- 1Department of Rheumatology and Immunology, West China Hospital, Sichuan University, Chengdu, China
- 2State Key Laboratory of Biotherapy and Cancer Center, West China Hospital, Sichuan University, Chengdu, China
- 3State Key Laboratory of Biotherapy, Department of Gastrointestinal Surgery, West China Hospital, Chengdu, China
- 4State Key Laboratory of Biotherapy, Department of Laboratory Medicine/Research Centre of Clinical Laboratory Medicine, West China Hospital, Chengdu, China
Brachydactyly type A1 (BDA1) is an autosomal dominant inherited disease characterized by the shortness/absence of the middle phalanges, which can be induced by mutations in the Indian hedgehog gene (IHH). Rheumatoid arthritis (RA) is a chronic, systemic autoimmune disease characterized by joint destruction, synovitis, and the presence of autoantibodies. In this study, the proband was diagnosed with both BDA1 and RA. We performed whole-exome sequencing in a four-generation Chinese family to investigate their inherited causal mutation to BDA1. A novel in-frame insertion variant in IHH: NM_002,181.4: c.383_415dup/p.(R128_H138dup) was identified in the BDA1 pedigree. This insertion of 11 amino acids was located in the highly conserved amino-terminal signaling domain of IHH and co-segregated with the disease status. This adds one to the total number of different IHH mutations found to cause BDA1. Moreover, we found a potential causal germline variant in CRY1 for a molecular biomarker of RA (i.e., a high level of anti-cyclic citrullinated peptide). Collectively, we identified novel variants in IHH for inherited BDA1, which highlights the important role of this gene in phalange development.
Introduction
Brachydactyly (BD) is a term referring to disproportionate shortening of fingers and toes and constitutes a heterogeneous group of abnormal development of phalanges, metacarpals, or both (Temtamy and Aglan, 2008) and can be divided into simplex and complex short-finger disease. Simplex includes five clinical types, A, B, C, D, and E, and complex is when there are other symptoms in addition to short fingers, such as facial abnormalities and hypertension. Typically, these include Robinow syndrome, Feingold syndrome, and Temtamy preaxial brachydactyly syndrome (Fitch, 1979; Lyu et al., 2019). BD type A (BDA) is confined to middle phalange shortening, and according to the number and location of affected digits, BDA includes five subtypes (BDA1-5). Except for types A3 and D, whose prevalence is approximately 2%, the other types are all rare diseases (Temtamy and Aglan, 2008). To date, the cause of BD is not fully understood, but simplex BD is mostly inherited in an autosomal dominant manner. Most BD patients will be treated only when it affects functions or for cosmetic reasons.
BD type A1 (BDA1, OMIM: 112500) is an autosomal dominant inherited disease characterized by the shortening or absence of the middle phalanges of most digits (Temtamy and Aglan, 2008). In addition to BDA1, some patients with complex syndromes may have scoliosis, nystagmus, developmental delay, or intellectual disability (Byrnes et al., 2009; Ho et al., 2018). Genetic attribution of BDA1 to IHH mutations was first revealed in 2001 (Gao et al., 2001). Since then, increasing genetic evidence has shown that several mutations have been identified in IHH, whose protein product plays a critical role in growth, patterns, and morphogenesis (Gao et al., 2001; Giordano et al., 2003; Byrnes et al., 2009; Jang et al., 2015; Ho et al., 2018; Shen et al., 2019; Yang et al., 2020).
IHH is an important member of the HH family, which is produced by prehypertrophic chondrocytes. It is related to the formation and differentiation of cartilages and bones (Daoussis et al., 2015; Bechtold et al., 2019; Al-Azab et al., 2020). When the IHH gene is mutated, it will weaken the transmission of hedgehog signals, thereby affecting cartilage development. On the other hand, many studies have found that in a variety of arthritis, including rheumatoid arthritis (RA) (Al-Azab et al., 2018), osteoarthritis (Wei et al., 2012), and ankylosing spondylitis (Daoussis et al., 2015), the IHH expression level is abnormally elevated. In summary, IHH is a key regulator involved in bones and cartilages.
RA is a common chronic autoimmune disease characterized by progressive joint destruction and autoantibodies and has a high disability rate (Weyand and Goronzy, 2021). The mechanism underlying RA is complex, and both genetic and environmental factors can lead to its pathogenesis (Aletaha and Smolen, 2018). In RA, anti-citrullinated protein antibodies (ACPAs) and the rheumatoid factor (RF) have been incorporated into the classification criteria. ACPAs are a key diagnostic indicator of RA and can be positive in the early stage of disease (Van Venrooij et al., 2011). Cyclic citrullinated peptide (CCP) is a test substrate for detecting ACPAs with high specificity and sensitivity. Up to 70% of RA patients are positive for anti-CCP antibodies, and the titer level is highly correlated with the prognosis of RA (Ge and Holmdahl, 2019).
In this study, we investigated the clinical and genetic characteristics of a four-generation Chinese family segregating BDA1. We present herein the clinical and radiographic findings associated with a novel IHH insertion variant in 12 affected family members and summarize all BDA1-related IHH mutations, expanding the clinical and genetic spectrum of BDA1. Additionally, we aimed to investigate the potential germline variant to the high level of the RA-related indicator (i.e., anti-CCP).
Materials and Methods
Subjects
The BDA1-affected family was recruited from West China Hospital for shorted and malformed digits. There were 27 individuals in the BDA1-affected family, including 12 affected individuals. We examined five of these subjects, including bilateral or right radiographs of the hands and feet. Peripheral blood samples were obtained from five affected individuals as well as from three healthy family members. Whole-exome sequencing (WES) was performed on seven individuals of this family, four affected and three normal people. Our study was approved by the Ethics Committee of the West China Hospital, Sichuan University.
Whole-Exome Sequencing and Variant Calling
Genomic DNA was extracted from peripheral blood using standard protocols of the Blood DNA Mini Kit (Foregene Co., Ltd., Chengdu, China). A minimum of 200 ng of DNA was used for constructing sequencing libraries, which were generated using the Agilent SureSelect Human All Exon V6 kit (Agilent Technologies, CA, United States) following the manufacturer’s recommendations. The captured pools were combined and sequenced on the Illumina Novaseq 6000 platform (2 × 150 bp). Variant calling was performed with a sophisticated pipeline as described previously (Shu et al., 2018; Luo et al., 2021a; Luo et al., 2021b; Chen et al., 2021; Zhang et al., 2021; Liao et al., 2022). Briefly, by using Burrows–Wheeler Aligner (BWA) and GATK4.0 with default parameters, 550 million uniquely mapped reads with MAPQ ≥ 30 were generated, covering 97.97% of target regions at least 30×. A variant allele frequency of 30–70% (variant reads/total reads) was defined as heterozygous, and a variant allele frequency >90% was defined as homozygous. Functional prediction was conducted with SIFT, Polyphen2, and LRT.
Sanger Sequencing Validation
The variant location was amplified in a single PCR by using primers specific for the region upstream of IHH exon 2 (5′-GCGCCTACACCTGCACCTC-3′ and 5′-GGCGGGCTCTTCACCTTCTC-3′). The candidate IHH variant was validated by Sanger sequencing following the standard protocol (Moriyama et al., 2021); alleles were isolated by T-cloning and sequenced by a sequencing primer (5′-CAAGGACCGCCTGAACTCGC-3′), and its pathogenicity was classified following ACMG/AMP Guidelines (Richards et al., 2015).
Results
Clinical Presentation
The proband (III-6) was diagnosed with severe RA at the age of 45 years. Upon physical examination, we found that her fingers and toes shortened to varying degrees, and the proximal interphalangeal joints, metacarpophalangeal (MCP) joints, and wrist joints on both sides were swollen and tender. Laboratory tests as the initial presentation showed a high level of RF (49.0 IU/ml, normal: <20), anti-CCP antibody (486 U/ml, normal: <17), immunoglobulin (Ig) G (19.2 g/L, normal: 8.00–15.50), IgA (4,340 mg/L, normal: 836–2900), C reactive protein (CRP) (9.92 mg/L, normal:<5), and positive antibodies, including antinuclear antibodies (ANA) (+1:320, grain type), anti-SSA (++), and anti-Ro-52 (+++) (Table 1). The blood routine test, the urine routine test, hepatic and renal functions, calcium and phosphorous levels in the serum, anti-neutrophil cytoplasmic antibodies, anticardiolipin antibodies, serum C3 and C4 levels, and IgM had normal limits. In addition, X-ray results showed that she had osteoporosis in both hands and feet, bone hyperplasia in hands, the absence of middle phalanges of the second and fifth figures in both hands and of the fourth finger in the left hand, the absence of phalanges of the second to fifth toes in both feet, and shortening of middle phalanges of the third and fourth fingers in the right and of the third finger in left hands; the MCP spaces are uneven across all hands and multiple cystic shadows below the surface of the wrist joint (Figure 1). Therefore, this proband was diagnosed as both BDA1 and RA. Subsequently, we collected her family history and constructed the analysis on the pedigree of the four-generation family for BDA1. Totally, 27 individuals have available information of fingers, including 12 individuals with self-reported shortened fingers (Figure 2). Finally, blood samples were collected from seven family members (four self-reported affected and three normal individuals). After X-ray evaluation, all these four affected individuals manifested shortening of the middle phalanges of all digits. The proband’s father (II-5) showed the absence of the middle phalanges of the bilateral fifth fingers and the second to fifth toes and the shortening of the middle and distal phalanges of the bilateral second to fourth fingers (Figure 1). One of her sons (IV-3) presented with middle phalanges without bilateral fifth fingers and bilateral third to fifth toes, shortening of all distal phalanges of limbs, second to fourth middle phalanges in hands, and second middle phalanges in feet (Figure 1). Her little son (IV-4) exhibited that the fifth middle phalanges in both hands and feet were absent, all distal phalanges of limbs and middle phalanges of the bilateral third and fourth fingers were shortened, and the middle phalanges of the second to fourth toes and the second finger of the right hand were fused to the distal phalanges (Figure 1). In addition, although no clinical signs or symptoms (i.e., joint pain and swelling, morning stiffness) of RA have been self-reported for other family members, the proband’s brother (a nonaffected BDA1 individual, III-8) showed ANA (+1:100, grain type), anti-Ro-52 (+), RF 35.50 IU/ml and anti-CCP >500.0 U/ml (Table 1), which is the molecular sign of RA and is even higher than that of the proband with RA.
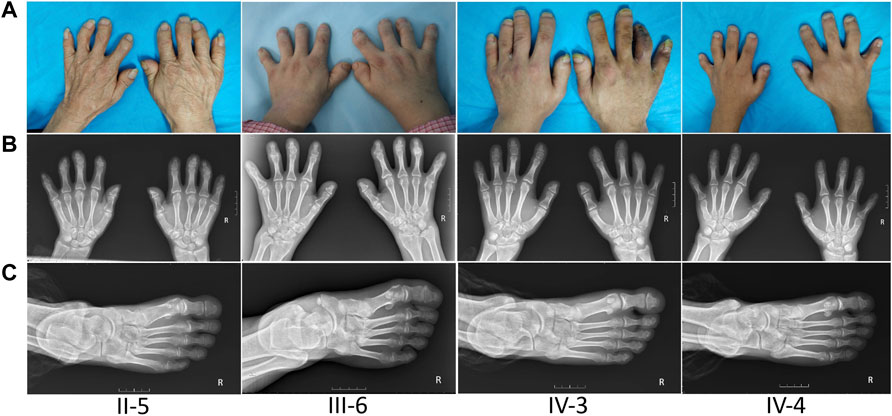
FIGURE 1. Appearance and radiological findings of other family members with BDA1. (A) Shortened fingers and radial deviation of other affected individuals in this family. (B) Radiographic images of other patients’ hands: absence of middle phalanges of the second, fourth, and/or fifth fingers (II-5, II-6, IV-3, IV-4) in both hands, and the middle phalanges of the second finger of the right hand were fused to the distal phalanges (IV-4). (C) Radiographic images of other patients’ right foot: abnormally shortened toes and the absence of phalanges.
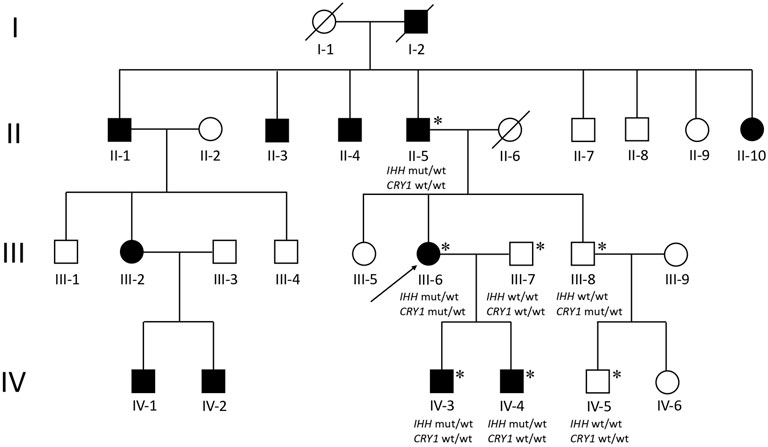
FIGURE 2. Pedigree of a four-generation family with BDA1. Filled symbols represent affected individuals; open symbols represent unaffected individuals; squares depict males, and circles depict females. The proband is indicated by an arrow. Diagonal lines indicate the proband. Asterisks represent participants who participated in WES testing. Wt, wild type; mut, mutant.
Genetic Analysis
We performed WES for for BDA1 patients (II-5, III-6, IV-3, and IV-4) and three normal family members (III-7, III-8, and IV-5) to screen out the causal mutation for this BDA1 family (Figure 2). A total of 42,106 nonsilent variants were identified in coding regions or splicing sites. Due to the low prevalence of BDA1, we excluded variants with a minor allele frequency greater than 1% in any ethnic population according to a public database with 141,456 individuals [i.e., gnomAD (Karczewski et al., 2020)], leaving 3,338 variants for further analysis. Given that all the affected individuals carry the variant allele while normal individuals have the wild-type genotype, 28 variants co-segregated with the disease phenotype in this family. We focused on the five variants that are not presented in the gnomAD database (https://gnomad.broadinstitute.org/), including RAVER2 NM_018,211.4.2:c.C125G/p.(P42R), PRDM9 NM_020,227.4:c.G979A/p.(E327K), KRT84 NM_ 033,045.4:c.G263C/p.(G88A), IFNGR2 NM_ 005,534.4:c.G943A/p.(D315N), and IHH NM_002,181.4:c.383_415dup/p.(R128_H138dup) (Figure 3) in all affected family members (Supplementary Table S1). Functional prediction revealed two potential damaging nonsynonymous variants in PRDM9 and IFNGR2. Moreover, the insertion of 11 amino acids in the conserved domain of IHH, which has been validated with Sanger sequencing (Figure 3D), is likely pathogenic (i.e., PM2, PM4, PP1, and PP4) according to the AMP/ACMG guidelines for the interpretation of sequence variants (Richards et al., 2015) and may impact its protein structure (Figure 3E). Intriguingly, previous studies have shown that variants in IHH can induce BDA1; thus, we considered the causal status of the IHH variant in this BDA1 family.
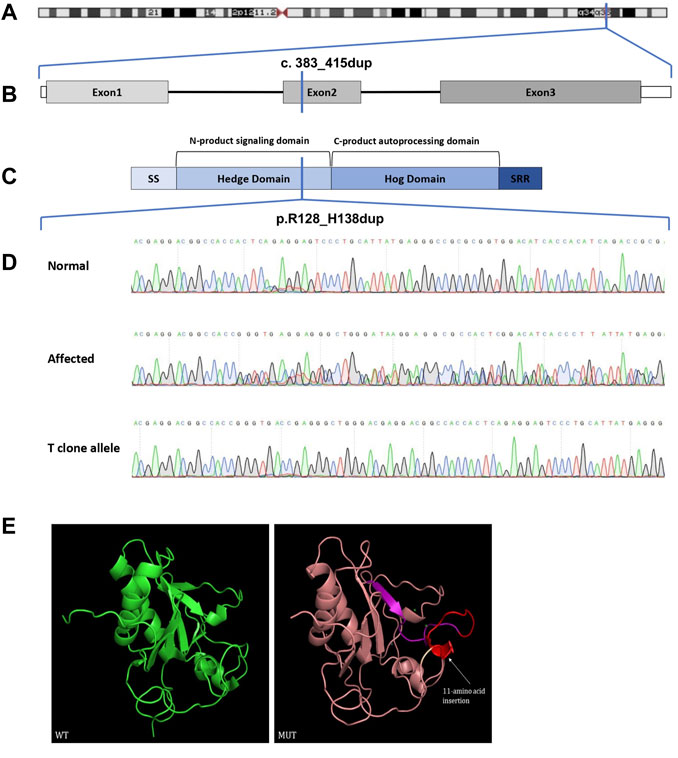
FIGURE 3. Chromosome location, gene structure, protein structure, and sequence analysis of the Indian Hedgehog. (A) Chromosome 2 and the location of IHH drawn to scale. (B) Genomic DNA map for IHH. (C) IHH protein structure with key domains, regions, and the mutation indicated (SS: signal sequence; SRR: sterol recognition region). (D) Sanger sequencing chromatograms. (E) Three-dimensional structure modeling of wild-type and mutant proteins. The insert 11-amino acid sequence (red) is same as the forward sequence (purple). WT, wild-type; MUT, mutant.
Potential Causal Variant for High Anti-CCP Levels
The proband was diagnosed with RA, and her elder brother (III-8, a nonaffected BDA1 individual) also showed ANA (+1:100, grain type), anti-Ro-52 (+), RF 35.50 IU/ml, and anti-CCP >500.0 U/ml. Although this individual exhibited a very high anti-CCP level, which is relatively unique in RA patients, he had no clinical signs or symptoms (i.e., joint pain and swelling, morning stiffness). We considered that this individual may have a high risk of developing RA. Therefore, with the same co-segregation strategy (two affected vs. five unaffected individuals) as BDA1, we identified three germline variant candidates for individuals with abnormal anti-CCP levels in this family. These variants were not present in the gnomAD database and were predicted to be damaging with different methods (i.e., SIFT, Polyphen2, and LRT), including p.I353V in CRY1, p.R119Q in TMEM119, and p.G573D in RBM26 (Supplementary Table S1). No pathogenic history of RA was self-reported from other members, and no available sample for determining anti-CCP levels prevented us from further narrowing down the candidates. However, a few reports have linked CRY1 to RA, thus making it the top candidate for familial high anti-CCP levels.
Discussion
Locus 2q35-q36 was identified in response to BDA1 in a previous study (Gao et al., 2001; Kirkpatrick et al., 2003). The IHH gene within this locus has not been revealed as one of the causes of BDA1. Since then, a series of variants in IHH have been reported to be associated with BDA1 according to HGMD (http://www.hgmd.cf.ac.uk/). In this study, we reported the clinical characterization of four related subjects affected by BDA1. Radiographic investigation of the hands and feet revealed that all four individuals had bilateral generalized shortness of fingers and toes caused by missing or shortened middle phalanges of digits 2–5. By WES, we identified that these subjects are associated with a novel IHH insertion mutation p. R128_H138dup, which is the only report.
IHH encodes a signaling protein of the Hedgehog family and is essential for bone formation (St-Jacques et al., 1999). Ihh knockout mice exhibit embryonic lethality that may be attributed to reduced chondrocyte proliferation, maturation of chondrocytes at inappropriate positions, and failure of osteoblast development in endochondral bones (St-Jacques et al., 1999). The IHH protein contains two major domains, including the Hedgehog amino-terminal signaling domain and the C-product autoprocessing domain, which have proteolytic enzyme activity and cholesterol functions. IHH is very conserved among different vertebrates. For instance, it translated into a protein with a length of 411 amino acid residues in both humans and mice, with 95% identity and 97% positivity, supporting its important role in skeletal development in all vertebrates. The majority of reported mutations are located in three hotspots without any truncating variants, including codon 95, 100, and 131, which are adjacent to special locations on the surface of the crystal structure in the conserved Hedge domain (Gao et al., 2001). Consistently, in a mouse model with mutated p.E95K of IHH, both Ihh +/E95K and Ihh E95K/E95K mice showed shortened middle phalanges (Gao et al., 2009) compared with normal phalanges in mice that are heterozygous for Ihh null mutation heterozygous and embryonic lethality for homozygous Ihh knockout mice (St-Jacques et al., 1999). This evidence suggested a dominant effect rather than loss of functions of IHH variants. In our study, R128_H138dup includes hot spot 131 and thus may interfere with the interaction of IHH with its binding partners (e.g., PTCH1 and HIP1) (Gao et al., 2001; Gao et al., 2009), according to the predicted protein structure (Figure 3E).
Moreover, two members of this family had very high anti-CCP levels, including the proband who was diagnosed with RA. After filtering the candidate variants with a co-segregation strategy, only CRY1 out of three candidates was reported to be related to RA. CRY1 is present in immune and endocrine cells and is expressed in a circadian manner in human cells (Esquifino et al., 2007). When arthritis is induced in a mouse model, CRY1 knockout mice will experience maximal exacerbation of joint swelling and upregulation of essential cytokines of arthritis, including TNF-α and IL-6 (Hashiramoto et al., 2010). In addition, a positive association of IHH expression with RA was noticed in a previous study (Al-Azab et al., 2018). Speculatively, the CRY1 variant may increase the anti-CCP level, while the combination of IHH and CRY1 variants induces RA development with a high anti-CCP level. However, due to the unavailability of samples from other family members, this hypothesis should be estimated through experimental procedures in the future.
Data Availability Statement
The datasets for this article are not publicly available due to concerns regarding participant/patient anonymity. Requests to access the datasets should be directed to the corresponding author.
Ethics Statement
The studies involving human participants were reviewed and approved by Ethics Committee of West China Hospital, Sichuan University. The patients/participants provided their written informed consent to participate in this study. Written informed consent was obtained from the individual(s) for the publication of any potentially identifiable images or data included in this article.
Author Contributions
FZ and HL designed the study and wrote the manuscript. HL, QX, and GY undertook patient care, collecting samples and clinical information. FZ, XX, YS, WC, and HX undertook bioinformatics analysis. HX, GY, and QX revised the manuscript. All authors reviewed the manuscript and approved the final version.
Funding
This work was supported by the Sichuan Science and Technology Program (Grant numbers 2021JDRC0045, 2021JDRC0169, 2021YJ0472, 2021YFS0164, and 2022YFS0202), the Clinical Research Incubation Project of West China Hospital, Sichuan University (Grant numbers 2019HXFH038 and 2021HXFH018), and the 1.3.5 Project for Disciplines of Excellence, West China Hospital, Sichuan University (Grant number ZYJC21024 and ZYYC20003).
Conflict of Interest
The authors declare that the research was conducted in the absence of any commercial or financial relationships that could be construed as a potential conflict of interest.
Publisher’s Note
All claims expressed in this article are solely those of the authors and do not necessarily represent those of their affiliated organizations or those of the publisher, the editors, and the reviewers. Any product that may be evaluated in this article or claim that may be made by its manufacturer is not guaranteed or endorsed by the publisher.
Acknowledgments
We thank all the family members for their cooperation in this study.
Supplementary Material
The Supplementary Material for this article can be found online at: https://www.frontiersin.org/articles/10.3389/fgene.2022.814786/full#supplementary-material
References
Al-Azab, M., Wang, B., Elkhider, A., Walana, W., Li, W., Yuan, B., et al. (2020). Indian Hedgehog Regulates Senescence in Bone Marrow-Derived Mesenchymal Stem Cell through Modulation of ROS/mTOR/4EBP1, p70S6K1/2 Pathway. Aging 12, 5693–5715. doi:10.18632/aging.102958
Al-Azab, M., Wei, J., Ouyang, X., Elkhider, A., Walana, W., Sun, X., et al. (2018). TL1A Mediates Fibroblast-like Synoviocytes Migration and Indian Hedgehog Signaling Pathway via TNFR2 in Patients with Rheumatoid Arthritis. Eur. Cytokine Netw. 29, 27–35. doi:10.1684/ecn.2018.0405
Aletaha, D., and Smolen, J. S. (2018). Diagnosis and Management of Rheumatoid Arthritis. Jama 320, 1360–1372. doi:10.1001/jama.2018.13103
Bechtold, T. E., Kurio, N., Nah, H. D., Saunders, C., Billings, P. C., and Koyama, E. (2019). The Roles of Indian Hedgehog Signaling in TMJ Formation. Int. J. Mol. Sci. 20. doi:10.3390/ijms20246300
Byrnes, A. M., Racacho, L., Grimsey, A., Hudgins, L., Kwan, A. C., Sangalli, M., et al. (2009). Brachydactyly A-1 Mutations Restricted to the central Region of the N-Terminal Active Fragment of Indian Hedgehog. Eur. J. Hum. Genet. 17, 1112–1120. doi:10.1038/ejhg.2009.18
Chen, H. N., Shu, Y., Liao, F., Liao, X., Zhang, H., Qin, Y., et al. (2021). Genomic Evolution and Diverse Models of Systemic Metastases in Colorectal Cancer. Gut 71 (2), 322–332. doi:10.1136/gutjnl-2020-323703
Daoussis, D., Filippopoulou, A., Liossis, S.-N., Sirinian, C., Klavdianou, K., Bouris, P., et al. (2015). Anti-Tnfα Treatment Decreases the Previously Increased Serum Indian Hedgehog Levels in Patients with Ankylosing Spondylitis and Affects the Expression of Functional Hedgehog Pathway Target Genes. Semin. Arthritis Rheum. 44, 646–651. doi:10.1016/j.semarthrit.2015.01.004
Esquifino, A. I., Cano, P., Jiménez-Ortega, V., Fernández-Mateos, P., and Cardinali, D. P. (2007). Neuroendocrine-immune Correlates of Circadian Physiology: Studies in Experimental Models of Arthritis, Ethanol Feeding, Aging, Social Isolation, and Calorie Restriction. Endocr 32, 1–19. doi:10.1007/s12020-007-9009-y
Fitch, N. (1979). Classification and Identification of Inherited Brachydactylies. J. Med. Genet. 16, 36–44. doi:10.1136/jmg.16.1.36
Gao, B., Guo, J., She, C., Shu, A., Yang, M., Tan, Z., et al. (2001). Mutations in IHH, Encoding Indian Hedgehog, Cause Brachydactyly Type A-1. Nat. Genet. 28, 386–388. doi:10.1038/ng577
Gao, B., Hu, J., Stricker, S., Cheung, M., Ma, G., Law, K. F., et al. (2009). A Mutation in Ihh that Causes Digit Abnormalities Alters its Signalling Capacity and Range. Nature 458, 1196–1200. doi:10.1038/nature07862
Ge, C., and Holmdahl, R. (2019). The Structure, Specificity and Function of Anti-citrullinated Protein Antibodies. Nat. Rev. Rheumatol. 15, 503–508. doi:10.1038/s41584-019-0244-4
Giordano, N., Gennari, L., Bruttini, M., Mari, F., Meloni, I., Baldi, C., et al. (2003). Mild Brachydactyly Type A1 Maps to Chromosome 2q35-Q36 and Is Caused by a Novel IHH Mutation in a Three Generation Family. J. Med. Genet. 40, 132–135. doi:10.1136/jmg.40.2.132
Hashiramoto, A., Yamane, T., Tsumiyama, K., Yoshida, K., Komai, K., Yamada, H., et al. (2010). Mammalian Clock Gene Cryptochrome Regulates Arthritis via Proinflammatory Cytokine TNF-α. J. Immunol. 184, 1560–1565. doi:10.4049/jimmunol.0903284
Ho, R., Mcintyre, A. D., Kennedy, B. A., and Hegele, R. A. (2018). Whole-exome Sequencing Identifies a Novel IHH Insertion in an Ontario Family with Brachydactyly Type A1. SAGE Open Med. Case Rep. 6, 2050313x18818711. doi:10.1177/2050313X18818711
Jang, M.-A., Kim, O.-H., Kim, S. W., and Ki, C.-S. (2015). Identification of p.Glu131Lys Mutation in the IHH Gene in a Korean Patient with Brachydactyly Type A1. Ann. Lab. Med. 35, 387–389. doi:10.3343/alm.2015.35.3.387
Karczewski, K. J., Francioli, L. C., Tiao, G., Cummings, B. B., Alföldi, J., Wang, Q., et al. (2020). The Mutational Constraint Spectrum Quantified from Variation in 141,456 Humans. Nature 581, 434–443. doi:10.1038/s41586-020-2308-7
Kirkpatrick, T. J., Au, K. S., Mastrobattista, J. M., Mccready, M. E., Bulman, D. E., and Northrup, H. (2003). Identification of a Mutation in the Indian Hedgehog (IHH) Gene Causing Brachydactyly Type A1 and Evidence for a Third Locus. J. Med. Genet. 40, 42–44. doi:10.1136/jmg.40.1.42
Liao, X., Xia, X., Su, W., Yan, H., Ma, Y., Xu, L., et al. (2022). Association of Recurrent APOBEC3B Alterations with the Prognosis of Gastric-type Cervical Adenocarcinoma. Gynecol. Oncol. 165 (1), 105–113. doi:10.1016/j.ygyno.2022.01.036
Luo, H., Xia, X., Kim, G. D., Liu, Y., Xue, Z., Zhang, L., et al. (2021). Characterizing Dedifferentiation of Thyroid Cancer by Integrated Analysis. Sci. Adv. 7. doi:10.1126/sciadv.abf3657
Luo, H., Liao, X., Qin, Y., Hou, Q., Xue, Z., Liu, Y., et al. (2021). Longitudinal Genomic Evolution of Conventional Papillary Thyroid Cancer with Brain Metastasis. Front. Oncol. 11, 620924. doi:10.3389/fonc.2021.620924
Lyu, Z. J., Wang, Z. H., Lu, S. X., Liu, P. R., and Tian, J. (2019). Brachydactyly and the Molecular Mechanisms of Digit Formation. Yi Chuan 41, 1073–1083. doi:10.16288/j.yczz.19-100
Moriyama, T., Yang, W., Smith, C., Pui, C.-H., Evans, W. E., Relling, M. V., et al. (2021). Comprehensive Characterization of Pharmacogenetic Variants in TPMT and NUDT15 in Children with Acute Lymphoblastic Leukemia. Pharmacogenetics and Genomics 32 (2), 60–66. doi:10.1097/FPC.0000000000000453
Richards, S., Aziz, N., Bale, S., Bick, D., Das, S., Gastier-Foster, J., et al. (2015). Standards and Guidelines for the Interpretation of Sequence Variants: a Joint Consensus Recommendation of the American College of Medical Genetics and Genomics and the Association for Molecular Pathology. Genet. Med. 17, 405–424. doi:10.1038/gim.2015.30
Shen, L., Ma, G., Shi, Y., Ruan, Y., Yang, X., Wu, X., et al. (2019). p.E95K Mutation in Indian Hedgehog Causing Brachydactyly Type A1 Impairs IHH/Gli1 Downstream Transcriptional Regulation. BMC Genet. 20, 10. doi:10.1186/s12863-018-0697-5
Shu, Y., Zhang, W., Hou, Q., Zhao, L., Zhang, S., Zhou, J., et al. (2018). Prognostic Significance of Frequent CLDN18-Arhgap26/6 Fusion in Gastric Signet-Ring Cell Cancer. Nat. Commun. 9, 2447. doi:10.1038/s41467-018-04907-0
St-Jacques, B., Hammerschmidt, M., and Mcmahon, A. P. (1999). Indian Hedgehog Signaling Regulates Proliferation and Differentiation of Chondrocytes and Is Essential for Bone Formation. Genes Dev. 13, 2072–2086. doi:10.1101/gad.13.16.2072
Temtamy, S. A., and Aglan, M. S. (2008). Brachydactyly. Orphanet J. Rare Dis. 3, 15. doi:10.1186/1750-1172-3-15
Van Venrooij, W. J., Van Beers, J. J. B. C., and Pruijn, G. J. M. (2011). Anti-CCP Antibodies: the Past, the Present and the Future. Nat. Rev. Rheumatol. 7, 391–398. doi:10.1038/nrrheum.2011.76
Wei, F., Zhou, J., Wei, X., Zhang, J., Fleming, B. C., Terek, R., et al. (2012). Activation of Indian Hedgehog Promotes Chondrocyte Hypertrophy and Upregulation of MMP-13 in Human Osteoarthritic Cartilage. Osteoarthritis and Cartilage 20, 755–763. doi:10.1016/j.joca.2012.03.010
Weyand, C. M., and Goronzy, J. J. (2021). The Immunology of Rheumatoid Arthritis. Nat. Immunol. 22, 10–18. doi:10.1038/s41590-020-00816-x
Yang, Q., Wang, J., Tian, X., Shen, F., Lan, J., Zhang, Q., et al. (2020). A Novel Variant of IHH in a Chinese Family with Brachydactyly Type 1. BMC Med. Genet. 21, 60. doi:10.1186/s12881-020-01000-6
Keywords: brachydactyly type A1, IHH, insertion variation, rheumatoid arthritis, anti-cyclic citrullinated peptide
Citation: Zeng F, Liu H, Xia X, Shu Y, Cheng W, Xu H, Yin G and Xie Q (2022) Case Report: Brachydactyly Type A1 Induced by a Novel Variant of in-Frame Insertion in the IHH Gene. Front. Genet. 13:814786. doi: 10.3389/fgene.2022.814786
Received: 14 November 2021; Accepted: 30 March 2022;
Published: 20 May 2022.
Edited by:
Aideen McInerney-Leo, The University of Queensland, AustraliaReviewed by:
Jinchen Li, Central South University, ChinaKaren E. Heath, University Hospital La Paz, Spain
Copyright © 2022 Zeng, Liu, Xia, Shu, Cheng, Xu, Yin and Xie. This is an open-access article distributed under the terms of the Creative Commons Attribution License (CC BY). The use, distribution or reproduction in other forums is permitted, provided the original author(s) and the copyright owner(s) are credited and that the original publication in this journal is cited, in accordance with accepted academic practice. No use, distribution or reproduction is permitted which does not comply with these terms.
*Correspondence: Heng Xu, eHVoZW5nODE5MTZAc2N1LmVkdS5jbg==; Geng Yin, eWluZ2VuZzE5NzVAMTYzLmNvbQ==; Qibing Xie, eGllcWliaW5nMTk3MUAxNjMuY29t
†These authors have contributed equally to this work and share first authorship