- 1College of Horticulture Science and Engineering, Shandong Agricultural University, Tai’an, China
- 2Collaborative Innovation Center of Fruit & Vegetable Quality and Efficient Production in Shandong, Tai’an, China
- 3Key Laboratory of Biology of Horticultural Crops in Huanghuai Region, Ministry of Agriculture and Rural Affairs, Tai’an, China
- 4State Key Laboratory of Crop Biology, Tai’an, China
- 5School of Environment, Tsinghua University, Qingdao, China
Quantitative real-time PCR (qRT-PCR) is widely used in the detection of gene expression level. However, there is no suitable ginger reference gene for qPCR analysis. Therefore, it is the primary task to select and validate the appropriate ginger reference gene to normalize the expression of target genes. In this study, 14 candidate reference genes were selected and analyzed in different tissues (leaf, and rhizome), different development stages, different varieties, and abiotic stress (ABA and salt stress). Expression stability was calculated using geNorm and NormFinder, Bestkeeper, and RefFinder. For abiotic stress and total conditions, 28S and COX were identified as the most stable genes. In addition, RPII was the most stable in the different development stages and different varieties. TEF2 and RPL2 were the least stably expressed in the tissue and all the conditions. In order to verify the feasibility of these genes as reference genes, we used the most stable and least stable reference genes to normalize the expression levels of ZoSPS genes under different conditions. This work can provide theoretical support for future research on ginger gene expression.
Introduction
Gene expression analysis is an important means to reveal plant signal transduction, metabolic pathways, tissue development, and plant stress response (Crisp et al., 2017; Guo et al., 2017; Zachgo et al., 2018). Quantitative real-time PCR (qRT-PCR) is currently the main method for studying gene expression, because of its high sensitivity, strong specificity, and accurate quantification. However, this technique requires stable expression of endogenous genes as expression correction (Liang et al., 2018; Su et al., 2019). Therefore, choosing a suitable reference gene plays an important role in avoiding errors in experimental operations.
A good reference gene should be stably expressed in tissues and cells, and its expression level is not influenced by internal and external conditions (Kozera and Rapacz, 2013). Ribosomal RNAs, actin, ubiquitin, elongation factor-1α, tubulin, etc., are often used as reference genes. However, the stability of these commonly used reference genes varies in different species and conditions (Tian et al., 2015; Zhang et al., 2015). Moreover, due to the limitation of the genome of non-model plants, the reference genes reported in model plants were often selected (Li H. et al., 2018); however, its stability as a housekeeper gene needs to be further explored. If an inappropriate reference gene was selected, the target gene expression will be misdescribed (Petriccione et al., 2015). Therefore, we should choose a suitable reference gene according to actual conditions.
Ginger is widely cultivated in China. It has important uses in daily diet, industrial product production, traditional Chinese medicine, and so on (Wang et al., 2015; Marx et al., 2017; Shukla et al., 2019). However, there is a lack of research on ginger in the world, mainly due to the limitation of genome and the weak foundation of molecular biology. Fortunately, the development of transcriptome technology has enabled ginger to develop at the gene level. However, there is no report on the selection of ginger reference genes, so it is urgent to find a reference gene suitable for ginger gene expression analysis.
In this study, 14 candidate genes [RNA polymerase-II (RPII), clathrin adaptor complex (CAC), exocyst complex component sec3 (SEC3), tonoplastic intrinsic proteins-41 (TIP41), ADP-ribosylation factor (ARF), ribosomal protein L (RPL2), ubiquitin extension protein (UBI), translation elongation factor 2 (TEF2), cytochrome c oxidase subunit Vc (COX), actin (ACT), 28SrRNA (28S), elongation factor 1 α promoter (EF1α), glyceraldehyde 3-phosphate dehydrogenase (GAPDH), and tubulin α-2 (TUB)] were selected for screening and identification, and their expression stability was tested by qRT-PCR. The tissues (leaf and rhizome) were collected 60, 110, 140, and 180 days after sowing. In addition, abiotic stress (ABA treatment and salt stress) and samples of different varieties were also studied. In this study, the expression stability of candidate genes was calculated using geNorm, NormFinder, and Best Keeper. Finally, in order to validate the reference genes, the most and least stable genes or genome combinations were selected to standardize the expression levels of ZoSPS genes in different tissues, stages, varieties, and abiotic stress.
Materials and Methods
Plant Material, Culture Conditions, and Sample Collection
Zingiber officinale Roscoe cultivar “Shannong 1” (S1), “Laiwu big ginger” (LBG), and “Laiwu small ginger” (LSG) were sown in pots (diameter: 25 cm, height: 30 cm) on May 1, 2019. Tissues (leaf and rhizome) were collected 60, 110, 140, and 180 days after sowing. On August 13, S1 with similar growth was selected for ABA treatment [foliar spraying of ABA (100 μmol L–1)] and salt stress [soil applying NaCl (100 mmol L–1) to simulate salt stress]. Ginger leaves treated with ABA and salt stress were collected on August 20. All samples were collected, washed, and surface dried, then stored at −80°C. Each sample was repeated three times.
Total RNA Extraction and cDNA Synthesis
Total RNA was extracted using a RNA Isolation Kit (TianGen, China). A total of 400 ng RNA was reverse transcribed to cDNA using a cDNA synthesis kit (TianGen, China).
Identification of Candidate Reference Genes
A total 14 candidate reference genes, including RNA polymerase-II (RPII), clathrin adaptor complex (CAC), exocyst complex component sec3 (SEC3), tonoplastic intrinsic proteins-41 (TIP41), ADP-ribosylation factor (ARF), ribosomal protein L (RPL2), ubiquitin extension protein (UBI), translation elongation factor 2 (TEF2), cytochrome c oxidase subunit Vc (COX), actin (ACT), 28SrRNA (28S), elongation factor 1 α promoter (EF1α), glyceraldehyde 3-phosphate dehydrogenase (GAPDH), and tubulin α-2 chain (TUB) were selected based on previous reports in other plant species (Chandna et al., 2012; Park et al., 2012; Xu et al., 2012; Tang et al., 2017). Preliminary sequence information of the 14 selected ginger reference genes was blasted using our unpublished transcriptome data.
qRT-PCR Primer Design and Validation
Quantitative primers for the 14 genes were designed by the Premier 5.0 program. A standard curve using a series of gradient-diluted cDNAs was generated to calculate the gene-specific PCR amplification efficiency (E) and correlation coefficients (R2) for each gene, qPCR was performed using the ABI Q6 Real-Time PCR system and a TB GREEN Premix Ex Taqreal-time PCR Kit (Takara, China), and the presence of a single amplification product of the expected size for each gene was verified by electrophoresis on a 1.5% agarose gel. PCR was performed under the following conditions: 95°C for 30 s, followed by 40 cycles at 95°C for 5 s for denaturation and 60°C for 30 s for annealing and extension. The experiments used independent RNA samples from three biological replicates, and mean Ct values were calculated. The primers are shown in Table 1.
Data Analysis
The Ct values of each reference gene were used to evaluate their expression levels. Expression stability was analyzed using the geNorm (Vandesompele et al., 2002), NormFinder (Andersen et al., 2004), BestKeeper (Pfaffl et al., 2004), and RefFinde1.
Validation of the Candidate Reference Genes
In order to verify the results of our experiments, the most stable and unstable reference genes were selected to validate the expression of the sucrose phosphate synthase (SPS) gene in different tissue samples (leaf, and rhizome), different stages, different varieties, and abiotic stress. The primers for ZoSPS were forward 5′ CAGAATGCCAAGGATTAGC3′ and reverse 5′ CCGTATGAGACCGTGAAT3′, and the SPS gene (GeneBank accession no. GI161176315) was blasted using our unpublished transcriptome data (Verma et al., 2011). The qRT-PCR experimental method was the same as described above, and the relative expression level was calculated by 2–ΔΔct method. Data from three biological replicates were analyzed using analysis of variance (ANOVA).
Results
Reference Gene Expression Analysis
The target specificity of 14 reference genes was detected by gel electrophoresis (Supplementary Figure S1). There was a single band around 100 bp in 14 genes. In addition, the 14 genes produced a single peak on their respective melting curves, which indicates that the primers are very specific (Supplementary Figure S2).
The amplification efficiency of 14 candidate primer pairs was assessed. Each primer pair was amplified, and cDNA was serially diluted 5 times in 10-fold iterations. The amplification efficiencies of 14 candidate reference genes ranged between 96 and 101%, and the regression coefficients (R2) were about 0.99 (Table 1). These results showed that all the 14 candidate primers met the basic requirements of reference genes.
The average Ct distributions of 14 genes in ginger samples were plotted (Figure 1). The results indicated that 14 candidate reference genes have average Ct thresholds between 8 and 35. Here, 28S, TUB, RPL2, ARF, GAPDH, SEC3, EF1α, and UBI have average Ct thresholds lower than 25. The gene with the highest average Ct value was TEF2.
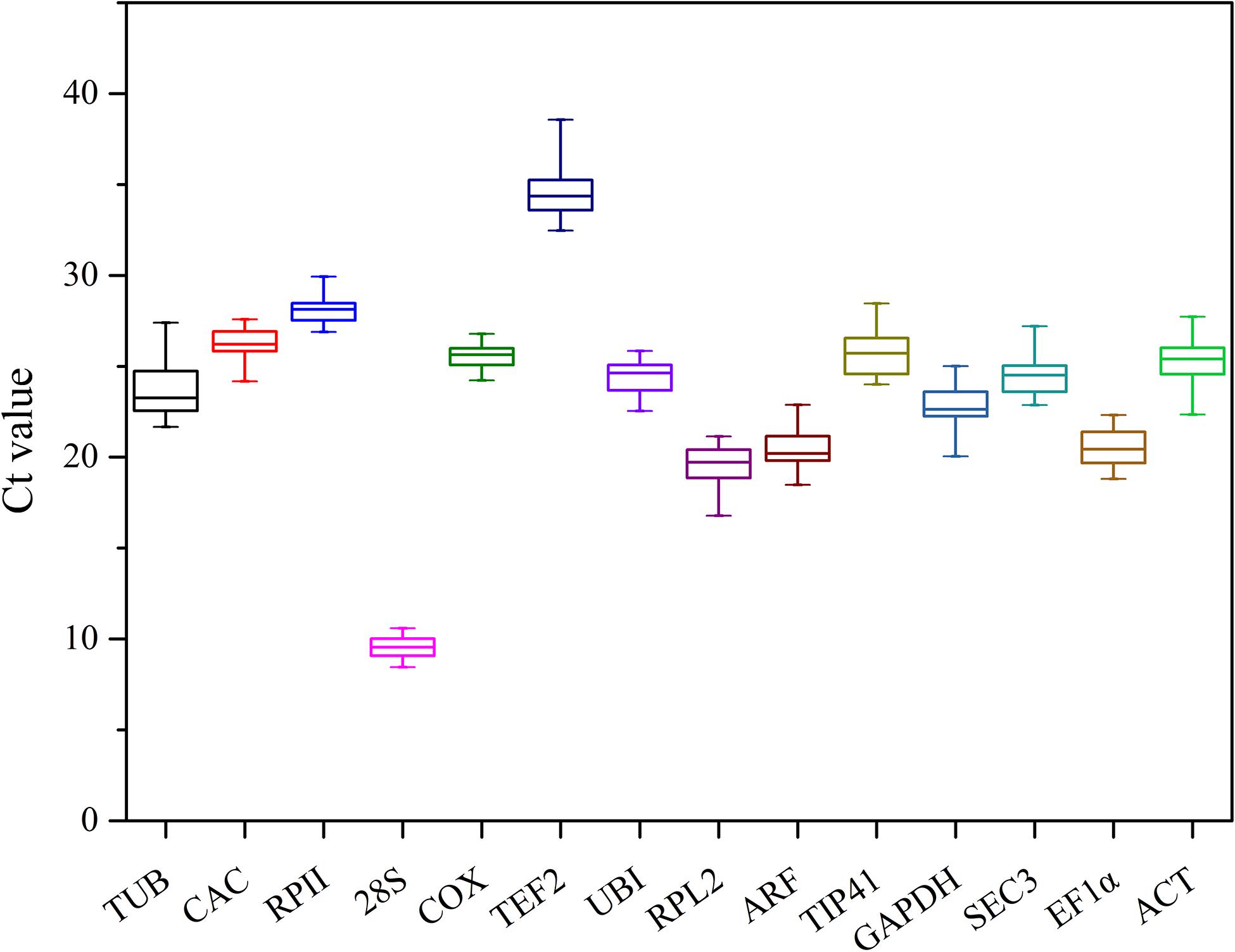
Figure 1. Expression levels of 14 candidate reference genes in ginger. The lines across the box indicate median values; boxes depict 25/75 percentiles. Whisker caps indicate the minimum and maximum values.
Evaluation of Reference Gene Stability Using Genorm, Bestkeeper, and NormFinder
GeNorm Analysis
GeNorm calculates the M value through pairwise comparison in stepwise iterations and screens out genes with better stability (Vandesompele et al., 2002). The lower the M value, the more stable the expression for the gene. The M value of the stable gene should be lower than 1.5 (Vandesompele et al., 2002). This study showed that the M values of all candidate genes under different conditions were lower than 1.5. Under different development stages, ACT and RPII had the lowest M value (0.396) and were the most stable genes in rhizomes. In addition, COX and RPII (0.416) were the most stable genes in leaf; however, the least stable genes were RPL2 and TUB (1.009 and 1.166) in rhizome and leaf, respectively (Figure 2). From the perspective of different ginger varieties, SEC3 and 28S (0.259) were the most stably expressed genes in rhizome, and TIP41 and RPII (0.145) were the most stable genes in leaf, while the least stable genes were ACT and TEF (0.862 and 1.185) in rhizome and leaf, respectively. Under salt stress, COX and 28S (0.396) were the most stably expressed, but RPL2 was the least stable (Figure 2). Under ABA treatment, COX and 28S (0.424) were the most stably expressed genes, while RPL2 was the least stably expressed (Figure 2). Under the total conditions, COX and RPII (0.519) were the most stably expressed genes, whereas TEF2 was the least stable.
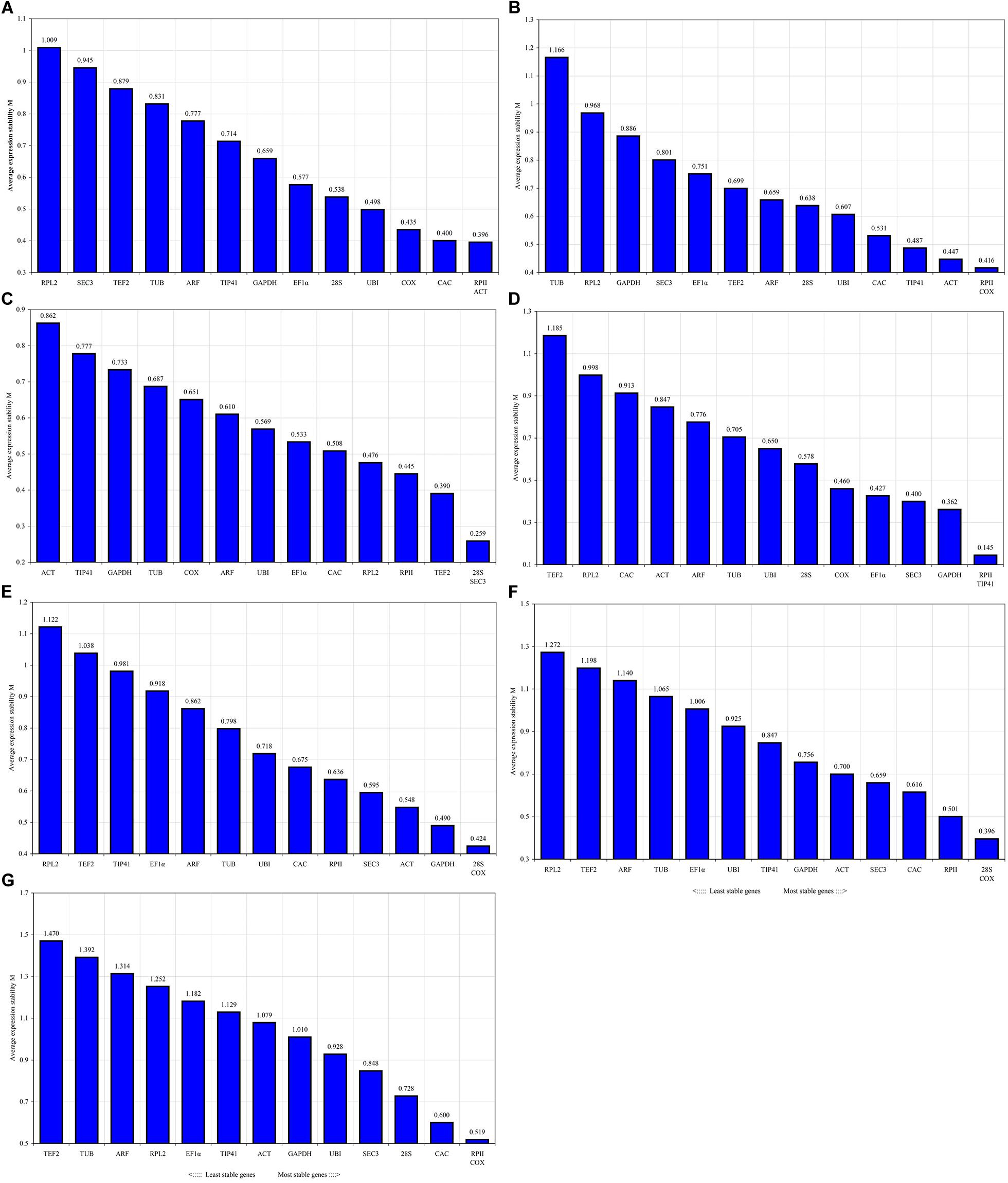
Figure 2. Gene expression stability calculated by geNorm. Mean expression stability (M) was calculated following stepwise exclusion of the least stable gene in different conditions. The least stable genes are on the left and the most stable genes on the right. (A) Gene expression stability in rhizome at different developmental stages. (B) In leaf at different developmental stages. (C) In rhizome of different varieties. (D) In leaf of different varieties. (E) Under ABA treatment. (F) Under salt stress. (G) Total conditions.
The pairwise variation (V) between the normalization factors is used to determine the optimal number of reference genes required. Assuming a cutoff of Vn/n + 1 ≤ 0.15, it was determined that the genes required for normalization in different periods and different varieties were the top two reference genes. Under total conditions, the number of genes increased to four (Supplementary Figure S3).
NormFinder Analysis
Like geNorm, the larger value, the lower stability. The most stable genes was RPII (rhizome and leaf) for different growth stages, SEC3 (rhizome) and TIP41 (leaf) for different varieties, 28S for ABA treatment and salt stress, and COX for total conditions. Additionally, the second and third stable genes were CAC, ACT (rhizome) and ACT, TIP41 (leaf) for different stages, RPII, EF1α (rhizome) and RPII, COX (leaf) for different varieties, COX and GAPDH for ABA treatment, COX and RPII for salt stress, and 28S and RPII for total conditions, respectively. TEF2 was the least stable genes for total conditions (Table 2).
BestKeeper
BestKeeper estimates the stability of candidate reference genes by standard deviation (SD) and variation coefficient (CV). The lower the SD value and the CV value, the stronger the stability of the reference gene, and the reference gene with an SD value > 1 is often regarded as an unstable expression gene. It was found that ARF and RPII were the most stably expressed genes for the different stages in rhizome, and RPII and TIP41 were the most stably expressed genes for the different stages in leaf. However, SEC3 and TUB were identified as the least stable genes in rhizome and leaf, respectively. Under different varieties, the most stably expressed genes were RPII (rhizome) and TIP41 (leaf), and TEF2 (rhizome) and RPII (leaf) were ranked in the second positions, whereas ACT (rhizome) and TEF2 (leaf) were identified as the least stably expressed genes. In addition, RPII was the most stably expressed under ABA treatment, followed by 28S as the second positions. However, RPL2 with the SD values > 1 was unstably expressed. Under salt stress, 28S was the most stably expressed gene, while RPL2 was treated as the unstably expressed genes. Nevertheless, TEF2 displayed a higher SD value under total conditions. 28S, COX, and RPII owing a lower SD value was considered as the stably expressed genes (Table 3).
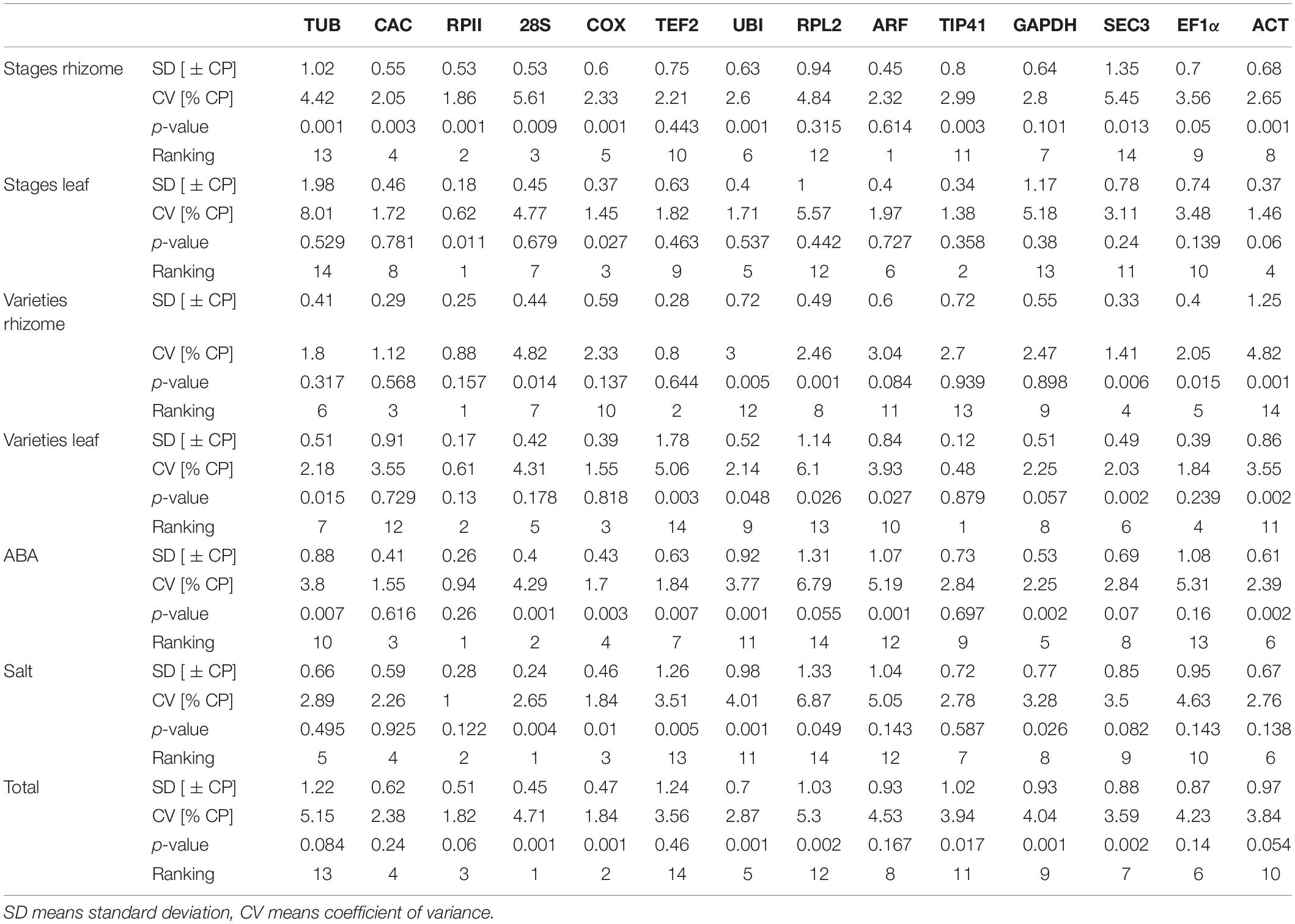
Table 3. The ranking of 14 candidate reference genes at different conditions according to BestKeeper.
Comprehensive Analysis
We use RefFinder to determine the comprehensive ranking of candidate reference genes (Table 4). RefFinder is an online software, which combines the algorithms of GeNorm, Bestkeeper, NormFinder, and other software. RefFinder ranks each individual gene in a variety of ways to calculate the stability value of the reference gene, and the smaller the stability value, the more stable the gene. RPII was identified as the most stably expressed for different stages and different varieties. However, 28S and COX were identified as the most stably expressed genes under ABA treatment and salt stress and for total samples. Nevertheless, TEF2 and RPL2 were the most unstably expressed genes in ginger.
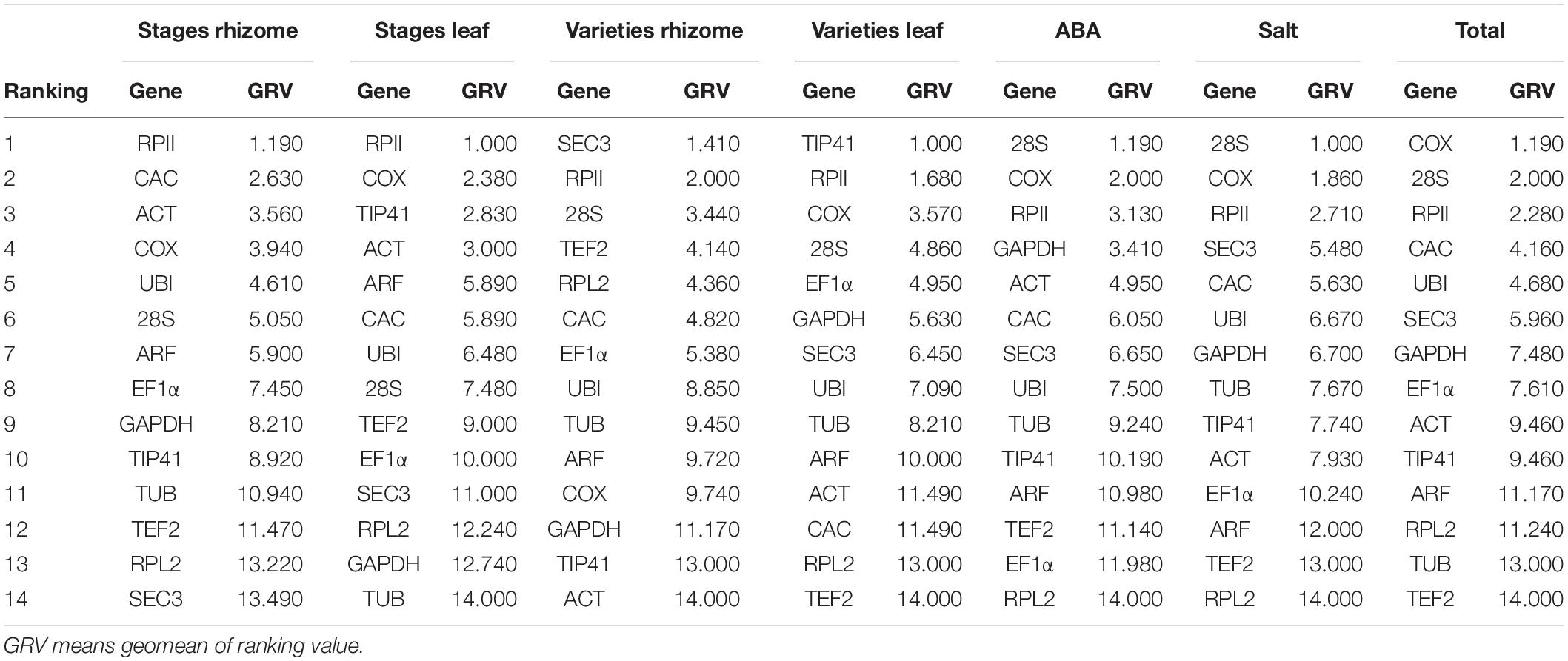
Table 4. The ranking of 14 candidate reference genes at different conditions using RefFinder software.
Validation of Candidate Reference Genes
In order to verify the reliability of the reference genes selected in this study, we selected the SPS gene for verification. As is well known, SPS is the key control gene in the synthesis of sucrose (Sawitri et al., 2018). It plays an important role in sink-source metabolism and sucrose accumulation (Li X. et al., 2018; Verma et al., 2019), and its expression and gene-coding enzyme activity were significantly different in different tissues and at different stages (Laporte et al., 2001; Lester et al., 2001). Therefore, we chose the SPS gene as the target gene to verify the feasibility of the reference gene identified in this study. Under different developmental stages, the expression of ZoSPS first increased and then decreased when normalized with the two stable genes (RPII and 28S), and the highest expression was 140 days after sowing. However, the expression pattern changed significantly when normalized with the least stable genes (RPL2 and TEF2) (Figure 3). Under different varieties, the expression of ZoSPS was S1 > LBG > LXG when the most stable reference genes (RPII and 28S) were used to normalize, whereas the expression of SPS in LXG leaves was significantly increased using the least stable gene (TEF2 and RPL2) (Figure 3). Through normalization detection of reference genes under ABA treatment and salt stress, we found that both 28S and COX could be used as reference genes for normalization, but the expression of SPS normalized by TEF2 was significantly decreased compared to 28S and COX under ABA treatment (Figure 3). In addition, the relative expression of SPS normalized by TEF2 and RPL2 was increased compared to 28S and COX under salt stress (Figure 3). Our previous studies showed that the SPS activity increased first and then decreased with the growth of ginger, which is consistent with the relative expression of ZoSPS normalized by the most stable combination (RPII and 28S) at different stages in this study, indicating the reliability of using RPII and 28S as housekeeping genes.
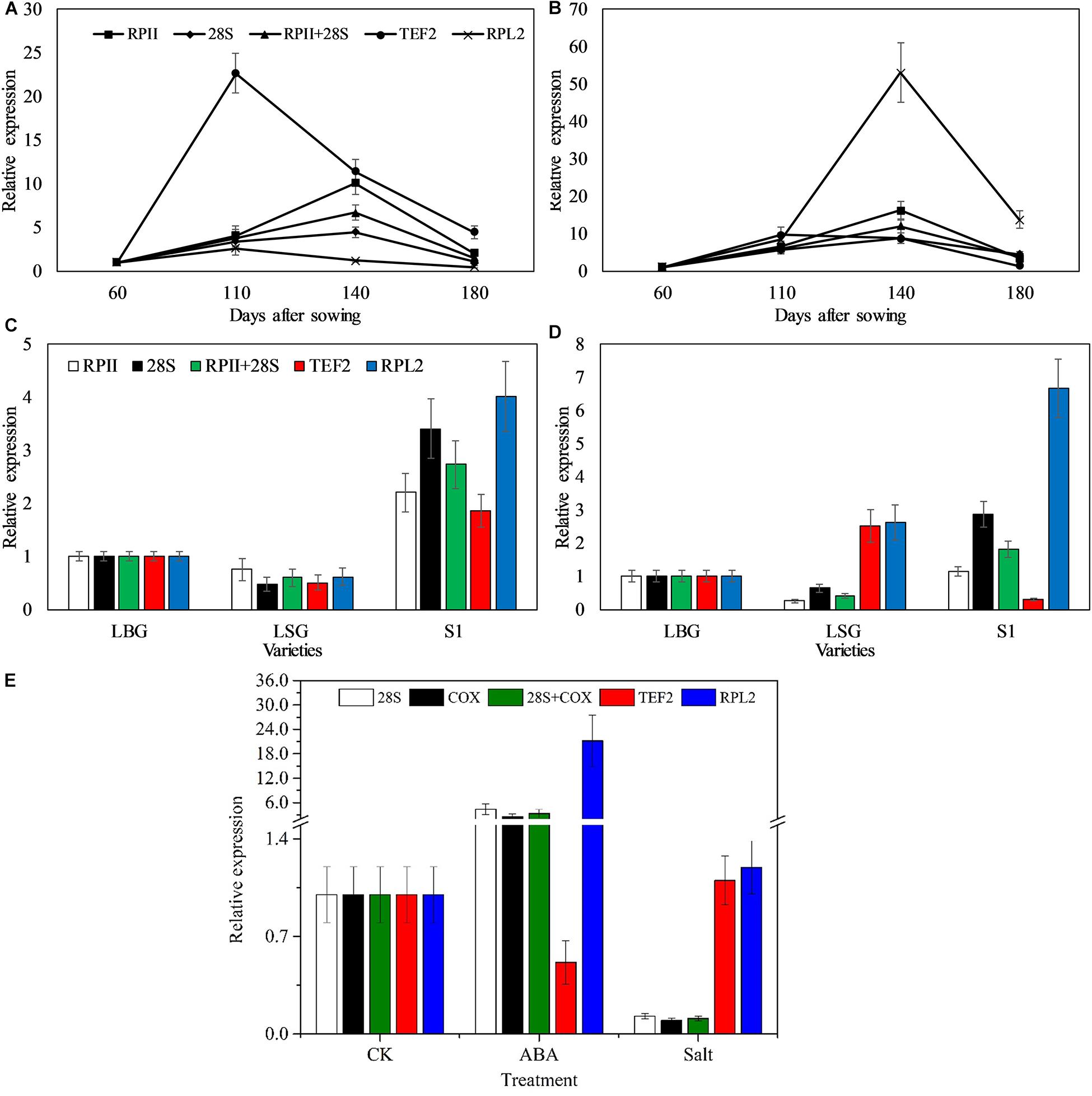
Figure 3. Relative expression of ZoSPS in different conditions. RPII, 28S, and COX were used as one or two most stable reference genes; TEF2 and RPL2 were used as the least stable reference gene. (A) In rhizome at different developmental stages. (B) In leaf at different developmental stages. (C) In rhizome of different varieties. (D) In leafs of different varieties. (E) ABA and salt stress treatment.
Discussion
Due to the lack of molecular biology research in ginger, there is no suitable reference gene for PCR analysis. This is the first time to screen and identify a series of candidate genes through stability analysis to determine the best reference gene for ginger. In addition, there are many problems in the process of ginger cultivation, such as long cultivation cycle, numerous varieties, and salt stress. Quantitative detection of the expression of key genes by qRT-PCR can solve the response mechanism of ginger under different conditions. The optimal reference gene can ensure the accurate presentation of gene expression in qRT-PCR analysis (Wang et al., 2020).
Appropriate reference genes contribute to more accurate expression of target genes (Connors et al., 2019). The stability of commonly used reference genes in different plants or different environments is different. For example, GAPDH of Cyamopsis tetragonoloba can be stably expressed under high temperature and salt stress (Jaiswal et al., 2018), but the stability of GAPDH of Setaria viridis was the lowest (Martins et al., 2016).
We used the geNorm, NormFinder, and BestKeeper programs to analyze the stability of candidate reference genes. However, there were some differences in the stability rankings of the reference genes given by the three softwares, mainly because of the different statistical algorithms. These analysis differences also appeared in other studies (Li et al., 2016; Hu et al., 2018). Through comprehensive analysis, the most stable RPII, 28S, and COX and the least stable RPL2 and TEF2 were selected for subsequent verification.
Commonly used reference genes (actin, tubulin, ubiquitin, etc.) have always been very popular. However, these genes have defects, and their expression varies greatly in different tissues, different developmental stages, and different experimental conditions (Chapman and Waldenström, 2015; Li et al., 2019). This is mainly related to the variability of reference genes in different environments and species. In addition, in the studies of reference gene stability, these commonly used reference genes are often not the most optimal choice (Chapman and Waldenström, 2015; Adeyinka et al., 2019; Gao et al., 2020), and this study has the same findings. In the qRT-PCR research on ginger, common reference genes (TUB, actin, EF1α, etc.) have been used (Girija et al., 2017; Jiang et al., 2017; Chen et al., 2020), but this study found that these are not the best choice, and some common reference genes even rank lower in this study. Therefore, the selection of appropriate reference genes in ginger is very important for the standardization of target genes.
This study found that the RPII gene ranked first or second by the RefFinder program was proved to be the best reference gene for different stages, different varieties, ABA treatment, and salt stress. In addition, 28S also normalized the expression of target genes. RPII is the enzyme that transcribes mRNA from protein-encoding genes (Adelman and Lis, 2012), which is the main reason why they are continuously expressed and show minimal changes. Xu et al. (2012) found that RPII is a relatively stable gene in radish. Therefore, RPII can be stably expressed in different periods and different varieties of ginger. 28SrRNA is a ribosomal RNA with weak mutation and could not highly modify the total RNA level (Thellin et al., 1999). The expression level of 28SrRNA was very stable compared with the commonly used reference genes (Stürzenbaum and Kille, 2001), so it was often used as a reference gene for quantitative research (González et al., 2002; Klok et al., 2002). This study found that 28S can also be stably expressed under different conditions. There are few studies about COX. Park et al. (2012) found that it can be stably expressed in sweet potato, which is consistent with the results of ABA treatment and salt stress in this study.
TEF2 is a regulatory protein of the translation extension step (Browne and Proud, 2002), which catalyzes the movement of ribosomes along mRNA. However, TEF2 is regulated by a variety of mechanisms (Yin et al., 2003; Jørgensen et al., 2006) and is sensitive to oxidative stress (Parrado et al., 1999). TEF2 promotes protein synthesis in the stress response and helps cells reduce the adverse effects of oxidative stress (Argüelles et al., 2013). Although Xu et al. (2012) found that it can be stably expressed in sweet potato, this study found that TEF2 would break the normal expression of ginger target genes. RPL is the largest subunit in the ribosome, the lack of ribosomal protein will affect the function of the ribosome to a certain extent, and the loss of important ribosomal protein will cause the disorder of intracellular life activities, the abnormality of the organ, and even the death of the organism. Many studies found that RPLs also showed high expression stability in different stages and abiotic stress (Park et al., 2012; Fu et al., 2013; An et al., 2016). However, in this study, RPL2 is the least stable gene, indicating that the expression stability of common reference genes is significantly different in different crops and under different conditions, which proves the importance of selecting ginger stable housekeeping genes.
Conclusion
This study reports the selection and verification of reference genes for qPCR in ginger under different tissues, different varieties, and abiotic stress. The stability values of 14 candidate reference genes were analyzed by geNorm, NormFinder, Bestkeeper, and RefFinder. Different programs give slightly different gene stability rankings, but in general, RPII and 28S are the most stable reference genes in different stages and different varieties, and 28S and COX are the most stable reference genes in abiotic stress and total conditions, whereas RPL2 and TEF2 are the least stable. In addition, the reliability of the identified reference genes was verified through determining the expression pattern of ZoSPS. Moreover, this study can provide theoretical support for future research on ginger gene expression.
Data Availability Statement
All datasets presented in this study are included in the article/Supplementary Material.
Author Contributions
YLv, XL, and KX designed the experiments and wrote the manuscript. YLv and KX selected the material. YLv and YLi performed the experiments. YLv and XL analyzed the data. All authors read and approved the final manuscript.
Funding
This study was supported by the China Agriculture Research System (Grant No. CARS-24-A-09), National Natural Science Foundation of China (Grant No. 31972399), Taishan Industrial Experts Programme, China (Grant No. tscy20190105), and Shandong Province’s Dual-class Discipline Construction Project, China (Grant No. SYL2017YSTD06).
Conflict of Interest
The authors declare that the research was conducted in the absence of any commercial or financial relationships that could be construed as a potential conflict of interest.
Acknowledgments
The English version of this manuscript has been revised by Ke Liu, who lives in the United States all year round and has extensive writing experience.
Supplementary Material
The Supplementary Material for this article can be found online at: https://www.frontiersin.org/articles/10.3389/fgene.2020.586098/full#supplementary-material
Footnotes
References
Adelman, K., and Lis, J. T. (2012). Promoter-proximal pausing of RNA polymerase II: emerging roles in metazoans. Nat. Rev. Genet. 13, 720–731. doi: 10.1038/nrg3293
Adeyinka, O. S., Tabassum, B., Nasir, I. A., Yousaf, I., Sajid, I. A., Shehzad, K., et al. (2019). Identification and validation of potential reference gene for effective dsRNA knockdown analysis in Chilo partellus. Sci. Rep. 9:13629.
An, X., Hou, M., and Liu, Y. (2016). Reference gene selection and evaluation for gene expression studies using qRT-PCR in the white-backed planthopper, Sogatella furcifera (Hemiptera: Delphacidae). J. Econ. Entomol. 109, 879–886. doi: 10.1093/jee/tov333
Andersen, C. L., Jensen, J. L., and Ørntoft, T. F. (2004). Normalization of real-time quantitative reverse transcription-PCR data: a model-based variance estimation approach to identify genes suited for normalization, applied to bladder and colon cancer data sets. Cancer Res. 64, 5245–5250. doi: 10.1158/0008-5472.can-04-0496
Argüelles, S., Camandola, S., Hutchison, E. R., Cutler, R. G., Ayala, A., and Mattson, M. P. (2013). Molecular control of the amount, subcellular location, and activity state of translation elongation factor 2 in neurons experiencing stress. Free Radic. Biol. Med. 61, 61–71. doi: 10.1016/j.freeradbiomed.2013.03.016
Browne, G. J., and Proud, C. G. (2002). Regulation of peptide-chain elongation in mammalian cells. Eur. J. Biochem. 269, 5360–5368. doi: 10.1046/j.1432-1033.2002.03290.x
Chandna, R., Augustine, R., and Bisht, N. C. (2012). Evaluation of candidate reference genes for gene expression normalization in Brassica juncea using real time quantitative RT-PCR. PLoS One 7:e36918. doi: 10.1371/journal.pone.0036918
Chapman, J. R., and Waldenström, J. (2015). With Reference to reference genes: a systematic review of endogenous controls in gene expression studies. PLoS One 10:e0141853. doi: 10.1371/journal.pone.014185
Chen, Z. X., Tang, N., Li, H. H., Liu, G. H., and Tang, L. (2020). Genome-wide transcriptomic analysis during rhizome development of ginger (Zingiber officinale Roscoe.) reveals hormone and transcriptional regulation involved in cellulose production. Sci. Horticult. 264:109154. doi: 10.1016/j.scienta.2019.109154
Connors, E., Soto-Dávila, M., Hossain, A., Vasquez, I., Gnanagobal, H., and Santander, J. (2019). Identification and validation of reliable Aeromonas salmonicida subspecies salmonicida reference genes for differential gene expression analyses. Infect. Genet. Evol. 73, 314–321. doi: 10.1016/j.meegid.2019.05.011
Crisp, P. A., Ganguly, D. R., Smith, A. B., Murray, K. D., Estavillo, G. M., Searle, I., et al. (2017). Rapid recovery gene downregulation during excess-light stress and recovery in Arabidopsis. Plant Cell 29, 1836–1863. doi: 10.1105/tpc.16.00828
Fu, W., Xie, W., Zhang, Z., Wang, S., Wu, Q., Liu, Y., et al. (2013). Exploring valid reference genes for quantitative real-time PCR analysis in Plutella xylostella (Lepidoptera: Plutellidae). Int. J. Biol. Sci. 9:792. doi: 10.7150/ijbs.5862
Gao, P., Wang, J. Z., and Wen, J. B. (2020). Selection of reference genes for tissue/organ samples of adults of Eucryptorrhynchus scrobiculatus. PLoS One 15:e0228308. doi: 10.1371/journal.pone.0228308
Girija, A., Devakumar, L. J. P. S., Vijayanathan, M., and Vasudevan, S. E. (2017). Nitric oxide as a bioactive molecule in the regulation of chalcone synthase during jasmonic acid mediated defense signaling in ginger. Plant Cell Tissue Organ Cult. 128, 715–721. doi: 10.1007/s11240-016-1129-8
González, M. A.-C., Echevarría, C., Vidal, J., and Cejudo, F. J. (2002). Isolation and characterisation of a wheat phosphoenolpyruvate carboxylase gene. modell. encoded protein. Plant Science 162, 233–238. doi: 10.1016/s0168-9452(01)00548-9
Guo, N., Qian, Y., Zhang, Q., Chen, X., Zeng, G., Zhang, X., et al. (2017). Alternative transcription start site selection in Mr-OPY2 controls lifestyle transitions in the fungus Metarhizium robertsii. Nat. Commun. 8:1565.
Hu, X., Zhang, L., Nan, S., Miao, X., Yang, P., Duan, G., et al. (2018). Selection and validation of reference genes for quantitative real-time PCR in Artemisia sphaerocephala based on transcriptome sequence data. Gene 657, 39–49. doi: 10.1016/j.gene.2018.03.004
Jaiswal, P. S., Kaur, N., and Randhawa, G. (2018). Identification of reference genes for real-time PCR gene expression studies during seed development and under abiotic stresses in Cyamopsis tetragonoloba (L.) Taub. bioRxiv [preprint] doi: 10.1101/313437
Jiang, Y. S., Liao, Q. H., Zou, Y., Liu, Y. Q., and Lan, J. B. (2017). Transcriptome analysis reveals the genetic basis underlying the biosynthesis of volatile oil, gingerols, and diarylheptanoids in ginger (Zingiber officinale Rosc.). Botan. Stud. 58:41.
Jørgensen, R., Merrill, A. R., and Andersen, G. R. (2006). The life and death of translation elongation factor 2. Biochem. Soc. Trans. 34(Pt 1), 1–6. doi: 10.1042/bst0340001
Klok, E. J., Wilson, I. W., Wilson, D., Chapman, S. C., Ewing, R. M., Somerville, S. C., et al. (2002). Expression profile analysis of the low-oxygen response in Arabidopsis root cultures. Plant Cell 14, 2481–2494. doi: 10.1105/tpc.004747
Laporte, M. M., Galagan, J. A., Prasch, A. L., Vanderveer, P. J., Hanson, D. T., Shewmaker, C. K., et al. (2001). Promoter strength and tissue specificity effects on growth of tomato plants transformed with maize sucrose-phosphate synthase. Planta 212, 817–822. doi: 10.1007/s004250000433
Lester, G. E., Arias, L. S., and Gomez-Lim, M. (2001). Muskmelon fruit soluble acid invertase and sucrose phosphate synthase activity and polypeptide profiles during growth and maturation. J. Am. Soc. Horticult. Sci. 126, 33–36. doi: 10.21273/jashs.126.1.33
Li, H., Huang, M., Tan, D., Liao, Q., Zou, Y., and Jiang, Y. (2018). Effects of soil moisture content on the growth and physiological status of ginger (Zingiber officinale Roscoe). Acta Physiol. Plantarum 40:125.
Li, M. Y., Wang, F., Jiang, Q., Wang, G. L., Tian, C., and Xiong, A. S. (2016). Validation and comparison of reference genes for qPCR normalization of celery (Apium graveolens) at different development stages. Front. Plant Sci. 7:313. doi: 10.3389/fpls.2016.00313
Li, S., Lauri, A., Ziemann, M., Busch, A., Bhave, M., and Zachgo, S. (2009). Nuclear activity of ROXY1, a glutaredoxin interacting with TGA factors, is required for petal development in Arabidopsis thaliana. Plant Cell 21, 429–441. doi: 10.1105/tpc.108.064477
Li, X., Du, J., Guo, J., Wang, H., Ma, S., Lü, J., et al. (2018). The functions of cucumber sucrose phosphate synthases 4 (CsSPS4) in carbon metabolism and transport in sucrose- and stachyose-transporting plants. J. Plant Physiol. 228, 150–157. doi: 10.1016/j.jplph.2018.05.013
Li, Z. Y., Lu, H. J., He, Z. H., Wang, C., Wang, Y. C., and Ji, X. Y. (2019). Selection of appropriate reference genes for quantitative real-time reverse transcription PCR in Betula platyphylla under salt and osmotic stress conditions. PLoS One 14:e0225926. doi: 10.1371/journal.pone.0225926
Liang, W., Zou, X., Carballar-Lejarazú, R., Wu, L., Sun, W., Yuan, X., et al. (2018). Selection and evaluation of reference genes for qRT-PCR analysis in Euscaphis konishii Hayata based on transcriptome data. Plant Methods 14:42.
Martins, P. K., Mafra, V., and Souza, W. R. D. (2016). Selection of reliable reference genes for RT-qPCR analysis during developmental stages and abiotic stress in Setaria viridis. Sci. Rep. 6:28348.
Marx, W., Isenring, E. A., and Lohning, A. E. (2017). Determination of the concentration of major active anti-emetic constituents within commercial ginger food products and dietary supplements. Eur. J. Integrat. Med. 10, 19–24. doi: 10.1016/j.eujim.2017.02.001
Park, S.-C., Kim, Y.-H., Ji, C. Y., Park, S., Jeong, J. C., Lee, H.-S., et al. (2012). Stable internal reference genes for the normalization of real-time PCR in different sweetpotato cultivars subjected to abiotic stress conditions. PLoS One 7:e51502. doi: 10.1371/journal.pone.0051502
Parrado, J., Bougria, M., Ayala, A., Castaño, A., and Machado, A. (1999). Effects of aging on the various steps of protein synthesis: fragmentation of elongation factor 2. Free Radic. Biol. Med. 26, 362–370. doi: 10.1016/s0891-5849(98)00202-0
Petriccione, M., Mastrobuoni, F., Zampella, L., and Scortichini, M. (2015). Reference gene selection for normalization of RT-qPCR gene expression data from Actinidia deliciosa leaves infected with Pseudomonas syringae pv. actinidiae. Sci. Rep. 5:16961.
Pfaffl, M. W., Tichopad, A., Prgomet, C., and Neuvians, T. P. (2004). Determination of stable housekeeping genes, differentially regulated target genes and sample integrity: bestkeeper – excel-based tool using pair-wise correlations. Biotechnol. Lett. 26, 509–515. doi: 10.1023/b:bile.0000019559.84305.47
Sawitri, W. D., Afidah, S. N., Nakagawa, A., Hase, T., and Sugiharto, B. (2018). Identification of UDP-glucose binding site in glycosyltransferase domain of sucrose phosphate synthase from sugarcane (Saccharum officinarum) by structure-based site-directed mutagenesis. Biophys. Rev. 10, 293–298. doi: 10.1007/s12551-017-0360-9
Shukla, A., Naik, S., Goud, V. V., and Das, C. (2019). Supercritical CO2 extraction and online fractionation of dry ginger for production of high-quality volatile oil and gingerols enriched oleoresin. Indust. Crops products 130, 352–362. doi: 10.1016/j.indcrop.2019.01.005
Stürzenbaum, S. R., and Kille, P. (2001). Control genes in quantitative molecular biological techniques: the variability of invariance. Comp. Biochem. Physiol. Part B 130, 281–289. doi: 10.1016/s1096-4959(01)00440-7
Su, W., Yuan, Y., Zhang, L., Jiang, Y., Gan, X., Bai, Y., et al. (2019). Selection of the optimal reference genes for expression analyses in different materials of Eriobotrya japonica. Plant Methods 15:7.
Tang, X., Zhang, N., Si, H., and Calderón-Urrea, A. (2017). Selection and validation of reference genes for RT-qPCR analysis in potato under abiotic stress. Plant Methods 13:85.
Thellin, O., Zorzi, W., Lakaye, B., Borman, B. D., Coumans, B., Hennen, G., et al. (1999). Housekeeping genes as internal standards: use and limits. J. Biotechnol. 75, 291–295. doi: 10.1016/s0168-1656(99)00163-7
Tian, C., Jiang, Q., Wang, F., Wang, G.-L., Xu, Z.-S., and Xiong, A.-S. (2015). Selection of suitable reference genes for qPCR normalization under abiotic stresses and hormone stimuli in carrot leaves. PLoS one 10:e0117569. doi: 10.1371/journal.pone.0117569
Vandesompele, J., Preter, K. D., Pattyn, F., Poppe, B., Roy, N. V., Paepe, A. D., et al. (2002). Accurate normalization of real-time quantitative RT-PCR data by geometric averaging of multiple internal control genes. Genome Biol. 3:research0034.
Verma, A. K., Upadhyay, S. K., Verma, P. C., Solomon, S., and Singh, S. B. (2011). Functional analysis of sucrose phosphate synthase (SPS) and sucrose synthase (SS) in sugarcane (Saccharum) cultivars. Plant Biol. 13, 325–332. doi: 10.1111/j.1438-8677.2010.00379.x
Verma, I., Roopendra, K., Sharma, A., Chandra, A., and Kamal, A. (2019). Expression analysis of genes associated with sucrose accumulation and its effect on source–sink relationship in high sucrose accumulating early maturing sugarcane variety. Physiol. Mol. Biol. Plants 25, 207–220. doi: 10.1007/s12298-018-0627-z
Wang, C. Z., Qi, L. W., and Yuan, C. S. (2015). Cancer chemoprevention effects of ginger and its active constituents: potential for new drug discovery. Am. J. Chin. Med. 43, 1351–1363. doi: 10.1142/s0192415x15500767
Wang, G., Cheng, H., Li, M., Zhang, C., Deng, W., and Li, T. (2020). Selection and validation of reliable reference genes for Tolypocladium guangdongense gene expression analysis under differentially developmental stages and temperature stresses. Gene 734:144380. doi: 10.1016/j.gene.2020.144380
Xu, Y., Zhu, X., Gong, Y., Xu, L., Wang, Y., and Liu, L. (2012). Evaluation of reference genes for gene expression studies in radish (Raphanus sativus L.) using quantitative real-time PCR. Biochem. Biophys. Res. Commun. 424, 398–403. doi: 10.1016/j.bbrc.2012.06.119
Yin, X., Fontoura, B. M., Morimoto, T., and Carroll, R. B. (2003). Cytoplasmic complex of p53 and eEF2. J. Cell. Physiol. 196, 474–482. doi: 10.1002/jcp.10329
Zachgo, S., Lauri, A., Ziemann, M., Busch, A., and Bhave, M. (2018). Nuclear activity of ROXY1, a glutaredoxin interacting with TGA factors, is required for petal development in Arabidopsis thaliana. Plant Cell 21, 429–441. doi: 10.1105/tpc.108.064477
Keywords: gene expression, ginger, normalization, qRT-PCR, reference gene
Citation: Lv Y, Li Y, Liu X and Xu K (2020) Identification of Ginger (Zingiber officinale Roscoe) Reference Genes for Gene Expression Analysis. Front. Genet. 11:586098. doi: 10.3389/fgene.2020.586098
Received: 22 July 2020; Accepted: 09 October 2020;
Published: 03 November 2020.
Edited by:
Reyazul Rouf Mir, Sher-e-Kashmir University of Agricultural Sciences and Technology, IndiaReviewed by:
Arif Ashraf, University of Massachusetts Amherst, United StatesHui Song, Qingdao Agricultural University, China
Copyright © 2020 Lv, Li, Liu and Xu. This is an open-access article distributed under the terms of the Creative Commons Attribution License (CC BY). The use, distribution or reproduction in other forums is permitted, provided the original author(s) and the copyright owner(s) are credited and that the original publication in this journal is cited, in accordance with accepted academic practice. No use, distribution or reproduction is permitted which does not comply with these terms.
*Correspondence: Kun Xu, eHVrdW5Ac2RhdS5lZHUuY24=; MTAwNjM5NzA5MEBxcS5jb20=