- 1Hospital Israelita Albert Einstein, São Paulo, SP, Brazil
- 2Instituto da Criança (Children’s Hospital), Hospital Das Clínicas HCFMUSP, Faculdade de Medicina da Universidade de São Paulo, São Paulo, Brazil
- 3VarsOmics, Sociedade Beneficente Israelita Brasileira Albert Einstein, São Paulo, SP, Brazil
- 4Programa de Pós Graduação em Tecnologias Energéticas e Nucleares (PROTEN), UFPE, Recife, Brazil
- 5Departamento de Morfologia e Genética, Universidade Federal de São Paulo, São Paulo, SP, Brazil
- 6Departamento de Medicina Translacional, Área de Genética Médica e Medicina Genômica, Faculdade de Ciências Médicas, Universidade Estadual de Campinas, Campinas, SP, Brazil
Hearing loss (HL) is a common sensory deficit in humans and represents an important clinical and social burden. We studied whole-genome sequencing data of a cohort of 2,097 individuals from the Brazilian Rare Genomes Project who were unaffected by hearing loss to investigate pathogenic and likely pathogenic variants associated with nonsyndromic hearing loss (NSHL). We found relevant frequencies of individuals harboring these alterations: 222 heterozygotes (10.59%) for sequence variants, 54 heterozygotes (2.58%) for copy-number variants (CNV), and four homozygotes (0.19%) for sequence variants. The top five most frequent genes and their corresponding combined allelic frequencies (AF) were GJB2 (AF = 1.57%), STRC (AF = 1%), OTOA (AF = 0.69%), TMPRSS3 (AF = 0.41%), and OTOF (AF = 0.29%). The most frequent sequence variant was GJB2:c.35del (AF = 0.72%), followed by OTOA:p. (Glu787Ter) (AF = 0.61%), while the most recurrent CNV was a microdeletion of 57.9 kb involving the STRC gene (AF = 0.91%). An important fraction of these individuals (n = 104; 4.96%) presented variants associated with autosomal dominant forms of NSHL, which may imply the development of some hearing impairment in the future. Using data from the heterozygous individuals for recessive forms and the Hardy–Weinberg equation, we estimated the population frequency of affected individuals with autosomal recessive NSHL to be 1:2,222. Considering that the overall prevalence of HL in adults ranges from 4–15% worldwide, our data indicate that an important fraction of this condition may be associated with a monogenic origin and dominant inheritance.
1 Introduction
Hearing loss (HL) is the most common sensory deficit in humans. Congenital forms of HL may affect up to 1 in 500 newborns, and approximately 80% of prelingual deafness has a monogenic basis; on the other hand, age-related HL may affect up to 80% of individuals by the age of 80 and has a multifactorial basis in which cumulative noise exposure or other extrinsic damage along with individual genetic predisposition leads to impairment in cochlear transduction of acoustic signals (Yamasoba et al., 2013). Between these two extreme ages, several Mendelian forms of later-onset HL have been described. Overall, studies estimate that more than 360 million people worldwide may suffer the clinical and social burden of HL (Shearer & Smith, 2015).
HL may be classified as syndromic when presented with additional clinical manifestations involving other organs or systems or nonsyndromic when HL is the sole clinical finding. Nonsyndromic hearing loss (NSHL) comprises approximately 70–80% of genetic deafness. HL may also be clinically classified according to type, onset, severity, clinical progression, and acoustic frequency (Shearer et al., 2017).
There are important initiatives to map all genetic forms of HL, such as the Online Mendelian Inheritance in Man® (OMIM) database and the Hereditary Hearing Loss Homepage (G Van Camp & R J H Smith). However, studies with comprehensive genetic tests that include the great majority of known hearing impairment genes still leave over 50% of cases unsolved (Shearer and Smith, 2015), indicating that approximately half of the genetic forms of HL have yet to be elucidated.
Whole genome sequencing is likely the most unbiased method for the diagnosis of monogenic diseases because it does not involve a capture step targeting specific areas, thus providing a much broader net for molecular diagnoses. This method allows the study of sequence variants (including single-nucleotide variants [SNVs] and insertions–deletions [indels]) and copy-number variants (CNVs) not only in the coding regions of chromosomes but also in the great majority of noncoding regions and mitochondrial DNA (Lionel et al., 2018). Therefore, this powerful diagnostic tool may enrich the understanding of the underlying molecular mechanisms of the great majority of known monogenic forms of NSHL.
In this study, we aimed to assess pathogenic and likely pathogenic variants associated with NSHL in a cohort of unaffected Brazilian individuals referred for genome sequencing.
2 Materials and methods
2.1 Case selection: The Brazilian Rare Genomes Project
The Brazilian Rare Genomes Project is a public‒private partnership between Hospital Israelita Albert Einstein and the Brazilian Health Ministry that aims to offer large-scale genomic studies for patients with suspected rare diseases of genetic etiology. The project involves 16 public health institutions from nine states of Brazil.
In the current study, we preselected 2,199 patients who had been referred for molecular investigation with genome sequencing from 2020 to 2021. All clinical data were provided by the participating centers and were collected through a comprehensive pretest form filled in by the attending physician on the online platforms REDCap (Harris et al., 2009; Harris et al., 2019) and PhenoTips (Girdea et al., 2013); medical reports, clinical notes, and pictures of patients were provided to the laboratory in selected cases and were used to gather clinical data for this work if available. Patients were not evaluated by specialists from our team.
All clinical features were tabulated and categorized according to the Human Phenotype Ontology (HPO) database (Köhler et al., 2021). Participating patients were divided into two groups:
1) Patients with HL (HP:0000365, HP:0000399, HP:0000407, HP:0008527, HP:0008610, HP:0008619, HP:0008625, HP:0011476, HP:0011474, HP:0000405, HP:0040119, HP:0008513, HP:0012716, HP:0000408, HP:0008504, HP:0000410, HP:0001757, HP:0008573, HP:0008598, HP:0000365, HP:0008587, HP:0008596, HP:0005101, HP:0000364, HP:0008615, and HP:0009900) and
2) Patients without HL: all population assumptions and projections based on the data gathered in this study were based on this group of patients, considered “unaffected.”
2.2 Ethics statement
All patients or their legal guardians provided written consent before genome analysis. The project and related studies adhered to the Declaration of Helsinki principles for research on human beings and were granted ethics committee approval from all institutions involved (Plataforma Brasil; CAAE#29567220.4.1001.0071).
2.3 Molecular analysis and bioinformatics
Blood collection kits along with strict instructions for a blood draw and sample storage were sent to all participating centers. Peripheral blood samples were collected from all patients and shipped to our core facility in São Paulo, Brazil. DNA from the proband and, when available, from parents was immediately extracted using a QIAsymphony DNA Mini Kit and QIAsymphony automated system (Qiagen, Valencia, CA, United States); we used a NanoDrop 2000 (thresholds: 260/280 ratio ≈1.8 and 260/230 ratio between 1.8 and 2.2) and Qubit® 4 fluorometer using the Qubit® dsDNA HS assay (both Life Technologies, Carlsbad, CA, United States) for DNA quality assessment and quantification, respectively.
DNA fragmentation (Covaris ME220 ultrasonicator) and library preparation (Illumina TruSeq® DNA PCR-Free Library Prep protocol HS [Illumina Inc. San Diego, CA, United States]) strictly followed the best laboratory practices and instructions from the manufacturers. Sequencing was performed on an Illumina NovaSeq® 6000 platform. Complete details regarding genome sequencing steps and validation are in the preprint (Campos Coelho et al., 2021).
Genome data from every patient were aligned to the GRCh38/hg38 reference genome, and variants (SNVs, indels, and CNVs) were called using the DRAGEN Germline pipeline (Illumina, version 3.6.3 or superior); variants were annotated using in-house protocols and analyzed on the Varstation ® Platform (version 2.0, São Paulo, Brazil, www.varstation.com). Quality control (QC)-passing samples were those with a percentage of mapped reads >98%, autosome median coverage ≥20X, uniformity of coverage ≥80%, estimated cross-contamination level <2%, autosome callability ≥95%, percentage of bases that met Q30 scores (Q30 score) ≥ 90%, percentage of chimeric (supplementary) reads <5%, and percentage of mapped reads marked as duplicate <10%.
We assessed the evidence for consanguinity by including questions in the recruitment forms and quantifying the number of runs of homozygosity (NROH) with lengths ≥1 million base pairs (MB), the sum of all NROH lengths (SROH), and the frequency of ROH (FROH). The ROH coordinates were generated during alignment and variant calling using the DRAGEN Germline pipeline (Illumina, version 3.6.3 or superior; see Section 2.3 for more details). We calculated the NROH and SROH for each individual using scripts for R software version 4.1.3. We considered evidence for consanguinity if the individual’s SROH was greater than 123 MB; probable consanguinity ≥79 MB and ≤123 MB; probable nonconsanguinity <79 MB, and no evidence of consanguinity for ≤22 MB SROH (Matalonga et al., 2020).
2.4 Gene selection, gene-disease classification, and clinical impact
The list of genes associated with NSHL was defined based on the aggregated data available in 1) the Online Mendelian Inheritance in Man® (OMIM) database (Online Mendelian Inheritance in Man OMIM®, 2021); 2) a review by Shearer et al. (2017); 3) the Hereditary Hearing Loss Homepage (G Van Camp & R J H Smith); and 4) other available literature.
We used a systematic approach to collect available scientific evidence to qualitatively define the clinical validity of gene-disease associations for all genes, following the framework proposed by the Clinical Genome Resource—ClinGen (Strande et al., 2017). The gene-disease classifications proposed by ClinGen and adopted in this study are as follows: limited, moderate, strong, and definite; for contradictory evidence, genes were classified as disputed and refuted. Several genes studied in our work had already been classified by the ClinGen Gene Curation Working Group and were used as standards for these genes. For the remaining genes not previously classified by ClinGen, our team replicated the ClinGen approach to curate the gene-disease validity.
Using data available in OMIM and the literature, we reviewed the following molecular and clinical characteristics for every gene: inheritance pattern, predominant age of onset of hearing impairment (prelingual or postlingual), type of HL (conductive, sensorineural, mixed, and central), predominant severity (mild: 26–40 dB, moderate: 41–70 dB, severe 71–90 dB, and profound >90 dB), clinical progression (progressive and stable), and predominantly affected frequencies (low: <500 Hz, middle: 501–2000 Hz, and high: >2000 Hz). For the purpose of this study, we grouped moderate (41–55 dB) and moderately severe (56–70 dB) HL into a single group of moderate HL (41–70 dB).
2.5 Variant preselection and classification
We used an in-house script based on Python 3.8 to search for variants of potential clinical interest in genes associated with NSHL for all patients using MANE and RefSeq transcript coordinates as references; we shared this script code in the GITHUB repository at the following address: https://github.com/Varstation/non-syndromic_hearing_loss. These variants were searched in variant call format (VCF) files for every patient according to the following selection criteria:
1) For SNVs and small indels, we preselected all variants previously reported in the ClinVar database that were classified as pathogenic, likely pathogenic, or variants that had a conflicting pathogenicity classification for every patient; variants reported in ClinVar as benign or likely benign were excluded from this preselection process.
2) For CNVs, all copy-number variants (microdeletions or microduplications) that included at least one base pair harbored by coding regions were preselected using AnnotSV (v.2.5) (Geoffroy et al., 2018; Geoffroy et al., 2021); CNVs encompassing solely noncoding regions were not preselected. All CNVs were visually confirmed in BAM files using the Integrative Genomics Viewer (IGV) tool (Robinson et al., 2011).
3) Smaller structural variants (SVs) were also selected using a different approach: unbalanced SVs (microdeletions and microduplications, which may be considered small CNVs), affecting whole genes or predicted leading to frameshift as indicated by AnnotSV. This approach was also used because detecting small CNVs may be a more challenging task and complements the previous step. All unbalanced SVs were visually confirmed in BAM files using IGV. In this study, unbalanced SVs (small CNVs) and CNVs were considered in the same group of CNVs.
4) For mitochondrial variants, only selected variants already associated with HL were searched (Lott et al., 2013).
Regarding sequence variants, we excluded from our analysis 1) variants not present in ClinVar, 2) variants with insufficient read coverage (less than 20X), and 3) variants with a call rate <95% considering the whole sample. For CNVs, we excluded events potentially associated with syndromic conditions: 1) CNVs that involve critical regions for known forms or contiguous-gene microdeletion or microduplication syndromes cataloged by ClinGen (including data from the International Standards for Cytogenomic Arrays—ISCA— Consortium) and 2) CNVs larger than 1 Mb (one megabase).
The complete list of searched nuclear genes is shown in Supplementary Table S1, and the list of mitochondrial variants is shown in Supplementary Table S2.
Sequence variants were classified according to ACMG guidelines (Richards et al., 2015) incorporating later ClinGen working group recommendations. CNVs were classified following the standards of the joint consensus recommendation for the interpretation and reporting of constitutional copy number variants of the American College of Medical Genetics and Genomics (ACMG) and ClinGen (Riggs et al., 2020). For the classification of CNVs, we used the ClinGen web-based CNV classification calculator (cnvcalc.clinicalgenome.org/cnvcalc/).
2.6 Homozygous genotype frequency estimation
We used the Hardy–Weinberg equation to estimate variant homozygous genotype frequencies (q2) considering the respective carrier frequencies (2pq) observed in this study (Edwards and Hardy, 2008). For this purpose, we considered random mating and the approximation p∼1.
2.7 Statistical analysis
We calculated allele counts and frequencies by directly counting genotypes extracted from filtered VCF files using Unix Bash and R software version 4.1 scripts. Next, we verified which ClinVar variants detected in our sample were also detected in the Brazilian ABraOM cohort (Naslavsky et al., 2017). Then, we compared the allele counts using a two-tailed Fisher exact test to assess whether there were significant differences in allele distribution between the samples. The resulting p-values were adjusted using the false discovery rate (FDR) method for multiple comparisons, and the significance level was set at α = 0.05.
3 Results
3.1 Selected cases
Figure 1 shows the workflow for selecting patients, genes, and genetic variants for downstream analysis. Among the 2,199 preselected patients, 13 failed the sample QC (mostly due to median autosome coverage <20X) and were removed from the analysis. Among the remaining 2,186, 89 patients presented with HL: 88 (98.9%) presented with other systemic involvement, such as neurological manifestations (69/88, 78.4%) or growth anomalies (60/88, 68.2%) and were clinically classified as having a syndromic form of HL; only one patient (1.1%) presented isolated (e.g., nonsyndromic) HL; all 89 patients were excluded from our cohort and population calculations.
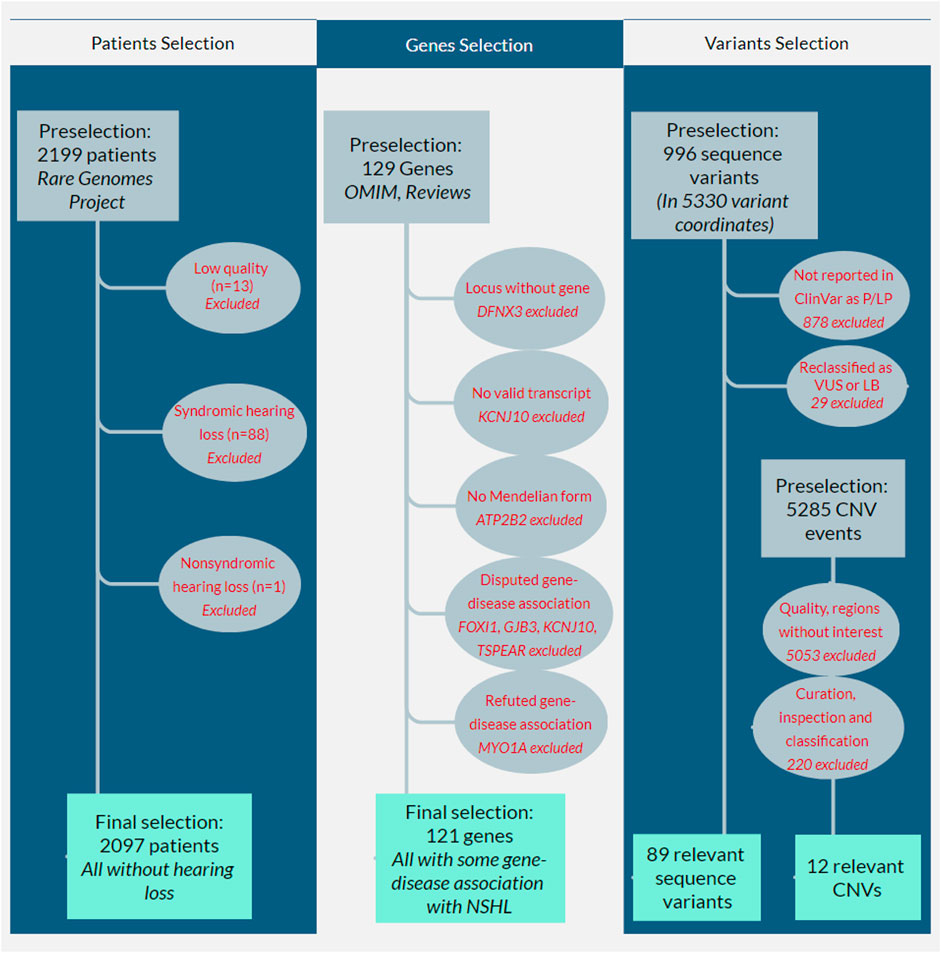
FIGURE 1. Flowcharts showing the selection workflow for patients, genes, and variants. The first column shows the patient selection workflow: 2,199 patients who had been referred for molecular investigation using whole genome sequencing from 2020–2021 were preselected; 13 failed sample quality controls, and 89 patients presented with hearing loss (88 syndromic hearing loss and one with nonsyndromic hearing loss [NSHL]): all were excluded from our cohort and population calculations. The second column shows gene selection: 129 genes associated with NSHL were preselected from the OMIM database, reviews (Shearer et al., 2017; Van Camp and Smith, 2021), and other available literature; three of them were initially excluded because one of them (DFNX3) refers to a locus without a known gene, another (KCNJ10) did not present a valid transcript in MANE or RefSeq databases, and the other (ATP2B2) was not associated with a Mendelian form of hearing loss; four genes (FOXI1, GJB3, KCNJ10, and TSPEAR) were excluded for presenting disputed gene-disease association and another gene (MYO1A) refuted association. The third column shows the variant selection workflow: 1) for sequence variants, 996 variants were preselected in 5,330 variant coordinates, but only 118 were reported in ClinVar as pathogenic or likely pathogenic (P/LP); the remaining 878 were excluded; after our internal curation and reclassification, 29 variants were excluded because they were reclassified as variants of unknown significance (VUS, n = 28) or likely benign (LB, n = 1); 2) for copy-number variants (CNV), the filtering process of 5,285 preselected CNV events eliminated 5,053 CNVs; our internal curation, visual inspection, and classification eliminated another 220.
Therefore, 2,097 patients did not present any form of HL and were included in our definite cohort of unaffected individuals. All data, calculations, and estimates in this work refer to this group of individuals.
Self-reported information regarding consanguinity was available for 1,615 individuals (77.0%). Among those, 1,424 (67.9%) reported no history of known consanguinity in the family, whereas 191 (9.1%) provided positive indications for consanguinity in the family. SROH and NROH were available for 2,069 individuals (98.7%). Mean overall NROH = 5.3 ± 6.6, mean SROH = 30.3 ± 76.4 MB, and mean FROH = 1.1% ± 2.7%. Self-report did not always match the classification using the ROH metrics. Our preliminary data suggest that approximately 8.0% (data not shown) of individuals had a mismatch between self-report and SROH data. Among patients with more than 79 MB following Matalonga et al.’s (2020) classification (n = 221, 10.5%), mean NROH = 20.5 ± 9.7, SROH = 213.2 ± 127.1 MB, and FROH = 7.4% ± 4.4%. Therefore, our sample seems to have a minority of individuals who likely have consanguineous parents, and they present remarkable variation in their ROH metrics.
3.2 Disease-gene classification and clinical characteristics
We preselected a total of 129 genes of known association with NSHL; three of them were initially excluded from our evaluation because one of them (DFNX3) refers to a locus without a known gene, another (KCNJ10) did not contain a valid transcript in the MANE or RefSeq databases, and the other (ATP2B2) was not associated with a Mendelian form of HL (classified as a modifier by OMIM). We also eliminated four genes (FOXI1, GJB3, KCNJ10, and TSPEAR) with gene-disease associations classified as disputed by the ClinGen team and another gene (MYO1A) classified as refuted. Additionally, we eliminated the autosomal recessive (AR) form of GJB6, classified as refuted by ClinGen, keeping only its autosomal dominant (AD) form. The complete list of the seven excluded genes, evidence, and other details are available in Supplementary Table S3.
The remaining 121 genes (including the AD form of GJB6) were considered to present at least a minimal level of gene-disease association with NSHL and were individually curated. Considering these 121 genes, 42 present exclusively AD inheritance, 65 exclusively AR, six X-linked (XL), and eight present both AD and AR inheritances.
Regarding the 42 genes presenting exclusively AD inheritance, 27 (64.3%) presented a ClinGen gene-disease curation, and the remaining genes (n = 15, 35.7%) were curated by our group. Overall, the gene-disease association was classified as definite for 26.2% (n = 11), strong for 4.8% (n = 2), moderate for 19% (n = 8), and limited for 50% (n = 21). The great majority of AD inheritance genes (n = 35, 83.3%) were associated with predominantly postlingual-onset HL, while seven genes (16.7%; CRYM, GREB1L, KITLG, PLS1, SIX1, SLC12A2, and WFS1) were associated with prelingual onset. Progressive involvement was observed for the majority of these genes (85.7%, n = 36); the remaining genes presented a predominantly stable (7.1%, n = 3) or variable progression (4.8%, n = 2); for one gene (2.4%; GREB1L), progression was not clear in the literature. High and middle-high frequencies were predominantly affected in 54.8% (n = 23), followed by flatter involvement for all frequencies in 28.6% (n = 12), and for 16.7% (n = 7), the involvement of low, low-middle, or middle frequencies was more predominant.
For the 65 genes with exclusively AR inheritance, the great majority (55, 84.6%) presented a ClinGen gene-disease curation, and the remaining (n = 10, 15.4%) were curated by our group. Gene-disease association was classified as definite for 41.5% (n = 27), strong for 7.7% (n = 5), moderate for 15.4% (n = 10), and limited for 35.4% (n = 23). In contrast to AD forms, the majority of genes associated with AR HL (84.6%, n = 55) were associated with the predominant prelingual onset and 15.4% (n = 10) with postlingual onset. A stable hearing impairment was observed for the majority of these genes (58.5%, n = 38); the remaining presented a predominantly progressive (29.2%, n = 19) or variable progression (1.5%, n = 1; CDC14A); for seven genes (10.8%; ESRP1, GAB1, GRAP, MET, SPNS2, TMEM132E, and WHRN), the progression was not clear in the literature. A flatter pattern involving all frequencies was predominant in 56.9% (n = 37), while predominant high or middle-high frequencies were observed in 18.5% (n = 12) and middle frequencies in 1.5% (n = 1; GRXCR1), while for 23% (n = 15), a predominant frequency was not clear in the literature. A minority of AR forms were associated with mild-moderate HL (6.1%, n = 4), and the majority involved more severe phenotypes to varying degrees between moderate and profound involvement (93.8%, n = 61).
A minority of six genes presented XL inheritance, four (66.7%) of which presented ClinGen gene-disease curation. Half of the X chromosome genes presented gene-disease curation classified as definite (AIFM1, POU3F4, and SMPX), and the other half had limited evidence (COL4A6, GPRASP2, and PRPS1). Two genes (33.3%; AIFM1 and SMPX) presented postlingual onset and the remaining prelingual onset. All genes were associated with progressive involvement, a flatter pattern involving all frequencies and generally moderate to profound manifestation.
The remaining eight genes (COL11A2, GJB2, MYO6, MYO7A, PTPRQ, TBC1D24, TECTA, and TMC1) were associated with both AD and AR inheritance. Except for MYO7A (AR) and PTPRQ (AD), ClinGen gene-disease curation was available for all remaining forms of all genes. For the TECTA gene, both AD and AR modes are associated with the prelingual onset; for all remaining genes, AD forms are associated with the postlingual onset and milder phenotypes, while AR forms are associated with the prelingual onset and more severe HL.
Table 1 shows a summary of the 121 genes associated with NSHL, inheritance pattern, predominant age of onset, and predominant frequencies. The complete list of genes studied in this work along with individual gene-disease classification and the resources used in this curation are available in Supplementary Table S1.
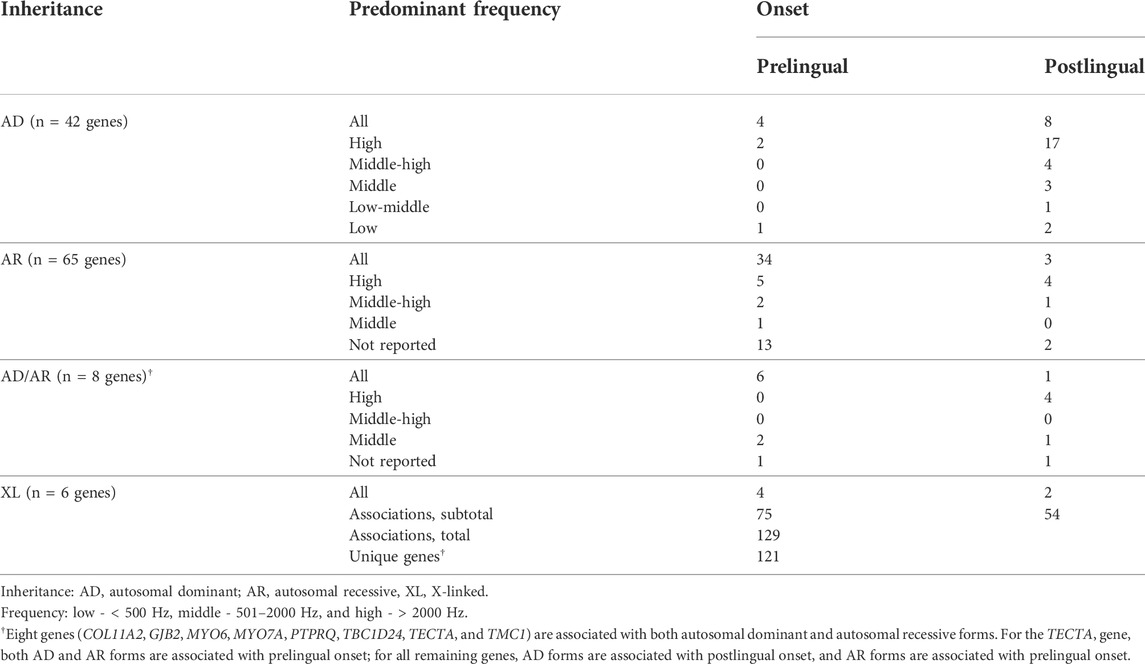
TABLE 1. Summary of genes involved in nonsyndromic hearing loss categorized by inheritance, predominant sound frequency impairment, and onset.
3.3 Sequence variants
Initially, a total of 5,330 variant coordinates dispersed throughout the 121 nuclear genes and 23 mitochondrial genes (5099 autosomal, 119 on the X chromosome, and 112 mitochondrial) were preselected according to the criteria mentioned previously. Following variant read depth quality control (QC), the genotypes from 5,087 autosomal, 104 on the X chromosome, and all 112 mitochondrial coordinates were retrieved. We did not detect any mitochondrial variants from the list of variants associated with HL.
Following the sample, variant, and call rate QC, 996 variants were detected in either of the two study groups (993 autosomal and three on the X chromosome). Among these variants, a total of 118 variants were reported in the ClinVar database and have been classified as pathogenic or likely pathogenic (P/LP) by at least one submitter. These variants were then individually curated and classified according to the ACMG criteria by our team of geneticists: 29 variants were excluded because they were reclassified as variants of unknown significance (n = 28) or likely benign (n = 1).
Finally, we retained a total of 89 clinically relevant sequence variants associated with nonsyndromic HL that were detected in the unaffected patients. They were distributed among 36 distinct genes; 44 variants were classified as pathogenic and 45 as likely pathogenic according to ACMG criteria. All variants were harbored by autosomal genes; no variants on the X chromosome were classified as P/LP. The complete list of variants, details on nomenclature, and respective frequencies are available in Supplementary Tables S4 and 5 (genotypes per variant) (genotypes per gene). The list of excluded variants along with all molecular data and criteria used for final classification is available in Supplementary Table S6.
We identified 222 heterozygotes (10.6%) and four homozygotes (0.2%) for these 89 P/LP variants in the group of 2,097 patients without HL. The top six most frequent variants in heterozygosity and the absolute number of heterozygous and allelic frequencies (AF) are as follows:
1) GJB2(NM_004004.6): c.35del; p. (Gly12fs); n = 28 (AF = 0.72%)
2) OTOA(NM_144672.4): c.2359G>T; p. (Glu787Ter); n = 25 (AF = 0.61%)
3) GJB2(NM_004004.6): c.109G>A; p. (Val37Ile); n = 23 (AF = 0.55%)
4) TMPRSS3(NM_001256317.3): c.1273G>A; p. (Ala425Thr); n = 13 (AF = 0.31%)
5) MPZL2(NM_005797.4): c.72del; p. (Ile24fs); n = 8 (AF = 0.19%)
6) GJB2(NM_004004.6): c.617A>G; p. (Asn206Ser); n = 6 (AF = 0.14%)
Among the heterozygotes, the majority (n = 123, 55.4%) were carriers for P/LP variants in genes associated exclusively with AR inheritance; 89 (40.1%) presented variants harbored by genes associated with both AD and AR forms. The remaining 10 (4.5%) harbored monoallelic P/LP variants for five genes associated exclusively with AD forms of HL (genes GJB6, KCNQ4, POU4F3, TJP2, and WFS1).
Regarding the four homozygotes, each had a different variant in homozygosity: 1) GJB2(NM_004004.6): c.35del; p. (Gly12fs) (AF = 0.72%); 2) DCDC2(NM_016356.5): c.383C>G; p. (Ser128Ter) (AF = 0.07%); 3) TBC1D24(NM_001199107.2): c.724C>T; p. (Arg242Cys) (AF = 0.07%); and 4) TJP2(NM_004817.4): c.1234C>T; p. (Arg412Ter) (AF = 0.05%). The TJP2 gene is exclusively associated with autosomal dominant inheritance, DCDC2 exclusively with autosomal recessive inheritance, and the remaining two with both forms of inheritance.
The combined frequencies for all the different P/LP variants for the top ten most frequent genes are shown in Table 2.
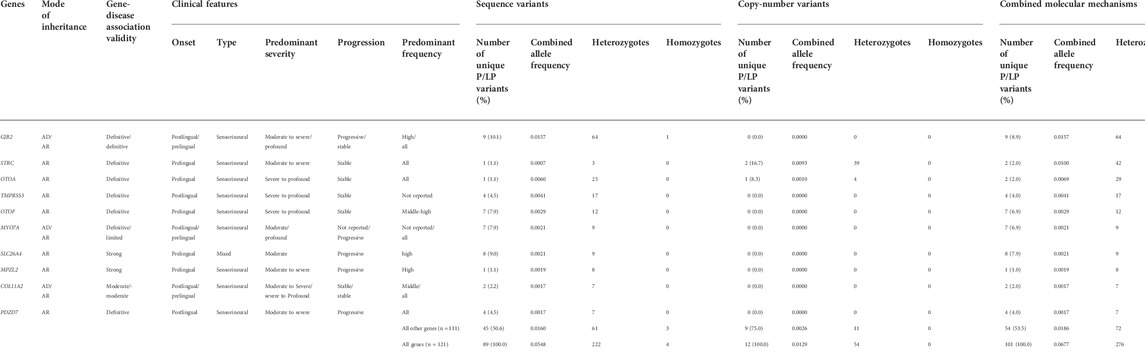
TABLE 2. Summarized clinical and molecular data regarding pathogenic and likely pathogenic (P/LP) variants for the 10 genes with the highest heterozygous genotype frequency. Three genes are associated with both autosomal dominant and autosomal recessive (AD/AR) forms of inheritance, and clinical features regarding both inheritance mechanisms are separated by “/” if they differ; the number of unique variants, combined allele frequencies for P/LP variants, number of heterozygotes, and number of homozygotes are shown for sequence variants, copy-number variants (CNVs), and combined molecular mechanisms (sequence variants plus CNVs). Additionally, see Supplementary Tables S4 and S7 for details of the variants.
Here, thirteen of the 89 P/LP variants detected in the no HL group were also detected in the Brazilian ABRaOM study. We compared the distribution of allele counts between their cohort and ours using Fisher’s exact test. After correction for multiple comparisons, the differences in allelic counts were not statistically significant for 12 of them. Only OTOA (NM_144,672.4): c.2359G>T; p. (Glu787Ter) appeared to be less frequent in our sample when compared to that observed by ABraOM (FDR adjusted Fisher’s exact test p-value column in Supplementary Table S4).
3.4 Copy-number variants
A preselection of 5,285 CNV events affecting 1,757 independent gene loci was detected among 1,259 patients (51 with HL, 1,208 without HL). This initial group of CNVs underwent a filtering process to eliminate all events harbored by patients with HL and all CNVs restricted to noncoding regions, after which 213 CNVs and 19 structural variants (small CNVs) remained. All 232 alterations underwent curation, visual inspection in BAM files, and classification according to the joint recommendation of ACMG and ClinGen (Riggs et al., 2020).
Finally, a total of 12 different heterozygous CNVs in 54 individuals were considered clinically relevant: four were classified as pathogenic and eight were classified as likely pathogenic (Supplementary Table S7). The most recurrent pathogenic CNV observed in our cohort and present in heterozygosity in 38 individuals (AF = 0.91%) was a microdeletion of approximately 57.9 kb (coordinates: chr15:43600849_43658715) involving exons 1 through 26 (out of a total of 29 exons) of the STRC gene, associated with the AR form of NSHL, along with the CATSPER2 gene (not associated with any Mendelian disorder in the OMIM database; however, biallelic deletions concomitantly involving STRC and CATSPER2 are associated with HL and male infertility, OMIM:611102). Another individual had a nonrecurring microdeletion also involving STRC (46.6 Kb; exons 22 through 29 and the PPIP5K1 gene; chr15:43557296_43603898; n = 1; AF = 0.024%).
The second-most recurrent pathogenic CNV was a microdeletion of approximately 244.2 Kb present in heterozygosity in four individuals (AF = 0.095%) involving the OTOA gene (associated with the AR form of NSHL) and the surrounding METTL9 gene (not associated with any Mendelian disorder in OMIM). The other CNVs identified all in heterozygosity in our work are as follows:
1) Pathogenic microdeletion of 805.5 Kb (chr16:15394361_16199828) involving the ABCC1 gene (AD form of NSHL): n = 2, AF = 0.048%;
2) Likely pathogenic microdeletion of 10.4 Kb (chr11:78476555_78486991) involving exons 7 through 10 of the NARS2 gene (AR form of NSHL): n = 2; AF = 0.048%;
3) Pathogenic microdeletion of 400.5 Kb (chr17:18624545_19025057) involving the GRAP gene (AR form of NSHL): n = 1; AF = 0.024%;
4) Likely pathogenic microdeletion of 19.6 Kb (chr15:51437517_51457141) involving exons 36 through 43 of the DMXL2 gene (AD form of NSHL): n = 1; AF = 0.024%;
5) Likely pathogenic microdeletion of 17.7 Kb (chr7:24702446_24720157) involving exons 3 through 8 of the GSDME gene (AD form of NSHL): n = 1; AF = 0.024%;
6) Likely pathogenic microdeletion of 10 kb (chr11:17603104_17613104) involving exons 33 through 38 of the OTOG gene (AR form of NSHL): n = 1; AF = 0.024%;
7) Likely pathogenic microduplication of 10.9 Kb (chr12:80288802_80299740) involving exon 27 of the OTOGL gene (AR form of NSHL): n = 1; AF = 0.024%;
8) Likely pathogenic microdeletion of 11.8 Kb (chr22:37719702_37731543) involving exon 5 of the TRIOBP gene (AR form of NSHL): n = 1; AF = 0.024%;
9) Intragenic likely pathogenic microdeletion of 11.6 Kb (chr11:121163035_121174624) involving exons 16 through 19 of the TECTA gene (AR/AD form of NSHL): n = 1; AF = 0.024%.
CNVs excluded for being of unknown significance or syndromic are shown in Supplementary Table S8.
3.5 Recessive HL frequency estimation
We used the Hardy–Weinberg equation to roughly estimate autosomal recessive HL frequency in our population (q2) based on the respective carrier frequencies (2pq) for all P/LP sequence variants and CNVs and the approximation of p∼1. Only genes associated with AR or both AD/AR forms were included in this estimate. According to this method, we estimated the following top five frequencies of autosomal recessive NSHL per gene per 1,000 people: GJB2: 0.25; STRC: 0.1; OTOA: 0.05; TMPRSS3: 0.02, and OTOF: 0.01. The combined frequency of these five recessive forms of NSHL is estimated to be 0.42 per 1,000 people, while the combined frequency considering all recessive forms of NSHL is 0.45/1,000 or 1:2,222. Supplementary Table S9 shows the estimate for each pathogenic/likely pathogenic variant included in this specific analysis.
4 Discussion
Herein, we studied molecular alterations (including sequence variants, copy-number variants, and mitochondrial DNA variants) associated with NSHL using data from genome sequencing of a cohort of 2,097 unaffected patients. We identified 276 heterozygotes (13.16%) and four homozygotes (0.2%) for P/LP variants harbored by genes associated with NSHL. Among the heterozygotes, 222 individuals (10.59%) were heterozygotes for 89 P/LP sequence variants and 54 (2.58%) were heterozygotes for 13 P/LP copy-number variants. All four homozygotes harbored P/LP sequence variants.
An important fraction of our cohort (n = 104, 4.96%) of unaffected individuals presented P/LP variants in genes associated with either exclusively AD or both AR/AD forms of NSHL. This finding may imply that at least a fraction of these individuals present mild hearing impairment or may develop it in the future, especially if we consider that 1) AD genes manifest primarily as postlingual onset (some forms in later decades of life), have progressive involvement, and predominantly affect high and middle-high frequencies and 2) the AD form of AR/AD genes is also associated with postlingual onset and milder phenotypes (Table 1). Considering that epidemiological studies have shown that the overall prevalence of HL in adults ranges from 4–15% worldwide (Hoffman et al., 2017; Sheffield and Smith, 2019), our data indicate that an important fraction of this condition may be associated with monogenic origin and dominant inheritance.
Among these 104 heterozygotes for dominant alleles for NSHL, the majority (61.5%, n = 64; 3.05% of the entire cohort) harbored monoallelic pathogenic variants in the GJB2 gene. This gene is associated with both AR and AD forms of NSHL. Although a full penetrance and progressive course have been described for both forms of this gene, the clinical impact may be heterogeneous, varying from mild to severe and primarily affecting the perception of high frequencies (Smith and Ranum, 1993). The combined allele frequency of 1.41% for the top three most frequent variants in GJB2 (c.35del, p.Val37Ile, and p.Asn206Ser) observed in our study (0.72, 0.55, and 0.14%, respectively) is close to the 1.05% observed in gnomAD (0.63, 0.4, and 0.02%) and the 1.49% in ABraOM (0.98, 0.34, and 0.17%). This gene is followed in the number of heterozygotes by MYO7A (n = 9; 0.43% of the cohort) and COL11A2 (n = 7; 0.33% of the cohort), both associated with AR/AD forms of NSHL: AD forms of these genes are also described as having postlingual onset. A remarkable finding regarding dominant alleles is that five individuals (0.24%) harbored heterozygous P/LP copy-number variants involving the genes ABCC1 (n = 2), TECTA (n = 1), DMXL2 (n = 1), and GSDME (n = 1).
We also observed a relevant frequency of heterozygotes (n = 262, 12.5%) with recessive alleles for NSHL. This finding is important for genetic counseling, especially for consanguineous couples. AR inheritance represents a relevant fraction of genetic forms of HL and is generally associated with prelingual and more severe clinical involvement (Table 1). GJB2 was also the gene in which most subjects harbored variants for recessive forms; it is important to note that this gene is associated with both AD and AR inheritances. Biallelic pathogenic variants in GJB2 are generally described in different populations as the most common genetic cause of sensorineural HL (Azaiez et al., 2004; Kenna et al., 2010). Following this gene is STRC, in which we detected two distinct forms of pathogenic CNVs within its coding region. The first form was recurrent and was present in 38 individuals. The second form was nonrecurrent and was found in a single patient. Alongside the CNVs, we detected three P/LP sequence variants, totaling 42 heterozygotes for variants in STRC. This gene has also been described as the second most common form of autosomal recessive HL in European populations (Del Castillo et al., 2022).
We used the Hardy–Weinberg equation to estimate the number of affected individuals (homozygotes or compound heterozygotes) with AR forms of HL based on the combined allele frequencies per gene observed in this study and estimated the population frequency of AR NSHR to be 1:2,222 (or 0.45/1,000). This approach presents limitations in certain populations because the frequency of carriers may vary widely in different groups due to founder effects, endogamy, nonrandom mating, and cultural, religious, social, and/or geographical isolation (Antonarakis, 2019).
Fareed et al. (2021) demonstrated how consanguinity influences NSHL occurrence. They found the rare pathogenic OTOF (NM_194,248.3): c.2122C>T; p. (Arg708Ter) (rs80356590) in a homozygous state among some individuals from an Indian sample with a consanguineous background. In our cohort, it occurred only once in a male heterozygous individual. His medical records state that he does not have consanguineous parents and we experimentally confirmed this: the sum length of his runs of homozygosity larger than 1 MB (SROH ≥1.0 MB) is approximately 7.0 MB. Matalonga et al., 2020 proposed a minimum of SROH ≥79 MB as evidence of probable consanguinity. In contrast to the sample examined by Fareed et al. (2021), ours was not specifically focused on consanguineous individuals. As such, they constitute a minority (but a relevant portion) of our study (approximately 9.0% or 10.0% depending on the form of assessment).
Recessive forms of NSHL represent an important fraction of congenital deafness, which can be detected by neonatal screening. However, there are other frequent genetic etiologies (such as syndromic forms of HL, de novo or inherited AD or XL, and de novo or inherited mitochondrial variants) and nongenetic etiologies (congenital infections such as rubella and syphilis; jaundice; and anoxia). Considering this complex etiology, our estimation of 1:2,222 may represent a fraction of congenital HL.
The incidence of congenital HL in Brazil is estimated at 4:1,000 births, with 20% (0.8:1,000 or 1:1,250) of cases having a genetic etiology (Schüffner et al., 2020). Interestingly, our estimate (1:2,222) is not very far from the epidemiological study.
We did not find any P/LP variants in the X chromosome or mitochondrial DNA. We believe that the primary reason for this is that both XL and mitochondrial inheritance are indeed uncommon causes of NSHL (Shearer and Smith, 2015), accounting for approximately 2–5% and 1% of all cases, respectively, and we would expect very low frequencies or even the absence of these variants in a cohort of unaffected individuals, such as ours. However, many HL studies do not even investigate the X chromosome or the mitochondrial genome, and both likely harbor variants yet to be found.
All 2,097 patients analyzed for statistics and population assumptions in this study were not affected with HL, as we eliminated all 89 patients with HL phenotypes, but they are not true “controls,” since they comprise a convenience sample referred by participating research centers from Brazil to undergo genome sequencing because they presented clinical manifestations (other than hearing impairment) for rare diseases suspected of genetic etiology. This fact may explain the higher consanguinity frequency in our cohort. Although we acknowledge that the ideal group of patients for the purpose of our study and for more precise population assumptions would comprise asymptomatic adult individuals, we compared the frequencies of variants found in this study to the frequencies found in the ABraOM repository, which contains genomic variants from elderly individuals from Brazil, and did not find relevant differences for the great majority of variants co-occurring in both databases (Supplementary Table S4). This may suggest that despite our sample being enriched with individuals with rare diseases, the frequencies for HL-associated pathogenic variants in our sample of individuals without HL may be representative of the frequency in the general Brazilian population as well.
The approach of using groups of symptomatic individuals with heterogeneous conditions as controls has been widely used before, such as in several studies that used molecular data from the United States National Institutes of Health (NIH) Heart, Lung and Blood Institute (NHLBI)-sponsored Exome Sequencing Project, composed primarily of symptomatic individuals, as control groups (Fu et al., 2013). In this study, we used the same strategy of examining datasets of people with diverse symptomatic conditions and without HL as a valid approach for use as controls for HL.
Gene selection for common genetic conditions that multiple genes are capable of producing the same phenotype, such as NSHL, may be a challenging task. Our first step in preselecting genes associated with a diverse group of genetic disorders was very inclusive and comprised several genes with limited evidence of gene-disease association, most of which presented few clinical reports and/or limited functional studies, and some genes with contradictory evidence of the association with HL. As we did not find a previously published systematic approach for gene-disease classification for NSHL, we applied an existing framework proposed by ClinGen and observed that half (21/42) of the genes were associated with AD NSHL, and more than one-third (23/65) of AR genes, half (3/6) of X-linked genes, and one-fourth (4/16) of all forms of the eight AR/AD genes exhibited limited gene-disease association. A limited gene-disease validity does not necessarily delegitimize studies with this group of genes, but it implies that the literature remains limited either in the number of individuals described or experimental data (Strande et al., 2017). Therefore, these results and conclusions regarding genes with limited association with diseases must be interpreted with caution.
An accurate clinical validity of gene-disease association is likely the first important step in understanding the genomics of NSHL. The second step is understanding the clinical impact of the variants harbored by these genes. Therefore, we carefully reviewed the available evidence and eliminated genes that were only weakly associated with NSHL: some genes were removed from the analysis either because their association with NSHL was classified as disputed or refuted (such as the AR NSHL-associated variants of GJB6). Among the disputed genes is GJB3. One relevant variant in GJB3 (NM_024009.3: c.196_198del; p.Asp66del) was quite frequent, with six heterozygotes (AF = 0.0014), but was eliminated from our analysis because we considered this gene not to exhibit a minimal level of gene-disease association.
Another challenging task in this study was selecting relevant variants associated with HL because, on the one hand, exaggeratedly inclusive approaches may overestimate frequencies, especially when including variants by presumptively assuming a deleterious mechanism without proper functional studies or relevant reports by other researchers. On the other hand, restricted inclusions may underestimate frequencies, especially for hypomorphic alleles (such as missense variants) that do not result in clear loss of function or variants not previously reported in the literature or genomic databases.
Both the aforementioned approaches represent relevant biases and may influence correct population assumptions, especially for rare molecular mechanisms and genes with limited evidence for gene-disease association. As a means to balance this conflict, we chose an approach that restricted the selection of variants for those already observed and classified as P/LP by at least one participant in the ClinVar database, which is the largest and most used public archive of reports of the relationships among human variations and phenotypes from participating centers from the whole world (Landrum et al., 2018). These preselected variants were then curated by our team of researchers and strictly reclassified following the ACMG criteria for variant classification. The direct consequence of this approach, though, is that we have lost all variants not present in the ClinVar database.
In our previous study, we used exome sequencing to search for the carrier status for AR conditions, including AR forms of HL, in a cohort of 320 patients (Quaio et al., 2021). We found a total of 27 occurrences of P/LP variants associated with either syndromic or NSHL. Among these variants, five (19.5%) were not found in the ClinVar database: two were harbored by genes with definite association with NSHL (DIAPH1 and GIPC3), one was harbored by a gene with strong association (MYO3A), one had limited association (TBC1D24), and another one was associated with a syndromic form of HL (PHYH). This suggests that we may have lost approximately 15% of potentially relevant variants harbored by genes associated with HL in the current study, as we restricted the scope of variants solely to those present in ClinVar.
Large-scale genomic studies of NSHL in non-North American and non-European populations are limited in the literature. We did not find comprehensive studies in Latin America for robust comparisons to our results. We hope that our pioneer study stimulates other groups in future attempts to better understand the molecular events associated with NSHL, especially those from countries with underrepresented populations in genomic studies.
In conclusion, we collected whole-genome sequencing data from a cohort of 2,097 Brazilian individuals unaffected by HL to investigate the molecular findings associated with NSHL and determined relevant frequencies of molecular alterations (sequence variants and CNVs) associated with this group of conditions and estimated a combined frequency of all recessive forms of NSHL of one case per 2,222 individuals. This approach demonstrates the potential for determining clinical and social burdens at the population level and addresses health policies, confirming the clinical utility of obtaining genomic data for the investigation and management of hearing impairment.
Data availability statement
The original contributions presented in the study are included in the article/Supplementary Material, further inquiries can be directed to the corresponding authors.
Ethics statement
The studies involving human participants were reviewed and approved by Hospital Israelita Albert Einstein. Written informed consent to participate in this study was provided by the participants’ legal guardian/next of kin.
Author contributions
CQ: conceptualization, investigation, formal analysis, writing—original draft, review, and editing. AC: data curation, software, formal analysis, writing—original draft, review, and editing. LM: software, data curation, and writing—review and editing. RG: software and data curation. KC: conceptualization, investigation, and writing—review and editing. JC: investigation and writing—review and editing. RM: investigation, supervision, and writing—review and editing. MC: software and data curation. RD: software and data curation. BA: resources and methodology. MN: resources and methodology. AT: investigation and writing—review and editing. ML: resources and methodology. TM: resources and methodology. MM: investigation and writing—review and editing. GC: resources and methodology. AR: resources and methodology. AF: resources and methodology. GC: resources and methodology. EP: investigation and writing—review and editing. LV: investigation and writing—review and editing. CM: investigation and writing—review and editing. JP: investigation and writing—review and editing. MD: investigation and writing—review and editing. MC: supervision, project administration, and funding acquisition. TA: conceptualization, project administration, investigation, formal analysis, and writing—review and editing. JO: supervision, project administration, and funding acquisition.
Funding
This study was funded by Hospital Israelita Albert Einstein in a public‒private partnership with the Programa de Apoio ao Desenvolvimento Institucional do Sistema Único de Saúde (PROADI-SUS) from the Brazilian Ministry of Health (Law 12.101/2009, protocol number 25000.083098/2019–71).
Conflict of interest
The following authors are employees (received salary and other bonuses) of Hospital Israelita Albert Einstein: CQ; AC; LM; RG; KC; JC; RM; MC; RD; BA; MN; AT; ML; TM; MM; GC; AR; AF; GC; EP; LV; CM; JP; MD; MC; TA; and JO.
Publisher’s note
All claims expressed in this article are solely those of the authors and do not necessarily represent those of their affiliated organizations, or those of the publisher, the editors, and the reviewers. Any product that may be evaluated in this article, or claim that may be made by its manufacturer, is not guaranteed or endorsed by the publisher.
Supplementary material
The Supplementary Material for this article can be found online at: https://www.frontiersin.org/articles/10.3389/fgene.2022.921324/full#supplementary-material
References
Antonarakis, S. E. (2019). Carrier screening for recessive disorders. Nat. Rev. Genet. 20 (9), 549–561. doi:10.1038/s41576-019-0134-2
Azaiez, H., Chamberlin, G. P., Fischer, S. M., Welp, C. L., Prasad, S. D., Taggart, R. T., et al. (2004). GJB2: The spectrum of deafness-causing allele variants and their phenotype. Hum. Mutat. 24 (4), 305–311. doi:10.1002/humu.20084
Campos Coelho, A. V., Cordeiro de Azevedo, B. M., Lucon, D. R., Nóbrega, M. S., de Souza Reis, R., de Alexandre, R. B., et al. (2021). The Brazilian rare genomes project: validation of whole genome sequencing for rare diseases diagnosis. medRxiv 2010, 21264436. doi:10.1101/2021.10.01.21264436
Del Castillo, I., Morín, M., Domínguez-Ruiz, M., and Moreno-Pelayo, M. A. (2022). Genetic etiology of non-syndromic hearing loss in Europe. Hum. Genet. 141 (3-4), 683–696. doi:10.1007/s00439-021-02425-6
Edwards, A. W. F., and Hardy, G. H. (2008). Hardy-Weinberg equilibrium. Genetics 179 (3), 1143–1150. doi:10.1534/genetics.104.92940
Fareed, M., Sharma, V., Singh, I., Rehman, S. U., Singh, G., and Afzal, M. (2021). Whole-exome sequencing reveals a rare variant of OTOF gene causing congenital non-syndromic hearing loss among large muslim families favoring consanguinity. Front. Genet. 12, 641925. doi:10.3389/fgene.2021.641925
Fu, W., O'Connor, T. D., Jun, G., Kang, H. M., Abecasis, G., Leal, S. M., et al. (2013). Analysis of 6, 515 exomes reveals the recent origin of most human protein-coding variants. Nature 493 (7431), 216–220. doi:10.1038/nature11690
Geoffroy, V., Guignard, T., Kress, A., Gaillard, J. B., Solli-Nowlan, T., Schalk, A., et al. (2021). AnnotSV and knotAnnotSV: a web server for human structural variations annotations, ranking and analysis. Nucleic Acids Res. 49 (W1), W21–W28. doi:10.1093/nar/gkab402
Geoffroy, V., Herenger, Y., Kress, A., Stoetzel, C., Piton, A., Dollfus, H., et al. (2018). AnnotSV: an integrated tool for structural variations annotation. Bioinformatics 34 (20), 3572–3574. doi:10.1093/bioinformatics/bty304
Girdea, M., Dumitriu, S., Fiume, M., Bowdin, S., Boycott, K. M., Chénier, S., et al. (2013). PhenoTips: patient phenotyping software for clinical and research use. Hum. Mutat. 34 (8), 1057–1065. doi:10.1002/humu.22347
Harris, P. A., Taylor, R., Minor, B. L., Elliott, V., Fernandez, M., O'Neal, L., et al. (2019). The REDCap consortium: building an international community of software platform partners. J. Biomed. Inf., 95, 103208. doi:10.1016/j.jbi.2019.103208
Harris, P. A., Taylor, R., Thielke, R., Payne, J., Gonzalez, N., and Conde, J. G. (2009). Research electronic data capture (REDCap)—a metadata-driven methodology and workflow process for providing translational research informatics support. J. Biomed. Inf., 42(2), 377–381. doi:10.1016/j.jbi.2008.08.010
Hoffman, H. J., Dobie, R. A., Losonczy, K. G., Themann, C. L., and Flamme, G. A. (2017). Declining prevalence of hearing loss in US adults aged 20 to 69 years. JAMA Otolaryngol. Head. Neck Surg. 143 (3), 274–285. doi:10.1001/jamaoto.2016.3527
Kenna, M. A., Feldman, H. A., Neault, M. W., Frangulov, A., Wu, B. L., Fligor, B., et al. (2010). Audiologic phenotype and progression in GJB2 (Connexin 26) hearing loss. Arch. Otolaryngol. Head. Neck Surg. 136 (1), 81–87. doi:10.1001/archoto.2009.202
Köhler, S., Gargano, M., Matentzoglu, N., Carmody, L. C., Lewis-Smith, D., Vasilevsky, N. A., et al. (2021). The human phenotype Ontology in 2021. Nucleic Acids Res. 49 (D1), D1207–d1217. doi:10.1093/nar/gkaa1043
Landrum, M. J., Lee, J. M., Benson, M., Brown, G. R., Chao, C., Chitipiralla, S., et al. (2018). ClinVar: improving access to variant interpretations and supporting evidence. Nucleic Acids Res. 46 (D1), D1062-D1067–D1067. doi:10.1093/nar/gkx1153
Lionel, A. C., Costain, G., Monfared, N., Walker, S., Reuter, M. S., Hosseini, S. M., et al. (2018). Improved diagnostic yield compared with targeted gene sequencing panels suggests a role for whole-genome sequencing as a first-tier genetic test. Genet. Med. 20 (4), 435–443. doi:10.1038/gim.2017.119
Lott, M. T., Leipzig, J. N., Derbeneva, O., Xie, H. M., Chalkia, D., Sarmady, M., et al. (2013). mtDNA variation and analysis using mitomap and mitomaster. Curr. Protoc. Bioinforma. 44, 1–2126. doi:10.1002/0471250953.bi0123s44
Matalonga, L., Laurie, S., Papakonstantinou, A., Piscia, D., Mereu, E., Bullich, G., et al. (2020). Connect genome-phenome analysis platform and URD-cat data ContributorsImproved diagnosis of rare disease patients through systematic detection of runs of homozygosity. J. Mol. Diagn. 22 (9), 1205–1215. doi:10.1016/j.jmoldx.2020.06.008
Naslavsky, M. S., Yamamoto, G. L., de Almeida, T. F., Ezquina, S. A. M., Sunaga, D. Y., Pho, N., et al. (2017). Exomic variants of an elderly cohort of brazilians in the aBraOM database. Hum. Mutat. 38 (7), 751–763. doi:10.1002/humu.23220
Online Mendelian Inheritance in Man OMIM® (2021). McKusick-nathans Institute of genetic medicine. Baltimore, MD): Johns Hopkins University. {November, 2021}. World Wide Web URL: https://omim.org/.
Quaio, C., Chung, C. H., Perazzio, S. F., Dutra, A. P., Moreira, C. M., Filho, G. M. N., et al. (2021). Frequency of carriers for rare recessive mendelian diseases in a brazilian cohort of 320 patients. Am. J. Med. Genet. C Semin. Med. Genet. 187, 364–372. n/a. doi:10.1002/ajmg.c.31932
Richards, S., Aziz, N., Bale, S., Bick, D., Das, S., Gastier-Foster, J., et al. (2015). Standards and guidelines for the interpretation of sequence variants: a joint consensus recommendation of the american college of medical genetics and genomics and the association for molecular pathology. Genet. Med. 17 (5), 405–424. doi:10.1038/gim.2015.30
Riggs, E. R., Andersen, E. F., Cherry, A. M., Kantarci, S., Kearney, H., Patel, A., et al. (2020). Technical standards for the interpretation and reporting of constitutional copy-number variants: A joint consensus recommendation of the american college of medical genetics and genomics (ACMG) and the clinical genome resource (ClinGen). Genet. Med. 22 (2), 245–257. doi:10.1038/s41436-019-0686-8
Robinson, J. T., Thorvaldsdottir, H., Winckler, W., Guttman, M., Lander, E. S., Getz, G., et al. (2011). Integrative genomics viewer. Nat. Biotechnol. 29 (1), 24–26. doi:10.1038/nbt.1754
Schüffner, R., Nascimento, K. L., Dias, F. A., Silva, P., Pires, W., Cipriano, N. M., et al. (2020). Molecular study of hearing loss in minas Gerais, Brazil. Braz. J. Otorhinolaryngol. 86 (3), 327–331. doi:10.1016/j.bjorl.2018.12.005
Shearer, A. E., Hildebrand, M. S., and Smith, R. J. (2017). Hereditary hearing loss and deafness overview. GeneReviews®[Internet]. Seattle, WA: University of Washington, Seattle.
Shearer, A. E., and Smith, R. J. (2015). Massively parallel sequencing for genetic diagnosis of hearing loss: the new standard of care. Otolaryngol. Head. Neck Surg. 153 (2), 175–182. doi:10.1177/0194599815591156
Sheffield, A. M., and Smith, R. (2019). The epidemiology of deafness. Cold Spring Harb. Perspect. Med. 9 (9), a033258. doi:10.1101/cshperspect.a033258
Smith, R. J. H., and Ranum, P. T. (1993). Nonsyndromic hearing loss and deafness, DFNA3. Editors M. P. Adam, H. H. Ardinger, R. A. Pagon, S. E. Wallace, L. J. H. Bean, G. Mirzaaet al. GeneReviews((R))https://www.ncbi.nlm.nih.gov/pubmed/20301708.
Strande, N. T., Riggs, E. R., Buchanan, A. H., Ceyhan-Birsoy, O., DiStefano, M., Dwight, S. S., et al. (2017). Evaluating the clinical validity of gene-disease associations: an evidence-based framework developed by the clinical genome resource. Am. J. Hum. Genet. 100 (6), 895–906. doi:10.1016/j.ajhg.2017.04.015
Van Camp, G., and Smith, R. J. H. (2021). Hereditary hearing loss homepage. Retrieved from: https://hereditaryhearingloss.org.
Keywords: nonsyndromic hearing loss, hearing loss, deafness, genomics, whole genome sequencing, GJB2 (C×26) gene mutations, STRC gene
Citation: Quaio CRDC, Coelho AVC, Moura LMS, Guedes RLM, Chen K, Ceroni JRM, Minillo RM, Caraciolo MP, Reis RS, Mascaro BC, Nobrega MS, Teixeira ACB, Martinelli ML, Mota TR, Matta MC, ColichioGBC, Roncalho AL, Ferreira AFM, Campilongo GP, Perrone E, Virmond LA, Moreno CA, Prota JRM, França M, Cervato MC, Almeida TF and Oliveira JB (2022) Genomic study of nonsyndromic hearing loss in unaffected individuals: Frequency of pathogenic and likely pathogenic variants in a Brazilian cohort of 2,097 genomes. Front. Genet. 13:921324. doi: 10.3389/fgene.2022.921324
Received: 15 April 2022; Accepted: 28 July 2022;
Published: 30 August 2022.
Edited by:
Matthew E. R. Butchbach, Nemours Children’s Health Delaware, United StatesReviewed by:
Terry-Lynn Young, Memorial University of Newfoundland, CanadaPu Dai, PLA General Hospital, China
Mohd Fareed, Indian Institute of Integrative Medicine (CSIR), India
Copyright © 2022 Quaio, Coelho, Moura, Guedes, Chen, Ceroni, Minillo, Caraciolo, Reis, Mascaro, Nobrega, Teixeira, Martinelli, Mota, Matta, Colichio, Roncalho, Ferreira, Campilongo, Perrone, Virmond, Moreno, Prota, França, Cervato, Almeida and Oliveira. This is an open-access article distributed under the terms of the Creative Commons Attribution License (CC BY). The use, distribution or reproduction in other forums is permitted, provided the original author(s) and the copyright owner(s) are credited and that the original publication in this journal is cited, in accordance with accepted academic practice. No use, distribution or reproduction is permitted which does not comply with these terms.
*Correspondence: Caio Robledo D’ Angioli Costa Quaio, Y2Fpby5xdWFpb0BlaW5zdGVpbi5icg==; Joao Bosco de Oliveira Filho, am9hby5ib3Njb0BlaW5zdGVpbi5icg==