- 1Institute of Medicinal Plant Development (IMPLAD), Chinese Academy of Medical Sciences and Peking Union Medical College, Beijing, China
- 2School of Life Sciences and Engineering, Southwest University of Science and Technology, Mianyang, China
- 3Triticeae Research Institute, Sichuan Agricultural University, Chengdu, China
Radix Bupleuri (roots of Bupleurum spp.) is an important medicinal herb. Triterpenoid saponins of saikosaponins generally constitute the main class of secondary metabolites of plants in the Bupleurum genus. However, the molecular regulatory mechanism underlying their biosynthesis remains elusive. In this study, we observed significantly different saikosaponin biosynthesis between Bupleurum chinense and Bupleurum scorzonerifolium at the seedling stage. The sequential and expression characterization of 232 genes in the triterpenoid saponin biosynthetic pathway, which includes the mevalonate (MVA) pathway and methylerythritol phosphate (MEP) pathway, between B. chinense and B. scorzonerifolium was also investigated. Sixty of these genes may be involved in saikosaponin biosynthesis. Manipulation of these genes, especially those of the β-AS, P450, and UGT families, may improve saikosaponin production.
Introduction
Radix Bupleuri (roots of Bupleurum spp.) is one of the most important medicinal herbs in Eurasia and North Africa used as a treatment for fever, chronic hepatitis, nephrotic syndrome, inflammatory diseases, menstrual disorders, and digestive ulcers (Pistelli et al., 1996; Guo et al., 2000; Ikegami et al., 2006; Mabberley, 2008). In addition, this herb has been used for more than 2,000 years in China (Tan et al., 2008). In the Chinese Pharmacopoeia, the official botanical origin of Bupleuri Radix is the roots of Bupleurum chinense DC. or Bupleurum scorzonerifolium Willd. (Chinese Pharmacopoeia Commission, 2015). Several groups of secondary metabolites have been isolated from Bupleurum species, including triterpenoid saponins (saikosaponins), steroidal saponins, lignans, essential oils, and polysaccharides (Ashour and Wink, 2011). Among these, saikosaponins generally represent the main class of secondary metabolites and constitute to up to 7% of the total dry weight of roots of plants in the Bupleurum genus (Ashour and Wink, 2011). Owing to their wide range of pharmacological activities, including their immunomodulatory activity, anti-inflammatory activity, antioxidant and hepatoprotective activity, cytotoxicity, antitumor activity, and antiviral activity, these triterpenoid saponins, especially saikosaponins a and d, are the most important pharmacological constituent in Bupleurum root extracts (Zhao and Xiao, 2007; Lin et al., 2013; Liang et al., 2014).
To date, more than 130 glycosylated oleanane-type and ursane-type saponins have been isolated from the genus Bupleurum L. (Ashour and Wink, 2011; Wang et al., 2017b; Chelghoum et al., 2018). A previous study on the species B. chinense, B. scorzonerifolium, and Bupleurum falcatum L. demonstrated that saikosaponins are mainly distributed in the tissues of the cork and cortex of roots (Liang et al., 2014). Similar results were reported based on histochemical studies (Tan et al., 2008). In addition, the synthesis and accumulation of saikosaponins is strongly influenced by intrinsic factors, including growth stage, developmental phase, and root structure, and by environmental conditions, such as drought, fertility, and light deficiency (Tan et al., 2008; Zhu et al., 2009a,b; Gong et al., 2017). The combination of cultural practices together with manipulation of the expression of genes involved in triterpenoid saponin biosynthesis may be a more effective way to improve the total yield of saikosaponins.
In the biosynthetic pathway of triterpenoid saponins in higher plants, the mevalonate (MVA) pathway in the cytosol and the methylerythritol phosphate (MEP) pathway in the plastids are essential biosynthetic processes for formation of the triterpenoid backbone of the five-carbon intermediates isopentenyl diphosphate (IPP) and dimethylallyl diphosphate (DMAPP). In transcriptome studies, genes involved in the biosynthetic pathway of saikosaponins were identified in Bupleurum kaoi, B. chinense, and B. scorzonerifolium (Chen et al., 2007; Sui et al., 2011; Sui et al., 2015). Moreover, genes involved in the biosynthesis of saikosaponins such as squalene epoxidase, β-amylase, cytochrome P450, and uridine diphosphate glycosyl transferases have been cloned in B. kaoi, B. falcatum, and B. chinense, and their expression profiles have been identified (Lin et al., 2006; Kim et al., 2011; Gao et al., 2016). Overexpression of the BcbZIP134 gene in B. chinense and BfSS1 in B. falcatum has been reported (Kim et al., 2011; Xu et al., 2019). Additionally, cytochrome P450 monooxygenase (P450) of CYP716Y1 from B. falcatum was combined with oxidosqualene cyclase, P450, and glycosyltransferase genes to construct a synthetic biological platform for the production of bioactive triterpene sapo(ge)nins in yeast (Moses et al., 2014). However, the molecular regulatory mechanism underlying triterpenoid saponin biosynthesis remains elusive.
In this study, we investigated the sequential and expression characterization of 232 genes in the MVA pathway and MEP pathway in B. chinense and B. scorzonerifolium to identify putative genes involved in the biosynthesis of saikosaponins in Bupleurum L.
Materials and Methods
Plant Materials
The two experimental materials, the commercial varieties Chuanbeichai No. 1 (CBC1) and Chuanhongchai No. 1 (CHC1), which are varieties of B. chinense and B. scorzonerifolium, respectively, were used. All of the plants were bred via systemic selection and purifying selection from farmholding populations by Dr. Jianhe Wei from the Institute of Medicinal Plant Development (IMPLAD), Chinese Academy of Medical Sciences and Peking Union Medical College, and Dr. Da-bin Hou from Southwest University of Science and Technology.
For each genotype, seeds were placed on moist filter paper before germination. Germinated seedlings were then grown in modified Hoagland’s nutrient solution. Five-day-old and 15-day-old CBC1 and CHC1 plants were utilized for isoform sequencing (iso-seq) analysis, transcriptome analysis, and saikosaponin a (SS a) and d (SS d) content assays. For 5-day-old seedlings, whole fresh roots were harvested (S1). For 15-day-old seedlings, 5 mm of the root tip without the region of differentiation (S2) and with the region of differentiation (S3) were harvested separately.
Extraction of Saikosaponins and HPLC Analysis
Samples S1, S2, and S3 (three replications each) were dried for 72 h using a freeze-drier (LGJ-18, Beijing Songyuan Huaxing Technology Development Co., Ltd., China). The SS a and SS d content was determined using a Waters HPLC (high-performance liquid chromatography) system (Waters 1525 Binary HPLC Pump, United States) and an ASB-vensil C18 column (250 mm × 4.6 mm, 5 μm). Reference standards of SS a and SS d were purchased from the National Institutes for Food and Drug Control, Beijing, China. The methods and conditions for determination have been reported previously (Xu et al., 2019).
Iso-seq and Transcriptome Analyses
The leaf and root samples were mixed and utilized for iso-seq library construction. The iso-seq analysis followed the method published by Wang et al. (2017a). Transcriptome analyses of samples S1, S2, and S3 were performed on an Illumina HiSeq 2500 platform (Illumina, San Diego, CA, United States) as previously described (Yang et al., 2018). Both iso-seq and transcriptome analyses were performed at the Novogene Bioinformatics Institute (Novogene, Beijing, China). Three replications were included in this study.
Candidate Gene Selection
Gene sequences of 20 families involved in the MVA pathway and MEP pathway were selected from iso-seq and transcriptome data with annotated gene names. These genes include glycosyltransferases (UGT), P450, β-amyrin synthase (β-AS), squalene synthase (SS), squalene epoxidase (SE), farnesyl diphosphate synthase (FPS), mevalonate-5-pyrophosphate decarboxylase (MVD), phosphomevalonate kinase (PMK), mevalonate kinase (MK), 3-hydroxy-3-methylglutaryl-CoA reductase (HMGCR), 3-hydroxy-3-methylglutaryl-CoA synthase (HMGS), acetyl-CoA C-acetyltransferase (AACT), 1-deoxy-D-xylulose-5-phosphate synthase (DXS), 1-deoxy-D- xylulose-5-phosphate reductoisomerase (DXR), 2-C-methyl-D-erythritol 4-phosphate cytidylyl transferase (CMS), 4-(cytidine 5′-diphospho)-2-C-methyl-D-erythritol kinase (CMK), 2-C-methyl-D-erythritol-2,4-cyclodiphosphate synthase (MCS), 4-hydroxy-3-methyl but-2-(E)-enyl diphosphate synthase (HDS), 4-hydroxy-3-methyl but-2-(E)-enyl diphosphate reductase (IDS), isopentenyl diphosphate isomerase (IDI), and geranyl diphosphate synthase (GPS). CAP3 software was used to identify overlaps between different sequences and to remove redundant fragments1. The conserved domains of each family were analyzed by using SMART online software2 and used as a query to search the NCBI non-redundant protein database via BLASTX to further validate the potential candidate genes3. The isoelectric points (PIs) and molecular weights (MWs) of deduced proteins were calculated using the ExPASy Compute pI/Mw tool4, and the subcellular localizations were predicted using Cell-PLoc 2.05. Differential expression analyses were performed by the DESeq2 R package (Anders and Huber, 2010) to identify differentially expressed genes (DEGs) among S1, S2, and S3 within each species and between the two species. The differentially expressed unigenes were further filtered based on their count number (at least one stage was greater than 1) and the log2(fold change) [the log2(fold change) between two stages was greater than 2].
Results
SS a and SS d were detected in the roots of B. chinense plants at the 1-day-old stage, whereas they significantly accumulated in the region of differentiation at the 15-day-old stage (Table 1). In B. scorzonerifolium, no peaks of SS a and SS d were identified in any of the samples during the HPLC analysis. Therefore, the genes showed significantly different expression in S3 in B. chinense, but insignificant or the opposite expression in B. scorzonerifolium would be interesting.
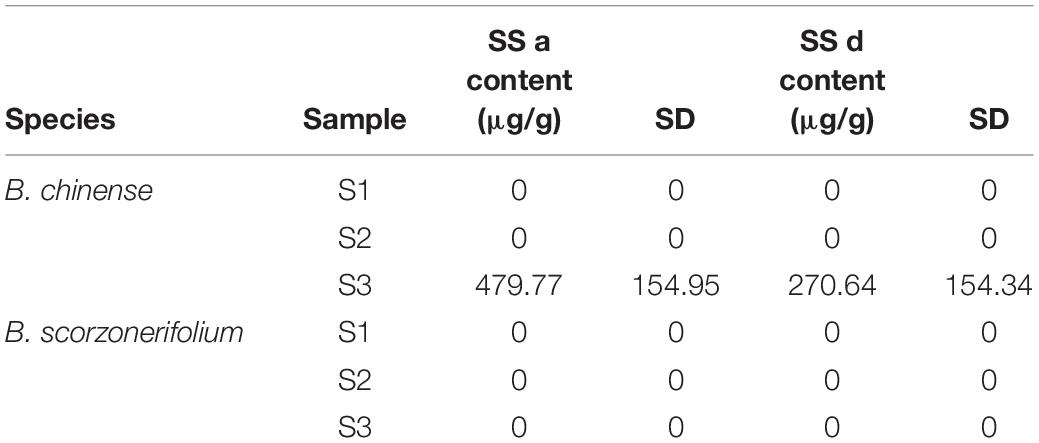
Table 1. Contents of saikosaponin a and saikosaponin d in the roots of B. chinense and B. scorzonerifolium.
A total of 223 genes with complete open reading frames (ORFs) were identified from the transcriptome database of B. chinense and B. scorzonerifolium (Supplementary Table S1). One to 96 genes were identified for a single gene family whose members are involved in triterpenoid saponin biosynthesis, although the MCS family had zero genes. In the differential expression analysis of B. chinense, 86 genes showed significantly different expression in the S3 sample (Figures 1, 2). Among these genes, 1 to 36 genes were identified for gene families including UGT, P450, β-AS, SS, FPS, GPS, PMK, HMGCR, HMGS, AACT, IDS, HDS, CMS, DXR, and DXS. No gene with significantly different expression was detected for SE, MVD, IDI, MK, and CMK. Five genes involved the gene families β-AS, SS, CMS, HDS, and IDS; these genes exhibited significantly similar expression in B. scorzonerifolium. Seven genes in the FPS, HMGCR, DXS, and P450 gene families showed significantly reversed expression. The BcDXS15975 gene in the DXS family was identified only in B. chinense and was not detected in any of the samples of B. scorzonerifolium.
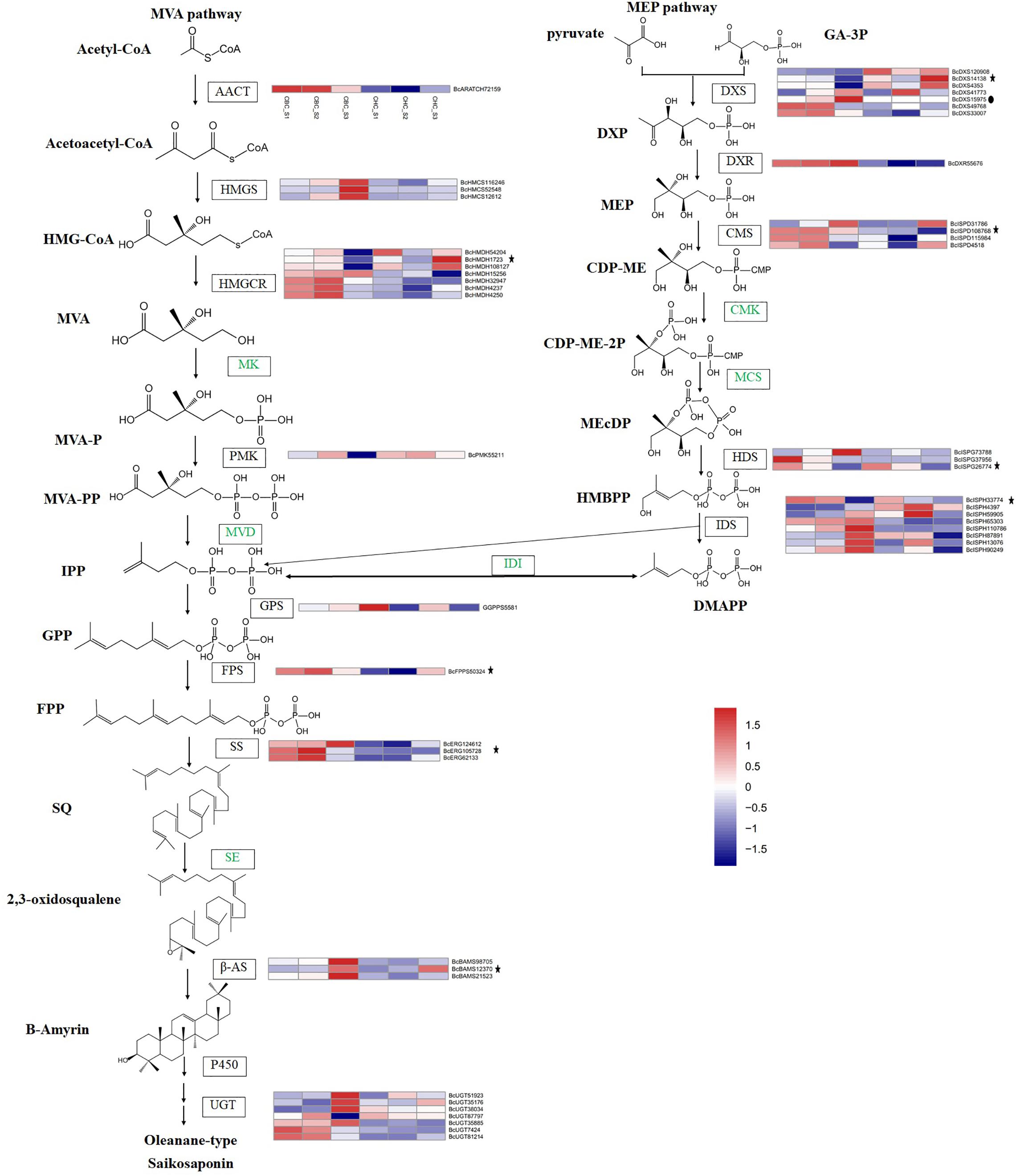
Figure 1. Putative triterpenoid backbone biosynthesis pathway (MVA and MEP) in Bupleurum chinense DC. and Bupleurum scorzonerifolium Willd. and gene expression profiles of the key enzymes. All genes outside of these pathways showed significantly differential expression in S3 of B. chinense. The genes with asterisks also showed significantly differential expression in S3 of B. scorzonerifolium. The genes with solid dots indicate those detected only in B. chinense DC. The samples include S1 (F), S2 (T), and S3 (M) in B. chinense (CBC) and B. scorzonerifolium (CHC).
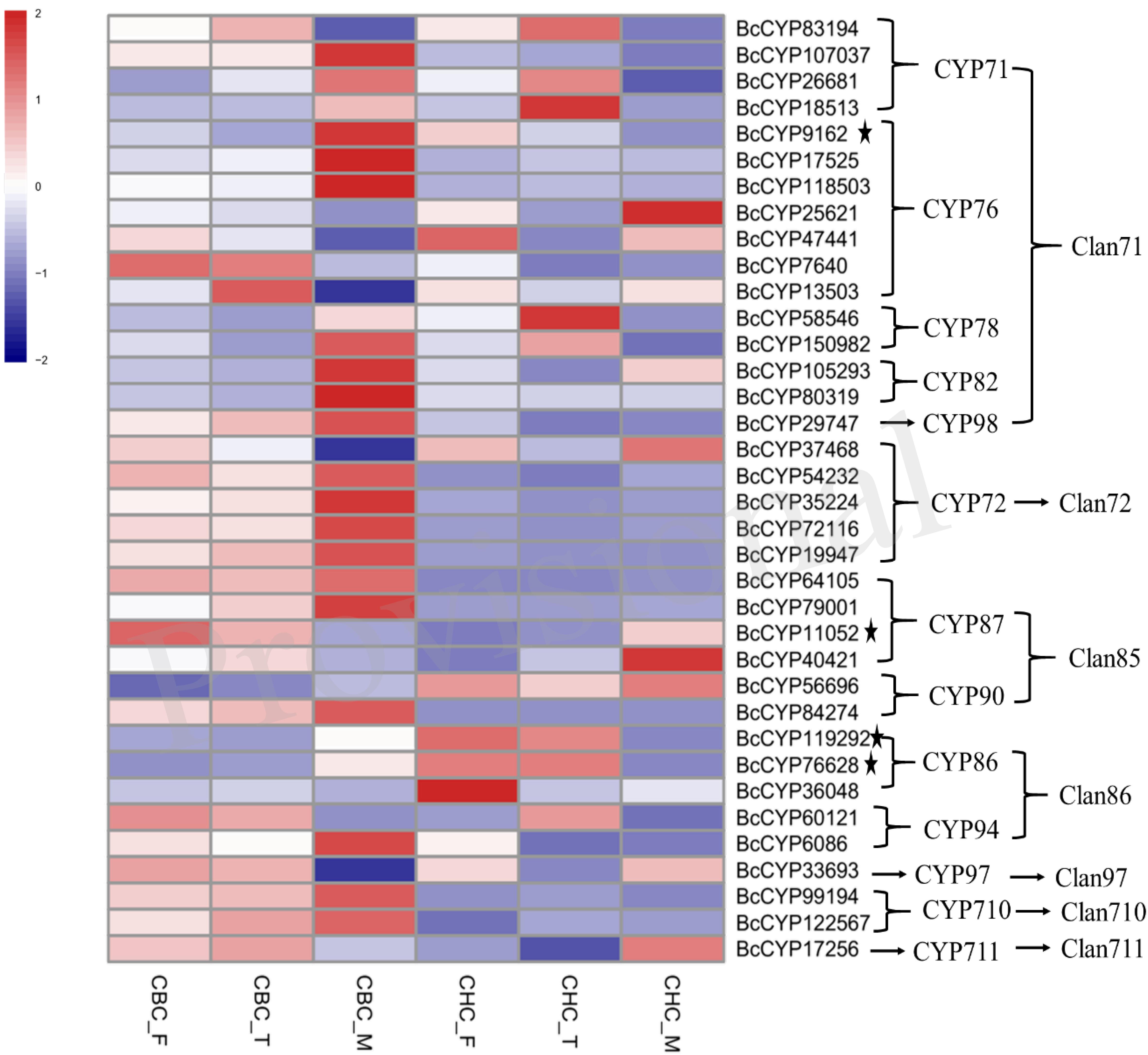
Figure 2. Gene expression profiles of candidate P450s involved in the biosynthesis of triterpenoid saponins. All these genes showed significantly differential expression in S3 of Bupleurum chinense DC. The genes with asterisks also showed significantly differential expression in S3 of Bupleurum scorzonerifolium Willd. The sample information is listed in Figure 1.
Discussion
Triterpenoids are derived from C5 isoprene units of IPP and DMAPP through a “head-to-tail” connection (Hillier and Lathe, 2019). Both of these triterpenoid backbones can be synthesized through the MVA pathway in the cytoplasm or the MEP pathway in the plastids. For the MVA pathway, three HMGS genes and one HMGCR gene (BcHMDH15256) showed significantly upregulated expression in S3 of the B. chinense transcriptome, whereas one AACT gene, seven HMGCR genes, and one PMK gene showed significantly downregulated expression. For these genes, the expression differences among S1, S2, and S3 were insignificant in B. scorzonerifolium, with the exception of the BcHMDH1723 gene (Figure 1). In addition, BcHMDH1723 showed the opposite expression trend between B. chinense and B. scorzonerifolium. We did not detect genes with significantly different expression in the MK family or the MVD family.
For the MEP pathway, 12 unigenes encoding enzymes (five DXS genes, three CMS genes, two HDS genes, and two IDS genes) showed significantly downregulated expression in S3 of the B. chinense transcriptome, whereas the expression of two DXS genes, one DXR gene, one CMS gene, one HDS gene, and six IDS genes significantly increased. The CMS gene BcISPD108768, the HDS gene BcISPG26774, and the IDS gene BcISPH33774 showed similar trends in the B. scorzonerifolium transcriptome, whereas the DXS gene BcDXS14138 showed the opposite trend. The DXS gene BcDXS15975 was detected only in the B. chinense transcriptome.
Both the MVA and MEP pathways produce the C5 unit IPP, which can be transformed into its isomer DMAPP by IDI (Xue et al., 2019). In the present study, we did not find that the IDI gene showed significant expression differences among S1, S2, and S3. IPP and DMAPP are assembled into GPP and FPP by GPS and FPS prenyltransferases, respectively. Expression of the GPS gene BcGGPPS5581 increased in S3 of the B. chinense transcriptome, and expression of the FPS gene BcFPPS50324 decreased. SS catalyzes the joining of two units of FPP in a “tail-to-tail” fashion of SQ. Kim et al. (2011) found that overexpression of BfSS1 in B. falcatum more powerfully regulates downstream genes than does MeJA treatment in triterpene and phytosterol biosynthesis. In the present study, three candidate genes in the SS family showed significant expression differences in S3 of the B. chinense transcriptome, and the expression of all these candidate genes among the three samples in the B. scorzonerifolium transcriptome was not significant. SQ is oxidized by SE to give rise to 2,3-oxidosqualene. We cloned the full-length SE gene in B. chinense (Gao et al., 2016). However, no candidate gene was identified in the differential expression analysis.
In Bupleurum, the first committed step in the synthesis of triterpenoid saponins involves the cyclization of 2,3-oxidosqualene by β-AS. Therefore, β-AS is presumed to be the enzyme that catalyzes the first committed step in saikosaponin biosynthesis. A previous study indicated that β-AS in B. kaoi and B. chinense exhibits tissue-specific responses to both MeJA and PEG (Lin et al., 2013; Zhang et al., 2016). We have also cloned the promoter of β-AS in B. chinense and evaluated its activity previously (Gao et al., 2015). We found three β-AS genes (BcBAMS98705, BcBAMS12370, and BcBAMS21523) with significantly increased expression in S3 of B. chinense. The BcBAMS12370 gene showed a similar expression trend in B. scorzonerifolium, whereas the expression of the other two genes was non-significant.
For the production of saikosaponins in Bupleurum, P450 enzymes catalyze the oxidation of β-amyrin to form 13,28-epoxy and the C11/C12 double-bond structure or two double bonds at C11/C12 and C13/C18 together with hydroxylation at C16 and C23. Previous studies have indicated that the CYP90, CYP72, CYP710, and CYP711 subfamilies of the P450 family may involve triterpenoid-metabolizing enzymes (Ohnishi et al., 2006; Hamberger and Bak, 2013; Yu et al., 2017). We found that 37 P450 genes showed significant expression in the three samples of B. chinense, and four of these genes exhibited a significant opposite trend in B. scorzonerifolium (Figure 2). These genes are members of 13 families of 7 clans in the P450 family. Among these genes, two unigenes belong to the CYP90 family, five unigenes belong to the CYP72 family, two unigenes belong to the CYP710 family, and the unigene BcCYP17256 belongs to the CYP711 family. Members of the CYP716 family contribute to the diversification of eudicot triterpenoid biosynthesis (Miettinen et al., 2017). Moses et al. (2014) found that the CYP716Y1 gene from B. falcatum catalyzes the C-16α hydroxylation of triterpenes in yeast. However, we did not identify any CYP716 gene that showed significantly different expression in S3 of B. chinense.
At the last step of saikosaponin biosynthesis, UGT catalyzes the glycosylation of hydroxylated β-amyrin at C3. Glycosylation contributes to the highly diverse nature of terpenoids in plants (Field and Osbourn, 2008). Therefore, UGT is presumed to be the key enzyme involved in the modification of saikosaponins. A transcriptome analysis of B. chinense revealed that, among 196 UGT genes, three were the most likely candidates involved in saikosaponin biosynthesis (Sui et al., 2011). Three BkUGT85A proteins that contain a highly conserved region of a motif of glycosyltransferases, which are involved in the production of secondary metabolites in plants, were identified in B. kaoi by Lin et al. (2013). In the present study, four UGT genes showed significantly increased expression in S3 of B. chinense, whereas four genes showed the opposite expression trend. The expression differences among all genes in B. scorzonerifolium were non-significant.
Conclusion
In this study, unigenes showed significantly different expression in the S3 sample in B. chinense, while insignificant or the opposite expression in B. scorzonerifolium may be involved in saikosaponin biosynthesis. Manipulation of these genes, especially those of the β-AS, P450, and UGT families, may improve saikosaponin production. The combined expression of these genes and reconstituting the synthesis of monoglycosylated saponins in yeast may provide a platform for the production of bioactive saikosaponins.
Data Availability Statement
The datasets generated for this study can be found in NCBI PRJNA645610 for B. chinense (SRR12223465-SRR12223473) and PRJNA662700 for B. scorzonerifolium (SRR12647620-SRR12647628).
Author Contributions
MY wrote the manuscript. HC performed the data analysis. S-HL seeded Chuanbeichai No.1 and Chuanhongchai No.1. Y-CL performed the HPLC analysis. CS performed the transcriptome analysis. J-HW bred out the commercial varieties of Chuanbeichai No.1 and Chuanhongchai No.1. J-HW and D-BH funded the whole project and helped MY to complete the manuscript. All authors contributed to the article and approved the submitted version.
Funding
This work was supported by the National Natural Science Foundation of China under grant number 81603223, the China Agriculture Research System under grant number CARS-21, the CAMS Innovation Fund for Medical Sciences (CIFMS) (2016-I2M-2-003), the National Transgenic Major Project of China (2019ZX08010004-005), the Natural Science Foundation of Science and Technology Department of Sichuan Province (2019YJ0443 and 2019YFH0072), and Young Elite Scientists Sponsorship Program by CACM (2019-QNRC2-C15).
Conflict of Interest
The authors declare that the research was conducted in the absence of any commercial or financial relationships that could be construed as a potential conflict of interest.
Supplementary Material
The Supplementary Material for this article can be found online at: https://www.frontiersin.org/articles/10.3389/fgene.2020.583245/full#supplementary-material
Footnotes
- ^ http://doua.prabi.fr/software/cap3
- ^ http://smart.embl-heidelberg.de/
- ^ http://www.ncbi.nlm.nih.gov/
- ^ http://web.expasy.org/compute_pi/
- ^ http://www.csbio.sjtu.edu.cn/bioinf/Cell-PLoc-2/
References
Anders, S., and Huber, W. (2010). Differential expression analysis for sequence count data. Genome Biol. 11:R106. doi: 10.1186/gb-2010-11-10-r106
Ashour, M. L., and Wink, M. (2011). Genus : a review of its phytochemistry, pharmacology and modes of action. J. Pharm. Pharmacol. 63, 305–321. doi: 10.1111/j.2042-7158.2010.01170.x
Chelghoum, M., Smati, D., Mitaine-Offer, A.-C., Paululat, T., and Lacaille-Dubois, M.-A. (2018). Four new triterpene saponins from Bupleurum rigidum L. Phytochem. Lett. 27, 223–228. doi: 10.1016/j.phytol.2018.07.025
Chen, L.-R., Chen, Y.-J., Lee, C.-Y., and Lin, T.-Y. (2007). MeJA-induced transcriptional changes in adventitious roots of Bupleurum kaoi. Plant Sci. 173, 12–24. doi: 10.1016/j.plantsci.2007.03.013
Chinese Pharmacopoeia Commission (2015). The Pharmacopoeia of the People’s Republic of China, 2015 Edition Part I. Beijing: China Medical Science Press.
Field, B., and Osbourn, A. E. (2008). Metabolic diversification—independent assembly of operon-like gene clusters in different plants. Science 320, 543–547. doi: 10.1126/science.1154990
Gao, K., Wu, S.-R., Wang, L., Xu, Y.-H., Wei, J.-H., and Sui, C. (2015). Cloning and analysis of β-amyrin synthase gene in Bupleurum chinense. Genes Genom. 37, 767–774. doi: 10.1007/s13258-015-0307-0
Gao, K., Xu, J.-S., Sun, J., Xu, Y.-H., Wei, J.-H., and Sui, C. (2016). Molecular cloning and expression of squalene epoxidase from a medicinal plant. Bupleurum chinense. Chinese Herb. Med. 8, 67–74. doi: 10.1016/s1674-6384(16)60010-2
Gong, J., Liu, M., Xu, S., Jiang, Y., Pan, Y., Zhai, Z., et al. (2017). Effects of light deficiency on the accumulation of saikosaponins and the ecophysiological characteristics of wild Bupleurum chinense DC. in China. Ind. Crops Products 99, 179–188. doi: 10.1016/j.indcrop.2017.01.040
Guo, Y., Matsumoto, T., Kikuchi, Y., Ikejima, T., Wang, B., and Yamada, H. (2000). Effects of a pectic polysaccharide from a medicinal herb, the roots of Bupleurum falcatum L. on interleukin 6 production of murine B cells and B cell lines. Immunopharmacology 49, 307–316. doi: 10.1016/s0162-3109(00)00245-9
Hamberger, B., and Bak, S. (2013). Plant P450s as versatile drivers for evolution of species-specific chemical diversity. Philos. Trans. R. Soc. B-Biol. Sci. 368:20120426. doi: 10.1098/rstb.2012.0426
Hillier, S. G., and Lathe, R. (2019). Terpenes, hormones and life: isoprene rule revisited. J. Endocrinol. 242, R9–R22. doi: 10.1530/JOE-19-0084
Ikegami, F., Sumino, M., Fujii, Y., Akiba, T., and Satoh, T. (2006). Pharmacology and toxicology of Bupleurum root-containing Kampo medicines in clinical use. Hum. Exp. Toxicol. 25, 481–494. doi: 10.1191/0960327106het654oa
Kim, Y. S., Cho, J. H., Park, S., Han, J.-Y., Back, K., and Choi, Y.-E. (2011). Gene regulation patterns in triterpene biosynthetic pathway driven by overexpression of squalene synthase and methyl jasmonate elicitation in Bupleurum falcatum. Planta 233, 343–355. doi: 10.1007/s00425-010-1292-9
Liang, Z., Oh, K., Wang, Y., Yi, T., Chen, H., and Zhao, Z. (2014). Cell type-specific qualitative and quantitative analysis of saikosaponins in three Bupleurum species using laser microdissection and liquid chromatography–quadrupole/time of flight-mass spectrometry. J. Pharm. Biomed. Anal. 97, 157–165. doi: 10.1016/j.jpba.2014.04.033
Lin, T.-Y., Chiou, C.-Y., and Chiou, S.-J. (2013). Putative genes involved in saikosaponin biosynthesis in Bupleurum species. Int. J. Mol. Sci. 14, 12806–12826. doi: 10.3390/ijms140612806
Lin, W.-Y., Peng, P.-H., and Lin, T.-Y. (2006). “Cloning and characterization of beta-amyrin synthase from Bupleurum kaoi,” in Proceedings of the 8th International Congress of Plant Molecular Biology, (Adelaide, SA: Book of Abstracts, ISPMB).
Mabberley, D. J. (2008). [Plant-book]; Mabberley’s plant-book: a portable dictionary of plants, their classifications and uses; utilizing Kubitzki’s” The families and genera of vascular plants” (1990-) and current botanical literature; arranged according to the principles of molecular systematics. Cambridge: Cambridge University Press.
Miettinen, K., Pollier, J., Buyst, D., Arendt, P., Csuk, R., Sommerwerk, S., et al. (2017). The ancient CYP716 family is a major contributor to the diversification of eudicot triterpenoid biosynthesis. Nat. Commun. 8, 1–13. doi: 10.1038/ncomms14153
Moses, T., Pollier, J., Almagro, L., Buyst, D., Van Montagu, M., Pedreño, M. A., et al. (2014). Combinatorial biosynthesis of sapogenins and saponins in Saccharomyces cerevisiae using a C-16α hydroxylase from Bupleurum falcatum. Proc. Natl. Acad. Sci. U.S.A. 111, 1634–1639. doi: 10.1073/pnas.1323369111
Ohnishi, T., Szatmari, A.-M., Watanabe, B., Fujita, S., Bancos, S., Koncz, C., et al. (2006). C-23 hydroxylation by Arabidopsis CYP90C1 and CYP90D1 reveals a novel shortcut in brassinosteroid biosynthesis. Plant cell 18, 3275–3288. doi: 10.1105/tpc.106.045443
Pistelli, L., Bertoli, A., Bilia, A. R., and Morelli, I. (1996). Minor constituents from Bupleurum fruticosum roots. Phytochemistry 41, 1579–1582. doi: 10.1016/0031-9422(95)00749-0
Sui, C., Chen, M., Xu, J., Wei, J., Jin, Y., Xu, Y., et al. (2015). Comparison of root transcriptomes and expressions of genes involved in main medicinal secondary metabolites from Bupleurum chinense and Bupleurum scorzonerifolium, the two Chinese official Radix bupleuri source species. Physiol. Plant. 153, 230–242. doi: 10.1111/ppl.12254
Sui, C., Zhang, J., Wei, J., Chen, S., Li, Y., Xu, J., et al. (2011). Transcriptome analysis of Bupleurum chinense focusing on genes involved in the biosynthesis of saikosaponins. BMC Genom. 12:539. doi: 10.1186/1471-2164-12-539
Tan, L. L., Cai, X., Hu, Z. H., and Ni, X. L. (2008). Localization and dynamic change of saikosaponin in root of Bupleurum chinense. J. Int. Plant Biol. 50, 951–957. doi: 10.1111/j.1744-7909.2008.00668.x
Wang, T., Wang, H., Cai, D., Gao, Y., Zhang, H., Wang, Y., et al. (2017a). Comprehensive profiling of rhizome-associated alternative splicing and alternative polyadenylation in moso bamboo (Phyllostachys edulis). Plant J. 91, 684–699. doi: 10.1111/tpj.13597
Wang, Y., Guo, Q., Cheng, Z., Zeng, K., Liang, H., Tu, P., et al. (2017b). New saikosaponins from the roots of Bupleurum chinense. Phytochem. Lett. 21, 183–189. doi: 10.1016/j.phytol.2017.06.005
Xu, J., Wu, S.-R., Xu, Y.-H., Ge, Z.-Y., Sui, C., and Wei, J.-H. (2019). Overexpression of BcbZIP134 negatively regulates the biosynthesis of saikosaponins. Plant Cell Tissue Organ Culture (PCTOC) 137, 297–308. doi: 10.1007/s11240-019-01571-0
Xue, L., He, Z., Bi, X., Xu, W., Wei, T., Wu, S., et al. (2019). Transcriptomic profiling reveals MEP pathway contributing to ginsenoside biosynthesis in Panax ginseng. BMC Genom. 20:383. doi: 10.1186/s12864-019-5718-x
Yang, L., Jin, Y., Huang, W., Sun, Q., Liu, F., and Huang, X. (2018). Full-length transcriptome sequences of ephemeral plant Arabidopsis pumila provides insight into gene expression dynamics during continuous salt stress. BMC Genom. 19:717. doi: 10.1186/s12864-018-5106-y
Yu, J., Tehrim, S., Wang, L., Dossa, K., Zhang, X., Ke, T., et al. (2017). Evolutionary history and functional divergence of the cytochrome P450 gene superfamily between Arabidopsis thaliana and Brassica species uncover effects of whole genome and tandem duplications. Bmc Genom. 18:733. doi: 10.1186/s12864-017-4094-7
Zhang, Y., Zhou, Z., Xia, P., Liang, Z., Liu, S., and Liu, Z. (2016). Expression of key enzyme genes and content of saikosaponin in saikosaponin biosynthesis under drought stress in Bupleurum chinense. China J. Chinese Mater. Med. 41, 643–647.
Zhao, Z., and Xiao, P. (2007). Encyclopedia on Contemporary Medicinal Plants. Shang Hai: World Publishing Corporation.
Zhu, Z., Liang, Z., and Han, R. (2009a). Growth and saikosaponin production of the medicinal herb Bupleurum chinense DC. under different levels of nitrogen and phosphorus. Ind. Crops Products 29, 96–101. doi: 10.1016/j.indcrop.2008.04.010
Keywords: Bupleurum, saikosaponin biosynthesis, transcriptome analysis, gene expression, P450
Citation: Yu M, Chen H, Liu S-H, Li Y-C, Sui C, Hou D-B and Wei J-H (2020) Differential Expression of Genes Involved in Saikosaponin Biosynthesis Between Bupleurum chinense DC. and Bupleurum scorzonerifolium Willd. Front. Genet. 11:583245. doi: 10.3389/fgene.2020.583245
Received: 14 July 2020; Accepted: 16 September 2020;
Published: 16 October 2020.
Edited by:
Penghao Wang, Murdoch University, AustraliaReviewed by:
Feng Wang, University of Helsinki, FinlandJunfeng Chen, Shanghai University of Traditional Chinese Medicine, China
Copyright © 2020 Yu, Chen, Liu, Li, Sui, Hou and Wei. This is an open-access article distributed under the terms of the Creative Commons Attribution License (CC BY). The use, distribution or reproduction in other forums is permitted, provided the original author(s) and the copyright owner(s) are credited and that the original publication in this journal is cited, in accordance with accepted academic practice. No use, distribution or reproduction is permitted which does not comply with these terms.
*Correspondence: Jian-He Wei, amh3ZWlAaW1wbGFkLmFjLmNu; Da-Bin Hou, eXV3ZW4wMDczQDEyNi5jb20=