- 1Institute of Animal Husbandry and Veterinary Science, Wuhan Academy of Agricultural Science, Wuhan, China
- 2Key Laboratory of Agricultural Animal Genetics, Breeding and Reproduction of Ministry of Education, Huazhong Agricultural University, Wuhan, China
- 3Institute of Animal Husbandry and Veterinary Medicine, Fujian Academy of Agricultural Sciences, Fuzhou, China
The key genes of avian gonadal development are of great significance for sex determination. Transcriptome sequencing analysis of Mule duck gonad as potential sterile model is expected to screen candidate genes related to avian gonad development. In this study, the embryonic gonadal tissues of Mule ducks, Jinding ducks, and Muscovy ducks were collected and identified. Six sample groups including female Mule duck (A), male Mule duck (B), female Jinding duck (C), male Jinding duck (D), female Muscovy duck (E), and male Muscovy duck (F) were subjected to RNA sequencing analysis. A total of 9,471 differential genes (DEGs) and 691 protein-protein interaction pairs were obtained. Totally, 12 genes (Dmrt1, Amh, Sox9, Tex14, Trim71, Slc26a8, Spam1, Tdrp, Tsga10, Boc, Cxcl14, and Hsd17b3) were identified to be specifically related to duck testicular development, and 11 genes (Hsd17b1, Cyp19a1, Cyp17a1, Hhipl2, Tdrp, Uts2r, Cdon, Axin2, Nxph1, Brinp2, and Brinp3) were specifically related to duck ovarian development. Seven genes (Stra8, Dmc1, Terb1, Tex14, Tsga10, Spam1, and Plcd4) were screened to be specifically involved in the female sterility of Mule ducks; eight genes (Gtsf1, Nalcn, Tat, Slc26a8, Kmo, Plcd4, Aldh4a1, and Hgd) were specifically involved in male sterility; and five genes (Terb1, Stra8, Tex14 Tsga10 and Spam1) were involved in both female and male sterility. This study provides an insight into the differential development between male and female gonads of ducks and the sterility mechanism of Mule ducks through function, pathway, and protein interaction analyses. Our findings provide theoretical basis for the further research on sex determination and differentiation of birds and the sterility of Mule ducks.
Introduction
Avian gonad development can be divided into three stages. The first stage refers to the early embryonic stage when the genital ridges exhibiting “bipotential” trait form, and the sex-determining switch genes drive genital ridges to develop towards ovaries or testes. The second stage is the early and middle embryonic stage when the genital ridges further develop to form the testis or ovary, accompanied with the formation of a regulatory network composed of abundant genes to induce the sex differentiation of specific cells in the testis or ovary. The third stage refers to the one when male and female characteristics are maintained by hormones, and in this stage a series of genes are involved in the regulation of sexual characteristics maintenance (Barske and Capel 2010; Guioli, et al., 2020). Several critical genes reported to play important roles in gonadal development in mammals may also act in chick gonadal development (Oréal, et al., 2002; Feng, et al., 2007). For example, the SRY-like HMG box gene 9 (Sox9) is marker gene of testicular development, and cytochrome P450 family 19 subfamily A member 1(Cyp19a1) is ovarian developmental marker genes. In mammals, the expression of Sox9 is activated by Sry, whereas the expression of Sox9 must be initiated by another male-specific gene (probably a Z-linked gene) due to the lack of Sry in birds. Aromatase encoded by Cyp19a1 is a key enzyme in the estrogen synthesis pathway (Elbrecht and Smith 1992), and aromatase is associated with the production of cortical layer and proliferation of germ cells and Sertoli cells. The expression pattern and expression stage of other sex-related genes such as Anti-Müllerian hormone gene (Amh), Steroidogenic factor-1 (Sf1), Wilm’s tumor1 gene (Wt1), and Gata binding protein 4 (Gata4) in birds were different from those in other species (Nachtigal, et al., 1998). The Pitx2 gene related to asymmetric organ development has been reported to regulate the development of asymmetric gonad in chickens, and translocation experiments have revealed that this gene causes the degeneration of the right ovary in hens (Guioli and Lovell-Badge 2007). Although these genes mentioned above play a certain role in avian gonad development and participate in some developmental regulatory networks, they are not sex-determining genes. Therefore, it is necessary to screen related genes so as to gain insights into avian gonadal development.
Hybridization between distant genera can combine biological characteristics of distant species and break species constraints, thus driving genome evolution and new species formation (Grant et al., 2005; Mallet 2005; Mallet 2007). Nevertheless, there often exists reproductive isolation for evolutionarily well-differentiated taxa, therefore resulting in sterility of hybrids (Wünsch and Pfennig 2013). Chickens and quails belong to the genus Gallus and Coturnix, respectively, both of which belong to the Phasianidae of Galliformes of Aves. They have the same number of chromosomes (2n = 78) and their chromosome structures are highly conserved (Shibusawa, et al., 2001). The hybrid between chicken and quail belongs to intergeneric cross, and the sex differentiation is male-oriented from the 10th day of incubation. All the females die in the embryonic period and the hatched hybrids are only male with sterility trait (Ishishita, et al., 2016). Mule ducks are the offspring of intergeneric hybridization between Cairina moschata and Anas platyrhynchos domestica, and are also the products of distant hybridization. The comparison of the morphology of testicular germ cells between domestic ducks and Mule ducks showed that spermatogonia and primary spermatocytes were observed in seminiferous tubules of 52-week-old Mule ducks with secondary spermatocytes, spermatocytes, and sperms undetected. In contrast, the testes of all 9-week-old male domestic ducks contained sperms, indicating that meiotic failure is one of the reasons for Mule ducks sterility (Huang and Sung 1988). Additionally, the comparison the testicular morphology between Mule ducks and Peking ducks showed that the spermatogonia and spermatocytes of Mule ducks are disorderly arranged with no mature sperms found in the testis (Li, et al., 2020). Although infertility phenotypes have been observed in adult Mule duck, the genes responsible for sterility in Mule ducks remain largely unclear. By comparing the morphological characteristics of the 1/4, 1/2, and 3/4 of embryonic stage of the Mule ducks with those of their parents, our previous study (unpublished) found that male and female individuals formed the seminiferous tubules of the testis and the cortex and medulla of the ovary at the 1/2 embryonic stage, respectively. Especially in this stage (1/2 stage), the expression level of Dmrt1 was highest, which was higher in males than in females, suggesting that the 1/2 embryonic stage might be a critical period for the development of gonads in Mule ducks. However, the underlying factors affecting sterility in early gonadal development remains to be investigated. Transcriptome sequencing technology can quickly and comprehensively screen related genes in specific avian gonadal development stages based on information about transcripts. In this study, we investigated the genes related to the early-stage gonad development of the Mule ducks with Jinding ducks and Muscovy ducks as parents. We constructed transcriptome libraries of Mule ducks, Jinding ducks and Muscovy ducks during 1/2 embryonic period via high-throughput sequencing. Our study screened 32 candidate genes responsible for sterility and sexual differentiation in the early gonadal development of Mule ducks. Our findings lay a foundation for elucidating the mechanism underlying avian gonad development.
Materials and methods
Sex identification
PCR amplification was performed with gonadal tissue DNA as a template, and gene-specific PCR primers were designed according to the Chd1 gene on the sex chromosomes. The sex could be identified according to the observed size of particular bands. Only one band with a size of about 467 bp was considered as male, and two bands with a size of 467 bp and 326 bp were identified as female (Supplementary Figure S1). PCR was conducted in a 10 μl system containing 5 μl of 2x M5 HiPer plus Taq HiFi PCR mix (TaKaRa) 0.2 μl of each forward and reverse primer (Supplementary Table S1, 10 μM), 1 μl of DNA (approximate concentration of 100 ng/μl), and 3.6 μl RNase-free ddH2O. PCR was performed with 35 cycles of denaturing at 98°C for 10 s, annealing at 51°C for 20 s, and extension at 72°C for 30 s. PCR products were analyzed by 1.5% agarose gel electrophoresis (120 V, 200 mA, 20 min).
Experiment material
The 500 eggs of Jinding ducks (domestic ducks), Muscovy ducks and their hybridization offspring Mule ducks were collected, respectively. When eggs were hatched to the 1/2 embryonic stage, the gonadal tissues were collected to extract DNA for sex identification. After sex identification, 10–20 paired gonads were combined into one sample. Six groups were respectively marked as A, female Mule ducks; B, male Mule ducks; C, female Jinding ducks; D, male Jinding ducks; E, female Muscovy ducks; F, male Muscovy ducks and a total of 18 samples were constructed with three biological replicates per group. The sample collection was approved by the Ethics Committee of Wuhan Academy of Agricultural Science.
Total RNA extraction
Total RNA was extracted from all tissues using TRIzol Reagent (Invitrogen, Carlsbad, CA, United States) according to the manufacturer’s instructions. The integrity of the obtained RNA was evaluated using an Agilent Bioanalyzer 2100 system (Agilent Technologies, CA, United States).
Library preparation for RNA sequencing
After quality evaluation of total RNA, high-quality total RNA samples were purified to obtain mRNA ending with Poly-A using the oligo (dT) magnetic beads, followed by fragmentation (about 300 bp) and cDNA synthesis with RNA as template. The RNA library was prepared using a total amount of 1 μg RNA per sample. The sequencing library was generated using NEB Next UltraTM RNA Library prep Kit for Illumina (NEB, United States) according to the instruction manual. A total of 18 cDNA libraries were constructed and paired-end sequenced using Illumina high-throughput sequencing platforms.
Bioinformatics analysis
The software Cutadapt was used to remove adapter sequences and low-quality nucleotides from the raw data to obtain clean data. After quality control, clean reads were aligned to the Anas platyrhynchos reference genome (ftp://ftp.ensembl.org/pub/release100/fasta/anas_platyrhynch-os/dna/) using HISAT2 software (http://ccb.jhu.edu/software/hisat2/index.shtml). The Read Count value aligned to each gene using HTSeq software was used as the original expression level of the gene. Expression levels were normalized using the FPKM method (Trapnell, et al., 2010). Principal component analysis (PCA) of samples based on gene expression levels was performed using DESeq package in R. Hierarchical clustering of genes and samples was performed using pheatmap package in R. The Euclidean distance was used for calculating distances and the complete linkage was used in clustering. Gene ontology (GO) and KEGG pathway enrichment analyses were conducted using Blast2GO (Götz, et al., 2008) and bi-directional best-hit (BBH) assignment method on KEGG Automatic Annotation Server (KAAS) (Moriya, et al., 2007). The hypergeometric test was performed followed by FDR correction and items with corrected p-values less than 0.05 were considered to be significant or enriched. The data background is genes in the whole genome. The protein-protein interaction (PPI) prediction of DEGs was based on the retrieved associations from STRING (Jensen, et al., 2009). Interaction score greater than 0.95 were screened and drawn with Cytoscape (Koonin and Rogozin 2003).
Quantitative real-time PCR (qRT-PCR)
RNA was reverse transcribed into cDNAs using the PrimeScriptTM RT reagent kit with gDNA Eraser (TaKaRa, Japan) following the manufacturer’s recommended procedures, and the resultant cDNAs were stored at -20°C. The quantitative real-time PCR (qRT-PCR) was conducted on an CFX-96/384 (Bio-Red, Hercules, CA, United States) in a 10 μl system containing 5 μl of 2 × SYBR Green qPCR Master Mix (Bimake, United States), 0.2 μl of each forward and reverse primer (Supplementary Table S1) (10 μM), 1 μl of cDNA (approximate concentration of 100 ng/μl), and 3.6 μl RNase-free ddH2O. The qRT-PCR was performed with 40 cycles of denaturing at 95°C for 15 s, annealing at 51°C for 30 s, and extension at 72°C for 20 s. Gapdh was used as an internal control. The experiments were conducted with at least four biological replicates and at least three technical replicates per biological replicate. Primer specificity was estimated by melting curves, and qPCR data were analyzed using 2-ΔΔCt method (Livak and Schmittgen 2001).
Result
mRNA expression profiles of embryonic gonads of Mule ducks, jinding ducks, and muscovy ducks
A total of 18 sequencing libraries of six sample groups were constructed (A, female Mule ducks; B, male Mule ducks;C, female Jinding ducks; D, male Jinding ducks; E, female Muscovy ducks; F, male Muscovy ducks) with three biological replicates per group. A total of 798216036 clean reads were obtained after paired-end sequencing on the Illumina sequencing platform, and the reference genome alignment results showed that the unique alignment rate was above 87% (Supplementary Table S2). The raw reads of our transcriptome data have been deposited into the NCBI Short Read Archive (SRA, http://www.ncbi.nlm.nih.gov/sra/) under accession number PRJNA874907.
Screening of differentially expressed gene (DEGs)
In order to evaluate the robustness of each group, we performed Spearman’s correlation analyses. The results showed that the average correlation coefficient of samples within the same group was above 0.9, and the average correlation coefficient between the Muscovy duck group and the other two groups was around 0.7 (Figure 1A). In addition, the principal component analysis (PCA) results showed a close distance within the same breed, while the samples from different breeds were more scattered (Figure 1B), and thus these samples were qualified for subsequent analysis.
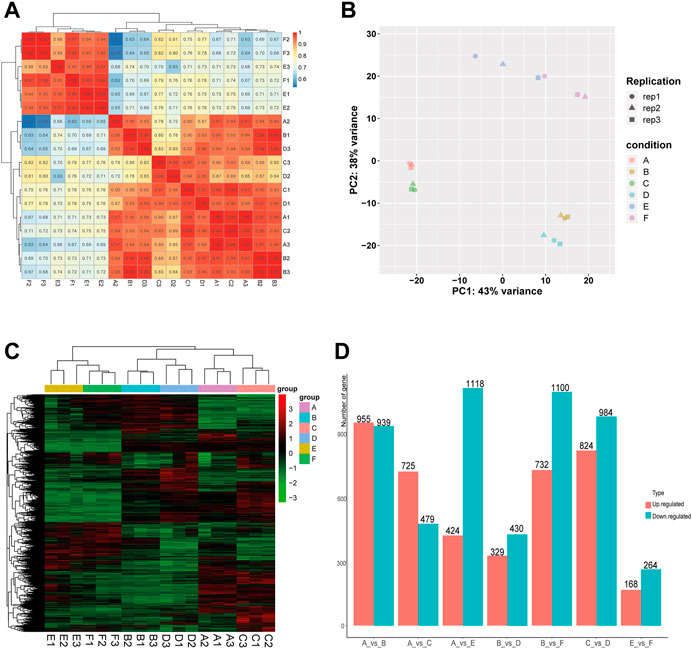
FIGURE 1. Correlation analysis of samples and screening of DEGs in the comparison between or within breeds (Mule ducks, Jinding ducks and Muscovy ducks). (A) Heatmap of correlations among groups. (B) Principal Component Analysis (PCA). Different shapes represent different replicates in the same group, and different colors represent different groups. (C) Hierarchical clustering analysis of DEGs. Columns indicate samples, and rows denote genes. The red represents the high gene expression and the green represents the low gene expression. (D) Statistics analysis of DEGs. The abscissa represents comparison groups, and the ordinate represents the number of DEGs. Red indicates up-regulated genes, and blue denotes down-regulated genes. A: female Mule ducks, B: male Mule ducks, C: female Jinding ducks, D: male Jinding ducks, E: female Muscovy ducks, F: male Muscovy ducks.
To identify differentially expressed genes (DEGs), the hierarchical clustering analysis was performed with the threshold of p-value < 0.05 and absolute value of log2 FoldChange >1 (Figure 1C). A total of 9,471 DEGs were identified, including 1894 DEGs in the comparison of A vs. B, 1808 DEGs in C vs. D, 432 DEGs in E vs. F, 1542 DEGs in A vs. E, 1204 DEGs in A vs. C, 1832 DEGs in B vs. F, and 759 DEGs in B vs. D. The number of DEGs (432) identified in the comparison between male and female in Muscovy ducks was the smallest, while it was the largest between female and male in Mule ducks (1894). A total of 955, 732, and 725 up-regulated DEGs and 939, 1100, and 479 down-regulated DEGs were identified respectively in the comparison of male vs. female of Mule ducks, Muscovy ducks, Jinding ducks (Figure 1D). The volcano map of DEGs in each comparison was shown in Supplementary Figure.S2. As shown in the Veen diagram in Supplementary Figures S3, 131 shared genes were identified among A vs. B, C vs. D, and E vs. F, 139 shared genes were identified between A vs. C and A vs. E, 100 shared genes were identified between B vs. D and B vs. F.
GO enrichment analysis
GO enrichment analysis of DEGs in intra-breed comparison (A vs. B, C vs. D, and E vs. F) was performed. The top 10 shared GO terms in which DEGs were enriched mainly included ion transport or multicellular organismal process-related biological processes (BP), plasma membrane or extracellular space-related cellular component (CC), and ion channel activation related molecular function (MF) (Figures 2A–C). In A vs. B, of 64 DEGs involved in developmental process, 28 were up-regulated, 36 were down-regulated, of which 2 up-regulated DEGs (Hsd17b3 and Amh) and one down-regulated DEG Cyp17a1 were significantly enriched in sex differentiation. The GO enrichment analysis results also showed that three up-regulated DEGs (Boc, Myocd, and Brinp1) and three down-regulated DEGs (Brinp3, Cdon, and Brinp2) were significantly enriched in positive regulation of cell differentiation or developmental process. In C vs. D, one up-regulated DEG (Spam1) and four down-regulated DEGs (Tsga10, Tdrp, Slc26a8, and Tex14) were significantly enriched in developmental process and reproductive process. Additionally, there were four shared DEGs (Axin2, Cxcl14, Uts2r, and Trim71) among A vs. B, C vs. D, and E vs. F were significantly enriched in estrogen biosynthetic process, calcium ion transport, sex differentiation, and multicellular organism development.
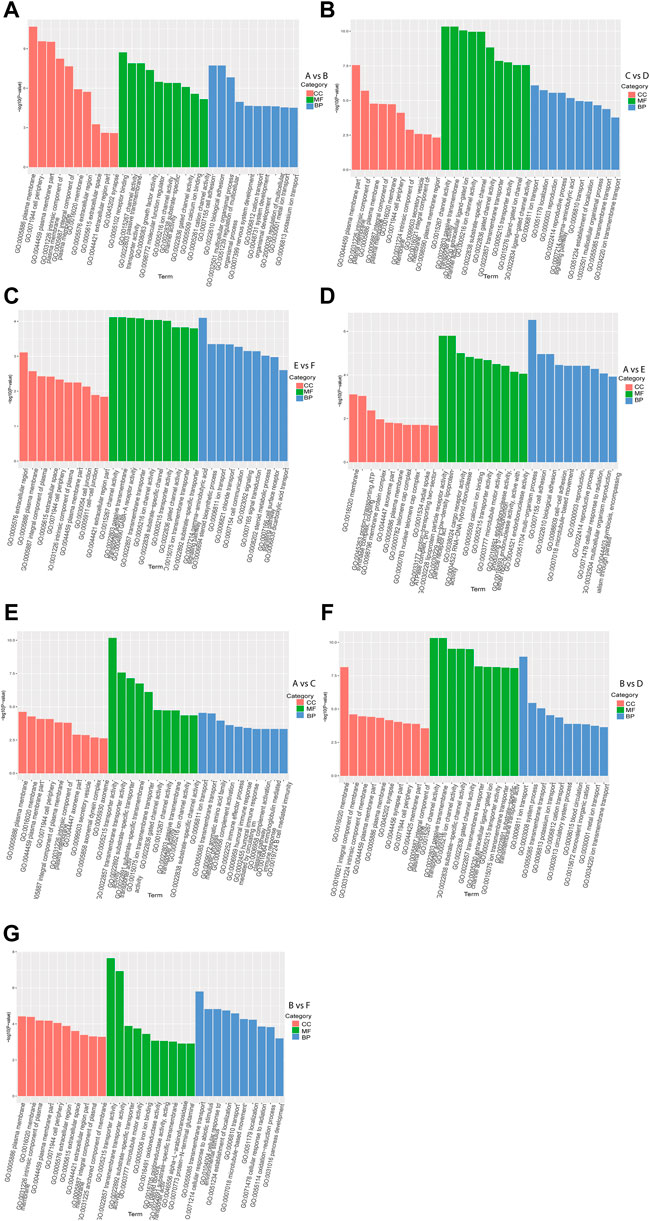
FIGURE 2. GO functional enrichment analysis of DEGs. The x-axis represents the secondary GO terms, the y-axis represents the -log10 p-value of enrichment of each term. Intra-breed comparisons between females and males include A vs. B. (A), C vs. D. (B), and E vs. F. (C). Comparisons between Mule ducks and Muscovy ducks or Jinding ducks of same sex include A vs. E. (D), A vs. C. (E), B vs. D. (F), and B vs. F. (G). BP, biological processes; CC, cellular component; MF, molecular function.
In addition, in inter-breed female comparison (A vs. E and A vs. C), the top 10 shared GO terms in which DEGs were enriched mainly included immune response-or reproduction-related BP, telomere cap complex- or axonemal dynein complex-related CC, as well as ion channel activation- or receptor activation-related MF (Figures 2D,E). In A vs. E, a total of 7 DEGs were involved in reproductive process, of which Tsga10, Stra8, Terb1, Spam1, Tex14 were up-regulated, and Plcd4, Bmp15 were down-regulated. The down-regulated DEG Plcd4 was also enriched in such biological processes as sexual reproduction and gamete generation. In A vs. C, four up-regulated DEGs (Tra8, Dmc1, Terb1, and Tex14) were significantly enriched in meiotic cell cycle. In inter-breed male comparison of B vs. D, three down-regulated DEGs (Tsga10, Gtsf1, and Slc26a8) were enriched in biological processes of male gamete generation and spermatogenesis, and in B vs. F, five up-regulated DEGs (Tsga10, Stra8, Terb1, Plcz1, and Tex14) and two down-regulated DEGs (Plcd4 and Hsd17b3) were enriched in reproductive process (Figures 2F,G). Besides, four up-regulated DEGs (Hgd, Tat, Kynu, and Kmo) were involved in aromatic amino acid family catabolic process in B vs. F. It should be noticed that up-regulated DEG Aldh4a1 was enriched in proline catabolic process.
KEGG pathway analysis of DEGs
KEGG pathway analysis of each comparison group was performed. The results showed that the DEGs in intra-breed comparison were enriched in such signaling pathways as Calcium signaling pathway, Steroid hormone biosynthesis, and TGF-beta signaling pathway. Specifically, the DEGs in A vs. B, C vs. D, and E vs. F were also significantly enriched in GnRH signaling pathway, MAPK signaling pathway, Drug metabolism-cytochrome P450, respectively (Figures 3A–C). For inter-breed comparison, such signaling pathways as Drug metabolism-cytochrome P450 and Oxidative phosphorylation were significantly enriched with DEGs in A vs. E (Figure 3D). Signaling pathways such as Tyrosine metabolism and Steroid hormone biosynthesis were significantly enriched with DEGs in A vs. C (Figure 3E). Notably, most DEGs in B vs. D and B vs. F were enriched in amino acid metabolism-related pathways such as Tyrosine metabolism, beta-Alanine metabolism, Histidine metabolism pathways (Figures 3F,G). Furthermore, Calcium signaling pathway and Oxidative phosphorylation were significantly enriched with DEGs in B-vs-D.
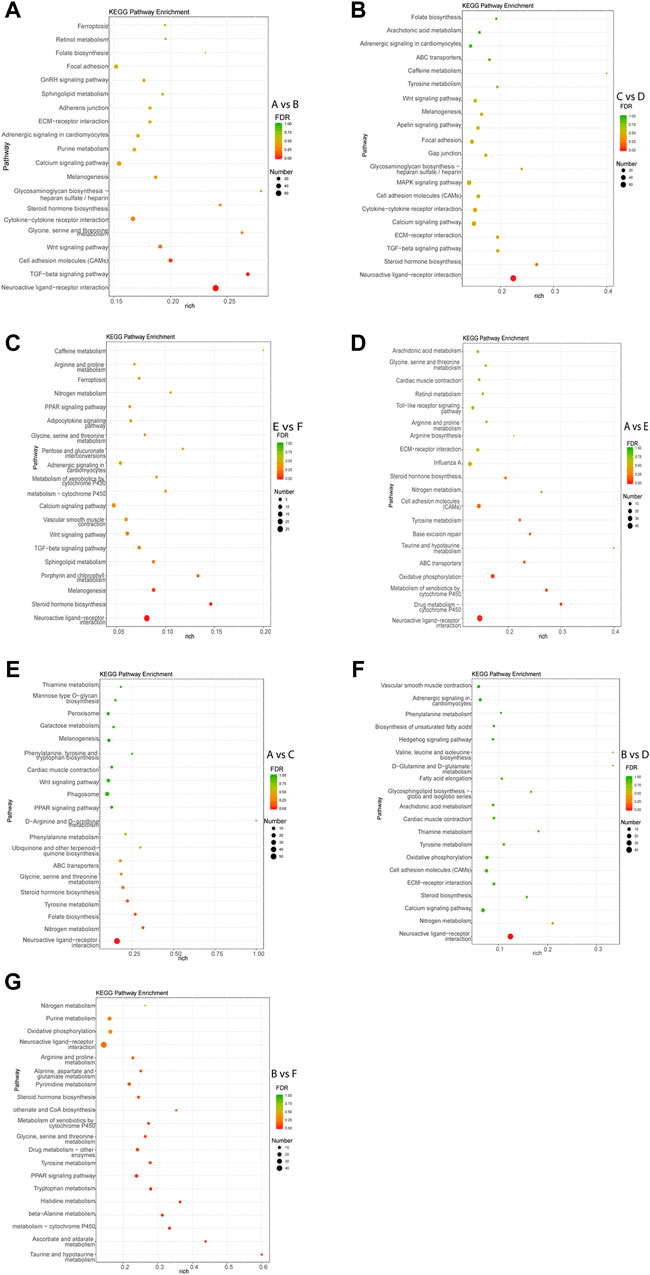
FIGURE 3. Top 20 pathways enriched with DEGs by KEGG pathway enrichment analysis. The Rich factor of x-axis is the ratio of the number of DEGs enriched in the pathway to the number of all annotated genes in the pathway. The size of the circle indicates the number of DEGs enriched in the pathway. The FDR (false discovery rate) indicates the enrichment degree of DEGs in a certain pathway. The closer to zero the FDR, the higher the enrichment degree. Intra-breed comparisons between females and males include A vs. B. (A), C vs. D. (B), and E vs. F. (C). Comparisons between Mule ducks and Muscovy ducks or Jinding ducks of same sex include A vs. E. (D), A vs. C. (E), B vs. D. (F), and B vs. F. (G).
Protein interaction analysis
In order to reveal the relationship between genes, the DEGs were scanned by the STRING to construct the protein-protein interaction (PPI) network. The results showed that a total of 691 gene pairs were searched including 122 in A vs. E, 65 in A vs. C, 176 in B vs. F, 34 in B vs. D, 164 in A vs. B, 119 in C vs. D, and 11 in E vs. F. The PPI network contained 1893 DEGs and 164 (Score>0.95) interaction pairs in A vs. B (Figure 4A). Five genes in the subnetwork constructed by DEGs in A vs. B (HSD17B1, HSD17B2, CYP19A1, CYP17A1, and CYP11A1) were annotated to Steroid hormone biosynthesis. These five genes in the subnetwork in C vs. D were also annotated to Steroid hormone biosynthesis (Figure 4B). The interaction score of HSD17B1-CYP19A1 and CYP11A1-STAR was 0.981 and 0.986, respectively. Seven genes in another subnetwork in A vs. B (NOG, BMPR2, BMP6, BMP7, BMP2, BMPR1B, and CHRD) were annotated to TGF-beta signaling pathway (p-value = 7.7 E-5),and the interaction score of NOG-BMP7 was 0.989. In addition, 3 DEGs (CYP19A1, HSD17B1, and SRD5A1) in the subnetwork in A vs. E were annotated to the redox biological processes and lipid metabolism signaling pathway (Figure 4C). The resultant PPI network in B vs. F contained 1832 DEGs and 176 (Score>0.95) interaction pairs. And four genes (AGXT2, GPT2, ALDH4A1, and AGXT) in the subnetwork were annotated to amino acid metabolism-related pathways such as Alanine, aspartate, and glutamate metabolism pathways (Figure 4D). The PPI networks of DEGs in the comparisons of E vs. F, A vs. C, and B vs. D were shown in Supplementary Figure S4. And The protein interaction scores of some subnetworks were shown in Supplementary Table S3.
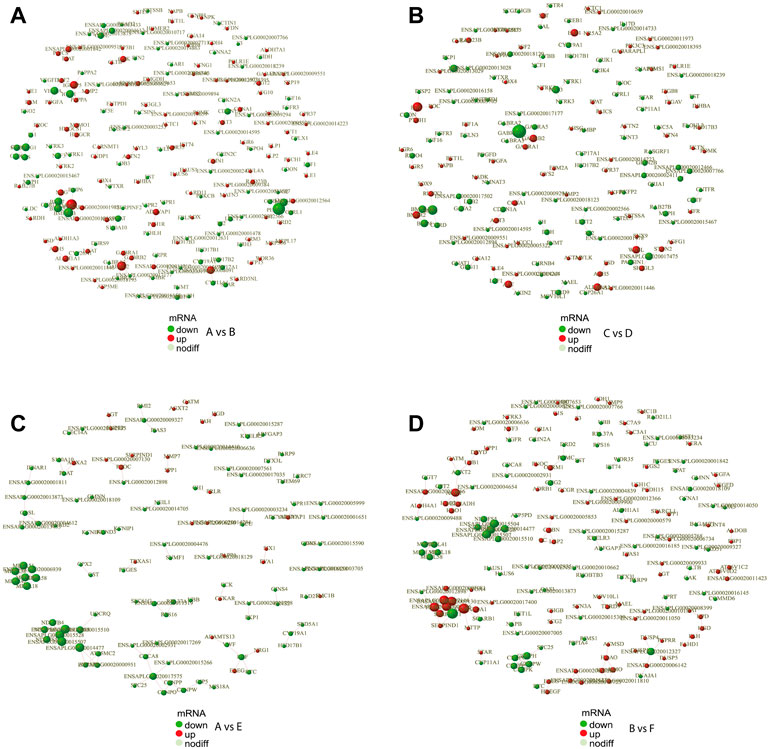
FIGURE 4. PPI network of DEGs. PPI network exhibits both protein interactions and expression levels. The dots indicate protein-encoding genes. Red indicates up-regulated genes, and green denotes down-regulated genes. PPI, protein-protein interaction; DEGs, differentially expressed genes. (A) PPI network of DEGs between female and male Mule ducks. (B) PPI network of DEGs between female and male Jinding ducks. (C) PPI network of DEGs between Mule duck and Muscovy duck females. (D) PPI network of DEGs between Mule duck and Muscovy duck males.
Screening of key candidate genes related to gonadal development and sterility of Mule ducks
A total of 34 key candidate genes were identified as gonadal development- or infertility-related genes according to our clustering analyses, functional enrichment analyses, and the published literature (Figure 5), of which 22 sex-biased DEGs (female-biased or male-biased), namely, Dmrt1, Amh, Sox9, Tex14, Trim71, Slc26a8, Spam1, Tdrp, Tsga10, Boc, Cxcl14, Hsd17b3, Hsd17b1, Cyp19a1, Cyp17a1, Hhipl2, Uts2r, Cdon, Axin2, Nxph1, Brinp2, and Brinp3 were identified as sex-related genes in Mule ducks, Jinding ducks, and Muscovy ducks. Most of these 22 sex-related genes were annotated to sexual differentiation- and reproduction-related biological processes, of which, seven genes (Cxcl14, Amh, Boc, Sox9, Trim71, Dmrt1, and Hsd17b3) were strongly expressed in male gonads of at least two duck breeds and simultaneously lowly expressed in female gonads of all three duck breeds. The 11 genes (Tdrp, Hsd17b1, Cyp19a1, Cyp17a1, Hhipl2, Uts2r, Cdon, Axin2, Nxph1, Brinp2, and Brinp3) were highly expressed in female gonads of at least two duck breeds and meanwhile lowly expressed in male gonads of all three breeds. In addition, seven genes including Stra8, Dmc1, Terb1, Tex14, Tsga10, Spam1, and Plcd4 were involved in meiotic cell cycle, reproductive process, and gamete generation, and they were identified as the genes causing female sterility in Mule ducks. The expression of three genes (Stra8, Terb1, and Tex14) in female gonads were lower in Mule ducks than in other two breeds. Thirteen genes including Gtsf1, Nalcn, Tat, Slc26a8, Kmo, Plcd4, Aldh4a1, Hgd, Tsga10, Hsd17b3, Stra8, Terb1, and Tex14 involved in male gamete generation, spermatogenesis, sperm motility, proline catabolic process, and reproductive process were identified as the genes causing male sterility in Mule ducks. Among them, the expression of three genes (Kmo, Aldh4a1, and Hgd) in male gonads was lower in Mule ducks than in other two breeds. and the expression of five genes (Tsga10, Gtsf1, Nalcn, Tat, and Slc26a8) in male gonads of Mule ducks was lower than that of Muscovy ducks but higher than that of Jinding ducks.
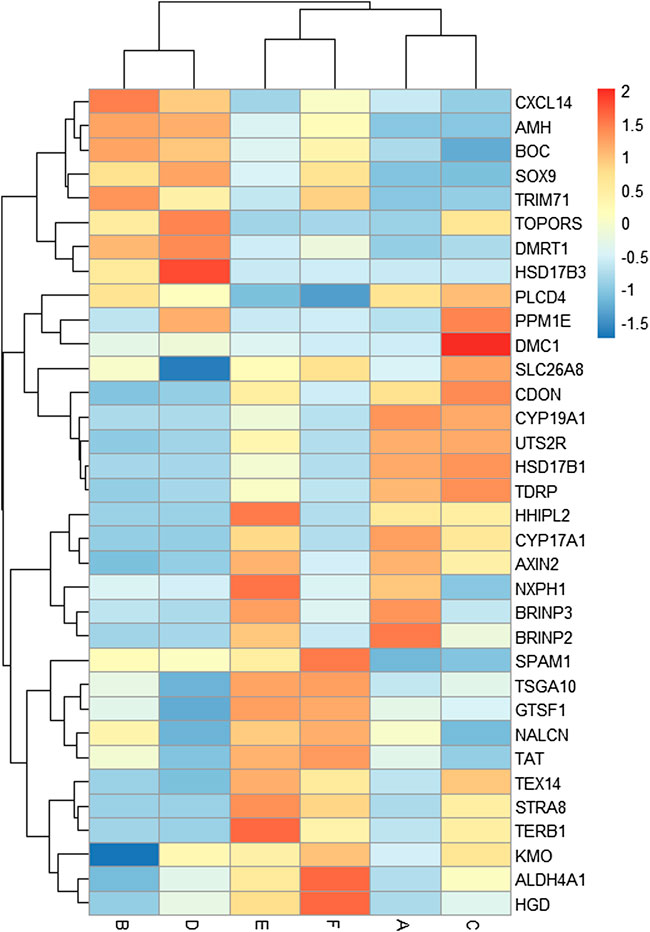
FIGURE 5. Heat map of the expression levels of key candidate genes related to gonadal development and sterility in Mule ducks. Red represents a high expression level, and blue indicates a low expression level. Columns and rows represent samples and genes, respectively. The color scale represents fold changes in gene expression.
Verification of DEGs by qRT-PCR
Eleven DEGs were randomly selected for validation by qRT-PCR. As shown in Figure 6, six genes, namely, Cyp17a1, Hhipl2, hsd17β1, Nxph1, ENSAPLG00020014497, and ENSAPLG0002-0010883, were more highly expressed in males than in females of both three breeds. ENSAPLG000200-14475 was more highly expressed in both females and males of Mule ducks than in those of Muscovy ducks. Particularly, the expression level of topors was higher in Mule duck females than males. The expression of Nxph1 was higher in the females of Mule ducks and Muscovy ducks than their males. Eleven DEGs identified by qRT-PCR coincided with the RNA-Seq results, indicating the reliability of RNA-Seq results.
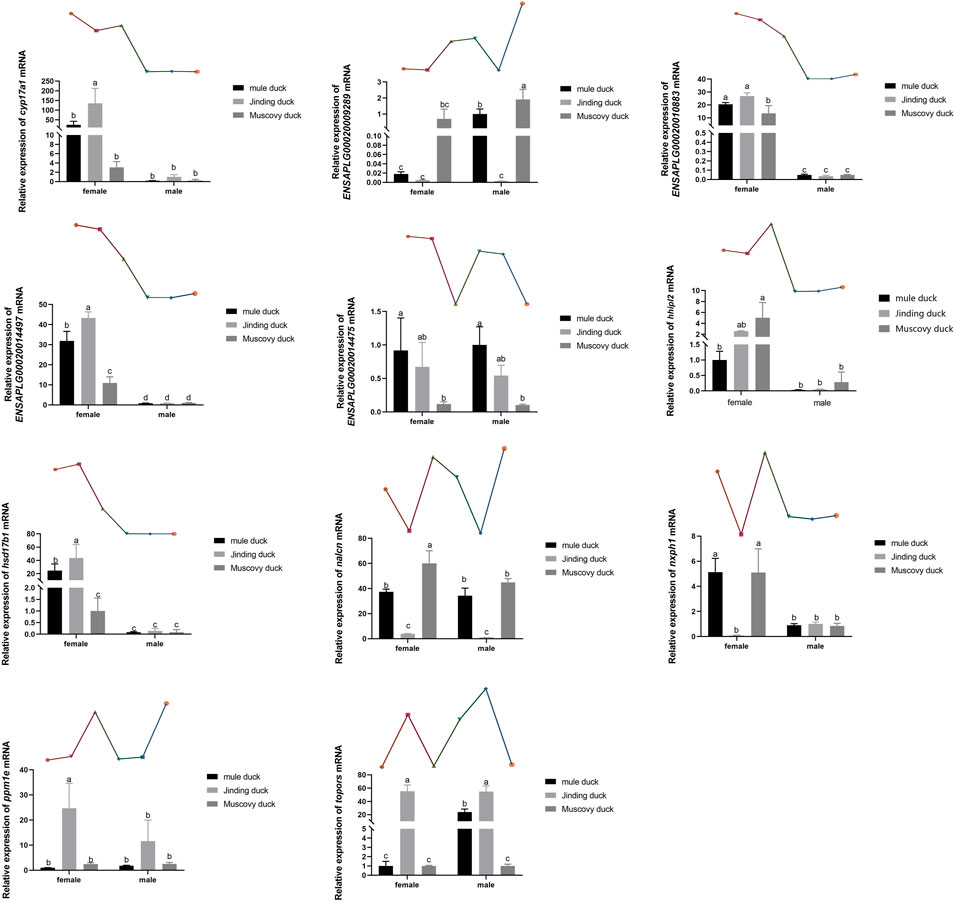
FIGURE 6. qRT-PCR validation of 11 DEGs identified by RNA-Seq. The gene expression level in the RNA-seq data indicated by line chart corresponds to that the qRT-PCR results displayed in bar chart. The data are expressed as mean ± standard deviation (SD). Each group has three biological replicates, and the lower-case letters above the bars represent significant differences.
Discussion
From initiation of sex differentiation, sex-biased genes drive the gonad to develop toward the testis or ovary, which plays a key role in the formation and functions of testis or ovary. Z-linked Dmrt1 has been reported to have important effect on gonadal development in multiple species (mammals, chicken, and fish) (Chen, et al., 2014). Our data showed that the expression levels of Dmrt1, Amh, and Sox9 were higher in males than in females of all three duck breeds. The transcriptome analysis has revealed that Amh is significantly up-regulated after male differentiation by comparison before or after gonad differentiation in Muscovy ducks (Bai, et al., 2020). Moreover, the expression of AmhR2 in male spermatogenic cords is disrupted after knockdown of Dmrt1 during sexual differentiation in birds (Cutting, et al., 2014), implying that Dmrt1 acts upstream of Amh to induce male initial differentiation and continues to play roles after differentiation. Additionally, the expression of Amh is regulated by the testicular factor Sox9 (Arango, et al., 1999), and Dmrt1 has been reported to possibly bind to the enhancer of Sox9 to regulate sex differentiation (Primakoff and Myles 2002). Based on these findings, it can be speculated that Dmrt1 might regulate Sox9, thus controlling the expression of Amh during sex differentiation of birds, and thus gene regulation plays an important role in the process of gonadal differentiation and development (Figure 7).
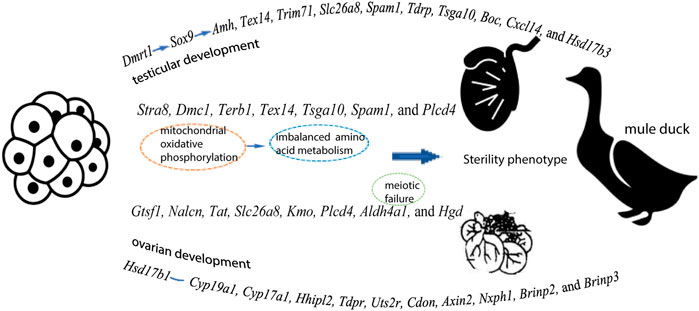
FIGURE 7. A speculation explaining the gene expression pattern in testes and ovaries, as well as the sterility in Mule ducks
Spermatogenesis can be divided into three principal stages: mitotic proliferation of spermatogonia, genome reduction of meiotic spermatocytes, and morphological transformation from haploid spermatids to sperm. Incomplete cytokinesis makes daughters connected by stable intercellular bridge to form germline cysts, thus sharing gene products (Braun, et al., 1989; Greenbaum, et al., 2011). Testis-expressed 14 (Tex14) is a novel protein localized to intercellular bridges of germ cells (Greenbaum, et al., 2006), and a study of adult mouse testis has shown that the Tex14 protein is essential for blocking cytokinesis and converting transient midbody loops into stable intercellular bridges (Kim, et al., 2015). During spermatogenesis of Tex14−/− mice, although diploid spermatogonia are subjected to transport, proliferation, and early meiotic marker expression, these processes stopped before the first meiosis, suggesting Tex14 mutation was likely to prevent the function of intercellular bridge, thereby leading to meiosis defects, eventually causing sterility (Greenbaum, et al., 2006). Our data showed that Tex14 was highly expressed in male Mule ducks but lowly expressed in females. Besides, Trim71 deletion leads to a reduction in the undifferentiated spermatogonia population, and blocks the differentiation of male reproductive system of mice (Du, et al., 2020). In this study, the expression of Trim71 was higher in males of three breeds than in females. Spam1, sperm membrane protein in mammals, is widely conserved across species (Lathrop, et al., 1990) and it is expressed in both testis and epididymis (Zheng, et al., 2001; Zhang, et al., 2004). As a multifunctional protein, Spam1 with multiple domains plays multiple roles in fertilization. Testis development-related protein (Tdrp) is a nuclear factor, and in Tdrp-deletion mouse model, many sperm motility indices such as average path velocity (VAP), straight line velocity (VSL), and curvilinear velocity (VCL) are significantly lowered (p < 0.05) (Mao, et al., 2016), implying that deletion of Tdrp impairs sperm motility. In our study, Spam1, Tsga10, Tdrp, Slc26a8, and Tex14 were linked to sexual reproduction and reproductive process. Boc and Cxcl14 displayed a high expression in males but a low expression in females in all three breeds, and our GO analysis showed that these two genes were involved in positive regulation of cell differentiation or developmental process.
The final step in androgen biosynthesis in the testis is the conversion of androstenedione (Δ4) into testosterone (T, Testosterone), and this step is catalyzed by 17β-HSD-3 (Twesten, et al., 2000). The increasing evidence has revealed that 17β-HSD-3 is expressed in Leydig cells, whose mutations cause a rare 46, XY disorder of sex development (DSD) (Folsom, et al., 2019), and it was first reported in 1971 that the phenotype of 17β-HSD-3 deficiency was an autosomal recessive inheritance (Saez, et al., 1971; Saez, et al., 1972). Our data showed that Hsd17b3 located on the Z chromosome of ducks was more highly expressed in males than in females in Mule ducks and Jinding ducks. Besides, Hsd17b3 was significantly enriched in sex differentiation GO term. In contrast, Hsd17b1 was more highly expressed in females than in males in all three breeds, which was consistent with the observation during the gonad developmental stage in flatfish (Zou, et al., 2020). Aromatase encoded by Cyp19a1 can convert androstenedione and testosterone into estrone (E1) and estradiol (E2), respectively. Hsd17b1 can efficiently catalyze the conversion of E1 with low biological activity into E2 with high biological activity (Day, et al., 2008). Overexpression of human-derived Hsd17b1 leads to masculinization of internal and external genitalia in female mice (Saloniemi, et al., 2009). In this study, the interaction score between Hsd17b1 and Cyp19a1 was 0.981, and these two genes were annotated to the Steroid hormone biosynthesis signaling pathway. Cytochrome P450 family 17 subfamily A member 1 (Cyp17a1), as an important member of the cytochrome P450 subfamily, exhibits both hydroxylase and lyase activities, and it is expressed in the Leydig cells and the granulosa cells of ovarian follicles (Zhou, et al., 2007). Mutation of Cyp17a1 results in female-to-male sex reversal, but sex reversal was successfully rescued by 17β-estradiol (E2) or testosterone (T) in XX Nile tilapia (Yang, et al., 2021). Consistent with the expression pattern of Hsd17b1, Cyp17a1 was highly expressed in females but lowly expressed in males in all three duck breeds, and Cyp17a1 was involved in sex differentiation, suggesting that Hsd17b1 and Cyp17a1 might be key candidate genes related to gonadal differentiation and development. Furthermore, five genes (Hhipl2, Tdrp, Uts2r, Cdon, and Axin2) exhibited high expression in females but low expression in males in all three duck breeds, of which Uts2r and Cdon were involved in multicellular organismal process. Nxph1, Brinp2, and Brinp3 were highly expressed in females but lowly expressed in males in Mule ducks and Muscovy ducks. of which Brinp2 and Brinp3 were involved in regulation of developmental process.
Only after meiosis can diploid progenitor cells produce haploid gametes (oocytes and sperm). In mammals, meiosis is initiated in different life stages for different sexes, and female germ cells enter meiosis shortly after sex determination during embryogenesis, while male germ cells do not enter meiosis until puberty (Goetz, et al., 1984; Spiller, et al., 2012). Our inter-breed female comparison of Mule ducks, Jinding ducks, and Muscovy ducks revealed that Stra8, Dmc1, Terb1, and Tex14 were involved in meiotic cell cycle, of which 3 genes (Stra8, Terb1, and Tex14) were more highly expressed in Jinding duck females and Muscovy duck females than in Mule duck females. Stimulated by retinoic acid gene 8 (Stra8) is considered a key regulator of meiosis in mammals (Anderson, et al., 2008). In female mice, Stra8 is expressed in embryonic ovarian germ cells before entering meiosis (Menke, et al., 2003). Although early mitosis of germ cells is normal in female embryos lacking Stra8 gene function, these germ cells are unable to undergo DNA replication before entering meiosis and chromosome condensation, synapsis, and recombination processes during meiotic prophase, implying that initiation of the meiotic program is dependent on the function of Stra8 in female embryonic germ cells (Baltus, et al., 2006). DNA meiotic recombinase 1 (Dmc1), a meiosis-specific gene, is crucial for assembling DNA double strand breaks (DSBs) site in homologous recombination and for searching DNA sequences located on homologous chromatids (Cao, et al., 2021). Dmc1-knockout mice have no follicles and a smaller ovary (Biswas, et al., 2021). Follicular development and spermatogenesis completely fail after Dmc1 homozygote knockout in mice, and in humans, Dmc1 is required for spermatogenesis but not for oogenesis (Cao, et al., 2021). In addition to the effect of Spam1 on spermatogenesis mentioned above, the expression of Spam1 in the reproductive tract of female mice was 3–10 times lower than that of males, and its expression in the uterus was unsteady during the estrous cycle (Zhang and Martin-DeLeon 2003). In this study, Spam1 was expressed lower in female Mule ducks than in female Muscovy ducks, and it was involved in sexual reproduction, reproduction, and gamete generation.
Our inter-breed comparison of males (B vs. D and B vs. F) revealed that DEGs were significantly enriched in biological processes such as male gamete generation, spermatogenesis, proline catabolic process. Of these DEGs, the expression of four genes (Gtsf1, Nalcn, Tat, and Slc26a8) were higher in male Mule ducks than in male Jinding ducks but lower than in male Muscovy ducks. The three genes (Kmo, Aldh4a1, and Hgd) were lowly expressed in male Mule ducks whereas highly expressed in males of the other two breeds. These results suggested that the above-mentioned seven genes were related to duck male sterility. Gametocyte-specific factor 1 (Gtsf1) is evolutionarily highly conserved. Primary spermatocytes of Gtsf1−/− male mice stopped proliferation before the zygotene of the first meiotic prophase, thus causing massive germ cell apoptosis on postnatal day 14, eventually leading to male sterility (Yoshimura, et al., 2009). One previous study has shown that sperms with the phospholipase C delta 4 (Plcd4) gene homozygote deleted fail to initiate acrosome reaction, thereby resulting in male sterility or small number per litter in mice (Fukami, et al., 2001). DEGs in the comparison of B vs. D were involved in calcium signaling pathway in this study. Notably, the Slc26a8 gene is expressed in the plasma membrane of male germ cells at the spermatocyte and sperm stages (Toure, et al., 2001). The deletion of Slc26a8 results in the sperm capacitation impairment, flagellum structure defects, abnormal mitochondrial sheath assembly, and sperm motility reduction, thus causing sterility in male mice (Rode, et al., 2012). The Kmo-encoded enzyme operates at a key branch point in the kynurenine pathway, and the deletion of Kmo gene alters mitochondrial morphology and function (Maddison, et al., 2020). These previous findings indicate that sterility of Mule ducks might be related to mitochondria. Mitochondria are recognized as critical sites of energy conversion and metabolism in sperm, and they play critical roles in calcium homeostasis maintenance, spermatogenesis, apoptosis, and fertilization (Friedman and Nunnari 2014). Interestingly, mitochondria are identified as a major source of reactive oxygen species (ROS) in mammalian sperm (Koppers, et al., 2008). The oxidative phosphorylation (OXPHOS) in sperm mitochondria produces an appropriate amount of ROS, which is necessary for sperm tyrosine phosphorylation and cholesterol efflux, sperm maturation, capacitation, and sperm-oocyte binding (Samanta, et al., 2018). Approximately 30–40% of male infertility cases are associated with the elevated mitochondrial ROS levels (Jose-Miller, et al., 2007). The OXPHOS complex is present in the folded and curved part of the mitochondrial inner membrane. Pyruvate has been reported to be converted to acetyl-CoA to provide fuel for OXPHOS, and perturbation of OXPHOS makes spermatogenesis highly sensitive (Varuzhanyan and Chan 2020). In this study, DEGs in both B vs. D and B vs. F were enriched in oxidative phosphorylation pathway, particularly, enriched in the signaling pathway of pantothenate and CoA biosynthesis in B vs. F, implying that abnormal oxidative phosphorylation of mitochondria in germ cells might be responsible for the sterile phenotype of Mule ducks. As shown in Figure 3, many amino acid metabolism pathways were significantly enriched in the comparison of males across different breeds. Aldh4a1 belongs to the aldehyde dehydrogenase superfamily, and it is associated with type II hyperprolinemia (HPII) (Geraghty, et al., 1998; van de Ven, et al., 2014), whose patients suffer from fibroblast mitochondrial dysfunction. Aldh4a1 expression is significantly decreased in oocytes of postpartum mouse (Esteves, et al., 2011). Proline acts as a central amino acid in cellular redox control (Yen and Curran 2021). Antioxidant drugs can alleviate function impairment of sperm mitochondria induced by proline catabolism disorder (Yen and Curran 2021). More studies of amino acids have shown that homocysteine level is increased in sperm from patients with severe disruption of semen proteins (Kralikova, et al., 2017), that d-Aspartate (D-Asp) and N-methyl-d-aspartate (NMDA) promote spermatogenesis by stimulating the biosynthesis of sex steroid hormones (Di Fiore, et al., 2014), that D-Asp and NMDA can increase the expression of OXPHOS complex (Falvo, et al., 2022). Additionally, tyrosine phosphorylation is regulated by redox signaling (Chiarugi and Cirri 2003). Based on these findings, we speculated that in 1/2 embryonic stage of Mule ducks, inordinate amino acid metabolism caused by mitochondrial OXPHOS could be considered to be one of the reasons for sterile phenotype of Mule ducks (Figure 7).
Five genes (Tsga10, Terb1, Stra8, Tex14, and Spam1) were predicted to be associated with sterility in male and female Mule ducks. Our data showed that the expression level of these five genes was significantly lower in Mule ducks than that in Muscovy ducks for both males and females. In this study, Tsga10 and Spam1 were found to be potentially related to the female sterility in Mule ducks, of which testis-specific gene antigen 10 (Tsga10) has been reported to be highly expressed in various species, especially in the middle and main parts of sperm tails (Modarressi, et al., 2004). In Tsga10+/− mice generated by CRISPR/Cas9, the disordered mitochondrial sheath resulted in the reduced sperm motility (Luo, et al., 2021). It has been reported that Stra8, Terb1, and Tex14 involved in meiosis of germ cells are also closely related to male sterility (Greenbaum, et al., 2006; Anderson, et al., 2008; Shibuya, et al., 2014). Our inter-breed female comparison showed that Terb1, Stra8, and Tex14 were enriched in meiotic cell cycle. However, our inter-breed male comparison indicated that no genes were enriched in meiotic cell cycle, instead, some genes were enriched in mitochondrial oxidative phosphorylation and amino acid metabolism. Such difference might be due to the fact that male germ cells did not enter the meiotic process until puberty, but our samples were derived from the 1/2 embryonic stage (before meiosis). Furthermore, the imbalance between OXPHOS and spermatogonia glycolysis could lead to meiosis failure (Li Y, et al., 2021), which suggested that amino acid metabolism disturbance induced by mitochondrial oxidative phosphorylation might be reason for meiosis failure in male Mule ducks, further resulting in male Mule duck sterility (Figure 7). It should be noted that merely a few amino acid metabolism pathways were enriched by DEGs in inter-breed female comparison in this study (Figure 3). One previous study has shown that the maintenance of redox homeostasis can promote meiotic maturation of porcine oocytes (Li Z, et al., 2021). These findings jointly suggested that amino acid metabolism imbalance induced by mitochondrial oxidative phosphorylation might be one of the early causes behind meiotic failure, which was consistent with our speculation on the reason for male Mule duck sterility.
Conclusion
Taken together, the transcriptome analysis of 1/2 embryonic gonads of three duck breeds was performed by RNA-seq. A total of 9471 DEGs were identified by intra- and inter-breed comparison between males and females of Mule ducks, Muscovy ducks, and Jinding ducks. Twelve genes (Dmrt1, Amh, Sox9, Tex14, Trim71, Slc26a8, Spam1, Tdrp, Tsga10, Boc, Cxcl14, and Hsd17b3) were identified to be specifically related to testicular development of ducks, and 11 genes (Hsd17b1, Cyp19a1, Cyp17a1, Hhipl2, Tdrp, Uts2r, Cdon, Axin2, Nxph1, Brinp2, and Brinp3) specifically related to the female. Seven genes (Stra8, Dmc1, Terb1, Tex14, Tsga10, Spam1, and Plcd4) were screened to be specifically responsible for the female sterility of Mule ducks, eight genes (Gtsf1, Nalcn, Tat, Slc26a8, Kmo, Plcd4, Aldh4a1 and Hgd) for male sterility, and five genes (Terb1, Stra8, Tex14 Tsga10 and Spam1) for both female and male sterility. This study lays a solid foundation for elucidating the mechanism of avian gonad development.
Data availability statement
The datasets presented in this study can be found in online repositories. The names of the repository/repositories and accession number(s) can be found in the article/Supplementary Material.
Ethics statement
The animal study was reviewed and approved by the Ethics Committee of Wuhan Academy of Agricultural Science.
Author contributions
YY and XL participated in data acquisition, data analysis, and manuscript drafting, contributed equally to the study; PG and YY contributed to conception and design of the study, as well as revised the manuscript; SY, XC, LW, YQ, QX, and LL participated in validation and data curation, as well as summarized the data from the literature search. All authors contributed to manuscript revision, read, and approved the submitted version.
Funding
This work was supported by the Fundamental Research Funds for the Central Universities (Grant No. 2662020DKPY004), the Key Laboratory of Animal Embryo Engineering and Molecular Breeding of Hubei Province (KLAEMB-2022–03) and the National Waterfowl Industry Technology System (CARS-42–48).
Conflict of interest
The authors declare that the research was conducted in the absence of any commercial or financial relationships that could be construed as a potential conflict of interest.
Publisher’s note
All claims expressed in this article are solely those of the authors and do not necessarily represent those of their affiliated organizations, or those of the publisher, the editors and the reviewers. Any product that may be evaluated in this article, or claim that may be made by its manufacturer, is not guaranteed or endorsed by the publisher.
Supplementary material
The Supplementary Material for this article can be found online at: https://www.frontiersin.org/articles/10.3389/fgene.2022.1037810/full#supplementary-material
References
Anderson, E. L., Baltus, A. E., Roepers-Gajadien, H. L., Hassold, T. J., de Rooij, D. G., van Pelt, A. M., et al. (2008). Stra8 and its inducer, retinoic acid, regulate meiotic initiation in both spermatogenesis and oogenesis in mice. Proc. Natl. Acad. Sci. U. S. A. 105 (39), 14976–14980. doi:10.1073/pnas.0807297105
Arango, N. A., Lovell-Badge, R., and Behringer, R. R. (1999). Targeted mutagenesis of the endogenous mouse mis gene promoter: In vivo definition of genetic pathways of vertebrate sexual development. Cell 99 (4), 409–419. doi:10.1016/s0092-8674(00)81527-5
Bai, D. P., Chen, Y., Hu, Y. Q., He, W. F., Shi, Y. Z., Fan, Q. M., et al. (2020). Transcriptome analysis of genes related to gonad differentiation and development in Muscovy ducks. BMC Genomics 21 (1), 438. doi:10.1186/s12864-020-06852-z
Baltus, A. E., Menke, D. B., Hu, Y. C., Goodheart, M. L., Carpenter, A. E., de Rooij, D. G., et al. (2006). In germ cells of mouse embryonic ovaries, the decision to enter meiosis precedes premeiotic DNA replication. Nat. Genet. 38 (12), 1430–1434. doi:10.1038/ng1919
Barske, L. A., and Capel, B. (2010). Sex determination: An avian sexual revolution. Nature 464 (7286), 171–172. doi:10.1038/464171a
Biswas, L., Tyc, K., El Yakoubi, W., Morgan, K., Xing, J., and Schindler, K. (2021). Meiosis interrupted: The genetics of female infertility via meiotic failure. Reproduction 161 (2), R13–r35. doi:10.1530/rep-20-0422
Braun, R. E., Behringer, R. R., Peschon, J. J., Brinster, R. L., and Palmiter, R. D. (1989). Genetically haploid spermatids are phenotypically diploid. Nature 337 (6205), 373–376. doi:10.1038/337373a0
Cao, D., Shi, F., Guo, C., Liu, Y., Lin, Z., Zhang, J., et al. (2021). A pathogenic DMC1 frameshift mutation causes nonobstructive azoospermia but not primary ovarian insufficiency in humans. Mol. Hum. Reprod. 27 (9), gaab058. doi:10.1093/molehr/gaab058
Chen, S., Zhang, G., Shao, C., Huang, Q., Liu, G., Zhang, P., et al. (2014). Whole-genome sequence of a flatfish provides insights into ZW sex chromosome evolution and adaptation to a benthic lifestyle. Nat. Genet. 46 (3), 253–260. doi:10.1038/ng.2890
Chiarugi, P., and Cirri, P. (2003). Redox regulation of protein tyrosine phosphatases during receptor tyrosine kinase signal transduction. Trends biochem. Sci. 28 (9), 509–514. doi:10.1016/s0968-0004(03)00174-9
Cutting, A. D., Ayers, K., Davidson, N., Oshlack, A., Doran, T., Sinclair, A. H., et al. (2014). Identification, expression, and regulation of anti-Müllerian hormone type-II receptor in the embryonic chicken gonad. Biol. Reprod. 90 (5), 106. doi:10.1095/biolreprod.113.116491
Day, J. M., Foster, P. A., Tutill, H. J., Parsons, M. F., Newman, S. P., Chander, S. K., et al. (2008). 17beta-hydroxysteroid dehydrogenase Type 1, and not Type 12, is a target for endocrine therapy of hormone-dependent breast cancer. Int. J. Cancer 122 (9), 1931–1940. doi:10.1002/ijc.23350
Di Fiore, M. M., Santillo, A., and Chieffi Baccari, G. (2014). Current knowledge of D-aspartate in glandular tissues. Amino Acids 46 (8), 1805–1818. doi:10.1007/s00726-014-1759-2
Du, G., Wang, X., Luo, M., Xu, W., Zhou, T., Wang, M., et al. (2020). mRBPome capture identifies the RNA-binding protein TRIM71, an essential regulator of spermatogonial differentiation. Development 147 (8), dev184655. doi:10.1242/dev.184655
Elbrecht, A., and Smith, R. G. (1992). Aromatase enzyme activity and sex determination in chickens. Science 255 (5043), 467–470. doi:10.1126/science.1734525
Esteves, T. C., Balbach, S. T., Pfeiffer, M. J., Araúzo-Bravo, M. J., Klein, D. C., Sinn, M., et al. (2011). Somatic cell nuclear reprogramming of mouse oocytes endures beyond reproductive decline. Aging Cell 10 (1), 80–95. doi:10.1111/j.1474-9726.2010.00644.x
Falvo, S., Santillo, A., Chieffi Baccari, G., Cioffi, F., and Di Fiore, M. M. (2022). d-aspartate and N-methyl-d-aspartate promote proliferative activity in mouse spermatocyte GC-2 cells. Reprod. Biol. 22 (1), 100601. doi:10.1016/j.repbio.2021.100601
Feng, Y., Zhang, S., Peng, X., Yuan, J., Yang, Y., Zhan, H., et al. (2007). Expression analysis of genes putatively involved in chicken gonadal development. Acta Biol. hung. 58 (2), 163–172. doi:10.1556/ABiol.58.2007.2.3
Folsom, L. J., Hjaige, M., Liu, J., Eugster, E. A., and Auchus, R. J. (2019). Germ cell neoplasia in situ complicating 17β-hydroxysteroid dehydrogenase type 3 deficiency. Mol. Cell. Endocrinol. 489, 3–8. doi:10.1016/j.mce.2018.11.014
Friedman, J. R., and Nunnari, J. (2014). Mitochondrial form and function. Nature 505 (7483), 335–343. doi:10.1038/nature12985
Fukami, K., Nakao, K., Inoue, T., Kataoka, Y., Kurokawa, M., Fissore, R. A., et al. (2001). Requirement of phospholipase Cdelta4 for the zona pellucida-induced acrosome reaction. Science 292 (5518), 920–923. doi:10.1126/science.1059042
Geraghty, M. T., Vaughn, D., Nicholson, A. J., Lin, W. W., Jimenez-Sanchez, G., Obie, C., et al. (1998). Mutations in the Delta1-pyrroline 5-carboxylate dehydrogenase gene cause type II hyperprolinemia. Hum. Mol. Genet. 7 (9), 1411–1415. doi:10.1093/hmg/7.9.1411
Goetz, P., Chandley, A. C., and Speed, R. M. (1984). Morphological and temporal sequence of meiotic prophase development at puberty in the male mouse. J. Cell Sci. 65, 249–263. doi:10.1242/jcs.65.1.249
Götz, S., García-Gómez, J. M., Terol, J., Williams, T. D., Nagaraj, S. H., Nueda, M. J., et al. (2008). High-throughput functional annotation and data mining with the Blast2GO suite. Nucleic Acids Res. 36 (10), 3420–3435. doi:10.1093/nar/gkn176
Grant, P. R., Grant, B. R., and Petren, K. (2005). Hybridization in the recent past. Am. Nat. 166 (1), 56–67. doi:10.1086/430331
Greenbaum, M. P., Iwamori, T., Buchold, G. M., and Matzuk, M. M. (2011). Germ cell intercellular bridges. Cold Spring Harb. Perspect. Biol. 3 (8), a005850. doi:10.1101/cshperspect.a005850
Greenbaum, M. P., Yan, W., Wu, M. H., Lin, Y. N., Agno, J. E., Sharma, M., et al. (2006). TEX14 is essential for intercellular bridges and fertility in male mice. Proc. Natl. Acad. Sci. U. S. A. 103 (13), 4982–4987. doi:10.1073/pnas.0505123103
Guioli, S., and Lovell-Badge, R. (2007). PITX2 controls asymmetric gonadal development in both sexes of the chick and can rescue the degeneration of the right ovary. Development 134 (23), 4199–4208. doi:10.1242/dev.010249
Guioli, S., Zhao, D., Nandi, S., Clinton, M., and Lovell-Badge, R. (2020). Oestrogen in the chick embryo can induce chromosomally male ZZ left gonad epithelial cells to form an ovarian cortex that can support oogenesis. Development 147 (4), dev181693. doi:10.1242/dev.181693
Huang, M. C., and Sung, Y. Y. (1988). Cytogenetical analysis of the germ cell in the domestic drake and mule drake. Proc. Natl. Sci. Counc. Repub. China. B 12 (4), 202–208.
Ishishita, S., Kinoshita, K., Nakano, M., and Matsuda, Y. (2016). Embryonic development and inviability phenotype of chicken-Japanese quail F1 hybrids. Sci. Rep. 6, 26369. doi:10.1038/srep26369
Jensen, L. J., Kuhn, M., Stark, M., Chaffron, S., Creevey, C., Muller, J., et al. (2009). STRING 8--a global view on proteins and their functional interactions in 630 organisms. Nucleic Acids Res. 37, D412–D416. doi:10.1093/nar/gkn760
Jose-Miller, A. B., Boyden, J. W., and Frey, K. A. (2007). Infertility. Am. Fam. Physician 75 (6), 849–856.
Kim, H. J., Yoon, J., Matsuura, A., Na, J. H., Lee, W. K., Kim, H., et al. (2015). Structural and biochemical insights into the role of testis-expressed gene 14 (TEX14) in forming the stable intercellular bridges of germ cells. Proc. Natl. Acad. Sci. U. S. A. 112 (40), 12372–12377. doi:10.1073/pnas.1418606112
Koonin, E. V., and Rogozin, I. B. (2003). Getting positive about selection. Genome Biol. 4 (8), 331. doi:10.1186/gb-2003-4-8-331
Koppers, A. J., De Iuliis, G. N., Finnie, J. M., McLaughlin, E. A., and Aitken, R. J. (2008). Significance of mitochondrial reactive oxygen species in the generation of oxidative stress in spermatozoa. J. Clin. Endocrinol. Metab. 93 (8), 3199–3207. doi:10.1210/jc.2007-2616
Kralikova, M., Crha, I., Huser, M., Melounova, J., Zakova, J., Matejovicova, M., et al. (2017). The intracellular concentration of homocysteine and related thiols is negatively correlated to sperm quality after highly effective method of sperm lysis. Andrologia 49 (7), e12702. doi:10.1111/and.12702
Lathrop, W. F., Carmichael, E. P., Myles, D. G., and Primakoff, P. (1990). cDNA cloning reveals the molecular structure of a sperm surface protein, PH-20, involved in sperm-egg adhesion and the wide distribution of its gene among mammals. J. Cell Biol. 111, 2939–2949. doi:10.1083/jcb.111.6.2939
Li, L., Zhang, L., Zhang, Z., Keyhani, N. O., Xin, Q., Miao, Z., et al. (2020). Comparative transcriptome and histomorphology analysis of testis tissues from mulard and Pekin ducks. Arch. Anim. Breed. 63 (2), 303–313. doi:10.5194/aab-63-303-2020
Li, Y., Miao, Y., Chen, J., and Xiong, B. (2021). SIRT6 maintains redox homeostasis to promote porcine oocyte maturation. Front. Cell Dev. Biol. 9, 625540. doi:10.3389/fcell.2021.625540
Li, Z., Wang, S., Gong, C., Hu, Y., Liu, J., Wang, W., et al. (2021). Effects of environmental and pathological hypoxia on male fertility. Front. Cell Dev. Biol. 9, 725933. doi:10.3389/fcell.2021.725933,
Livak, K. J., and Schmittgen, T. D. (2001). Analysis of relative gene expression data using real-time quantitative PCR and the 2(-Delta Delta C(T)) Method. Methods 25 (4), 402–408. doi:10.1006/meth.2001.1262
Luo, G., Hou, M., Wang, B., Liu, Z., Liu, W., Han, T., et al. (2021). Tsga10 is essential for arrangement of mitochondrial sheath and male fertility in mice. Andrology 9 (1), 368–375. doi:10.1111/andr.12889
Maddison, D. C., Alfonso-Núñez, M., Swaih, A. M., Breda, C., Campesan, S., Allcock, N., et al. (2020). A novel role for kynurenine 3-monooxygenase in mitochondrial dynamics. PLoS Genet. 16 (11), e1009129. doi:10.1371/journal.pgen.1009129
Mallet, J. (2005). Hybridization as an invasion of the genome. Trends Ecol. Evol. 20 (5), 229–237. doi:10.1016/j.tree.2005.02.010
Mao, S., Wu, F., Cao, X., He, M., Liu, N., Wu, H., et al. (2016). TDRP deficiency contributes to low sperm motility and is a potential risk factor for male infertility. Am. J. Transl. Res. 8 (1), 177–187.
Menke, D. B., Koubova, J., and Page, D. C. (2003). Sexual differentiation of germ cells in XX mouse gonads occurs in an anterior-to-posterior wave. Dev. Biol. 262 (2), 303–312. doi:10.1016/s0012-1606(03)00391-9
Modarressi, M. H., Behnam, B., Cheng, M., Taylor, K. E., Wolfe, J., and van der Hoorn, F. A. (2004). Tsga10 encodes a 65-kilodalton protein that is processed to the 27-kilodalton fibrous sheath protein. Biol. Reprod. 70 (3), 608–615. doi:10.1095/biolreprod.103.021170
Moriya, Y., Itoh, M., Okuda, S., Yoshizawa, A. C., and Kanehisa, M. (2007). Kaas: An automatic genome annotation and pathway reconstruction server. Nucleic Acids Res. 35, W182–W185. Web Server issue. doi:10.1093/nar/gkm321
Nachtigal, M. W., Hirokawa, Y., Enyeart-VanHouten, D. L., Flanagan, J. N., Hammer, G. D., and Ingraham, H. A. (1998). Wilms' tumor 1 and Dax-1 modulate the orphan nuclear receptor SF-1 in sex-specific gene expression. Cell 93 (3), 445–454. doi:10.1016/s0092-8674(00)81172-1
Oréal, E., Mazaud, S., Picard, J. Y., Magre, S., and Carré-Eusèbe, D. (2002). Different patterns of anti-Müllerian hormone expression, as related to DMRT1, SF-1, WT1, GATA-4, Wnt-4, and Lhx9 expression, in the chick differentiating gonads. Dev. Dyn. 225 (3), 221–232. doi:10.1002/dvdy.10153
Primakoff, P., and Myles, D. G. (2002). Penetration, adhesion, and fusion in mammalian sperm-egg interaction. Science 296 (5576), 2183–2185. doi:10.1126/science.1072029
Rode, B., Dirami, T., Bakouh, N., Rizk-Rabin, M., Norez, C., Lhuillier, P., et al. (2012). The testis anion transporter TAT1 (SLC26A8) physically and functionally interacts with the cystic fibrosis transmembrane conductance regulator channel: A potential role during sperm capacitation. Hum. Mol. Genet. 21 (6), 1287–1298. doi:10.1093/hmg/ddr558
Saez, J. M., De Peretti, E., Morera, A. M., David, M., and Bertrand, J. (1971). Familial male pseudohermaphroditism with gynecomastia due to a testicular 17-ketosteroid reductase defect. I. Studies in vivo. J. Clin. Endocrinol. Metab. 32 (5), 604–610. doi:10.1210/jcem-32-5-604
Saez, J. M., Morera, A. M., De Peretti, E., and Bertrand, J. (1972). Further in vivo studies in male pseudohermaphroditism with gynecomastia due to a testicular 17-ketosteroid reductase defect (compared to a case of testicular feminization). J. Clin. Endocrinol. Metab. 34 (3), 598–600. doi:10.1210/jcem-34-3-598
Saloniemi, T., Welsh, M., Lamminen, T., Saunders, P., Mäkelä, S., Streng, T., et al. (2009). Human HSD17B1 expression masculinizes transgenic female mice. Mol. Cell. Endocrinol. 301 (1-2), 163–168. doi:10.1016/j.mce.2008.10.047
Samanta, L., Agarwal, A., Swain, N., Sharma, R., Gopalan, B., Esteves, S. C., et al. (2018). Proteomic signatures of sperm mitochondria in varicocele: Clinical use as biomarkers of varicocele associated infertility. J. Urol. 200 (2), 414–422. doi:10.1016/j.juro.2018.03.009
Shibusawa, M., Minai, S., Nishida-Umehara, C., Suzuki, T., Mano, T., Yamada, K., et al. (2001). A comparative cytogenetic study of chromosome homology between chicken and Japanese quail. Cytogenet. Cell Genet. 95 (1-2), 103–109. doi:10.1159/000057026
Shibuya, H., Ishiguro, K., and Watanabe, Y. (2014). The TRF1-binding protein TERB1 promotes chromosome movement and telomere rigidity in meiosis. Nat. Cell Biol. 16 (2), 145–156. doi:10.1038/ncb2896
Spiller, C. M., Bowles, J., and Koopman, P. (2012). Regulation of germ cell meiosis in the fetal ovary. Int. J. Dev. Biol. 56, 779–787. doi:10.1387/ijdb.120142pk
Toure, A., Morin, L., Pineau, C., Becq, F., Dorseuil, O., and Gacon, G. (2001). Tat1, a novel sulfate transporter specifically expressed in human male germ cells and potentially linked to rhogtpase signaling. J. Biol. Chem. 276 (23), 20309–20315. doi:10.1074/jbc.M011740200
Trapnell, C., Williams, B. A., Pertea, G., Mortazavi, A., Kwan, G., van Baren, M. J., et al. (2010). Transcript assembly and quantification by RNA-Seq reveals unannotated transcripts and isoform switching during cell differentiation. Nat. Biotechnol. 28 (5), 511–515. doi:10.1038/nbt.1621
Twesten, W., Holterhus, P., Sippell, W. G., Morlot, M., Schumacher, H., Schenk, B., et al. (2000). Clinical, endocrine, and molecular genetic findings in patients with 17beta-hydroxysteroid dehydrogenase deficiency. Horm. Res. 53 (1), 26–31. doi:10.1159/000023509
van de Ven, S., Gardeitchik, T., Kouwenberg, D., Kluijtmans, L., Wevers, R., and Morava, E. (2014). Long-term clinical outcome, therapy and mild mitochondrial dysfunction in hyperprolinemia. J. Inherit. Metab. Dis. 37 (3), 383–390. doi:10.1007/s10545-013-9660-9
Varuzhanyan, G., and Chan, D. C. (2020). Mitochondrial dynamics during spermatogenesis. J. Cell Sci. 133 (14), jcs235937. doi:10.1242/jcs.235937
Wünsch, L. K., and Pfennig, K. S. (2013). Failed sperm development as a reproductive isolating barrier between species. Evol. Dev. 15 (6), 458–465. doi:10.1111/ede.12054
Yang, L., Zhang, X., Liu, S., Zhao, C., Miao, Y., Jin, L., et al. (2021). Cyp17a1 is required for female sex determination and male fertility by regulating sex steroid biosynthesis in fish. Endocrinology 162 (12), bqab205. doi:10.1210/endocr/bqab205
Yen, C. A., and Curran, S. P. (2021). Incomplete proline catabolism drives premature sperm aging. Aging Cell 20 (2), e13308. doi:10.1111/acel.13308
Yoshimura, T., Toyoda, S., Kuramochi-Miyagawa, S., Miyazaki, T., Miyazaki, S., Tashiro, F., et al. (2009). Gtsf1/Cue110, a gene encoding a protein with two copies of a CHHC Zn-finger motif, is involved in spermatogenesis and retrotransposon suppression in murine testes. Dev. Biol. 335 (1), 216–227. doi:10.1016/j.ydbio.2009.09.003
Zhang, H., Jones, R., and Martin-DeLeon, P. A. (2004). Expression and secretion of rat SPAM1(2B1 or PH-20) in the epididymis: Role of testicular lumicrine factors. Matrix Biol. 22 (8), 653–661. doi:10.1016/j.matbio.2003.11.010
Zhang, H., and Martin-DeLeon, P. A. (2003). Mouse Spam1 (PH-20) is a multifunctional protein: Evidence for its expression in the female reproductive tract. Biol. Reprod. 69 (2), 446–454. doi:10.1095/biolreprod.102.013854
Zheng, Y., Deng, X., and Martin-DeLeon, P. A. (2001). Lack of sharing of Spam1 (Ph-20) among mouse spermatids and transmission ratio distortion. Biol. Reprod. 64 (6), 1730–1738. doi:10.1095/biolreprod64.6.1730
Zhou, L. Y., Wang, D. S., Kobayashi, T., Yano, A., Paul-Prasanth, B., Suzuki, A., et al. (2007). A novel type of P450c17 lacking the lyase activity is responsible for C21-steroid biosynthesis in the fish ovary and head kidney. Endocrinology 148 (9), 4282–4291. doi:10.1210/en.2007-0487
Keywords: transcriptome, sex, sterility, embryonic gonad development, gene, Mule ducks
Citation: Yang Y, Li X, Ye S, Chen X, Wang L, Qian Y, Xin Q, Li L and Gong P (2022) Identification of genes related to sexual differentiation and sterility in embryonic gonads of Mule ducks by transcriptome analysis. Front. Genet. 13:1037810. doi: 10.3389/fgene.2022.1037810
Received: 06 September 2022; Accepted: 10 October 2022;
Published: 26 October 2022.
Edited by:
Shahin Eghbalsaied, Islamic Azad University, Isfahan, IranReviewed by:
Xiaoyun He, Institute of Animal Sciences, (CAAS), ChinaMin Xiao, The Obstetrics and Gynecology Hospital of Fudan University, China
Copyright © 2022 Yang, Li, Ye, Chen, Wang, Qian, Xin, Li and Gong. This is an open-access article distributed under the terms of the Creative Commons Attribution License (CC BY). The use, distribution or reproduction in other forums is permitted, provided the original author(s) and the copyright owner(s) are credited and that the original publication in this journal is cited, in accordance with accepted academic practice. No use, distribution or reproduction is permitted which does not comply with these terms.
*Correspondence: Ping Gong, Z29uZ3BpbmcwOUBmb3htYWlsLmNvbQ==
†These authors have contributed equally to this work and share first authorship