- GenPhySE, Université de Toulouse, INRAE, ENVT, Castanet-Tolosan, France
The search for the genetic determinism of prolificacy variability in sheep has evidenced several major mutations in genes playing a crucial role in the control of ovulation rate. In the Noire du Velay (NV) sheep population, a recent genetic study has evidenced the segregation of such a mutation named FecLL. However, based on litter size (LS) records of FecLL non-carrier ewes, the segregation of a second prolificacy major mutation was suspected in this population. In order to identify this mutation, we have combined a case/control genome-wide association study with ovine 50k SNP chip genotyping, whole genome sequencing, and functional analyses. A new single nucleotide polymorphism (OARX:50977717T > A, NC_019484) located on the X chromosome upstream of the BMP15 gene was evidenced to be highly associated with the prolificacy variability (P = 1.93E-11). The variant allele was called FecXN and shown to segregate also in the Blanche du Massif Central (BMC) sheep population. In both NV and BMC, the FecXN allele frequency was estimated close to 0.10, and its effect on LS was estimated at +0.20 lamb per lambing at the heterozygous state. Homozygous FecXN carrier ewes were fertile with increased prolificacy in contrast to numerous mutations affecting BMP15. At the molecular level, FecXN was shown to decrease BMP15 promoter activity and supposed to impact BMP15 expression in the oocyte. This regulatory action was proposed as the causal mechanism for the FecXN mutation to control ovulation rate and prolificacy in sheep.
Introduction
There is now an accumulation of evidence that the oocyte plays a central role in controlling the ovarian folliculogenesis from the early stages up to ovulation. Among the local factors produced by the oocyte itself, members of the bone morphogenetic protein/growth and differentiation factor (BMP/GDF) family play an integral role in this control (Persani et al., 2014). Among them, the most important are surely BMP15 and GDF9. Knockout mice models gave the first evidence of the importance of these two oocyte-derived factors acting individually as homodimers and/or through a synergistic cooperation to control the ovarian function (Elvin et al., 1999; Yan et al., 2001).
In humans also, a focus was done on BMP15 and GDF9 about their implication in various ovarian dysfunctions. Indeed, numerous heterozygous missense mutations have been identified in both genes associated with primary or secondary amenorrhea in different cohorts of women affected by primary ovarian insufficiency (POI) all over the world. Particularly, the 10-fold higher prevalence of BMP15 variants among patients with POI compared with the control population supports the causative role of these mutations (Persani et al., 2014). Alterations of BMP15 and GDF9 were also searched in association with polycystic ovary syndrome (PCOS). Here, again, several missense variants were discovered in both genes, but the pathogenic role of these mutations remains controversial in the etiology of this syndrome. However, several studies have reported an aberrant expression of BMP15 and GDF9 in the ovary of PCOS patients (Teixeira Filho et al., 2002; Wei et al., 2014). Interestingly, some BMP15 polymorphisms situated in the 5′UTR are significantly associated with the over-response to recombinant FSH applied during assisted reproductive treatment and with the risk to develop ovarian hyper-stimulation syndrome (OHSS, Morón et al., 2006; Hanevik et al., 2011). Finally, polymorphisms in BMP15 and GDF9 genes were also searched in association with dizygotic twinning in humans. If no convincing results were obtained for BMP15, some loss-of-function variants of GDF9 were observed significantly more frequently in mothers of twins compared to the control population (Palmer et al., 2006).
In parallel, the search for the genetic determinism of ovulation rate and prolificacy variability in sheep has also highlighted the crucial role of BMP15 and GDF9 by evidencing numerous independent loss-of-function mutations, all altering the coding sequence of these two genes (Persani et al., 2014; Abdoli et al., 2016). Depending on the mutation and its hetero- or homozygous state, the phenotype controlled by these mutations in BMP15 and GDF9 goes from the early blockade of folliculogenesis and subsequent sterility to an extraordinary increase of ovulation rate (OR) and, thus, litter size (LS) of carrier ewes (Galloway et al., 2000; Hanrahan et al., 2004; Silva et al., 2011; Demars et al., 2013). Thus, sheep exhibiting an extremely high prolificacy are of great interest for identifying genes and mutations involved in molecular pathways controlling ovarian function. These animal models have a double interest in agriculture for genetic improvement of the prolificacy and in human clinics for providing valuable candidate genes in the genetic determinism of female infertility or subfertility as described above.
The Noire du Velay (NV) population is a French local sheep breed mainly reared in the Haute-Loire and Loire departments. Ewes present naturally out-of-season breeding ability, very good maternal characteristics, and a quite high prolificacy (mean LS = 1.62 lamb per lambing). Large variation in LS has been observed in this breed, and a recent genetic study has evidenced the segregation of an autosomal mutation named FecLL controlling this trait (Chantepie et al., 2018). This variant, located in intron 7 of the B4GALNT2 gene and associated with its ectopic ovarian expression, was originally discovered in the Lacaune meat sheep breed, increasing OR and prolificacy (Drouilhet et al., 2013). For the segregation study, more than 2700 NV ewes with LS records were genotyped at the FecL locus (Chantepie et al., 2018). Surprisingly, the distribution of LS and the existence of highly prolific ewes among the FecLL non-carriers have suggested the possible segregation of a second prolificacy major mutation in this population as previously observed in the Lacaune breed carrying both FecLL and FecXL (Bodin et al., 2007; Drouilhet et al., 2013). In order to validate this hypothesis, after specific genotyping excluding all other known mutations affecting OR and LS and segregating in French sheep populations, we have performed a genome-wide association study (GWAS) based on a case/control design. Completed by the whole-genome sequencing of two specifically chosen animals based on their genotypes, we have identified a new regulatory variant called FecXN proposed to affect the oocyte-dependent expression of BMP15 in association with increased prolificacy in sheep.
Materials and Methods
Animals
Ewes (Ovis aries) from the NV breed (n = 2266) were genotyped on blood DNA at the FecL locus as previously described (Chantepie et al., 2018). In order to test the hypothesis of the segregation of a second major mutation controlling LS in this breed, a first set of 80 ewes with at least 5 LS records (mean LS = 1.84, ranging from 1.00 to 3.50) were selected among the FecL+ homozygous genotype (n = 2151, mean LS = 1.58). Subsequently, for the NV breed, the effect of the FecXN mutation on LS was estimated on 2252 ewes, considering the genotype at the FecL locus. The presence of the FecXN mutation in other breeds was checked on a diversity panel of 725 animals from 26 French sheep breeds (Rochus et al., 2018; Table 3). For the BMC population, the effect of the FecXN mutation on LS was estimated on 2456 ewes. For gene expression analysis, 10 homozygous ewes at the FecXN locus (five carriers and five non-carriers of the N allele) were bought from private breeders (six NV and four BMC) and reared at the INRA experimental facility (agreement number: D3142901). All experimental procedures were approved (approval number 01171.02) by the French Ministry of Teaching and Scientific Research and local ethical committee C2EA-115 (Science and Animal Health) in accordance with the European Union Directive 2010/63/EU on the protection of animals used for scientific purposes.
Biological Samples
All blood sampling from the numerous sheep breeds studied were collected from the jugular vein (5 ml per animal) by the Venoject system with EDTA and directly stored at −20°C for further use. Part of these blood samples (GWAS and diversity panel) was used for extraction of genomic DNA as described (Bodin et al., 2007). All other samples were used for direct genotyping on whole blood without DNA purification (Chantepie et al., 2018).
For ovary collection and oocyte isolation, the estrus cycles of all adult NV and BMC ewes were synchronized with intravaginal sponges impregnated with flugestone acetate (FGA, 30 mg, CEVA) for 14 days. Ovaries were collected at slaughtering during the follicular phase 36 h after FGA sponge removal. Cumulus-oocyte complexes (COC) were immediately recovered from all visible 1–3 mm follicles by aspiration using a 1 ml syringe with a 26 G needle and placed in McCoy’s 5A culture medium (Sigma-Aldrich). COC were mechanically dissociated by several pipetting and washing cycles in 150 μl drops of McCoy’s 5A medium, and finally, denuded oocytes devoid of granulosa cells were recovered in 1X PBS. Only intact oocytes with a good homogeneity of the cytoplasm were grouped to obtain two to three pools of five oocytes per animal and stored at −80°C before RNA extraction.
Genotyping Analyses
The FecLL mutation (OAR11:36938224T > A, NC_019468) was genotyped directly on whole blood samples by the KAPA-KASP assay as previously described (Chantepie et al., 2018). As a prerequisite before GWAS, a set of 30 highly prolific FecL+/FecL+ ewes was controlled for the absence of other evidenced major mutations affecting sheep prolificacy in French populations. Using the same KAPA-KASP assay, FecXL and FecXGr alleles in BMP15 were genotyped as described (Chantepie et al., 2018). FecBB in the exon 7 of the BMPR1B gene (OAR6:29382188A > G, NC_019463.1) was genotyped using forced restriction fragment length polymorphism (RFLP) as described by Wilson et al. (2001).
Whole-genome genotyping was performed on 80 ovine genomic DNA samples using the OvineSNP50 Genotyping Beadchip from Illumina according to the manufacturer’s protocol at the Laboratoire d’Analyses Geìneìtiques pour les espèces Animales (LABOGENA, Jouy en Josas, France)1. From the data set, individuals with a call rate < 0.98 were excluded. SNP exclusion thresholds were call frequency < 0.95 and minor allele frequency (MAF) < 0.01 or a significant deviation from Hardy–Weinberg equilibrium (HWE) in the controls (p < 1.10–6). Non-polymorphic SNP positions and markers with no position on the OARv3.1 reference genome map were also discarded. Finally, from the available design of 54,241 SNPs available on the Illumina OvineSNP50 Beadchip and 80 selected NV ewes, the final data set was reduced to 47,446 SNPs analyzed in 79 individuals.
The FecXN mutation (OARX: 50977717T > A, NC_019484) was genotyped by a RFLP analysis using the Mse1 restriction enzyme (New England Biolabs) after a first step of Terra PCR Direct Polymerase Mix amplification (Takara) using 1 μl sample of total blood. The accuracy of the FecXN RFLP genotyping was validated by Sanger sequencing on few samples using the same amplification primers.
PCR amplifications were conducted independently for each locus studied on an ABI2400 thermocycler (Applied Biosystems) with the following conditions: 5 min at 94°C, 32 cycles of 30 s at the specific melting temperature, 30 s at 72°C and 30 s at 94°C, followed by 5 min at 72°C. The primers used in this study are listed in Supplementary Table S2.
Whole-Genome Sequencing (WGS) Analysis
DNA sequencing libraries were constructed from 1 μg of genomic DNA using TruSeq DNA PCR-free Library Prep kit (Illumina). Sequencing was run on an Illumina HiSeq 2500 apparatus using a paired-end read length of 2 × 150 pb with the Illumina Reagent Kits as previously described (Demars et al., 2017). WGS was performed at the Genotoul-GeT core facility (INRA Toulouse)2. The raw reads of Illumina DNA sequencing were preprocessed by removing adapter sequences. After quality control, the FastQ files and metadata were submitted to the European Nucleotide Archive (ENA) at EMBL-EBI (accession number PRJEB35553). Reads mapping and variants calling were performed using the local instance of Galaxy3 at the Toulouse Midi-Pyrénées bioinformatics platform4. The cleaned paired reads were combined and mapped against the ovine genome assembly (Oar_v3.1.86) using BWA-MEM (Galaxy version 0.7.17.1). The resulting BAM files were sorted using Samtools_sort (Galaxy version 1.0.0). Sorted and indexed BAM files were visualized through the Integrative Genome Viewer, IGV software version 2.4.10 (Robinson et al., 2011). A GFF3 annotation file was obtained from Ensembl (Ovis_aries.Oar_V3.1.78). We applied GATK version 3.5-0 to perform SNP and InDel discovery and genotyping across the two samples simultaneously using standard filtering parameters according to GATK Best Practices recommendations (DePristo et al., 2011; Van der Auwera et al., 2013). Variants effect and annotation were realized by SNPEff version 4.1, and filtering of interesting variants was performed using the SNPSift tool.
In vitro Transcription and Translation of BMP15
The full-length cDNA of ovine BMP15 [1480 bp (-297, + 1183) referring to ATG start codon] with or without the FecXN mutation in position -290 was generated from oocyte-derived RNA after a reverse transcription (RT) step (described in RNA extraction and RT paragraph, primers are listed in Supplementary Table S2). The resulting PCR products were inserted by TA cloning into pGEM-T Easy plasmid (Promega) possessing T7 and SP6 promoters. The orientation of insertion and exclusion of unexpected PCR-induced mutations were controlled by Sanger sequencing.
In vitro transcription and translation were realized from 500 ng of cDNA pGEM-T construct using the TnT T7 Quick Coupled Transcription/Translation kit (Promega) and Transcend Biotin-Lysine-tRNA following the manufacturer’s protocol. Reactions for each construct were run in duplicate in six independent TnT experiments. The resulting BMP15 protein was detected using a Transcend non-radioactive translation detection system with chemiluminescent method (Promega) after reducing SDS-PAGE on a gradient (4–15%) polyacrylamide gel (Promega) and transfer onto nitrocellulose membrane. Chemiluminescent signal was capture by a ChemiDoc MP imaging system and images were analyzed with the Image Lab Software (Bio-Rad).
BMP15 Promoter Activity
The promoter sequence of the ovine BMP15 gene was amplified by PCR on genomic DNA from both homozygous FecX+ and homozygous FecXN ewes. Two sizes of fragments were generated for cloning the BMP15 promoter in front of the luciferase (Luc) reporter gene, a long (lg) form of 732 bp [(-743, -11) bp referring to ATG start codon] and a short (sh) form of 341bp [(-443, -102) bp]. The PCR products were engineered for digestion using KpnI and HindIII restriction enzymes (New England Biolabs) and inserted into the pGL4.23 vector (Promega). The four resulting constructs (lgBMP15+-Luc, lgBMP15N-Luc, shBMP15+-Luc, shBMP15N-Luc) were controlled by Sanger sequencing. Primers used to generate these constructs are listed in Supplementary Table S2. Twenty-four hours after seeding (3.104 cells/well, 24 wells plate), CHO (Chinese hamster ovary) cells were transfected using Lipofectamine 3000 (Invitrogen) with 500 ng/well of pGL4.23 constructs either empty or containing BMP15 promoter fragment. Forty-eight hours after transfection, cells were lysed and assayed for luciferase activity (Luciferase reporter assay kit, Promega). Luminescence in relative light units (RLU) was measured by a Glomax microplate reader (Promega). Each construct was assayed in triplicate in six independent transfection experiments.
RNA Extraction, Reverse Transcription, and Quantitative PCR
Total RNA from pools of five oocytes were extracted using the Nucleospin RNA XS kit according to the manufacturer’s protocol (Macherey–Nagel) and including a DNase1 treatment. The low quantity of RNA recovered did not allow quantification. So the equivalent of 1.25 oocyte was reverse-transcribed using SuperScript II reverse transcriptase (Invitrogen) and anchored oligo(dT)22 primer (1 μl at 10 μM). Primer design using Beacon designer 8.20 (Premier Biosoft), SYBR green real-time PCR cycling conditions using a QuantStudio 6 Flex Real-Time PCR system (Thermo Fisher Scientific) and amplification efficiency calculation [E = e(–1/slope)] were as previously described in Talebi et al. (2018a). Primer sequences, amplicon length, and amplification efficiency are listed in Supplementary Table S2. RNA transcript abundance was quantified using the ΔCt method with the mean expression of GAPDH and SDHA as internal references and following the formula R = [ErefCt ref/EtargetCt target]. The two reference genes were validated by the Bestkeeper algorithm (Pfaffl et al., 2004).
Data Analysis
Single-marker association analyses were conducted using a Fisher’s exact test, and a Bonferroni correction has been applied to check for significance levels. The chromosome- and genome-wide values have been established as mentioned by Balding (2006). Statistical analyses were done using PLINK1.9 software under a case/control design (Chang et al., 2015). Among the 79 data sets of 47,446 SNPs analyzed, the LS trait was considered as the case when mean LS ≥ 2.18 (n = 39) and control when LS ≤ 1.45 (n = 40). Haplotypic association analysis on the X chromosome were performed using FastPhase software (Scheet and Stephens, 2006). Empirical significance levels were calculated using maximum statistic permutation approach [max (T), n = 1000].
Allele effect on LS was estimated in NV and BMC breeds on data extracted from the French national database for genetic evaluation and research managed by the Institut de l’Elevage (French Livestock Production Institute) and the CTIG (Centre de Traitement de l’Information Génétique, Jouy-en-Josas, France). Only females born after 2000 were retained (27,754 NV ewes with 122,110 LS records and 110,848 BMC ewes with 461,405 LS records) with their pedigree over five generations. The FecXN genotype effect on the subset of 79 case/control animals was assessed by one-way ANOVA, followed by the Newman–Keuls post hoc test. For the large animal cohort analyses, the linear mixed models used were as similar as possible to those of the national genetic evaluation system (Poivey et al., 1995). In the present study, the following fixed effects were considered: (i) the genotype at the FecX locus; (ii) the month of birth (12 levels); (iii) a physiological status effect combining parity, age at first lambing, rearing mode, and postpartum interval (44 levels); and (iv) a combination of the flock year and season effect. Two random effects were added to the model: a permanent environmental effect and an animal additive genetic effect. Moreover, an additional fixed effect of the reproduction type was considered for the BMC breed for which some hormonal treatments are used each year (87 and 13% for natural and induced estrus, respectively). For the NV breed, because the FecLL allele is also segregating in the population (Chantepie et al., 2018), the effect of the genotype at this locus (2252 known and 25,502 unknown genotypes) as well as its interaction with the genotypes at the FecX locus were considered. All these models were fitted using the ASReml software (Gilmour et al., 2009).
The comparison between FecX alleles for BMP15 protein quantification was analyzed using Student’s t-test, using Welch’s correction for unequal variance. For reporter luciferase assays, differences between constructs were analyzed by one-way ANOVA followed by Newman–Keuls post hoc test. QPCR data for BMP15 and GDF9 expression in oocytes were analyzed by two-way ANOVA considering genotype and breed effects. P < 0.05 was the threshold for statistical significance. All these experimental data are presented as means ± SEM and were analyzed using Prism 6 (GraphPad Software Inc.).
Results
Genetic Association Analyses
A first set of genomic DNA from 30 NV ewes without the FecLL prolific allele at the B4GALNT2 locus (LS records ranging from 2.00 to 3.00) was genotyped for already known mutations affecting sheep prolificacy at the three other loci, BMPR1B, GDF9, and BMP15. Using a specific RFLP assay (BMPR1B, Wilson et al., 2001) or Sanger sequencing of coding parts (GDF9 and BMP15, Talebi et al., 2018b), none of the known mutations was evidenced (data not shown). Thus, to establish the genetic determinism of the remaining LS variation in this population, 80 ewes were genotyped by an Illumina Ovine SNP50 Genotyping Beadchip. The allele frequencies of the most highly prolific ewes (cases, n = 40, mean LS = 2.47) and lowly prolific ewes (controls, n = 40, mean LS = 1.23) were compared to identify loci associated with LS using GWAS according to the procedures described in section “Materials and Methods.” Finally, genotype data were obtained from 79 animals (39 cases, 40 controls). Six markers located on OARX were significantly associated with LS variation at the genome-wide level after Bonferroni correction (Figure 1A and Table 1). Importantly, at the chromosome-wide level, a cluster of 26 significant markers encompassed the location of the BMP15 candidate gene (Figure 1B). In order to better characterize this locus on the X chromosome, we have determined, for each individual, the most likely linkage phase across 80 markers (10 Mb) including the significant region. After haplotype clusterization, a specific segment of 3.5 Mb (50,639,087–54,114,793 bp, OARv3.1 genome assembly) was identified to be more frequent in highly prolific cases than in controls (fcases = 0.51 vs. fcontrols = 0.037, P = 1.92E–11, Chi-square test) (Figure 2). This identified segment contained the BMP15 gene (50,970,938–50,977,454 bp, OARv3.1) well known to play a crucial role in ovarian function and to be a target of numerous mutations in its coding region controlling prolificacy (Persani et al., 2014).
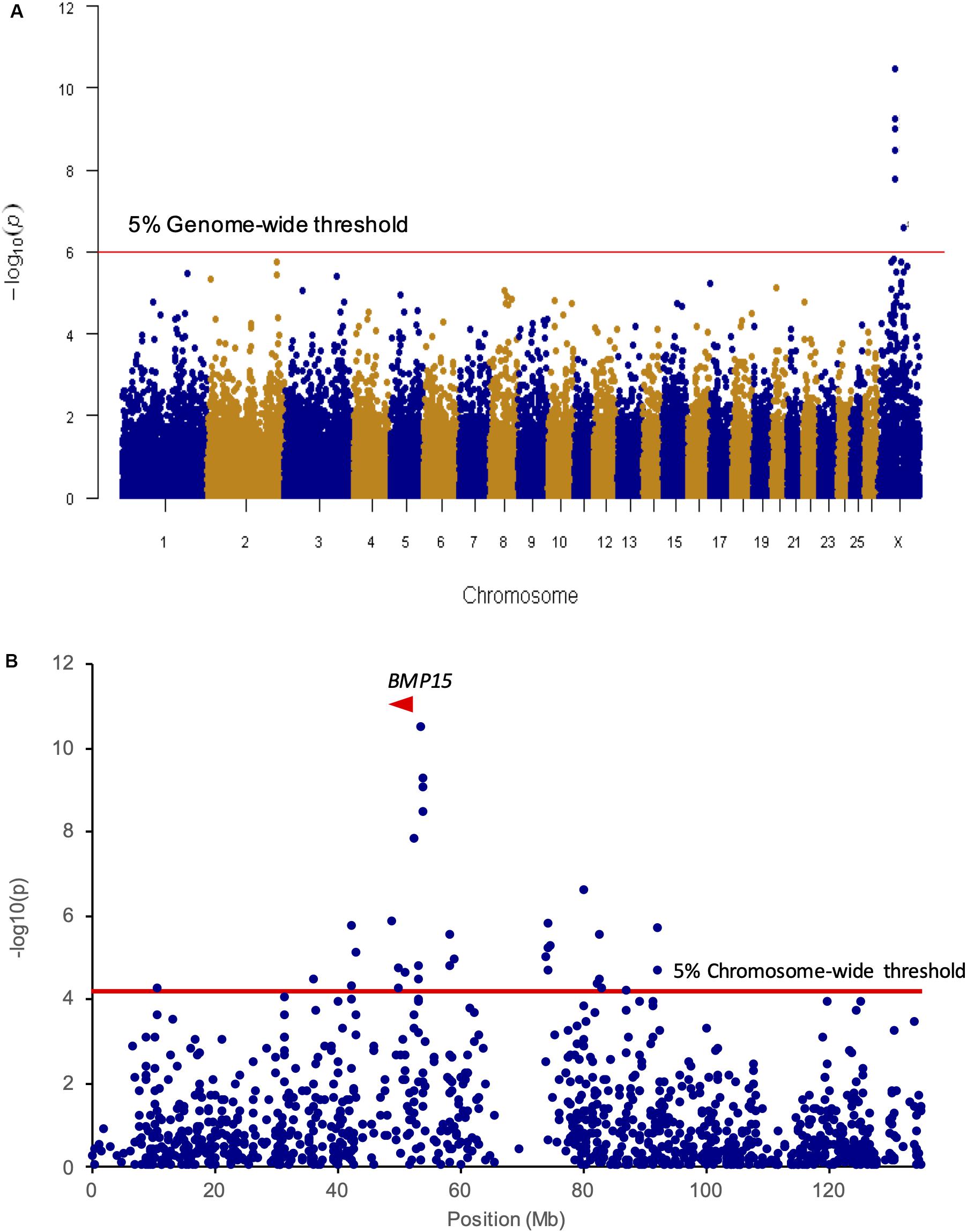
Figure 1. Genome- and chromosome-wide association results. (A) Genome-wide association results for litter size in the NV sheep population. Manhattan plot shows the combined association signals [-log10(p-value)] on the y-axis vs. SNPs position in the sheep genome on the x-axis and ordered by chromosome number (assembly OARv3.1). Red line represents the 5% genome-wide threshold. (B) OARX chromosome-wide association results. The curve shows the combined association signals [-log10(p-value)] on the y-axis vs. SNPs position on the X chromosome on the x-axis (assembly OARv3.1). Red line represents the 5% chromosome-wide threshold. The BMP15 gene location is indicated by a red arrowhead.
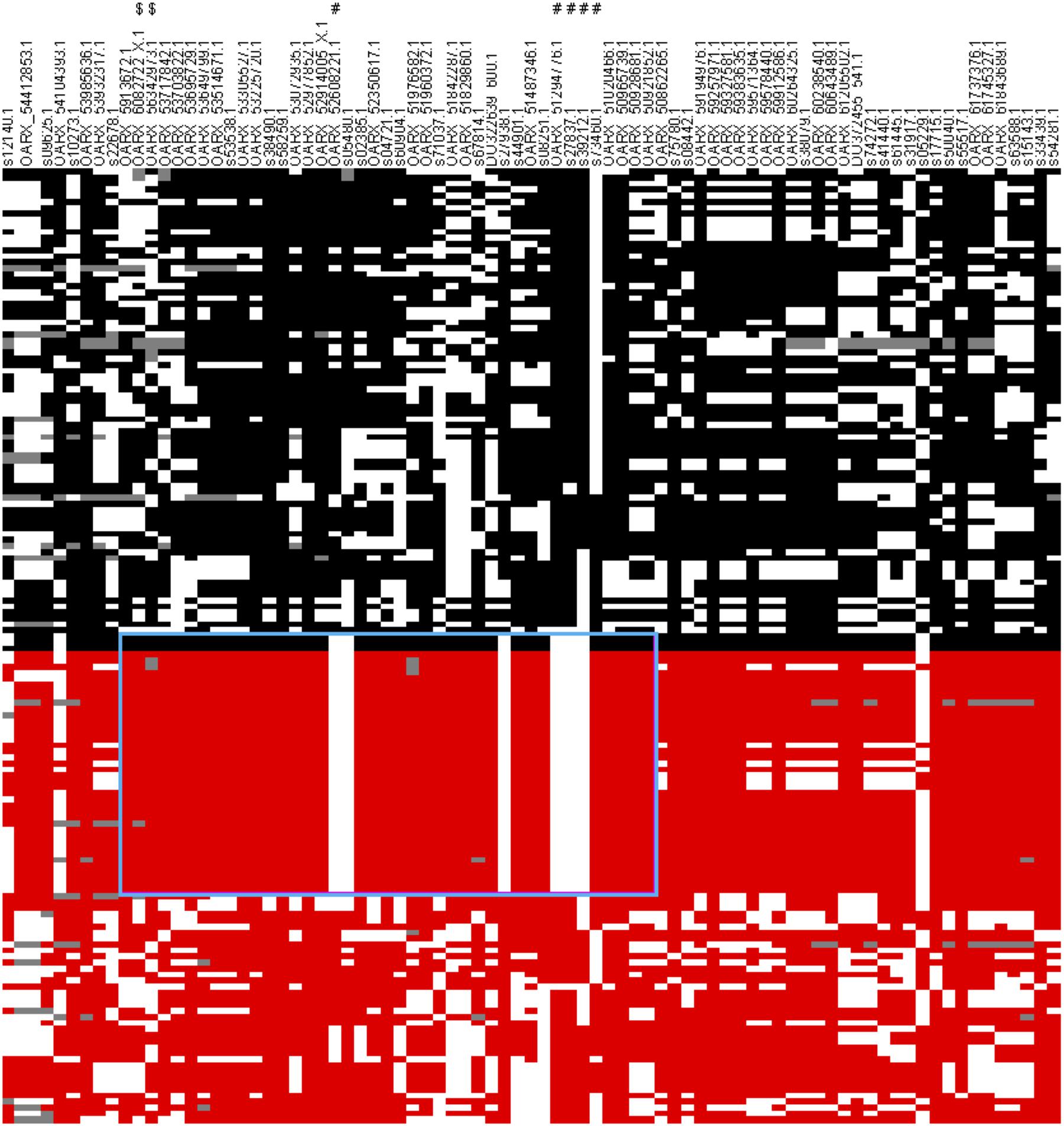
Figure 2. Clusterization of haplotypes reconstructed at the OARX locus. Eighty markers encompassing the OARX region of interest (50.6–54.1 Mb) were selected to construct haplotypes from 39 cases and 40 control animals. Each column represents one SNP, and each line represents one haplotype. For one marker (i) allele 1 is in black in controls or in red in cases, (ii) allele 2 is in white when the phase was unambiguous, and (iii) gray color represents unphased SNP. Haplotypes were ordered to distinguish controls vs. cases and clusterized to classify similar clades of haplotypes. The # sign flags SNP significantly associated with LS at genome-wide level. The $ sign flags SNP flanking the BMP15 genes (50,970,938–50,977,454 bp). The specific haplotype preferentially selected in highly prolific ewes (cases) is symbolized by the cyan rectangle.
Characterization of the Mutation
Although the BMP15 gene could be considered as a positional and functional candidate gene, no mutation was evidenced by Sanger sequencing of the BMP15 coding regions of the most prolific ewes studied. In order to find the potential causal mutation, we sequenced the whole genome of two specifically chosen ewes based on the shortest haplotype within the region (homozygous reference vs. homozygous variant) and their opposite extreme phenotypes (LS 1.1 vs. 2.8).
Variant search analysis and annotations through the GATK toolkit was limited to the OARX: 50,639,087–54,114,793 bp region. We detected 60 SNPs and 90 small insertions and deletions (INDELs) with quality score > 30 (Supplementary Table S1). Among them, we particularly focused on the 85 variants located within annotated genes (upstream, exon, intron, splice acceptor or donor, and downstream localization). After filtering these 85 variants for allele sharing with other breeds based on SheepGenome DB5 and 68 publicly available domestic sheep genomes (International Sheep Genomics Consortium)6, none of them were removed, all being NV breed-specific. Finally, and based on prolificacy gene knowledge, we were particularly interested in one SNP (T > A) identified in the upstream region of the BMP15 gene at position 50,977,717 bp on OARX v3.1. We then developed an RFLP assay to specifically genotype for this polymorphism. Among the 79 animals of the GWAS, 31 ewes were heterozygous and six homozygous for the A variant allele. As shown in Table 2, most of the A carrier ewes were in the highly prolific Case group (34 among 39), and only three set in the Control group. When associating the LS performance of the 79 ewes to their genotype at the OARX: 50977717T > A SNP, the A non-carriers exhibited a mean LS of 1.36, heterozygous T/A a mean LS of 2.32, and homozygous A/A a mean LS of 2.73, indicating that the A allele of this polymorphism was strongly associated with increased LS in NV (T/A or A/A vs. T/T, P < 1E–3, one-way ANOVA). Furthermore, this polymorphism appears to be in total linkage disequilibrium with the six more significant markers from the GWAS analysis (Figure 3). Genotype information at the OARX: 50977717T > A locus was introduced in the GWAS analysis. This SNP appeared as the most significant marker associated with the prolificacy phenotype (Punadjusted = 1.93E–11, PChromosome–wide corrected = 1.62E–14 and PGenome–wide corrected = 9.13E–07), suggesting that it could be the causal mutation (Supplementary Figure S1). In accordance with the Fec gene nomenclature, the mutant allele identified upstream of the BMP15 gene in NV sheep was named FecXN.
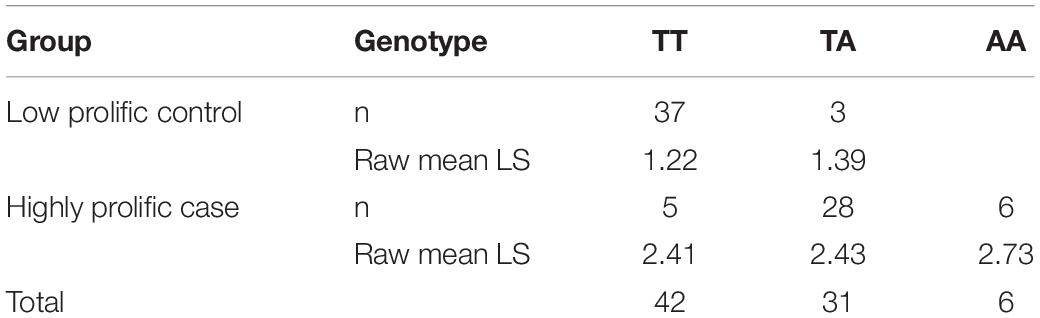
Table 2. Distribution of OARX:50977717T > A SNP genotypes and associated LS in case and control groups.
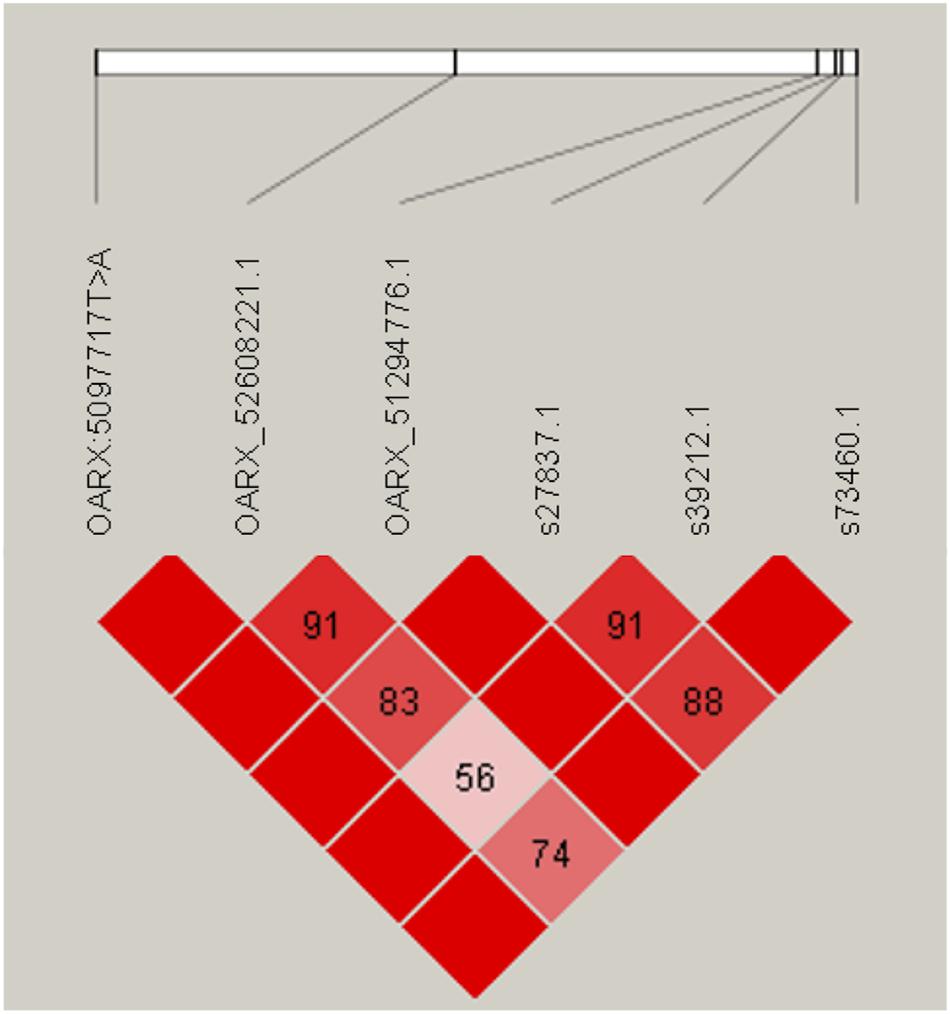
Figure 3. Linkage disequilibrium (LD) plot. Generated by Haploview, pairwise LD between SNP markers (OARX: 50977717T > A and the five genome-wide significant LS-associated markers) is represented by the D’ value. Strong LD is represented in dark red boxes (D’ = 100) and weaker LD (D’ < 100) in lighter red boxes.
As described for other prolific alleles, such as FecBB, FecXG, FecGH, FecXGr, and FecLL, a given mutation can segregate in several sheep populations (Davis et al., 2002; Mullen et al., 2013; Chantepie et al., 2018; Ben Jemaa et al., 2019). We have tested the FecXN allele presence in a diversity of 26 sheep breeds representing 725 animals (Rochus et al., 2018). Among the breeds tested, the FecXN genotyping has confirmed the segregation of this mutation in NV breed and revealed its presence in the Blanche du Massif Central (BMC) and Lacaune breeds (Table 3). Additionally, the FecXN variant was absent from the Ensembl variant database7 compiling information from (i) dbSNP, (ii) whole-genome sequencing information from the NextGen project (180 animals from various Iranian and Moroccan breeds), and (iii) the International Sheep Genome Consortium (551 animals from 39 breeds all over the world).
FecXN Genotype Frequency and Effect on Prolificacy
Large cohorts of ewes, chosen at random, were genotyped in order to accurately estimate the allele frequencies in the NV and the BMC populations (Table 4). The frequency of the N prolific allele at the FecX locus was similar in both populations, 0.11 and 0.10, with a distribution of 19.4 and 17.6% heterozygous, 1.5 and 1% homozygous carriers in NV and BMC, respectively. The genotype frequencies were consistent with the Hardy–Weinberg equilibrium (HWE) in both breeds (NV P = 0.28 and BMC P = 0.76).
Based on the raw mean LS observations, the FecXN carrier ewes clearly exhibited increased LS compared to non-carriers in both populations (Table 4). The L prolific allele at the FecL locus is also segregating in NV (Chantepie et al., 2018). Results of the linear mixed model showed that, for the NV breed, one copy of the FecXN allele significantly increased LS by + 0.22, and two copies increased LS by +0.65, and a single copy of the FecLL allele increased LS by + 0.41, and two copies by + 0.72. Based on the 80 ewes genotyped heterozygous at both loci, it appeared that the effects of FecXN and FecLL on LS were not fully additive, the expected LS being significantly slightly reduced by -0.05 (0.58 instead of 0.63) due to a significant interaction between the two loci (P < 0.001) (Figure 4A). For the BMC population, compared to FecX+/FecX+ ewes, FecXN/FecX+ exhibited increased LS by + 0.18 and FecXN/FecXN by + 0.30 under natural estrus (Figure 4B). The use of PMSG for estrus synchronization increased LS significantly among FecX+/FecX+ ewes (+ 0.23) and FecXN/FecX+ ewes (+ 0.18) while the effect on FecXN/FecXN ewes was negative (-0.13). The combined effect of the first copy of the FecXN allele and the use of PMSG treatment was not fully additive, the interaction being significant (P < 0.001) although low (0.35 instead of 0.41) (Figure 4B).
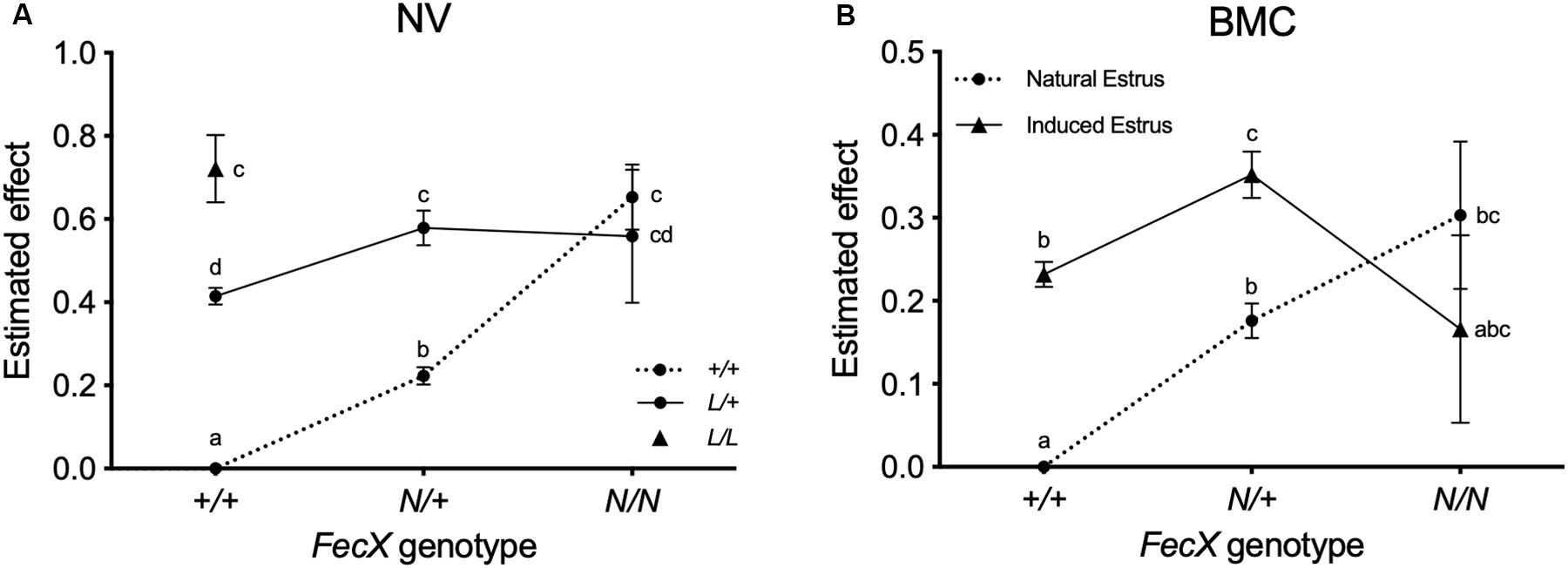
Figure 4. Estimated effect of FecXN. (A) Estimated effect of FecXN knowing FecLL genotype in Noire du Velay (NV). (B) Estimated effect of FecXN according to induced or natural estrus in Blanche du Massif Central (BMC). Estimated increased LS and the corresponding standard errors from the linear mixed models with interaction between genotypes at the FecX and FecL loci for NV, and between FecX genotype and estrus type for BMC are given relative to L and N alleles non-carrier ewes (+/+). Different letters indicate significant differences (P < 0.01).
Functional Effects of the FecXN Mutation
As described above, FecXN is located upstream of the coding region of the BMP15 gene when referencing to the ovine genome v3.1 (ensembl.org) or v4.0 (ncbi.nlm.nih.gov). In both versions of the ovine genome, the BMP15 gene annotation begins at the ATG start site and FecXN is located 290 pb upstream, possibly in the 5′UTR and/or the proximal promoter region. As a first approach, we took advantage of RNA sequencing data from ovine oocytes publicly available at EMBL-EBI (Bonnet et al., 2013). After reads mapping against the ovine genome (v3.1) using STAR2 aligner within the Galaxy pipeline and visualization with Integrative Genome Viewer (IGV), Figure 5 shows the location of FecXN within the possible 5′UTR of the BMP15 gene when expressed in the oocyte. Consequently, we have first tested the potential functional impact of FecXN on the in vitro stability and translatability of the BMP15 mRNA. Thus, the reference (T, FecX+) and variant (A, FecXN) forms of the ovine BMP15 cDNA (-297, +1183 referring to ATG start codon) were cloned in a pGEM-T vector for subsequent in vitro T7 promoter-dependent transcription/translation experiment using reticulocyte lysate solution. As shown in Figure 6, the Western blotting of the BMP15 proteins produced from both forms, and their chemiluminescent quantification revealed that the FecXN mutation had no significant impact on the overall stability and translatability of the BMP15 mRNA in this condition.
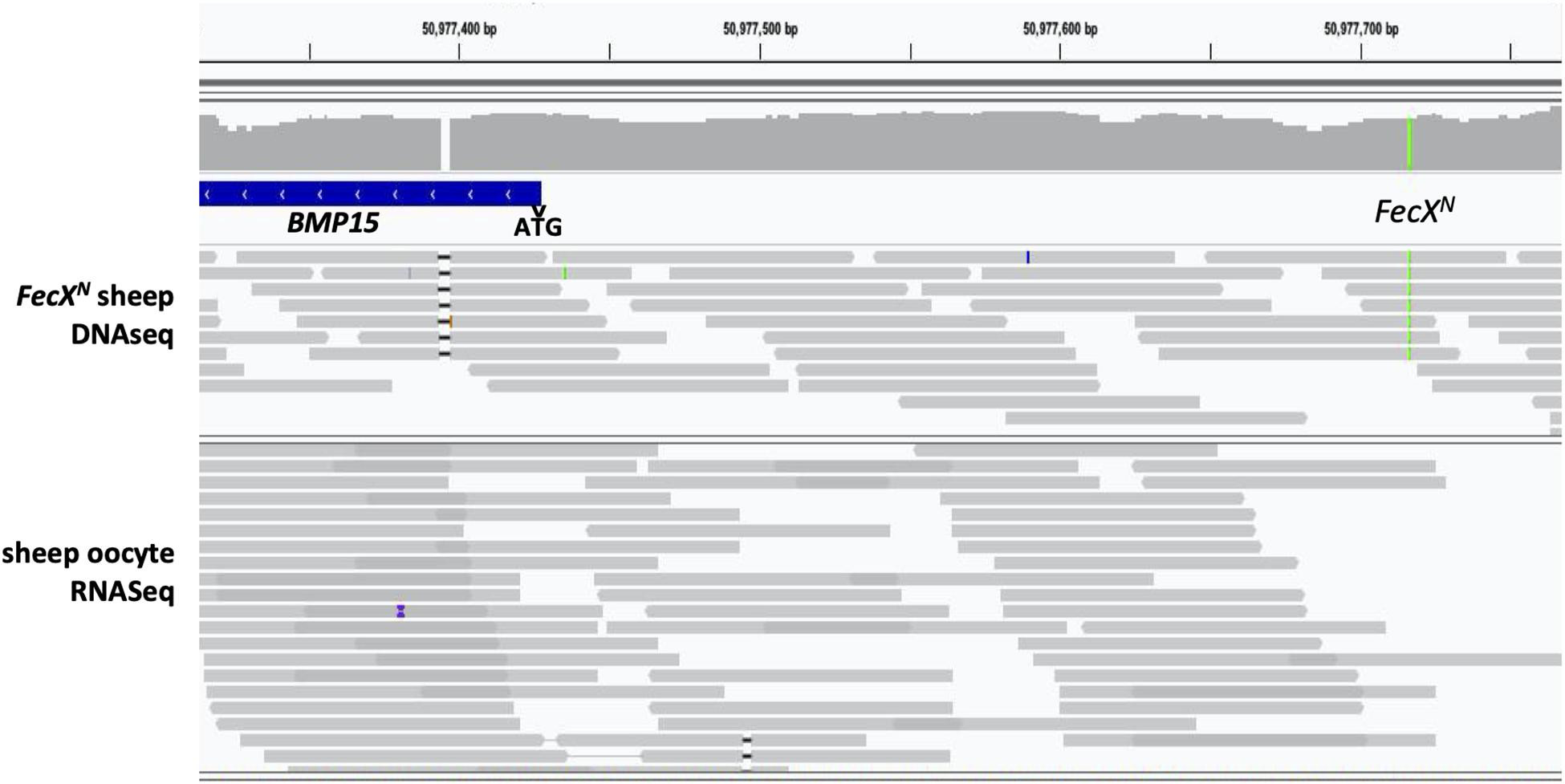
Figure 5. Localization of FecXN in the BMP15 upstream region. Integrative Genomics Viewer (IGV v2.4.10) snapshot of the ovine BMP15 gene upstream region alignments (coordinates from OAR v3.1 assembly) with reads from whole genome DNA sequencing (DNASeq) of a homozygous FecXN carrier ewe (green line) and total mRNA sequencing of ovine oocytes from small antral follicles (RNASeq, Bonnet et al., 2013), indicating a possible localization of FecXN in the 5′UTR region of BMP15 (ATG start codon is indicated by an arrowhead and BMP15 gene annotation is on the minus strand).
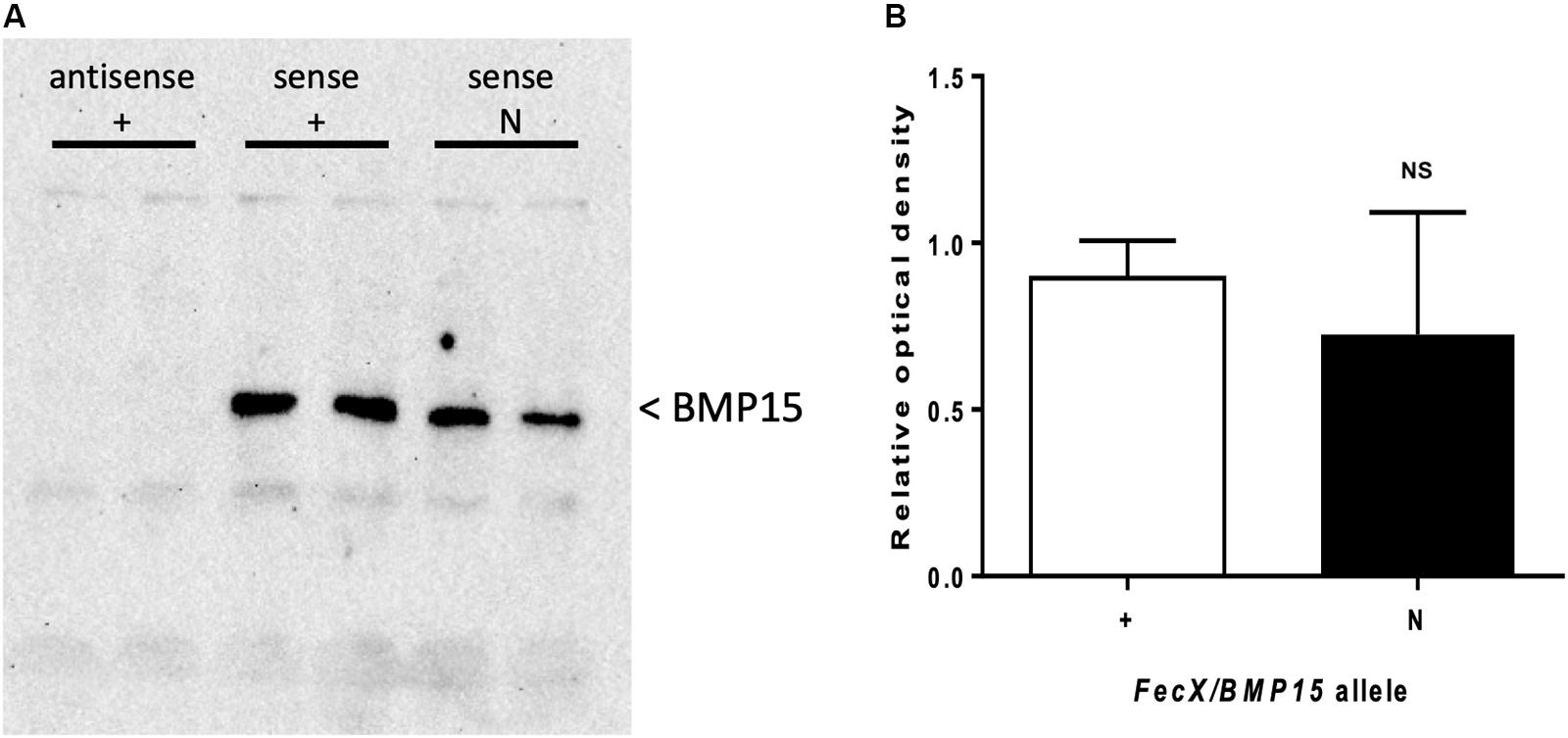
Figure 6. Effect of FecXN mutation on the ovine BMP15 protein produced in vitro. In vitro transcription and translation were realized in duplicate in six independent experiments from antisense BMP15 cDNA carrying the wild-type allele (antisense +) as negative control or sense BMP15 cDNA with (sense N) or without FecXN (sense +). (A) A representative western blot experiment revealing the BMP15 protein. (B) Chemiluminescent signal of BMP15 was captured by a ChemiDoc MP imaging system and images were quantified (relative to sense +) and analyzed with the Image Lab Software (Bio-Rad). Means (± SEM) were not significantly different (NS) after Student’s T-test with Welch’s correction.
As a second hypothesis, we have tested the FecXN impact on the BMP15 promoter activity. Two promoter regions were tested [(-743, -11) bp and (-443, -102) bp referring to ATG start codon] cloned in front of the luciferase reporter gene and transiently expressed in CHO cells cultured in vitro. As shown by the luciferase assays (Figure 7), the FecXN variant was able to significantly reduce the luciferase activity in the context of both the long or the short BMP15 promoters, indicating the possible inhibitory impact of FecXN on BMP15 gene expression. To go further with this hypothesis, in vivo BMP15 gene expression was measured directly on isolated oocytes pools from NV and BMC homozygous ewe carriers and non-carriers of the FecXN allele. Even if the mean expression of BMP15 checked by real-time qPCR was decreased by twofold in the oocytes of FecXN carriers, this difference appeared as not significant (P = 0.166, genotype effect, two-way ANOVA) due to a large variability between animals. In parallel, the mean expression of the second oocyte-specific prolificacy major gene GDF9 seemed unaffected by the presence of FecXN (Figure 8).
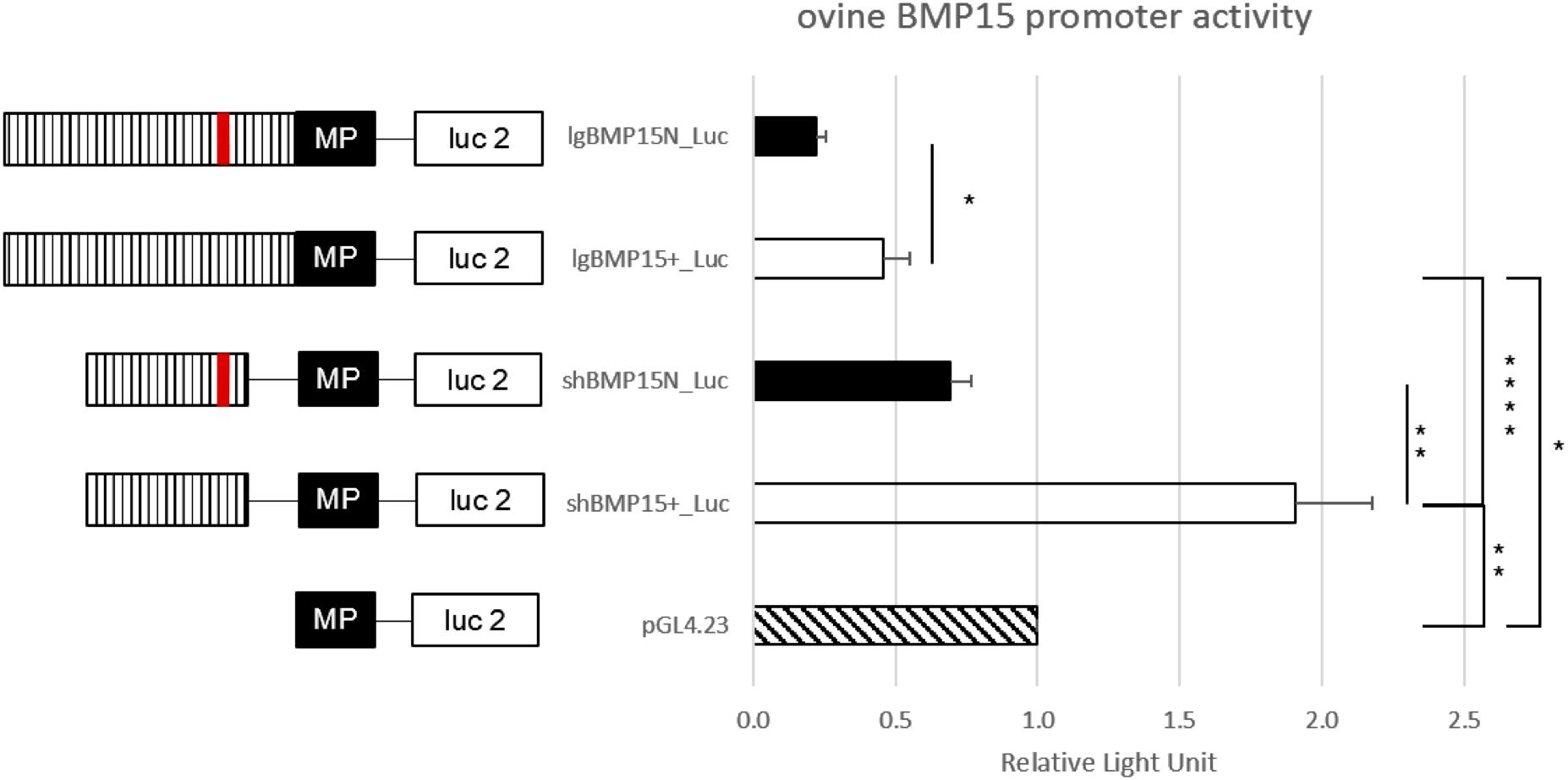
Figure 7. Functional effect of FecXN mutation on the BMP15 promoter activity. In vitro reporter luciferase assay from CHO cells transiently transfected with empty vector or wild-type BMP15 promoter (BMP15+) or mutant BMP15 promoter (BMP15N). Two fragments were generated, a long (lg) form of 732 bp (-743, -11 bp referring to ATG start codon) and a short (sh) form of 341 bp (-443, -102 bp). Results are expressed as means ± SEM of the relative light unit (RLU) from six independent transfection experiments in triplicate. Asterisk indicates significant difference after one-way ANOVA *P < 0.05; **P < 0.01; ****P < 0.0001. MP, minimal promotor of pGL4.23; Luc 2, luciferase reporter gene; hatched bar, BMP15 promotor; red line, FecXN mutation.
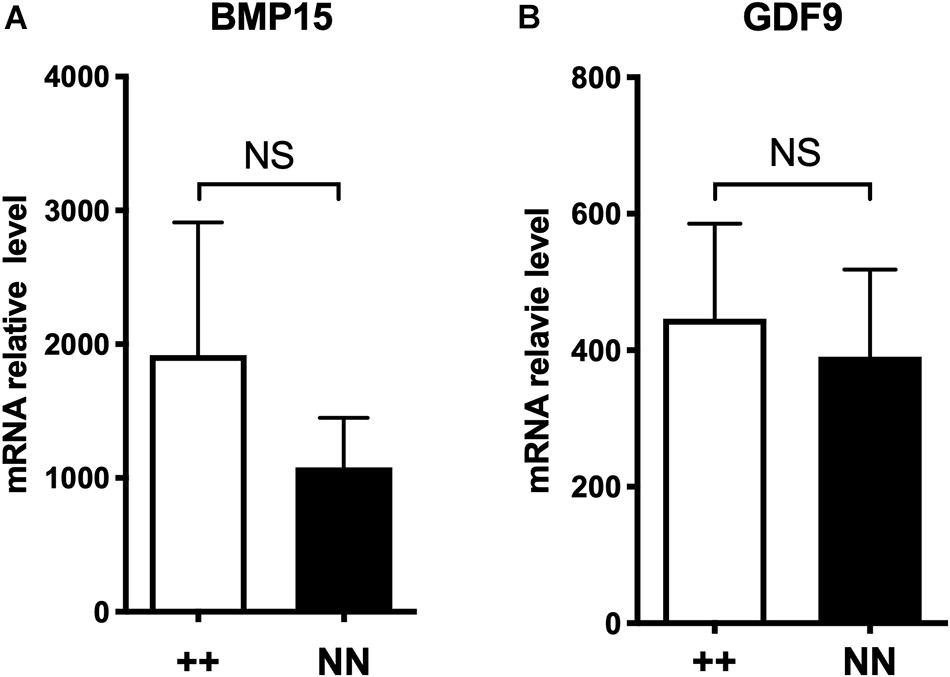
Figure 8. Effect of FecXN mutation on BMP15 and GDF9 expression in ovine oocyte. Quantitative real-time PCR results of BMP15 (A) and GDF9 (B) expression in oocyte pools from growing (1–3 mm) follicles (two pools of five oocytes per animal) from homozygous FecXN carrier (N/N, n = 5) and non-carrier ewes (+/+, n = 5) during the follicular phase of the estrus cycle. Results are expressed as means ± SEM of the mRNA relative level for each genotype, using GAPDH and SDHA as internal references. Raw data were analyzed by two-way ANOVA. Means (± SEM) were not significantly different (NS).
Discussion
The present study identified the g.50977717T > A variant on the ovine chromosome X upstream of the BMP15 gene as the most likely causative mutation for the increased prolificacy of the NV ewes. The highly significant genetic association with the extreme LS phenotype, the significant effect of the A variant on increasing prolificacy by + 0.2 lamb per lambing in a large set of NV ewes, also found in the BMC genetic background, and the demonstrated action on BMP15 transcriptional activity all support the causality of this mutation named FecXN.
The BMP15 gene is at the top of the list of candidate genes controlling ovarian function, ovulation rate, and thus prolificacy in the ovine species with 10 independent mutations identified out of the 17 already known. Indeed, eight SNPs and two small INDELs, all within the open reading frame, were evidenced affecting prolificacy and BMP15 function. Among these mutations, two SNPs and the two INDELS impaired the protein production either by generating premature stop codon (FecXH, Galloway et al., 2000; FecXG, Hanrahan et al., 2004) or by breaking the reading frame (FecXR, Martinez-Royo et al., 2008, FecXBar, Lassoued et al., 2017). The six other SNPs generate non-conservative amino acid substitutions, one being not functionally tested (L252P, Amini et al., 2018), and all others leading to a loss of function of BMP15 ranging from inhibited protein production (FecXL, Bodin et al., 2007) and impaired interaction with GDF9 (FecXI and FecXB, Liao et al., 2004) to altered cell signaling activity (FecXGr and FecXO, Demars et al., 2013). In contrast with the 10 mutations described above, the FecXN variant evidenced in the present study is not located in the open reading frame of BMP15 and does not alter the protein sequence. However, no other polymorphism genetically linked to FecXN was found in the BMP15 coding sequence when checked by whole genome or local Sanger sequencing of the BMP15 gene from FecXN carrier animals. Of course, this does not rule out the possibility of a polymorphism lying in another gene nearby with a still unknown role in the ovarian function and prolificacy. Nevertheless, we did not find any polymorphism (SNP and INDEL) altering the coding sequence of genes annotated in the significantly LS-associated genetic region of 3.5 Mb on OARX (Supplementary Table S1), leaving BMP15 as the most obvious candidate.
Whatever the version of the ovine reference genome (Oar_v3.1, Oar_v4.0, or even the last Oar_rambouillet_v1.0), the annotation of the BMP15 gene always starts at the ATG-initiating codon. Using publicly available transcriptome data from ovine oocytes RNAseq analysis, we were able to show that FecXN located 290 bp upstream of BMP15 could stand in its 5′UTR region. From our in vitro functional analyses, FecXN was not demonstrated to influence the translatability of the BMP15 mRNA, but on the contrary, it was shown to decrease the BMP15 promoter activity. Little is known about transcription factors able to regulate BMP15 expression. Several regulatory elements were evidenced in pig BMP15 promoter hosting consensus-binding sites for LHX8, NOBOX, and PITX1 transcription factors. However, only LHX8 was demonstrated as functionally activating the porcine BMP15 promoter activity (Wan et al., 2015). In human, a regulatory mutation in the 5′UTR of BMP15 (c.-9C > G) was associated to non-syndromic premature ovarian failure (Dixit et al., 2006), and also iatrogenic ovarian hyperstimulation syndrome (Morón et al., 2006). This mutation was shown to enhance the fixation of the PITX1 factor transactivating the BMP15 promoter (Fonseca et al., 2014). However, the FecXN position does not fit with the syntenic location of porcine LHX8 and human PITX1 binding sites on the ovine BMP15 promoter. Using the MatInspector promoter analysis tool (Genomatix), we were only able to hypothesize an alteration by FecXN of a putative TATA-box-like sequence (TTAAATA > TTATATA). Unfortunately, our electromobility shift assay attempts using CHO nuclear extracts failed to demonstrate the binding of any factor at the FecXN position, preventing us from defining the precise molecular mechanism by which FecXN decreases BMP15 promoter activity.
The inhibition of the promoter activity suggests a transcriptional regulatory role of FecXN on BMP15 gene expression. That is the reason why BMP15 mRNA accumulation in homozygous FecXN/FecXN oocytes was checked and compared to non-carrier oocytes. The twofold decrease of BMP15 mean expression in oocytes of FecXN carriers was not statistically significant. However, the moment we have chosen during the follicular phase of the late folliculogenesis for the comparative analysis between FecX+ and FecXN oocytes from 1 to 3 mm antral follicles without controlling the atretic status might not be optimal to visualize a highly significant differential expression of BMP15. To this could be added an induced effect on oocyte by our experimental procedures, progestagen treatment for estrus cycle synchronization and/or oocyte denudation (Rose et al., 2013; Menchaca et al., 2018). The BMP15 gene expression in ovine oocytes begins during the primary stage of follicular development, and its expression increases up to the antral stages where it stays stable (McNatty et al., 2005; Bonnet et al., 2011; Kona et al., 2016; Bonnet and Fabre, unpublished data). Moreover, the streak ovaries phenotype of infertile ewes carrying homozygous mutations in BMP15 have evidenced its crucial role in controlling the primary to secondary follicle transition (Galloway et al., 2000; Bodin et al., 2007; Lassoued et al., 2017). Consequently, it would certainly be appropriate to follow the BMP15 expression in FecXN carrier ewes from these early stages of folliculogenesis to better decipher the mutation impact on ovarian physiology. Nevertheless, the fact that FecXN could inhibit the BMP15 gene expression fits well with the physiological and molecular models associating BMP system loss of function and increased sheep prolificacy (Fabre et al., 2006; Demars et al., 2013).
One copy of the FecXN allele significantly increased by +0.30 to +0.50 the raw mean LS of NV ewes. When corrected for different environmental effects and more particularly for the genotype at the FecL locus, the estimated effect of FecXN on LS was +0.22 lamb per lambing for the first copy and + 0.43 for the second copy. The FecXN effect is in the range of already known prolific alleles in various sheep breeds (Jansson, 2014) and explains about 20% of the genetic variance of prolificacy without considering information at the FecL locus and up to 50% when polygenic effect is corrected for the presence of FecLL, confirming its status of major gene. The effect of FecXN on LS seems relatively independent of the genetic background. Indeed, the estimated positive effect of the first copy of FecXN on prolificacy in NV was confirmed in the BMC breed with + 0.18 lamb per lambing based on natural estrus. Moreover, the same robust effect was observed even in the presence of PMSG for synchronizing the estrus cycles preceding the lambing. The same observation is made for other mutations controlling sheep prolificacy. For instance, the FecLL allele exhibited a similar effect on LS in NV (+0.41, present study; +0.42, Chantepie et al., 2018) Lacaune, +0.47 (Martin et al., 2014), and D’man (+0.30, Ben Jemaa et al., 2019), and this was also observed for the FecBB allele introgressed in several populations (Kumar et al., 2008).
By genotyping a diversity panel, we also evidenced the presence of 2 FecXN carrier animals in the Lacaune meat strain, which will require further genotyping of numerous animals. If this is confirmed, the Lacaune meat breed will be another population, as Belclare, in which three different natural prolific mutations are segregating (Hanrahan et al., 2004; Bodin et al., 2007; Drouilhet et al., 2013). The presence of FecLL in both NV and Lacaune and the presence of FecXN in NV, BMC, and Lacaune also raise the question of the origin of these mutations. From population structure analysis, it was shown that NV, BMC, and Lacaune shared the same origin within the European southern sheep populations that may explain the segregation of the same mutations in these populations (Rochus et al., 2018).
Conclusion
In conclusion, through a case/control GWAS strategy and genome sequencing, we have identified in the NV breed a second prolific mutation named FecXN affecting the expression of the BMP15 gene, a well-known candidate gene controlling OR and LS in sheep. This work confirms the relevance of the whole genome approaches to decipher the genetic determinism of the prolificacy trait. Homozygous FecXN/FecXN animals were still hyperprolific as previously observed for FecXGr and FecXO but in contrast with sterile animals observed for the seven other FecX homozygous variants in BMP15. As an upstream regulatory mutation, FecXN also contrasts with these nine other prolific causal mutations all evidenced in the coding part of BMP15 and altering the protein function. Thanks to this new sheep model, the genetic etiology of ovarian pathologies in women could be improved by searching polymorphisms, not only in the coding region, but also in the regulatory parts driving the BMP15 expression within the oocyte.
Data Availability Statement
The datasets generated for this study can be found in the European Nucleotide Archive (ENA) at EMBL-EBI (accession number PRJEB35553).
Ethics Statement
All experimental procedures were approved (approval number 01171.02) by the French Ministry of Teaching and Scientific Research and local ethical committee C2EA-115 (Science and Animal Health) in accordance with the European Union Directive 2010/63/EU on the protection of animals used for scientific purposes. Written informed consent for participation was not obtained from the owners because breeders in selection schemes are affiliated to selection organisms and give their tacit agreement for animal participation to these organisms collaborating with research institutes.
Author Contributions
LC conducted the investigation, validation, formal analysis and visualization of the data, and wrote the original draft. LB participated in the conceptualization, formal analysis, data curation, and final writing, review, and editing of the manuscript. JS, FW, FP-P, JR, and LD participated in the investigation and getting the biological resources. LD helped in writing, review, and editing of the final manuscript. SF was implicated in the conceptualization, supervision, funding acquisition, investigation, formal analysis and writing, review, and editing of the final manuscript. All authors contributed to the article and approved the submitted version.
Funding
LC was supported by a Ph.D. grant co-funded by the APIS-GENE through the Proligen project and the European Funds for Regional Development (FEDER) through the Interreg POCTEFA programme in the framework of the PIRINNOVI project (EFA103/15). Part of the NV sampling was supported by the DEGERAM project co-funded by the FEDER Massif Central, the Règions: Aquitaine, Midi- Pyrènèes, Limousin and Auvergne, and the French government.
Conflict of Interest
The authors declare that the research was conducted in the absence of any commercial or financial relationships that could be construed as a potential conflict of interest.
Acknowledgments
This manuscript has been released as a preprint at BioRxiv (Chantepie et al., 2019). We thank Claire Chantaduc, Didier Cathalan, and Kévin Chile from ROM Seilection managing the NV and BMC populations for their precious help in the planning of blood sampling. We grateful to the breeders who made their animals available for this study.
Supplementary Material
The Supplementary Material for this article can be found online at: https://www.frontiersin.org/articles/10.3389/fgene.2020.00585/full#supplementary-material
FIGURE S1 | Genome-wide and chromosome-wide association results integrating the SNP OARX: 50977717T > A. (A) The SNP OARX: 50977717T > A genotypes were included in the SNP50 Beadchip data for genome-wide association analysis for litter size in the NV sheep population. Manhattan plot shows the combined association signals [-log10(p-value)] on the y-axis vs. SNPs position in the sheep genome on the x-axis and ordered by chromosome number (assembly OARv3.1). Red line represents the 5% genome-wide threshold. (B) OARX chromosome-wide association results. The curve shows the combined association signals [-log10(p-value)] on the y-axis vs. SNPs position on the X chromosome on the x-axis (assembly OARv3.1). Red line represents the 5% chromosome-wide threshold. In both panels, the position of the SNP OARX:50977717T > A is indicated by a red dot. In (B), the BMP15 gene location is indicated by a red arrowhead.
TABLE S1 | List of variants found in the OARX: 50639087–54114793 region. Listing of 60 SNPs and 90 small INDELs with quality score > 30.
TABLE S2 | List of primers used in the study. Locations of primers are based on the OARv3.1 ovine genome assembly available on ensembl.org.
Footnotes
- ^ www.labogena.fr
- ^ get.genotoul.fr
- ^ https://galaxyproject.org
- ^ http://sigenae-workbench.toulouse.inra.fr
- ^ http://sheepgenomesdb.org/
- ^ http://www.sheephapmap.org/
- ^ http://www.ensembl.org
References
Abdoli, R., Zamani, P., Mirhoseini, S. Z., Ghavi Hossein-Zadeh, N., and Nadri, S. (2016). A review on prolificacy genes in sheep. Reprod. Domest. Anim. 51, 631–637. doi: 10.1111/rda.12733
Amini, H.-R., Ajaki, A., Farahi, M., Heidari, M., Pirali, A., Forouzanfar, M., et al. (2018). The novel T755C mutation in BMP15 is associated with the litter size of Iranian Afshari. Ghezel, and Shal breeds. Arch. Anim. Breed. 61, 153–160. doi: 10.5194/aab-61-153-2018
Balding, D. J. (2006). A tutorial on statistical methods for population association studies. Nat. Rev. Genet. 7, 781–791. doi: 10.1038/nrg1916
Ben Jemaa, S., Ruesche, J., Sarry, J., Woloszyn, F., Lassoued, N., and Fabre, S. (2019). The high prolificacy of D’man sheep is associated with the segregation of the FecLL mutation in the B4GALNT2 gene. Reprod. Domest. Anim. 54, 531–537. doi: 10.1111/rda.13391
Bodin, L., Di Pasquale, E., Fabre, S., Bontoux, M., Monget, P., Persani, L., et al. (2007). A novel mutation in the bone morphogenetic protein 15 gene causing defective protein secretion is associated with both increased ovulation rate and sterility in lacaune sheep. Endocrinology 148, 393–400. doi: 10.1210/en.2006-0764
Bonnet, A., Bevilacqua, C., Benne, F., Bodin, L., Cotinot, C., Liaubet, L., et al. (2011). Transcriptome profiling of sheep granulosa cells and oocytes during early follicular development obtained by Laser Capture Microdissection. BMC Genomics 12:417. doi: 10.1186/1471-2164-12-417
Bonnet, A., Cabau, C., Bouchez, O., Sarry, J., Marsaud, N., Foissac, S., et al. (2013). An overview of gene expression dynamics during early ovarian folliculogenesis: specificity of follicular compartments and bi-directional dialog. BMC Genomics 14:904. doi: 10.1186/1471-2164-14-904
Chang, C. C., Chow, C. C., Tellier, L. C., Vattikuti, S., Purcell, S. M., and Lee, J. J. (2015). Second-generation PLINK: rising to the challenge of larger and richer datasets. Gigascience 4:7. doi: 10.1186/s13742-015-0047-8
Chantepie, L., Bodin, L., Sarry, J., Woloszyn, F., Plisson-Petit, F., Ruesche, J., et al. (2018). Presence of causative mutations affecting prolificacy in the noire du velay and mouton vendeen sheep breeds. Livestock Sci. 216, 44–50. doi: 10.1016/j.livsci.2018.07.007
Chantepie, L., Bodin, L., Sarry, J., Woloszyn, F., Plisson-Petit, F., Ruesche, J., et al. (2019). Genome-wide identification of a regulatory mutation in BMP15 controlling prolificacy in sheep. BioRxiv [Preprint]. doi: 10.1101/858860
Davis, G. H., Galloway, S. M., Ross, I. K., Gregan, S. M., Ward, J., Nimbkar, B. V., et al. (2002). DNA tests in prolific sheep from eight countries provide new evidence on origin of the Booroola (FecB) mutation. Biol. Reprod. 66, 1869–1874. doi: 10.1095/biolreprod66.6.1869
Demars, J., Cano, M., Drouilhet, L., Plisson-Petit, F., Bardou, P., Fabre, S., et al. (2017). Genome-wide identification of the mutation underlying fleece variation and discriminating ancestral hairy species from modern woolly sheep. Mol. Biol. Evol. 34, 1722–1729. doi: 10.1093/molbev/msx114
Demars, J., Fabre, S., Sarry, J., Rossetti, R., Gilbert, H., Persani, L., et al. (2013). Genome-wide association studies identify two novel BMP15 mutations responsible for an atypical hyperprolificacy phenotype in sheep. PLoS Genet. 9:e1003482. doi: 10.1371/journal.pgen.1003482
DePristo, M. A., Banks, E., Poplin, R., Garimella, K. V., Maguire, J. R., Hartl, C., et al. (2011). A framework for variation discovery and genotyping using next-generation DNA sequencing data. Nat. Genet. 43, 491–498. doi: 10.1038/ng.806
Dixit, H., Rao, L. K., Padmalatha, V. V., Kanakavalli, M., Deenadayal, M., Gupta, N., et al. (2006). Missense mutations in the BMP15 gene are associated with ovarian failure. Hum. Genet. 119, 408–415. doi: 10.1007/s00439-006-0150-0
Drouilhet, L., Mansanet, C., Sarry, J., Tabet, K., Bardou, P., Woloszyn, F., et al. (2013). The highly prolific phenotype of Lacaune sheep is associated with an ectopic expression of the B4GALNT2 gene within the ovary. PLoS Genet. 9:e1003809. doi: 10.1371/journal.pgen.1003809
Elvin, J. A., Yan, C., Wang, P., Nishimori, K., and Matzuk, M. M. (1999). Molecular characterization of the follicle defects in the growth differentiation factor 9-deficient ovary. Mol. Endocrinol. 13, 1018–1034. doi: 10.1210/mend.13.6.0309
Fabre, S., Pierre, A., Mulsant, P., Bodin, L., Di Pasquale, E., Persani, L., et al. (2006). Regulation of ovulation rate in mammals: contribution of sheep genetic models. Reprod. Biol. Endocrinol. 4:20. doi: 10.1186/1477-7827-4-20
Fonseca, D. J., Ortega-Recalde, O., Esteban-Perez, C., Moreno-Ortiz, H., Patiño, L. C., Bermúdez, O. M., et al. (2014). BMP15 c.-9C>G promoter sequence variant may contribute to the cause of non-syndromic premature ovarian failure. Reprod. BioMed. Online 29, 627–633. doi: 10.1016/j.rbmo.2014.07.018
Galloway, S. M., McNatty, K. P., Cambridge, L. M., Laitinen, M. P., Juengel, J. L., Jokiranta, T. S., et al. (2000). Mutations in an oocyte-derived growth factor gene (BMP15) cause increased ovulation rate and infertility in a dosage-sensitive manner. Nat. Genet. 25, 279–283. doi: 10.1038/77033
Gilmour, A. R., Gogel, B. J., Cullis, B. R., and Thompson, R. (2009). ASReml User-Guide Release 3.0. Hemel Hempstead: VSN International Ltd.
Hanevik, H. I., Hilmarsen, H. T., Skjelbred, C. F., Tanbo, T., and Kahn, J. A. (2011). A single nucleotide polymorphism in BMP15 is associated with high response to ovarian stimulation. Reprod. BioMed. Online 23, 97–104. doi: 10.1016/j.rbmo.2011.02.015
Hanrahan, J. P., Gregan, S. M., Mulsant, P., Mullen, M., Davis, G. H., Powell, R., et al. (2004). Mutations in the genes for oocyte-derived growth factors GDF9 and BMP15 are associated with both increased ovulation rate and sterility in cambridge and belclare sheep (Ovis aries). Biol. Reprod. 70, 900–909. doi: 10.1095/biolreprod.103.023093
Jansson, T. (2014). Genes Involved in Ovulation Rate and Litter Size in Sheep. Bachelor Thesis, Swedish University of Agricultural Sciences, Uppsala.
Kona, S. S. R., Praveen Chakravarthi, V., Siva Kumar, A. V. N., Srividya, D., Padmaja, K., and Rao, V. H. (2016). Quantitative expression patterns of GDF9 and BMP15 genes in sheep ovarian follicles grown in vivo or cultured in vitro. Theriogenology 85, 315–322. doi: 10.1016/j.theriogenology.2015.09.022
Kumar, S., Mishra, A. K., Kolte, A. P., Arora, A. L., Singh, D., and Singh, V. K. (2008). Effects of the Booroola (FecB) genotypes on growth performance, ewe’s productivity efficiency and litter size in Garole×Malpura sheep. Anim. Reprod. Sci. 105, 319–331. doi: 10.1016/j.anireprosci.2007.03.012
Lassoued, N., Benkhlil, Z., Woloszyn, F., Rejeb, A., Aouina, M., Rekik, M., et al. (2017). FecX (Bar) a Novel BMP15 mutation responsible for prolificacy and female sterility in Tunisian Barbarine Sheep. BMC Genet. 18:43. doi: 10.1186/s12863-017-0510-x
Liao, W. X., Moore, R. K., and Shimasaki, S. (2004). Functional and molecular characterization of naturally occurring mutations in the oocyte-secreted factors bone morphogenetic protein-15 and growth and differentiation factor-9. J. Biol. Chem. 279, 17391–17396. doi: 10.1074/jbc.M401050200
Martin, P., Raoul, J., and Bodin, L. (2014). Effects of the FecL major gene in the lacaune meat sheep population. Genet. Sel. Evol. 46:48. doi: 10.1186/1297-9686-46-48
Martinez-Royo, A., Jurado, J. J., Smulders, J. P., Martí, J. I., Alabart, J. L., Roche, A., et al. (2008). A deletion in the bone morphogenetic protein 15 gene causes sterility and increased prolificacy in rasa aragonesa sheep. Anim. Genet. 39, 294–297. doi: 10.1111/j.1365-2052.2008.01707.x
McNatty, K. P., Juengel, J. L., Reader, K. L., Lun, S., Myllymaa, S., Lawrence, S. B., et al. (2005). Bone morphogenetic protein 15 and growth differentiation factor 9 co-operate to regulate granulosa cell function in ruminants. Reproduction 129, 481–487. doi: 10.1530/rep.1.00517
Menchaca, A., Cuadro, F., Dos Santos-Neto, P. C., Bosolasco, D., Barrera, N., de Brun, V., et al. (2018). Oocyte developmental competence is improved by relatively greater circulating progesterone concentrations during preovulatory follicular growth. Anim. Reprod. Sci. 195, 321–328. doi: 10.1016/j.anireprosci.2018.06.010
Morón, F. J., de Castro, F., Royo, J. L., Montoro, L., Mira, E., Sáez, M. E., et al. (2006). Bone morphogenetic protein 15 (BMP15) alleles predict over-response to recombinant follicle stimulation hormone and iatrogenic ovarian hyperstimulation syndrome (OHSS). Pharmacogenet. Genomics 16, 485–495. doi: 10.1097/01.fpc.0000215073.44589.96
Mullen, M. P., Hanrahan, J. P., Howard, D. J., and Powell, R. (2013). Investigation of prolific sheep from UK and Ireland for evidence on origin of the mutations in BMP15 (FecX(G), FecX(B)) and GDF9 (FecG(H)) in Belclare and Cambridge sheep. PLoS One 8:e53172. doi: 10.1371/journal.pone.0053172
Palmer, J. S., Zhao, Z. Z., Hoekstra, C., Hayward, N. K., Webb, P. M., Whiteman, D. C.D. C., et al. (2006). Novel variants in growth differentiation factor 9 in mothers of dizygotic twins. J. Clin. Endocrinol. Metab. 91, 4713–4716. doi: 10.1210/jc.2006-0970
Persani, L., Rossetti, R., Di Pasquale, E., Cacciatore, C., and Fabre, S. (2014). The fundamental role of bone morphogenetic protein 15 in ovarian function and its involvement in female fertility disorders. Hum. Reprod. Update 20, 869–883. doi: 10.1093/humupd/dmu036
Pfaffl, M. W., Tichopad, A., Prgomet, C., and Neuvians, T. P. (2004). Determination of stable housekeeping genes, differentially regulated target genes and sample integrity: bestkeeper–Excel-based tool using pair-wise correlations. Biotechnol. Lett. 26, 509–515. doi: 10.1023/b:bile.0000019559.84305.47
Poivey, J. P., Tiphine, L., Berny, F., and Jullien, E. (1995). Estimation of breeding values under BLUP animal model methodology for meat sheep. Rencontres Recherches Ruminants 2, 453–456.
Robinson, J. T., Thorvaldsdóttir, H., Winckler, W., Guttman, M., Lander, E. S., Getz, G., et al. (2011). Integrative genomics viewer. Nat. Biotech. 29, 24–26. doi: 10.1038/nbt.1754
Rochus, C. M., Tortereau, F., Plisson-Petit, F., Restoux, G., Moreno-Romieux, C., Tosser-Klopp, G., et al. (2018). Revealing the selection history of adaptive loci using genome-wide scans for selection: an example from domestic sheep. BMC Genomics 19:71. doi: 10.1186/s12864-018-4447-x
Rose, R. D., Gilchrist, R. B., Kelly, J. M., Thompson, J. G., and Sutton-McDowall, M. L. (2013). Regulation of sheep oocyte maturation using cAMP modulators. Theriogenology 79, 142–148. doi: 10.1016/j.theriogenology.2012.09.020
Scheet, P., and Stephens, M. (2006). A fast and flexible statistical model for large-scale population genotype data: applications to inferring missing genotypes and haplotypic phase. Am. J. Hum. Genet. 78, 629–644. doi: 10.1086/502802
Silva, B. D. M., Castro, E. A., Souza, C. J. H., Paiva, S. R., Sartori, R., Franco, M. M., et al. (2011). A new polymorphism in the growth and differentiation factor 9 (GDF9) gene is associated with increased ovulation rate and prolificacy in homozygous sheep. Anim. Genet. 42, 89–92. doi: 10.1111/j.1365-2052.2010.02078.x
Talebi, R., Ahmadi, A., Afraz, F., Sarry, J., Plisson-Petit, F., Genêt, C., et al. (2018a). Transcriptome analysis of ovine granulosa cells reveals differences between small antral follicles collected during the follicular and luteal phases. Theriogenology 108, 103–117. doi: 10.1016/j.theriogenology.2017.11.027
Talebi, R., Ahmadi, A., Afraz, F., Sarry, J., Woloszyn, F., and Fabre, S. (2018b). Detection of single nucleotide polymorphisms at major prolificacy genes in the Mehraban sheep and association with litter size. Ann. Anim. Sci. 18, 685–698. doi: 10.2478/aoas-2018-0014
Teixeira Filho, F. L., Baracat, E. C., Lee, T. H., Suh, C. S., Matsui, M., Chang, R. J., et al. (2002). Aberrant expression of growth differentiation factor-9 in oocytes of women with polycystic ovary syndrome. J. Clin. Endocrinol. Metab. 87, 1337–1344. doi: 10.1210/jcem.87.3.8316
Van der Auwera, G. A., Carneiro, M. O., Hartl, C., Poplin, R., del Angel, G., Levy-Moonshine, A., et al. (2013). From FastQ data to high confidence variant calls: the genome analysis toolkit best practices pipeline. Curr. Protoc. Bioinformatics 43, 11.10.1–11.10.33. doi: 10.1002/0471250953.bi1110s43
Wan, Q., Wang, Y., and Wang, H. (2015). Identification and Analysis of Regulatory Elements in Porcine Bone Morphogenetic Protein 15 Gene Promoter. Int. J. Mol. Sci. 16, 25759–25772. doi: 10.3390/ijms161025759
Wei, L.-N., Huang, R., Li, L.-L., Fang, C., Li, Y., and Liang, X.-Y. (2014). Reduced and delayed expression of GDF9 and BMP15 in ovarian tissues from women with polycystic ovary syndrome. J. Assist. Reprod. Genet. 31, 1483–1490. doi: 10.1007/s10815-014-0319-8
Wilson, T., Wu, X. Y., Juengel, J. L., Ross, I. K., Lumsden, J. M., Lord, E. A., et al. (2001). Highly prolific Booroola sheep have a mutation in the intracellular kinase domain of bone morphogenetic protein IB receptor (ALK-6) that is expressed in both oocytes and granulosa cells. Biol. Reprod. 64, 1225–1235. doi: 10.1095/biolreprod64.4.1225
Keywords: GWAS, major gene, prolificacy, sheep, BMP15
Citation: Chantepie L, Bodin L, Sarry J, Woloszyn F, Plisson-Petit F, Ruesche J, Drouilhet L and Fabre S (2020) Genome-Wide Identification of a Regulatory Mutation in BMP15 Controlling Prolificacy in Sheep. Front. Genet. 11:585. doi: 10.3389/fgene.2020.00585
Received: 16 January 2020; Accepted: 14 May 2020;
Published: 19 June 2020.
Edited by:
Luis Varona, University of Zaragoza, SpainReviewed by:
Shahin Eghbalsaied, Islamic Azad University, IranBrian Kirkpatrick, University of Wisconsin–Madison, United States
Copyright © 2020 Chantepie, Bodin, Sarry, Woloszyn, Plisson-Petit, Ruesche, Drouilhet and Fabre. This is an open-access article distributed under the terms of the Creative Commons Attribution License (CC BY). The use, distribution or reproduction in other forums is permitted, provided the original author(s) and the copyright owner(s) are credited and that the original publication in this journal is cited, in accordance with accepted academic practice. No use, distribution or reproduction is permitted which does not comply with these terms.
*Correspondence: Stéphane Fabre, c3RlcGhhbmUuZmFicmVAaW5yYWUuZnI=
†Present address: Louise Chantepie, Institut de l’Elevage, Castanet-Tolosan, France