- 1School of Life Sciences, Sun Yat-sen University, Guangzhou, China
- 2College of Life Sciences, South China Agricultural University, Guangzhou, China
- 3Research Institute of Sun Yat-sen University, Shenzhen, China
Amentotaxus argotaenia (Taxaceae) is a vulnerable coniferous species with preference for shade and moist environment. Accurate estimation of genetic variation is crucial for its conservation, especially in the context of global warming. In this study, we acquired a transcriptome from A. argotaenia leaves using Illumina sequencing and de novo assembled 62,896 unigenes, of which 5510 EST-SSRs were detected. Twenty-two polymorphic EST-SSRs were successfully developed and further used to investigate genetic variation, linkage disequilibrium, and bottleneck signatures of A. argotaenia. The results showed that A. argotaenia had moderate genetic variation and high genetic differentiation, which may provide raw material to protect against climatic changes and accelerate local adaptation, respectively. No bottlenecks were found to occur in A. argotaenia. Our study not only showed that these EST markers are very effective in population genetic analysis but also lay a solid foundation for further investigating adaptive evolution and conservation strategies of A. argotaenia.
Introduction
The genus Amentotaxus Pilger (Taxaceae) has six species, and five of them have been listed as endangered, vulnerable, or near threatened (Fu et al., 1999a; Farjon and Filer, 2013; Hilton-Taylor et al., 2013). Among all Amentotaxus species, Amentotaxus argotaenia (Hance) Pilger has the widest distribution but with small isolated populations occurring in southern and central China, northern Vietnam, and Laos (Farjon and Filer, 2013). Its preferred environments are limestone mountains, forests, ravines, and shady and damp stream banks, at altitudes of 300–1100 m (Fu et al., 1999a; Lin et al., 2007). The natural regeneration of the plant is infrequent due to slow growth rate and poorly dispersed seeds. Moreover, forest clearing and habitat loss have also been severely reducing its population size. A. argotaenia is listed as vulnerable in China and as near threatened in the International Union for Conservation of Nature Red List of Threatened Species (Hilton-Taylor et al., 2013). Since knowledge of population genetics is essential for the conservation and sustainable use of wild resources (Wayne and Morin, 2004; Hoban et al., 2013), we aim to examine the population genetic variation of A. argotaenia using novel molecular markers.
In comparison to genomic SSRs, expressed sequence tag (EST)-SSRs represent functional markers that may linked with functional genes inducing phenotypic effects (Ranade et al., 2014; Zhou et al., 2018a). They hence provide opportunities to examine functional diversity in relation to adaptive variation. Moreover, EST-SSRs are reported to be more reliable because they present lower frequencies of null alleles than do genomic SSRs (Yu and Li, 2008). With the advances in next-generation sequencing technology, EST-SSRs are becoming amenable to be identified by sequencing the transcriptomes (Zhou et al., 2018b). Next-generation sequencing is faster and more cost-effective than traditional approach, e.g., cDNA library construction method (Huang et al., 2015). However, currently, there is a shortage of EST-SSRs developed from A. argotaenia, although EST-SSRs have been identified for its congeneric species, Amentotaxus formosana (Li et al., 2016). Excavation and characterization of EST-SSRs for A. argotaenia may contribute to enhance our understanding of its population genetic diversity, structure, and the genetic basis of adaptive divergence. In addition, it will also provide resources to assess the association between transposable elements and SSR distribution as well as their roles in genome organization (La Rota et al., 2005; Wang et al., 2018a).
In this study, we constructed a leaf transcriptome of A. argotaenia using the Illumina sequencing platform. Based on the transcriptome sequencing data, we developed a set of EST-SSR markers and examined their polymorphisms. We then assessed the genetic variation of four populations of A. argotaenia using the novel EST-SSR markers. This work provides essential information for the conservation and management of A. argotaenia in the future.
Materials and Methods
Plant Materials and DNA Extraction
A total of 56 A. argotaenia individuals were sampled from four of its natural populations Jiuqushui (JQS; n = 15), Chuanping (CP; n = 13), Qiniangshan (QNS; n = 12), and Wugongshan (WGS; n = 16) located in China (Supplementary Table 1). Fresh leaves were collected and desiccated in sealed plastic bags with silica gel. Genomic DNA was isolated using the modified cetyltrimethylammonium bromide method (Su et al., 2005). DNA quality was evaluated using gel electrophoresis on 0.8% agarose gel.
RNA Extraction, cDNA Library Construction, and Transcriptome Sequencing
Fresh young leaves of one A. argotaenia individual from population CP, which is planted in a greenhouse at Sun Yat-sen University, were used to extract total RNAs using the method described by Fu et al. (2004). RNA integrity was evaluated on agarose gels followed by quantification on an Agilent 2100 Bioanalyzer (Agilent Technologies, Santa Clara, California, USA). The mRNAs were isolated from the total RNAs by using a Dynabeads mRNA DIRECT Kit (Invitrogen Life Technologies, Carlsbad, California, USA) and randomly fragmented. The fragmented mRNAs were converted into double-stranded cDNA by using random primers and reverse transcriptase. After end-repairing and tailing A, the cDNA fragments were ligated to Illumina paired-end adapters. The cDNA library was sequenced on an Illumina Hiseq2500 platform (Illumina, San Diego, California, USA) with insertion size of 400–500 bp.
Transcriptome Assembly, Functional Annotation, and Classification
We obtained a total of 25,257,542 paired-end reads from A. argotaenia. The reads were filtered by removing primer or adaptor sequences, and reads that contain unknown (“N”) or poor-quality bases (the mean quality per base < 15 with a 4-base wide sliding window) using the Trimmomatic software version 0.32 (Bolger et al., 2014). The resulting clean data were deposited in Sequence Read Archive of the National Center for Biotechnology Information (NCBI) (Bioproject no. PRJNA413732; Biosample no. SAMN07764634; https://www.ncbi.nlm.nih.gov/bioproject/PRJNA413732). The clean reads longer than 90 nt were de novo assembled into contigs and transcripts using the TRINITY software (https://github.com/trinityrnaseq/trinityrnaseq/releases) with default settings. The transcripts that cannot be prolonged at either end were defined as unigenes.
All unigenes were searched against Nt (NCBI nucleotide sequences), Nr (NCBI non-redundant database), and Swiss-Prot (a manually annotated and reviewed protein sequence database) through blast 2.2.30+ (ftp://ftp.ncbi.nlm.nih.gov/blast/executables/blast+/2.2.30/) with a cut-off E-value of 10−5. Protein domains of open reading frame within unigenes was identified by using HMMER hmmscan (hmmer-3.1b2-linux-intel-x86_64) and Pfam (the protein families database). Blast2GO version 3.0 was used to perform Gene Ontology (GO) annotations defined by molecular function, cellular component, and biological process ontologies (http://www.blast2go.com/b2ghome). We further used the Kyoto Encyclopedia of Genes and Genomes (KEGG) database to performed pathways annotation and euKaryotic Ortholog Groups (KOG) database to predict possible functions.
Development of EST-SSRs
EST-SSRs were searched in the assembled unigenes using MIcroSAtellite (http://pgrc.ipk-gatersleben.de/misa/misa.html). The SSRs were assumed to contain mono-, di-, tri-, tetra-, penta-, and hexa-nucleotides with minimum repeat numbers of 10, 6, 5, 5, 5, and 5, respectively. Primer premier 5.0 (Clarke and Gorley, 2001) was used to design primers. Only those SSRs containing two to six repeat motifs were considered. Major parameters for primer design included the following: (1) primer length ranging from 16 to 27 bp, (2) GC content of 30%–70%, (3) melting temperature between 50 and 63°C, and (4) PCR product size ranging from 100 to 400 bp. PCR reactions were performed in 20 μl mixture containing 2 μl 10 × PCR buffer (Mg2+), 0.4 μl 10 mM dNTPs, 0.5 μl 10 mM each of primers, 1.25 U Taq polymerase, and 20 ng DNA template. The PCR protocol was as follows: 94°C for 5 min, followed by 30 cycles of 40 s at 94°C, 40 s at optimal annealing temperature and 30 s at 72°C, and a final elongation at 72°C for 10 min. Amplified products were screened on a 6.0% denaturing polyacrylamide gel, and fragment size was determined with 50-bp marker.
Evaluation of Polymorphic EST-SSRs and Population Genetic Analysis
GenAlex software (Peakall and Smouse, 2006) was used to calculate the EST-SSR genetic parameters, including the number of observed alleles (Na), the effective number of alleles (Ne), observed heterozygosities (Ho), expected heterozygosities (He), and probability of the deviation from the Hardy–Weinberg equilibrium. The polymorphism information content (PIC) value and null alleles were evaluated using CERVUS 3.0 (Kalinowski et al., 2007) and Micro-Checker (Van Oosterhout et al., 2004), respectively. Linkage disequilibrium across loci was determined using TASSEL version 3.0 (Bradbury et al., 2007) with squared correlation coefficient (r2 > 0.3) and the threshold of p values (< 0.001) based on Fisher’s exact test.
Arlequin version 3.5 (Excoffier and Lischer, 2010) was used to perform a Mantel test with 10,000 permutations to examine the pattern of isolation by distance. Using the same software, analysis of molecular variance was conducted to determine the amount of genetic variation at different levels.
Using EST-SSR data, we applied a Bayesian model-based clustering algorithm implemented in STRUCTURE 2.2 to infer population structure. We used the admixture model, setting the parameters as follows: burn-in periods = 10,000, MCMC replicates = 10,000, K = 1 to 8, and iterations = 10. The optimum number of clusters (K) was determined by calculating ΔK (Evanno et al., 2005).
We conducted the Wilcoxon’s sign-rank test and the mode-shift test to detect signatures of genetic bottleneck by running BOTTLENECK version 1.2.02 (Piry et al., 1999). The two-phase mutation model was selected because it is more suitable for microsatellite data than the other two models (Piry et al., 1999; Zhang and Zhou, 2013). We performed 1000 simulations under the two-phase mutation model with 70% single-step mutations and 30% multi-step mutations.
Results
Transcriptome Sequencing and De Novo Assembly
Approximately 23.5 million clean reads were obtained from the transcriptome of A. argotaenia with the length of 90–125 bp and GC content of 47%. The percentages of Q20 (base sequencing error probability < 1%) and Q30 (base sequencing error probability < 0.1%) bases were 100% and 97%, respectively. These clean reads were assembled into 80674 transcripts by using Trinity with an average length of 756 bp and an N50 of 1018 bp. The total length of transcripts reached 60,999,479 bp. After further assembly, a total of 62,896 unigenes were identified with an average length of 721 bp, a minimal length of 301 bp, and an N50 value of 947 bp. The sum of the length of the unigenes was 45,357,136 bp (Table 1). The length of 37.473% (23569) of the unigenes ranged from 301 to 400 bp, 61.956% (38,968) varied from 401 to 3000 bp, while 0.571% (359) was longer than 3000 bp (Supplementary Figure 1).
Functional Annotation and Categorization
We conducted the annotation of 62,896 unigenes in the seven public databases (Nr, Nt, KOG, Swiss-Prot, Pfam, KEGG, and GO), of which 36,671 were successfully annotated (Table 1). Of them, 13,140 (20.89%) were annotated in Nt, 32,183 (51.17%) in Nr, 26,309 (41.83%) in Swiss-Prot, 21,595 (34.33%) in Pfam, 31,283 (49.74%) in GO, 32,953 (52.39%) in KOG, and 15,072 (23.96%) in KEGG. A total of 490 unigene were identified in all seven databases (Table 1).
First, 31,283 unigenes were classified into three main GO categories: biological process, cellular component, and molecular function, including 44 functional groups. In the biological process category, there were 7313 unigenes assigned to “cellular process,” 5973 to “single-organism process,” 5808 to “metabolic process,” and 1 to “biological regulation.” In the cellular component category, “cell part” and “organelle” component-related functions were predominant, with 4835 unigenes assigned to the former and 2060 to the latter. In the molecular function category, “binding” and “catalytic activity” were the most enriched, comprising 2520 and 2358 unigenes, respectively (Figure 1).
Second, 32,953 unigenes were assigned to 25 KOG classifications. Among them, the largest group was “general function prediction only” (4286), followed by “function unknown” (3218), “signal transduction mechanisms” (2521), and “posttranslational modification, protein turnover, chaperones” (2442) (Supplementary Figure 2).
Third, a total of 15,072 unigenes were mapped to 38 KEGG pathways corresponding to six categories (Supplementary Figure 3). Most of them involved in “translation” (3007 unigenes; 19.95%), “carbohydrate metabolism” (2815; 18.68%), and “folding” (2425; 16.09%) pathways. The 38 KEGG pathways were related to such categories as metabolism, cellular processes, genetic information processing, environmental information processing, and others.
Characterization of EST-SSRs
We identified 5510 EST-SSRs from 4830 SSR-containing unigene sequences, of which 362 were compound SSRs. With the exception of mononucleotide repeats (64.50%), trinucleotide repeats were the most common type with a frequency of 22.25%, followed by di- (9.18%), tetra- (1.82%), penta- (1.00%), and hexanucleotide (1.25%) repeats (Table 2). EST-SSRs with 10 tandem repeats (1719, 31.19%) were the most common, followed by >11 tandem repeats (1134; 20.58%) and 9 tandem repeats (42; 0.76%) (Table 2). Motif A/T (98.11%) was dominant in mononucleotide repeats, whereas AT/TA (50.79%) was the most abundant in dinucleotide repeats, followed by AG/CT (35.18%) and AC/GT (14.03%). Trinucleotide motifs AAG/CTT, AGG/CCT, AGC/CTG, AAT/ATT, and ACT/AGT had frequencies of 21.21%, 17.94%, 15.42%, 14.68%, and 0.33%, respectively.
We also examined the linkage of the 22 EST-SSR loci. Of 231 combinations, four pairs of loci (SHS-1306 vs SHS-26840, SHS-1306 vs SHS-38297, SHS-28717 vs SHS-32587, and SHS-34629 vs SHS-38297) were found to be linked with r2 > 0.3 and p < 0.001.
Polymorphic EST-SSRs Identification and Estimation of Genetic Diversity
We randomly selected 60 EST-SSR primers to evaluate their application and the polymorphism across 12 A. argotaenia individuals from four populations. Twenty-two of the microsatellite loci exhibited allelic polymorphism, whereas 16 were identified as monomorphic (Table 3). We then used the 22 polymorphic EST-SSR markers to perform population genetic analysis (Supplementary Figure 5).
The number of observed alleles and the effective number of alleles varied from 1 to 7 and from 1 to 4.694 per locus, respectively. The observed heterozygosity ranged from 0 to 1.000 (average = 0.250), while the expected heterozygosity ranged from 0.000 to 0.787 (average = 0.390). The mean value of PIC was 0.455, with the minimum of 0.084 and the maximum of 0.707. Fourteen loci were identified as null allele. Six, 9, 14, and 16 EST-SSRs showed significant deviations from the Hardy–Weinberg equilibrium in populations JQS, CP, QNS, and WGS, respectively (Table 4).
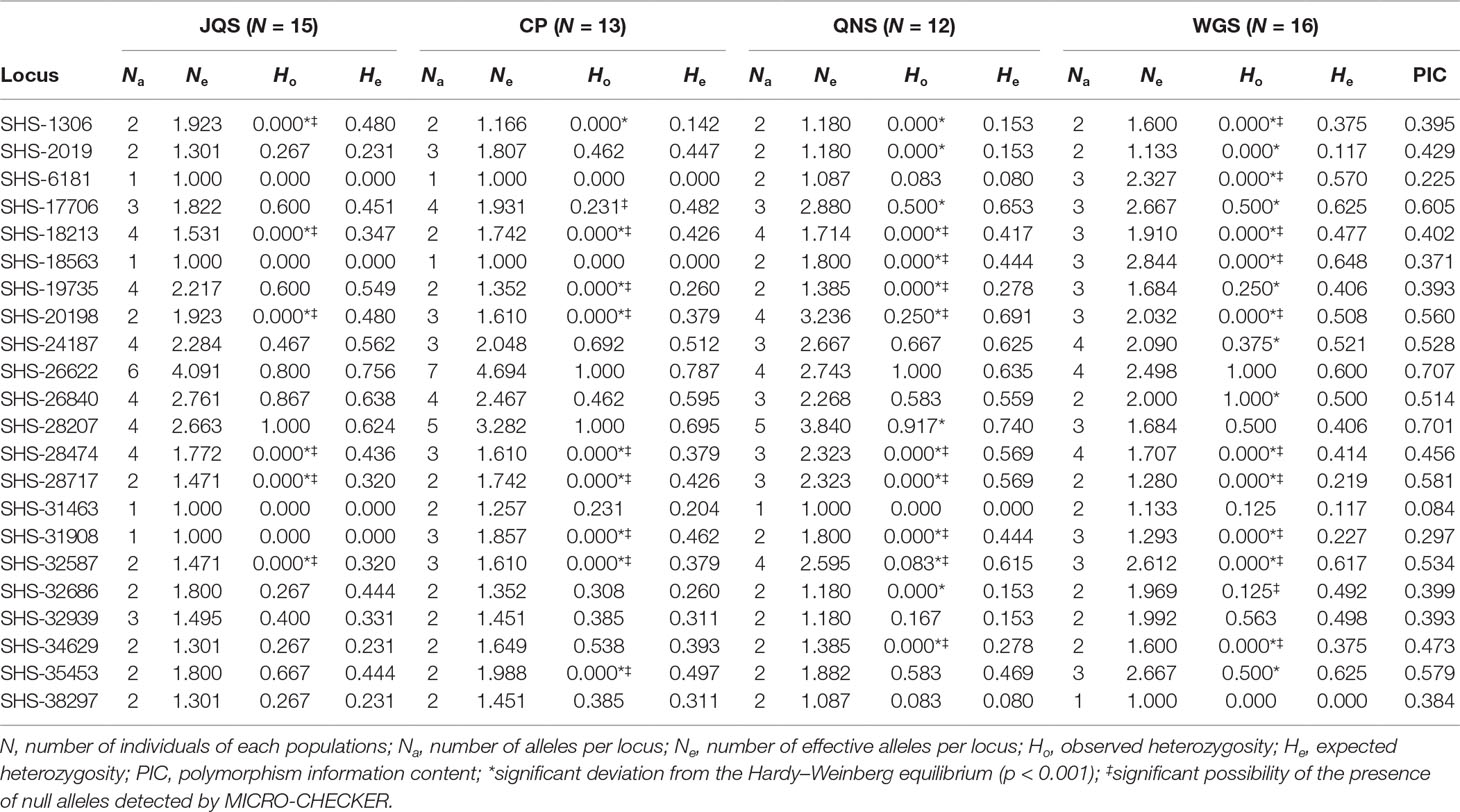
Table 4 Genetic diversity statistics for four A. argotaenia populations based on 22 polymorphic EST-SSR primers.
Population Genetic Structure and Differentiation
Genetic differentiation (Fst) based on EST-SSRs was 0.28198. Analysis of molecular variance revealed that 71.80% of the genetic variation occurred within populations, while 10.37% and 17.83% were attributed to among populations within groups and among groups, respectively (Supplementary Table 2). In addition, the result of Mantel test showed that there was no significant correlation between genetic and geographical distances (p = 0.3306).
ΔK demonstrated that the uppermost K equaled 3 (Supplementary Figure 4). Amentataxus argotaenia populations were assigned to three groups. Group I contained populations JQS and CP, group II contained population WGS, and group III contained population QNS (Figure 2).
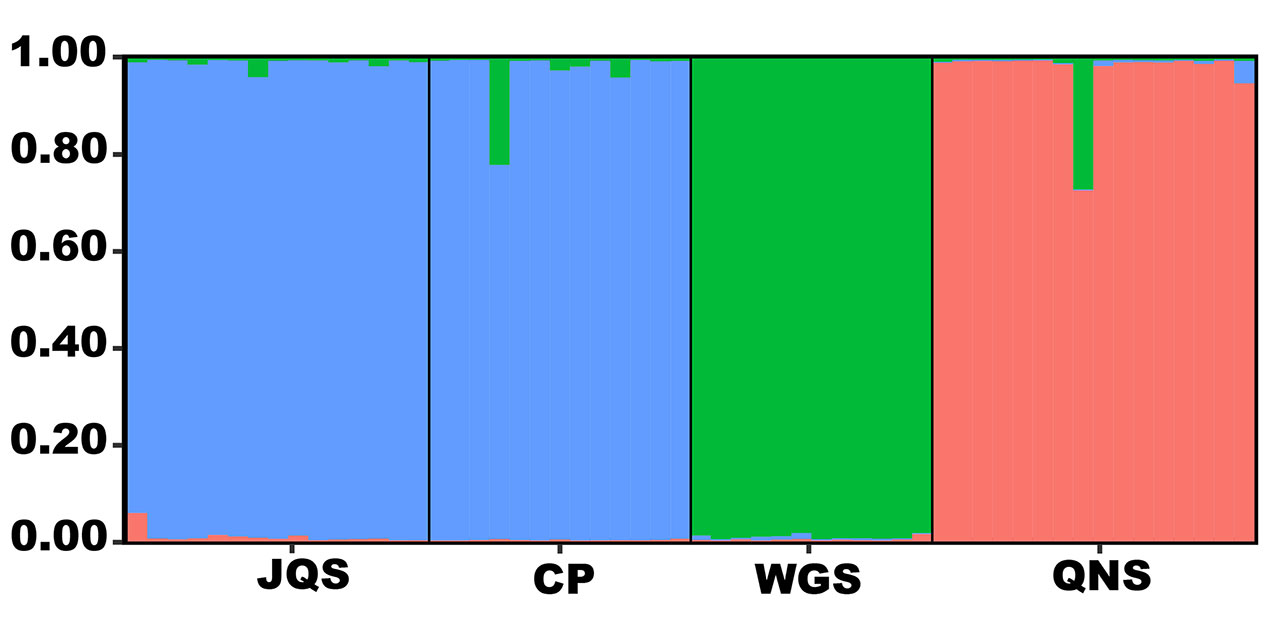
Figure 2 Population genetic structure analysis of A. argotaenia based on EST-SSRs. JQS, Population Jiuqushui; CP, Population Chuanping; WGS, Population Wugongshan; QNS, Population Qiniangshan.
Bottleneck Signature
At species level, both Wilcoxon’s sign-rank test and mode-shift analysis indicated that A. argotaenia had not experienced a recent bottleneck (Supplementary Table 3).
Discussion
Characterization of Transcriptome
In this study, we sequenced, assembled, and annotated the transcriptome of A. argotaenia using the next-generation sequencing approach. A total of 62,896 unigenes were de novo assembled with the unigene mean and N50 length of 721 and 947 bp, respectively (Table 1). More than half of the unigenes can be successfully annotated through seven databases (Nr, Nt, KOG, Swiss-Prot, Pfam, KO, and GO), of which 490 were simultaneously identified (Table 1). Most of the annotated unigenes were unique in A. argotaenia compared to its closely related conifer Torreya grandis (Zeng et al., 2018). Moreover, the annotated results of NR database indicated that A. argotaenia exhibited only 27.5% unigene identity to another conifer Picea sitchensis. These unique unigenes may represent the species-specific genetic signature of A. argotaenia potentially underlying its speciation process or evolution (Frech and Chen, 2011). Similar results have been found in the case of Picea abies (Nystedt et al., 2013). In addition, 31,283, 32,953, and 15,072 unigenes of A. argotaenia were assigned into 44 functional groups in GO, 25 classifications in KOG, and 38 pathways in KEGG, respectively. These results indicate that the identified A. argotaenia unigenes have wide-ranging functions and will be valuable for analyzing the functional diversity of A. argotaenia.
Frequency and Distribution of EST-SSRs
The mean length of unigenes (721 bp) of A. argotaenia was considerably longer than that of other conifers, including Pinus pinaster (495 bp) (Canales et al., 2014), Platycladus orientalis (686 bp) (Hu et al., 2016), and P. abies (472 bp) (Chen et al., 2012). Zalapa et al. (2012) pointed out that longer sequences will increase the probability to properly design EST-SSR primers. In accordance with this, we developed 5510 EST-SSRs from the transcriptome of A. argotaenia, which was significantly greater than those of A. formosana (4955) (Li et al., 2016) and Pinus densiflora (1953) (Liu et al., 2015). The most common motif for dinucleotides in A. argotaenia was found to be AT/TA. The same result was obtained in Picea spp. (Rungis et al., 2004), Pinus dabeshanensis (Xiang et al., 2015), and Pseudolarix amabilis (Geng et al., 2015). Ranade et al. (2014) emphasized that AT/TA was often ranked as the most abundant dimer motif in gymnosperms (especially in the 3′ untranslated regions). A factor related to this phenomenon may be increased A + T contents. In addition, the most common trinucleotide motif in A. argotaenia was AAG/CTT, which is similar to that in Cryptomeria japonica (Ueno et al., 2012), Pinus taeda (Wegrzyn et al., 2014), and Pinus halepensis (Pinosio et al., 2014), but unlike in P. dabeshanensis (AGC) (Xiang et al., 2015). It has been noted that AAG/CTT is the target for methylation in plants (Law and Jacobsen, 2010).
Validation and Polymorphism of EST-SSRs and Population Genetic Variation
Thirty-eight of the 60 EST-SSR primers designed for A. argotaenia enabled to amplify expected products with a success rate of 63.33%, which is relatively high in comparison to previous studies (Dantas et al., 2015; Ueno et al., 2015). Then 22 polymorphic EST-SSRs were used to investigate standard genetic diversity and population genetic structure of four A. argotaenia populations. Only four pairs of them were tightly linked among each other. Moderate genetic variation was observed based on the EST-SSRs (PIC = 0.455) according to the evaluation criteria of polymorphism (moderate: 0.25 < PIC < 0.5) (Botstein et al., 1980). This judgment was also lent support by such genetic parameters as the average of observed alleles, observed heterozygosity, expected heterozygosity, and the percentage of polymorphic band. In contrast, previous researches detected low levels of genetic diversity in A. argotaenia by using ISSRs and genomic SSRs (Ge et al., 2005; Ge et al., 2015). These inconsistencies highlighted the importance to investigate genetic variation of A. argotaenia using multiple markers.
Accurate estimate of genetic diversity is very useful for conservation and management of genetic resources (Cardoso et al., 1998; Wang et al., 2018b). Compared to other conifers, we observed a moderate EST-SSR variation in A. argotaenia (Supplementary Table 4). Similar levels of the EST-SSR variation were found in its closely related species A. formosana as well (Li et al., 2016). The moderate level of functional diversity, together with the finding that A. argotaenia did not experience a recent bottleneck, implies that the species still has essential evolutionary potential to adapt to the changing environment (Frankham, 2010).
We detected a marked genetic differentiation among A. argotaenia populations in comparison to other conifers (Supplementary Table 4). Similar findings were obtained by using chloroplast intergenic spacer, mitochondrial intron, and genomic microsatellite data (Ge et al., 2015). The dispersal distance of pollen and seed in conifers is generally less than 2 and 20 km, respectively (Fu et al., 1999b; Cain et al., 2000; Sima, 2004; Zhang et al., 2005; Lu, 2006). As for A. argotaenia, its pollen and seed exchanges may be further hindered because of preferring to grow under forest canopies (Ge et al., 2015). It is thus reasonable to speculate that the genetic differentiation pattern of A. argotaenia is highly linked to restricted between-population gene flow (genetic exchange via pollen and seed). Moreover, the establishment of climate- or habitat-linked genotypes should also be considered, since we used functional markers to perform studies (Jump and Peñuelas, 2005; Ortego et al., 2012).
Conclusion
We generated the leaf transcriptome of A. argotaenia by using Illumina sequencing technology. A total of 62,896 unigenes were assembled, annotated, and classified. Based on the transcriptome data, 5510 EST-SSRs were identified from 4830 SSR-containing unigene sequences. Among them, 60 were randomly selected for the development of potential functional markers. Consequently, 22 polymorphic EST-SSR markers were developed and used to reveal a moderate level of functional diversity, along with marked genetic structure and the lack of genetic bottleneck, in A. argotaenia. This study has provided effective EST-SSR markers for measuring the evolutionary potential of A. argotaenia in response to environmental changes.
Data Availability Statement
All Illumina clean data generated for this study was deposited at the Sequence Read Archive (SRA) of the National Center for Biotechnology Information (https://www.ncbi.nlm.nih.gov/sra/SRX3296043[accn]). The Bioproject number and Biosample number for clean data are PRJNA413732 and SAMN07764634, respectively. Thirty-eight EST-SSR sequences generated for this study were deposited at GenBank with accession numbers MG209531-MG209568.
Author Contributions
The author XR conducted the experiments and ZW completed the data analysis. The two authors contributed equally to this work. YS designed the experiments and wrote the manuscript, and TW corrected the manuscript.
Funding
This work was supported by the National Natural Science Foundation of China (31370364, 31570652, 31670200, 31770587, and 31872670); the Natural Science Foundation of Guangdong Province, China (2016A030313320 and 2017A030313122); Science and Technology Planning Project of Guangdong Province, China (2017A030303007); Project of Department of Science and Technology of Shenzhen City, Guangdong, China (JCYJ20160425165447211, JCYJ20170413155402977, and JCYJ20170818155249053); and Science and Technology Planning Project of Guangzhou City, China (201804010389).
Conflict of Interest
The authors declare that the research was conducted in the absence of any commercial or financial relationships that could be construed as a potential conflict of interest.
Acknowledgments
The authors thank Dr. Q. Fan of the School of Life Sciences, Sun Yat-sen University, for assistance with the collection of plant materials.
Supplementary Material
The Supplementary Material for this article can be found online at: https://www.frontiersin.org/articles/10.3389/fgene.2019.01014/full#supplementary-material
Supplementary Figure 1 | Length distribution of assembled unigenes generated from A. argotaenia transcriptome.
Supplementary Figure 2 | Functional classification of A. argotaenia unigenes based on KOG annotation.
Supplementary Figure 3 | Functional classification of A. argotaenia unigenes based on KEGG annotation.
Supplementary Figure 4 | The optimum number of clusters (K) estimated with STRUCTURE analysis based on LnP(D) (A) and hoc statistic ΔK (B).
Supplementary Figure 5 | Amplification products generated using EST-SSR primer pair, SHS-26622, were separated by electrophoresis in 6% denaturing polyacrylamide gel. The expected allele size was 228 bp and the annealing temperature was 57°C. Lanes 1-15, 16-28, 29-40, and 41-56 were products of A. argotaenia individuals from populations JQS (Jiuqushui), CP (Chuanping), QNS (Qiniangshan), and WGS (Wugongshan), respectively; Lane M: 50 bp ladder.
References
Bolger, A. M., Lohse, M., Usadel, B. (2014). Trimmomatic: a flexible trimmer for Illumina sequence data. Bioinformatics 30, 2114–2120. doi: 10.1093/bioinformatics/btu170
Botstein, D., White, R. L., Skolnick, M., Davis, R. W. (1980). Construction of a genetic linkage map in man using restriction fragment length polymorphisms. Am. J. Hum. Genet. 32, 314–331. doi: 10.1016/0165-1161(81)90274-0
Bradbury, P. J., Zhang, Z. W., Kroon, D. E., Casstevens, T. M., Ramdoss, Y., Buckler, E. S. (2007). TASSEL: software for association mapping of complex traits in diverse samples. Bioinformatics 23, 2633–2635. doi: 10.1093/bioinformatics/btm308
Cain, M. L., Milligan, B. G., Strand, A. E. (2000). Long-distance seed dispersal in plant populations. Am. J. Bot. 87, 1217–1227. doi: 10.2307/2656714
Canales, J., Bautista, R., Label, P., Gomez-Maldonado, J., Lesur, I., Fernandez-Pozo, N., et al. (2014). De novo assembly of maritime pine transcriptome: implications for forest breeding and biotechnology. Plant Biotechnol. J. 12, 286–299. doi: 10.1111/pbi.12136
Cardoso, M. A., Provan, J., Powell, W., Ferreira, P. C. G., De Oliveira, D. E. (1998). High genetic differentiation among remnant populations of the endangered Caesalpinia echinata Lam. (Leguminosae–Caesalpinioideae). Mol. Ecol. 7, 601–608. doi: 10.1046/j.1365-294x.1998.00363.x
Chen, J., Uebbing, S., Gyllenstrand, N., Lagercrantz, U., Lascoux, M., Källman, T. (2012). Sequencing of the needle transcriptome from Norway spruce (Picea abies Karst L.) reveals lower substitution rates, but similar selective constraints in gymnosperms and angiosperms. BMC Genomics 13, 589. doi: 10.1186/1471-2164-13-589
Dantas, L. G., Esposito, T., Sousa, A. C. B. D., Félix, L., Amorim, L. L. B., Benko-Iseppon, A. M., et al. (2015). Low genetic diversity and high differentiation among relict populations of the neotropical gymnosperm Podocarpus sellowii (Klotz.) in the Atlantic Forest. Genetica 143, 21–30. doi: 10.1007/s10709-014-9809-y
Evanno, G., Regnaut, S., Goudet, J. (2005). Detecting the number of clusters of individuals using the software structure: a simulation study. Mol. Ecol. 14, 2611–2620. doi: 10.1111/j.1365-294X.2005.02553.x
Excoffier, L., Lischer, H. E. L. (2010). Arlequin suite ver 3.5: a new series of programs to perform population genetics analyses under Linux and Windows. Mol. Ecol. Resour. 10, 564–567. doi: 10.1111/j.1755-0998.2010.02847.x
Farjon, A., Filer, D., (2013). An atlas of the world’s conifers: an analysis of their distribution, biogeography, diversity and conservation status. Leiden: Brill. doi: 10.1163/9789004211810
Frech, C., Chen, N. (2011). Genome comparison of human and non-human malaria parasites reveals species subset-specific genes potentially linked to human disease. PLoS Comput. Biol. 7, e1002320. doi: 10.1371/journal.pcbi.1002320
Frankham, R. (2010). Challenges and opportunities of genetic approaches to biological conservation. Biol. Conserv. 143, 1919–1927. doi: 10.1016/j.biocon.2010.05.011
Fu, L. G., Li, N., Mill, R. R., (1999a). “Taxaceae,” in Flora of China. Eds. Wu, Z. Y., Raven, P. H. (Beijing: Science Press and St. Louis, Missouri: Missouri Botanical Garden Press), 89–98.
Fu, L. G., Li, N., Mill, R. R., (1999b). “Cephalotaxaceae,” in Flora of China. Eds. Wu, Z. Y., Raven, P. H. (Beijing: Science Press and St. Louis, Missouri: Missouri Botanical Garden Press), 85–88.
Fu, X. H., Deng, S. L., Su, G. H., Zeng, Q. L., Shi, S. H. (2004). Isolating high-quality RNA from mangroves without liquid nitrogen. Plant Mol. Biol. Rep. 22, 197. doi: 10.1007/BF02772728
Ge, X. J., Hung, K. H., Ko, Y. Z., Hsu, T. W., Gong, X., Chiang, T. Y., et al. (2015). Genetic divergence and biogeographical patterns in Amentotaxus argotaenia species complex. Plant Mol. Biol. Rep. 33, 264–280. doi: 10.1007/s11105-014-0742-0
Ge, X. J., Zhou, X. L., Li, Z. C., Hsu, T. W., Schaal, B. A., Chiang, T. Y. (2005). Low genetic diversity and significant population structuring in the relict Amentotaxus argotaenia complex (Taxaceae) based on ISSR fingerprinting. J. Plant Res. 118, 415–422. doi: 10.1007/s10265-005-0235-1
Geng, Q. F., Liu, J., Sun, L., Liu, H., Ou-Yang, Y., Cai, Y., et al. (2015). Development and characterization of polymorphic microsatellite markers (SSRs) for an endemic plant, Pseudolarix amabilis (Nelson) Rehd. (Pinaceae). Molecules 20, 2685–2692. doi: 10.3390/molecules20022685
Hilton-Taylor, C., Yang, Y., Rushforth, K., Liao, W., (2013). Amentotaxus argotaenia. The IUCN Red List of Threatened Species. Available at: http://www.iucnredlist.org (Accessed July 3, 2017).
Hoban, S. M., Hauffe, H. C., Pérez-Espona, S., Arntzen, J. W., Bertorelle, G., Bryja, J., et al. (2013). Bringing genetic diversity to the forefront of conservation policy and management. Conserv. Genet. Resour. 5, 593–598. doi: 10.1007/s12686-013-9859-y
Hu, X. G., Liu, H., Jin, Y., Sun, Y. Q., Li, Y., Zhao, W., et al. (2016). De novo transcriptome assembly and characterization for the widespread and stress-tolerant conifer Platycladus orientalis. PLoS ONE 11, e0148985. doi: 10.1371/journal.pone.0148985
Huang, L. K., Yan, H. D., Zhang, X. X., Zhang, X. Q., Wang, J., Frazier, T., et al. (2015). Identifying differentially expressed genes under heat stress and developing molecular markers in orchardgrass (Dactylis glomerata L.) through transcriptome analysis. Mol. Ecol. Resour. 15, 1497–1509. doi: 10.1111/1755-0998.12418
Jump, A. S., Peñuelas, J. (2005). Running to stand still: adaptation and the response of plants to rapid climate change. Ecol. Lett. 8, 1010–1020. doi: 10.1111/j.1461-0248.2005.00796.x
Kalinowski, S. T., Taper, M. L., Marshall, T. C. (2007). Revising how the computer program CERVUS accommodates genotyping error increases success in paternity assignment. Mol. Ecol. 16, 1099–1106. doi: 10.1111/j.1365-294X.2007.03089.x
La Rota, M., Kantety, R. V., Yu, J.-K., Sorrells, M. E. (2005). Nonrandom distribution and frequencies of genomic and EST-derived microsatellite markers in rice, wheat, and barley. BMC Genomics 6, 23. doi: 10.1186/1471-2164-6-23
Law, J. A., Jacobsen, S. E. (2010). Establishing, maintaining and modifying DNA methylation patterns in plants and animals. Nat. Rev. Genet. 11, 204–220. doi: 10.1038/nrg2719
Li, C. Y., Chiang, T. Y., Chiang, Y. C., Hsu, H. M., Ge, X. J., Huang, C. C., et al. (2016). Cross-species, amplifiable EST-SSR markers for Amentotaxus species obtained by next-generation sequencing. Molecules 21, 67. doi: 10.3390/molecules21010067
Lin, C., Chan, M. H., Chen, F. S., Wang, Y. N. (2007). Age structure and growth pattern of an endangered species, Amentotaxus formosana Li. J. Integr. Plant Biol. 49, 157–167. doi: 10.1111/j.1744-7909.2007.00429.x
Liu, L., Zhang, S., Lian, C. (2015). De novo transcriptome sequencing analysis of cDNA library and large-scale unigene assembly in Japanese Red Pine (Pinus densiflora). Int. J. Mol. Sci. 16, 29047–29059. doi: 10.3390/ijms161226139
Lu, C. H. (2006). Roles of animals in seed dispersal of Pinus: a review. Chin. J. Ecol. 25, 557–562. doi: 10.1093/rheumatology/kex446
Nystedt, B., Street, N. R., Wetterbom, A., Zuccolo, A., Lin, Y. C., Scofield, D. G., et al. (2013). The Norway spruce genome sequence and conifer genome evolution. Nature 497, 579–584. doi: 10.1038/nature12211
Ortego, J., Riordan, E. C., Gugger, P. F., Sork, V. L. (2012). Influence of environmental heterogeneity on genetic diversity and structure in an endemic southern Californian oak. Mol. Ecol. 21, 3210–3223. doi: 10.1111/j.1365-294X.2012.05591.x
Peakall, R., Smouse, P. E. (2006). GenALEx 6: genetic analysis in Excel. Population genetic software for teaching and research. Mol. Ecol. Notes 6, 288–295. doi: 10.1111/j.1471-8286.2005.01155.x
Pinosio, S., González-Martínez, S. C., Bagnoli, F., Cattonaro, F., Grivet, D., Marroni, F., et al. (2014). First insights into the transcriptome and development of new genomic tools of a widespread circum-Mediterranean tree species, Pinus halepensis Mill. Mol. Ecol. Resour. 14, 846–856. doi: 10.1111/1755-0998.12232
Piry, S., Luikart, G., Cornuet, J. M. (1999). Bottleneck: a computer program for detecting recent reductions in the effective size using allele frequency data. J. Hered. 90, 502–503. doi: 10.1093/jhered/90.4.502
Ranade, S. S., Lin, Y. C., Zuccolo, A., Peer, Y. V. D., García-Gil, M. D. R. (2014). Comparative in silico analysis of EST-SSRs in angiosperm and gymnosperm tree genera. BMC Plant Biol. 14, 220. doi: 10.1186/s12870-014-0220-8
Rungis, D., Bérubé, Y., Zhang, J., Ralph, S., Ritland, C. E., Ellis, B. E., et al. (2004). Robust simple sequence repeat markers for spruce (Picea spp.) from expressed sequence tags. Theor. Appl. Genet. 109, 1283–1294. doi: 10.1007/s00122-004-1742-5
Sima, Y. K. (2004). Studies of conservation biology on Cephalotaxus oliveri, a rare medicinal plant. Kunming, China: Yunnan University Press.
Su, Y. J., Wang, T., Zheng, B., Jiang, Y., Chen, G. P., Ouyang, P. Y., et al. (2005). Genetic differentiation of relictual populations of Alsophila spinulosa in southern China inferred from cpDNA trnL–F noncoding sequences. Mol. Phylogenet. Evol. 34, 323–333. doi: 10.1016/j.ympev.2004.10.016
Ueno, S., Moriguchi, Y., Uchiyama, K., Ujino-Ihara, T., Futamura, N., Sakurai, T., et al. (2012). A second generation framework for the analysis of microsatellites in expressed sequence tags and the development of EST-SSR markers for a conifer, Cryptomeria japonica. BMC Genomics 13, 136. doi: 10.1186/1471-2164-13-136
Ueno, S., Wen, Y., Tsumura, Y. (2015). Development of EST-SSR markers for Taxus cuspidata from publicly available transcriptome sequences. Biochem. Syst. Ecol. 63, 20–26. doi: 10.1016/j.bse.2015.09.016
Van Oosterhout, C., Hutchinson, W. F., Wills, D. P. M., Shipley, P. (2004). MICRO-CHECKER: software for identifying and correcting genotyping errors in microsatellite data. Mol. Ecol. Notes 4, 535–538. doi: 10.1111/j.1471-8286.2004.00684.x
Wang, C. R., Yan, H. D., Li, J., Zhou, S. F., Liu, T., Zhang, X. Q., et al. (2018a). Genome survey sequencing of purple elephant grass (Pennisetum purpureum Schum ‘Zise’) and identification of its SSR markers. Mol. Breed. 38, 94. doi: 10.1007/s11032-018-0849-3
Wang, Y. L., Liang, Q. L., Hao, G. Q., Chen, C. L., Liu, J. Q. (2018b). Population genetic analyses of the endangered alpine Sinadoxa corydalifolia (Adoxaceae) provide insights into future conservation. Biodivers. Conserv. 27, 2275–2291. doi: 10.1007/s10531-018-1537-7
Wayne, R. K., Morin, P. A. (2004). Conservation genetics in the new molecular age. Front. Ecol. Envion. 2, 89–97. doi: 10.1890/1540-9295(2004)002[0089:CGITNM]2.0.CO;2
Wegrzyn, J. L., Liechty, J. D., Stevens, K. A., Wu, L. S., Loopstra, C. A., Vasque-Gross, H. A., et al. (2014). Unique features of the Loblolly Pine (Pinus taeda L.) megagenome revealed through sequence annotation. Genetics 196, 891–909. doi: 10.1534/genetics.113.159996
Xiang, X. Y., Zhang, Z. X., Wang, Z. G., Zhang, X. P., Wu, G. L. (2015). Transcriptome sequencing and development of EST-SSR markers in Pinus dabeshanensis, an endangered conifer endemic to China. Mol. Breed. 35, 158. doi: 10.1007/s11032-015-0351-0
Yu, H., Li, Q. (2008). Exploiting EST databases for the development and characterization of EST-SSRs in the Pacific oyster (Crassostrea gigas). J. Hered. 99, 208–214. doi: 10.1093/jhered/esm124
Zalapa, J. E., Cuevas, H., Zhu, H., Steffan, S., Senalik, D., Zeldin, E., et al. (2012). Using next-generation sequencing approaches to isolate simple sequence repeat (SSR) loci in the plant science. Am. J. Bot. 99, 193–208. doi: 10.3732/ajb.1100394
Zeng, J., Chen, J., Kou, Y. X., Wang, Y. J. (2018). Application of EST-SSR markers developed from the transcriptome of Torreya grandis (Taxaceae), a threatened nut-yielding conifer tree. PeerJ 6, e5606. doi: 10.7717/peerj.5606
Zhang, D. Q., Zhou, N. (2013). Genetic diversity and population structure of the endangered conifer Taxus wallichiana var. mairei (Taxaceae) revealed by Simple Sequence Repeat (SSR) markers. Biochem. Syst. Ecol. 49, 107–114. doi: 10.1016/j.bse.2013.03.030
Zhang, Q., Chiang, T. Y., George, M., Liu, J. Q., Abbott, R. J. (2005). Phylogeography of the Qinghai–Tibetan Plateau endemic Juniperus przewalskii (Cupressaceae) inferred from chloroplast DNA sequence variation. Mol. Ecol. 14, 3513–3524. doi: 10.1111/j.1365-294X.2005.02677.x
Zhou, S. F., Wang, C. R., Yin, G. H., Zhang, Y., Shen, X. Y., Pennerman, K. K., et al. (2018a). Phylogenetics and diversity analysis of Pennisetum species using Hemarthria EST-SSR markers. Grassland Sci. 65, 13–22. doi: 10.1111/grs.12208
Keywords: Amentotaxus argotaenia, transcriptome, EST-SSR, genetic variation, population structure
Citation: Ruan X, Wang Z, Wang T and Su Y (2019) Characterization and Application of EST-SSR Markers Developed From the Transcriptome of Amentotaxus argotaenia (Taxaceae), a Relict Vulnerable Conifer. Front. Genet. 10:1014. doi: 10.3389/fgene.2019.01014
Received: 31 March 2019; Accepted: 24 September 2019;
Published: 18 October 2019.
Edited by:
Jacob A. Tennessen, Harvard University, United StatesReviewed by:
Jian-Feng Mao, Beijing Forestry University, ChinaLinkai Huang, Sichuan Agricultural University, China
Copyright © 2019 Ruan, Wang, Wang and Su. This is an open-access article distributed under the terms of the Creative Commons Attribution License (CC BY). The use, distribution or reproduction in other forums is permitted, provided the original author(s) and the copyright owner(s) are credited and that the original publication in this journal is cited, in accordance with accepted academic practice. No use, distribution or reproduction is permitted which does not comply with these terms.
*Correspondence: Ting Wang, dGluZ3dhbmdAc2NhdS5lZHUuY24=; Yingjuan Su, c3V5akBtYWlsLnN5c3UuZWR1LmNu
†These authors have contributed equally to this work