- 1Department of Pediatrics, Samsung Medical Center, Sungkyunkwan University School of Medicine, Seoul, South Korea
- 2Department of Laboratory Medicine and Genetics, Hanyang University Guri Hospital, Hanyang University College of Medicine, Guri, South Korea
- 3Samsung Genome Institute, Sungkyunkwan University School of Medicine, Seoul, South Korea
- 4Department of Neurology, Samsung Medical Center, Sungkyunkwan University School of Medicine, Seoul, South Korea
- 5Green Cross Genome, Yongin, South Korea
Microcephaly is a prevalent phenotype in patients with neurodevelopmental problems, often with genetic causes. We comprehensively investigated the clinical phenotypes and genetic background of microcephaly in 40 Korean patients. We analyzed their clinical phenotypes and radiologic images and conducted whole exome sequencing (WES) and analysis of copy number variation (CNV). Infantile hypotonia and developmental delay were present in all patients. Thirty-four patients (85%) showed primary microcephaly. The diagnostic yield from the WES and CNV analyses was 47.5%. With WES, we detected pathogenic or likely pathogenic variants that were previously associated with microcephaly in 12 patients (30%); nine of these were de novo variants with autosomal dominant inheritance. Two unrelated patients had mutations in the KMT2A gene. In 10 other patients, we found mutations in the GNB1, GNAO1, TCF4, ASXL1, SMC1A, VPS13B, ACTG1, EP300, and KMT2D genes. Seven patients (17.5%) were diagnosed with pathogenic CNVs. Korean patients with microcephaly show a genetic spectrum that is different from that of patients with microcephaly of other ethnicities. WES along with CNV analysis represents an effective approach for diagnosis of the underlying causes of microcephaly.
Introduction
Microcephaly is a prevalent phenotypic feature of patients with neurodevelopmental problems (Vargas et al., 2001; Abuelo, 2007). It is defined as an occipitofrontal circumference (OFC) lower than the third percentile or more than two standard deviations below the mean for sex, age, and ethnicity. Patients with microcephaly show various neurological manifestations, including psychomotor problems, delayed developments, and epilepsy, which are frequently accompanied by facial dysmorphism, skeletal anomalies, and congenital structural anomalies in the major organs (Vargas et al., 2001). Recognition of microcephaly can prompt clinicians to investigate its causes.
The fundamental size of the brain is determined by neuronal progenitor cells formed at conception and the cell divisions. During cell division, microcephaly may be caused by deficiency of neuropils, increased apoptosis of progenitor cells, or improper mitosis of these cells, which is defined as primary microcephaly (Gilmore and Walsh, 2013). Most primary microcephaly cases occur due to the failure of neurogenesis or destructive prenatal events associated with environmental or maternal conditions. In contrast, children with secondary microcephaly are born with normal head circumference (HC) and then show a progressive decrease in HC with age. Secondary microcephaly represents abnormal neuronal development after birth or a perinatal brain insult (Woods and Parker, 2013). Children with microcephaly have OFCs at the outer limits of the normal distribution and low for their age group. Both categories of microcephaly have been associated with various causative factors, including congenital infection, perinatal problems such as hypoxia or a maternal medical condition, or genetic causes (Abuelo, 2007), and the distinction between them helps clinicians to isolate the preferential etiologies. Additionally, accompanying anomalies or facial dysmorphism can provide clues to determine the exact cause of microcephaly (von der Hagen et al., 2014).
In previous studies, genetic causes of microcephaly showed an autosomal recessive inheritance pattern (Darvish et al., 2010; Sajid Hussain et al., 2013). With the development of next-generation sequencing, the genetic causes of neurodevelopmental disorders, including microcephaly, have been identified (Najmabadi et al., 2011; Hamdan et al., 2014; Thevenon et al., 2016). To date, only three studies have used whole exome sequencing (WES) to investigate genetic causes of microcephaly in microcephaly cohorts (Rump et al., 2016; Boonsawat et al., 2019; Shaheen et al., 2019). One study determined potential causative genes in 11 out of 38 patients who had highly heterogeneous clinical and radiologic phenotypes. It revealed that autosomal recessive disorders were highly prevalent among patients with microcephaly (Rump et al., 2016; Shaheen et al., 2019) identified potentially causal genetic variants in 104 out of 137 families. They found variants in novel and previously reported genes that were associated with microcephaly with established disease phenotypes. Additionally, the authors showed overlap in the genetic causes of microcephaly with genes underlying microcephalic primordial dwarfism. A recent comprehensive genetic analysis based on chromosomal microarray (CMA) and WES detected causative variants in 48.4% of the patients (30/62) and analyzed the differences in clinical severity and genetic variants between primary and secondary microcephaly (Boonsawat et al., 2019). Each of these studies covered different characteristics of race, consanguinity, and findings of brain imaging. However, there has been no study of an Asian population based exclusively on a microcephaly cohort.
Therefore, in this study, we aimed to comprehensively analyze the genomic and phenotypic features of Korean patients with microcephaly. We attempted to delineate the clinical features associated with the potential causative genes to contribute toward our understanding of the genetic features of patients with microcephaly.
Materials and Methods
Patients
We recruited 40 pediatric patients with microcephaly, using a definition of microcephaly of OFC >2 standard deviations below the mean for same sex, age-matched Korean references, between January 2014 and December 2018 at the Samsung Medical Center in Seoul, Korea. All affected individuals were evaluated for clinical features and diagnosed with microcephaly by two pediatric neurologists. We excluded patients with microcephaly that resulted from cerebral insults related to defined perinatal problems such as congenital infection, teratogens, physical environment, or maternal medical conditions assessed via laboratory tests, brain imaging, and detailed medical history. We performed WES for all patients and CMA or low-depth whole genome sequencing (LD-WGS) to analyze copy number variation (CNV) (Figure 1). Among the patients that participated in this study, we performed trio WES for 23 patients whose parents' samples were available. The other 17 patients were subjected to proband-only WES. We measured weights, heights, and HCs in the same examination and evaluated concordance between the body gauges. Clinical phenotypes included the following: infantile hypotonia, facial dysmorphism, skeletal and organ anomalies, hearing loss, developmental delay/cognitive impairment, seizure, and affected epilepsy syndrome. Development and cognitive function were assessed using the Bayley Scales of Infant Development (BSID-III) or the Wechsler Scale of Intelligence for children (WISC-V), depending on the age of the patient. If these scales were not available, we estimated developmental stage or cognitive function based on the clinical status assessed by two pediatric neurologists using the same standards.
WES
Genomic DNA was extracted from peripheral blood leukocytes using a Wizard Genomic DNA Purification Kit following the manufacturer's instructions (Promega, Madison, WI, USA). SureSelect Human All Exon V5 (Agilent Technologies, Santa Clara, CA, USA) was used for library preparation, and sequencing was performed on an Illumina HiSeq2500 platform (Illumina Inc., San Diego, CA, USA) to generate 2 × 100-bp paired-end reads. Sequence reads were aligned against the Human Reference Genome build GRCh37 using BWA 0.7.12; duplicated reads were marked with Picard Tools 1.130. Local alignment, base quality recalibration, and variant calling were conducted using the Genome Analysis Tool Kit v3.4.0, and annotation and variant effect prediction were performed with SnpEff v4.1g.
Variant Filtering Steps and Data Analysis
Variants were filtered and prioritized using a four-step strategy to generate a short candidate variant list for experimental validation (Supplementary Figure 1). Initially, we removed variants with <10× coverage. However, before removing <10× variants, ClinVar (https://www.ncbi.nlm.nih.gov/clinvar/) and Human Gene Mutation Database (HGMD, http://www.hgmd.org/) were checked for any previously reported pathogenic or likely pathogenic variants, and such variants were not filtered out regardless of whether they were <10×. Next, variants were limited to those with low population frequency. The minor allele frequency (MAF) threshold was carefully chosen and variants with an MAF ≥ 1% in the Genome Aggregation Database (gnomAD) (http://gnomad.broadinstitute.org/) or the Korean Reference Genome Database (KRGDB) (http://coda.nih.go.kr/coda/KRGDB/index.jsp) were removed. The third step was to prioritize variants causing missense, non-sense, frameshifts, and in-frame insertions/deletion variants, or changes affecting consensus splice site sequences. Finally, we performed a gene-specific analysis with an in silico gene panel composed of 885 genes, for filtering selected phenotype traits based on the Human Phenotype Ontology (HPO)-terms for Microcephaly (HP:0000252) or Online Mendelian Inheritance in Man (OMIM) microcephaly phenotype genes (Supplementary Table 1). To delineate candidate genetic variants, an additional allele analysis was performed under the following conditions: (1) triplicate data with no pathogenic variants (PVs) nor likely pathogenic variants (LPVs), (2) de novo, compound heterozygous, homozygous, or hemizygous variants, (3) ≤ 2 alleles in gnomAD or ≤ 8 alleles if recessive, (4) a CADD score of ~15 or higher and all deleterious predictions in SIFT (http://sift.jcvi.org), PolyPhen2 (http://genetics.bwh.harvard.edu/pph2), and MutationTaster (http://mutationtaster.org/) if missense variants, (5) affected genes with data from animal models and/or functional studies suggesting neurodevelopmental roles.
CMA and LD-WGS
CMA was performed using an Illumina HumanCytoSNP-12 platform (Illumina, San Diego, CA, USA). The array employs both Copy Number Changes (CNC) and Single Nucleotide Polymorphism (SNP) probes on a whole genome array with a 30-Kb resolution. A higher resolution was used for all regions known to be associated with balanced and unbalanced structural variants. The data were analyzed in the Illumina KaryoStudio version 1.4.3.0 (Illumina, San Diego, CA, USA) and were reported using NCBI human genome build 37.1 (hg19) following ISCN nomenclature.
The LD-WGS-based CNV analysis was performed by GC Genome (Yongin, Korea). Briefly, genomic DNA was extracted from peripheral blood leukocytes, sheared to a target size of 250 bp, and sequenced on a NextSeq 500 platform (Illumina, CA, USA) in a 75-bp single-read mode. CNV calling for 3.1 million sequence reads was performed using the DNAcopy software v1.38. Using the target-specific reference mapping ratio values for the target sample and the normal controls, we calculated the copy number status of each targeted sample. The February 2009 Human reference sequence (GRCh37/hg19) was used for genome assembly.
Interpretation of Candidate Genetic Variants by WES and CNVs by CMA or LD-WGS
Candidate variants were classified according to the standards and guidelines of the American College of Medical Genetics and Genomics (ACMG) and Association for Molecular Pathology (AMP) (Richards et al., 2015). These guidelines recommend classifying variants into five categories: PV, LPV, variant of uncertain significance (VUS), likely benign variant (LBV), and benign variant (BV) based on combined lines of weighted evidence, including population, computational, functional, segregation, de novo, allelic, and other data. To assess the frequency of a variant in a control or general population, we used the KRGDB, which consists of publicly available race-matched control data from WGS of 1100 Korean individuals, as well as other public databases such as the 1,000 Genomes Project database, ExAC database, and gnomAD. A primary literature review was conducted using various sources cited in the HGMD professional version (release 2018.02), ClinVar, and PubMed, to determine the potential pathogenicity of all identified variants. Various in silico tools, including SIFT, PolyPhen2, and MutationTaster, all of which use missense prediction algorithms, were used to determine the predicted impact of a missense change.
To interpret CNVs, we used DGV (http://dgv.tcag.ca/dgv/app/home), DECIPHER (https://decipher.sanger.ac.uk/), and dbVar (https://www.ncbi.nlm.nih.gov/dbvar/) databases as well as OMIM (https://www.omim.org/). Additionally, the clinical findings were compared with those reported in the literature.
Confirmation and Validation of Candidate Variants
Candidate variants identified in WES data were confirmed using standard PCR and Sanger sequencing methods. Primer sequences are available upon request. Sequence data were aligned to the reference sequence using the Sequencher software (Gene Codes Corporation, Ann Arbor, MI, USA).
Results
Clinical Phenotypes of Patients
Among 40 patients (female:male = 22:18) with microcephaly, 34 patients presented with primary microcephaly at birth and the remaining six were identified as cases of secondary microcephaly based on the gradual development of a small HC after birth (Table 1). With proportionate microcephaly identified in 15 patients (37.5%), all body gauges were below the sex and age-matched 3rd percentile value. The other 25 patients (62.5%) showed disproportionate microcephaly, with height and/or weight in the normal range for sex and age. All patients with short stature (n = 26) displayed type 3 failure to thrive during their infancy. Common features observed in all patients were infantile hypotonia and developmental delay. The average age at the time of participation in this study was 5.28 ± 4.60 years. Thirty-two patients showed noticeable developmental delay or intellectual disability. Of the remaining patients, one showed mild intellectual disability and seven displayed a delay (5 months below age) in reaching developmental milestones. Patients of a preschool age (n = 25) also lagged in achieving developmental milestones, and eight patients of a schoolgoing age received special education. Four patients were treated for autism spectrum disorder (n = 3) or behavioral disorder (n = 1) in a neuropsychiatric clinic. Seven patients showed frequent dystonia or other involuntary movements like chorea, athetoid movements, or tremor.
Seventeen patients (42.5%) showed various accompanying anomalies, including congenital heart anomaly (n = 8), skeletal anomaly (n = 4), and cleft lip and/or palate (n = 5) (Table 1). Three patients developed sensorineural hearing loss without a discernible etiology. Dysmorphic features were evident in more than half of the patients (n = 28, 70%); these features were clear in 17 patients (42.5%) and notably vague in 11 patients (27.5%). Twenty-seven patients (67.5%) had seizures. One of these patients had only one episode of seizure provoked by febrile illness. Among the 26 patients with epilepsy, 11 showed intractable epilepsy, with frequent seizures despite multiple anti-epileptic medications. There were eight patients diagnosed with epileptic encephalopathy, including six patients with Lennox-Gastaut syndrome (LGS). Three of the patients with LGS showed infantile spasms, and one patient had a neonatal seizure.
Brain MRIs were performed in all patients, and abnormal structural lesions were found in six patients. Three patients had agenesis or hypoplasia of the corpus callosum with microcephaly. In another three patients, holoprosencephaly, subependymal heterotopia, and hypoplasia of the pons and cerebellum were found, respectively. Thirty-four patients showed normal brain structure using MRI. Further details of all patients can be found in Supplementary Table 2.
Genetic Evaluation of Patients
A molecular diagnosis was established in 19 patients (47.5%) from 39 families. In 12 patients, including one sibling pair (30%), 11 PVs or LPVs were identified by WES (Table 2). The inheritance patterns of these mutations were autosomal dominant (n = 9), autosomal recessive (n = 1), and X-linked dominant (n = 1). Another seven patients (17.5%) showed a pathogenic CNV by CMA or LD-WGS (Table 3). Using WES, PVs or LPVs were detected in 10 genes previously associated with microcephaly: GNB1, GNAO1, TCF4, ASXL1, SMC1A, KMT2A, VPS13B, ACTG1, EP300, and KMT2D. Five PVs or LPVs were novel variants, and nine PVs or LPVs were de novo variants. Identified variants except BV and LBV are described in Supplementary Table 3.
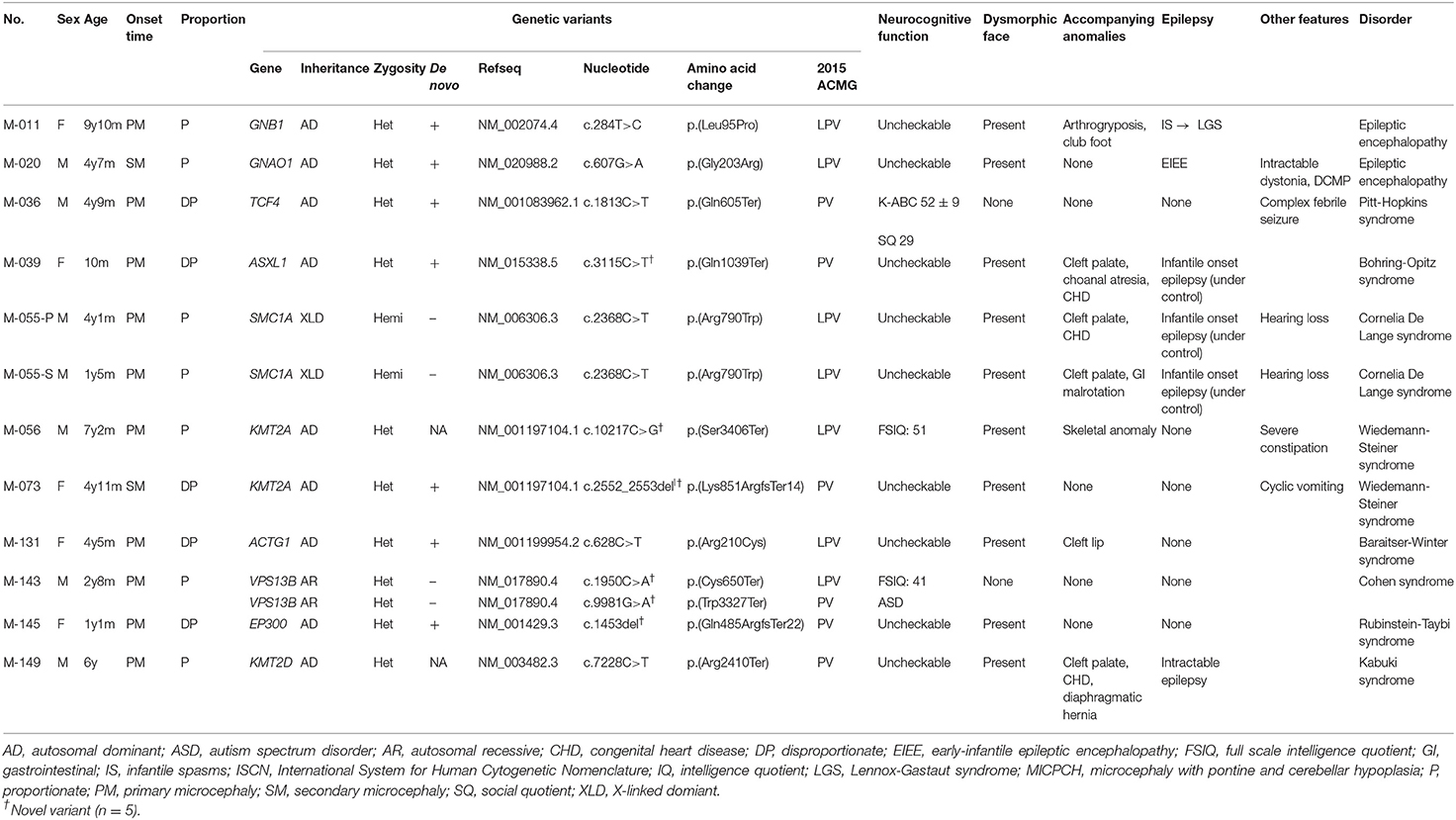
Table 2. Clinical characteristics of 12 patients having pathogenic or likely pathogenic variants via whole exome sequencing.
Clinical Phenotypes of the Patients With a PV/LPV Determined by WES
There was a 9-year-old girl (M-011) with an LPV in the GNB1 gene. She presented with infantile spasms at the age of 4 months and was treated with anti-epileptic medications. Her epileptic spasms disappeared during infancy, but she failed to reach developmental milestones. Gradually, different types of seizures and involuntary movements developed, despite administration of a number of anti-epileptic medications. A de novo LPV (c.284T>C;p.Leu95Pro) that was absent from the control databases was identified in the GNB1 gene. This c.284T>C variant was previously associated with de novo severe neurodevelopmental disability, hypotonia, and seizures (Petrovski et al., 2016).
In a 4-year-old boy (M-020) suffering from intractable dystonia with severe failure to thrive and profound psychomotor retardation, a de novo LPV (c.607G>A;p.Gly203Arg) that was absent from the control databases was identified in the GNAO1 gene. A de novo c.607G>A change in GNAO1 has been previously reported many times in patients with epileptic encephalopathy, suggesting that it is a mutation hotspot (Arya et al., 2017).
In patient M-039, a PV (c.3115C>T;p.Gln1039Ter) of the ASXL1 gene was identified. She was admitted to the neonatal intensive care unit for congenital hypotonia. As her respiration was unstable and weak, a tracheostomy was performed and a home ventilator support was provided for her. She showed severe feeding problems and extreme failure to thrive. Additionally, she suffered from intractable epilepsy and severe dystonia. The c.3115C>T variant in ASXL1 was absent in the control population and occurred de novo. This c.3115C>T variant was previously reported mainly as a somatic variant in patients with myelodysplastic syndrome (Wang et al., 2013), but no germline variant has been reported.
In the SMC1A gene, known as a causative gene of Cornelia de Lange syndrome (Ansari et al., 2014), an LPV (c.2368C>T;p.Arg790Trp) was identified in a male sibling pair (M-055-P, M-055-S) clinically suspected of Cornelia de Lange syndrome. The pair showed distinctive facial features, namely long eyelashes, synophrys, small nose, wide nasal bridge, low-set ears, and small chin. They could not speak meaningful words and showed severe growth retardation in not only HC, but also height and weight. The c.2368C>T variant in SMC1A was hemizygous. The patients' mother had this variant; in her case, it was heterozygous. There were two unaffected maternal uncles, who were unmarried. They displayed normal intelligence and facial features, and were not tested for the variant.
Clinical Phenotypes of Patients With a Pathogenic CNV
Of the 40 patients, 7 (17.5%) were found to have a pathogenic CNV by CMA or LD-WGS (Table 3). The size of duplication or deletion region was between 312 Kb and 28.43 Mb.
Patient M-004 presented with severe intellectual disability, autistic behaviors, epilepsy, and dysmorphic facial features, and showed an 801-Kb deletion at 7q11.21. This region contains the AUTS2 gene, and the deletion of the AUTS2 gene has been reported to be associated with autism, intellectual disability, short stature, and microcephaly (Beunders et al., 2013).
M-017 presented with primary microcephaly and congenital heart disease, and showed a 2,790-Kb deletion at 9q34.3. This region was previously associated with 9q subtelomeric syndrome characterized by severe developmental delay, microcephaly, congenital heart disease, and seizure (Stewart and Kleefstra, 2007).
M-048 presented with primary microcephaly and congenital heart disease, and displayed a 28.43-Mb duplication at 16p13.3p11.2. This region was registered at OMIM as being associated with chromosome 16p11.2 duplication syndrome (MIM 614671). Patients with a 16p11.2 duplication have been reported to exhibit motor delays, congenital anomalies, seizures, and microcephaly (Shinawi et al., 2010).
M-074 presented with primary microcephaly, epilepsy, and cleft palate, and carried a 368-Kb duplication at 6q25.3. This region contains the ARID1B gene, whose mutation is responsible for Coffin-Siris syndrome. This syndrome is characterized by mental retardation and associated with coarse facial features, hypertrichosis, sparse scalp hair, and hypoplastic or absent fifth fingernails or toenails. Patients with a ARID1B duplication have been reported to show moderate developmental delays, hypotonia, and high-arched palate (Hoyer et al., 2012).
A girl (M-075) showing microcephaly with pontine and cerebellar hypoplasia evidenced by brain MRI was found to have a 2.8-Mb deletion at Xp11.4p11.3 (Figure 2). This region contains the CASK gene, whose deletion has been associated with mental retardation and microcephaly with disproportionate pontine and cerebellar hypoplasia in females (Hayashi et al., 2012).
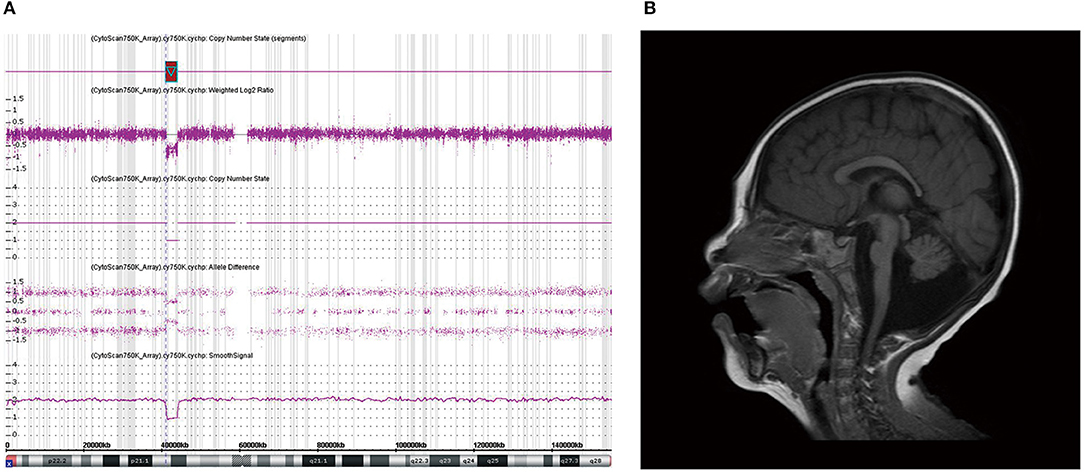
Figure 2. Chromosomal microarray (CMA) and brain magnetic resonance imaging (MRI) of patient M-075. (a) CMA showing a 2.8-Mb deletion at Xp11.4p11.3 containing the CASK gene. (b) Brain MRI of patient (M-075) demonstrating the microcephaly with pontine and cerebellar hypoplasia in the T2-weighted sagittal view.
M-116 presented with intellectual disability and autistic features, midline hypoplasia of the face, hypertrichosis, and bush eyebrows, and showed a 312-Kb deletion at 2q23.1. This region contains the MBD5 gene, whose deletion has been associated with intellectual disability, seizures, significant speech impairment, and behavioral problems (Bonnet et al., 2013).
Additional Candidate Microcephaly Genes
An allele search in familial trio exome data revealed four additional candidate genes (Table 4). In a patient (M-001) with primary microcephaly, we found a de novo variant (c.2123A>C;p.Tyr708Ser) in the JARID2 gene. JARID2 (jumonji and AT-rich interaction domain containing 2) is highly homologous to mouse Jumonji (Jmj). In mice, Jmj is required for normal morphogenesis of the neural tube (Takeuchi et al., 1995). This c.2123A>C variant in JARID2 was absent from the control databases.
RFX7 (regulatory factor X7) is a member of the regulatory factor X (RFX) family of transcription factors (Aftab et al., 2008). RFX7 is required for the formation of cilia in the neural tube (Manojlovic et al., 2014). In one patient (M-100), we found a de novo variant (c.2727_2730dup;p.Gly911PhefsTer58) in the RFX7 gene that was absent from the control databases.
LMNB1 (lamin B1) encodes one of the two B-type lamin proteins. Lamin B1, a key component of the nuclear lamina, plays an important role in brain development (Mahajani et al., 2017). A heterozygous tandem genomic duplication of LMNB1 is known to be the cause of autosomal dominant adult-onset demyelinating leukodystrophy. In one patient (M-118), we found a de novo variant (c.1091T>C;p.Leu364Pro) in this gene that was absent from the control databases.
HPRT1 (hypoxanthine-guanine phosphoribosyltransferase 1) encodes hypoxanthine-guanine phosphoribosyl transferase, an enzyme involved in purine metabolism. An HPRT1 gene mutation is the cause of Lesch-Nyhan syndrome, which is an X-linked recessive disorder (Gibbs and Caskey, 1987). In one patient (M-122), we identified a hemizygous variant (c.151C>T;p.Arg51Ter) that was inherited from his mother. This c.151C>T variant was absent from the control databases and was previously reported in a Japanese patient with Lesch-Nyhan syndrome (Fujimori et al., 1990). The patient in this study did not have the clinical characteristics of that syndrome.
Discussion
This study used WES and CNV analyses to identify genetic causes of microcephaly in Korean patients. The overall diagnostic rate was 47.5%, with 12 cases (30%) identified by using WES and 7 cases identified by using CNV analysis. Nine variants among all the variants found by using WES, including five novel ones, were de novo and exhibited autosomal dominant traits. Of the 40 patients included in this study, 34 patients (85%) had primary microcephaly. Six showed various structural anomalies as evidenced by brain MRIs, whereas the others showed normal brain structures despite microcephaly. Hypotonia and developmental delay were the most frequent accompanying symptoms. Seizure was the next most frequent symptom (n = 27, 65%), and 11 patients had intractable seizures.
Microcephaly can present as non-syndromic or with various hereditary syndromic features (von der Hagen et al., 2014). According to the OMIM database, there are reportedly over 900 OMIM phenotypes and genes related to microcephaly. In the present study, the diagnostic yield of WES (PVs and LPVs) was 30% and was comparable to that found in previous reports of genetic causes of microcephaly (Rump et al., 2016; Boonsawat et al., 2019; Shaheen et al., 2019). In general, the diagnostic yield of WES in neurodevelopmental disorders ranges from 17.5 to 29% (Vissers et al., 2017; Marques Matos et al., 2019). The yield depends on several factors such as phenotypic distinctiveness of the disease, genes associated with the phenotype, sequence read depth, bioinformatics filtering, and/or level of clinical medical review (Farwell et al., 2015; Di Resta and Ferrari, 2018; Prodan Zitnik et al., 2018; Trinh et al., 2019). Some neurological disorders are more closely associated with muscle disease, ataxia, and epilepsy, which are known to have heterogeneous genetic causes (Farwell et al., 2015; Marques Matos et al., 2019). Thus, WES should be the first-line test for complex diseases presumed to have extensive genetic causes, based on considerations of diagnostic yield and economic cost (Vissers et al., 2017; Marques Matos et al., 2019). Similar to those identified in previous reports, the variants found in this study were highly diverse. This study reconfirms the utility of WES in determination of the genetic causes of microcephaly.
In this study, the CNV analysis increased the diagnostic rate from 30 to 47.5%. This rate was similar to that reported in a recent study by Boonsawat et al. (2019). The diagnostic rate of pathogenic CNVs in neurodevelopmental disorders, including primary microcephaly, has been reported to be 13.2–20.8% (Shoukier et al., 2013; Jang et al., 2019). According to a previous study, combined WES and CNV analyses enable better diagnosis of rare neurological disorders, with a 47.8% diagnostic rate, compared to that observed with WES alone (32.7%) (Jiao et al., 2019), which is consistent with the results of this study. A recent study showed 11 definite and 7 probable CNVs in 53 patients with primary microcephaly (Tsoutsou et al., 2017). Therefore, WES with CNV analysis may be the most effective approach to diagnose the underlying causes of microcephaly. A diagnosis of microcephaly via WES and CNV analysis can help to determine the genetic mechanism of the disease and predict the prognosis. Additionally, it may provide information to patients for future reproductive decisions and genetic counseling, including opportunities for targeted therapies.
In the present study, most of the detected variants showed an autosomal dominant inheritance pattern. Two variants were associated with autosomal recessive and X-linked dominant inheritance, respectively. Previous studies dealt with congenital microcephaly in consanguineous families and mainly focused on neurodevelopmental defects in the fetal period (Darvish et al., 2010; Sajid Hussain et al., 2013; Shaheen et al., 2019). They classified cases of microcephaly as autosomal recessive primary microcephaly (MCPH), and 18 loci in MCPH genes have been revealed (Jayaraman et al., 2018). In our patients, no mutations were identified in MCPH1, WDR62, CDK5RAP2, CEP152, ASPM, CENPJ, STIL, CEP63, CEP135, CASC5, PHC1, CDK6, CENPE, SASS6, MFSD2A, ANKLE2, CIT, or WDFY3 genes. Our results suggest that autosomal dominant disorders are highly prevalent among Korean patients with microcephaly. The microcephaly-associated genes identified in this study were diverse, and no genes were found to be predominant causes of microcephaly. This suggests that various genes may lead to microcephaly, as reported previously (Shaheen et al., 2019). These genes are involved in neuronal myelination, neurotransmission, or regulation of neuronal excitability, rather than being involved in centrosome-related neurogenesis and DNA damage repair process (Woods and Parker, 2013).
Among 19 patients who were established their genetic diagnosis in this study, 14 patients were concluded as syndromic microcephaly. Syndromic microcephaly can occur in both primary and secondary microcephaly and most are accompanied by other organ anomalies (von der Hagen et al., 2014). Through these characteristic phenotypes, including other organ anomalies, dysmorphism, laboratory findings, we can predict a putative cause of the patient with microcephaly indirectly. However, even with syndromic microcephaly, it is not easy to approach the genetic diagnosis only with clinical information which the patient has. Some of the patients with syndromic microcephaly confirmed genetically in this study also had no other organ anomalies, but only had intellectual disabilities or vague dysmorphism. For this reason, it was difficult to conclude the genetic diagnosis with only developmental delay and small head circumference. In the other two studies of microcephaly that included syndromic and non-syndromic microcephaly, various genetic findings were found through WES and CMA similar to our study (Rump et al., 2016; Boonsawat et al., 2019). On the other hand, the results of study on non-syndromic microcephaly by Shaheen et al. (2019) showed different genetic spectrum of microcephaly.
In this study, 34 patients showed normal brain structures despite a small brain size. In the six patients (15%) with structural abnormalities observed by brain MRI, only one patient was found to have a causative genetic mutation in the CASK gene. The CASK gene encodes a membrane-associated guanylate kinase and is involved in neurotransmitter regulation, axon branching, and dendritic outgrowth. The patient with this gene variant showed a wide range of X-linked intellectual disabilities, including microcephaly with pontine and cerebellar hypoplasia (MICPCH) syndrome, nystagmus, dysmorphic faces, and hypotonia (Hayashi et al., 2017). As this is located on the X chromosome, most cases of CASK mutation are exhibited by females, and affected males are very rare and show severe manifestations (Cristofoli et al., 2018). We found a CASK mutation through CMA in a female patient with MICPCH syndrome. She was born at term without perinatal complications. Her HC at birth was 32.2 cm, and the fourth ventricle was extensively dilated according to a brain ultrasonogram performed on the second day of birth. She showed poor growth in all body indices and dysmorphic facial features such as arched eyebrows, midline hypoplasia, long philtrum, and micrognathia. Her brain MRI findings were consistent with the criteria for microcephaly and pontocerebellar hypoplasia, and her achievement of developmental milestones being assessed at 1 year of age showed at the 5-month level (Figure 2).
Racially homogeneous Korean patients with microcephaly, with no consanguineous family members, were enrolled in this study. This is the first study delineating the genetic causes of microcephaly in East Asia. The patients in this study showed the same clinical characteristics that were reported in previous studies, including infantile hypotonia and developmental delay. Epilepsy was observed in 65% of the patients, and approximately half of these patients showed an intractable clinical course. Additionally, movement disorders, behavioral problems, and autism spectrum disorder were noted, which were similar to the features reported in a recent study (Boonsawat et al., 2019). However, our study showed different genetic peculiarities from those reported previously for patients in the Middle East or Europe, in which autosomal recessive inheritance was frequent. In our study, 75% of the detected variants were autosomal dominant; only one sibling pair had an SMC1A gene variant with X-linked inheritance. These results suggest that the genetic spectrum of microcephaly may differ between ethnic groups, even if clinical characteristics are similar. Therefore, consideration of the racial background may be helpful to interpret WES results.
This study had several limitations. First, the number of patients in our study was small to determine the detection rate for genetic causes of microcephaly. Second, a functional study of detected VUSs was not performed. Although not classified as PVs or LPVs, some of the VUSs found in this study may be useful in determination of the genetic cause of microcephaly through additional studies to verify gene function. Additionally, we did not identify cases of somatic mosaicism and balanced translocation, which is difficult to confirm by WES or CMA. However, somatic mosaicism and balanced translocation have been rarely reported as genetic causes of microcephaly.
We conclude that conducting WES and CMA for patients with microcephaly is useful and time-/cost-effective for genetic diagnosis and treatment considerations. Unlike other studies, autosomal recessive microcephaly was rare, and autosomal dominant was the predominant mode of inheritance in Korean patients. This study confirms that microcephaly is a condition with genotypically and phenotypically heterogeneous causes. Interdisciplinary cooperation between molecular geneticists and clinicians is important to facilitate the elucidation of the underlying causes of microcephaly.
Data Availability Statement
The datasets generated for this study can be found in the ClinVar: submission name as Korean microcephaly.
Ethics Statement
Written informed consent was obtained from the individuals or legal guardian for the publication of any potentially identifiable images or data included in this article.
Author Contributions
JeL, BK, and C-SK conceived and designed the study. JeL and JiL enrolled the participants and analyzed their clinical data. JP, CL, and AK analyzed the data of genetic evaluation. JiL and JP wrote the manuscript. WP and C-SK reviewed the analysis of genetic variants. JeL approved the submitted version of the manuscript. All authors contributed to the article and approved the submitted version.
Funding
This research was supported by the Basic Science Research Program of the National Research Foundation of Korea (NRF) funded by the Ministry of Science and ICT (NRF-2014M3C9A2064619 and NRF-2017R1A2B4005276). The funding body had no role in this study design, data collection, or analysis, decision to publish, or preparation of the manuscript.
Conflict of Interest
C-SK, who previously belonged to Sungkyunkwan University School of Medicine during the period when this study was performed, was employed by the company Green Cross Genome from 2019.
The remaining authors declare that the research was conducted in the absence of any commercial or financial relationships that could be construed as a potential conflict of interest.
Acknowledgments
We are grateful to the patients and their families for their participation in this study and we thank the research coordinator for collecting samples and processing genetic evaluations.
Supplementary Material
The Supplementary Material for this article can be found online at: https://www.frontiersin.org/articles/10.3389/fgene.2020.543528/full#supplementary-material
Supplementary Figure 1. Generation process of a candidate variant list using a four-step strategy.
Supplementary Table 1. List of genes included in silico gene panel for microcephaly.
Supplementary Table 2. Clinical phenotypes of all patients.
Supplementary Table 3. List of pathogenic variant, likely pathogenic variant, and variant of uncertain significance with in-silico analysis for microcephaly phenotype genes.
References
Abuelo, D. (2007). Microcephaly syndromes. Semin. Pediatr. Neurol. 14, 118–127. doi: 10.1016/j.spen.2007.07.003
Aftab, S., Semenec, L., Chu, J. S., and Chen, N. (2008). Identification and characterization of novel human tissue-specific RFX transcription factors. BMC Evol. Biol. 8:226. doi: 10.1186/1471-2148-8-226
Ansari, M., Poke, G., Ferry, Q., Williamson, K., Aldridge, R., Meynert, A. M., et al. (2014). Genetic heterogeneity in cornelia de lange syndrome (CdLS) and CdLS-like phenotypes with observed and predicted levels of mosaicism. J. Med. Genet. 51, 659–668. doi: 10.1136/jmedgenet-2014-102573
Arya, R., Spaeth, C., Gilbert, D. L., Leach, J. L., and Holland, K. D. (2017). GNAO1-associated epileptic encephalopathy and movement disorders: c.607G>A variant represents a probable mutation hotspot with a distinct phenotype. Epileptic Disord. 19, 67–75. doi: 10.1684/epd.2017.0888
Beunders, G., Voorhoeve, E., Golzio, C., Pardo, L. M., Rosenfeld, J. A., Talkowski, M. E., et al. (2013). Exonic deletions in AUTS2 cause a syndromic form of intellectual disability and suggest a critical role for the C terminus. Am. J. Hum. Genet. 92, 210–220. doi: 10.1016/j.ajhg.2012.12.011
Bonnet, C., Ali Khan, A., Bresso, E., Vigouroux, C., Beri, M., Lejczak, S., et al. (2013). Extended spectrum of MBD5 mutations in neurodevelopmental disorders. Eur. J. Hum. Genet. 21, 1457–1461. doi: 10.1038/ejhg.2013.22
Boonsawat, P., Joset, P., Steindl, K., Oneda, B., Gogoll, L., Azzarello-Burri, S., et al. (2019). Elucidation of the phenotypic spectrum and genetic landscape in primary and secondary microcephaly. Genet. Med. 21, 2043–2058. doi: 10.1038/s41436-019-0464-7
Cristofoli, F., Devriendt, K., Davis, E. E., van Esch, H., and Vermeesch, J. R. (2018). Novel CASK mutations in cases with syndromic microcephaly. Hum. Mutat. 39, 993–1001. doi: 10.1002/humu.23536
Darvish, H., Esmaeeli-Nieh, S., Monajemi, G. B., Mohseni, M., Ghasemi-Firouzabadi, S., Abedini, S. S., et al. (2010). A clinical and molecular genetic study of 112 Iranian families with primary microcephaly. J. Med. Genet. 47, 823–828. doi: 10.1136/jmg.2009.076398
Di Resta, C., and Ferrari, M. (2018). Next generation sequencing: from research area to clinical practice. EJIFCC 29, 215–220.
Farwell, K. D., Shahmirzadi, L., El-Khechen, D., Powis, Z., Chao, E. C., Tippin Davis, B., et al. (2015). Enhanced utility of family-centered diagnostic exome sequencing with inheritance model-based analysis: results from 500 unselected families with undiagnosed genetic conditions. Genet. Med. 17, 578–586. doi: 10.1038/gim.2014.154
Fujimori, S., Kamatani, N., Nishida, Y., Ogasawara, N., and Akaoka, I. (1990). Hypoxanthine guanine phosphoribosyltransferase deficiency: nucleotide substitution causing lesch-nyhan syndrome identified for the first time among Japanese. Hum. Genet. 84, 483–486. doi: 10.1007/bf00195826
Gibbs, R. A., and Caskey, C. T. (1987). Identification and localization of mutations at the lesch-nyhan locus by ribonuclease A cleavage. Science 236, 303–305. doi: 10.1126/science.3563511
Gilmore, E. C., and Walsh, C. A. (2013). Genetic causes of microcephaly and lessons for neuronal development. Wiley Interdiscip. Rev. Dev. Biol. 2, 461–478. doi: 10.1002/wdev.89
Hamdan, F. F., Srour, M., Capo-Chichi, J. M., Daoud, H., Nassif, C., Patry, L., et al. (2014). De novo mutations in moderate or severe intellectual disability. PLoS Genet. 10:e1004772. doi: 10.1371/journal.pgen.1004772
Hayashi, S., Okamoto, N., Chinen, Y., Takanashi, J., Makita, Y., Hata, A., et al. (2012). Novel intragenic duplications and mutations of CASK in patients with mental retardation and microcephaly with pontine and cerebellar hypoplasia (MICPCH). Hum. Genet. 131, 99–110. doi: 10.1007/s00439-011-1047-0
Hayashi, S., Uehara, D. T., Tanimoto, K., Mizuno, S., Chinen, Y., Fukumura, S., et al. (2017). Comprehensive investigation of CASK mutations and other genetic etiologies in 41 patients with intellectual disability and microcephaly with pontine and cerebellar hypoplasia (MICPCH). PLoS ONE 12:e0181791. doi: 10.1371/journal.pone.0181791
Hoyer, J., Ekici, A. B., Endele, S., Popp, B., Zweier, C., Wiesener, A., et al. (2012). Haploinsufficiency of ARID1B, a member of the SWI/SNF-a chromatin-remodeling complex, is a frequent cause of intellectual disability. Am. J. Hum. Genet. 90, 565–572. doi: 10.1016/j.ajhg.2012.02.007
Jang, W., Kim, Y., Han, E., Park, J., Chae, H., Kwon, A., et al. (2019). Chromosomal microarray analysis as a first-tier clinical diagnostic test in patients with developmental delay/intellectual disability, autism spectrum disorders, and multiple congenital anomalies: a prospective multicenter study in Korea. Ann. Lab. Med. 39, 299–310. doi: 10.3343/alm.2019.39.3.299
Jayaraman, D., Bae, B. I., and Walsh, C. A. (2018). The genetics of primary microcephaly. Annu. Rev. Genomics Hum. Genet. 19, 177–200. doi: 10.1146/annurev-genom-083117-021441
Jiao, Q., Sun, H., Zhang, H., Wang, R., Li, S., Sun, D., et al. (2019). The combination of whole-exome sequencing and copy number variation sequencing enables the diagnosis of rare neurological disorders. Clin. Genet. 96, 140–150. doi: 10.1111/cge.13548
Mahajani, S., Giacomini, C., Marinaro, F., de Pietri Tonelli, D., Contestabile, A., and Gasparini, L. (2017). Lamin B1 levels modulate differentiation into neurons during embryonic corticogenesis. Sci. Rep. 7:4897. doi: 10.1038/s41598-017-05078-6
Manojlovic, Z., Earwood, R., Kato, A., Stefanovic, B., and Kato, Y. (2014). RFX7 is required for the formation of cilia in the neural tube. Mech. Dev. 132, 28–37. doi: 10.1016/j.mod.2014.02.001
Marques Matos, C., Alonso, I., and Leao, M. (2019). Diagnostic yield of next-generation sequencing applied to neurological disorders. J. Clin. Neurosci. 67, 14–18. doi: 10.1016/j.jocn.2019.06.041
Najmabadi, H., Hu, H., Garshasbi, M., Zemojtel, T., Abedini, S. S., Chen, W., et al. (2011). Deep sequencing reveals 50 novel genes for recessive cognitive disorders. Nature 478, 57–63. doi: 10.1038/nature10423
Petrovski, S., Kury, S., Myers, C. T., Anyane-Yeboa, K., Cogne, B., Bialer, M., et al. (2016). Germline de novo mutations in GNB1 cause severe neurodevelopmental disability, hypotonia, and seizures. Am. J. Hum. Genet 98, 1001–1010. doi: 10.1016/j.ajhg.2016.03.011
Prodan Zitnik, I., Cerne, D., Mancini, I., Simi, L., Pazzagli, M., Di Resta, C., et al. (2018). Personalized laboratory medicine: a patient-centered future approach. Clin. Chem. Lab. Med. 56, 1981–1991. doi: 10.1515/cclm-2018-0181
Richards, S., Aziz, N., Bale, S., Bick, D., Das, S., Gastier-Foster, J., et al. (2015). Standards and guidelines for the interpretation of sequence variants: a joint consensus recommendation of the American college of medical genetics and genomics and the association for molecular pathology. Genet. Med. 17, 405–424. doi: 10.1038/gim.2015.30
Rump, P., Jazayeri, O., van Dijk-Bos, K. K., Johansson, L. F., van Essen, A. J., Verheij, J. B., et al. (2016). Whole-exome sequencing is a powerful approach for establishing the etiological diagnosis in patients with intellectual disability and microcephaly. BMC Med. Genomics 9:7. doi: 10.1186/s12920-016-0167-8
Sajid Hussain, M., Marriam Bakhtiar, S., Farooq, M., Anjum, I., Janzen, E., Reza Toliat, M., et al. (2013). Genetic heterogeneity in Pakistani microcephaly families. Clin. Genet. 83, 446–451. doi: 10.1111/j.1399-0004.2012.01932.x
Shaheen, R., Maddirevula, S., Ewida, N., Alsahli, S., Abdel-Salam, G. M. H., Zaki, M. S., et al. (2019). Genomic and phenotypic delineation of congenital microcephaly. Genet. Med. 21, 545–552. doi: 10.1038/s41436-018-0140-3
Shinawi, M., Liu, P., Kang, S. H., Shen, J., Belmont, J. W., Scott, D. A., et al. (2010). Recurrent reciprocal 16p11.2 rearrangements associated with global developmental delay, behavioural problems, dysmorphism, epilepsy, and abnormal head size. J. Med. Genet. 47, 332–341. doi: 10.1136/jmg.2009.073015
Shoukier, M., Klein, N., Auber, B., Wickert, J., Schroder, J., Zoll, B., et al. (2013). Array CGH in patients with developmental delay or intellectual disability: are there phenotypic clues to pathogenic copy number variants? Clin. Genet. 83, 53–65. doi: 10.1111/j.1399-0004.2012.01850.x
Stewart, D. R., and Kleefstra, T. (2007). The chromosome 9q subtelomere deletion syndrome. Am. J. Med. Genet. C Semin. Med. Genet. 145C, 383–392. doi: 10.1002/ajmg.c.30148
Takeuchi, T., Yamazaki, Y., Katoh-Fukui, Y., Tsuchiya, R., Kondo, S., Motoyama, J., et al. (1995). Gene trap capture of a novel mouse gene, jumonji, required for neural tube formation. Genes Dev. 9, 1211–1222. doi: 10.1101/gad.9.10.1211
Thevenon, J., Duffourd, Y., Masurel-Paulet, A., Lefebvre, M., Feillet, F., El Chehadeh-Djebbar, S., et al. (2016). Diagnostic odyssey in severe neurodevelopmental disorders: toward clinical whole-exome sequencing as a first-line diagnostic test. Clin. Genet. 89, 700–707. doi: 10.1111/cge.12732
Trinh, J., Kandaswamy, K. K., Werber, M., Weiss, M. E. R., Oprea, G., Kishore, S., et al. (2019). Novel pathogenic variants and multiple molecular diagnoses in neurodevelopmental disorders. J. Neurodev. Disord. 11:11. doi: 10.1186/s11689-019-9270-4
Tsoutsou, E., Tzetis, M., Giannikou, K., Braoudaki, M., Mitrakos, A., Amenta, S., et al. (2017). Application of high-resolution array comparative genomic hybridization in children with unknown syndromic microcephaly. Pediatr. Res. 82, 253–260. doi: 10.1038/pr.2017.65
Vargas, J. E., Allred, E. N., Leviton, A., and Holmes, L. B. (2001). Congenital microcephaly: phenotypic features in a consecutive sample of newborn infants. J. Pediatr. 139, 210–214. doi: 10.1067/mpd.2001.115314
Vissers, L., van Nimwegen, K. J. M., Schieving, J. H., Kamsteeg, E. J., Kleefstra, T., Yntema, H. G., et al. (2017). A clinical utility study of exome sequencing versus conventional genetic testing in pediatric neurology. Genet. Med. 19, 1055–1063. doi: 10.1038/gim.2017.1
von der Hagen, M., Pivarcsi, M., Liebe, J., von Bernuth, H., Didonato, N., Hennermann, J. B., et al. (2014). Diagnostic approach to microcephaly in childhood: a two-center study and review of the literature. Dev. Med. Child Neurol. 56, 732–741. doi: 10.1111/dmcn.12425
Wang, J., Ai, X., Gale, R. P., Xu, Z., Qin, T., Fang, L., et al. (2013). TET2, ASXL1 and EZH2 mutations in Chinese with myelodysplastic syndromes. Leuk. Res. 37, 305–311. doi: 10.1016/j.leukres.2012.10.004
Keywords: microcephaly, whole exome sequencing (WES), chromosomal microarray, low-depth whole genome sequencing, Korea
Citation: Lee J, Park JE, Lee C, Kim AR, Kim BJ, Park W-Y, Ki C-S and Lee J (2021) Genomic Analysis of Korean Patient With Microcephaly. Front. Genet. 11:543528. doi: 10.3389/fgene.2020.543528
Received: 17 March 2020; Accepted: 30 December 2020;
Published: 28 January 2021.
Edited by:
Prashant Kumar Verma, All India Institute of Medical Sciences, Rishikesh, IndiaReviewed by:
Chiara Di Resta, Vita-Salute San Raffaele University, ItalyByungChan Lim, Seoul National University Hospital, South Korea
Copyright © 2021 Lee, Park, Lee, Kim, Kim, Park, Ki and Lee. This is an open-access article distributed under the terms of the Creative Commons Attribution License (CC BY). The use, distribution or reproduction in other forums is permitted, provided the original author(s) and the copyright owner(s) are credited and that the original publication in this journal is cited, in accordance with accepted academic practice. No use, distribution or reproduction is permitted which does not comply with these terms.
*Correspondence: Jeehun Lee, amhsZWUwMTAxQHNra3UuZWR1
†These authors share first authorship