- 1Laboratório de Helmintologia e Malacologia Médica, Instituto René Rachou, Fundação Oswaldo Cruz, Belo Horizonte, Brazil
- 2Allergy and Infectious Diseases Laboratory, Division of Molecular Therapeutics and Formulation, School of Pharmacy, University of Nottingham, Nottingham, United Kingdom
- 3Institute of Parasitology, BFS, Justus Liebig University, Giessen, Germany
- 4Environmental Genomics Group, Instituto Tecnológico Vale, Belém, Brazil
Mitogen-activated protein kinases (MAPKs) play a regulatory role and influence various biological activities, such as cell proliferation, differentiation, and survival. Our group has demonstrated through functional studies that Schistosoma mansoni c-Jun N-terminal kinase (SmJNK) MAPK is involved in the parasite’s development, reproduction, and survival. SmJNK can, therefore, be considered a potential target for the development of new drugs. Considering the importance of SmJNK in S. mansoni maturation, we aimed at understanding of SmJNK regulated signaling pathways in the parasite, correlating expression data with S. mansoni development. To better understand the role of SmJNK in S. mansoni intravertebrate host life stages, RNA interference knockdown was performed in adult worms and in schistosomula larval stage. SmJNK knocked-down in adult worms showed a decrease in oviposition and no significant alteration in their movement. RNASeq libraries of SmJNK knockdown schistosomula were sequenced. A total of 495 differentially expressed genes were observed in the SmJNK knockdown parasites, of which 373 were down-regulated and 122 up-regulated. Among the down-regulated genes, we found transcripts related to protein folding, purine nucleotide metabolism, the structural composition of ribosomes and cytoskeleton. Genes coding for proteins that bind to nucleic acids and proteins involved in the phagosome and spliceosome pathways were enriched. Additionally, we found that SmJNK and Smp38 MAPK signaling pathways converge regulating the expression of a large set of genes. C. elegans orthologous genes were enriched for genes related to sterility and oocyte maturation, corroborating the observed phenotype alteration. This work allowed an in-depth analysis of the SmJNK signaling pathway, elucidating gene targets of regulation and functional roles of this critical kinase for parasite maturation.
Introduction
Schistosomiasis is one of the most common human parasitic diseases whose socioeconomic impact affects people in developing nations in the tropics and sub-tropics. Preventive chemotherapy (PC) with Praziquantel is required in 52 countries, where only in 2017, 220 million people needed PC for schistosomiasis; however, only 44.9% of this demand was covered (WHO, 2018). Despite the control efforts, schistosomiasis continues to be a major public health problem, principally due to schistosomiasis-related morbidity (French et al., 2018; Nascimento et al., 2019).
Schistosoma mansoni kinome containing 252 eukaryotic kinase proteins (ePKs) was first described by Andrade and co-authors (Andrade et al., 2011) based on an earlier version of S. mansoni genome (Berriman et al., 2009). Recently, 351 kinase genes with evidence of being transcribed in nearly all adult stages were described, of which 268 were PKs and an additional 83 were non-PKs (Grevelding et al., 2017). Although protein kinases have been recognized for years as suitable targets for drug development (Cohen, 2002; Cai et al., 2017), experimental functional evidence exists for only 40 S. mansoni proteins, showing that there is still need for further research.
The c-Jun N-terminal kinase signaling pathway is involved in the developmental regulation of various organisms. Its role has already been demonstrated in oocyte maturation and embryogenesis of Xenopus laevis (Bagowski et al., 2001) and in the spindle assembly during the mouse oocyte meiotic maturation (Huang et al., 2011). In S. mansoni, SmJNK (Smp_172240) was previously characterized, demonstrating its importance for the establishment of infection in the mammalian host (Andrade et al., 2014). SmJNK knockdown caused damage to the adult worms’ tegument and impaired the maturation of vitelline organs. This resulted in lower oviposition and greater susceptibility to the host immune system. Furthermore, the SmJNK ortholog in Schistosoma haematobium was pointed as one of the prioritized druggable kinase targets due to its essentiality based on lethal gene knock-down or knock-out phenotypes in other organisms (Stroehlein et al., 2015).
Here, we sought to assess the role of SmJNK in adult worms in vitro, further advancing in unraveling the functions of this critical protein and putative druggable target. Also, we elucidated genes that are target of regulation by SmJNK knockdown, aiming to identify novel parasite-specific targets in addition to contributing to a better understanding of this signaling pathway. Additionally, we checked for a crosstalk between SmJNK pathway and the previously reported data from Smp38 (Avelar et al., 2019). Finally, we identified specific targets that correlate with previously observed phenotypes of developmental impairment (Andrade et al., 2014; Avelar et al., 2019) which is highly desirable for the design of new anti-schistosome drugs.
Methods
Parasites
S. mansoni adult worms of LE strain were recovered from hamster periportal perfusion 40 days after cercariae percutaneous infection (Pellegrino and Siqueira, 1956). Schistosomula were obtained by mechanical transformation of cercariae as previously described (Milligan and Jolly, 2011). Cercariae were supplied by the Mollusk Room ‘Lobato Paraense’ of the René Rachou Institute–FIOCRUZ, where the parasite cycle is routinely maintained. The sporocysts were prepared following the protocol previously described (Mourão et al., 2009). This work was approved by the Oswaldo Cruz Foundation’s Ethics Committee for Animal Use (CEUA) under number LW12/16, according to the Brazilian national guidelines set out in Law 11794/08.
DsRNA Synthesis
For the dsRNA synthesis, an SmJNK mRNA fragment corresponding to a region of approximately 570 bp, previously cloned into pGEM-T Easy vector, was amplified by PCR. Primers and cycling conditions used in this study were previously designed and established by Andrade and collaborators (Andrade et al., 2014). After amplification, PCR products were separated on 1% agarose gels, purified using QIAquick Gel Extraction Kit (Qiagen) and used as the template for dsRNA synthesis. DsRNA synthesis was performed using the T7 RiboMAX Express RNAi Systems kit (Promega) according to the supplier’s protocol. DsRNA integrity and annealing were verified on 1% agarose electrophoresis.
SmJNK Knockdown in Adult Worms by RNA Interference
After perfusion, males and females adult worms were washed and separated manually. Then, eight males and eight females were placed separately in each well containing 100 μL of RPMI 1640 medium with 25 μg of dsRNA, in two technical replicates. The worms were electroporated with specific SmJNK dsRNA or unspecific GFP dsRNA into 4 mm cuvettes at 125 V for 20 ms and cultivated in 24-well plates with 1 mL RPMI 1640 medium supplemented with 10% heat-inactivated Fetal Bovine Serum and 2% Penicillin/Streptomycin. Unless stated otherwise, all culture reagents were from Gibco, Thermo Fisher Scientific. Worm motility was assessed using the WormAssay software (Marcellino et al., 2012) for 10 days, in which eight worms were cultured in 24-well tissue culture plates containing 1 mL of medium. Similarly, to count the number of eggs laid, eight worm pairs were electroporated and cultured in 6-well plates, the medium was changed daily, and the eggs counted. Also, on days 3, 5, 7, and 10 after electroporation, two worm pairs per day were removed and macerated with TRIzol Reagent (Thermo Fisher Scientific) for RNA extraction as described below. SmJNK expression in adult worms was assessed by quantitative real-time PCR (RT-qPCR). Experiments were performed in three biological replicates. Data were analyzed using unpaired t-test with Welch’s correction.
SmJNK Knockdown in Schistosomula by RNA Interference
Schistosomula cultures (∼500,000 worms/condition) were maintained in bottles with 10 mL of Glasgow Minimum Essential Medium (GMEM) (Sigma-Aldrich) supplemented with 0.2 µM triiodothyronine (Sigma-Aldrich); 0.1% glucose; 0.1% lactalbumin (Sigma-Aldrich); 20 mM HEPES; 0.5% MEM vitamin solution (Gibco, Thermo Fisher Scientific); 5% Schneider’s Insect Medium (Sigma-Aldrich); 0.5 µM Hypoxanthine (Sigma-Aldrich), 1 µM hydrocortisone (Sigma-Aldrich), 1% Penicillin/Streptomycin (Gibco, Thermo Fisher Scientific), and 2% heat-inactivated Fetal Bovine Serum (Gibco, Thermo Fisher Scientific). SmJNK dsRNA was added shortly after schistosomula transformation to a final concentration of 100 nM. Cultures were kept in a Bio-Oxygen Demand incubator (B.O.D.) at 37°C, 5% CO2, and 95% humidity. Two independent biological replicates were performed. Data were analyzed using unpaired t-test with Welch’s correction.
Total RNA Extraction
RNA extraction was performed three, five, seven, and ten days after adult worm electroporation with SmJNK dsRNA. For schistosomula, the RNA extraction was performed two days after exposure to SmJNK dsRNA, as previously shown, reduction in transcript levels was greater at this exposure time (Andrade et al., 2014). For RNA extraction, TRIzol Reagent (Invitrogen) was used associated with the RNeasy Mini Kit (Qiagen) according to the manufacturer’s guidelines. Samples were treated with Turbo DNase (Ambion, Thermo Fisher Scientific) for removal of genomic DNA. RNAs were quantified using a Qubit Fluorometer 2.0 (Invitrogen) and, for RNASeq, RNA integrity was assessed using the Agilent RNA 6000 Pico kit and BioAnalyzer 2100 (Agilent Technologies).
Gene Expression Analysis by Quantitative Real-Time PCR (RT-qPCR)
The cDNA was synthesized using SuperScript™ III Reverse Transcriptase (Invitrogen, Thermo Fisher Scientific) or Illustra Ready-To-Go RT-PCR Beads (GE Healthcare). qPCR was performed using Power SYBR® Green Master mix (Applied Biosystems, Thermo Fisher Scientific) on an ABI 7500 RT-PCR system (Applied Biosystems, Thermo Fisher Scientific) to evaluate SmJNK knockdown. Primers and cycling conditions used in this study were previously designed and established by Andrade and collaborators (Andrade et al., 2014). Cytochrome C oxidase I gene (Smp_900000) was used to normalize RNA input from schistosomula (Andrade et al., 2014). FAD dependent oxidoreductase domain containing protein (SmFAD, Smp_089880) and actin-related protein 10 (Sm-arp 10, Smp_093230) genes were used to normalize RNA input from adult worms (Supplementary Table S1). In each plate, two internal controls were included to assess both reagent and genomic DNA contamination (RNA samples). Post-RNAi, SmJNK transcript levels were analyzed using the relative 2-ΔΔCt method (Livak and Schmittgen, 2001) and expressed as the percentage of difference compared to the untreated or unspecific control.
To evaluate SmJNK expression among the different stages of S. mansoni we used qPCR absolute quantification using copy number standards, employing a curve containing five points of an SmJNK clone 10-fold dilution. The copy number of each dilution was calculated by the ratio of the clone molecular mass to the Avogadro constant as described (Lee et al., 2006), and the absolute copy number of the SmJNK transcript at each stage was estimated by interpolation of the sample Ct using a standard curve, and expressed as copy number per ng of total RNA.
High-Throughput mRNA Sequencing (RNASeq)
After confirming SmJNK knockdown, 2 µg of total RNA extracted from untreated and SmJNK knocked-down schistosomula, two days after dsRNA exposure, were subjected to mRNA purification, fragmentation, cDNA synthesis, and library construction. Next generation barcode multiplexed sequencing libraries were constructed using the TruSeq stranded mRNA kit (Illumina) and pooled in equimolar concentrations. Libraries were sequenced as 100-base paired-end reads using HiSeq 2500 (Illumina) using a HiSeq Rapid SBS sequencing kit v.2 (Illumina). Raw sequence data were preprocessed using the standard Illumina pipeline to segregate multiplexed reads. Sequence quality was assessed using the FastQC program (http://www.bioinformatics.babraham.ac.uk/projects/fastqc). Reads were then mapped to the S. mansoni reference genome (v. 7) using the STAR program (v. 2.6.1d) using default parameters except the following: –outSJfilterReads Unique, –sjdbOverhang 99, –outFilterType BySJout, –outFilterMultimapNmax 20, –alignSJoverhangMin 8, –alignIntronMin 25, and –outSAMattributes All (Dobin et al., 2013).
Screening of Differentially Expressed Genes
Downstream analysis was carried out using the R statistical software package (v. 3.3.2) (R Development Core Team, 2011). To ascertain genes that significantly change after SmJNK dsRNA exposure, we used DESeq2 (v. 1.12.4) (Love et al., 2014) to normalize the data and performed statistical analysis. Genes with adjusted p-value <0.01 were considered significantly differentially expressed. Clustering analysis was performed using the heatmap function from the gplots (v. 3.0.1) package and PCA plots were generated using the ggplot2 (v. 2.1.1) package.
Gene ontology (GO) (Ashburner et al., 2000; The Gene Ontology Consortium, 2017) and the Kyoto Encyclopedia of Genes and Genomes (KEGG) (Kanehisa and Goto, 2000; Kanehisa et al., 2016; Kanehisa et al., 2017) pathway analysis were used to identify the enriched molecular functions and associated biological pathways of differentially expressed genes (DEGs). The g:Profiler tool (version e95_eg42_p13_f6e58b9) (Reimand et al., 2007; Raudvere et al., 2019) was applied to perform the GO enrichment and KEGG pathway analysis. The g:SCS method was applied for computing multiple testing correction using the padjusted values with a 0.05 threshold. The ReViGO tool (Supek et al., 2011) was used to cluster, summarize, and visualize the list of significantly enriched GO terms based on their semantic similarities (Allowed similarity: 0.4).
We also compared DEGs of schistosomula SmJNK knocked-down with those found in schistosomula Smp38 knocked-down (Avelar et al., 2019). The down-regulated DEGs that overlap in both datasets were subjected to the BioMart tool (https://parasite.wormbase.org/info/Tools/biomart.html) from WormBase ParaSite (Howe et al., 2017) to search for orthologs in the nematode Caenorhabditis elegans. The orthologous genes were then analyzed using the Worm Enrichr enrichment analysis tool for C. elegans (https://amp.pharm.mssm.edu/WormEnrichr/) to search for the RNAi phenotypes associated with this gene list (Chen et al., 2013; Kuleshov et al., 2016).
Quantitative Real-Time PCR Validation of Differentially Expressed Genes
Quantitative PCR was performed to validate the expression levels of selected differentially expressed genes. Ten genes were selected randomly; whereas five of them are among the DEGs exhibiting large fold changes (Supplementary Table S1). Specific primers were designed using PrimerQuest tool (https://www.idtdna.com/Primerquest/Home/Index) and/or Primer3 software (http://primer3.ut.ee/) and obtained from Integrated DNA Technologies (IDT). The sequences of all primers and the final concentrations established for each qPCR reaction are presented in Supplementary Table S1. PCR efficiency for each pair of specific primers was estimated by titration analysis to be 100% ± 10%. RNA extraction, purification, cDNA synthesis, and RT-qPCR reactions were carried as described above. SmFAD and Sm-arp 10 genes were used to normalize RNA input (Supplementary Table S1).
Results
SmJNK Expression Levels Among S. Mansoni Developmental Stages
The expression profile of SmJNK in developmental stages of S. mansoni (cercariae, two and seven days schistosomula, adult males, adult females, and sporocysts) was investigated by quantitative PCR. Absolute quantification was employed to assess SmJNK expression among the different developmental stages. The SmJNK gene exhibited the highest expression levels (∼4,000 copies/ng of total RNA) in two days schistosomula. This was six times the amount presented in adult males that exhibited the second highest expression levels (∼635 copies/ng of total RNA) (Figure 1A). Cercariae, sporocysts and seven days transformed schistosomula presented approximately the same amount of SmJNK transcripts.
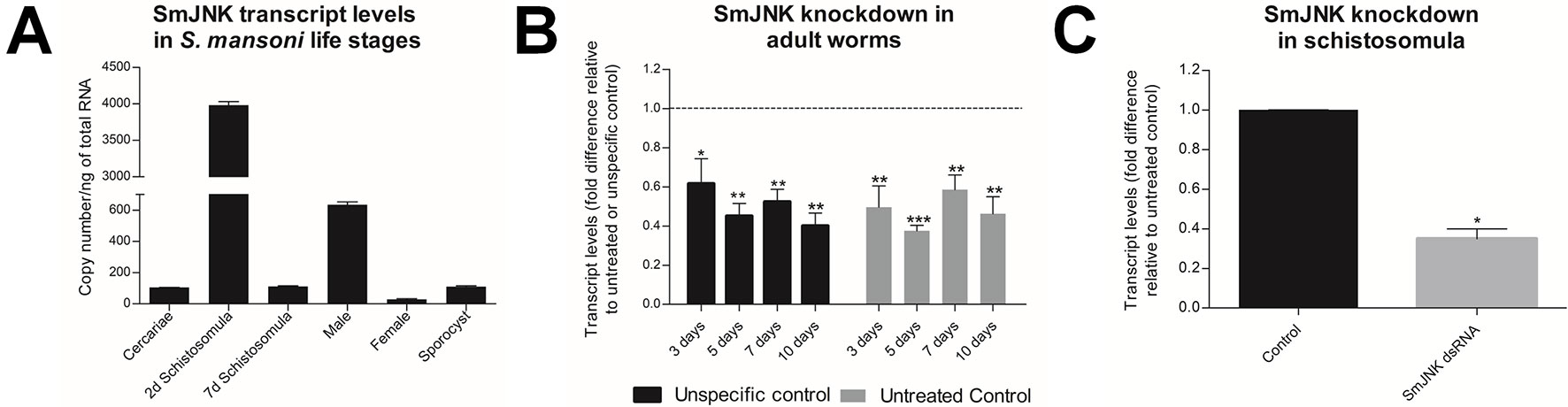
Figure 1 SmJNK expression profile among developmental stages of S. mansoni and after RNAi of adult worms in vitro. (A) SmJNK transcript levels in the different S. mansoni life stages; cercariae, two and seven days schistosomula, males, females, and sporocysts. Bar graph depicting the absolute SmJNK transcript levels presented as copy number per ng of total RNA. Bars represent the standard deviation of the mean of three technical replicates. (B) Bar graph depicting the relative SmJNK transcript levels in adult worms three, five, seven, and ten days after electroporation with SmJNK dsRNA. Bars represent the relative values of SmJNK transcripts compared to the untreated control (▪), or to the unspecific control-GFP (▪). The dashed line represents the values of the normalized controls. Data are represented as mean fold-difference (+/− SE). Asterisks represent statistically significant differences. Data were analyzed using Unpaired t-test with Welch’s correction (N=3, *p < 0.05, **p < 0.01, ***p < 0.001). (C) SmJNK transcript levels in schistosomula exposed to SmJNK dsRNA before RNASeq experiments. Bar graph depicting relative SmJNK transcripts levels in schistosomula two days after exposure to SmJNK dsRNA (▪) compared to the untreated control (▪). Data are represented as mean fold-differences (+/− SE). Transcript levels were determined by RT-qPCR and data analyzed using the ΔΔCt method (*p < 0.05, N = 2). Unpaired t test with Welch’s correction.
SmJNK Knockdown in Adult Schistosomes
Adult worms electroporated with SmJNK dsRNA showed up to 62% reduction in SmJNK transcript levels on the fifth day after electroporation (Figure 1B). After the successful establishment of SmJNK knockdown in adults, we evaluated phenotypic changes, including worm movement and oviposition. SmJNK knockdown in adult worms did not result in any significant changes in male nor female movement for ten days (Supplementary Figures S1A, B). However, a significant reduction of 67% in egg laying was observed in worms electroporated with SmJNK dsRNA (Figure 2).
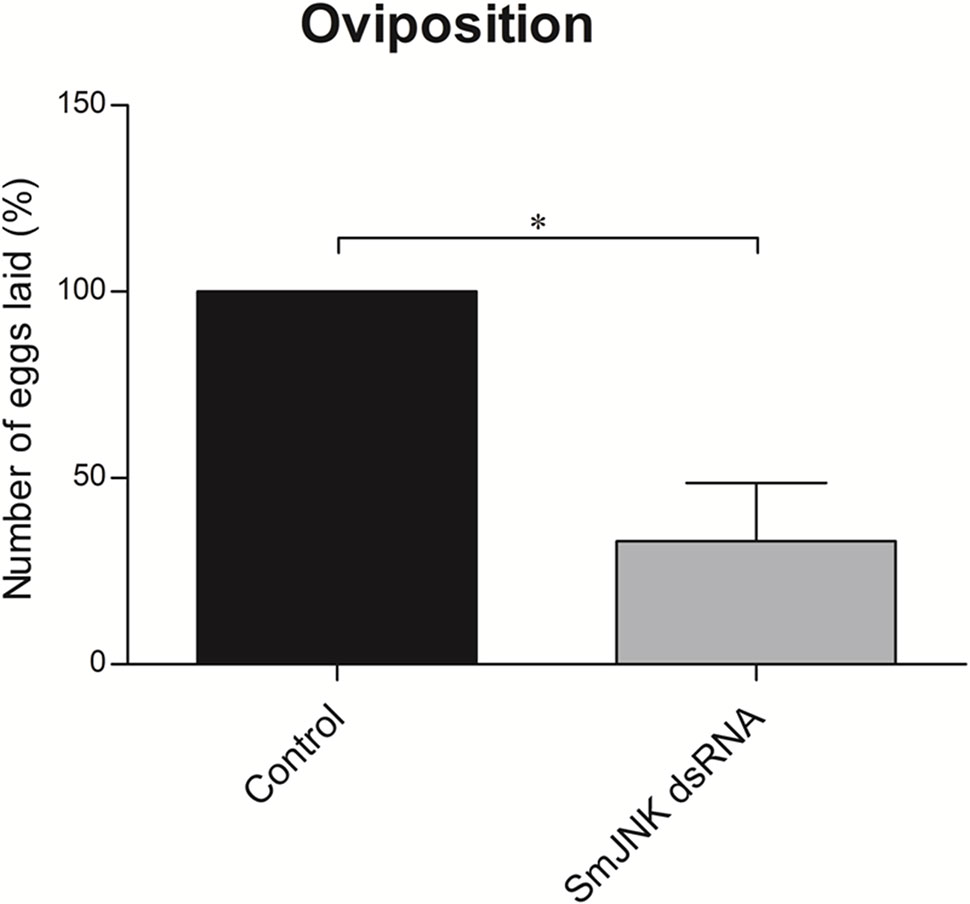
Figure 2 In vitro SmJNK knockdown effects in adult schistosome oviposition. Bar graph depicting the percentage of eggs released during 10 days after electroporation with SmJNK dsRNA (▪) compared to untreated control (▪). Data were analyzed using Unpaired t-test with Welch’s correction (N = 3; *p < 0.05).
SmJNK Knockdown in Schistosomula and Quality Assessment of RNASeq Libraries
After confirming successful SmJNK knockdown by RT-qPCR in schistosomula (Figure 1C), four paired-end libraries were generated yielding a total of 158 M paired-end reads with 100 bps as average sequence length, with 38–40% guanine-cytosine (GC) content (Table 1). FastQC quality assessment indicated that RNASeq data presented a high quality and were adequate for downstream transcriptome analysis. Raw reads were submitted to Sequence Read Archive (SRA; http://www.ncbi.nlm.nih.gov/sra), under accession numbers: PRJNA354932 and PRJNA492452. Sequence mapping to S. mansoni reference genome (v. 7) resulted in uniquely mapped reads ranging from 80.94 - 84.16%.
The correlation degree between the biological samples was investigated by hierarchical clustering using the heatmap function of the DESeq2 package and means of the Euclidean distances. Clustering analysis grouped libraries from biological replicates in the same branch (Figure 3A). Principal component analysis (PCA) was also performed with the genes by multidimensional scaling of the data matrix (Figure 3B). The PCA plot of the first two components showed a clear separation between the control and SmJNK dsRNA treated samples in the first dimension.
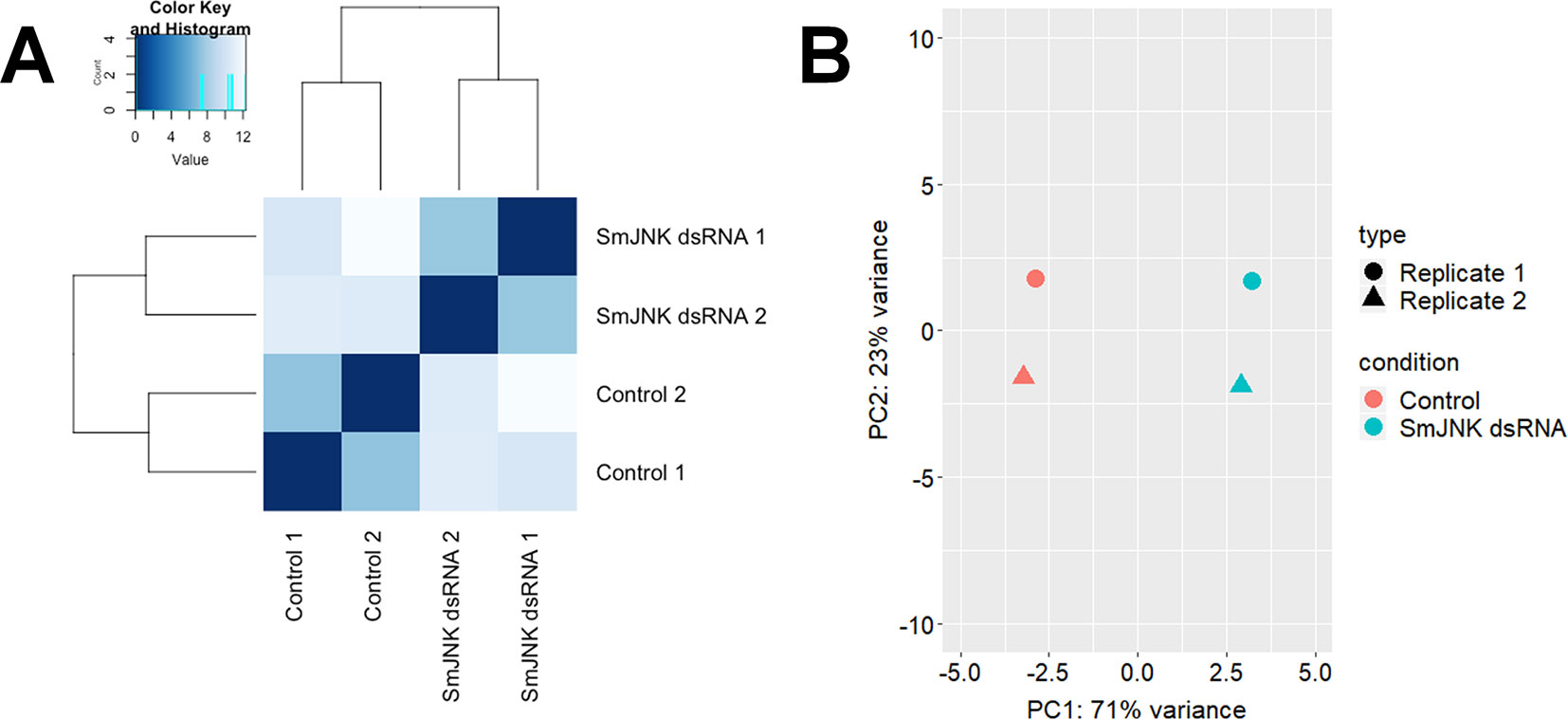
Figure 3 Correlation plots of SmJNK knocked-down schistosomula libraries. (A) Heatmap showing the Euclidean distances between the RNASeq libraries of schistosomula treated with SmJNK dsRNA and the untreated control. (B) Graph of the first two principal components (PCA) of schistosomula transcriptome expression patterns treated with SmJNK dsRNA compared to the untreated control. The colors represent each treatment: Control (orange) and SmJNK dsRNA (light blue), and the shapes represent each of the two biological replicates (circle and triangle).
Potential SmJNK Regulated Gene Targets Identified in Schistosomula After RNAi
After checking for data consistency and sample quality, the transcriptional profile of schistosomula two days after SmJNK dsRNA exposure was compared to the profile of the untreated control using the DESeq2 package in R. We found 495 DEGs using the DESeq2 package (padj <0.01) (Figure 4A). Ten of the DEGs identified among the transcripts were selected for validation by RT-qPCR (Figure 4B) (Supplementary Table S1). All the analyzed genes showed consistent expression trend between the RNASeq and RT-qPCR analyses, although with some differences in log2FoldChange values, which is expected between two different methodologies and among different biological replicates. Significant correlation (R2 = 0.7474, p = 0.0012) between the log2FoldChange of the employed methodologies confirms the accuracy of the data (Figure 4C).
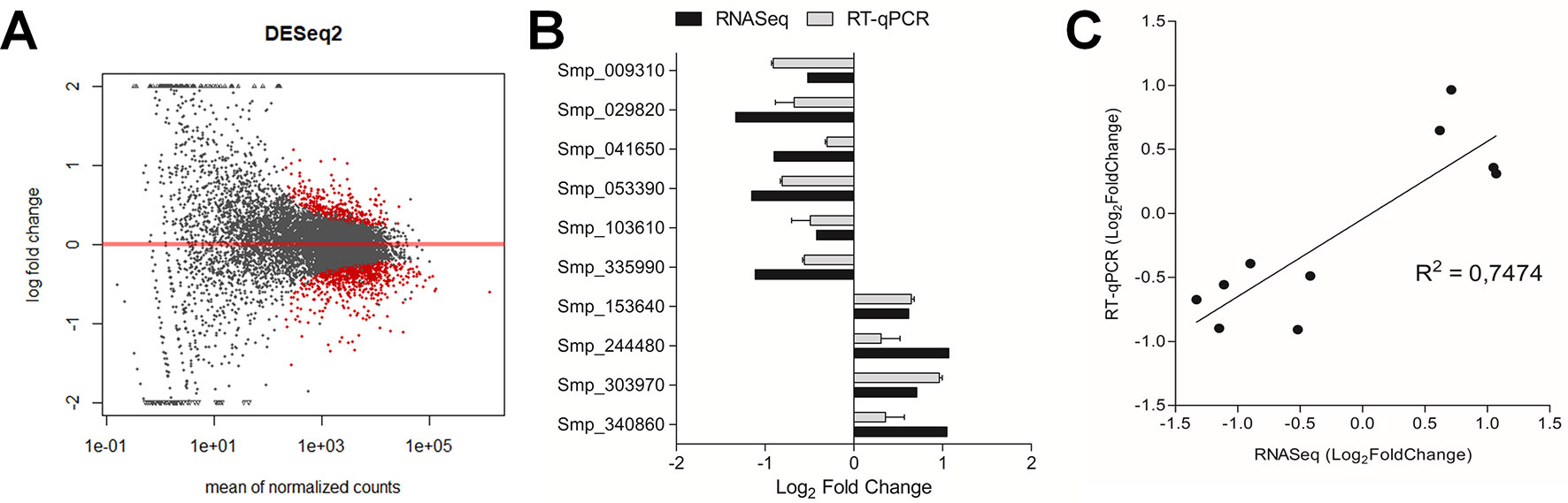
Figure 4 Differentially expressed genes (DEGs) after SmJNK knockdown in schistosomula. (A) MA plot depicting a DESeq2 analysis to identify DEGs between SmJNK dsRNA and untreated control. The log-fold change for each transcript is plotted against the mean of normalized counts; each point corresponds to one gene. Significantly altered gene expressions are highlighted in red (padj < 0.01). (B) RT-qPCR validation of the differentially expressed genes in response to SmJNK knockdown. Ten DEGs were selected from a range of up-regulated and down-regulated genes. Expression levels were quantified by RT-qPCR (gray) and the results were compared to those obtained by the RNASeq approach (black). (C) Pearson’s correlation of Log2FoldChange in differentially expressed transcripts between RNASeq and RT-qPCR analysis.
A list of all statistically significant DEGs from schistosomula exposed to SmJNK dsRNA can be found in the Supplementary Table S2. Of the 495 DEGs detected in the samples in which SmJNK expression was reduced, 373 are down-regulated and 122 up-regulated in comparison to untreated controls.
Gene Set Enrichment Analysis
The g:Profiler tool was used to elucidate the functional roles of the 495 DEGs. DEGs were categorized into three GO categories (GO Biological Process, GO Molecular Function, and GO Cell Component) and KEGG Pathways. The significantly enriched subcategories are shown in Figure 5 and Supplementary Table S3. We observed a decrease in expression for genes encoding proteins related to (1) the ribosome structural composition, (2) cytoskeleton-related, (3), purine nucleotide metabolism (4) protein folding, (5) splicing mechanisms, (6) phagosomes, and (7) binding to nucleic acids. For DEGs that showed increased expression, no subcategory showed significant enrichment (Figure 5A).
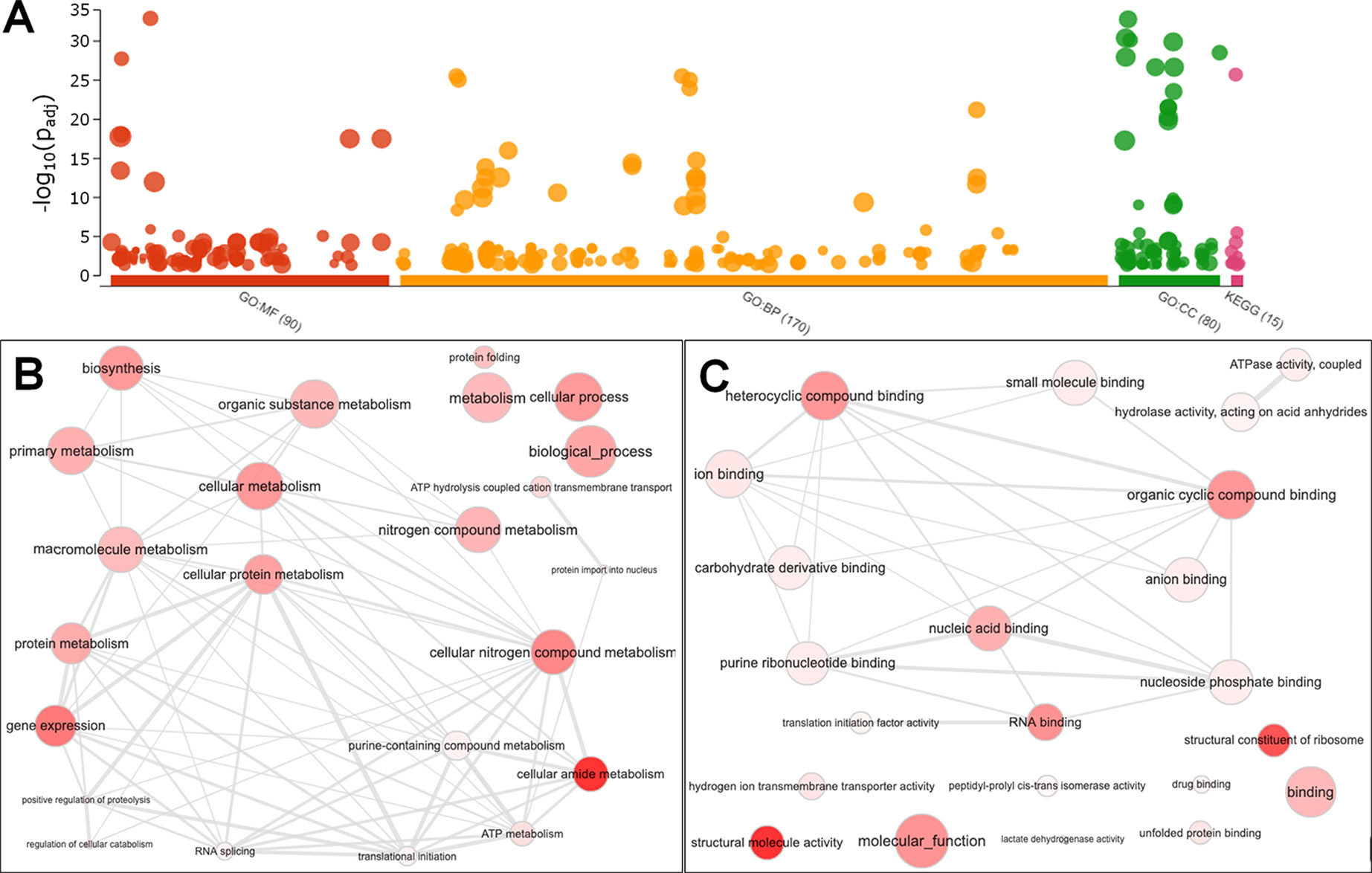
Figure 5 Gene Ontology Enrichment of down-regulated genes from SmJNK knockdown schistosomula. (A) Manhattan plot illustrating the enrichment analysis results separated into four categories: GO : MF (Molecular Function), GO : BP (Biological Process), GO : CC (Cellular Component), and KEGG Pathways. The number in the source name in the x-axis labels shows how many significantly enriched terms were found. The circle corresponds to term size. The term location on the x-axis is fixed and terms from the same GO subtree are located closer to each other. Interactive graph of the representative subset of the GO terms related to biological process (B) and molecular function (C) enriched in down-regulated genes from SmJNK knockdown schistosomula. Bubble colors indicate the p-value and size indicates the frequency of the GO term in the underlying Gene Ontology Annotation database. Highly similar GO terms are linked by edges, where the line width indicates the degree of similarity.
After summarizing the list of GO terms using the ReViGO tool, we found not redundant GO terms and ordered according to the log10 p-value. Focusing on enriched GO categories from Biological Process categories found among the down-regulated genes, we found that 23 GOs were representative in SmJNK knockdown schistosomula, due to their uniqueness, we highlight: protein folding, biosynthetic process, adenosine triphosphate (ATP) hydrolysis coupled cation transmembrane transport, ATP metabolic process, regulation of cellular catabolic process, gene expression, nitrogen compound metabolic process, RNA splicing, cellular amide metabolic process, protein import into nucleus, positive regulation of proteolysis, translational initiation, and cellular nitrogen compound metabolic process (Figure 5B, Table 2). The GO terms included in the molecular function category were summarized in 22 GO terms. We draw attention to structural constituent of ribosome, hydrogen ion transmembrane transporter activity, unfolded protein binding, lactate dehydrogenase activity, peptidyl-prolyl cis-trans isomerase activity, drug binding, translation initiation factor activity, and nucleic acid binding (Figure 5C, Table 2).
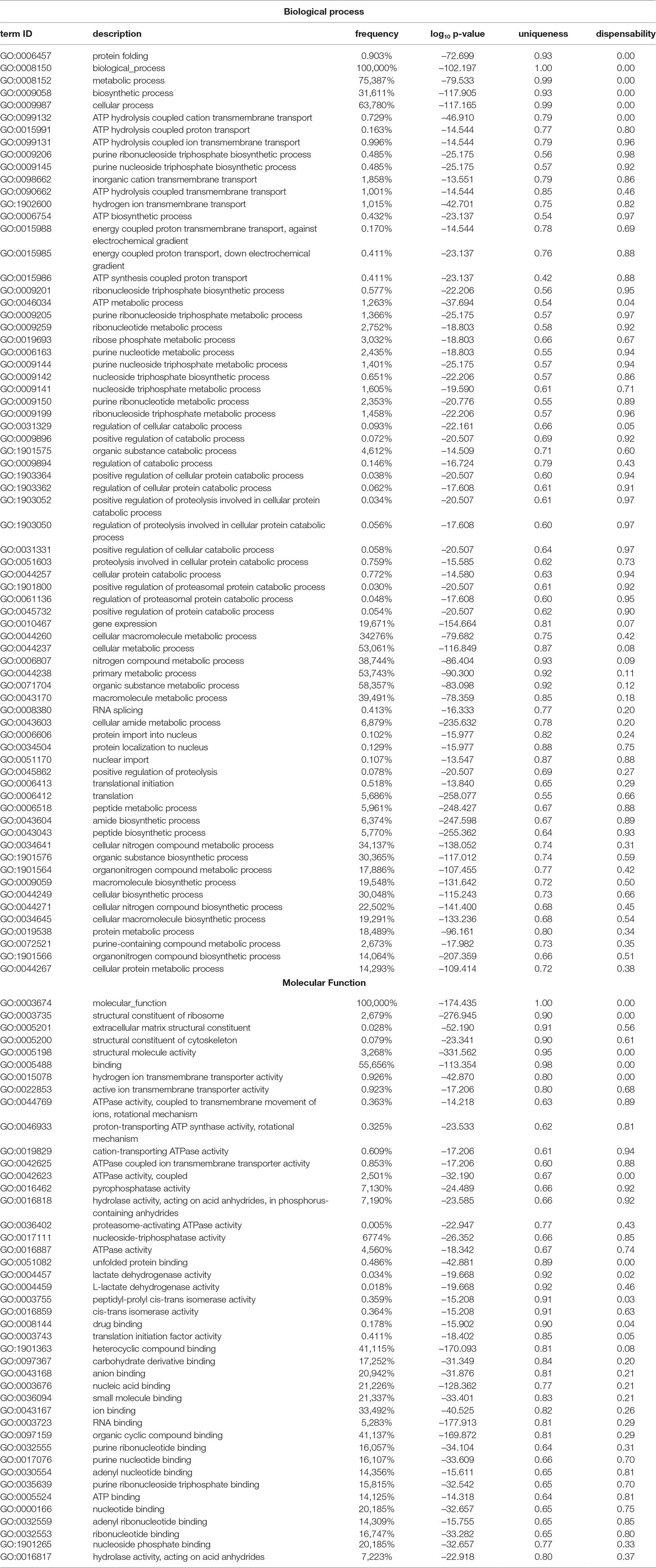
Table 2 List of enriched gene ontology (GO) from DEGs down-regulated in schistosomula SmJNK knockdown. The analysis is separated into two GO categories: Biological Process and Molecular Function. The dispensable GO terms are depicted in italic. The values of log10p-value refers to log10 of padjusted values obtained using g:Profiler toll.
Crosstalk Between SmJNK and Smp38 MAPK Pathways
Since the JNK and p38 MAPK signaling pathways are triggered by multiple stimuli that simultaneously activate both pathways and consequently share several upstream regulators (Wagner and Nebreda, 2009), we compared the DEGs found here with those found for Smp38 knocked-down schistosomula (Avelar et al., 2019). The SmJNK and Smp38 MAPK pathways in S. mansoni presented an intersection of 311 and 89 genes with decreased and increased expression, respectively (Figure 6).
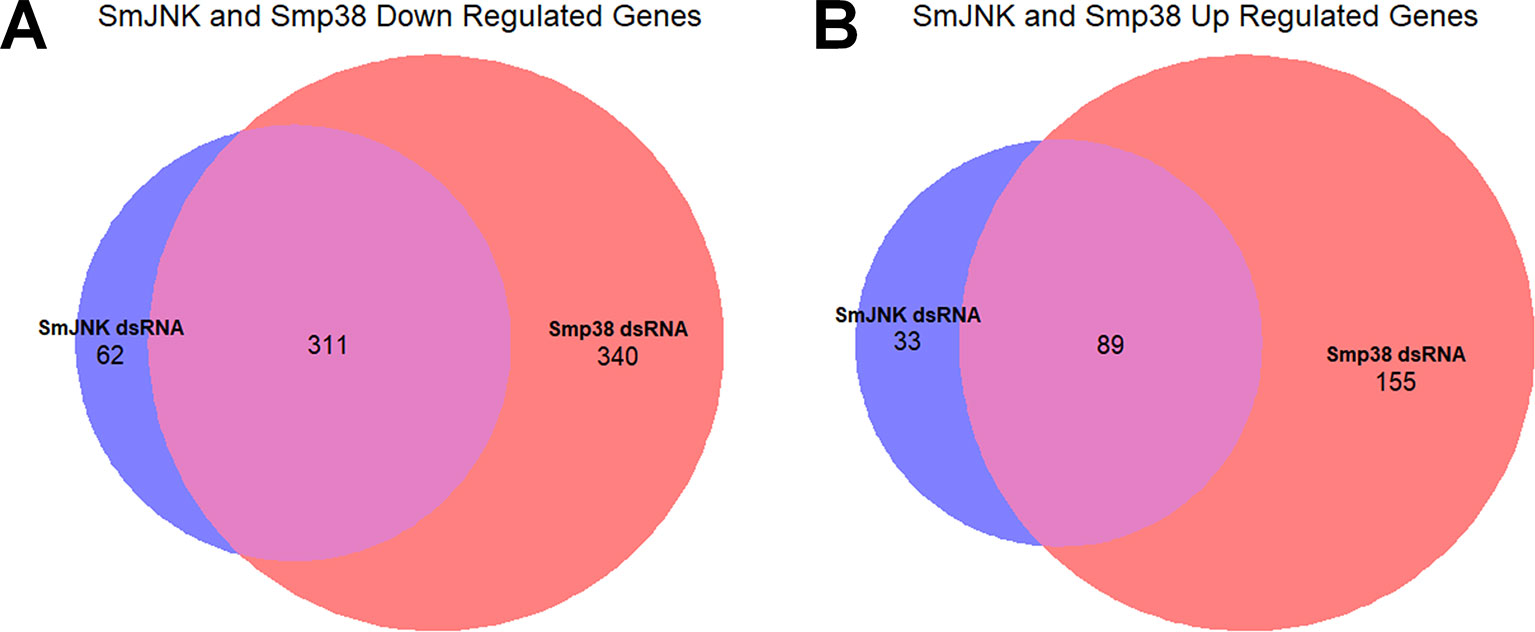
Figure 6 Overlap of DEGs in schistosomula knocked-down for SmJNK and Smp38 MAPKs. Venn diagram representing the number of genes with a decrease (A) or increase (B) in gene expression after exposure to SmJNK or Smp38 dsRNAs. In red are represented DEGs regulated by Smp38, in blue SmJNK, and in pink are the overlapping DEGs in both datasets.
Of the 311 down-regulated genes identified in common for SmJNK and Smp38 knockdown schistosomula, we found orthologs for 221 of them, which correspond to 291 different genes in C. elegans, since some of the genes present more than one ortholog. The C. elegans orthologs are enriched in genes related to the RNAi phenotypes as described: sterile, embryonic lethal, maternal sterile, larval arrest, transgene subcellular localization variant, slow growth, among others (Figure 7 and Supplementary Table S4).
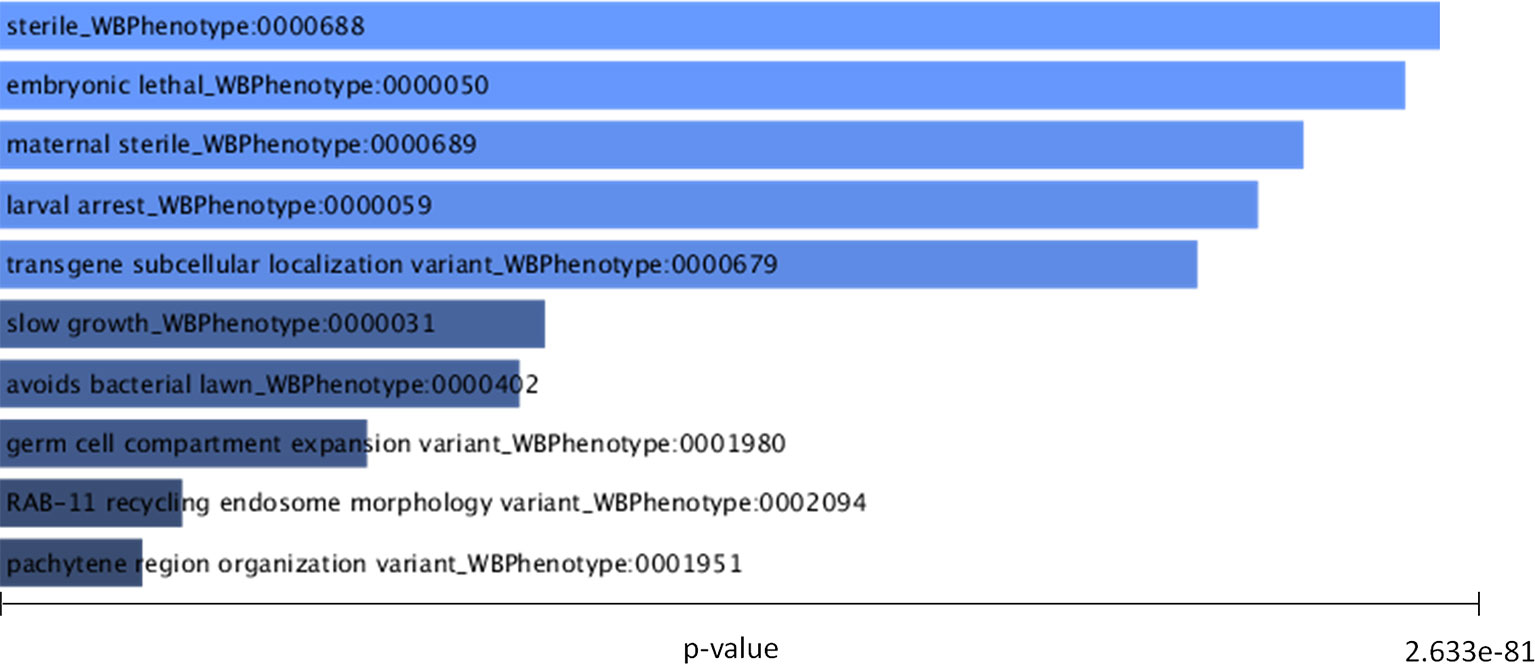
Figure 7 C. elegans RNAi phenotypes enriched in orthologues from down-regulated genes in SmJNK and Smp38 knockdown schistosomula. Worm Enrichr analysis was performed to identify the most significantly enriched RNAi phenotypes listed based on the P-value. The length of the bar represents the significance of that specific term. In addition, the brighter the color, the more significant that term is.
Discussion
We advanced the understanding of SmJNK gene function by demonstrating that SmJNK is involved in parasite reproduction in vitro. We observed a significantly lower number of eggs recovered from SmJNK knocked-down adult worms compared to the control group. It was previously shown that SmJNK knockdown decreased parasite survival and maturation within the definitive host, and that female worms treated with SmJNK dsRNA showed undifferentiated oocytes (Andrade et al., 2014). SmJNK is highly expressed in the ovary of paired females (Lu et al., 2016; Lu et al., 2017), evidencing its role in female reproduction. Therefore, in in vitro adult females, SmJNK knockdown may influence oocyte maturation, since the ovary is already fully formed prior to the SmJNK knockdown. This is particularly promising as the eggs are fundamentally related to the disease pathology. Granulomas are the result of host immune response to schistosome eggs. Its formation is triggered by immune cells migration to encapsulate the eggs, resulting in damage of liver tissue (fibrosis and, ultimately, cirrhosis) (Espírito Santo et al., 2008; King and Dangerfield-Cha, 2008; Burke et al., 2009). Additionally, in in vitro cultured adult worms, SmJNK does not seem to have a role in parasite movement or viability.
Protein kinases domains are highly conserved between organisms (Manning et al., 2002). Therefore, any drug development effort needs to consider cross-binding of a small molecules that could result in undesired potential side effects. Inhibitors need to be specific and to target essential proteins. The elucidation of gene targets regulated by SmJNK, in addition to contributing to a better understanding of the JNK signaling pathway in S. mansoni parasite, also aims at searching for parasite-specific targets. Among the identified DEGs are some proteins with unknown function, these genes could be schistosome-specific. Such proteins may be related to the previously observed phenotypes of parasite development and oviposition impairment.
We observed the down-regulation of genes related to the structural composition of ribosomes in SmJNK knockdown parasites. Ribosomal proteins are major components of ribosomes and play critical roles in protein biosynthesis. Variations observed in the expression of ribosomal proteins in different human tissues are probably related to extraribosomal functions (Bortoluzzi et al., 2001), since ribosomal proteins and RNAs are typically synthesized in stoichiometric amounts (Mager, 1988). Also, the differential expression of ribosomal proteins has been reported in several pathological (Bévort and Leffers, 2000) and stress conditions (Wang et al., 2013).
Genes related to the cytoskeleton showed decreased expression when SmJNK was knocked-down. Integrin-linked kinase (ILK) an activator of the SmJNK, extracellular signal-regulated kinase (SmERK), and Akt pathways, is involved in cytoskeletal reorganization and cell survival. The dysregulation of SmILK may contribute to errors in cell division and genomic instability (Fielding and Dedhar, 2009). The SmILK signaling pathway has an influence on egg production, ovarian structure, and oocyte integrity in female schistosomes (Gelmedin et al., 2017), with similar phenotypes to those observed for SmJNK knockdown (Andrade et al., 2014).
Among genes with decreased expression after SmJNK knockdown, we observed genes related to protein folding, such as chaperonins and heat shock proteins (HSPs). Cellular chaperones such as HSPs confer resistance to stress, promoting cell survival. Prolonged oxidative stress causes an increase in misfolded proteins that aggregate in the endoplasmic reticulum. The objective of the endoplasmic reticulum stress response is to inhibit or retard protein synthesis. This is achieved by the phosphorylation of eukaryotic translation initiation factor 2 (eIF2a) by protein kinase R–like endoplasmic reticulum kinase or by JNK (Nagiah et al., 2016). SmJNK knockdown may have inhibited this stress response, resulting in the observed down-regulation of chaperone and HSPs gene expression.
In rat fibroblasts, positive regulation of the p38 and JNK signaling pathways promotes increased expression of the plasminogen activator inhibitor 1 (PAI-1) (Nutter et al., 2015). JNK modulates positively the PAI-1 expression in the neurodegenerative amyloid pathology observed in Alzheimer’s disease (Gerenu et al., 2017), whereas JNK inhibition significantly attenuates the induction of PAI-1 (Eurlings et al., 2017). PAI-1 was not detected among DEGs in SmJNK and Smp38 knockdown schistosomula. However, the expression of plasminogen activator inhibitor 1 RNA-binding protein (SERBP1, Smp_009310), which plays a role in regulating the PAI-1 mRNA stability, was down-regulated (Figure 4B). Schistosoma inhibits host coagulation during infection through stimulation of fibrinolytic pathways (Mebius et al., 2013). The interaction between parasites and the hosts’ fibrinolytic system results in the regulation of several functions related to parasite survival mechanisms. For example, the degradation of immunoglobulins and complement components, the activation of matrix metalloproteinases, the stimulation of adhesion, and the degradation of proteins for nutrition (González-Miguel et al., 2016). The detected reduction in the SERBP1 transcript levels, could influence PAI-1 stability, therefore, may interfere in parasite feeding and/or reduce mobility in the host, thus, be related to the parasite survival impairment (Andrade et al., 2014).
SmJNK knockdown also down-regulated genes involved in RNA splicing. Alternative splicing events alter the protein repertoire of the cells being regulated by the expression patterns of the splicing factors (Grosso et al., 2008). The splicing control is as complex and relevant as the transcriptional control (Kornblihtt et al., 2004). It has already been shown that JNK signaling pathway controls splicing events in human T cells (Martinez et al., 2015). This pathway also seems to act in the regulation of alternative splicing in response to extracellular stimuli, promoting changes in splicing patterns (Pelisch et al., 2005). Moreover, Schistosoma presents trans-splicing, which is a peculiar mechanism that shares the proteins from the spliceosome, could affect up to 58% of transcripts in the cercarial stage (Boroni et al., 2018). It has been suggested as a target for parasite impairment and would be affected by the alterations detected in categories related to RNA splicing (Mourão et al., 2013).
The ability to deal with adverse environmental conditions is a fundamental need for all organisms, especially parasites. It is, therefore, not surprising that stress-activated protein kinase pathways are among the oldest and most conserved metazoan signaling modules (Twumasi-Boateng et al., 2012). The JNK and p38 MAPK pathways share several upstream regulators and, consequently, multiple stimuli simultaneously activate both pathways (Wagner and Nebreda, 2009). Several studies demonstrate that the extracellular signal-regulated kinase (ERK), JNK, and p38 MAPK signaling pathways act cooperatively, amplifying and integrating signals from various stimuli; promoting appropriate physiological responses including cell proliferation, differentiation, development, inflammatory responses, and apoptosis in mammalian cells (Zhang et al., 2002).
Orthologs of SmJNK and Smp38 in C. elegans regulate phenotypes that result in sterile nematodes. Hence, oocyte morphology alterations, decreased number or lack of this structure, and gonad alterations are redundant terms among the enriched RNAi phenotypes in the orthologs. The JNK (named kgb-1) deletion in C. elegans has a sterile phenotype, characterized by the massive presence of immature oocytes (Smith et al., 2002). The C. elegans kgb-1 deleted mutant presents low reproduction rate and shortened lifespan, concomitantly with reduced expression of genes for protein biosynthesis, chaperones, and enzymes involved in ubiquitination/proteasomal degradation (Gerke et al., 2014). All those findings corroborate the phenotypes and transcription profile associated with SmJNK and Smp38 knockdown.
Here we have shown that SmJNK activity is related to the reduction of egg production in vitro. This is in accordance with previously observed phenotype alterations in vivo, that demonstrated that SmJNK and Smp38 inhibitors could lead to sterile females, thereby reducing schistosomiasis pathology in the host. Our results point to other key regulatory proteins that are not well conserved between host and parasite, encouraging the development of small-molecule inhibitors.
Data Availability Statement
The datasets generated for this study can be found in PRJNA354932 and PRJNA492452.
Ethics Statement
This work was approved by the Oswaldo Cruz Foundation’s Ethics Committee for Animal Use (CEUA) under number LW12/16 , according to the Brazilian national guidelines set out in Law 11794/08.
Author Contributions
SG, GO, and MM contributed to conception and design of the study. SG and NT performed the experiments. SG performed the bioinformatic and statistical analysis. GO and MM contributed with reagents, materials, and analysis tools. SG, FF, and MM wrote the manuscript. All authors contributed to manuscript revision and read and approved the submitted version.
Funding
This work has been supported by funding from the European Commission’s Seventh Framework Programme for research, under Grant agreement no. 602080 (AParaDDisE), FAPEMIG (CBB-APQ-0520-13), CNPq grant (302518/2018-5) to MM; and Coordenação de Aperfeiçoamento de Pessoal de Nível Superior–Brasil (CAPES)–Finance Code 001, PCDD Programa CAPES/Nottingham University (003/2014), CNPq grants (470673/2014-1, 309312/2012-4, 304138/2014-2), CAPES (REDE 21/2015), and FAPEMIG (PPM-3500189-13) to GO. SG and NT fellowship was financed by the Coordenação de Aperfeiçoamento de Pessoal de Nível Superior-Brasil (CAPES). The authors thank the support of the Programa de Pós-graduação em Ciências da Saúde, IRR.
Conflict of Interest
The authors declare that the research was conducted in the absence of any commercial or financial relationships that could be construed as a potential conflict of interest.
Acknowledgments
The authors thank the Mollusk Room ‘Lobato Paraense’ of the René Rachou Institute–FIOCRUZ for proving the cercaria used in this study and the Program for Technological Development in Tools for Health-PDTIS FIOCRUZ for use of its facilities. We also thank Anna Salim and Flávio Araújo for their assistance in the RNASeq experimental design and libraries construction, Núbia Fernandes Braga for kindly provide RNA extracted from sporocysts, and Fábio Passetti and Ricardo Junqueira for sequencing the RNASeq libraries.
Supplementary Material
The Supplementary Material for this article can be found online at: https://www.frontiersin.org/articles/10.3389/fgene.2019.01036/full#supplementary-material
References
Andrade, L. F., Mourão, M. D. M., Geraldo, J. A., Coelho, F. S., Silva, L. L., Neves, R. H., et al. (2014). Regulation of schistosoma mansoni development and reproduction by the mitogen-activated protein kinase signaling pathway. PLoS Negl. Trop. Dis. 8, e2949. doi: 10.1371/journal.pntd.0002949
Andrade, L. F., Nahum, L. A., Avelar, L. G., Silva, L. L., Zerlotini, A., Ruiz, J. C., et al. (2011). Eukaryotic protein kinases (ePKs) of the helminth parasite schistosoma mansoni. BMC Genomics 12, 215. doi: 10.1186/1471-2164-12-215
Ashburner, M., Ball, C. A., Blake, J. A., Botstein, D., Butler, H., Cherry, J. M., et al. (2000). Gene ontology: tool for the unification of biology. The Gene Ontology Consortium. Nat. Genet. 25, 25–29. doi: 10.1038/75556
Avelar, L., das, G. A., Gava, S. G., Neves, R. H., Silva, M. C. S., Araújo, N., et al., et al. (2019). Smp38 MAP Kinase Regulation in Schistosoma mansoni: roles in survival, oviposition, and protection against oxidative stress. Front. Immunol. 10, 21. doi: 10.3389/fimmu.2019.00021
Bagowski, C. P., Xiong, W., Ferrell, J. E. (2001). c-Jun N-terminal kinase activation in Xenopus laevis eggs and embryos. A possible non-genomic role for the JNK signaling pathway. J. Biol. Chem. 276, 1459–1465. doi: 10.1074/jbc.M008050200
Berriman, M., Haas, B. J., LoVerde, P. T., Wilson, R. A., Dillon, G. P., Cerqueira, G. C., et al. (2009). The genome of the blood fluke Schistosoma mansoni. Nature 460, 352–358. doi: 10.1038/nature08160
Bévort, M., Leffers, H. (2000). Down regulation of ribosomal protein mRNAs during neuronal differentiation of human NTERA2 cells. Differentiation 66, 81–92. doi: 10.1046/j.1432-0436.2000.660203.x
Boroni, M., Sammeth, M., Gava, S. G., Jorge, N. A. N., Macedo, A. M., Machado, C. R., et al. (2018). Landscape of the spliced leader trans-splicing mechanism in Schistosoma mansoni. Sci. Rep. 8, 3877. doi: 10.1038/s41598-018-22093-3
Bortoluzzi, S., D’Alessi, F., Romualdi, C., Danieli, G. A. (2001). Differential expression of genes coding for ribosomal proteins in different human tissues. Bioinformatics 17, 1152–1157. doi: 10.1093/bioinformatics/17.12.1152
Burke, M. L., Jones, M. K., Gobert, G. N., Li, Y. S., Ellis, M. K., McManus, D. P. (2009). Immunopathogenesis of human schistosomiasis. Parasite Immunol. 31, 163–176. doi: 10.1111/j.1365-3024.2009.01098.x
Cai, P., McManus, D. P., You, H. (2017). Signalling pathways in schistosomes: novel targets for control interventions against schistosomiasis. Emerg. Top. Life Sci. 1, 633–639. doi: 10.1042/ETLS20170093
Chen, E. Y., Tan, C. M., Kou, Y., Duan, Q., Wang, Z., Meirelles, G., et al. (2013). Enrichr: interactive and collaborative HTML5 gene list enrichment analysis tool. BMC Bioinformatics 14, 128. doi: 10.1186/1471-2105-14-128
Cohen, P. (2002). Protein kinases–the major drug targets of the twenty-first century? Nat. Rev. Drug Discov. 1, 309–315. doi: 10.1038/nrd773
Dobin, A., Davis, C. A., Schlesinger, F., Drenkow, J., Zaleski, C., Jha, S., et al. (2013). STAR: Ultrafast universal RNA-seq aligner. Bioinformatics 29, 15–21. doi: 10.1093/bioinformatics/bts635
Espírito Santo, M. C. C., Azeredo, L. M., Teles, H. M. S., Gryschek, R. C. B., Ferreira, C. S., Amato Neto, V. (2008). Abdominal ultrasound in the evaluation of fibrosis and portal hypertension in an area of schistosomiasis low endemicity. Rev. Inst. Med. Trop. Sao Paulo 50, 117–119. doi: 10.1590/S0036-46652008000200010
Eurlings, I. M. J. J., Reynaert, N. L., Van De Wetering, C., Aesif, S. W., Mercken, E. M., de Cabo, R., et al. (2017). Involvement of c-Jun N-Terminal Kinase in TNF-α-driven remodeling. Am. J. Respir. Cell Mol. Biol. 56, 393–401. doi: 10.1165/rcmb.2015-0195OC
Fielding, A. B., Dedhar, S. (2009). The mitotic functions of integrin-linked kinase. Cancer Metastasis Rev. 28, 99–111. doi: 10.1007/s10555-008-9177-0
French, M. D., Evans, D., Fleming, F. M., Secor, W. E., Biritwum, N.-K., Brooker, S. J., et al. (2018). Schistosomiasis in Africa: improving strategies for long-term and sustainable morbidity control. PLoS Negl. Trop. Dis. 12, e0006484. doi: 10.1371/journal.pntd.0006484
Gelmedin, V., Morel, M., Hahnel, S., Cailliau, K., Dissous, C., Grevelding, C. G. (2017). Evidence for integrin – venus kinase receptor 1 alliance in the ovary of schistosoma mansoni females controlling cell survival. PLoS Pathog. 13, e1006147. doi: 10.1371/journal.ppat.1006147
Gerenu, G., Martisova, E., Ferrero, H., Carracedo, M., Rantamäki, T., Ramirez, M. J., et al. (2017). Modulation of BDNF cleavage by plasminogen-activator inhibitor-1 contributes to Alzheimer’s neuropathology and cognitive deficits. Biochim. Biophys. Acta Mol. Basis Dis. 1863, 991–1001. doi: 10.1016/j.bbadis.2017.01.023
Gerke, P., Keshet, A., Mertenskötter, A., Paul, R. J. (2014). The JNK-Like MAPK KGB-1 of Caenorhabditis elegans promotes reproduction, lifespan, and gene expressions for protein biosynthesis and germline homeostasis but interferes with hyperosmotic stress tolerance. Cell Physiol. Biochem. 34, 1951–1973. doi: 10.1159/000366392
González-Miguel, J., Siles-Lucas, M., Kartashev, V., Morchón, R., Simón, F. (2016). Plasmin in parasitic chronic infections: friend or Foe? Trends Parasitol. 32, 325–335. doi: 10.1016/j.pt.2015.12.012
Grevelding, C. G., Langner, S., Dissous, C. (2017). Kinases: Molecular Stage directors for schistosome development and differentiation. Trends Parasitol. xx, 1–15. doi: 10.1016/j.pt.2017.12.001
Grosso, A. R., Gomes, A. Q., Barbosa-Morais, N. L., Caldeira, S., Thorne, N. P., Grech, G., et al. (2008). Tissue-specific splicing factor gene expression signatures. Nucleic Acids Res. 36, 4823–4832. doi: 10.1093/nar/gkn463
Howe, K. L., Bolt, B. J., Shafie, M., Kersey, P., Berriman, M. (2017). WormBase ParaSite - a comprehensive resource for helminth genomics. Mol. Biochem. Parasitol. 215, 2–10. doi: 10.1016/j.molbiopara.2016.11.005
Huang, X., Tong, J., Wang, Z., Yang, C., Qi, S.-T., Guo, L., et al. (2011). JNK2 Participates in Spindle Assembly during Mouse Oocyte Meiotic Maturation. Microsc. Microanal. 17, 197–205. doi: 10.1017/S1431927610094456
Kanehisa, M., Furumichi, M., Tanabe, M., Sato, Y., Morishima, K. (2017). KEGG: New perspectives on genomes, pathways, diseases and drugs. Nucleic Acids Res. 45, D353–D361. doi: 10.1093/nar/gkw1092
Kanehisa, M., Goto, S. (2000). KEGG: kyoto encyclopedia of genes and genomes. Nucleic Acids Res. 28, 27–30. doi: 10.1093/nar/27.1.29
Kanehisa, M., Sato, Y., Kawashima, M., Furumichi, M., Tanabe, M. (2016). KEGG as a reference resource for gene and protein annotation. Nucleic Acids Res. 44, D457–D462. doi: 10.1093/nar/gkv1070
King, C. H., Dangerfield-Cha, M. (2008). The unacknowledged impact of chronic schistosomiasis. Chronic Illn. 4, 65–79. doi: 10.1177/1742395307084407
Kornblihtt, A. R., de la Mata, M., Fededa, J. P., Munoz, M. J., Nogues, G. (2004). Multiple links between transcription and splicing. RNA 10, 1489–1498. doi: 10.1261/rna.7100104
Kuleshov, M. V., Jones, M. R., Rouillard, A. D., Fernandez, N. F., Duan, Q., Wang, Z., et al. (2016). Enrichr: a comprehensive gene set enrichment analysis web server 2016 update. Nucleic Acids Res. 44, W90–W97. doi: 10.1093/nar/gkw377
Lee, C., Kim, J., Shin, S. G., Hwang, S. (2006). Absolute and relative QPCR quantification of plasmid copy number in Escherichia coli. J. Biotechnol. 123, 273–280. doi: 10.1016/j.jbiotec.2005.11.014
Livak, K. J., Schmittgen, T. D. (2001). Analysis of relative gene expression data using real-time quantitative PCR and the 2(-Delta Delta C(T)) Method. Methods 25, 402–408. doi: 10.1006/meth.2001.1262
Love, M. I., Huber, W., Anders, S. (2014). Moderated estimation of fold change and dispersion for RNA-seq data with DESeq2. Genome Biol. 15, 1–21. doi: 10.1186/s13059-014-0550-8
Lu, Z., Sessler, F., Holroyd, N., Hahnel, S., Quack, T., Berriman, M., et al. (2016). Schistosome sex matters: a deep view into gonad-specific and pairing-dependent transcriptomes reveals a complex gender interplay. Sci. Rep. 6, 31150. doi: 10.1038/srep31150
Lu, Z., Sessler, F., Holroyd, N., Hahnel, S., Quack, T., Berriman, M., et al. (2017). Data Descriptor: a gene expression atlas of adult Schistosoma mansoni and their gonads. Sci. Data 4, 1–9. doi: 10.1038/sdata.2017.118
Mager, W. H. (1988). Control of ribosomal protein gene expression. Biochim. Biophys. Acta 949, 1–15. doi: 10.1016/0167-4781(88)90048-6
Manning, G., Whyte, D. B., Martinez, R., Hunter, T., Sudarsanam, S. (2002). The protein kinase complement of the human genome. Science 298, 1912–34. doi: 10.1126/science.1075762
Marcellino, C., Gut, J., Lim, K. C., Singh, R., McKerrow, J., Sakanari, J. (2012). WormAssay: a novel computer application for whole-plate motion-based screening of macroscopic parasites. PLoS Negl. Trop. Dis. 6, e1494. doi: 10.1371/journal.pntd.0001494
Martinez, N. M., Agosto, L., Qiu, J., Mallory, M. J., Gazzara, M. R., Barash, Y., et al. (2015). Widespread JNK-dependent alternative splicing induces a positive feedback loop through CELF2-mediated regulation of MKK7 during T-cell activation. Genes Dev. 29, 2054–2066. doi: 10.1101/gad.267245.115
Mebius, M. M., van Genderen, P. J. J., Urbanus, R. T., Tielens, A. G. M., de Groot, P. G., van Hellemond, J. J. (2013). Interference with the host haemostatic system by schistosomes. PLoS Pathog. 9, 1–8. doi: 10.1371/journal.ppat.1003781
Milligan, J. N., Jolly, E. R. (2011). Cercarial transformation and in vitro cultivation of Schistosoma mansoni schistosomules. J. Vis. Exp. 54, 4–7. doi: 10.3791/3191
Mourão, M., de, M., Bitar, M., Lobo, F. P., Peconick, A. P., Grynberg, P., et al., et al. (2013). A directed approach for the identification of transcripts harbouring the spliced leader sequence and the effect of trans-splicing knockdown in Schistosoma mansoni. Mem. Inst. Oswaldo Cruz 108, 707–717. doi: 10.1590/0074-0276108062013006
Mourão, M. M., de Moraes Mourão, M., Dinguirard, N., Franco, G. R., Yoshino, T. P. (2009). Phenotypic screen of early-developing larvae of the blood fluke, Schistosoma mansoni, using RNA interference. PLoS Negl. Trop. Dis. 3, e502. doi: 10.1371/journal.pntd.0000502
Nagiah, S., Phulukdaree, A., Chuturgoon, A. A. (2016). Lon protease and eiF2α are involved in acute, but not prolonged, antiretroviral induced stress response in HepG2 cells. Chem. Biol. Interact. 252, 82–86. doi: 10.1016/j.cbi.2016.03.021
Nascimento, G. L., Pegado, H. M., Domingues, A. L. C., Ximenes, R. A., de, A., Itria, A., et al., et al. (2019). The cost of a disease targeted for elimination in Brazil: the case of schistosomiasis mansoni. Mem. Inst. Oswaldo Cruz 114, 1–9. doi: 10.1590/0074-02760180347
Nutter, F. H., Haylor, J. L., Khwaja, A. (2015). Inhibiting ERK activation with CI-1040 leads to compensatory upregulation of alternate MAPKs and plasminogen activator inhibitor- 1 following subtotal nephrectomy with no impact on kidney fibrosis. PLoS One 10, 1–18. doi: 10.1371/journal.pone.0137321
Pelisch, F., Blaustein, M., Kornblihtt, A. R., Srebrow, A. (2005). Cross-talk between signaling pathways regulates alternative splicing: A novel role for JNK. J. Biol. Chem. 280, 25461–25469. doi: 10.1074/jbc.M412007200
Pellegrino, J., Siqueira, A. F. (1956). A perfusion technic for recovery of Schistosoma mansoni from experimentally infected guinea pigs. Rev. Bras. Malariol. Doencas Trop. 8, 589–597.
Raudvere, U., Kolberg, L., Kuzmin, I., Arak, T., Adler, P., Peterson, H., et al. (2019). g:Profiler: a web server for functional enrichment analysis and conversions of gene lists (2019 update). Nucleic Acids Res. 47, W191–W198. doi: 10.1093/nar/gkz369
Reimand, J., Kull, M., Peterson, H., Hansen, J., Vilo, J. (2007). g:Profiler–a web-based toolset for functional profiling of gene lists from large-scale experiments. Nucleic Acids Res. 35, W193–W200. doi: 10.1093/nar/gkm226
Smith, P., Leung-Chiu, W. M., Montgomery, R., Orsborn, A., Kuznicki, K., Gressman-Coberly, E., et al. (2002). The GLH proteins, Caenorhabditis elegans P granule components, associate with CSN-5 and KGB-1, proteins necessary for fertility, and with ZYX-1, a predicted cytoskeletal protein. Dev. Biol. 251, 333–347. doi: 10.1006/dbio.2002.0832
Stroehlein, A. J., Young, N. D., Jex, A. R., Sternberg, P. W., Tan, P., Boag, P. R., et al. (2015). Defining the Schistosoma haematobium kinome enables the prediction of essential kinases as anti-schistosome drug targets. Sci. Rep. 5, 17759. doi: 10.1038/srep17759
Supek, F., Bošnjak, M., Škunca, N., Šmuc, T. (2011). REVIGO summarizes and visualizes long lists of gene ontology terms. PLoS One 6, e21800. doi: 10.1371/journal.pone.0021800
The Gene Ontology Consortium (2017). Expansion of the Gene Ontology knowledgebase and resources. Nucleic Acids Res. 45, D331–D338. doi: 10.1093/nar/gkw1108
Twumasi-Boateng, K., Wang, T. W., Tsai, L., Lee, K. H., Salehpour, A., Bhat, S., et al. (2012). An age-dependent reversal in the protective capacities of JNK signaling shortens Caenorhabditis elegans lifespan. Aging Cell 11, 659–667. doi: 10.1111/j.1474-9726.2012.00829.x
Wagner, E. F., Nebreda, A. R. (2009). Signal integration by JNK and p38 MAPK pathways in cancer development. Nat. Rev. Cancer 9, 537–549. doi: 10.1038/nrc2694
Wang, J., Lan, P., Gao, H., Zheng, L., Li, W., Schmidt, W. (2013). Expression changes of ribosomal proteins in phosphate- and iron-deficient Arabidopsis roots predict stress-specific alterations in ribosome composition. BMC Genomics 14, 783. doi: 10.1186/1471-2164-14-783
WHO (2018). Weekly epidemiological record:Schistosomiasis and soil- transmitted helminthiases: numbers of people treated in 2017. Wkly. Epidemiol. Rec. III, 445–452. doi: 10.1016/j.actatropica.2012.04.013
Keywords: Schistosoma mansoni, mitogen-activated protein kinases, c-Jun N-terminal kinase, RNA interference, signaling pathways, gene expression
Citation: Gava SG, Tavares NC, Falcone FH, Oliveira G and Mourão MM (2019) Profiling Transcriptional Regulation and Functional Roles of Schistosoma mansoni c-Jun N-Terminal Kinase. Front. Genet. 10:1036. doi: 10.3389/fgene.2019.01036
Received: 28 June 2019; Accepted: 27 September 2019;
Published: 18 October 2019.
Edited by:
Jose F Tort, University of the Republic, UruguayReviewed by:
Sergio Verjovski-Almeida, Butantan Institute, BrazilPengfei Cai, QIMR Berghofer Medical Research Institute, Australia
Thomas Quack, University of Giessen, Germany
Copyright © 2019 Gava, Tavares, Falcone, Oliveira and Mourão. This is an open-access article distributed under the terms of the Creative Commons Attribution License (CC BY). The use, distribution or reproduction in other forums is permitted, provided the original author(s) and the copyright owner(s) are credited and that the original publication in this journal is cited, in accordance with accepted academic practice. No use, distribution or reproduction is permitted which does not comply with these terms.
*Correspondence: Marina Moraes Mourão, bWFyaW5hLm1vdXJhb0BmaW9jcnV6LmJy