- Department of Urology, Cancer Hospital of China Medical University, Liaoning Cancer Hospital and Institute, Shenyang, China
N6-methyladenosine (m6A) is a dynamic, reversible post-transcriptional modification, and the most common internal modification of eukaryotic messenger RNA (mRNA). Considerable evidence now shows that m6A alters gene expression, thereby regulating cell self-renewal, differentiation, invasion, and apoptotic processes. M6A methylation disorders are directly related to abnormal RNA metabolism, which may lead to tumor formation. M6A methyltransferase is the dominant catalyst during m6A modification; it removes m6A demethylase, promotes recognition by m6A binding proteins, and regulates mRNA metabolic processes. Bladder cancer (BC) is a urinary system malignant tumor, with complex etiology and high incidence rates. A well-differentiated or moderately differentiated pathological type at initial diagnosis accounts for most patients with BC. For differentiated superficial bladder urothelial carcinoma, the prognosis is normally good after surgery. However, due to poor epithelial cell differentiation, BC urothelial cell proliferation and infiltration may lead to invasive or metastatic BC, which lowers the 5-years survival rate and significantly affects clinical treatments in elderly patients. Here, we review the latest progress in m6A RNA methylation research and investigate its regulation on BC occurrence and development.
Introduction
Bladder cancer (BC) is one of the most common malignant tumors of the urinary system; it ranks first among urological tumors in terms of incidence rate, and is the 9th highest incidence cancer in the world (Lenis et al., 2020; Li et al., 2021a). In recent years, BC treatment strategies have improved such that surgical resection combined with radiotherapy or chemotherapy are highly effective treatments (Jain et al., 2021; Tran et al., 2021). However, while immunotherapy has demonstrated strong prospects for solid tumor treatment, it remains to be clinically applied to BC (Afonso et al., 2020; van Puffelen et al., 2020; Wu and Abraham 2021). Immunotherapy is limited as it inhibits non-muscular and muscular invasive BC at the laboratory level (Schneider et al., 2019; Witjes et al., 2021). For patients with non-muscle invasive tumors (NMIBC), transurethral resection combined with postoperative bladder perfusion chemotherapy or BCG treatment strategies is usually adopted (Charpentier et al., 2021; Li et al., 2021e). However, 20–30% of NMIBC patients will progress to muscle invasive bladder cancer (MIBC), and 50% will develop distant metastases within 2 years of radical surgery (Jiang et al., 2021a; Liu et al., 2021a). For locally advanced or advanced MIBC patients, gemcitabine combined with cisplatin (GC regimen) remains the standard treatment, however, BC fatality rates have only dropped by 1.5% in the past 15 years (Kaur et al., 2021; Roviello et al., 2021). Due to its high recurrence and metastasis rate, the 5-years survival rate for patients with MIBC remains very low (Meeks et al., 2020; Patel et al., 2020; Jiang et al., 2021a). Therefore, while novel treatment strategies must be explored and BC molecular mechanisms clarified, recent evidence has suggested that m6A mechanisms actively participate in BC (Mu et al., 2021).
N6-methyladenosine (m6A) is one of the most common internal transcription modifications in eukaryotic messenger RNA (mRNA) (Huang et al., 2021; Oerum et al., 2021). The molecule was first identified in the 1970s, but recent studies have shown that m6A-associated mutations are closely related to BC occurrence (Liu et al., 2021b). In 2011, the fat-mass and obesity-associated protein (FTO) was reported to have functions in m6A demethylase and suggested that m6A modification was dynamically reversible (Zheng et al., 2020; Gu et al., 2021a; Tan et al., 2021; Zhao et al., 2021). Studies have since summarized the related modifications of m6A as methyltransferase complexes, demethylases, and corresponding readers coordinated regulation, which are classified as “writers,” “erasers,” and “readers,” respectively (Tang et al., 2021). M6A is abundant in 3′ untranslated regions (UTRs), stop codons, and long exon regions. The process has a high degree of evolutionary conservation, but with unclear biological functions (Yao et al., 2021; Zhao et al., 2021). M6A is co-catalyzed by the methylation modification enzymes, METTL3 and METL14. Also, WTAP and KIAA1429 function as m6A regulators to participate in catalytic processes (Wu et al., 2020; He and He 2021). Interestingly, the METTL3-METTL14 complex is more potent than individual components in catalyzing m6A formation (Song et al., 2021a; Uddin et al., 2021). M6A methylation is also demethylated by the FTO and AlkB homolog 5 (ALKBH5) demethylases (Ye et al., 2021). M6A modification is involved in all mRNA metabolic processes, including maturation, transport, splicing, translation, and degradation (Song et al., 2020; Li et al., 2021b Li et al., 2021c; Lou et al., 2021). M6A RNA methylation exerts critical biological functions in mammals, such as tissue development, circadian rhythms, DNA damage responses, gender identification, and tumor occurrence and development (Xu et al., 2020a; Li et al., 2020; Ma and Ji 2020; Gu et al., 2021b; Wu and Wang 2021). In this review, we discuss the potential mechanisms of m6A methylation-related regulators in BC initiation and development.
M6A Methylation Regulators
M6A modification adds a methyl group to the N6 position of adenosine and is an evolutionarily conserved RNA modification (Han and Choe 2020; Zhang et al., 2020; Zhou et al., 2020). Approximately 0.3% of adenosine in mRNA is modified by m6A, with an average of three m6A modification sites in every transcript. M6A methylation mainly occurs in RRACH sequences (where R = A or G, H = A, C, or U), stop codons, 3′UTRs, and internal long exons, to regulate RNA transcription, processing, translation, and metabolism (Huang et al., 2020a; Chen and Wong 2020; Liang et al., 2020; Scarrow et al., 2020). The modification is controlled by m6A regulatory enzymes, amongst which, methyltransferases or m6A “writers” actively catalyze modifications, m6A “erasers,” with demethylase activity, eliminate m6A modifications, and m6A “readers” recognize modification (He et al., 2019; Huang et al., 2020b; Lee et al., 2020; Zhao et al., 2020) bases and convey information, thereby establishing an efficient and orderly m6A regulatory network (Figure 1).
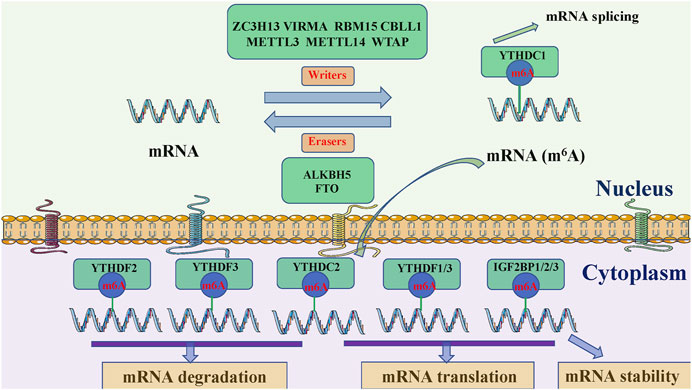
FIGURE 1. Potential m6A methylation mechanisms in RNA. M6A methylation is catalyzed by the writer complex, including METTL3, METTL14, WTAP, VIRMA, RBM15, ZC3H13, and CBLL1. The demethylases, FTO and ALKBH5 remove m6A modifications. Reader proteins (YTHDC1, YTHDF2, YTHDF3, YTHDC2, YTHDF1/3, and IGF2BP1/2/3) recognize m6A and determine target RNA targets. METTL3, methyltransferase 3, N6-adenosine-methyltransferase complex catalytic subunit; METTL14, methyltransferase 14, N6-adenosine-methyltransferase subunit; WTAP, WT1 associated protein; VIRMA, vir like m6A methyltransferase associated; RBM15, RNA binding motif protein 15; ZC3H13, zinc finger CCCH-type containing 13; CBLL1, Cbl proto-oncogene like 1; FTO, FTO α-ketoglutarate dependent dioxygenase; ALKBH5, alkB homolog 5, RNA demethylase; YTHDC1/2, YTH domain containing 1/2; YTHDF1/2/3, YTH N6-methyladenosine RNA binding protein 1/2/3; IGF2BP1/2/3, insulin like growth factor 2 mRNA binding protein 1/2/3.
The methyltransferase complex primarily includes methyltransferase-like 3 (METTL3), METTL14, vir like m6A methyltransferase associated (VIRMA), RNA binding motif protein 15 (RBM15), zinc finger CCCH-type containing 13 (ZC3H13), Cbl proto-oncogenes like 1 (CBLL1), and Wilm’s tumor 1-associated protein (WTAP). All proteins co-ordinate and regulate m6A control (Chen et al., 2019a; Ma et al., 2019; Williams et al., 2019). METTL3 functions as a core component where METTL14 combines with it to form a stable heterodimer to catalyze m6A RNA methylation via synergistic effects (Chen et al., 2019b; Yue et al., 2019). WTAP anchors the METTL3/14 complex on target RNA and promotes its nuclear accumulation (Lan et al., 2019; Liu et al., 2019). The KIAA1429-RBM15 complex was recently verified as a new component of the m6A “writer” complex, while RBM15 recruits the complex to target sites (Niu et al., 2018; Wang et al., 2018). METTL16 is also a novel m6A molecule targeting U6 small nuclear RNA (snRNA) and regulates S-adenosylmethionine homeostasis by elevating S-adenosylmethionine synthase expression during methionine starvation (Frye et al., 2018; Yang et al., 2018; Zhang 2018).
M6A demethylases include FTO and ALKBH5. FTO was identified as regulating steady-state energy levels and positively correlating with obesity risk (Deng et al., 2018a; Huang and Yin 2018). ALKBH5 is a homolog of FTO, and belongs to the Fe2+and α-ketoglutarate-dependent AlkB oxygenase family (Deng et al., 2018b; Dai et al., 2018). FTO and ALKBH5 both recognize m6A-modified nuclear RNA as a substrate, and catalyze the removal of m6A methyl modifications (Meyer and Jaffrey 2017; Wang et al., 2017).
M6A reading proteins are divided into three categories: proteins contain an evolutionarily conserved YTH domain which folds into a hydrophobic aromatic structure directly binding to m6A (Liao et al., 2018; Patil et al., 2018). YTH domain proteins are composed of YTHDF (YTHDF1, YTHDF2, and YTHDF3) and YTHDC subtypes (YTHDC1 and YTHDC2). YTHDF subtype proteins are mainly distributed in the cytoplasm.
Heterogeneous nuclear ribonucleoproteins (hnRNPs) mainly include three types, namely hnRNPC, hnRNPG, and hnRNPA2B1. The “m6A switch” phenomenon disrupts RNA hairpin structures and exposes single-stranded hnRNP binding motifs (Aguilo and Walsh 2017; Wu et al., 2017). These proteins bind to transcripts containing m6A via the m6A switch, thereby affecting mRNA localization and alternative splicing (Batista 2017; Roignant and Soller 2017).
Insulin-like growth factor 2 mRNA-binding proteins 1–3 (IGF2BP1–3) also recognize the GGC (m6A) sequences via the K homology domain, and enhance the stability and translation of downstream mRNAs in an m6A-dependent manner under normal and stress conditions (Adhikari et al., 2016).
M6A Roles and Disease Mechanisms
The potential roles and mechanisms of m6A-related regulators are shown (Figure 2).
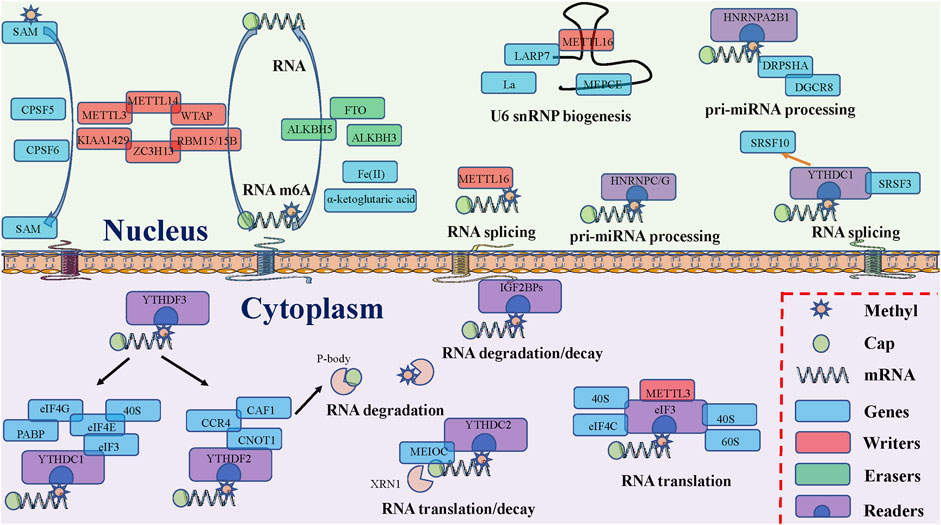
FIGURE 2. The potential functions of RNA m6A modification related proteins. “Writers,” “Erasers”, and “Readers” rely on several crucial factors to install, remove, and recognize m6A modifications and participate in various RNA metabolism steps, including splicing, export, translation, degradation, and decay.
M6A Methyltransferases
RNA m6A methylation is controlled by METTL3, METTL14, WTAP, RBM15 RBM15B, and Cbl proto-oncogene E3, and is catalyzed by methyltransferase complexes composed of CBLL1, VIRMA, and ZC3H13. Of these proteins, METTL3 and METTL14 exhibit m6A methyltransferase activity (Zhou et al., 2021a; Maldonado Lopez and Capell 2021; Pan et al., 2021). WTAP promotes m6A functionby recruiting METTL3 and METTL14 into nuclear speckles (Deng et al., 2021). RBM15 and RBM15B bind METTL3 and WTAP and guides them to specific RNA sites for m6A modification (Meng et al., 2021). VIRMA preferentially mediates mRNA methylation near 3′UTR and stop codon regions (Zhu et al., 2021a). ZC3H13, together with other cofactors such as WTAP, control nuclear m6A methylation (Zhou et al., 2021b).
METTL16 is a novel RNA methyltransferase that independently induces the m6A modification of the 3′UTR of mRNAs (Satterwhite and Mansfield 2021), which have crucial roles in maintaining mRNA stability and splicing. M6A methyltransferases also display carcinogenic roles in several cancers. METTL3-induced miR-222-3p up-regulation suppresses STK4 and promotes malignant behaviors in thyroid carcinoma cells (Lin et al., 2021). METTL3 also up-regulates the m6A modification of adenomatous polyposis coli (APC), leading to its mRNA degradation. Decreased APC increases β-catenin, cyclin D1, c-Myc, and PKM2 expression, resulting in mouse aerobic glycolysis, cell proliferation, and enhanced esophageal squamous cell carcinoma (ESCC) formation (Wang et al., 2021a). METTL3 also induces PLX4032 resistance to melanoma by promoting m6A-dependent EGFR translation (Bhattarai et al., 2021). METTL14 also aggravates podocyte injury and glomerulopathy progression via N-methyladenosine-dependent Sirt1 down-regulation (Lu et al., 2021). METTL14 promotes glomerular endothelial cell injury and diabetic nephropathy via m6A modification of the α-klotho protein (Li et al., 2021d). METTL16 promotes cell proliferation by up-regulating cyclin D1 expression in gastric cancer (Wang et al., 2021b). WTAP up-regulation reduces PERP levels via m6A modification, which in turn promotes pancreatic cancer growth and metastasis (Wang et al., 2020a). WTAP expression is significantly increased in HCC and promotes liver cancer development. WTAP-guided m6A modifications may also promote HCC progression via the HuR-ETS1-p21/p27 regulatory axis (Chen et al., 2019c).
M6A Demethyltransferase
RNA m6A methylation is a reversible process, with its demethylation reliant on demethylases. FTO catalyzes m6A demethylation and displays strict substrate selectivity near alternatively spliced exons and poly-A sites (Lan et al., 2020; Jiang et al., 2021b; He and He 2021). ALKBH5 functions with FTO to ensure balanced m6A modifications in the transcriptome (Chen et al., 2021a; Purslow et al., 2021; Wu et al., 2021). ALKBH3 was identified as another m6A demethylase with easier binding to tRNA m6A sites than mRNA or rRNA sites (Esteve-Puig et al., 2021; Wollen et al., 2021). FTO expression is increased in breast cancer and promotes cell growth and metastasis (Niu et al., 2019). FTO also mediates m6A demethylation in the 3′UTR of BNIP3 mRNA and induces its degradation via a YTHDF2 independent manner. The FTO-mediated epigenetic up-regulation of LINC00022 also promotes tumorigenesis in ESCCs (Cui et al., 2021). ALKBH5-HOXA10 loop-mediated JAK2 m6A demethylation causes cisplatin resistance in epithelial ovarian cancer (Nie et al., 2021). ALKBH5 promotes the cadmium-induced transformation of human bronchial epithelial cells by regulating PTEN expression in an m6A-dependent manner (Li et al., 2021e). ALKBH5 was also identified in cell and animal models as related to patient prognoses and the suppression of esophageal cancer malignancies. The protein also demethylates pri-miR-194-2 and inhibits it in an m6A/DGCR8-dependent manner (Chen et al., 2021b).
M6A Binding Proteins
M6A modifications exert biological functions by binding to m6A-binding proteins (Dai et al., 2021; Tsuchiya et al., 2021). YTHDF1 knockout reduces the overall level of IFN-induced A-to-I RNA editing, thereby activating the Double stranded RNA sensing pathway and promoting IFN-stimulated gene expression (Terajima et al., 2021). YTHDF1 deficiency also inhibits viral replication in cells by modulating IFN responses. YTHDF2 inhibits cardiac hypertrophy through a Myh7 mRNA decoy in an m6A-dependent manner (Xu et al., 2021). YTHDF1 also correlates with the immune microenvironment and predicts clinical outcomes and therapeutic efficacy in breast cancer (Hu et al., 2021). YTHDF1 and YTHDF2 are associated with better patient survival rates and an inflamed tumor-immune microenvironment in non-small-cell lung cancer (Tsuchiya et al., 2021). Highly expressed YTHDF3 promotes cancer cell interactions with brain endothelial cells and astrocytes, blood-brain barrier extravasation, angiogenesis, and growth (Chang et al., 2020). Mechanistically, YTHDF3 enhances the translation of m6A-rich ST6GALNAC5, GJA1, and EGFR transcripts. MiR-30d is a new target modified by YTHDC1 via m6A, with miR-30d inhibiting pancreatic tumors by inhibiting aerobic glycolysis (Hou et al., 2021). YTHDC2 contains an RNA helicase domain, recognizes m6A methylated adenosine at nucleotide 331, and cooperates with the cellular La antigen to support HCV IRES-dependent translation (Kim and Siddiqui 2021).
M6A Roles and Mechanisms in BC
Recent studies reported that m6A-modified mRNA is dysregulated in several cancers, with in vivo and in vitro anti-cancer effects identified. Dysregulated m6A-related factors may alter m6A modifications in tumors and interfere with cancer progression. In the following sections, we summarize m6A regulatory factor roles in BC (Table 1).
M6A Modification of Related Protein Expression Up-Regulates METTL3 in BC
METTL3 was the first discovered methyltransferase and forms a complex with METTL14 and WTAP to promote RNA methylation. METTL3 in human tissue is highly expressed and conserved, especially in the testes. Recent studies reported that METTL3 is significantly highly expressed in chronic myeloid leukemia (Ianniello et al., 2021), thymic epithelial tumors (Iaiza et al., 2021), esophageal cancer (Han et al., 2021), and prostate cancer (Chen et al., 2021b), suggesting a close relationship with malignant tumor development. Previous studies also suggested that METTL3 is significantly up-regulated in BC. METTL3 knockdown significantly reduces BC proliferation, invasion, and survival rates in vitro, and tumorigenicity in vivo. In contrast, METTL3 overexpression promotes BC cell growth and invasion (Cheng et al., 2019). AF4/FMR2 are two critical regulators of the NF-κB pathway (IKBKB and RELA) and MYC and were verified as downstream targets of METTL3-mediated m6A modification. Yang et al. reported that METTL3 and CDCP1 were up-regulated in BC tissue, and their expression levels were interrelated with respect to BC progression (Yang et al., 2019). METTL3-m6A-CDCP1 axis repression inhibits the growth and progression of chemically transformed and BC cells. This axis and chemical carcinogens exert a synergistic impact on promoting the malignant transformation of urothelial cells and BC occurrence. Han et al. (2019) indicated that METTL3 exerts carcinogenic effects in BC by interacting with DGCR8 and positively regulating pri-miR221/222 processes in an m6A-dependent manner. Xie et al. (2020) discovered that the tumor-promoting functions and specific regulatory mechanisms of the m6A axis are composed of the core “writer” protein, METTL3 and the main “reading” protein, YTHDF2. METTL3 consumption damages cancer proliferation and metastasis. The METTL3/YTHDF2 m6A axis directly degrades the mRNA of the tumor suppressors, SETD7 and KLF4 and promotes BC development. Ying et al. (2020) showed that the RCas9-METTL3 system mediates the effective site-specific m6A installation on CDCP1 mRNA and promotes BC progression. Wang et al. (2021c) suggested that METTL3 absence inhibits tyrosine kinase endothelium (TEK) and vascular endothelial growth factor A (VEGF-A) by reducing the abundance of m6A peaks at specific sites. METTL3 consumption down-regulates mRNA and protein expression levels of TEK and VEGF-A. Also, activation of TEK-VEGF-A-mediated tumor development and angiogenesis requires METTL3-mediated m6A modification. Mu et al. reported that cisplatin blocks G-CSF methylation by targeting METTL3 and reducing fibrocystic-myeloid-derived suppressor cells during IAIC (Mu, et al., 2021).
4.1.1 FTO
FTO is the first obesity susceptibility gene confirmed by whole genome scanning and is localized to human chromosome 16q 12.2, is approximately 430 kb, and contains nine exons and eight introns. The protein is widely expressed in the hypothalamus, adipose tissue, pancreatic islets, and other tissues (Zarza-Rebollo et al., 2021) (Zhou et al., 2021b). Song et al. (2021b) showed that USP18 post-translational deubiquitination up-regulates FTO protein expression, while FTO promotes BC occurrence and progression via its demethylase activity on PYCR1 to stabilize its transcript. Thus, the UPS18/FTO/PYCR1 signaling network could act as a potential therapeutic target for BC. In addition, FTO regulates the MALAT/miR-384/MAL2 axis via m6A RNA modification to initiate BC. Thus, FTO has the potential to be a prognostic biomarker for BC (Tao et al., 2021).
4.1.2 IGF2BP1
Several IGF2BP molecules were identified thanks to molecular detection and proteomic approaches. These proteins exert key biological roles in cell polarization, proliferation, migration, and differentiation, and are closely related to the development of many tumors (Bell et al., 2013). The IGF2BP family includes IGF2BP1, IGF2BP2, and IGF2BP3, and all of which are highly conserved onco-embryonic proteins mainly expressed in embryonic tissue. Their expression levels are extremely low, or negligible in adult tissue (Du et al., 2021). Xie et al. (2021) showed that IGF2BP1 binds circPTPRA in the BC cell cytoplasm, with the ectopic expression of circPTPRA eliminating the promotion of IGF2BP1-induced growth and metastasis in BC cells.
4.1.3 IGF2BP3
Huang et al. (2020c) reported that IGF2BP3 expression is elevated in BC tissue and is closely related to a poor prognosis in BC patients. Overexpressed IGF2BP3 significantly promotes cell cycle and BC cell proliferation by activating the JAK/STAT signaling pathway and inhibiting apoptosis.
4.2 M6A Modification of Related Protein Expression Down-Regulates METTL14 in BC
Gu at al. reported that METL14 expression decreases in BC and bladder tumor-initiating cells (TIC). METL14 knockout significantly promotes cell proliferation, self-renewal, metastasis, and tumor initiation of bladder TIC (Gu et al., 2019). METTL14 and m6A modifications are involved in Notch1 mRNA stability. In addition, isorhapontigenin reduces vimentin protein levels by increasing METTL14 expression and up-regulating METTL14 mRNA by activating the transcription factor, FOXO3a, thereby impacting on BC progression (Zhang et al., 2021).
4.2.1 FTO
Using real-time fluorescent quantitative PCR and TCGA analysis, Wen et al. (2020) observed that FTO mRNA expression levels in urothelial BC are significantly lower than normal tissue. FTO knockdown significantly promotes the proliferation and migration of 5,637 and T24 cells (Wen et al., 2020).
4.2.2 ALKBH5
ALKBH5 is an RNA demethylation modification enzyme during m6A modification processes (Cai et al., 2021; Peng et al., 2021; Wu, et al., 2021) and mainly reverses m6A methylation (Wang et al., 2020b; Cai et al., 2021). ALKBH5 is an Fe2+ and Q-ketoglutarate-dependent non-heme oxygenase, belongs to the ALKB family, and only displays demethylation activity for m6A modifications on single-stranded RNA/DNA. ALKBH5 exerts essential biological functions in several tumors and cancers. Jin et al. (2019) reported that METTL3 and ALKBH5 modulate ITGA6 expression in BC cells to alter cell adhesion, thereby indicating the carcinogenic effects of m6A-modified ITGA6 and its regulatory mechanisms on BC initiation and development. In addition, down-regulated ALKBH5 expression in BC tissue and cell lines is related to a poor prognosis in patients with BC. ALKBH5 knockdown promotes BC cell proliferation, migration, and invasion, and reduces ciplatin chemosensitivity (Yu et al., 2021). ALKBH5 inhibits cancer progression in an m6A-dependent manner via the glycolytic pathway as mediated by casein kinase 2, and promotes BC cell sensitivity to cisplatin.
4.3 M6A Methylation is a Putative Prognostic Biomarker for BC
The diagnostic value of m6A-related regulatory proteins in BC is summarized (Table 2). Chen et al. collected 62 fresh bladder transitional BC samples (BC group) and 20 normal bladder mucosa specimens (controls). When compared with controls, WTAP expression was significantly increased in the BC group (Chen and Wang 2018). These authors identified a significant difference in the risk of disease recurrence between patients with negative WTAP protein expression levels and those with positive expression. In addition, MTTL3 (Han, et al., 2019), ALKBH5 (Yu, et al., 2021), m6A (Gu, et al., 2019), IGF2BP3 (Huang et al., 2020c), and FTO (Tao, et al., 2021) levels are closely related to prognosis in BC patients. However, no research has yet analyzed the diagnostic potential of m6A-related regulatory protein expression levels in urine and plasma.
5 Perspectives and Conclusion
M6A methylation mechanism have greatly contributed to the field of epigenetics. Methyltransferases, demethylases, and reading proteins jointly regulate m6A levels in downstream genes, thereby promoting tumor initiation and progression (Xu et al., 2020b; Yan et al., 2021). M6A RNA methylation comprises the m6A methyltransferase, m6A demethylase, and m6A binding proteins which regulate mRNA precursor shear, mRNA stability, and translation. M6A RNA methylation is related to tumor cell growth, metastasis, and drug resistance. There is no doubt that m6A methylation has significant potential for the development of new human cancer therapies. Additionally, bioinformatics show that m6A participates in BC via multiple biological processes: m6A regulators contribute to malignant progression and impact on prognoses (Chen et al., 2019d), m6A contributes to tumor microenvironments (Zhu et al., 2021b), and m6A regulates lncRNA in BC carcinogenesis (Li et al., 2021f). Moreover, bioinformatics tools can be used to study associations between m6A and BC. RMVar (Luo et al., 2021) and RMdisease (Chen et al., 2021c) presented the m6A-associated mutations in BC, and the BC-associated m6A sites were estimated by the heterogeneous network in DURM (Tang et al., 2019). However, challenges remain. Mechanisms underpinning m6A modulators in certain cancers are unclear, especially as so few studies on m6A modified “readers” exist. The evidence suggests that m6A modulators and related pathways could function as therapeutic targets, therefore, more input from the clinic is required to verify these therapeutic effects. Moreover, m6A modified proteins have the dual effect of suppressing or causing cancer, thus controversial research results must be fully explored to characterize these discrepancies. However, in the era of next-generation sequencing, the generation and analysis of big data (omics) will expand and transform cancer biology.
In summary, thanks to high-throughput sequencing and other biotechnologies, a clear role of m6A methylation during BC has emerged. METTL3, METTL14, ALKBH5, FTO, YTHDF1/3, YTHDF2, IGF2BP1, and IGF2BP3 aberrant expression occur in BC, mainly affect mRNA stability, and regulate the growth and metastasis of tumor cells. However, many challenges remain. The role of epigenetic networks in BC initiation and progression requires further exploration. It is vital to fully evaluate the safety and effectiveness of m6A-related regulatory factors and pathways as novel tumor therapy targets. Furthermore, exploring correlations between m6A and BC drug sensitivity and long-term prognostics is also essential.
Author Contributions
Original draft preparation, allocation, revision, supplementation and editing: QL. All authors read and agreed to the final published version of the manuscript.
Conflict of Interest
The author declares that the research was conducted in the absence of any commercial or financial relationships that could be construed as a potential conflict of interest.
Publisher’s Note
All claims expressed in this article are solely those of the authors and do not necessarily represent those of their affiliated organizations, or those of the publisher, the editors and the reviewers. Any product that may be evaluated in this article, or claim that may be made by its manufacturer, is not guaranteed or endorsed by the publisher.
Acknowledgments
We thank the Liaoning Cancer Hospital and Institute (Shenyang).
Supplementary Material
The Supplementary Material for this article can be found online at: https://www.frontiersin.org/articles/10.3389/fgene.2021.825109/full#supplementary-material
Abbreviations
m6A, N6-methyladenosine; mRNA, messenger RNA; 3′UTR, 3′untranslated region; snRNA, small nuclear RNA; APC, adenomatous polyposis coli; METTL3, methyltransferase 3, N6-adenosine-methyltransferase complex catalytic subunit; METTL14, methyltransferase 14, N6-adenosine-methyltransferase subunit; WTAP, WT1 associated protein; VIRMA, vir like m6A methyltransferase associated; RBM15, RNA binding motif protein 15; ZC3H13, zinc finger CCCH-type containing 13; CBLL1, Cbl proto-oncogene like 1; FTO, FTO alpha-ketoglutarate dependent dioxygenase; ALKBH5, alkB homolog 5, RNA demethylase; YTHDC1/2, YTH domain containing 1/2; YTHDF1/2/3, YTH N6-methyladenosine RNA binding protein 1/2/3; IGF2BP1/2/3, insulin like growth factor 2 mRNA binding protein 1/2/3.
References
Adhikari, S., Xiao, W., Zhao, Y.-L., and Yang, Y.-G. (2016). m6A: Signaling for mRNA Splicing. RNA Biol. 13, 756–759. doi:10.1080/15476286.2016.1201628
Afonso, J., Santos, L. L., Longatto-Filho, A., and Baltazar, F. (2020). Competitive Glucose Metabolism as a Target to Boost Bladder Cancer Immunotherapy. Nat. Rev. Urol. 17, 77–106. doi:10.1038/s41585-019-0263-6
Aguilo, F., and Walsh, M. J. (2017). The N6-Methyladenosine RNA Modification in Pluripotency and Reprogramming. Curr. Opin. Genet. Development 46, 77–82. doi:10.1016/j.gde.2017.06.006
Batista, P. J. (2017). The RNA Modification N 6 -methyladenosine and its Implications in Human Disease. Genomics, Proteomics & Bioinformatics 15 (3), 154–163. doi:10.1016/j.gpb.2017.03.002
Bell, J. L., Wächter, K., Mühleck, B., Pazaitis, N., Köhn, M., Lederer, M., et al. (2013). Insulin-like Growth Factor 2 mRNA-Binding Proteins (IGF2BPs): post-transcriptional Drivers of Cancer Progression? Cell. Mol. Life Sci. 70, 2657–2675. doi:10.1007/s00018-012-1186-z
Bhattarai, P. Y., Kim, G., Poudel, M., Lim, S.-C., and Choi, H. S. (2021). METTL3 Induces PLX4032 Resistance in Melanoma by Promoting m6A-dependent EGFR Translation. Cancer Lett. 522, 44–56. doi:10.1016/j.canlet.2021.09.015
Cai, Y., Wu, G., Peng, B., Li, J., Zeng, S., Yan, Y., et al. (2021). Expression and Molecular Profiles of the AlkB Family in Ovarian Serous Carcinoma. Aging 13, 9679–9692. doi:10.18632/aging.202716
Chang, G., Shi, L., Ye, Y., Shi, H., Zeng, L., Tiwary, S., et al. (2020). YTHDF3 Induces the Translation of m6A-Enriched Gene Transcripts to Promote Breast Cancer Brain Metastasis. Cancer Cell 38, 857–871. doi:10.1016/j.ccell.2020.10.004
Charpentier, M., Gutierrez, C., Guillaudeux, T., Verhoest, G., and Pedeux, R. (2021). Noninvasive Urine-Based Tests to Diagnose or Detect Recurrence of Bladder Cancer. Cancers (Basel) 13 (7), 1650. doi:10.3390/cancers13071650
Chen, J., Fang, X., Zhong, P., Song, Z., and Hu, X. (2019a). N6-methyladenosine Modifications: Interactions with Novel RNA-Binding Proteins and Roles in Signal Transduction. RNA Biol. 16, 991–1000. doi:10.1080/15476286.2019.1620060
Chen, K., Song, B., Tang, Y., Wei, Z., Xu, Q., Su, J., et al. (2021a). RMDisease: a Database of Genetic Variants that Affect RNA Modifications, with Implications for Epitranscriptome Pathogenesis. Nucleic Acids Res. 49, D1396–D1404. doi:10.1093/nar/gkaa790
Chen, L., and Wang, X. (2018). Relationship between the Genetic Expression of WTAP and Bladder Cancer and Patient Prognosis. Oncol. Lett. 16, 6966–6970. doi:10.3892/ol.2018.9554
Chen, M., Nie, Z. Y., Wen, X. H., Gao, Y. H., Cao, H., and Zhang, S. F. (2019b). m6A RNA Methylation Regulators Can Contribute to Malignant Progression and Impact the Prognosis of Bladder Cancer. Biosci. Rep. 39, 12. doi:10.1042/BSR20192892
Chen, M., and Wong, C.-M. (2020). The Emerging Roles of N6-Methyladenosine (m6A) Deregulation in Liver Carcinogenesis. Mol. Cancer 19, 44. doi:10.1186/s12943-020-01172-y
Chen, P., Li, S., Zhang, K., Zhao, R., Cui, J., Zhou, W., et al. (2021b). N(6)-methyladenosine Demethylase ALKBH5 Suppresses Malignancy of Esophageal Cancer by Regulating microRNA Biogenesis and RAI1 Expression. Oncogene 40 (37), 5600–5612. doi:10.1038/s41388-021-01966-4
Chen, X.-Y., Zhang, J., and Zhu, J.-S. (2019c). The Role of m6A RNA Methylation in Human Cancer. Mol. Cancer 18, 103. doi:10.1186/s12943-019-1033-z
Chen, Y., Pan, C., Wang, X., Xu, D., Ma, Y., Hu, J., et al. (2021c). Silencing of METTL3 Effectively Hinders Invasion and Metastasis of Prostate Cancer Cells. Theranostics 11, 7640–7657. doi:10.7150/thno.61178
Chen, Y., Peng, C., Chen, J., Chen, D., Yang, B., He, B., et al. (2019d). WTAP Facilitates Progression of Hepatocellular Carcinoma via m6A-HuR-dependent Epigenetic Silencing of ETS1. Mol. Cancer 18, 127. doi:10.1186/s12943-019-1053-8
Cheng, M., Sheng, L., Gao, Q., Xiong, Q., Zhang, H., Wu, M., et al. (2019). The m6A Methyltransferase METTL3 Promotes Bladder Cancer Progression via AFF4/NF-Κb/MYC Signaling Network. Oncogene 38, 3667–3680. doi:10.1038/s41388-019-0683-z
Cui, Y., Zhang, C., Ma, S., Li, Z., Wang, W., Li, Y., et al. (2021). RNA m6A Demethylase FTO-Mediated Epigenetic Up-Regulation of LINC00022 Promotes Tumorigenesis in Esophageal Squamous Cell Carcinoma. J. Exp. Clin. Cancer Res. 40, 294. doi:10.1186/s13046-021-02096-1
Dai, D., Wang, H., Zhu, L., Jin, H., and Wang, X. (2018). N6-methyladenosine Links RNA Metabolism to Cancer Progression. Cell Death Dis 9, 124. doi:10.1038/s41419-017-0129-x
Dai, X.-Y., Shi, L., Li, Z., Yang, H.-Y., Wei, J.-F., and Ding, Q. (2021). Main N6-Methyladenosine Readers: YTH Family Proteins in Cancers. Front. Oncol. 11, 635329. doi:10.3389/fonc.2021.635329
Deng, J., Zhang, J., Ye, Y., Liu, K., Zeng, L., Huang, J., et al. (2021). N6-methyladenosine-mediated Upregulation of WTAPP1 Promotes WTAP Translation and Wnt Signaling to Facilitate Pancreatic Cancer Progression. Cancer Res. doi:10.1158/0008-5472.can-21-0494
Deng, X., Su, R., Feng, X., Wei, M., and Chen, J. (2018a). Role of N6-Methyladenosine Modification in Cancer. Curr. Opin. Genet. Development 48, 1–7. doi:10.1016/j.gde.2017.10.005
Deng, X., Su, R., Weng, H., Huang, H., Li, Z., and Chen, J. (2018b). RNA N6-Methyladenosine Modification in Cancers: Current Status and Perspectives. Cell Res 28, 507–517. doi:10.1038/s41422-018-0034-6
Du, Q. Y., Zhu, Z. M., and Pei, D. S. (2021). The Biological Function of IGF2BPs and Their Role in Tumorigenesis. Invest. New Drugs 39 (6), 1682–1693. doi:10.1007/s10637-021-01148-9
Esteve-Puig, R., Climent, F., Piñeyro, D., Domingo-Domènech, E., Davalos, V., Encuentra, M., et al. (2021). Epigenetic Loss of m1A RNA Demethylase ALKBH3 in Hodgkin Lymphoma Targets Collagen, Conferring Poor Clinical Outcome. Blood 137 (7), 994–999. doi:10.1182/blood.2020005823
Frye, M., Harada, B. T., Behm, M., and He, C. (2018). RNA Modifications Modulate Gene Expression during Development. Science 361, 1346–1349. doi:10.1126/science.aau1646
Gu, C., Wang, Z., Zhou, N., Li, G., Kou, Y., Luo, Y., et al. (2019). Mettl14 Inhibits Bladder TIC Self-Renewal and Bladder Tumorigenesis through N6-Methyladenosine of Notch1. Mol. Cancer 18, 168. doi:10.1186/s12943-019-1084-1
Gu, J., Zhan, Y., Zhuo, L., Zhang, Q., Li, G., Li, Q., et al. (2021a). Biological Functions of m6A Methyltransferases. Cell Biosci 11, 15. doi:10.1186/s13578-020-00513-0
Gu, Y., Wu, X., Zhang, J., Fang, Y., Pan, Y., Shu, Y., et al. (2021b). The Evolving Landscape of N6-Methyladenosine Modification in the Tumor Microenvironment. Mol. Ther. 29 (5), 1703–1715. doi:10.1016/j.ymthe.2021.04.009
Han, H., Yang, C., Zhang, S., Cheng, M., Guo, S., Zhu, Y., et al. (2021). METTL3-mediated m6A mRNA Modification Promotes Esophageal Cancer Initiation and Progression via Notch Signaling Pathway. Mol. Ther. - Nucleic Acids 26, 333–346. doi:10.1016/j.omtn.2021.07.007
Han, J., Wang, J.-z., Yang, X., Yu, H., Zhou, R., Lu, H.-C., et al. (2019). METTL3 Promote Tumor Proliferation of Bladder Cancer by Accelerating Pri-miR221/222 Maturation in m6A-dependent Manner. Mol. Cancer 18, 110. doi:10.1186/s12943-019-1036-9
Han, S. H., and Choe, J. (2020). Diverse Molecular Functions of m6A mRNA Modification in Cancer. Exp. Mol. Med. 52, 738–749. doi:10.1038/s12276-020-0432-y
He, L., Li, H., Wu, A., Peng, Y., Shu, G., and Yin, G. (2019). Functions of N6-Methyladenosine and its Role in Cancer. Mol. Cancer 18, 176. doi:10.1186/s12943-019-1109-9
He, P. C., and He, C. (2021). m6 A RNA Methylation: from Mechanisms to Therapeutic Potential. EMBO J. 40 (3), e105977. doi:10.15252/embj.2020105977
Hou, Y., Zhang, Q., Pang, W., Hou, L., Liang, Y., Han, X., et al. (2021). YTHDC1-mediated Augmentation of miR-30d in Repressing Pancreatic Tumorigenesis via Attenuation of RUNX1-Induced Transcriptional Activation of Warburg Effect. Cell Death Differ 28 (11), 3105–3124. doi:10.1038/s41418-021-00804-0
Hu, Y., Pan, Q., Wang, M., Ai, X., Yan, Y., Tian, Y., et al. (2021). m6A RNA Methylation Regulator YTHDF1 Correlated with Immune Microenvironment Predicts Clinical Outcomes and Therapeutic Efficacy in Breast Cancer. Front. Med. 8, 667543. doi:10.3389/fmed.2021.667543
Huang, H., Weng, H., and Chen, J. (2020b). m6A Modification in Coding and Non-coding RNAs: Roles and Therapeutic Implications in Cancer. Cancer Cell 37, 270–288. doi:10.1016/j.ccell.2020.02.004
Huang, H., Weng, H., and Chen, J. (2020a). The Biogenesis and Precise Control of RNA m6A Methylation. Trends Genet. 36, 44–52. doi:10.1016/j.tig.2019.10.011
Huang, J., and Yin, P. (2018). Structural Insights into N 6 -methyladenosine (M 6 A) Modification in the Transcriptome. Genomics, Proteomics & Bioinformatics 16, 85–98. doi:10.1016/j.gpb.2018.03.001
Huang, W., Chen, T.-Q., Fang, K., Zeng, Z.-C., Ye, H., and Chen, Y.-Q. (2021). N6-methyladenosine Methyltransferases: Functions, Regulation, and Clinical Potential. J. Hematol. Oncol. 14, 117. doi:10.1186/s13045-021-01129-8
Huang, W., Li, Y., Zhang, C., Zha, H., Zhou, X., Fu, B., et al. (2020c). IGF2BP3 Facilitates Cell Proliferation and Tumorigenesis via Modulation of JAK/STAT Signalling Pathway in Human Bladder Cancer. J. Cell. Mol. Med. 24, 13949–13960. doi:10.1111/jcmm.16003
Iaiza, A., Tito, C., Ianniello, Z., Ganci, F., Laquintana, V., Gallo, E., et al. (2021). METTL3-dependent MALAT1 Delocalization Drives C-Myc Induction in Thymic Epithelial Tumors. Clin. Epigenet 13, 173. doi:10.1186/s13148-021-01159-6
Ianniello, Z., Sorci, M., Ceci Ginistrelli, L., Iaiza, A., Marchioni, M., Tito, C., et al. (2021). New Insight into the Catalytic -dependent and -independent Roles of METTL3 in Sustaining Aberrant Translation in Chronic Myeloid Leukemia. Cel Death Dis 12, 870. doi:10.1038/s41419-021-04169-7
Jain, P., Kathuria, H., and Momin, M. (2021). Clinical Therapies and Nano Drug Delivery Systems for Urinary Bladder Cancer. Pharmacol. Ther. 226, 107871. doi:10.1016/j.pharmthera.2021.107871
Jiang, D. M., Gupta, S., Kitchlu, A., Meraz-Munoz, A., North, S. A., Alimohamed, N. S., et al. (2021a). Defining Cisplatin Eligibility in Patients with Muscle-Invasive Bladder Cancer. Nat. Rev. Urol. 18, 104–114. doi:10.1038/s41585-020-00404-6
Jiang, X., Liu, B., Nie, Z., Duan, L., Xiong, Q., Jin, Z., et al. (2021b). The Role of m6A Modification in the Biological Functions and Diseases. Sig Transduct Target. Ther. 6, 74. doi:10.1038/s41392-020-00450-x
Jin, H., Ying, X., Que, B., Wang, X., Chao, Y., Zhang, H., et al. (2019). N6-methyladenosine Modification of ITGA6 mRNA Promotes the Development and Progression of Bladder Cancer. EBioMedicine 47, 195–207. doi:10.1016/j.ebiom.2019.07.068
Kaur, J., Choi, W., Geynisman, D. M., Plimack, E. R., and Ghatalia, P. (2021). Role of Immunotherapy in Localized Muscle Invasive Urothelial Cancer. Ther. Adv. Med. Oncol. 13, 17588359211045858. doi:10.1177/17588359211045858
Kim, G. W., and Siddiqui, A. (2021). N6-methyladenosine Modification of HCV RNA Genome Regulates Cap-independent IRES-Mediated Translation via YTHDC2 Recognition. Proc. Natl. Acad. Sci. U S A. 118, 10. doi:10.1073/pnas.2022024118
Lan, N., Lu, Y., Zhang, Y., Pu, S., Xi, H., Nie, X., et al. (2020). FTO - A Common Genetic Basis for Obesity and Cancer. Front. Genet. 11, 559138. doi:10.3389/fgene.2020.559138
Lan, Q., Liu, P. Y., Haase, J., Bell, J. L., Hüttelmaier, S., and Liu, T. (2019). The Critical Role of RNA m6A Methylation in Cancer. Cancer Res. 79, 1285–1292. doi:10.1158/0008-5472.can-18-2965
Lee, Y., Choe, J., Park, O. H., and Kim, Y. K. (2020). Molecular Mechanisms Driving mRNA Degradation by m6A Modification. Trends Genet. 36, 177–188. doi:10.1016/j.tig.2019.12.007
Lenis, A. T., Lec, P. M., Chamie, K., and Mshs, M. (2020). Bladder Cancer. JAMA 324, 1980–1991. doi:10.1001/jama.2020.17598
Li, H., Wu, H., Wang, Q., Ning, S., Xu, S., and Pang, D. (2021a). Dual Effects of N6-Methyladenosine on Cancer Progression and Immunotherapy. Mol. Ther. - Nucleic Acids 24, 25–39. doi:10.1016/j.omtn.2021.02.001
Li, L., Zhou, M., Chen, B., Wang, Q., Pan, S., Hou, Y., et al. (2021b). ALKBH5 Promotes Cadmium-Induced Transformation of Human Bronchial Epithelial Cells by Regulating PTEN Expression in an m6A-dependent Manner. Ecotoxicology Environ. Saf. 224, 112686. doi:10.1016/j.ecoenv.2021.112686
Li, M., Deng, L., and Xu, G. (2021c). METTL14 Promotes Glomerular Endothelial Cell Injury and Diabetic Nephropathy via m6A Modification of α-klotho. Mol. Med. 27, 106. doi:10.1186/s10020-021-00365-5
Li, M., Zha, X., and Wang, S. (2021d). The Role of N6-Methyladenosine mRNA in the Tumor Microenvironment. Biochim. Biophys. Acta (Bba) - Rev. Cancer 1875, 188522. doi:10.1016/j.bbcan.2021.188522
Li, R., Zhang, J., Gilbert, S. M., Conejo-Garcia, J., and Mule, J. J. (2021e). Using Oncolytic Viruses to Ignite the Tumour Immune Microenvironment in Bladder Cancer. Nat. Rev. Urol. 18 (9), 543–555. doi:10.1038/s41585-021-00483-z
Li, Y., Ge, Y.-z., Xu, L., Xu, Z., Dou, Q., and Jia, R. (2020). The Potential Roles of RNA N6-Methyladenosine in Urological Tumors. Front. Cel Dev. Biol. 8, 579919. doi:10.3389/fcell.2020.579919
Li, Z., Li, Y., Zhong, W., and Huang, P. (2021f). m6A-Related lncRNA to Develop Prognostic Signature and Predict the Immune Landscape in Bladder Cancer. J. Oncol. 2021, 7488188. doi:10.1155/2021/7488188
Liang, Z., L. Kidwell, R., Deng, H., and Xie, Q. (2020). Epigenetic N6-Methyladenosine Modification of RNA and DNA Regulates Cancer. Cancer Biol. Med. 17, 9–19. doi:10.20892/j.issn.2095-3941.2019.0347
Liao, S., Sun, H., and Xu, C. (2018). YTH Domain: A Family of N 6 -methyladenosine (M 6 A) Readers. Genomics, Proteomics & Bioinformatics 16, 99–107. doi:10.1016/j.gpb.2018.04.002
Lin, S., Zhu, Y., Ji, C., Yu, W., Zhang, C., Tan, L., et al. (2021). METTL3-Induced miR-222-3p Upregulation Inhibits STK4 and Promotes the Malignant Behaviors of Thyroid Carcinoma Cells. J. Clin. Endocrinol. Metab., dgab480. doi:10.1210/clinem/dgab480
Liu, H., Gu, J., Jin, Y., Yuan, Q., Ma, G., Du, M., et al. (2021a). Genetic Variants in N6-Methyladenosine Are Associated with Bladder Cancer Risk in the Chinese Population. Arch. Toxicol. 95, 299–309. doi:10.1007/s00204-020-02911-2
Liu, J., Harada, B. T., and He, C. (2019). Regulation of Gene Expression by N-Methyladenosine in Cancer. Trends Cel Biol. 29, 487–499. doi:10.1016/j.tcb.2019.02.008
Liu, S., Chen, X., and Lin, T. (2021b). Lymphatic Metastasis of Bladder Cancer: Molecular Mechanisms, Diagnosis and Targeted Therapy. Cancer Lett. 505, 13–23. doi:10.1016/j.canlet.2021.02.010
Lou, X., Wang, J.-J., Wei, Y.-Q., and Sun, J.-J. (2021). Emerging Role of RNA Modification N6-Methyladenosine in Immune Evasion. Cel Death Dis 12, 300. doi:10.1038/s41419-021-03585-z
Lu, Z., Liu, H., Song, N., Liang, Y., Zhu, J., Chen, J., et al. (2021). METTL14 Aggravates Podocyte Injury and Glomerulopathy Progression through N6-methyladenosine-dependent Downregulating of Sirt1. Cel Death Dis 12, 881. doi:10.1038/s41419-021-04156-y
Luo, X., Li, H., Liang, J., Zhao, Q., Xie, Y., Ren, J., et al. (2021). RMVar: an Updated Database of Functional Variants Involved in RNA Modifications. Nucleic Acids Res. 49, D1405–D1412. doi:10.1093/nar/gkaa811
Ma, S., Chen, C., Ji, X., Liu, J., Zhou, Q., Wang, G., et al. (2019). The Interplay between m6A RNA Methylation and Noncoding RNA in Cancer. J. Hematol. Oncol. 12, 121. doi:10.1186/s13045-019-0805-7
Ma, Z., and Ji, J. (2020). N6-methyladenosine (m6A) RNA Modification in Cancer Stem Cells. Stem Cells 38, 1511–1519. doi:10.1002/stem.3279
Maldonado López, A., and Capell, B. C. (2021). The METTL3-m6A Epitranscriptome: Dynamic Regulator of Epithelial Development, Differentiation, and Cancer. Genes (Basel) 12 (7), 1019. doi:10.3390/genes12071019
Meeks, J. J., Al-Ahmadie, H., Faltas, B. M., Taylor, J. A., Flaig, T. W., DeGraff, D. J., et al. (2020). Genomic Heterogeneity in Bladder Cancer: Challenges and Possible Solutions to Improve Outcomes. Nat. Rev. Urol. 17, 259–270. doi:10.1038/s41585-020-0304-1
Meng, Y., Zhang, Q., Wang, K., Zhang, X., Yang, R., Bi, K., et al. (2021). RBM15-mediated N6-Methyladenosine Modification Affects COVID-19 Severity by Regulating the Expression of Multitarget Genes. Cel Death Dis 12, 732. doi:10.1038/s41419-021-04012-z
Meyer, K. D., and Jaffrey, S. R. (2017). Rethinking m6A Readers, Writers, and Erasers. Annu. Rev. Cel Dev. Biol. 33, 319–342. doi:10.1146/annurev-cellbio-100616-060758
Mu, X., Wu, K., Zhu, Y., Zhu, Y., Wang, Y., Xiao, L., et al. (2021). Intra-arterial Infusion Chemotherapy Utilizing Cisplatin Inhibits Bladder Cancer by Decreasing the FIbrocytic Myeloid-Derived Suppressor Cells in an m6A-dependent Manner. Mol. Immunol. 137, 28–40. doi:10.1016/j.molimm.2021.06.012
Nie, S., Zhang, L., Liu, J., Wan, Y., Jiang, Y., Yang, J., et al. (2021). ALKBH5-HOXA10 Loop-Mediated JAK2 m6A Demethylation and Cisplatin Resistance in Epithelial Ovarian Cancer. J. Exp. Clin. Cancer Res. 40, 284. doi:10.1186/s13046-021-02088-1
Niu, Y., Lin, Z., Wan, A., Chen, H., Liang, H., Sun, L., et al. (2019). RNA N6-Methyladenosine Demethylase FTO Promotes Breast Tumor Progression through Inhibiting BNIP3. Mol. Cancer 18, 46. doi:10.1186/s12943-019-1004-4
Niu, Y., Wan, A., Lin, Z., Lu, X., and Wan, G. (2018). N6-Methyladenosine Modification: a Novel Pharmacological Target for Anti-cancer Drug Development. Acta Pharmaceutica Sinica B 8, 833–843. doi:10.1016/j.apsb.2018.06.001
Oerum, S., Meynier, V., Catala, M., and Tisné, C. (2021). A Comprehensive Review of m6A/m6Am RNA Methyltransferase Structures. Nucleic Acids Res. 49, 7239–7255. doi:10.1093/nar/gkab378
Pan, F., Lin, X.-R., Hao, L.-P., Chu, X.-Y., Wan, H.-J., and Wang, R. (2021). The Role of RNA Methyltransferase METTL3 in Hepatocellular Carcinoma: Results and Perspectives. Front. Cel Dev. Biol. 9, 674919. doi:10.3389/fcell.2021.674919
Patel, V. G., Oh, W. K., and Galsky, M. D. (2020). Treatment of Muscle‐invasive and Advanced Bladder Cancer in 2020. CA A. Cancer J. Clin. 70, 404–423. doi:10.3322/caac.21631
Patil, D. P., Pickering, B. F., and Jaffrey, S. R. (2018). Reading m6A in the Transcriptome: m6A-Binding Proteins. Trends Cel Biol. 28, 113–127. doi:10.1016/j.tcb.2017.10.001
Peng, B., Yan, Y., and Xu, Z. (2021). The Bioinformatics and Experimental Analysis of AlkB Family for Prognosis and Immune Cell Infiltration in Hepatocellular Carcinoma. PeerJ 9, e12123. doi:10.7717/peerj.12123
Purslow, J. A., Nguyen, T. T., Khatiwada, B., Singh, A., and Venditti, V. (2021). N 6-methyladenosine Binding Induces a Metal-Centered Rearrangement that Activates the Human RNA Demethylase Alkbh5. Sci. Adv. 7, 34. doi:10.1126/sciadv.abi8215
Roignant, J.-Y., and Soller, M. (2017). m 6 A in mRNA: An Ancient Mechanism for Fine-Tuning Gene Expression. Trends Genet. 33, 380–390. doi:10.1016/j.tig.2017.04.003
Roviello, G., Catalano, M., Santi, R., Palmieri, V. E., Vannini, G., Galli, I. C., et al. (2021). Immune Checkpoint Inhibitors in Urothelial Bladder Cancer: State of the Art and Future Perspectives. Cancers 13, 4411. doi:10.3390/cancers13174411
Satterwhite, E. R., and Mansfield, K. D. (2021). RNA Methyltransferase METTL16: Targets and Function. Wiley Interdiscip Rev RNA, e1681. doi:10.1002/wrna.1681
Scarrow, M., Chen, N., and Sun, G. (2020). Insights into the N6-Methyladenosine Mechanism and its Functionality: Progress and Questions. Crit. Rev. Biotechnol. 40, 639–652. doi:10.1080/07388551.2020.1751059
Schneider, A. K., Chevalier, M. F., and Derré, L. (2019). The Multifaceted Immune Regulation of Bladder Cancer. Nat. Rev. Urol. 16, 613–630. doi:10.1038/s41585-019-0226-y
Song, P., Tayier, S., Cai, Z., and Jia, G. (2021a). RNA Methylation in Mammalian Development and Cancer. Cell Biol Toxicol 37 (6), 811–831. doi:10.1007/s10565-021-09627-8
Song, T., Yang, Y., Jiang, S., and Peng, J. (2020). Novel Insights into Adipogenesis from the Perspective of Transcriptional and RNA N6‐Methyladenosine‐Mediated Post‐Transcriptional Regulation. Adv. Sci. 7, 2001563. doi:10.1002/advs.202001563
Song, W., Yang, K., Luo, J., Gao, Z., and Gao, Y. (2021b). Dysregulation of USP18/FTO/PYCR1 Signaling Network Promotes Bladder Cancer Development and Progression. Aging 13, 3909–3925. doi:10.18632/aging.202359
Tan, F., Zhao, M., Xiong, F., Wang, Y., Zhang, S., Gong, Z., et al. (2021). N6-methyladenosine-dependent Signalling in Cancer Progression and Insights into Cancer Therapies. J. Exp. Clin. Cancer Res. 40, 146. doi:10.1186/s13046-021-01952-4
Tang, L., Wei, X., Li, T., Chen, Y., Dai, Z., Lu, C., et al. (2021). Emerging Perspectives of RNA N6-Methyladenosine (m6A) Modification on Immunity and Autoimmune Diseases. Front. Immunol. 12, 630358. doi:10.3389/fimmu.2021.630358
Tang, Y., Chen, K., Wu, X., Wei, Z., Zhang, S.-Y., Song, B., et al. (2019). DRUM: Inference of Disease-Associated m6A RNA Methylation Sites from a Multi-Layer Heterogeneous Network. Front. Genet. 10, 266. doi:10.3389/fgene.2019.00266
Tao, L., Mu, X., Chen, H., Jin, D., Zhang, R., Zhao, Y., et al. (2021). FTO Modifies the m6A Level of MALAT and Promotes Bladder Cancer Progression. Clin. Transl Med. 11, e310. doi:10.1002/ctm2.310
Terajima, H., Lu, M., Zhang, L., Cui, Q., Shi, Y., Li, J., et al. (2021). N6-methyladenosine Promotes Induction of ADAR1-Mediated A-To-I RNA Editing to Suppress Aberrant Antiviral Innate Immune Responses. Plos Biol. 19, e3001292. doi:10.1371/journal.pbio.3001292
Tran, L., Xiao, J.-F., Agarwal, N., Duex, J. E., and Theodorescu, D. (2021). Advances in Bladder Cancer Biology and Therapy. Nat. Rev. Cancer 21, 104–121. doi:10.1038/s41568-020-00313-1
Tsuchiya, K., Yoshimura, K., Inoue, Y., Iwashita, Y., Yamada, H., Kawase, A., et al. (2021). YTHDF1 and YTHDF2 Are Associated with Better Patient Survival and an Inflamed Tumor-Immune Microenvironment in Non-small-cell Lung Cancer. Oncoimmunology 10, 1962656. doi:10.1080/2162402x.2021.1962656
Uddin, M. B., Wang, Z., and Yang, C. (2021). The m6A RNA Methylation Regulates Oncogenic Signaling Pathways Driving Cell Malignant Transformation and Carcinogenesis. Mol. Cancer 20, 61. doi:10.1186/s12943-021-01356-0
van Puffelen, J. H., Keating, S. T., Oosterwijk, E., van der Heijden, A. G., Netea, M. G., Joosten, L. A. B., et al. (2020). Trained Immunity as a Molecular Mechanism for BCG Immunotherapy in Bladder Cancer. Nat. Rev. Urol. 17, 513–525. doi:10.1038/s41585-020-0346-4
Wang, G., Dai, Y., Li, K., Cheng, M., Xiong, G., Wang, X., et al. (2021a). Deficiency of Mettl3 in Bladder Cancer Stem Cells Inhibits Bladder Cancer Progression and Angiogenesis. Front. Cel Dev. Biol. 9, 627706. doi:10.3389/fcell.2021.627706
Wang, J., Wang, J., Gu, Q., Ma, Y., Yang, Y., Zhu, J., et al. (2020a). The Biological Function of m6A Demethylase ALKBH5 and its Role in Human Disease. Cancer Cel Int 20, 347. doi:10.1186/s12935-020-01450-1
Wang, M., Liu, J., Zhao, Y., He, R., Xu, X., Guo, X., et al. (2020b). Upregulation of METTL14 Mediates the Elevation of PERP mRNA N6 Adenosine Methylation Promoting the Growth and Metastasis of Pancreatic Cancer. Mol. Cancer 19, 130. doi:10.1186/s12943-020-01249-8
Wang, S., Chai, P., Jia, R., and Jia, R. (2018). Novel Insights on m6A RNA Methylation in Tumorigenesis: a Double-Edged Sword. Mol. Cancer 17, 101. doi:10.1186/s12943-018-0847-4
Wang, S., Sun, C., Li, J., Zhang, E., Ma, Z., Xu, W., et al. (2017). Roles of RNA Methylation by Means of N6-Methyladenosine (m6A) in Human Cancers. Cancer Lett. 408, 112–120. doi:10.1016/j.canlet.2017.08.030
Wang, W., Shao, F., Yang, X., Wang, J., Zhu, R., Yang, Y., et al. (2021b). METTL3 Promotes Tumour Development by Decreasing APC Expression Mediated by APC mRNA N6-methyladenosine-dependent YTHDF Binding. Nat. Commun. 12, 3803. doi:10.1038/s41467-021-23501-5
Wang, X. K., Zhang, Y. W., Wang, C. M., Li, B., Zhang, T. Z., Zhou, W. J., et al. (2021c). METTL16 Promotes Cell Proliferation by Up‐regulating Cyclin D1 Expression in Gastric Cancer. J. Cel Mol Med 25, 6602–6617. doi:10.1111/jcmm.16664
Wen, L., Pan, X., Yu, Y., and Yang, B. (2020). Down-regulation of FTO Promotes Proliferation and Migration, and Protects Bladder Cancer Cells from Cisplatin-Induced Cytotoxicity. BMC Urol. 20, 39. doi:10.1186/s12894-020-00612-7
Williams, G. D., Gokhale, N. S., and Horner, S. M. (2019). Regulation of Viral Infection by the RNA Modification N6-Methyladenosine. Annu. Rev. Virol. 6, 235–253. doi:10.1146/annurev-virology-092818-015559
Witjes, J. A., Bruins, H. M., Cathomas, R., Compérat, E. M., Cowan, N. C., Gakis, G., et al. (2021). European Association of Urology Guidelines on Muscle-Invasive and Metastatic Bladder Cancer: Summary of the 2020 Guidelines. Eur. Urol. 79, 82–104. doi:10.1016/j.eururo.2020.03.055
Wollen, K. L., Hagen, L., Vågbø, C. B., Rabe, R., Iveland, T. S., Aas, P. A., et al. (2021). ALKBH3 Partner ASCC3 Mediates P-Body Formation and Selective Clearance of MMS-Induced 1-methyladenosine and 3-methylcytosine from mRNA. J. Transl Med. 19, 287. doi:10.1186/s12967-021-02948-6
Wu, B., Li, L., Huang, Y., Ma, J., and Min, J. (2017). Readers, Writers and Erasers of N6-Methylated Adenosine Modification. Curr. Opin. Struct. Biol. 47, 67–76. doi:10.1016/j.sbi.2017.05.011
Wu, G., Yan, Y., Cai, Y., Peng, B., Li, J., Huang, J., et al. (2021). ALKBH1-8 and FTO: Potential Therapeutic Targets and Prognostic Biomarkers in Lung Adenocarcinoma Pathogenesis. Front. Cel Dev. Biol. 9, 633927. doi:10.3389/fcell.2021.633927
Wu, J., and Abraham, S. N. (2021). The Roles of T Cells in Bladder Pathologies. Trends Immunol. 42, 248–260. doi:10.1016/j.it.2021.01.003
Wu, R., and Wang, X. (2021). Epigenetic Regulation of Adipose Tissue Expansion and Adipogenesis by N6 -methyladenosine. Obes. Rev. 22 (2), e13124. doi:10.1111/obr.13124
Wu, S., Zhang, S., Wu, X., and Zhou, X. (2020). m6A RNA Methylation in Cardiovascular Diseases. Mol. Ther. 28, 2111–2119. doi:10.1016/j.ymthe.2020.08.010
Xie, F., Huang, C., Liu, F., Zhang, H., Xiao, X., Sun, J., et al. (2021). CircPTPRA Blocks the Recognition of RNA N6-Methyladenosine through Interacting with IGF2BP1 to Suppress Bladder Cancer Progression. Mol. Cancer 20, 68. doi:10.1186/s12943-021-01359-x
Xie, H., Li, J., Ying, Y., Yan, H., Jin, K., Ma, X., et al. (2020). METTL3/YTHDF2 M 6 A axis Promotes Tumorigenesis by Degrading SETD7 and KLF4 mRNAs in Bladder Cancer. J. Cel Mol Med 24, 4092–4104. doi:10.1111/jcmm.15063
Xu, H., Wang, Z., Chen, M., Zhao, W., Tao, T., Ma, L., et al. (2021). YTHDF2 Alleviates Cardiac Hypertrophy via Regulating Myh7 mRNA Decoy. Cel Biosci 11, 132. doi:10.1186/s13578-021-00649-7
Xu, Y., Liu, J., Chen, W.-J., Ye, Q.-Q., Chen, W.-T., Li, C.-L., et al. (2020a). Regulation of N6-Methyladenosine in the Differentiation of Cancer Stem Cells and Their Fate. Front. Cel Dev. Biol. 8, 561703. doi:10.3389/fcell.2020.561703
Xu, Z., Peng, B., Cai, Y., Wu, G., Huang, J., Gao, M., et al. (2020b). N6-methyladenosine RNA Modification in Cancer Therapeutic Resistance: Current Status and Perspectives. Biochem. Pharmacol. 182, 114258. doi:10.1016/j.bcp.2020.114258
Yan, Y., Liang, Q., Xu, Z., and Yi, Q. (2021). Integrative Bioinformatics and Experimental Analysis Revealed Down-Regulated CDC42EP3 as a Novel Prognostic Target for Ovarian Cancer and its Roles in Immune Infiltration. PeerJ 9, e12171. doi:10.7717/peerj.12171
Yang, F., Jin, H., Que, B., Chao, Y., Zhang, H., Ying, X., et al. (2019). Dynamic m6A mRNA Methylation Reveals the Role of METTL3-m6A-CDCP1 Signaling axis in Chemical Carcinogenesis. Oncogene 38, 4755–4772. doi:10.1038/s41388-019-0755-0
Yang, Y., Hsu, P. J., Chen, Y.-S., and Yang, Y.-G. (2018). Dynamic Transcriptomic m6A Decoration: Writers, Erasers, Readers and Functions in RNA Metabolism. Cel Res 28, 616–624. doi:10.1038/s41422-018-0040-8
Yao, L., Yin, H., Hong, M., Wang, Y., Yu, T., Teng, Y., et al. (2021). RNA Methylation in Hematological Malignancies and its Interactions with Other Epigenetic Modifications. Leukemia 35 (5), 1243–1257. doi:10.1038/s41375-021-01225-1
Ye, F., Hu, Y., Gao, J., Liang, Y., Liu, Y., Ou, Y., et al. (2021). Radiogenomics Map Reveals the Landscape of m6A Methylation Modification Pattern in Bladder Cancer. Front. Immunol. 12, 722642. doi:10.3389/fimmu.2021.722642
Ying, X., Jiang, X., Zhang, H., Liu, B., Huang, Y., Zhu, X., et al. (2020). Programmable N6-Methyladenosine Modification of CDCP1 mRNA by RCas9-Methyltransferase like 3 Conjugates Promotes Bladder Cancer Development. Mol. Cancer 19, 169. doi:10.1186/s12943-020-01289-0
Yu, H., Yang, X., Tang, J., Si, S., Zhou, Z., Lu, J., et al. (2021). ALKBH5 Inhibited Cell Proliferation and Sensitized Bladder Cancer Cells to Cisplatin by m6A-Ck2α-Mediated Glycolysis. Mol. Ther. - Nucleic Acids 23, 27–41. doi:10.1016/j.omtn.2020.10.031
Yue, H., Nie, X., Yan, Z., and Weining, S. (2019). N6‐methyladenosine Regulatory Machinery in Plants: Composition, Function and Evolution. Plant Biotechnol. J. 17, 1194–1208. doi:10.1111/pbi.13149
Zarza-Rebollo, J. A., Molina, E., and Rivera, M. (2021). The Role of the FTO Gene in the Relationship between Depression and Obesity. A Systematic Review. Neurosci. Biobehavioral Rev. 127, 630–637. doi:10.1016/j.neubiorev.2021.05.013
Zhang, L., Hou, C., Chen, C., Guo, Y., Yuan, W., Yin, D., et al. (2020). The Role of N6-Methyladenosine (m6A) Modification in the Regulation of circRNAs. Mol. Cancer 19, 105. doi:10.1186/s12943-020-01224-3
Zhang, N., Hua, X., Tu, H., Li, J., Zhang, Z., and Max, C. (2021). Isorhapontigenin (ISO) Inhibits EMT through FOXO3A/METTL14/VIMENTIN Pathway in Bladder Cancer Cells. Cancer Lett. 520, 400–408. doi:10.1016/j.canlet.2021.07.041
Zhang, S. (2018). Mechanism of N6-Methyladenosine Modification and its Emerging Role in Cancer. Pharmacol. Ther. 189, 173–183. doi:10.1016/j.pharmthera.2018.04.011
Zhao, W., Qi, X., Liu, L., Ma, S., Liu, J., and Wu, J. (2020). Epigenetic Regulation of m6A Modifications in Human Cancer. Mol. Ther. - Nucleic Acids 19, 405–412. doi:10.1016/j.omtn.2019.11.022
Zhao, Y., Chen, Y., Jin, M., and Wang, J. (2021). The Crosstalk between m6A RNA Methylation and Other Epigenetic Regulators: a Novel Perspective in Epigenetic Remodeling. Theranostics 11, 4549–4566. doi:10.7150/thno.54967
Zheng, H.-x., Zhang, X.-s., and Sui, N. (2020). Advances in the Profiling of N6-Methyladenosine (m6A) Modifications. Biotechnol. Adv. 45, 107656. doi:10.1016/j.biotechadv.2020.107656
Zhou, G., Yan, K., Liu, J., Gao, L., Jiang, X., and Fan, Y. (2021a). FTO Promotes Tumour Proliferation in Bladder Cancer via the FTO/miR-576/CDK6 axis in an m6A-dependent Manner. Cell Death Discov. 7, 329. doi:10.1038/s41420-021-00724-5
Zhou, H., Yin, K., Zhang, Y., Tian, J., and Wang, S. (2021b). The RNA m6A Writer METTL14 in Cancers: Roles, Structures, and Applications. Biochim. Biophys. Acta (Bba) - Rev. Cancer 1876, 188609. doi:10.1016/j.bbcan.2021.188609
Zhou, Z., Lv, J., Yu, H., Han, J., Yang, X., Feng, D., et al. (2020). Mechanism of RNA Modification N6-Methyladenosine in Human Cancer. Mol. Cancer 19, 104. doi:10.1186/s12943-020-01216-3
Zhu, H., Jia, X., Wang, Y., Song, Z., Wang, N., Yang, Y., et al. (2021a). M6A Classification Combined with Tumor Microenvironment Immune Characteristics Analysis of Bladder Cancer. Front. Oncol. 11, 714267. doi:10.3389/fonc.2021.714267
Keywords: N6-methyladenosine, “writers”, “erasers”, “readers”, methylation, bladder cancer
Citation: Liu Q (2022) Current Advances in N6-Methyladenosine Methylation Modification During Bladder Cancer. Front. Genet. 12:825109. doi: 10.3389/fgene.2021.825109
Received: 30 November 2021; Accepted: 22 December 2021;
Published: 11 January 2022.
Edited by:
Yicheng Long, Cornell University, United StatesReviewed by:
Kunqi Chen, Fujian Medical University, ChinaCopyright © 2022 Liu. This is an open-access article distributed under the terms of the Creative Commons Attribution License (CC BY). The use, distribution or reproduction in other forums is permitted, provided the original author(s) and the copyright owner(s) are credited and that the original publication in this journal is cited, in accordance with accepted academic practice. No use, distribution or reproduction is permitted which does not comply with these terms.
*Correspondence: Qiang Liu, bHExNzQ5MDYwOTdAb3V0bG9vay5jb20=