- 1Department of Neurology, West China Hospital, Sichuan University, Chengdu, China
- 2Laboratory of Neurodegenerative Disorders, West China Hospital, Sichuan University, Chengdu, China
- 3Rare Disease Center, West China Hospital, Sichuan University, Chengdu, China
Background: The association between inflammation and neurodegeneration has long been observed in parkinson’s disease (PD) and multiple system atrophy (MSA). Previous genome-wide association studies (GWAS) and meta-analyses have identified several risk loci in inflammation-associated genes associated with PD.
Objective: To investigate whether polymorphisms in some inflammation-associated genes could modulate the risk of developing PD and MSA in a Southwest Chinese population.
Methods: A total of 2,706 Chinese subjects comprising 1340 PD, 483 MSA and 883 healthy controls were recruited in the study. Three polymorphisms (rs2074404 GG/GT/TT, rs17425622 CC/CT/TT, rs34043159 CC/CT/TT) in genes linked to inflammation in all the subjects were genotyped by using the Sequenom iPLEX Assay.
Results: The allele G of WNT3 rs2074404 can increase risk on PD (OR: 1.048, 95% CI: 1.182–1.333, p = 0.006), exclusively in the LOPD subgroup (OR: 1.166, 95% CI:1.025–1.327, p = 0.019), but not in EOPD or MSA. And the recessive model analysis also demonstrated an increased PD risk in GG genotype of this locus (OR = 1.331, p = 0.007). However, no significant differences were observed in the genotype distributions and alleles of HLA-DRB5 rs17425622 and IL1R2 rs34043159 between the PD patients and controls, between the MSA patients and controls, or between subgroups of PD or MSA and controls.
Conclusion: Our results suggested the allele G of WNT3 rs2074404 have an adverse effect on PD and particularly, on the LOPD subgroup among a Chinese population.
1 Introduction
Neurodegenerative diseases such as Parkinson’s disease (PD) and multiple system atrophy (MSA) imposed a severe burden on our aging society. More and more people would suffer from neurodegenerative disorders with increasing life expectancy (Dorsey et al., 2007). Although their causes were not yet fully understood, new genetic insights of PD and some genome-wide association studies (GWAS) have strengthened the evidence that PD has a considerable genetic component (Puschmann, 2013; Chang et al., 2017; Nalls et al., 2019; Blauwendraat et al., 2020). And MSA pedigrees with both autosomal dominant and autosomal recessive inheritance patterns have been reported as well (Wullner et al., 2004; Hara et al., 2007; Itoh et al., 2014).
To date, mechanisms underlying PD and MSA pathogenesis remain not fully understood, but recent studies have shown that neuroinflammation might play an important role in their pathogenesis (Jellinger, 2014; De Virgilio et al., 2016; Gelders et al., 2018). The association between inflammation and neurodegeneration has long been observed in PD (McGeer and McGeer, 2004; Phani et al., 2012; Gelders et al., 2018). In addition, recent studies have shown that inflammation contributions to the abnormal accumulation of α-synuclein, which targets dopamine neurons in the midbrain, forming Lewy bodies in PD (Ohama and Ikuta, 1976; Schain and Kreisl, 2017; Bras et al., 2020). In MSA, which is different from PD, such aggregates usually can be found in oligodendrocytes. However, it has been reported that neuroinflammation also closely links to oligodendroglial α-syncleinopathy (Hoffmann et al., 2019). Therefore, inflammation-related genes may play a part in the pathogenesis of such diseases.
WNT3 (Wnt Family Member 3) is within a protein-protein interaction network with PD risk genes, such as LRRK2, SNCA and MAPT. It might regulate the gene expression of these PD-related genes in multiple immune cells contributing to PD risk (Witoelar et al., 2017). HLA-DRB5 (Major Histocompatibility Complex, Class II, DR Beta 5), whose expression products play a central role in the immune system by presenting peptides derived from extracellular proteins, was found to have a possible pathogenic effect Alzheimer’s disease (AD) risk (Karch and Goate, 2015). IL1R2 (Interleukin 1 Receptor Type 2) is another inflammation-related gene and encodes a cytokine receptor. Polymorphisms, rs2074404 in WNT3 and rs17425622 in HLA-DRB5, have been reported to be associated with PD (Liu et al., 2011; Witoelar et al., 2017). However, such association was not investigated in patients from Chinese. And the previous results of the association between IL1R2 rs34043159 and PD in the Chinese population were contradictory (Chen et al., 2018; Li et al., 2018; Gao et al., 2020; Hu et al., 2020; Fang et al., 2021).
Hence, in this study, we sought to investigate whether these three polymorphisms in inflammation-associated genes could modulate the risk of developing PD and MSA in a Southwest Chinese population.
2 Material and Methods
2.1 Subjects
Two independent series of patients, the first cohort of PD diagnosed according to the United Kingdom PD Society Brain Bank Clinical Diagnostic Criteria for PD (Hughes et al., 1992), and the second cohort of MSA meeting the current consensus criteria for the disease (Gilman et al., 2008), were included in this study. Finally, 1823 Chinese subjects comprising 1340 PD, and 483 MSA, were enrolled from the Department of Neurology, West China Hospital of Sichuan University. Detailed clinical variables, such as sex, age, education level, onset age, and initial symptoms, were recorded. In addition, a group of 883 unrelated, age- and sex-matched healthy Chinese controls (HCs) was also recruited for the study as the control group. Neurologists confirmed that all the HCs did not have any neurological disorders. Signed and informed consent forms were obtained from all the subjects. The ethics committee of West China Hospital of Sichuan University approved this study.
2.2 Genetic Analysis
As described in our previous studies (Chen et al., 2015; Chen Y. et al., 2020; Chen et al., 2021), peripheral blood leukocytes from subjects were collected for genomic DNA extraction using standard phenol-chloroform procedures. The genotypes for WNT3 rs2074404, HLA-DRB5 rs17425622, and IL1R2 rs34043159 were determined with Sequenom iPLEX Assay technology (Sequenom, San Diego, CA, United States) following the manufacturer’s instructions. Generally, about 10 ng of genomic DNA from each sample was amplified through a locus-specific polymerase chain reaction with primers flanking the targeted sequence designed using Mass ARRAY Assay Design 3.0 software (Sequenom). A 384 element SpectroCHIP bioarray was used to purify and transfer the extension products. Parameters were obtained through matrix-assisted laser desorption/ionization time-of-flight mass spectrometry. Finally, the resultant data were processed and analyzed using Sequenom Mass ARRAY Workstation software. The allele frequencies in five continental populations obtained from 1,000 Genomes and location information of these SNPs was presented in Supplementary Table S1. In consideration of all the three variants located in the non-coding regions of genes, we further predicted where it mapped in the nuclear receptor response elements (NRRE) via NUBIScan15, a computer algorithm to predict DNA recognition sites for nuclear receptors in the regulatory regions of genes, with a threshold of 0.8 raw score by using 100-bp sequence both upstream and downstream of each SNP (Podvinec et al., 2002).
2.3 Statistical Analysis
The Hardy–Weinberg equilibrium (HWE) in controls was assessed based on the chi-square test. Age, gender and allele distribution differences between PD patients and controls, and minor allele frequency (MAF) of the SNP were tested by t-test or chi-square test when appropriate. As the risk of developing PD and MSA varied for males and females and for subjects of different ages, binary logistic regression analysis was performed to compare the genotype distributions of the SNP between the patients and controls with gender and age as covariates. A two-tailed p < 0.05 was considered statistically significant. The statistical power was calculated using PS Power and Sample Size Calculations software (version 3.0.43) (Dupont and Plummer, 1990).
3 Results
3.1 Clinical Data
A total of 2,706 subjects, including 1,340 PD, 483 MSA, and 883 healthy controls were recruited in this study, and the demographic information is shown in Table 1. The mean age of PD symptoms onset was 56.46 ± 11.47 years, that of MSA was 56.77 ± 8.92 years and that of controls upon recruitment was 56.19 ± 11.05 years. According to previous study (Bonifati et al., 2005), the PD group was divided into early-onset PD (EOPD, onset ≤ 50 years of age) with 379 (28.3%) patients and late-onset PD (LOPD, onset > 50 years of age) subgroup with 961 (71.7%). As for MSA, 254 (52.6%) patients had the MSA-C (MSA with predominant cerebellar features) type and 229 (47.4%) patients had the MSA-P (MSA with predominant parkinsonism) type.
3.2 Genetic Analysis
The genotype distributions and MAF for the three SNPs in both patients and controls did not deviate significantly from Hardy-Weinberg equilibrium (Table 2). The distributions of WNT3 rs2074404 GG genotype showed a higher prevalence in the PD patients than the controls (25.67 vs. 20.61%). After adjusted age and gender, further analysis suggested that the allele G of rs2074404 might increase risk on PD (OR: 1.182, 95% CI: 1.048–1.333, p = 0.006) and were also significant in the LOPD subgroup (OR: 1.166, 95% CI: 1.025–1.327, p = 0.019) (Table 3). In addition, it became highly significant in the recessive model for the PD and LOPD group (OR: 1.331, 95% CI: 1.082–1.637, p = 0.007) (Table 4). However, no significant differences were observed in the genotype distributions and alleles of HLA-DRB5 rs17425622 between the PD patients and controls, between the MSA patients and controls, or between subgroups of PD or MSA and controls (Supplementary Table S2). Likewise, no significant association with PD or MSA was observed for IL1R2 rs34043159 (Supplementary Table S3). In addition, there were no NRRE of WNT3, HLA-DRB and IL1R2 across any sites of the three SNPs via NUBIScan (Supplementary Figure S1).
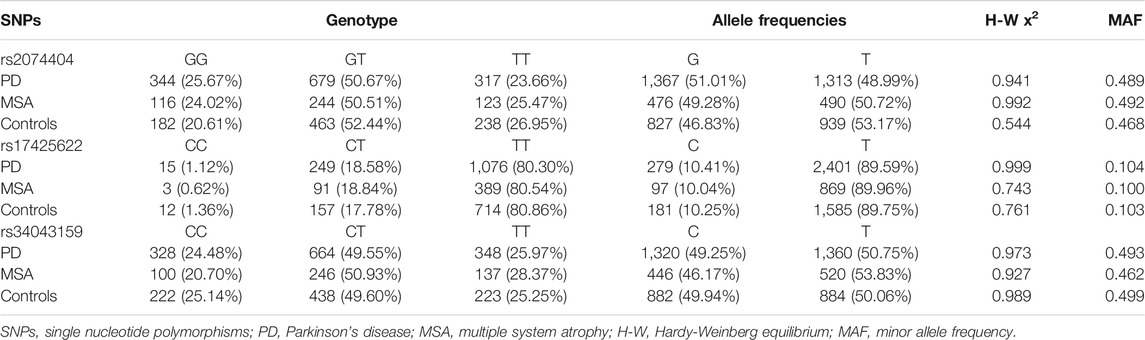
TABLE 2. Distribution of genotype and allele frequencies of rs2074404, rs17425622 and rs34043159 in PD, MSA patients and controls.
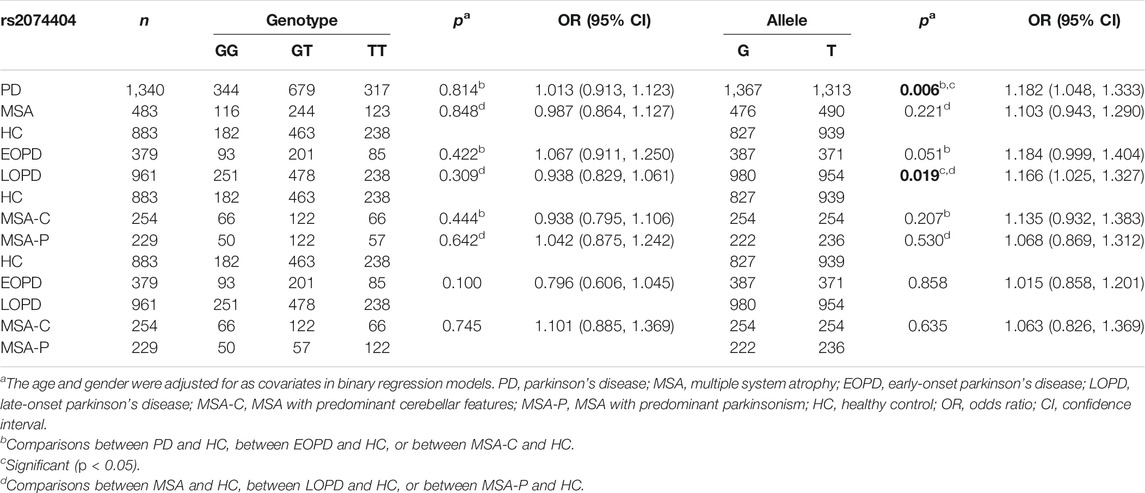
TABLE 3. Distributions of genotype and allele frequencies of WNT3 rs2074404 observed in PD, MSA and healthy-matched control.
4 Discussion
The current study explored the association between polymorphisms in inflammation-related genes and the risk of developing PD and MSA. We replicated the association of HLA-DRB5 rs17425622 and IL1R2 rs34043159 with PD, and first analyzed it with MSA. In addition, we firstly studied the possible modification effects of WNT3 rs2074404 on PD and MSA. The results indicated the WNT3 rs2074404 could modify the risk for PD, especially for LOPD rather than MSA or EOPD, but not in HLA-DRB5 rs17425622 and IL1R2 rs34043159.
WNT3, encode secreted signaling protein, that possesses significant functions during neural development, such as neural stem cells (NSC) proliferation, axonal guidance, and neuronal survival (Gonzalez-Fernandez et al., 2014; Duan et al., 2018). A previous study indicated WNT3 is a candidate risk gene of PD (Liu et al., 2011), and a protein-protein interaction network of WNT3 with known causative PD genes like LRRK2 was also perceived (Witoelar et al., 2017). The locus rs2074404 of WNT3 was previously found to be a CD (Coeliac disease) associated polymorphism (Dubois et al., 2010; Franke et al., 2010; Amundsen et al., 2014). It was also shown that gut inflammation is a main pathological feature occurring in both PD and CD and this inflammation may contribute to α-synuclein aggregation (Dzamko et al., 2017; Drobny et al., 2021). Additionally, some SNPs in genes responsible for binding bacterial metabolites and intestinal homeostasis were associated with PD (Gorecki et al., 2020). All above suggested CD have a link with neurodegenerative diseases, in some respects of genetics and intestinal flora (Voros et al., 2013; Cao et al., 2018; Spielman et al., 2018). Our study found the minor G allele of rs2074404 had a 1.182-bold increased risk of PD, which further supported there may be shared pathways and similar pathogenesis between CD and PD. Analyzing rs2074404 using two genetic models (dominant and recessive) showed that rs2074404 was significantly associated with PD in the recessive mode and with LOPD, suggesting that the G allele was a risk allele for PD.Nevertheless, it didn’t reach significance in the EOPD subgroup. One study indicated that there might be differences in inflammatory responses between EOPD and LOPD, and peripheral immune disorders were more severe in LOPD patients (Jiang et al., 2019). It was also possible that the long-term inflammatory stimulation due to rs2074404 variants increased the risk of LOPD instead of EOPD. Mechanistically, rs2074404, an intron variant mapped in WNT3, is not located on the NRRE predicted by NUBIScan, however, we found an NRRE component, ER6 (everted repeats separated by 6 base pairs), is not far upstream from it (Supplementary Figure S1), and the different allele of rs2074404 might affect the ER6 element binding and further influence WNT3 expression. Furthermore, we found that WNT3 rs2074404 has a three-dimensional interacting with rs916888 that also has a similar link to WNT3 rs415430 (Lu et al., 2017) (Supplementary Table S4), which has been reported to be associated with PD in an Ashkenazi Jewish population (Liu et al., 2011). However, whether rs2074404 affects the expression of WNT3, or the fact and potential mechanisms that rs2074404 or rs415430 in WNT3 were associated with the increased PD risk need more studies. Moreover, this locus makes no difference on MSA onset. Onset age might be one reason, because WNT3 rs2074404 increased the risk of development for PD, mainly in LOPD, but not in EOPD. For MSA, the mean onset age was younger than that in LOPD, which makes MSA suffer from a relative shorter-term inflammatory stimulation mediated by WNT3 rs2074404. Even now, the role of neuroinflammation in MSA cannot be ignored. As our previous study has shown, rs3135500 variant in the NOD2 gene whose products act a role in the immune response might increase the risk for MSA (Cao et al., 2018). However, there were no relevant studies on whether WNT3 rs2074404 could contribute to the development of MSA. In this study, it was an exploratory study, the smaller sample size of MSA patients might be another reason which contributing the negative results. It is worth point out that our study may be the first one to directly report that rs2074404 is a polymorphism for PD risk with specific data, Therefore, our findings need to be confirmed in more studies with larger sample-size.
IL1R2 encodes the receptor of IL1 (interleukin-1), a critical inflammation cytokine. The association between IL1 and dopaminergic dysfunction has been reported (Williams-Gray et al., 2016). And this locus has been found to be associated with PD and AD among the Chinese population in some researches (Li et al., 2018; Chen K. et al., 2020; Fang et al., 2021), but in some were not (Chen et al., 2018; Hu et al., 2020). In this current study, rs34043159 was also found to have no effect on both PD and MSA. And then, after a comprehensive searching, we pooled five previous studies including this locus in Chinese and the current analysis (Figure 1; Table 5) together which came out with a nonsignificant result similar to ours, whether performed by random-effects or fixed-effect model. However, using the sample from Chinese (Figure 1, 7,626 allele in patients and 9,322 allele in controls), the statistical power for the rs34043159 polymorphism was 0.677 if the MAF in controls was 38.3% and the OR of developing PD in patients relative to controls 1.08 (Chen et al., 2018; Li et al., 2018; Gao et al., 2020; Hu et al., 2020; Fang et al., 2021), indicated the lack of statistical power to adequately assess the small impact of this risk variant. Further investigations with a larger sample or from experimental evidence are needed to confirm if there is a link between it and PD. In addition, origin-specific effects of the risk locus or potential environmental factors may contribute to these inconsistent results. For MSA, this is the first study to explore rs34043159 on MSA and found out that it is not associated with MSA, whether other loci of IL1R2 region may make a difference on MSA onset need more evidence.
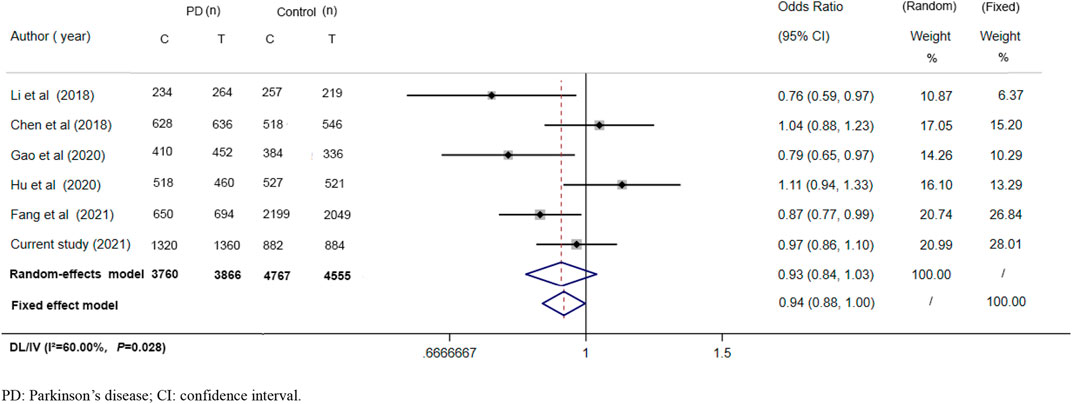
FIGURE 1. Forest plot for IL1R2 rs34043159 on PD risk in Chinese. PD: Parkinson’s disease; CI: confidence interval.
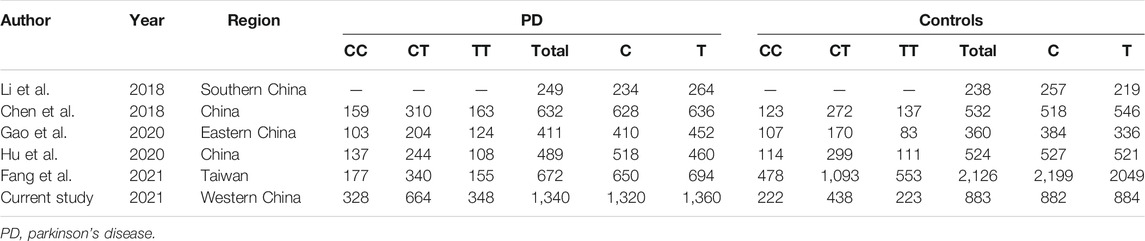
TABLE 5. Characteristics of the included studies for the association between IL1R2 rs34043159 and PD in Chinese.
For HLA-DRB5 rs17425622, it is a risk locus for UC (ulcerative colitis) (Anderson et al., 2011). Braak et al. (2006) suggested that the pathological process in PD originates in the gastrointestinal tract, and then a hypothesis called “gut-brain axis theory” has been proposed, which consists of bidirectional communication between the central nervous system and the enteric nervous system (Carabotti et al., 2015). Indeed, as mentioned above, some researches showed that chronically inflamed gut may trigger α-syn deposition, increase the permeability of the blood-brain barrier leading to neuroinflammation (Lema Tome et al., 2013; Pellegrini et al., 2018). However, whether the SNPs on inflammatory bowel disease genes would increase the risk of PD is worth further exploration. The association of rs17425622 with PD was first explored in a large-scale meta-analysis of genome-wide association, which showed the T allele of rs17425622 could reduce the risk of PD (OR: 0.79, p = 8.07e−5) in the USA population (Nalls et al., 2014). In addition, a recent study for mapping the HLA locus in PD among Europeans also validated associations for the SNPs rs17425622 (OR: 0.92, 95% CI (0.90, 0.95)) (Yu et al., 2021). However, our preliminary exploration of this locus in the Chinese PD and MSA population did not yield meaningful results. In this study, the statistical power for the rs17425622 polymorphism was 0.857 if the MAF in controls was 21.7% and the OR of developing PD in patients relative to controls 0.79 (Nalls et al., 2014), which indicated the sample size involved in our study was enough. The genetic heterogeneity of different people might be an essential reason for the divergent findings.
The strengths of our study are we included a relatively large and ethnically homogeneous cohort, and WNT3 rs2074404 related to PD in the Chinese population was tested for the first time. However, this study has some weaknesses or limitations. First, this genetic study did not take the gene-gene or gene-environment interaction into consideration. Second, our study was a single-center study. Thus, more multi-centers and larger studies are expected.
5 Conclusion
We found the allele G of WNT3 rs2074404 was a risk allele for PD and particularly, for the LOPD subgroup. However, we did not detect the significant association of IL1R2 rs34043159, HLA-DRB5 rs17425622 with PD and MSA in a Chinese population. More studies for the WNT3 signaling pathway would be beneficial in developing new therapeutic strategies.
Data Availability Statement
The original contributions presented in the study are included in the article/Supplementary Material, further inquiries can be directed to the corresponding authors.
Ethics Statement
The studies involving human participants were reviewed and approved by the West China Hospital of Sichuan University. The patients/participants provided their written informed consent to participate in this study.
Author Contributions
W-MS: Design, execution, data analysis, and writing. X-JG: Execution, blood sample collection, and DNA extraction; Y-BH, L-YZ, BC, and R-WO: Execution and clinical data collection. YW, X-PC, WS, BZ, Y-PC, and H-FS: Execution, patient enrollment. H-FS and Y-PC: Conception, design, organization, review, and critique. All authors contributed to the article and approved the submitted version.
Funding
This study was supported the National Key Research and Development Program of China (2018YFC1312001 and 2016YFC0901504), the National Natural Science Fund of China (Grant No. 81971188), the Science and Technology Bureau Fund of Sichuan Province (No. 2019YFS0216), and the 1.3.5 project for disciplines of excellence, West China Hospital, Sichuan University (Grant No. 2019HXFH046).
Conflict of Interest
The authors declare that the research was conducted in the absence of any commercial or financial relationships that could be construed as a potential conflict of interest.
Publisher’s Note
All claims expressed in this article are solely those of the authors and do not necessarily represent those of their affiliated organizations, or those of the publisher, the editors, and the reviewers. Any product that may be evaluated in this article or claim that may be made by its manufacturer is not guaranteed or endorsed by the publisher.
Acknowledgments
The authors thank the patients and healthy controls for their participation in the study. And this study could help to explore the underlying mechanism for the effect of inflammation on neurodegenerative diseases and further develop new therapeutic strategies for PD and MSA.
Supplementary Material
The Supplementary Material for this article can be found online at: https://www.frontiersin.org/articles/10.3389/fgene.2021.765833/full#supplementary-material
References
Amundsen, S. S., Viken, M. K., Sollid, L. M., and Lie, B. A. (2014). Coeliac Disease-Associated Polymorphisms Influence Thymic Gene Expression. Genes Immun. 15 (6), 355–360. doi:10.1038/gene.2014.26
Anderson, C. A., Boucher, G., Lees, C. W., Franke, A., D'Amato, M., Taylor, K. D., et al. (2011). Meta-analysis Identifies 29 Additional Ulcerative Colitis Risk Loci, Increasing the Number of Confirmed Associations to 47. Nat. Genet. 43 (3), 246–252. doi:10.1038/ng.764
Blauwendraat, C., Nalls, M. A., and Singleton, A. B. (2020). The Genetic Architecture of Parkinson's Disease. Lancet Neurol. 19 (2), 170–178. doi:10.1016/s1474-4422(19)30287-x
Bonifati, V., Rohe, C. F., Breedveld, G. J., Fabrizio, E., De Mari, M., Tassorelli, C., et al. (2005). Early-onset Parkinsonism Associated with PINK1 Mutations: Frequency, Genotypes, and Phenotypes. Neurology 65 (1), 87–95. doi:10.1212/01.wnl.0000167546.39375.82
Braak, H., de Vos, R. A. I., Bohl, J., and Del Tredici, K. (2006). Gastric α-synuclein Immunoreactive Inclusions in Meissner's and Auerbach's Plexuses in Cases Staged for Parkinson's Disease-Related Brain Pathology. Neurosci. Lett. 396 (1), 67–72. doi:10.1016/j.neulet.2005.11.012
Brás, I. C., Xylaki, M., and Outeiro, T. F. (2020). Mechanisms of Alpha-Synuclein Toxicity: An Update and Outlook. Prog. Brain Res. 252, 91–129. doi:10.1016/bs.pbr.2019.10.005
Cao, B., Chen, Y., Zhou, Q., Zhang, L., Ou, R., Wei, Q., et al. (2018). Functional Variant Rs3135500 in NOD2 Increases the Risk of Multiple System Atrophy in a Chinese Population. Front. Aging Neurosci. 10, 150. doi:10.3389/fnagi.2018.00150
Carabotti, M., Scirocco, A., Maselli, M. A., and Severi, C. (2015). The Gut-Brain axis: Interactions between Enteric Microbiota, central and Enteric Nervous Systems. Ann. Gastroenterol. 28 (2), 203–209.
Chang, D., Nalls, M. A., Nalls, M. A., Hallgrímsdóttir, I. B., Hunkapiller, J., van der Brug, M., et al. (2017). A Meta-Analysis of Genome-wide Association Studies Identifies 17 New Parkinson's Disease Risk Loci. Nat. Genet. 49 (10), 1511–1516. doi:10.1038/ng.3955
Chen, K., Tang, Y., Zhao, X., Hou, C., Li, G., and Zhang, B. (2020a). Association of IL1R2 Rs34043159 with Sporadic Alzheimer's Disease in Southern Han Chinese. Eur. J. Neurol. 27 (10), 1844–1847. doi:10.1111/ene.14319
Chen, X., Xiao, Y., Guo, W., Zhou, M., Huang, S., Mo, M., et al. (2019). Relationship between Variants of 17 Newly Loci and Parkinson's Disease in a Chinese Population. Neurobiol. Aging 73, e231–e234. doi:10.1016/j.neurobiolaging.2018.08.017
Chen, Y.-P., Ou, R.-W., Gu, X.-J., Zhang, L.-Y., Cao, B., Hou, Y.-B., et al. (2021). Multivariable Clinical-Genetic Model for Predicting Dyskinesia in Early-Onset Parkinson's Disease. Transl Neurodegener 10 (1), 26. doi:10.1186/s40035-021-00251-4
Chen, Y., Gu, X., Ou, R., Zhang, L., Hou, Y., Liu, K., et al. (2020b). Evaluating the Role of SNCA , LRRK2 , and GBA in Chinese Patients with Early‐Onset Parkinson's Disease. Mov Disord. 35 (11), 2046–2055. doi:10.1002/mds.28191
Chen, Y. P., Zhao, B., Cao, B., Song, W., Guo, X., Wei, Q.-Q., et al. (2015). Mutation Scanning of the COQ2 Gene in Ethnic Chinese Patients with Multiple-System Atrophy. Neurobiol. Aging 36 (2), e1227–e1222. doi:10.1016/j.neurobiolaging.2014.09.010
De Virgilio, A., Greco, A., Fabbrini, G., Inghilleri, M., Rizzo, M. I., Gallo, A., et al. (2016). Parkinson's Disease: Autoimmunity and Neuroinflammation. Autoimmun. Rev. 15 (10), 1005–1011. doi:10.1016/j.autrev.2016.07.022
Dorsey, E. R., Constantinescu, R., Thompson, J. P., Biglan, K. M., Holloway, R. G., Kieburtz, K., et al. (2007). Projected Number of People with Parkinson Disease in the Most Populous Nations, 2005 through 2030. Neurology 68 (5), 384–386. doi:10.1212/01.wnl.0000247740.47667.03
Drobny, A., Ngo, P. A., Neurath, M. F., Zunke, F., and López-Posadas, R. (2021). Molecular Communication between Neuronal Networks and Intestinal Epithelial Cells in Gut Inflammation and Parkinson's Disease. Front. Med. 8, 655123. doi:10.3389/fmed.2021.655123
Duan, R.-S., Liu, P.-P., Xi, F., Wang, W.-H., Tang, G.-B., Wang, R.-Y., et al. (2018). Wnt3 and Gata4 Regulate Axon Regeneration in Adult Mouse DRG Neurons. Biochem. Biophysical Res. Commun. 499 (2), 246–252. doi:10.1016/j.bbrc.2018.03.138
Dubois, P. C. A., Trynka, G., Franke, L., Hunt, K. A., Romanos, J., Curtotti, A., et al. (2010). Multiple Common Variants for Celiac Disease Influencing Immune Gene Expression. Nat. Genet. 42 (4), 295–302. doi:10.1038/ng.543
Dupont, W. D., and Plummer, W. D. (1990). Power and Sample Size Calculations. Controlled Clin. Trials 11 (2), 116–128. doi:10.1016/0197-2456(90)90005-m
Dzamko, N., Gysbers, A., Perera, G., Bahar, A., Shankar, A., Gao, J., et al. (2017). Toll-like Receptor 2 Is Increased in Neurons in Parkinson's Disease Brain and May Contribute to Alpha-Synuclein Pathology. Acta Neuropathol. 133 (2), 303–319. doi:10.1007/s00401-016-1648-8
Fang, H.-S., Wang, C.-C., Chao, C.-Y., Fan, W.-L., Su, S.-C., and Wu, Y.-R. (2021). Association of ITPKB, IL1R2 and COQ7 with Parkinson's Disease in Taiwan. J. Formos. Med. Assoc. doi:10.1016/j.jfma.2021.06.016
Franke, A., McGovern, D. P., Barrett, J. C., Wang, K., Radford-Smith, G. L., Ahmad, T., et al. (2010). Genome-wide Meta-Analysis Increases to 71 the Number of Confirmed Crohn's Disease Susceptibility Loci. Nat. Genet. 42 (12), 1118–1125. doi:10.1038/ng.717
Gao, T., Zheng, R., Ruan, Y., Fang, Y., Jin, C., Cao, J., et al. (2020). Association of ZNF184, IL1R2, LRRK2, ITPKB, and PARK16 with Sporadic Parkinson's Disease in Eastern China. Neurosci. Lett. 735, 135261. doi:10.1016/j.neulet.2020.135261
Gelders, G., Baekelandt, V., and Van der Perren, A. (2018). Linking Neuroinflammation and Neurodegeneration in Parkinson's Disease. J. Immunol. Res. 2018, 1–12. doi:10.1155/2018/4784268
Gilman, S., Wenning, G. K., Low, P. A., Brooks, D. J., Mathias, C. J., Trojanowski, J. Q., et al. (2008). Second Consensus Statement on the Diagnosis of Multiple System Atrophy. Neurology 71 (9), 670–676. doi:10.1212/01.wnl.0000324625.00404.15
González-Fernández, C., Fernández-Martos, C. M., Shields, S. D., Arenas, E., and Javier Rodríguez, F. (2014). Wnts Are Expressed in the Spinal Cord of Adult Mice and Are Differentially Induced after Injury. J. Neurotrauma 31 (6), 565–581. doi:10.1089/neu.2013.3067
Gorecki, A. M., Bakeberg, M. C., Theunissen, F., Kenna, J. E., Hoes, M. E., Pfaff, A. L., et al. (2020). Single Nucleotide Polymorphisms Associated with Gut Homeostasis Influence Risk and Age-At-Onset of Parkinson's Disease. Front. Aging Neurosci. 12, 603849. doi:10.3389/fnagi.2020.603849
Hara, K., Momose, Y., Tokiguchi, S., Shimohata, M., Terajima, K., Onodera, O., et al. (2007). Multiplex Families with Multiple System Atrophy. Arch. Neurol. 64 (4), 545–551. doi:10.1001/archneur.64.4.545
Hoffmann, A., Ettle, B., Battis, K., Reiprich, S., Schlachetzki, J. C. M., Masliah, E., et al. (2019). Oligodendroglial α‐synucleinopathy‐driven Neuroinflammation in Multiple System Atrophy. Brain Pathol. 29 (3), 380–396. doi:10.1111/bpa.12678
Hu, X., Mao, C., Hu, Z., Zhang, Z., Zhang, S., Yang, Z., et al. (2020). Association Analysis of 15 GWAS-Linked Loci with Parkinson's Disease in Chinese Han Population. Neurosci. Lett. 725, 134867. doi:10.1016/j.neulet.2020.134867
Hughes, A. J., Daniel, S. E., Kilford, L., and Lees, A. J. (1992). Accuracy of Clinical Diagnosis of Idiopathic Parkinson's Disease: a Clinico-Pathological Study of 100 Cases. J. Neurol. Neurosurg. Psychiatry 55 (3), 181–184. doi:10.1136/jnnp.55.3.181
Itoh, K., Kasai, T., Tsuji, Y., Saito, K., Mizuta, I., Harada, Y., et al. (2014). Definite Familial Multiple System Atrophy with Unknown Genetics. Neuropathology 34 (3), 309–313. doi:10.1111/neup.12092
Jellinger, K. A. (2014). Neuropathology of Multiple System Atrophy: New Thoughts about Pathogenesis. Mov Disord. 29 (14), 1720–1741. doi:10.1002/mds.26052
Jiang, S., Wang, Y., Gao, H., Luo, Q., Wang, D., Li, Y., et al. (2019). Cell Ratio Differences in Peripheral Blood between Early- and Late-Onset Parkinson's Disease: A Case-Control Study. Biomed. Res. Int. 2019, 1–6. doi:10.1155/2019/2072635
Karch, C. M., and Goate, A. M. (2015). Alzheimer's Disease Risk Genes and Mechanisms of Disease Pathogenesis. Biol. Psychiatry 77 (1), 43–51. doi:10.1016/j.biopsych.2014.05.006
Lema Tomé, C. M., Tyson, T., Rey, N. L., Grathwohl, S., Britschgi, M., and Brundin, P. (2013). Inflammation and α-Synuclein's Prion-like Behavior in Parkinson's Disease-Is There a Link? Mol. Neurobiol. 47 (2), 561–574. doi:10.1007/s12035-012-8267-8
Li, G., Cui, S., Du, J., Liu, J., Zhang, P., Fu, Y., et al. (2018). Association of GALC, ZNF184, IL1R2 and ELOVL7 with Parkinson's Disease in Southern Chinese. Front. Aging Neurosci. 10, 402. doi:10.3389/fnagi.2018.00402
Liu, X., Cheng, R., Verbitsky, M., Kisselev, S., Browne, A., Mejia-Sanatana, H., et al. (2011). Genome-wide Association Study Identifies Candidate Genes for Parkinson's Disease in an Ashkenazi Jewish Population. BMC Med. Genet. 12, 104. doi:10.1186/1471-2350-12-104
Lu, Y., Quan, C., Chen, H., Bo, X., and Zhang, C. (2017). 3DSNP: a Database for Linking Human Noncoding SNPs to Their Three-Dimensional Interacting Genes. Nucleic Acids Res. 45 (D1), D643–d649. doi:10.1093/nar/gkw1022
McGeer, P. L., and McGeer, E. G. (2004). Inflammation and Neurodegeneration in Parkinson's Disease. Parkinsonism Relat. Disord. 10 (Suppl. 1), S3–S7. doi:10.1016/j.parkreldis.2004.01.005
Nalls, M. A., Blauwendraat, C., Vallerga, C. L., Heilbron, K., Bandres-Ciga, S., Chang, D., et al. (2019). Identification of Novel Risk Loci, Causal Insights, and Heritable Risk for Parkinson's Disease: a Meta-Analysis of Genome-wide Association Studies. Lancet Neurol. 18 (12), 1091–1102. doi:10.1016/s1474-4422(19)30320-5
Nalls, M. A., Pankratz, N., Pankratz, N., Lill, C. M., Do, C. B., Hernandez, D. G., et al. (2014). Large-scale Meta-Analysis of Genome-wide Association Data Identifies Six New Risk Loci for Parkinson's Disease. Nat. Genet. 46 (9), 989–993. doi:10.1038/ng.3043
Ohama, E., and Ikuta, F. (1976). Parkinson's Disease: Distribution of Lewy Bodies and Monoamine Neuron System. Acta Neuropathol. 34 (4), 311–319. doi:10.1007/BF00696560
Pellegrini, C., Antonioli, L., Colucci, R., Blandizzi, C., and Fornai, M. (2018). Interplay Among Gut Microbiota, Intestinal Mucosal Barrier and Enteric Neuro-Immune System: a Common Path to Neurodegenerative Diseases? Acta Neuropathol. 136 (3), 345–361. doi:10.1007/s00401-018-1856-5
Phani, S., Loike, J. D., and Przedborski, S. (2012). Neurodegeneration and Inflammation in Parkinson's Disease. Parkinsonism Relat. Disord. 18 (Suppl. 1), S207–S209. doi:10.1016/S1353-8020(11)70064-5
Podvinec, M., Kaufmann, M. R., Handschin, C., and Meyer, U. A. (2002). NUBIScan, an In Silico Approach for Prediction of Nuclear Receptor Response Elements. Mol. Endocrinol. 16 (6), 1269–1279. doi:10.1210/mend.16.6.0851
Puschmann, A. (2013). Monogenic Parkinson's Disease and Parkinsonism: Clinical Phenotypes and Frequencies of Known Mutations. Parkinsonism Relat. Disord. 19 (4), 407–415. doi:10.1016/j.parkreldis.2013.01.020
Schain, M., and Kreisl, W. C. (2017). Neuroinflammation in Neurodegenerative Disorders-A Review. Curr. Neurol. Neurosci. Rep. 17 (3), 25. doi:10.1007/s11910-017-0733-2
Spielman, L. J., Gibson, D. L., and Klegeris, A. (2018). Unhealthy Gut, Unhealthy Brain: The Role of the Intestinal Microbiota in Neurodegenerative Diseases. Neurochem. Int. 120, 149–163. doi:10.1016/j.neuint.2018.08.005
Vörös, P., Sziksz, E., Himer, L., Ónody, A., Pap, D., Frivolt, K., et al. (2013). Expression of PARK7 Is Increased in Celiac Disease. Virchows Arch. 463 (3), 401–408. doi:10.1007/s00428-013-1443-z
Williams‐Gray, C. H., Wijeyekoon, R., Yarnall, A. J., Lawson, R. A., Breen, D. P., Evans, J. R., et al. (2016). S Erum Immune Markers and Disease Progression in an Incident P Arkinson's Disease Cohort ( ICICLE‐PD ). Mov Disord. 31 (7), 995–1003. doi:10.1002/mds.26563
Witoelar, A., Jansen, I. E., Wang, Y., Desikan, R. S., Gibbs, J. R., Blauwendraat, C., et al. (2017). Genome-wide Pleiotropy between Parkinson Disease and Autoimmune Diseases. JAMA Neurol. 74 (7), 780–792. doi:10.1001/jamaneurol.2017.0469
Wullner, U., Abele, M., Schmitz-Huebsch, T., Wilhelm, K., Benecke, R., Deuschl, G., et al. (2004). Probable Multiple System Atrophy in a German Family. J. Neurol. Neurosurg. Psychiatry 75 (6), 924–925. doi:10.1136/jnnp.2003.025155
Keywords: parkinsion’s disease, multiple system atrophy, Wnt3, HLA-DRB5, IL1R2, SNP
Citation: Su W-M, Gu X-J, Hou Y-B, Zhang L-Y, Cao B, Ou R-W, Wu Y, Chen X-P, Song W, Zhao B, Shang H-F and Chen Y-P (2021) Association Analysis of WNT3, HLA-DRB5 and IL1R2 Polymorphisms in Chinese Patients With Parkinson’s Disease and Multiple System Atrophy. Front. Genet. 12:765833. doi: 10.3389/fgene.2021.765833
Received: 27 August 2021; Accepted: 29 October 2021;
Published: 18 November 2021.
Edited by:
Jian-Hong Zhu, Wenzhou Medical University, ChinaReviewed by:
Ryan Scott Anderton, University of Notre Dame Australia, AustraliaJun Mitsui, The University of Tokyo, Japan
Copyright © 2021 Su, Gu, Hou, Zhang, Cao, Ou, Wu, Chen, Song, Zhao, Shang and Chen. This is an open-access article distributed under the terms of the Creative Commons Attribution License (CC BY). The use, distribution or reproduction in other forums is permitted, provided the original author(s) and the copyright owner(s) are credited and that the original publication in this journal is cited, in accordance with accepted academic practice. No use, distribution or reproduction is permitted which does not comply with these terms.
*Correspondence: Hui-Fang Shang, hfshang2002@126.com; Yong-Ping Chen, yongping.chen@wchscu.cn