- 1Key Laboratory of Gastrointestinal Cancer (Fujian Medical University), Ministry of Education, School of Basic Medical Sciences, Fujian Medical University, Fuzhou, China
- 2Fujian Key Laboratory of Tumor Microbiology, Department of Medical Microbiology, Fujian Medical University, Fuzhou, China
As a critical layer of epigenetics, RNA modifications demonstrate various molecular functions and participate in numerous biological processes. RNA modifications have been shown to be essential for embryogenesis and stem cell fate. As high-throughput sequencing and antibody technologies advanced by leaps and bounds, the association of RNA modifications with multiple human diseases sparked research enthusiasm; in addition, aberrant RNA modification leads to tumor angiogenesis by regulating angiogenesis-related factors. This review collected recent cutting-edge studies focused on RNA modifications (N6-methyladenosine (m6A), N5-methylcytosine (m5C), N7-methylguanosine (m7G), N1-methyladenosine (m1A), and pseudopuridine (Ψ)), and their related regulators in tumor angiogenesis to emphasize the role and impact of RNA modifications.
Introduction
The epitranscriptome has been thought of as an important layer for the regulation of gene expression, which was expected to become a key target in the treatment of cancers (Dong and Cui, 2020). Also, currently, over 180 different types of chemical modifications on ribonucleic acid (Boccaletto et al., 2022) have been discovered in all three life domains and viruses (Krug et al., 1976; Canaani et al., 1979; Deng et al., 2015) that widespread exist on several types of RNA, including small nucleolar RNA (snoRNA), noncoding RNA (ncRNA), ribosomal RNA (rRNA), transfer RNA (tRNA), and messenger RNA (mRNA). According to the incidence of cancer rising in the past few decades, the associations between RNA modifications with tumor development were widely studied to try find a new therapeutic target (Zhao et al., 2017a). However, the quantitative methods to detect RNA epigenetics with highly accuracy and sensitivity are still under development, and the function and mechanism of these chemical modifications on different types of RNAs is under exploration.
In recent decades, microRNAs (miRNAs) have been considered one of the factors of angiogenesis, affecting the processes associated with neovascularization that include angiogenesis and arteriogenesis (Weber, 2013; Welten et al., 2016). In the angiogenesis, the new capillaries will growth from the existing vasculature and postcapillary venules and then redistribute local blood flow toward ischemic areas, which contributes to relieving ischemia (van der Kwast et al., 2019). Under physiological conditions, angiogenesis is strictly regulated by various cytokines and is crucial to embryonic development, trauma repair, reproduction, and menstrual cycles (Ma et al., 2020), while pathological angiogenesis is a continuously uncontrolled process that leads to various diseases (Jeong et al., 2021) (Figure 1). Under hypoxia, vascular endothelial growth factor (VEGF) is a mitogen that directly stimulates endothelial cells (ECs) division and proliferation, thereby resulting in new capillaries. Apart from ECs, vascular smooth muscle cells, fibroblasts, and immune cells also play vital parts by affecting and regulating ECs function as well as secreting angiogenic factors (Schwartz and Mitchell, 1962; Noonan et al., 2008; Newman et al., 2011). Furthermore, direct angiogenic factors are highly correlated and are jointly involved in the formation of vascular networks in tumors (Viallard and Larrivée, 2017). In addition, angiogenesis-related factors interact and influence each other, for example, VEGF stimulates ECs to secrete fibroblast growth factor (FGF), which in turn activates the VEGF system for angiogenesis (Hosaka et al., 2020). The most widely studied VEGF family, especially VEGFA, is a key angiogenic factor regulating ischemic diseases. VEGFA expression was influenced by histone methylation, ncRNA, and other epigenetic regulatory networks. Lack of VEGF leads to embryonic body death, while overexpression of VEGF promotes tumor angiogenesis (Melincovici et al., 2018). VEGF was overexpressed in liver tissue dysplasia and premalignant cirrhosis stage, suggesting that VEGF is bound up with the grade of hepatocellular carcinoma (HCC) (Hamdy et al., 2020). In renal cancer tissues of different clinical stages, aberrant expression of VEGF may be correlated with the deterioration of disease (Hirsch et al., 2020). Besides, overexpression of VEGF is associated with clinicopathological stage in patients and is expected to be a major factor in the prediction of gastric cancer recurrence (Pang et al., 2018).
Because of the dynamics of RNA modifications, cells respond to external signals accurately and quickly. The ability to adapt to microenvironmental changes, such as stress and hypoxia, is a factor for tumor cell survival, which indicates that RNA modifications may play significant part in tumors. Angiogenesis is a major feature of tumors and mainly formed by genetic mutation or epigenetic alterations (Hanahan and Weinberg, 2011). Researchers showed that RNA modifications also participate in certain pathological processes, affecting gene transcription and chromatin integrity and even regulating signaling pathways of the cell cycle, proliferation, differentiation, apoptosis, and tumorigenesis (Nombela et al., 2021). Of note, substantial evidence indicates that abnormal expression of RNA modification-related regulators comprises of methyltransferases, demethylases, and binding proteins (Pan et al., 2018), which are associated with angiogenesis and tumorigenesis (Blanco et al., 2014a; Barbieri and Kouzarides, 2020a). Other RNA modifications participated in multiple biological processes, which have been proven in recent studies. For instance, 2ʹ-O methylation, which is hypermodified in rRNA, reducing efficient translation and affecting growth and sensitivity to antibiotics (Liang et al., 2009). m5C is commonly found in tRNA and rRNA and is associated with protein translation (Blanco et al., 2014a; Schosserer et al., 2015; Janin et al., 2019a). Next, pseudoreuridine, in yeast mRNA expression, increases under heat shock and starvation conditions (Carlile et al., 2014). m1A is primarily enriched within 5ʹ UTR, and located upstream vicinity of start codons contributes to the structural stability of RNA, which is associated with increased translation and induction of stress (Dominissini et al., 2016a).
Considering that angiogenesis is an important physiological and pathological process, RNA modification is the research front in epigenetics. Thus, in this review, we systematically summarized the RNA modification-associated angiogenesis pathways, including m6A, m5C, m7G, m1A, and Ψ (Figure 2).
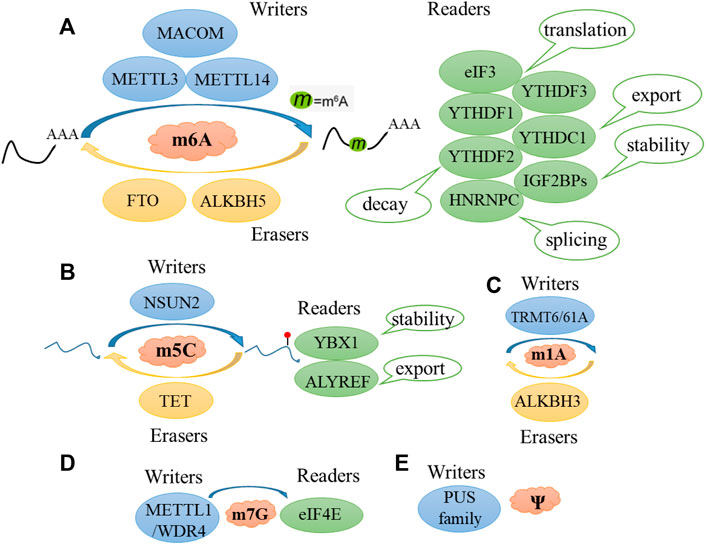
FIGURE 2. Major classes of RNA modification. Schematic diagram of m6A (A), m5C (B), m1A (C), m7G (D), Ψ (E), and regulated by methyltransferase (writers), demethylases (erasers), and some specific proteins (readers).
RNA Modification Type
RNA m6A Methylation
In 1974, N6-methyladenosine (m6A) was identified in human mRNA for the first time (Desrosiers et al., 1974). Also, previously, some methods have been developed to detect m6A modification, including dot blots (Wang et al., 2018), electrochemical immunosensor method (Yin et al., 2017), RNA photo-crosslinkers, and quantitative proteomics (Arguello et al., 2017); however, they cannot determine the precise location of m6A. The LC/MS analysis suggested that m6A is the most prevalent mRNA modification in mammals. With the advent of MeRIP-Seq (Meyer et al., 2012)/m6A-seq (Dominissini et al., 2012), the m6A conserved motif RRACH was discovered, where the most classical motif is GGACU (R = Guanine, Adenine, and H = Adenine, Cytosine, Uracil); meanwhile, miCLIP technology enabled correct identification of m6A residues at base-resolution (Linder et al., 2015). Further, at least 12 methods were developed to detect methylation based on the NGS platform (Wang and Jia, 2020). Right now, at least 178,049 human modification sites have been reported based on the high-resolution sequencing techniques (Tang et al., 2021a). Moreover, the evolutionary conservation of m6A was discussed among mammals (Dominissini et al., 2012), primates (Ma et al., 2017), and plants (Miao et al., 2021), reflecting the potential correlation between m6A richness with gene-specific structure and function (Song et al., 2021a).
RNA m6A modification is a reversible mark. The methyl group from S-adenosylmethionine can be added on the adenine by methyltransferase (writers), and the methyl group can be removed from the methylation sites by demethylases (erasers). Next, some specific proteins (readers) will recognize m6A that affects these regulatory mechanisms and exert important biological effects. Furthermore, m6A affects RNA metabolism in different stages, such as mRNA translation (Frye and Blanco, 2016), splicing (Liu et al., 2017), degradation (Shima et al., 2017), secondary structure (Kierzek et al., 2022), and stability (Wang et al., 2014). In general, m6A plays crucial roles of RNA processing in both the nucleus and cytoplasm (Zhao et al., 2017b).
m6A Writers
In eukaryotic cells, m6A methyltransferases are composed of three components: a main catalyze domain methyltransferase-like 3 (METTL3), a substrate recognition domain methyltransferase-like 14 (METTL14), and the m6A-METTL-associated complex (MACOM). METTL3 exhibits the S-adenosine methionine (SAM)-binding motif, and METTL14 demonstrates a methyltransferase domain also, which shares 22 percentage amino acids sequences with METTL3 (Śledź and Jinek, 2016). Next, MACOM comprises four components: RBM15, Zc3h13, WTAP, and VIRMA (He et al., 2019). RBM15 can recognize target mRNA and recruit the other components of the methyltransferase complex: WT1 associated protein (WTAP). WTAP not only acts as a splicing factor regulating the methylation and location of the heterodimer (Ping et al., 2014) but also regulates complex formation. Next, Zc3h13 recruits protein that can connect the other two adaptors, RBM15 and WTAP (Knuckles et al., 2018). In recent years, another active single methyltransferase, METTL16, was found to maintain SAM homeostasis, which can add a methyl group on the SAM synthase transcript, which contributes to the methylation of a small nucleolar (snoRNA) (Mendel et al., 2018).
m6A Erasers
RNA demethylases were not discovered until 2011. Jia and her colleagues (Jia et al., 2011a) proved the m6A is the substrate of fat mass and obesity-related proteins (FTO), and the FTO will induce the demethylation of m6A. FTO can oxidize m6A in an indirect way, which will generate two intermediates: hm6A and f6A (Jia et al., 2011b). ALKBH5 is another eraser, which is homolog of FTO (Zheng et al., 2013) and catalyzes the direct removal of m6A methylation, acting on mRNA preferentially (Zhou et al., 2020).
m6A Readers
Readers serve as the recognizer of m6A methylation that recruits complexes or cytokines to bind with RNA to execute different functions. Family proteins (YTHDF1-3 & YTHDC1-2) containing the YTH domain (Li et al., 2014) are one group of m6A readers. YTHDF1, in synergy with YTHDF3, facilitates translation and promotes mRNA binding to the ribosome. Next, YTHDF2 is the first recognized m6A reader, whose main substrate is the m6A on 5ʹ UTR, and it competes for ribosomes mediating mRNA translation (Du et al., 2016). YTHDC1 is primarily present in the nucleus to regulate gene splice exon selection, and YTHDC2 mediates mRNA degradation and regulates translation in a m6A-dependent manner (Kretschmer et al., 2018; Chen and Wong, 2020). Next, insulin-like growth factors (IGF2BPs) is another group of m6A readers that stabilize mRNA based on m6A-dependent manner (Huang et al., 2018). Also, eIF3 (Meyer et al., 2015) and other “indirect” readers recognize m6A to exert specific functions (Wu et al., 2019a). The aberrant expression of these m6A-associated downstream proteins were observed in tumorigenesis, angiogenesis, and immunoregulation.
Roles of m6A in Tumors
m6A methylation participating in the process of tumorigenesis was proved by many studies, including proliferation (Liu et al., 2018), invasion (Yue et al., 2019), and immune system evasion (Han et al., 2019). Based on the potential samples with lung and colon cancer, the METTL3 is considered as an oncogene to facilitate the development of the disease (Lin et al., 2016). Researchers reported that upregulated FTO enhanced the development and metastasis of breast cancer cell through downregulation of BCL2 interacting protein 3 (BNIP3) expression in both vitro and vivo experiment (Niu et al., 2019). Also, overexpression of YTHDC2 is observed in colon cancer, which is considered as a mark associated with poor prognosis (Tanabe et al., 2016).
RNA m5C Methylation
5-methylcytosine (m5C) is another common and dynamic RNA marker found in most organisms. Based on biochemical studies, the m5C methylated tRNA and rRNA were observed demonstrating various molecular functions (Blanco et al., 2014b; Janin et al., 2019a). For example, m5C alters the rRNA conformation to regulate synthesis of ribosome, thereby affecting translation fidelity. The tertiary structures of tRNA are maintained by m5C, which is evolutionarily conserved. Also, recent high-throughput studies based on bisulfite treatment or immunoprecipitation techniques proved that m5C modification also appears on mRNA (Huang et al., 2019).
Similar to this, m5C is also a reversible process. The m5C methylation can be added on RNAs by different enzymes, including the NSUNs family and DNMT2 (Cui et al., 2017). The ten-eleven translocator family is most likely to be a m5C eraser catalyzing the demethylation of m5C, but the mechanism of action remains to be demonstrated (Fu et al., 2014). In recent studies, the Aly/REF export factor (ALYREF) (Yang et al., 2017) was proven to demonstrate an ability to recognize m5C sites and can be considered as a m5C reader, which facilitates m5C-dependent export; furthermore, the same research group suggested that the m5C sites are substrates of the YB protein family YBX1 (Chen et al. 2019c) and YBX2, which can help mRNA stabilization and liquid-liquid phase separation in m5C-dependent manner, respectively.
Role of m5C in Tumors
Also, m5C is involved in tumor formation and various diseases. One study suggested that overexpression of NSUN2 may contribute to the proliferation of mouse fibroblasts (Perlaky et al., 1992). Meanwhile, NSUN2 is upregulated in lung cancer (Saijo et al., 2001), prostate cancer (Bantis et al., 2004), and breast carcinoma (Freeman et al., 1991), and it is used as a marker of poor prognosis. Also, to reduce the stability of p57 mRNA in gastric cancer, the NSUN2 is responsible for the m5C methylated sites on the 3’ -untranslated regions of p57, thus suppressing the expression of p57, which may lead to the development of gastric cancer (Mei et al., 2020). Furthermore, the overexpression of NSUN4 is strongly related to HCC (He et al., 2020). Also, downregulation of NSUN5 is observed in glioblastoma patients and is related to low survival (Janin et al., 2019b).
RNA m7G Methylation
N7-methylguanosine (m7G) is a commonly observed in positively charged methylated nucleotides under physiological conditions (Agris, 1996a) and was a constituent of mRNA cap structures (Ramanathan et al., 2016). Meanwhile, it is conserved between eukaryotes and archaea. As the part of 5′cap structure, m7G methylation participates in the mediation of multiple biological processes, such as pre-mRNA splicing, transcription termination, exosomal degradation, and mRNA export (Chen and Guo, 2016). Next, m7G is catalyzed by the METTL1/WDR4 complex and is recognized by eIF4E in humans, which affects the translation efficiency of transcripts at the m7G site (Lin et al., 2018).
RNA m1A Methylation
N1-methyladenosine (m1A) is a reversible modification with a high content in tRNA but a relatively low abundance in mRNA (Dominissini et al., 2016b; Shima and Igarashi, 2020). Also, m1A is a common positive electrostatic methylated modification under physiological conditions that produce electrochemical effects and is important for the biological functions and secondary structure of tRNA (Agris, 1996b). Next, m1A methylation in human mRNA is a rare modification that blocks Watson-Crick base pairing and alters the mRNA structure and initiation of translation (Li et al., 2016; Safra et al., 2017). Scientists use this feature to create high-throughput m1A sequencing technology (Hauenschild et al., 2015) to identify hundreds of m1A sites on mRNA (Li et al., 2017).
Next, currently, the TRMT6/61A complex is the only known methyltransferase which is responsible for mRNA m1A methylation (Dominissini et al., 2016b), which is demethylated by the AlkB homolog protein ALKBH3 (Woo and Chambers, 2019). In mitochondria, TRMT61B and TRMT10C methylate m1A sites in mt-tRNA transcripts (Chujo and Suzuki, 2012; Vilardo et al., 2012). In addition, m1A is also found in human and mouse 28S rRNAs, which is methylated by RRP8 (Waku et al., 2016).
Of note, ALKBH3 can demethylate m1A tRNA to promote protein synthesis, which may contribute to the cancer development (Ueda et al., 2017). Next, ALKBH1 demethylates other targeted tRNAs, affecting translation by reducing the synthesis of tRNAs in proteins (Liu et al., 2016).
Pseudouridine
Pseudouridine (Ψ), often termed as the fifth-class nucleotide, an isomerization of uracil, is the first identified posttranscriptional modification (Davis and Allen, 1957). Based on the Davis and Allen’s research, at least five percentage ribonucleic acid in transcriptome were identified as pseudouridine, and Ψ is considered as the most abundant RNA modification (Hamma and Ferré-D'Amaré, 2006). An important difference from methylation, pseuduridine is considered as an irreversible modification (Nielsen and Arison, 1989). Also, the reader of pseuduridine is still unknown.
Pseudouridylation plays a different role depending on the RNA type that is modified, and the presence of Ψ is crucial to the cancer. Dyskerin pseudouridine synthase 1 (DKC1), the enzyme catalyzing pseudouridylation, proved that it is disease associated. For example, its mutation will result in a loss of pseudouridines in mature rRNA, increase the risk to suffer the X-linked dyskeratosis congenita (X-DC), and fail the differentiation of hematopoietic stem cells to increase the risk of cancer (Kirwan and Dokal, 2008). In 28S rRNA, a reduction in pseudouridylation leads to the dysregulation of the critical mRNA translation of VEGF and p53 (Barbieri and Kouzarides, 2020b). In tRNA, aberrant expression of snoRNA24-guided Ψ influenced translation efficiency and increased stop codon readthrough frequencies, thereby driving the development of HCC (McMahon et al., 2019). Thus, pseudouridine will be a potential biomarker and is expected to be a potential anticancer target from a clinical perspective.
Role of RNA Modification in Angiogenesis
m6A in Physiological Angiogenesis
RNA m6A modification, as an important regulator of mRNA biology, often occurs in many physiological processes and plays a pivotal role in ontogeny (Liu et al., 2019). Also, currently, METTL3-mediated m6A methylation is vital in responding to hypoxic stress and promoting angiogenesis. In vitro experiments revealed that METTL3 participates in the regulation of endothelial cell viability, proliferation, and migration. Next, mechanistically, METTL3 methylates LRP6 and disheveled 1 (DVL1) for m6A to regulate Wnt signaling and further exert its angiogenic role (Yao et al., 2020). In osteogenesis, the high expression of METTL3 will activate the PI3K/AKT signaling pathway of endothelial progenitor cells to enhance the cell growth, migration ability, tube formation activity, and ultimately promoting EPCs angiogenesis (Jiang et al., 2021). Bone marrow mesenchymal stem cells (BMSCs) can secrete VEGF to promote local angiogenesis, while METTL3 is highly expressed in osteogenic differentiated BMSCs, and the knocking down of METTL3 leads to the lower expression of VEGFA (Tian et al., 2019). Another in vivo experiment showed that silencing the eraser FTO in ECs can induce hyper-methylation on critical proangiogenic genes, such as FAK, which were recognized by the m6A reader YTHDF2 to induce RNA decay, thus regulating ocular angiogenesis (Shan et al., 2020). In comparison, angiogenesis is regulated by miR-4729, which inhibits METTL14 to decrease the m6A methylation of TIE1 and further inhibit TIE1/VEGFA signaling pathways in vascular ECs (Liu et al., 2021). In addition, FTO plays a vital role in cardiac contraction function, remodeling, regenerative repair, and cardiac homeostasis. Under ischemic conditions, overexpression of FTO reduced the abnormal modification level of m6A in the whole heart and improved the expression of related proteins, thus reducing myocardial fibrosis and enhancing angiogenesis in ischemic myocardium (Mathiyalagan et al., 2019).
m6A in Pathological Angiogenesis
Vascular vessels are important channels of tumor metastasis, and angiogenesis is a hallmark of tumor aggressiveness (Goel et al., 2011). The progression of tumor angiogenesis is mutually controlled by a range of stimulators and suppressors. Also, current evidence exists that m6A affects tumor angiogenesis (Han et al., 2021).
In multiple types of cancer, overexpression of METTL3 promoted angiogenesis by enhancing oncogenes (Wang et al., 2020a). Also, the study found that METTL3 targets EphA2 and VEGFA through a IGF2BP3 dependent mechanism to promote the formation of vasculogenic mimicry in the colorectal cancer (CRC) activated PI3K/AKT and ERK1/2 signal pathway, thus promoting the development of tumors (Liu et al., 2022a). Also, METTL3 upregulated expression of JAK2 and then activated JAK2/STAT3 pathway in ox-LDL-induced human umbilical vein endothelial cells (HUVECs), and IGF2BP1 directly binds with JAK2 RNA on a m6A site, which promoted angiogenesis (Dong et al., 2021). Furthermore, a study also suggested that overexpression of METTL3 facilitated gastric cancer liver metastasis and angiogenesis in vivo, which correlated with methylation states of secreted heparin-binding growth factor (HDGF). Also, HDGF is the substrate of and the IGF2BP3, which stabilized HDGF mRNA and subsequently caused tumor angiogenesis (Wang et al., 2020a). Next, METTL3 can facilitate the maturity of miR-143-3p by splicing the precursor. Further, miR-143-3p can be a target to the vasohibin-1 (VASH1) to inhibit its translation, which leads to the hypoubiquitylation of VEGFA to inhibit its degradation and promotes angiogenesis in lung cancer (Wang et al., 2019). In bladder cancer tissues, TEK/PI3K/VEGF cascades were also enhanced by METTL3, which is involved in angiogenesis in tumor cells (Han et al., 2021). In recent studies, m6A methylation existing on circular RNAs (circRNAs) was proved, which contributes to the angiogenesis of CRC (Chen et al., 2020a; Chen et al., 2020b). One study found that ALKBH5 and YTHDF3 negatively regulated circ3823 and were significantly reduced in CRC. Circ3823 inhibited expression of miR-30c-5p to enhance the translation of TCF7. Further, the expression of MYC and CCND1 were induced by the TCF7 to facilitate CRC growth, metastasis, and angiogenesis (Guo et al., 2021). Furthermore, Chang et al. (2020) reported that the overexpressed YTHDF3 is observed in the clinical patients with brain metastases. The overexpressed YTHDF3 may facilitate the cancer cell to go through the blood-brain barrier because of its enhancement of the translation of several brain metastasis-associated m6A-enriched transcripts, namely, ST6GALNAC5, GJA1, and EGFR.
Also, gene ontology results suggested that FTO participates the regulation of angiogenesis. Next, low expression of FTO is correlated with microvessel density (MVD) in intrahepatic cholangiocarcinoma (ICC) (Rong et al., 2019), which is predicted to be associated with poor prognosis.
Moreover, studies showed that overexpression of YTHDF2 suppresses tumor growth in hepatocellular carcinoma. In terms of the relationship between YTHDF2 and tumor vessels, a study suggested that serine-type endopeptidase inhibitor 2 (SERPINE2) was upregulated in YTHDF2-silenced cells, thereby promoting angiogenesis and growth of HCC (Hou et al., 2019). Studies showed that microRNA-320b (miR-320b) can inhibit the proliferation of cancer cells and lead to apoptosis (Zhang et al., 2019). In lung cancer, the expression of IGF2BP2 was inhibited by overexpressing miR-320b. As the downstream of IGF2BP2, the stability of thymidine kinase 1 (TK1) mRNA is reduced subsequently because of the hypoexpressed IGF2BP2. Considering that the TK1 is important kinase in angiogenesis, the miR-320b suppressed cancer development by inhibiting IGF2BP2/TK1 manner (Ma et al., 2021). A recent report showed that IGF2BPs are associated with tumor progression and angiogenesis in colon cancer (Ye et al., 2016). Downregulated IGF2BP3 expression significantly reduced the m6A modification recognition of CCND1 and VEGF, which decreased both mRNA expression and stability. The m6A reader IGF2BP3 represses angiogenesis in colon cancer by regulating VEGF, thus inhibiting colon cancer angiogenesis (Yang et al., 2020). Downregulated WTAP expression in brain arteriovenous malformations (AVMs) and desmoplakin (DSP) mRNA is degraded rapidly because of the reduction in m6A methylation. In addition, the knockdown of WTAP will activate the Wilmsʹ tumor 1 (WT1) to degrade the β‐catenin, repressing the Wnt signaling pathway to suppress angiogenesis of ECs (Wang et al., 2020b).
In conclusion, N6-methyladenosine participates the regulation of angiogenesis under physiological or pathological conditions both (Figure 3).
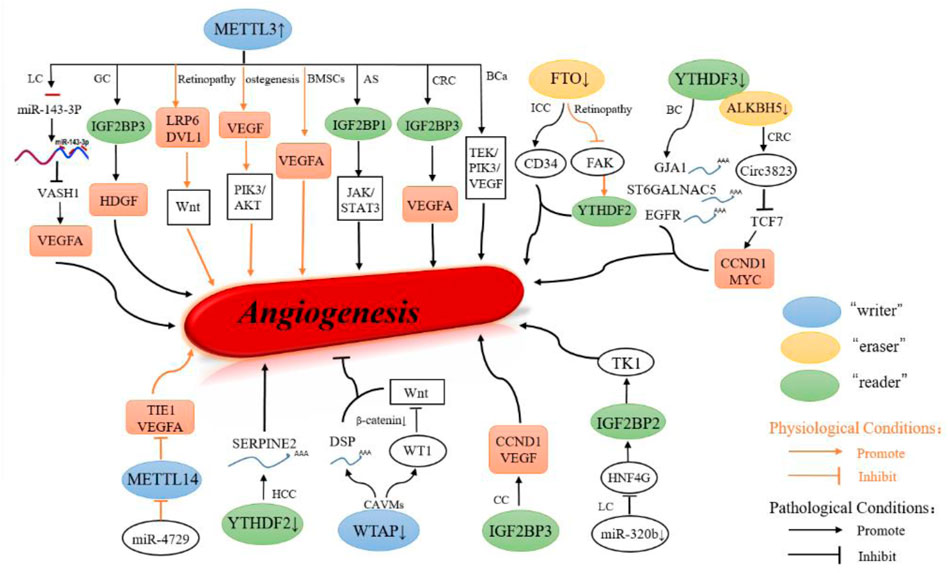
FIGURE 3. Role of m6A modification in angiogenesis. m6A is important to maintain the homeostasis of angiogenesis in physiological condition, and aberrant regulation of m6A regulators were observed in pathological condition.
m5C in Angiogenesis
In human diseases, 5-hydroxymethylation has also been found to be altered in cancer and angiogenesis (Ma et al., 2022). In recent years, the m5C writer NSUN2 has been studied most extensively. Knockout of NSUN2 significantly reduced the number of invaded cells and cell cord formation on Matrigel, thereby inhibiting the invasion, metastasis, and angiogenesis of HCC (Sun et al., 2020). To promote the UCB development, overexpression of NSUN2 and YBX1 stabilized the HDGF mRNA by regulating the m5C methylation site on its 3′ untranslated region (Chen et al., 2019a) (Figure 4).
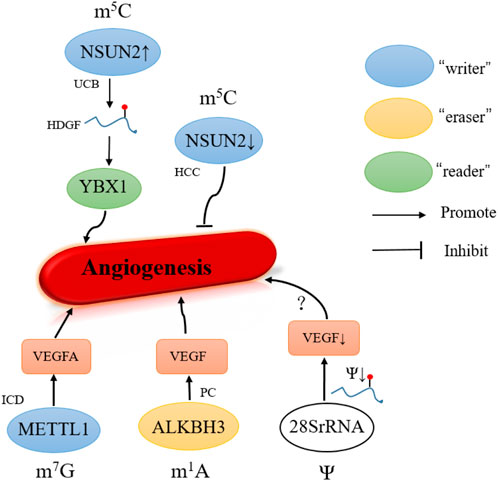
FIGURE 4. Role of other modifications in angiogenesis. UCB, urothelial carcinoma of the bladder; HCC, hepatocellular carcinoma; ICD, ischemic cardiovascular disease. PC, pancreatic cancer.
The molecular dynamics and function of RNA m5C modification are still in their infancy, and the role of m5C in angiogenesis and tumor progression is still poorly understood.
m7G in Angiogenesis
METTL1 is a key gene involved in m7G methylation that demonstrates promising functions in angiogenesis regulation and could be a treatment target of vascular diseases (Deng et al., 2020). In HUVECs, to enhance the angiogenesis, METTL1 upregulates m7G methylation of mRNA in a complex ischemic environment. Also, the METTL1 methylated adenine of VEGFA to enhance its translation to promote postischemic angiogenesis (Zhao et al., 2021). As a consequence, the METTL1 could be considered as a potentially innovative therapeutic target in peripheral arterial disease (PAD) in clinical treatment.
m1A in Angiogenesis
Also, ALKBH3, the demethylase of m1A modification, is named as prostate cancer antigen 1 (PCA-1) (Konishi et al., 2005). The knockdown of ALKBH3 can downregulate expression of VEGF to inhibit the cancer angiogenesis in vivo experiment. Also, the overexpressed ALKBH3 is observed in a few human cancers, and its expression is correlated with the TNM stage, such as pancreatic cancer (Yamato et al., 2012).
Compared to the extensively studied m6A modification, m1A still belongs to the new field of mRNA and ncRNA, and its function has not been widely explored. Therefore, whether m1A plays an important role in angiogenesis might be worth exploring.
Anti-Angiogenic Drugs With RNA Modifications
Many drugs were developed to be anti-angiogenesis in cancer, which is one important strategy in anticancer therapy. In this section, we try to summarize anti-angiogenic drugs which may associate with RNA modifications.
Erbitux (Cetuximab)
Cetuximab is an EGFR monoclonal antibody (Chen et al., 2018), which is designed to anti-angiogenesis in CRC, GC, and NHSCC. In the Cetuximab resistant CRC cell, the m6A reader hnRNPA2B1 activates the Wnt signaling pathway by stabilizing TCF4 mRNA based on m6A-dependent manner (Liu et al., 2022b).
Nexavar (Sorafenib)
Sorafenib is a kinase inhibitor which is used in the therapy of HCC, OC, RC and targets for VEGFR, PDGFR, and Raf (Chen et al., 2018). In the physiology condition, the METTL3 is responsible for the methylation site of FOXO3 mRNA to increase its stability. In the Sorafenib-resistant HCC cell, the downregulation of METTL3 affects FOXO3 and its medicated autophagy, which may contribute to the drug resistance (Lin et al., 2020).
Sutent (Sunitinib)
Sunitinib is an inhibitor of receptor tyrosine kinase, which provides multi-target therapy in NSCLC, HCC, and PC (Chen et al., 2018). In cells with Sunitinib resistance, METTL14 facilities the stabilization of TRAF1 mRNA to enhance angiogenesis (Chen et al., 2022).
Iressa (Gefitinib)
Gefitinib is an EGFR tyrosine kinase inhibitor, which is used in the NSCLC, with a promising therapeutic effect, and is used in EC and NHSCC also (Chen et al., 2018). In a recent study, the NSCLC Gefitin resistance derived from overexpression of VIRMA helps the methylation of HOXA1 to enhance its mRNA stability (Tang et al., 2021b).
Tarceva (Erlotinib)
Erlotinib is another kind of EGFR tyrosine kinase inhibitor which is used in the therapy for HCC, PC, and NSCLC patients (Chen et al., 2018). TUSC7, a suppressor of Notch signaling pathway, was inhibited by overexpressing YTHDF2 to contribute to Erlotinib resistance (Li et al., 2021).
Nivolumab (anti-PD-1)
PD-1 is an immunosuppressive molecule which acts as a vital role in promoting immune evasion that inhibits antitumor effects. Anti-PD-1/PD-L1 is mainly used for advanced melanoma (Machiraju et al., 2021) and advanced squamous NSCLC treatment (Dantoing et al., 2021). In CRC cells, FTO enables in upregulating the PD-1 expression and thus promoted immune escape (Zhang et al., 2021). Next, ALK-04 (ALKBH5 inhibitor) and suppression of METTL3 and METTL14 increased the efficacy of anti-PD-1 therapy (Li et al., 2020a; Wang et al., 2020c).
Conclusion and Perspective
In the past the decade, RNA modifications have been proven as an important layer of epigenetics, which regulates gene expression, the cell cycle, and proliferation, fetal development, and progression of disease. Angiogenesis is a vital process in fetal development and associates with tumor metastasis. Here, we reviewed recent studies to summarize the five types of RNA modification-related pathways in angiogenesis. Most studies focused on m6A, the most popular mRNA modification, to illustrate its regulatory pathway in physiological and pathological angiogenesis. Other modifications also participate in the regulation of angiogenesis, but they need to be further studied to show the complete regulatory network. In general, aberrant RNA modifications affect the RNA status of certain angiogenic factors or proteins in pathological angiogenesis to promote tumor angiogenesis.
Although the associations between RNA modifications and angiogenesis have been proven in these studies, they mostly focus on a single RNA modification and its corresponding regulators; thus, more aspects of the alteration of angiogenesis could be analyzed further: 1) RNA modification-associated mutations in angiogenesis. The correlations between carcinogenesis and variants of angiogenesis-related genes have been studied in the past few years (Crona et al., 2019; Sullivan et al., 2019). Also, several computational biology projects (Zheng et al., 2018; Song et al., 2020; Chen et al., 2021a; Wang et al., 2021a) suggested that RNA modification-associated mutations may induce diseases or contribute to disease development, and some identified variants were proven further by cohort studies (Meng et al., 2020; Wang et al., 2021b; Ruan et al., 2021). However, no study illustrated how variants affect the RNA modification status to alter angiogenesis. 2) Research on RNA modifications in angiogenesis. The crosstalk between protein modifications (PTMs) and DNA modifications in cancers has been well studied (Cedar and Bergman, 2009; Wu et al., 2019b; Hernandez-Valladares et al., 2019; Li et al., 2020b; Zhang et al., 2020), but RNA modification as an emerging epigenetic layer remains to be discovered. In a recent study for patients with CRC among different RNA modifications, the crosstalk between writers was introduced (Chen et al., 2021b). However, the detailed regulation of crosstalk between different RNA modifications sites in the pathological conditions is still unknown because of technical limitations in current sequencing methods and bioinformatics tools (Chen et al., 2019b; Liu and Chen, 2020; Song et al., 2021b; Xu et al., 2021; Liu et al., 2022c). Previous studies suggested that the different RNA modifications are involved in angiogenesis, and the association among them should be further analyzed. 3) The role of virus infection in angiogenesis. Most RNA modifications, including m6A and m5C, were proven to be presented on viral RNA, and virus infection has been reported to alter the host epitranscriptome (Courtney, 2021). Also, viral infection is associated with carcinogenesis, which promotes pathological angiogenesis and has been introduced in recent studies (Wu et al., 2022). However, whether infection alters RNA modification to promote angiogenesis is still unclear and should be considered in further studies.
Author Contributions
H-MC, HL, and S-XW wrote the manuscript; M-XL and W-JF prepared the figures; YZ and Y-TL revised the manuscript; S-XW and Y-TL conceptualized and supervised the manuscript. All authors read and agreed to the published version of the manuscript.
Funding
This work was supported by grants from the National Natural Science Foundation of China (U1905209), Joint Funds for the Innovation of Science and Technology in Fujian Province (2019Y9003), the Health and Education Joint Project of Fujian Province (2019-WJ-27), and Scientific Research Foundation for Advanced Talents of Fujian Medical University (XRCZX2020012).
Conflict of Interest
The authors declare that the research was conducted in the absence of any commercial or financial relationships that could be construed as a potential conflict of interest.
Publisher’s Note
All claims expressed in this article are solely those of the authors and do not necessarily represent those of their affiliated organizations, or those of the publisher, the editors, and the reviewers. Any product that may be evaluated in this article, or claim that may be made by its manufacturer, is not guaranteed or endorsed by the publisher.
References
Agris, P. F. (1996). The Importance of Being Modified: Roles of Modified Nucleosides and Mg2+ in RNA Structure and Function. Prog. Nucleic Acid. Res. Mol. Biol. 53, 79–129. doi:10.1016/s0079-6603(08)60143-9
Agris, P. F. (1996). The Importance of Being Modified: Roles of Modified Nucleosides and Mg2+ in RNA Structure and Function. Prog. nucleic acid Res. Mol. Biol. 53, 79–129. doi:10.1016/s0079-6603(08)60143-9
Arguello, A. E., DeLiberto, A. N., and Kleiner, R. E. (2017). RNA Chemical Proteomics Reveals the N6-Methyladenosine (m6A)-Regulated Protein-RNA Interactome. J. Am. Chem. Soc. 139, 17249–17252. doi:10.1021/jacs.7b09213
Bantis, A., Giannopoulos, A., Gonidi, M., Liossi, A., Aggelonidou, E., Petrakakou, E., et al. (2004). Expression of P120, Ki-67 and PCNA as Proliferation Biomarkers in Imprint Smears of Prostate Carcinoma and Their Prognostic Value. Cytopathology 15 (1), 25–31. doi:10.1046/j.0956-5507.2003.00090
Barbieri, I., and Kouzarides, T. (2020). Role of RNA Modifications in Cancer. Nat. Rev. Cancer 20, 303–322. doi:10.1038/s41568-020-0253-2
Barbieri, I., and Kouzarides, T. (2020). Role of RNA Modifications in Cancer. Nat. Rev. Cancer 20 (6), 303–322. doi:10.1038/s41568-020-0253-2
Blanco, S., Dietmann, S., Flores, J. V., Hussain, S., Kutter, C., Humphreys, P., et al. (2014). Aberrant Methylation of T RNA S Links Cellular Stress to Neuro‐Developmental Disorders. Embo J. 33, 2020–2039. doi:10.15252/embj.201489282
Blanco, S., Dietmann, S., Flores, J. V., Hussain, S., Kutter, C., Humphreys, P., et al. (2014). Aberrant Methylation of T RNA S Links Cellular Stress to Neuro‐developmental Disorders. Embo J. 33, 2020–2039. doi:10.15252/embj.201489282
Boccaletto, P., Stefaniak, F., Ray, A., Cappannini, A., Mukherjee, S., Purta, E., et al. (2022). MODOMICS: a Database of RNA Modification Pathways. 2021 Update. Nucleic acids Res. 50, D231–d235. doi:10.1093/nar/gkab1083
Canaani, D., Kahana, C., Lavi, S., and Groner, Y. (1979). Identification and Mapping of N6-Methyladenosine Containing Sequences in Simian Virus 40 RNA. Nucl. Acids Res. 6, 2879–2899. doi:10.1093/nar/6.8.2879
Carlile, T. M., Rojas-Duran, M. F., Zinshteyn, B., Shin, H., Bartoli, K. M., and Gilbert, W. V. (2014). Pseudouridine Profiling Reveals Regulated mRNA Pseudouridylation in Yeast and Human Cells. Nature 515 (7525), 143–146. doi:10.1038/nature138025
Cedar, H., and Bergman, Y. (2009). Linking DNA Methylation and Histone Modification: Patterns and Paradigms. Nat. Rev. Genet. 10, 295–304. doi:10.1038/nrg2540
Chang, G., Shi, L., Ye, Y., Shi, H., Zeng, L., Tiwary, S., et al. (2020). YTHDF3 Induces the Translation of m6A-Enriched Gene Transcripts to Promote Breast Cancer Brain Metastasis. Cancer Cell. 38 (6), 857–871. e7. doi:10.1016/j.ccell.2020.10.004
Chen, C., Huang, Z., Mo, X., Song, Y., Li, X., Li, X., et al. (2020). RETRACTED ARTICLE: The Circular RNA 001971/miR-29c-3p axis Modulates Colorectal Cancer Growth, Metastasis, and Angiogenesis through VEGFA. J. Exp. Clin. Cancer Res. 39, 91. doi:10.1186/s13046-020-01594-y
Chen, H., Yao, J., Bao, R., Dong, Y., Zhang, T., Du, Y., et al. (2021). Cross-talk of Four Types of RNA Modification Writers Defines Tumor Microenvironment and Pharmacogenomic Landscape in Colorectal Cancer. Mol. Cancer 20, 29. doi:10.1186/s12943-021-01322-w
Chen, K., Song, B., Tang, Y., Wei, Z., Xu, Q., Su, J., et al. (2021). RMDisease: a Database of Genetic Variants that Affect RNA Modifications, with Implications for Epitranscriptome Pathogenesis. Nucleic acids Res. 49, D1396–d1404. doi:10.1093/nar/gkaa790
Chen, K., Wei, Z., Zhang, Q., Wu, X., Rong, R., Lu, Z., et al. (2019). WHISTLE: a High-Accuracy Map of the Human N6-Methyladenosine (m6A) Epitranscriptome Predicted Using a Machine Learning Approach. Nucleic acids Res. 47, e41. doi:10.1093/nar/gkz074
Chen, L.-Y., Wang, L., Ren, Y.-X., Pang, Z., Liu, Y., Sun, X.-D., et al. (2020). The Circular RNA Circ-ERBIN Promotes Growth and Metastasis of Colorectal Cancer by miR-125a-5p and miR-138-5p/4EBP-1 Mediated Cap-independent HIF-1α Translation. Mol. Cancer 19, 164. doi:10.1186/s12943-020-01272-9
Chen, M., and Wong, C.-M. (2020). The Emerging Roles of N6-Methyladenosine (m6A) Deregulation in Liver Carcinogenesis. Mol. Cancer 19, 44. doi:10.1186/s12943-020-01172-y
Chen, W., Ding, H., Zhou, X., Lin, H., and Chou, K.-C. (2018). iRNA(m6A)-PseDNC: Identifying N6-Methyladenosine Sites Using Pseudo Dinucleotide Composition. Anal. Biochem. 561-562, 59–65. doi:10.1016/j.ab.2018.09.002
Chen, X., Li, A., Sun, B.-F., Yang, Y., Han, Y.-N., Yuan, X., et al. (2019). 5-methylcytosine Promotes Pathogenesis of Bladder Cancer through Stabilizing mRNAs. Nat. Cell. Biol. 21, 978–990. doi:10.1038/s41556-019-0361-y
Chen, X., Li, A., Sun, B. F., Yang, Y., and Yang, Y. G. J. N. C. B. (2019c). 5-methylcytosine Promotes Pathogenesis of Bladder Cancer through Stabilizing mRNAs.Nat Cell Biol 21, 978. doi:10.1038/s41556-019-0361-y
Chen, Y., and Guo, D. (2016). Molecular Mechanisms of Coronavirus RNA Capping and Methylation. Virol. Sin. 31 (1), 3–11. doi:10.1007/s12250-016-3726-4
Chen, Y., Lu, Z., Qi, C., Yu, C., Li, Y., Huan, W., et al. (2022). N6-methyladenosine-modified TRAF1 Promotes Sunitinib Resistance by Regulating Apoptosis and Angiogenesis in a METTL14-dependent Manner in Renal Cell Carcinoma. Mol. Cancer 21, 111. doi:10.1186/s12943-022-01549-1
Chujo, T., and Suzuki, T. (2012). Trmt61B Is a Methyltransferase Responsible for 1-methyladenosine at Position 58 of Human Mitochondrial tRNAs. Rna 18, 2269–2276. doi:10.1261/rna.035600.112
Courtney, D. G. (2021). Post-transcriptional Regulation of Viral RNA through Epitranscriptional Modification. Cells 10, 1129. doi:10.3390/cells10051129
Crona, D. J., Skol, A. D., Leppänen, V.-M., Glubb, D. M., Etheridge, A. S., Hilliard, E., et al. (2019). Genetic Variants of VEGFA and FLT4 Are Determinants of Survival in Renal Cell Carcinoma Patients Treated with Sorafenib. Cancer Res. 79, 231–241. doi:10.1158/0008-5472.Can-18-1089
Cui, X., Liang, Z., Shen, L., Zhang, Q., Bao, S., Geng, Y., et al. (2017). 5-Methylcytosine RNA Methylation in Arabidopsis Thaliana. Mol. Plant 10 (11), 1387–1399. doi:10.1016/j.molp.2017.09.013
Dantoing, E., Piton, N., Salaün, M., Thiberville, L., and Guisier, F. (2021). Anti-PD1/PD-L1 Immunotherapy for Non-small Cell Lung Cancer with Actionable Oncogenic Driver Mutations. Ijms 22, 6288. doi:10.3390/ijms22126288
Davis, F. F., and Allen, F. W. (1957). Ribonucleic Acids from Yeast Which Contain a Fifth Nucleotide. J. Biol. Chem. 227, 907–915. doi:10.1016/s0021-9258(18)70770-9
Deng, X., Chen, K., Luo, G.-Z., Weng, X., Ji, Q., Zhou, T., et al. (2015). Widespread Occurrence ofN6-Methyladenosine in Bacterial mRNA. Nucleic Acids Res. 43, 6557–6567. doi:10.1093/nar/gkv596
Deng, Y., Zhou, Z., Ji, W., Lin, S., and Wang, M. (2020). METTL1-mediated m7G Methylation Maintains Pluripotency in Human Stem Cells and Limits Mesoderm Differentiation and Vascular Development. Stem Cell. Res. Ther. 11, 306. doi:10.1186/s13287-020-01814-4
Desrosiers, R., Friderici, K., and Rottman, F. (1974). Identification of Methylated Nucleosides in Messenger RNA from Novikoff Hepatoma Cells. Proc. Natl. Acad. Sci. U.S.A. 71 (10), 3971–3975. doi:10.1073/pnas.71.10.3971
Dominissini, D., Moshitch-Moshkovitz, S., Schwartz, S., Salmon-Divon, M., Ungar, L., Osenberg, S., et al. (2012). Topology of the Human and Mouse m6A RNA Methylomes Revealed by m6A-Seq. Nature 485, 201–206. doi:10.1038/nature11112
Dominissini, D., Nachtergaele, S., Moshitch-Moshkovitz, S., Peer, E., Kol, N., Ben-Haim, M. S., et al. (2016). The Dynamic N1-Methyladenosine Methylome in Eukaryotic Messenger RNA. Nature 530 (7591), 441–446. doi:10.1038/nature16998
Dominissini, D., Nachtergaele, S., Moshitch-Moshkovitz, S., Peer, E., Kol, N., Ben-Haim, M. S., et al. (2016). The Dynamic N1-Methyladenosine Methylome in Eukaryotic Messenger RNA. Nature 530, 441–446. doi:10.1038/nature16998
Dong, G., Yu, J., Shan, G., Su, L., Yu, N., and Yang, S. (2021). N6-Methyladenosine Methyltransferase METTL3 Promotes Angiogenesis and Atherosclerosis by Upregulating the JAK2/STAT3 Pathway via m6A Reader IGF2BP1. Front. Cell. Dev. Biol. 9, 731810. doi:10.3389/fcell.2021.731810
Dong, Z., and Cui, H. (2020). The Emerging Roles of RNA Modifications in Glioblastoma. Cancers 12 (3), 736. doi:10.3390/cancers12030736
Du, H., Zhao, Y., He, J., Zhang, Y., Xi, H., Liu, M., et al. (2016). YTHDF2 Destabilizes m6A-Containing RNA through Direct Recruitment of the CCR4-Not Deadenylase Complex. Nat. Commun. 7, 12626. doi:10.1038/ncomms12626
Freeman, J. W., McGrath, P., Bondada, V., Selliah, N., Ownby, H., Maloney, T., et al. (1991). Prognostic Significance of Proliferation Associated Nucleolar Antigen P120 in Human Breast Carcinoma. Cancer Res. 51 (8), 1973.
Frye, M., and Blanco, S. (2016). Post-Transcriptional Modifications in Development and Stem Cells. Development 143 (21), 3871–3881. doi:10.1242/dev.136556
Fu, L., Guerrero, C. R., Zhong, N., Amato, N. J., Liu, Y., Liu, S., et al. (2014). Tet-mediated Formation of 5-hydroxymethylcytosine in RNA. J. Am. Chem. Soc. 136 (33), 11582–11585. doi:10.1021/ja505305z
Goel, S., Duda, D. G., Xu, L., Munn, L. L., Boucher, Y., Fukumura, D., et al. (2011). Normalization of the Vasculature for Treatment of Cancer and Other diseasesErratum in. Physiol. Rev. Rev. 9194 (32), 1071707–1081121. doi:10.1152/physrev.00038.2010
Guo, Y., Guo, Y., Chen, C., Fan, D., Wu, X., Zhao, L., et al. (2021). Circ3823 Contributes to Growth, Metastasis and Angiogenesis of Colorectal Cancer: Involvement of miR-30c-5p/TCF7 axis. Mol. Cancer 20 (1), 93. doi:10.1186/s12943-021-01372-0
Hamdy, M. N., Shaheen, K. Y., Awad, M. A., Barakat, E., Shalaby, S., Gupta, N., and Gupta, V. (2020). Vascular Endothelial Growth Factor (VEGF) as a Biochem- Ical Marker for the Diagnosis of Hepatocellular Carcinoma (HCC). Clin. Pract. 17, 1441–1453.
Hamma, T., and Ferré-D'Amaré, A. R. (2006). Pseudouridine Synthases. Chem. Biol., 13, 1125–1135. doi:10.1016/j.chembiol.2006.09.009
Han, D., Liu, J., Chen, C., Dong, L., Liu, Y., Chang, R., et al. (2019). Anti-tumour Immunity Controlled through mRNA m6A Methylation and YTHDF1 in Dendritic Cells. Nature 566 (7743), 270–274. doi:10.1038/s41586-019-0916-x
Han, X., Wang, M., Zhao, Y.-L., Yang, Y., and Yang, Y.-G. (2021). RNA Methylations in Human Cancers. Seminars cancer Biol. 75, 97–115. doi:10.1016/j.semcancer.2020.11.007
Hanahan, D., and Weinberg, R. A. (2011). Hallmarks of Cancer: the Next Generation. Cell. 144 (5), 646–674. doi:10.1016/j.cell.2011.02.013
Hauenschild, R., Tserovski, L., Schmid, K., Thüring, K., Winz, M. L., Sharma, S., et al. (2015). The Reverse Transcription Signature of N-1-Methyladenosine in RNA-Seq Is Sequence Dependent. Nucleic Acids Res. 43 (20), 9950–9964. doi:10.1093/nar/gkv895
He, L., Li, H., Wu, A., Peng, Y., Shu, G., and Yin, G. (2019). Functions of N6-Methyladenosine and its Role in Cancer. Mol. Cancer 18, 176. doi:10.1186/s12943-019-1109-9
He, Y., Yu, X., Li, J., Zhang, Q., Zheng, Q., and Guo, W. (2020). Role of m5C-Related Regulatory Genes in the Diagnosis and Prognosis of Hepatocellular Carcinoma. Am. J. Transl. Res. 12 (3), 912–922. doi:10.18632/aging.102669
Hernandez-Valladares, M., Wangen, R., Berven, F. S., and Guldbrandsen, A. (2019). Protein Post-Translational Modification Crosstalk in Acute Myeloid Leukemia Calls for Action. Cmc 26, 5317–5337. doi:10.2174/0929867326666190503164004
Hirsch, L., Flippot, R., Escudier, B., and Albiges, L. (2020). Immunomodulatory Roles of VEGF Pathway Inhibitors in Renal Cell Carcinoma. Drugs 80 (12), 1169–1181. doi:10.1007/s40265-020-01327-7
Hosaka, K., Yang, Y., Seki, T., Du, Q., Jing, X., He, X., et al. (2020). Therapeutic Paradigm of Dual Targeting VEGF and PDGF for Effectively Treating FGF-2 Off-Target Tumors. Nat. Commun. 11 (1), 3704. doi:10.1038/s41467-020-17525-6
Hou, J., Zhang, H., Liu, J., Zhao, Z., Wang, J., Lu, Z., et al. (2019). YTHDF2 Reduction Fuels Inflammation and Vascular Abnormalization in Hepatocellular Carcinoma. Mol. Cancer 18, 163. doi:10.1186/s12943-019-1082-3
Huang, H., Weng, H., Sun, W., Qin, X., Shi, H., Wu, H., et al. (2018). Recognition of RNA N6-Methyladenosine by IGF2BP Proteins Enhances mRNA Stability and Translation. Nat. Cell. Biol. 20, 285–295. doi:10.1038/s41556-018-0045-z
Huang, T., Chen, W., Liu, J., Gu, N., and Zhang, R. (2019). Genome-wide Identification of mRNA 5-methylcytosine in Mammals. Nat. Struct. Mol. Biol. 26 (5), 380–388. doi:10.1038/s41594-019-0218-x
Janin, M., Ortiz-Barahona, V., de Moura, M. C., Llinàs-Arias, P., Soler, M., Nachmani, D., et al. (2019). Epigenetic Loss of RNA-Methyltransferase NSUN5 in Glioma Targets Ribosomes to Drive a Stress Adaptive Translational Program. Acta Neuropathol. 138, 1053–1074. doi:10.1007/s00401-019-02062-4
Janin, M., Ortiz-Barahona, V., de Moura, M. C., Martínez-Cardús, A., Llinàs-Arias, P., Soler, M., et al. (2019). Epigenetic Loss of RNA-Methyltransferase NSUN5 in Glioma Targets Ribosomes to Drive a Stress Adaptive Translational Program. Acta Neuropathol. 138 (6), 1053–1074. doi:10.1007/s00401-019-02062-4
Jeong, J.-H., Ojha, U., and Lee, Y. M. (2021). Pathological Angiogenesis and Inflammation in Tissues. Arch. Pharm. Res. 44, 1–15. doi:10.1007/s12272-020-01287-2
Jia, G., Fu, Y., Zhao, X., Dai, Q., Zheng, G., Yang, Y., et al. (2011). N6-methyladenosine in Nuclear RNA Is a Major Substrate of the Obesity-Associated FTO. Nat. Chem. Biol. 7, 885–887. doi:10.1038/nchembio.687
Jia, G., Fu, Y., Zhao, X., Dai, Q., Zheng, G., Yang, Y., et al. (2011). N6-methyladenosine in Nuclear RNA Is a Major Substrate of the Obesity-Associated FTOErratum in. Nat. Chem. BiolNat Chem. Biol. 78 (1212), 8851008–8851887. doi:10.1038/nchembio.687
Jiang, W., Zhu, P., Huang, F., Zhao, Z., Zhang, T., An, X., et al. (2021). The RNA Methyltransferase METTL3 Promotes Endothelial Progenitor Cell Angiogenesis in Mandibular Distraction Osteogenesis via the PI3K/AKT Pathway. Front. Cell. Dev. Biol. 9, 720925. doi:10.3389/fcell.2021.720925
Kierzek, E., Zhang, X., Watson, R. M., Kennedy, S. D., Szabat, M., Kierzek, R., et al. (2022). Secondary Structure Prediction for RNA Sequences Including N6-Methyladenosine. Nat. Commun. 13, 1271. doi:10.1038/s41467-022-28817-4
Kirwan, M., and Dokal, I. (2008). Dyskeratosis Congenita: a Genetic Disorder of Many Faces. Clin. Genet. 73 (2), 103–112. doi:10.1111/j.1399-0004.2007.00923.x
Knuckles, P., Lence, T., Haussmann, I. U., Jacob, D., Kreim, N., Carl, S. H., et al. (2018). Zc3h13/Flacc Is Required for Adenosine Methylation by Bridging the mRNA-Binding Factor Rbm15/Spenito to the m6A Machinery Component Wtap/Fl(2)d. Genes. Dev. 32 (5-6), 415–429. doi:10.1101/gad.309146.117
Konishi, N., Nakamura, M., Ishida, E., Shimada, K., Mitsui, E., Yoshikawa, R., et al. (2005). High Expression of a New Marker PCA-1 in Human Prostate Carcinoma. Clin. Cancer Res. 11 (14), 5090–5097. doi:10.1158/1078-0432.CCR-05-0195
Kretschmer, J., Rao, H., Hackert, P., Sloan, K. E., Höbartner, C., and Bohnsack, M. T. (2018). The m6A Reader Protein YTHDC2 Interacts with the Small Ribosomal Subunit and the 5′-3′ Exoribonuclease XRN1. Rna 24, 1339–1350. doi:10.1261/rna.064238.117
Krug, R. M., Morgan, M. A., and Shatkin, A. J. (1976). Influenza Viral mRNA Contains Internal N6-Methyladenosine and 5'-terminal 7-methylguanosine in Cap Structures. J. Virol. 20, 45–53. doi:10.1128/jvi.20.1.45-53.1976
Li, F., Zhao, D., Wu, J., and Shi, Y. (2014). Structure of the YTH Domain of Human YTHDF2 in Complex with an m6A Mononucleotide Reveals an Aromatic Cage for m6A Recognition. Cell. Res. 24 (12), 1490–1492. doi:10.1038/cr.2014.153
Li, K., Peng, Z.-Y., Gao, S., Wang, Q.-S., Wang, R., Li, X., et al. (2021). M6A Associated TSUC7 Inhibition Contributed to Erlotinib Resistance in Lung Adenocarcinoma through a Notch Signaling Activation Dependent Way. J. Exp. Clin. Cancer Res. 40, 325. doi:10.1186/s13046-021-02137-9
Li, N., Kang, Y., Wang, L., Huff, S., Tang, R., Hui, H., et al. (2020). ALKBH5 Regulates Anti-PD-1 Therapy Response by Modulating Lactate and Suppressive Immune Cell Accumulation in Tumor Microenvironment. Proc. Natl. Acad. Sci. U.S.A. 117, 20159–20170. doi:10.1073/pnas.1918986117
Li, S., Shi, B., Liu, X., and An, H.-X. (2020). Acetylation and Deacetylation of DNA Repair Proteins in Cancers. Front. Oncol. 10, 573502. doi:10.3389/fonc.2020.573502
Li, X., Xiong, X., Wang, K., Wang, L., Shu, X., Ma, S., et al. (2016). Transcriptome-wide Mapping Reveals Reversible and Dynamic N1-Methyladenosine Methylome. Nat. Chem. Biol. 12, 311–316. doi:10.1038/nchembio.2040
Li, X., Xiong, X., Zhang, M., Wang, K., Chen, Y., Zhou, J., et al. (2017). Base-Resolution Mapping Reveals Distinct m1A Methylome in Nuclear- and Mitochondrial-Encoded Transcripts. Mol. Cell. 68 (5), 993–1005. e9. doi:10.1016/j.molcel.2017.10.019
Liang, X.-h., Liu, Q., and Fournier, M. J. (2009). Loss of rRNA Modifications in the Decoding Center of the Ribosome Impairs Translation and Strongly Delays Pre-rRNA Processing. RNA 15 (9), 1716–1728. doi:10.1261/rna.1724409
Lin, S., Choe, J., Du, P., Triboulet, R., and Gregory, R. I. (2016). The m6A Methyltransferase METTL3 Promotes Translation in Human Cancer Cells. Mol. Cell. 62 (3), 335–345doi:10.1016/j.molcel.2016.03.021
Lin, S., Liu, Q., Lelyveld, V. S., Choe, J., Szostak, J. W., and Gregory, R. I. (2018). Mettl1/Wdr4-Mediated m7G tRNA Methylome Is Required for Normal mRNA Translation and Embryonic Stem Cell Self-Renewal and Differentiation. Mol. Cell. 71 (2), 244–255. doi:10.1016/j.molcel.2018.06.001
Lin, Z., Niu, Y., Wan, A., Chen, D., Liang, H., Chen, X., et al. (2020). RNA m6A Methylation Regulates Sorafenib Resistance in Liver Cancer through FOXO 3‐mediated Autophagy. Embo J. 39, e103181. doi:10.15252/embj.2019103181
Linder, B., Grozhik, A. V., Olarerin-George, A. O., Meydan, C., Mason, C. E., and Jaffrey, S. R. (2015). Single-nucleotide-resolution Mapping of m6A and m6Am throughout the Transcriptome. Nat. Methods 12, 767–772. doi:10.1038/nmeth.3453
Liu, F., Clark, W., Luo, G., Wang, X., Fu, Y., Wei, J., et al. (2016). ALKBH1-Mediated tRNA Demethylation Regulates Translation. Cell. 167, 816–828. e816. doi:10.1016/j.cell.2016.09.038
Liu, H., Li, D., Sun, L., Qin, H., Fan, A., Meng, L., et al. (2022). Interaction of lncRNA MIR100HG with hnRNPA2B1 Facilitates m6A-dependent Stabilization of TCF7L2 mRNA and Colorectal Cancer Progression. Mol. Cancer 21, 74. doi:10.1186/s12943-022-01555-3
Liu, J., Eckert, M. A., Harada, B. T., Liu, S.-M., Lu, Z., Yu, K., et al. (2018). m6A mRNA Methylation Regulates AKT Activity to Promote the Proliferation and Tumorigenicity of Endometrial Cancer. Nat. Cell. Biol. 20 (9), 1074–1083. doi:10.1038/s41556-018-0174-4
Liu, J., Harada, B. T., and He, C. (2019). Regulation of Gene Expression by N6-Methyladenosine in Cancer. Trends Cell Biol. 29, 487–499. doi:10.1016/j.tcb.2019.02.008
Liu, K., and Chen, W. (2020). iMRM: a Platform for Simultaneously Identifying Multiple Kinds of RNA Modifications. Bioinforma. Oxf. Engl. 36, 3336–3342. doi:10.1093/bioinformatics/btaa155
Liu, L., Song, B., Chen, K., Zhang, Y., de Magalhães, J. P., Rigden, D. J., et al. (2022). WHISTLE Server: A High-Accuracy Genomic Coordinate-Based Machine Learning Platform for RNA Modification Prediction. Methods 203, 378–382. doi:10.1016/j.ymeth.2021.07.003
Liu, N., Zhou, K. I., Parisien, M., Dai, Q., Diatchenko, L., and Pan, T. (2017). N 6-methyladenosine Alters RNA Structure to Regulate Binding of a Low-Complexity Protein. Nucleic Acids Res. 45 (10), 6051–6063. doi:10.1093/nar/gkx141
Liu, T., Zhou, H., Lu, H., Luo, C., Wang, Q., Peng, Y., et al. (2021). MiR-4729 Regulates TIE1 mRNA m6A Modification and Angiogenesis in Hemorrhoids by Targeting METTL14. Ann. Transl. Med. 9 (3), 232. doi:10.21037/atm-20-339
Liu, X., He, H., Zhang, F., Hu, X., Bi, F., Li, K., et al. (2022). m6A Methylated EphA2 and VEGFA through IGF2BP2/3 Regulation Promotes Vasculogenic Mimicry in Colorectal Cancer via PI3K/AKT and ERK1/2 Signaling. Cell. Death Dis. 13, 483. doi:10.1038/s41419-022-04950-2
Ma, J., Song, B., Wei, Z., Huang, D., Zhang, Y., Su, J., et al. (2022). m5C-Atlas: a Comprehensive Database for Decoding and Annotating the 5-methylcytosine (m5C) Epitranscriptome. Nucleic acids Res. 50, D196–d203. doi:10.1093/nar/gkab1075
Ma, L., Zhao, B., Chen, K., Thomas, A., Tuteja, J. H., He, X., et al. (2017). Evolution of Transcript Modification by N6-Methyladenosine in Primates. Genome Res. 27, 385–392. doi:10.1101/gr.212563.116
Ma, Q., Reiter, R. J., and Chen, Y. (2020). Role of Melatonin in Controlling Angiogenesis under Physiological and Pathological Conditions. Angiogenesis 23, 91–104. doi:10.1007/s10456-019-09689-7
Ma, Y.-S., Shi, B.-W., Guo, J.-H., Liu, J.-B., Yang, X.-L., Xin, R., et al. (2021). microRNA-320b Suppresses HNF4G and IGF2BP2 Expression to Inhibit Angiogenesis and Tumor Growth of Lung Cancer. Carcinogenesis 42, 762–771. doi:10.1093/carcin/bgab023
Machiraju, D., Schäfer, S., and Hassel, J. C. (2021). Potential Reasons for Unresponsiveness to Anti-PD1 Immunotherapy in Young Patients with Advanced Melanoma. Life 11, 1318. doi:10.3390/life11121318
Mathiyalagan, P., Adamiak, M., Mayourian, J., Sassi, Y., Liang, Y., Agarwal, N., et al. (2019). FTO-dependent N 6 -Methyladenosine Regulates Cardiac Function during Remodeling and Repair. Circulation 139 (4), 518–532. doi:10.1161/CIRCULATIONAHA.118.033794
McMahon, M., Contreras, A., Holm, M., Uechi, T., Forester, C. M., Pang, X., et al. (2019). A Single H/ACA Small Nucleolar RNA Mediates Tumor Suppression Downstream of Oncogenic RAS. Elife 8, e48847. doi:10.7554/eLife.48847
Mei, L., Shen, C., Miao, R., Wang, J.-Z., Cao, M.-D., Zhang, Y.-S., et al. (2020). RNA Methyltransferase NSUN2 Promotes Gastric Cancer Cell Proliferation by Repressing p57Kip2 by an m5C-dependent Manner. Cell. Death Dis. 11 (4), 270. doi:10.1038/s41419-020-2487-z
Melincovici, C. S., Boşca, A. B., Şuşman, S., Mărginean, M., Mihu, C., Istrate, M., et al. (2018). Vascular Endothelial Growth Factor (VEGF) - Key Factor in Normal and Pathological Angiogenesis. Rom. J. Morphol. Embryol. 59 (2), 455
Mendel, M., Chen, K.-M., Homolka, D., Gos, P., Pandey, R. R., McCarthy, A. A., et al. (2018). Methylation of Structured RNA by the m6A Writer METTL16 Is Essential for Mouse Embryonic Development. Mol. Cell. 71 (6), 986–1000. e11. doi:10.1016/j.molcel.2018.08.004
Meng, Y., Li, S., Gu, D., Xu, K., Du, M., Zhu, L., et al. (2020). Genetic Variants in m6A Modification Genes Are Associated with Colorectal Cancer Risk. Carcinogenesis 41, 8–17. doi:10.1093/carcin/bgz165
Meyer, K. D., Patil, D. P., Zhou, J., Zinoviev, A., Skabkin, M. A., Elemento, O., et al. (2015). 5′ UTR m6A Promotes Cap-independent Translation. Cell. 163, 999–1010. doi:10.1016/j.cell.2015.10.012
Meyer, K. D., Saletore, Y., Zumbo, P., Elemento, O., Mason, C. E., and Jaffrey, S. R. (2012). Comprehensive Analysis of mRNA Methylation Reveals Enrichment in 3′ UTRs and Near Stop Codons. Cell. 149, 1635–1646. doi:10.1016/j.cell.2012.05.003
Miao, Z., Zhang, T., Xie, B., Qi, Y., and Ma, C. (2021). Evolutionary Implications of the RNA N6-Methyladenosine Methylome in Plants. Mol. Biol. Evol. 39, 299. doi:10.1093/molbev/msab299
Newman, A. C., Nakatsu, M. N., Chou, W., Gershon, P. D., and Hughes, C. C. W. (2011). The Requirement for Fibroblasts in Angiogenesis: Fibroblast-Derived Matrix Proteins Are Essential for Endothelial Cell Lumen Formation. MBoC 22, 3791–3800. doi:10.1091/mbc.E11-05-0393
Nielsen, J. B. K., and Arison, B. H. (1989). 3-Methylpseudouridine as a Fermentation Product. J. Antibiot. 42, 1248–1252. doi:10.7164/antibiotics.42.1248
Niu, Y., Lin, Z., Wan, A., Chen, H., Liang, H., Sun, L., et al. (2019). RNA N6-Methyladenosine Demethylase FTO Promotes Breast Tumor Progression through Inhibiting BNIP3. Mol. Cancer 18 (1), 46. doi:10.1186/s12943-019-1004-4
Nombela, P., Miguel-López, B., and Blanco, S. (2021). The Role of m6A, m5C and Ψ RNA Modifications in Cancer: Novel Therapeutic Opportunities. Mol. Cancer 20 (1), 18. doi:10.1186/s12943-020-01263-w
Noonan, D. M., De Lerma Barbaro, A., Vannini, N., Mortara, L., and Albini, A. (2008). Inflammation, Inflammatory Cells and Angiogenesis: Decisions and Indecisions. Cancer Metastasis Rev. 27, 31–40. doi:10.1007/s10555-007-9108-5
Pan, Y., Ma, P., Liu, Y., Li, W., and Shu, Y. (2018). Multiple Functions of m6A RNA Methylation in Cancer. J. Hematol. Oncol. 11, 48. doi:10.1186/s13045-018-0590-8
Pang, L., Wang, J., Fan, Y., Xu, R., Bai, Y., and Bai, L. (2018). Correlations of TNM Staging and Lymph Node Metastasis of Gastric Cancer with MRI Features and VEGF Expression. Cbm 23 (1), 53–59. doi:10.3233/CBM-181287
Perlaky, L., Valdez, B. C., Busch, R. K., Larson, R. G., Jhiang, S. M., Zhang, W. W., et al. (1992). Increased Growth of NIH/3T3 Cells by Transfection with Human P120 Complementary DNA and Inhibition by a P120 Antisense Construct. Cancer Res. 52 (2), 428
Ping, X.-L., Sun, B.-F., Wang, L., Xiao, W., Yang, X., Wang, W.-J., et al. (2014). Mammalian WTAP Is a Regulatory Subunit of the RNA N6-Methyladenosine Methyltransferase. Cell. Res. 24, 177–189. doi:10.1038/cr.2014.3
Ramanathan, A., Robb, G. B., and Chan, S.-H. (2016). mRNA Capping: Biological Functions and Applications. Nucleic Acids Res. 44, 7511–7526. doi:10.1093/nar/gkw551
Rong, Z.-X., Li, Z., He, J.-J., Liu, L.-Y., Ren, X.-X., Gao, J., et al. (2019). Downregulation of Fat Mass and Obesity Associated (FTO) Promotes the Progression of Intrahepatic Cholangiocarcinoma. Front. Oncol. 9, 369. doi:10.3389/fonc.2019.00369
Ruan, X., Tian, M., Kang, N., Ma, W., Zeng, Y., Zhuang, G., et al. (2021). Genome-wide Identification of m6A-Associated Functional SNPs as Potential Functional Variants for Thyroid Cancer. Am. J. Cancer Res. 11, 5402–5414.
Safra, M., Sas-Chen, A., Nir, R., Winkler, R., Nachshon, A., Bar-Yaacov, D., et al. (2017). The m1A Landscape on Cytosolic and Mitochondrial mRNA at Single-Base Resolution. Nature 551, 251–255. doi:10.1038/nature24456
Saijo, Y., Sato, G., Usui, K., Sato, M., Sagawa, M., Kondo, T., et al. (2001). Expression of Nucleolar Protein P120 Predicts Poor Prognosis in Patients with Stage I Lung Adenocarcinoma. Ann. Oncol. 12 (8), 1121–1125. doi:10.1023/a:10116177079994
Schosserer, M., Minois, N., Angerer, T. B., Amring, M., Dellago, H., Harreither, E., et al. (2015). Methylation of Ribosomal RNA by NSUN5 Is a Conserved Mechanism Modulating Organismal Lifespan. Nat. Commun. 6, 6158. doi:10.1038/ncomms7158
Schwartz, C. J., and Mitchell, J. R. A. (1962). Cellular Infiltration of the Human Arterial Adventitia Associated with Atheromatous Plaques. Circulation 26, 73–78. doi:10.1161/01.cir.26.1.73
Shan, K., Zhou, R.-m., Xiang, J., Sun, Y.-n., Liu, C., Lv, M.-w., et al. (2020). FTO Regulates Ocular Angiogenesis via m6A-YTHDF2-dependent Mechanism. Exp. eye Res. 197, 108107. doi:10.1016/j.exer.2020.108107
Shima, H., and Igarashi, K. (2020). N 1-methyladenosine (m1A) RNA Modification: the Key to Ribosome Control. J. Biochem. 167, 535–539. doi:10.1093/jb/mvaa026
Shima, H., Matsumoto, M., Ishigami, Y., Ebina, M., Muto, A., Sato, Y., et al. (2017). S-adenosylmethionine Synthesis Is Regulated by Selective N6-Adenosine Methylation and mRNA Degradation Involving METTL16 and YTHDC1. Cell. Rep. 21 (12), 3354–3363. doi:10.1016/j.celrep.2017.11.092
Śledź, P., and Jinek, M. (2016). Structural Insights into the Molecular Mechanism of the m6A Writer Complex. Elife 5, e18434. doi:10.7554/eLife.18434
Song, B., Chen, K., Tang, Y., Wei, Z., Su, J., de Magalhães, J. P., et al. (2021). ConsRM: Collection and Large-Scale Prediction of the Evolutionarily Conserved RNA Methylation Sites, with Implications for the Functional Epitranscriptome. Briefings Bioinforma. 22, 22. doi:10.1093/bib/bbab088
Song, B., Tang, Y., Chen, K., Wei, Z., Rong, R., Lu, Z., et al. (2020). m7GHub: Deciphering the Location, Regulation and Pathogenesis of Internal mRNA N7-Methylguanosine (m7G) Sites in Human. Bioinforma. Oxf. Engl. 36, 3528–3536. doi:10.1093/bioinformatics/btaa178
Song, Z., Huang, D., Song, B., Chen, K., Song, Y., Liu, G., et al. (2021). Attention-based Multi-Label Neural Networks for Integrated Prediction and Interpretation of Twelve Widely Occurring RNA Modifications. Nat. Commun. 12, 4011. doi:10.1038/s41467-021-24313-3
Sullivan, I., Riera, P., Andrés, M., Altés, A., Majem, M., Blanco, R., et al. (2019). Prognostic Effect of VEGF Gene Variants in Metastatic Non-small-cell Lung Cancer Patients. Angiogenesis 22, 433–440. doi:10.1007/s10456-019-09668-y
Sun, Z., Xue, S., Zhang, M., Xu, H., Hu, X., Chen, S., et al. (2020). Aberrant NSUN2-Mediated m5C Modification of H19 lncRNA Is Associated with Poor Differentiation of Hepatocellular Carcinoma. Oncogene 39 (45), 6906–6919. doi:10.1038/s41388-020-01475-w
Tanabe, A., Tanikawa, K., Tsunetomi, M., Takai, K., Ikeda, H., Konno, J., et al. (2016). RNA Helicase YTHDC2 Promotes Cancer Metastasis via the Enhancement of the Efficiency by Which HIF-1α mRNA Is Translated. Cancer Lett. 376 (1), 34–42. doi:10.1016/j.canlet.2016.02.022
Tang, J., Han, T., Tong, W., Zhao, J., and Wang, W. (2021). N6-methyladenosine (m6A) Methyltransferase KIAA1429 Accelerates the Gefitinib Resistance of Non-small-cell Lung Cancer. Cell. Death Discov. 7, 108. doi:10.1038/s41420-021-00488-y
Tang, Y., Chen, K., Song, B., Ma, J., Wu, X., Xu, Q., et al. (2021). m6A-Atlas: a Comprehensive Knowledgebase for Unraveling the N6-Methyladenosine (m6A) Epitranscriptome. Nucleic acids Res. 49, D134–d143. doi:10.1093/nar/gkaa692
Tian, C., Huang, Y., Li, Q., Feng, Z., and Xu, Q. (2019). Mettl3 Regulates Osteogenic Differentiation and Alternative Splicing of Vegfa in Bone Marrow Mesenchymal Stem Cells. Ijms 20, 551. doi:10.3390/ijms20030551
Ueda, Y., Ooshio, I., Fusamae, Y., Kitae, K., Kawaguchi, M., Jingushi, K., et al. (2017). AlkB Homolog 3-mediated tRNA Demethylation Promotes Protein Synthesis in Cancer Cells. Sci. Rep. 7, 42271. doi:10.1038/srep42271
van der Kwast, R. V. C. T., Quax, P. H. A., and Nossent, A. Y. (2019). An Emerging Role for isomiRs and the microRNA Epitranscriptome in Neovascularization. Cells 9 (1), 61. doi:10.3390/cells9010061
Viallard, C., and Larrivée, B. (2017). Tumor Angiogenesis and Vascular Normalization: Alternative Therapeutic Targets. Angiogenesis 20 (4), 409–426. doi:10.1007/s10456-017-9562-9
Vilardo, E., Nachbagauer, C., Buzet, A., Taschner, A., Holzmann, J., and Rossmanith, W. (2012). A Subcomplex of Human Mitochondrial RNase P Is a Bifunctional Methyltransferase-Extensive Moonlighting in Mitochondrial tRNA Biogenesis. Nucleic acids Res. 40, 11583–11593. doi:10.1093/nar/gks910
Waku, T., Nakajima, Y., Yokoyama, W., Nomura, N., Kako, K., Kobayashi, A., et al. (2016). NML-Mediated rRNA Base Methylation Links Ribosomal Subunit Formation to Cell Proliferation in a P53-dependent Manner. J. Cell. Sci. 129 (12), 2382–2393. doi:10.1242/jcs.183723
Wang, G., Dai, Y., Li, K., Cheng, M., Xiong, G., Wang, X., et al. (2021). Deficiency of Mettl3 in Bladder Cancer Stem Cells Inhibits Bladder Cancer Progression and Angiogenesis. Front. Cell. Dev. Biol. 9, 627706. doi:10.3389/fcell.2021.627706
Wang, H., Deng, Q., Lv, Z., Ling, Y., Hou, X., Chen, Z., et al. (2019). N6-methyladenosine Induced miR-143-3p Promotes the Brain Metastasis of Lung Cancer via Regulation of VASH1. Mol. Cancer 18 (1), 181. doi:10.1186/s12943-019-1108-x
Wang, L., Hui, H., Agrawal, K., Kang, Y., Li, N., Tang, R., et al. (2020). m 6 A RNA Methyltransferases METTL3/14 Regulate Immune Responses to anti‐PD‐1 therapyA RNA Methyltransferases METTL3/14 Regulate Immune Responses to Anti-PD-1 Therapy. Embo J. 39, e104514. doi:10.15252/embj.2020104514
Wang, L. j., Xue, Y., Li, H., Huo, R., Yan, Z., Wang, J., et al. (2020). Wilms' Tumour 1‐associating Protein Inhibits Endothelial Cell Angiogenesis by m6A‐dependent Epigenetic Silencing of Desmoplakin in Brain Arteriovenous Malformation. J. Cell. Mol. Medi 24 (9), 4981–4991. doi:10.1111/jcmm.15101
Wang, Q., Chen, C., Ding, Q., Zhao, Y., Wang, Z., Chen, J., et al. (2020). METTL3-mediated m6A Modification of HDGF mRNA Promotes Gastric Cancer Progression and Has Prognostic Significance. Gut 69, 1193–1205. doi:10.1136/gutjnl-2019-319639
Wang, X., Guan, D., Wang, D., Liu, H., Wu, Y., Gong, W., et al. (2021). Genetic Variants in m6A Regulators Are Associated with Gastric Cancer Risk. Arch. Toxicol. 95, 1081–1088. doi:10.1007/s00204-020-02958-1
Wang, X., Lu, Z., Gomez, A., Hon, G. C., Yue, Y., Han, D., et al. (2014). N6-Methyladenosine-Dependent Regulation of Messenger RNA Stability. Nature 505 (7481), 117–120. doi:10.1038/nature12730
Wang, Y., and Jia, G. (2020). Detection Methods of Epitranscriptomic Mark N6-Methyladenosine. Essays Biochem. 64, 967–979. doi:10.1042/ebc20200039
Wang, Y., Li, Y., Yue, M., Wang, J., Kumar, S., Wechsler-Reya, R. J., et al. (2018). N6-methyladenosine RNA Modification Regulates Embryonic Neural Stem Cell Self-Renewal through Histone Modifications. Nat. Neurosci. 21, 195–206. doi:10.1038/s41593-017-0057-1
Welten, S. M. J., Goossens, E. A. C., Quax, P. H. A., and Nossent, A. Y. (2016). The Multifactorial Nature of microRNAs in Vascular Remodelling. Cardiovasc Res. 110, 6–22. doi:10.1093/cvr/cvw039
Woo, H.-H., and Chambers, S. K. (2019). Human ALKBH3-Induced m1A Demethylation Increases the CSF-1 mRNA Stability in Breast and Ovarian Cancer Cells. Biochimica Biophysica Acta (BBA) - Gene Regul. Mech. 1862, 35–46. doi:10.1016/j.bbagrm.2018.10.008
Wu, R., Li, A., Sun, B., Sun, J.-G., Zhang, J., Zhang, T., et al. (2019). A Novel m6A Reader Prrc2a Controls Oligodendroglial Specification and Myelination. Cell. Res. 29 (1), 23–41. doi:10.1038/s41422-018-0113-8
Wu, S.-X., Ye, S.-S., Hong, Y.-X., Chen, Y., Wang, B., Lin, X.-J., et al. (2022). Hepatitis B Virus Small Envelope Protein Promotes Hepatocellular Carcinoma Angiogenesis via Endoplasmic Reticulum Stress Signaling to Upregulate the Expression of Vascular Endothelial Growth Factor A. J. Virol. 96, Jvi0197521. doi:10.1128/jvi.01975-21
Wu, Z., Huang, R., and Yuan, L. (2019). Crosstalk of Intracellular Post-translational Modifications in Cancer. Archives Biochem. biophysics 676, 108138. doi:10.1016/j.abb.2019.108138
Xu, Q., Chen, K., and Meng, J. (2021). WHISTLE: A Functionally Annotated High-Accuracy Map of Human m6A Epitranscriptome. Methods Mol. Biol. Clift. N.J.) 2284, 519–529. doi:10.1007/978-1-0716-1307-8_28
Yamato, I., Sho, M., Shimada, K., Hotta, K., Ueda, Y., Yasuda, S., et al. (2012). PCA-1/ALKBH3 Contributes to Pancreatic Cancer by Supporting Apoptotic Resistance and Angiogenesis. Cancer Res. 72 (18), 4829–4839. doi:10.1158/0008-5472.CAN-12-0328
Yang, X., Yang, Y., Sun, B.-F., Chen, Y.-S., Xu, J.-W., Lai, W.-Y., et al. (2017). 5-methylcytosine Promotes mRNA Export - NSUN2 as the Methyltransferase and ALYREF as an m5C Reader. Cell. Res. 27 (5), 606–625. doi:10.1038/cr.2017.55
Yang, Z., Wang, T., Wu, D., Min, Z., Tan, J., and Yu, B. (2020). RNA N6-Methyladenosine Reader IGF2BP3 Regulates Cell Cycle and Angiogenesis in Colon Cancer. J. Exp. Clin. Cancer Res. 39 (1), 203. doi:10.1186/s13046-020-01714-8
Yao, M.-D., Jiang, Q., Ma, Y., Liu, C., Zhu, C.-Y., Sun, Y.-N., et al. (2020). Role of METTL3-dependent N6-Methyladenosine mRNA Modification in the Promotion of Angiogenesis. Mol. Ther. 28, 2191–2202. doi:10.1016/j.ymthe.2020.07.022
Ye, S., Song, W., Xu, X., Zhao, X., and Yang, L. (2016). IGF2BP2 Promotes Colorectal Cancer Cell Proliferation and Survival through Interfering withRAF-1degradation by miR-195. FEBS Lett. 590, 1641–1650. doi:10.1002/1873-3468.12205
Yin, H., Wang, H., Jiang, W., Zhou, Y., and Ai, S. (2017). Electrochemical Immunosensor for N6-Methyladenosine Detection in Human Cell Lines Based on Biotin-Streptavidin System and Silver-SiO2 Signal Amplification. Biosens. Bioelectron. 90, 494–500. doi:10.1016/j.bios.2016.10.066
Yue, B., Song, C., Yang, L., Cui, R., Cheng, X., Zhang, Z., et al. (2019). METTL3-mediated N6-Methyladenosine Modification Is Critical for Epithelial-Mesenchymal Transition and Metastasis of Gastric Cancer. Mol. Cancer 18 (1), 142. doi:10.1186/s12943-019-1065-4
Zhang, B., Gu, X., Han, X., Gao, Q., Liu, J., Guo, T., et al. (2020). Crosstalk between DNA Methylation and Histone Acetylation Triggers GDNF High Transcription in Glioblastoma Cells. Clin. Epigenet 12, 47. doi:10.1186/s13148-020-00835-3
Zhang, S., Zhang, X., Sun, Q., Zhuang, C., Li, G., Sun, L., et al. (2019). LncRNA NR2F2-AS1 Promotes Tumourigenesis through Modulating BMI1 Expression by Targeting miR-320b in Non-small Cell Lung Cancer. J. Cell. Mol. Med. 23, 2001–2011. doi:10.1111/jcmm.14102
Zhang, Z., Zhang, C., Luo, Y., Zhang, G., Wu, P., Sun, N., et al. (2021). RNA N6 ‐methyladenosine Modification in the Lethal Teamwork of Cancer Stem Cells and the Tumor Immune Microenvironment: Current Landscape and Therapeutic Potential. Clin. Transl. Med 11, e525. doi:10.1002/ctm2.525
Zhao, B. S., Roundtree, I. A., and He, C. (2017). Post-Transcriptional Gene Regulation by mRNA Modifications. Nat. Rev. Mol. Cell. Biol. 18, 31–42. doi:10.1038/nrm.2016.132
Zhao, B. S., Roundtree, I. A., and He, C. (2017). Post-Transcriptional Gene Regulation by mRNA modificationsErratum in. Nat. Rev. Mol. Cell. BiolNat Rev. Mol. Cell. Biol. 1819 (112), 31808–31842. doi:10.1038/nrm.2016.132
Zhao, Y., Kong, L., Pei, Z., Li, F., Li, C., Sun, X., et al. (2021). m7G Methyltransferase METTL1 Promotes Post-ischemic Angiogenesis via Promoting VEGFA mRNA Translation. Front. Cell. Dev. Biol. 9, 642080. doi:10.3389/fcell.2021.642080
Zheng, G., Dahl, J. A., Niu, Y., Fedorcsak, P., Huang, C.-M., Li, C. J., et al. (2013). ALKBH5 Is a Mammalian RNA Demethylase that Impacts RNA Metabolism and Mouse Fertility. Mol. Cell. 49 (1), 18–29. doi:10.1016/j.molcel.2012.10.015
Zheng, Y., Nie, P., Peng, D., He, Z., Liu, M., Xie, Y., et al. (2018). m6Avar: a Database of Functional Variants Involved in m6A Modification. Nucleic acids Res. 46, D139–d145. doi:10.1093/nar/gkx895
Zhou, Z., Lv, J., Yu, H., Han, J., Yang, X., Feng, D., et al. (2020). Mechanism of RNA Modification N6-Methyladenosine in Human Cancer. Mol. Cancer 19, 104. doi:10.1186/s12943-020-01216-3
Glossary
m6A N6-methyladenosine;
m5C N5-methylcytosine
m7G N7-methylguanosine
m1A N1-methyladenosine
Ψ pseudopuridine
LC lung cancer
GC gastric cancer
BCa bladder cancer
ICC intrahepatic cholangiocarcinoma
BC breast cancer
CRC colorectal cancer
HCC hepatocellular carcinoma
CC colon cancer
CAVMs brain arteriovenous malformations
UCB urothelial carcinoma of the bladder
ICD ischemic cardiovascular disease
PC pancreatic cancer
ECs endothelial cells
BMSCs bone marrow mesenchymal stem cells
AS atherosclerosis
HUVECs human umbilical vein endothelial cells
VEGF vascular endothelial growth factor
FGF fibroblast growth factor
HDGF heparin-binding growth factor
MeRIP methylated RNA immunoprecipitation
miCLIP m6A individual-nucleotide-resolution cross-linking and immunoprecipitation
METTL3 methyltransferase-like 3
METTL14 methyltransferase-like 14
RBM15 RNA-binding protein 15
VIRMA virus like m6A methyltransferase associated protein
Zc3h13 zinc finger CCCH domain-containing protein 13
YTHDC YTH domain-containing
YTHDF YTH domain-containing family
FTO fat mass and obesity-related proteins
ALKBH5 alkB homologue 5
IGF2BPs Insulin-like growth factors
NSUN2 NOP2/Sun RNA methyltransferase family member 2
ALYREF Aly/REF export factor
YBX1 Y box binding protein 1
MVD microvessel density
PAD peripheral arterial disease
PCA-1 prostate cancer antigen 1
Keywords: RNA modifications, angiogenesis, tumor, m6A, m5C
Citation: Chen H-M, Li H, Lin M-X, Fan W-J, Zhang Y, Lin Y-T and Wu S-X (2022) Research Progress for RNA Modifications in Physiological and Pathological Angiogenesis. Front. Genet. 13:952667. doi: 10.3389/fgene.2022.952667
Received: 25 May 2022; Accepted: 20 June 2022;
Published: 22 July 2022.
Edited by:
Jia Meng, Xi’an Jiaotong-Liverpool University, ChinaReviewed by:
Marco Biggiogera, University of Pavia, ItalyLin Zhang, China University of Mining and Technology, China
Copyright © 2022 Chen, Li, Lin, Fan, Zhang, Lin and Wu. This is an open-access article distributed under the terms of the Creative Commons Attribution License (CC BY). The use, distribution or reproduction in other forums is permitted, provided the original author(s) and the copyright owner(s) are credited and that the original publication in this journal is cited, in accordance with accepted academic practice. No use, distribution or reproduction is permitted which does not comply with these terms.
*Correspondence: Shu-Xiang Wu, d3VzaHV4aWFuZ0Bmam11LmVkdS5jbg==; Yan-Ting Lin, dGluZ3lhbmxpbkBmam11LmVkdS5jbg==
†These authors have contributed equally to this work