- 1Tropical Futures Institute, James Cook University Singapore, Singapore, Singapore
- 2Facultad de Ciencias Veterinarias y Pecuarias, Universidad de Chile, Santiago, Chile
- 3Temasek Life Sciences Laboratory, National University of Singapore, Singapore, Singapore
- 4James Cook University, Singapore, Singapore
- 5Open Blue Sea Farms, Panama City, Panama
- 6Centre for Sustainable Tropical Fisheries and Aquaculture, James Cook University, Townsville, QLD, Australia
Background: Cobia (Rachycentron canadum) is a species of fish with high commercial potential particularly due to fast growth rates. The evidence of sexual size dimorphism favoring females indicate potential benefits in having a monosex culture. However, the involvement of genetic factors responsible for sexual development and gonadal maintenance that produces phenotypic sex in cobia is largely unknown.
Methods: In the present study, we performed transcriptome sequencing of cobia to identify sex-biased significantly differentially expressed genes (DEGs) in testes and ovaries. The reliability of the gonad transcriptome data was validated by qPCR analysis of eight selected significantly differential expressed sex-related candidate genes.
Results: This comparative gonad transcriptomic analysis revealed that 7,120 and 4,628 DEGs are up-regulated in testes or ovaries, respectively. Further functional annotation analyses identified 76 important candidate genes involved in sex determination cascades or sex differentiation, including 42 known testis-biased DEGs (dmrt1, amh and sox9 etc.), and 34 known ovary-biased DEGs (foxl2, sox3 and cyp19a etc.). Moreover, eleven significantly enriched pathways functionally related to sex determination and sex differentiation were identified, including Wnt signaling pathway, oocyte meiosis, the TGF-beta signaling pathway and MAPK signaling pathway.
Conclusion: This work represents the first comparative gonad transcriptome study in cobia. The putative sex-associated DEGs and pathways provide an important molecular basis for further investigation of cobia’s sex determination, gonadal development as well as potential control breeding of monosex female populations for a possible aquaculture setting.
Introduction
Cobia (Rachycentron canadum) is a tropical aquaculture species with high potential due to fast growth rate, high flesh quality, high survival rates, and ease of spawning and larviculture (Holt et al., 2007). Exhibiting extreme growth rates reaching between 4 and 8 kg in the first year, cobia was first proposed as a potential aquaculture species by Hassler and Rainville (1975). Since then, cobia aquaculture has increased in popularity with global production rising from only 9 tonnes in 1997 to nearly 30,000 tonnes in 2007 (FAO, 2009), and reaching an estimated 55,000 tonnes in Taiwan, Panama, China, and Vietnam in 2019 (Tveterås et al., 2019). In recent years, research in cobia aquaculture has been focused on addressing knowledge gaps in commercial production, nutrition, and the use of genomic techniques to enhance its aquaculture performance. This is a response to its apparent slow growth in production during the 2010s (Tveterås et al., 2019; Benetti et al., 2021).
Cobia are multiple batch spawners, and reach sexual maturity within 2 years of age under aquaculture settings. The year-round spawning of large numbers of high-quality eggs with high fertilization has been shown possible through the control and manipulation of water parameters (Stieglitz et al., 2012). Cobia displays a strong sexual growth dimorphism with females growing significantly faster and reaching larger sizes than males, both in wild and captivity (Shaffer and Nakamura, 1989; Dutney et al., 2017; Molina et al., 2018; Díaz-Muñoz et al., 2019). Sexual size dimorphism creates the potential for a monosex female cobia population culture to maximise commercial production, similar to giant freshwater prawn (Nair et al., 2006; Levy et al., 2017) and Nile tilapia (Chakraborty et al., 2011; Moniruzzaman et al., 2015). However, the genetic mechanisms underlying cobia’s rapid growth rates, particularly in females, remains unknown, and to date there is no reliable method (i.e., sex-specific DNA markers) to distinguish the genotypic sex of cobia. Apart from limiting the potential advantage of a monosex female population culture, this also results in another major complication in cobia aquaculture: a breeding program with inefficient selection of broodstock where fast growing individuals, notably females, dominate the pool of breeding candidates when selection is based purely on weight. Without the ability to accurately test for the genotypic sex of cobia, breeding programs are unable to capitalise on potential genetic gains in desired traits necessary for commercial production such as fast growth, disease resistance, and survival (Benetti et al., 2021).
Sex determination mechanisms in fish are diverse and complex, ranging from genotypic sex determination (GSD) to environmental sex determination (ESD) systems. For most gonochoristic fish, the mechanism of genetic sex determination is associated with either polygene or a critical gene on sex chromosomes or autosomes (Kikuchi and Hamaguchi, 2013). Currently, many master sex determination genes have been reported to play a key role in regulating sex development in fish species [(for review see (Chen et al., 2022):], such as dmy/dmrt1 in Japanese rice fish/medaka (Oryzias latipes) (Matsuda et al., 2002; Nanda et al., 2002), and sdY in rainbow trout (Oncorhynchus mykiss) (Yano et al., 2012). These sex determining genes dictate the direction in development of bipotential gonads as either an ovary or a testis (Stévant and Nef, 2019). Cobia is considered a gonochoristic species as the even representation of males and females in its farmed cohorts, suggesting that there is a genetic system (i.e., sex chromosomes and a master gene) controlling the sex determination of the species (Shaffer and Nakamura, 1989). Unfortunately, cytologically there is no distinguishable sex chromosomes observed between genders (Jacobina et al., 2011; Benetti et al., 2021) in cobia. In addition, genomic information of the species is still scarce, and limited knowledge is known about the molecular mechanisms of sex determination and sex differentiation within cobia.
Transcriptome screening is one of the most powerful and efficient methods for discovering functional genes (Vidotto et al., 2013), as well as genetic markers. Gonads are indispensable reproductive organs, and their development is commonly controlled by multiple sex-associated genes and pathways. With the rapid development of sequencing technology, RNA sequencing (RNA-Seq) of gonadal samples has been used to examine sex determination or differentiation in various fish species (Lin et al., 2017; Fu et al., 2020; Li et al., 2020; Chen et al., 2021; Lin et al., 2021; Gao et al., 2022). The transcriptomic information available in cobia is currently limited. To date, only a liver transcriptome (pooled RNA samples extracted from hepatic tissue of ninety fish) (Barbosa et al., 2020) and some RNASeq data recently published identifying immune related genes in the liver, spleen and head kidney infected by Photobacterium damselae subsp. Piscicida (Tran et al., 2018) and Streptococcus dysgalactiae (Maekawa et al., 2019) are available. RNASeq has also been used to identify genes responsible for salinity regulation and adaptation in the gill of cobia (Cao et al., 2020; Huang et al., 2021). In the present study, we screened differentially expressed genes (DEGs) between testes and ovaries in cobia via comparative gonad transcriptome analysis, and identified sex-associated key genes and pathways. This work will offer a data source to further investigate the underlying molecular regulatory mechanisms of sex determination and gonadal development in cobia, which has a potential to contribute to a fast growing all-female population at a commercial level.
Materials and methods
Samples collection
The gonad samples of cobia in this study were provided by Open Blue Sea Farms, the Republic of Panama. At the farm, the fish were cultured in 10 m3 circular HDPE tanks in flow through mode with incoming water treated by mechanical filtration, protein skimmer and UV filters. Water quality parameters were monitored daily, with averaged water temperatures of 27.5°C with range from 26°C in winter to 30°C in summer months, and also stable 35ppt full salinity water with pure oxygen injection through ceramic diffusers to maintain dissolved oxygen at 8 mg/L. In order to progressively track and determine if there is significant sexually dimorphic growth of male and female cobia, and especially the point of significant divergence in growth, the body weight and total length of 170 fish were individually PIT (Passive Integrated Transponder) tagged and their body weight were regularly weighted at twenty-one sampling points from an early age of ca. 3 months post hatching to 1 year old. The sex of individuals (77 males and 93 females) was determined through gonadal observations at the harvesting point and the gender fitted retrospectively to the growth data set. A total of twelve gonad tissues (six ovaries and six testes) for RNASeq were dissected from two-year-old adult fish of same cohort. After dissection, the determination of fish gender was performed by visual inspection of the gross morphology of gonads. The testes were clearly distinct from ovaries, and both sexes of the fish were at the spawning capable phase, late developing subphase (Lefebvre and Denson, 2012). Ovaries were observed to be well developed with vitelogenic eggs clearly visible and milt was present in testes (Figure 1D). All the samples were placed in RNAlater (Ambion) and stored at −80°C before RNA isolation.
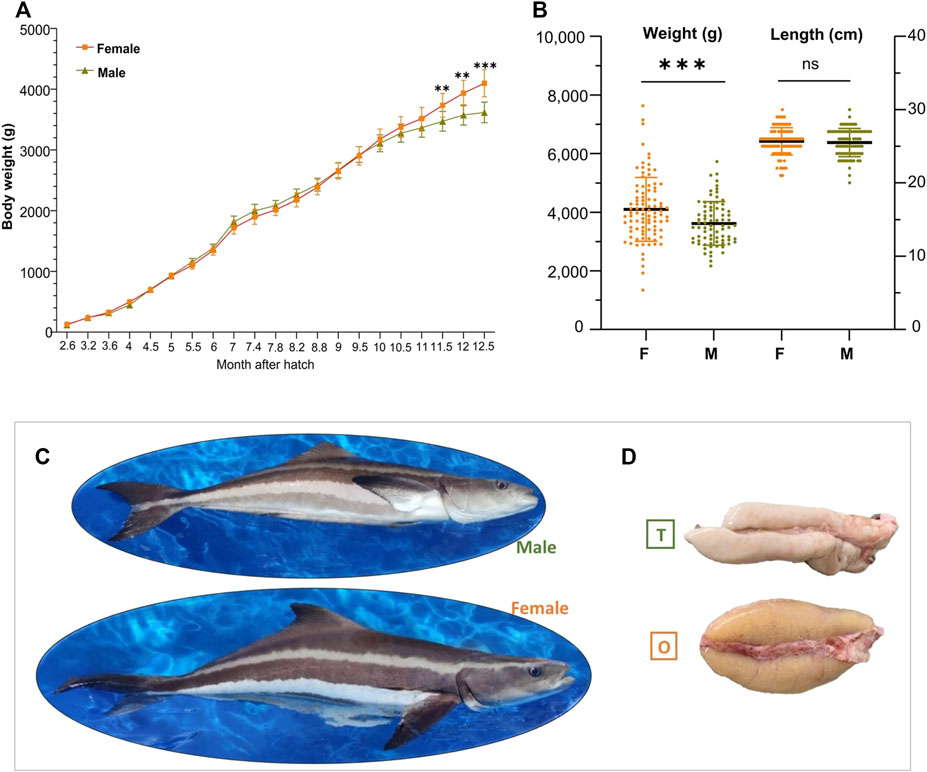
FIGURE 1. Sexual dimorphism in the growth of cobia. (A) Body weight of cobia at indicated time points after hatching (spawning date: 04/04/2018). (B) Body weight and body length of 1-year old adults (n = 170; M_Male_77&F_Female_93). “**”, p < 0.01 and “***”, p < 0.001. (C) Representative photos of 2-year old male and female cobia. (D) Photos of the cobia gonads at adult stage. T, testis; O, ovary.
RNA isolation, library construction and sequencing
Total RNA was extracted from each gonad tissue using RNeasy® Mini kit (Qiagen, 74104), following the manufacturer’s instructions. All RNA samples were treated with RNase free DNase-I (M610A, Promega) to remove genomic DNA contamination. The quality and the quantity of the total RNA was determined with an Agilent 2100 Bioanalyzer (RNA 6000 Nano chip assay) and a Qubit 3.0 (Quant-It dsRNA BR Assay). Only the RNA samples with RNA integrity number (RIN) > 8.5 were used for constructing the cDNA library in HiSeq. A total of ten libraries of gonadal samples (one testis and one ovary not included for library construction due to the poor quality of RNA) were generated using VAHTS mRNASeq V3 Library Prep Kit for Illumina (NR611, Vazyme), following manufacturer’s instructions. Briefly, mRNA with poly(A) was enriched by mRNA Capture Beads and fragmented by heating. Short mRNA was reverse-transcribed with random hexamer-primers to generate the first cDNA, and then the second cDNA was synthesized. cDNA fragments went through an end repair process, the addition of a single ‘A’ base to the 3’ end and then ligation of the adapters. Then the products were purified and size selected (350 bp range). At the end, fragments were enriched by PCR amplification and purified using VAHTSTM DNA Clean Beads. The quality and quantity of the PCR product was determined by the Agilent Bioanalyzer 2100 and Qubit 2.0 (Thermo). Finally, ten cobia sequencing libraries (five from testes and five from ovaries with RIN value >8.5) were sequenced on an Illumina Novaseq 6000 platform with 150 bp paired-end reads.
Sex-biased gene differential expression, functional GO and pathway enrichment analysis
High-quality clean data were produced from the raw data by removing reads containing adapters, more than 10 unknown nucleotides, or more than 50 low-quality (Q ≤ 20) bases. Paired-end clean reads were aligned with the cobia reference genome (unpublished data) using TopHat v2.0.12 (Kim et al., 2013). Genetic quantification of gene expression level was determined with HTSeq v0.6.1 by counting the reads numbers mapped to each gene (Anders et al., 2015). The expected number of fragments per kilobase of transcript sequence per million base pairs sequenced (FPKM) of each gene was calculated based on the length of the gene and reads count mapped to this gene. To characterize differentially expressed genes (DEGs) between ovary and testis, the raw reads number data sets were analyzed using the DESeq R package (1.18.0) (Wang et al., 2009). Genes with an adjusted p-value <0.05 and |log2FoldChange|≥0.7 were assigned as the threshold for indicating significant differential expression. Gene Ontology (GO) enrichment analysis of differentially expressed genes was implemented by the GOseq R package, in which gene length bias was corrected (Young et al., 2010). GO terms with corrected p-values < 0.05 were considered significantly enriched by differentially expressed genes. KOBAS software (Mao et al., 2005) was utilized to test the statistical enrichment of those differential expression genes in Kyoto Encyclopedia of Genes and Genomes (KEGG, http://www.genome.jp/kegg/) pathways (Kanehisa et al., 2016). The protein-protein interaction (PPI) network prediction (http://cn.string-db.org) was adopted for a set of selected candidate sex-related DEGs (Minimum required interaction score was 0.4.) and the obtained gene target network was imported into Cytoscape software (https://cytoscape.org/) for visual editing.
Validation of the DEGs by quantitative real-time PCR (qPCR)
The reliability of the gonad transcriptome data was validated by qPCR analysis of eight selected significantly differentially expressed sex-related candidate genes. The primers were designed using Primer3 (Rozen and Skaletsky, 2000) on the Geneious Prime 2021.2.2 (Biomatters) software. Total RNA was isolated from gonad samples of three males and three females using RNeasy® Plus Micro Kit (Qiagen) according to the manufacturer’s instructions. The RNA was then subjected to reverse transcription using a SensiFAST cDNA Synthesis Kit (Bioline). qPCR was performed on StepOnePlus™ Real-Time PCR System thermal cycler (Applied Biosystems) using KAPA SYBR FAST qPCR Master Mix (2X) (Sigma-Aldrich). Gene expression stability of all samples for ubq, ef1a, and b-actin was analysed using the geNorm (Vandesompele et al., 2002), plugin on the qbase+ 3.3 software (Biogazelle), of which, ubq was determined to be the most stable (M = 1.46), and was chosen as an internal control to determine relative expression. The relative gene expression levels were calculated using the 2−ΔΔCt method (Livak and Schmittgen, 2001), normalised by ubq. All statistical analysis between testes and ovaries were performed on SPSS Statistics v28.0.1.1 (IBM). Values with p < 0.05 were considered significant.
Results
Sexually dimorphic growth in cobia
To characterize the sexual dimorphism in growth of cobia, fish of both sexes were measured at twenty-one sampling points from ca. 3 months post hatching. As shown in Figure 1A, female-biased growth was observed after 9.5 months of age (avg. weight of M_2.93 kg; F_2.90 kg), with differences in weight between the sexes increasing as the trial continued. However, statistical differences were only observed from 11.5 months of age. At the end of the experiment, female fish were significantly (p < 0.001) heavier than males (13.5%), with an average body weight of 4.11 kg compared to 3.62 kg, for females and males, respectively (Figure 1B). The females also had longer average body length compared to the males although no significant difference was observed (Figures 1B, C). These results confirm that female cobia have higher growth rates and larger size at maturation than males. Figure 1D shows that testis is clearly distinct from ovary in the species at adult stage.
Overview of sequencing data and read mapping of testis and ovaries
RNASeq of all ten libraries produced 488,232,862 (73.23 Gb) and 601,664,668 (90.25 Gb) raw reads from testes and ovaries, respectively (Supplementary Table S1). All raw sequencing data were submitted to the Sequence Read Archive (SRA) of the NCBI database under BioProject accession number PRJNA853560. After removing low-quality reads, rRNA reads, reads containing adapters, and reads with >10 unknown nucleotides, 485,824,650 (72.87 Gb) and 598,371,962 (89.76 Gb) clean reads were remained for testis and ovaries, respectively. An average of 16.26 Gb (ranging from 11.55 to 37.95 Gb) clean reads were generated from each sample. The Q20 and Q30 ranged from 96.55% to 98.26% and 92.52%–94.93% respectively, indicating that the sequencing data were of high quality. All the filtered clean reads were then mapped individually against the annotated reference genome of a male cobia (unpublished data), with an averaged mapping rate of 83.76 per sample (Supplementary Table S1).
Identification and enrichment analysis of the differentially expressed genes (DEGs) in testes and ovaries of cobia
By comparison of the unigene expression levels in gonadal transcriptomes, a total of 11,748 DEGs were detected between the two sexes (Supplementary Table S2). Compared to the testes, there were 4,628 upregulated DEGs and 7,120 downregulated DEGs in the ovaries (Figure 2A). Further analysis indicated that the number of male-specific expressed genes was larger than those of females (739 vs. 26) (Figure 2B; Supplementary Table S2). In addition, the heatmap generated based on all the DEGs detected in ovaries and testes showed the transcriptomic profiles of testes was obviously different than the ovaries (Figure 2C).
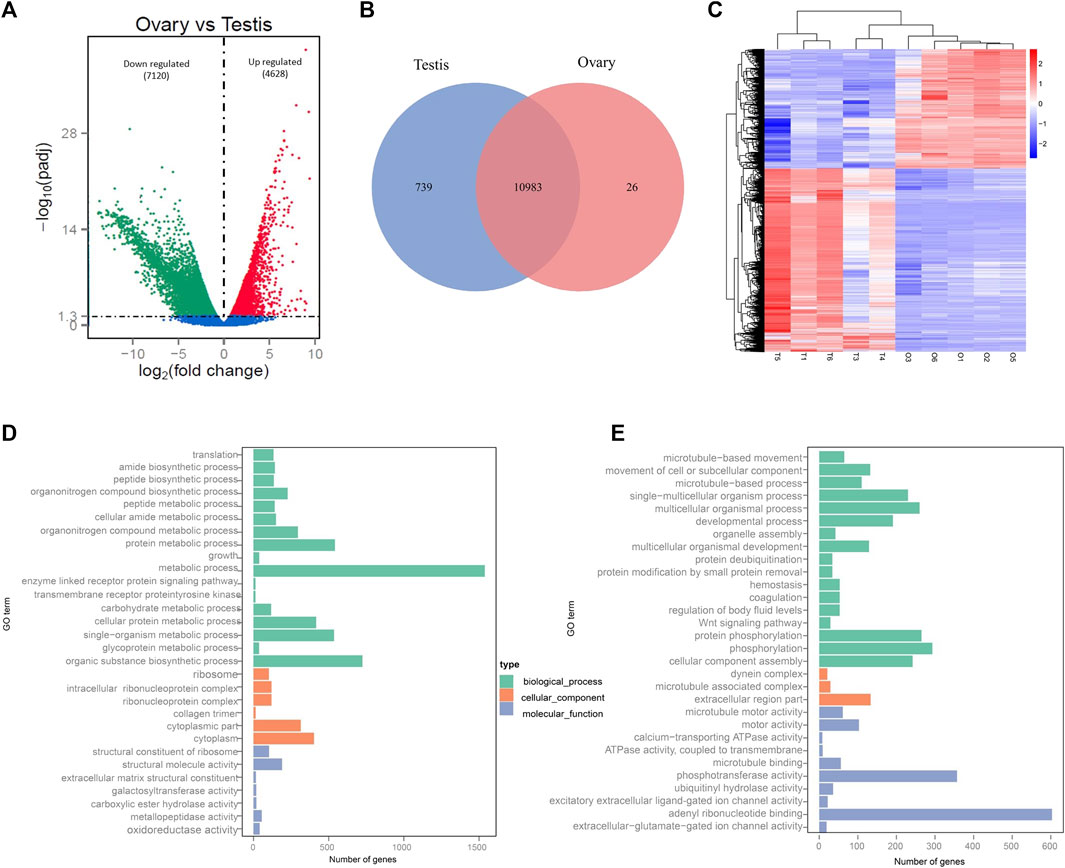
FIGURE 2. Identification and GO functional annotation of DEGs between testes and ovaries. (A) Number of up-/down-expressed DEGs in testes versus ovaries. (B) Venn diagram of testis-specific and ovarian-specific genes. (C) Heatmap analysis of hierarchical clustering of DEGs in males (T1, T3, T4, T5, T6) and females (O1, O2, O3, O5, O6). Each column represents an individual, and each row represents a gene. Blue and red colors in the heatmap correspond to low and high relative gene expression, respectively. (D) Top 30 enriched GO terms for ovary-biased DEGs. (E) Top 30 enriched GO terms for testis-biased DEGs.
Furthermore, GO functional annotation and KEGG pathway analysis were searched for all DEGs. A total of 8,543 unigenes (5,237 female-biased genes and 3,306 male-biased genes) were assigned to 4,265 GO terms (Supplementary Table S3). The DEGs were primarily classified within “biological processes”, followed by “molecular function” and then “cellular component”. More importantly, many DEGs were involved with sex-related GO terms, such as gonad development, gamete generation, germ cell development, sexual reproduction, sex differentiation, spermatogenesis, reproductive processes and meiotic cell cycle processes, etc. (Supplementary Table S3). In addition, the GO annotation of ovary-biased DEGs (Figure 2D; Supplementary Table S4) showed that the top three most significant GO terms were structural constituent of ribosome, ribosome and translation; while microtubule-based movement, microtubule motor activity and movement of cell or subcellular were the top three most significant GO terms for testis-biased DEGs (Figure 2E; Supplementary Table S5). KEGG pathway enrichment analysis indicated that the DEGs were annotated in 151 signaling pathways (Supplementary Table S6). Figure 3 shows the top 20 most significantly enriched pathways (p < 0.05) for ovary upregulated (Figure 3A) and downregulated DEGs (Figure 3B), respectively. Notably, eleven out of these top significantly enriched pathways functionally related to reproduction, sex determination and differentiation were identified, including Wnt signaling pathway, TGF-beta signaling pathway, MAPK signaling pathway and p53 signaling pathway (Supplementary Table S7) associated with female-biased DEGs; as well as endocytosis, adrenergic signaling in cardiomyocytes, neuroactive ligand-receptor interaction, Wnt signaling pathway, MAPK signaling pathway, FoxO signaling pathway, Insulin signaling pathway, oocyte meiosis and progesterone-mediated oocyte maturation associated with male-biased DEGs (Supplementary Table S8).
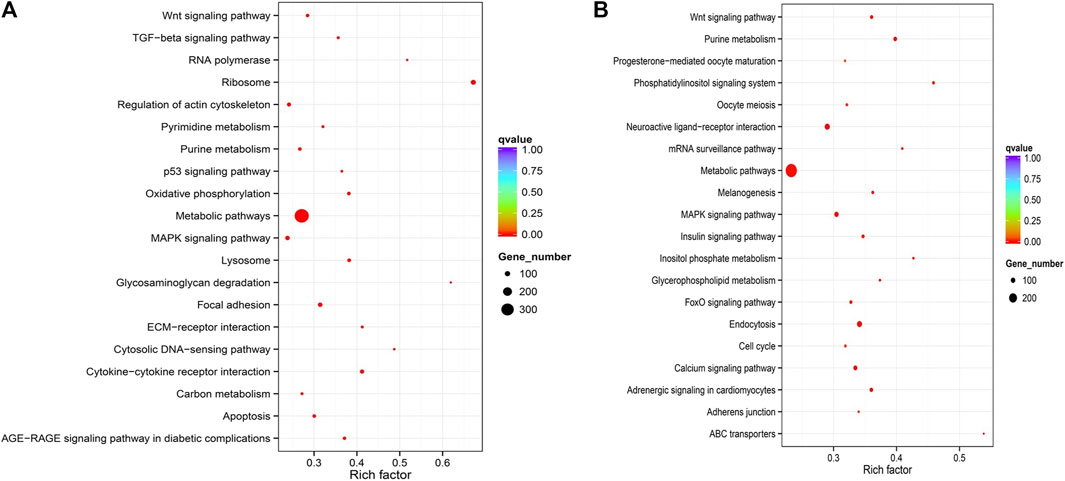
FIGURE 3. Top20 KEGG pathways enrichment of upregulated DEGs (Ovary vs Testis) (A) and downregulated DEGs (Ovary vs Testis) (B).
Analysis of the expression pattern of representative gonadal genes implicated in sex determination and sex differentiation of cobia
Based on the resources of annotation and enrichment analyses, the DEGs were further comparatively analysed. A total of seventy-six either well known candidate gonadal sex differentiation genes or genes involved in the sex determination cascade of teleosts were detected in the cobia gonadal transcriptome, which showed significant sexually dimorphic expression between the testes and ovaries (Table 1). Among them, forty-two were upregulated in the testes, while thirty-four exhibited significantly higher expressions in the ovaries (Table 1; Figure 4A). The interaction networks of the DEGs are shown in Figure 4B. The genes essential for testis differentiation and maintenance of male specified germ cells in fish included: doublesex and mab-3 related transcription factor genes (dmrt1), anti-Müllerian hormone (amh) and its receptor amhr2, SRY (sex-determining region Y)-box genes (sox9a, sox9b, sox8), piwi-like protein genes (piwil1 and piwil2), cytochromes P450 enzyme (cyp11b, cyp17a2), which were identified and significantly upregulated in the testes of cobia. The genes associated with spermatogenesis such as synaptonemal complex protein (sycp1, sycp2, sycp3), Kelch-like protein 10 (klhl10), tekt1 (Tektin-1), spermatogenesis-associated genes (spata1, spata6, spata13, spata17), outer dense fiber protein (odf3, odf3b and odf2), DEAD-box helicase (Ddx) family members (ddx4/vasa, ddx5), and others were also highly upregulated in testes. The expressions of genes related to ovary differentiation including: sox3, sox7, sox11, sox17, forkhead transcription factor L2 gene (foxl2), cytochromes P450 enzyme (cyp19a1, cyp11a1), factor in the germline alpha (figla), GATA Binding Protein 4 (gata4), Nuclear receptors superfamily genes (nr5a2, nr0b1/dax1)), Wnt signaling components (wnt4a, wnt5b), hydroxysteroid dehydrogenases (hsd17b1, hsd17b10, hsd3b), zona pellucida sperm-binding proteins (zp1, zp3, zp4), Wilm’s tumor 1 (wt1), gonadal soma derived factor 1 (gdf9) and bone morphogenetic protein (bmp2, bmp15), were highly upregulated in ovaries. Among these seventy-six representative reproductive related DEGs, we found that two cyp genes of cyp11b and cyp1a1, as well as two wnt family members, wnt1 and wnt4b, showed male-specific expression patterns.
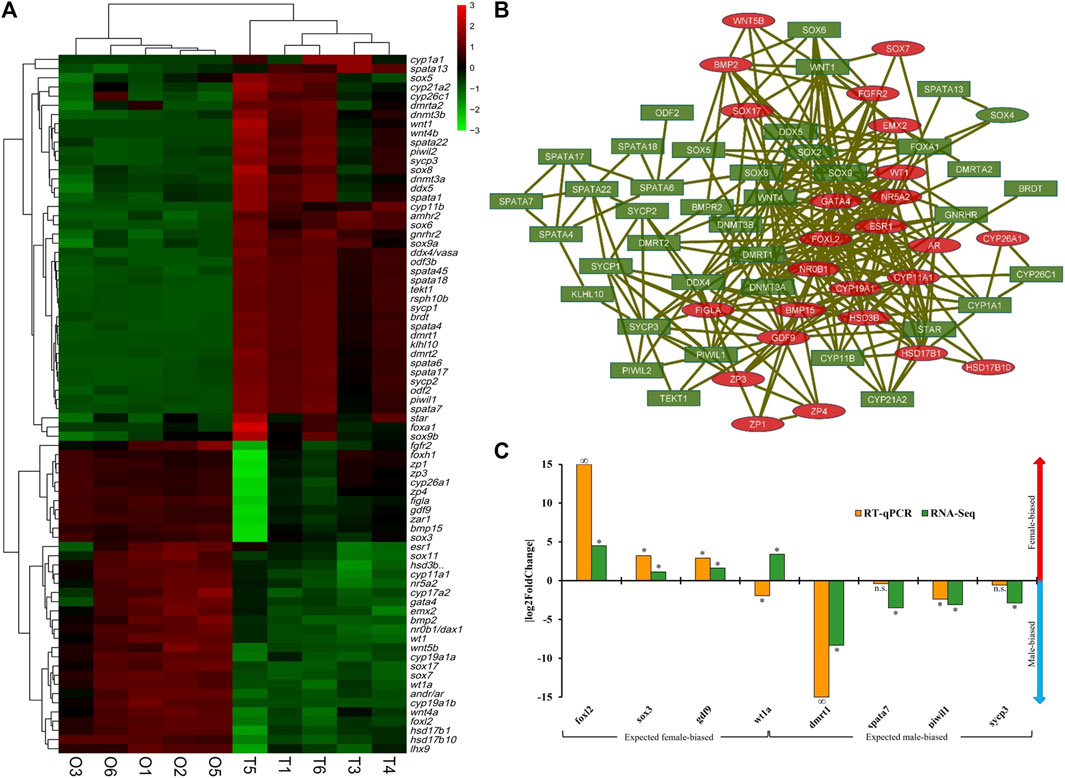
FIGURE 4. Sexually differentially expressed genes (DEGs) of cobia (p < 0.05). (A) The expression pattern of the representative gonadal genes implicated in sex determination/differentiation of cobia. In the heatmap, each row represents a gene listed on the right. Each column stands for a gonadal sample of males (T1, T3, T4, T5, T6) and females (O1, O2, O3, O5, O6). The expression of genes is colour coded from low (green) to high (red). M: male-biased, F: female-biased. (B) The testis upregulated (green with rectangle shapes) and ovary upregulated sex candidate genes (red with oval shapes) were filtered into the DEG PPI network complex. Nodes represent genes, edges represent correlation between nodes. (C) Validation of the expression patterns of selected significantly expressed DEGs from RNA-Seq, using qPCR. Positive |log2FoldChange| indicates female-biasness, negative values indicate male-biasness. ‘*’represents significant difference between testes and ovaries (p-value < 0.05), n.s. Represents non-significance, ‘∞’ represents infinite log2FoldChange for RT-qPCR results.
Verification of DEGs by qPCR
To validate the transcriptome data, expression profiles of four selected male-biased genes (dmrt1, spata7, piwil1, sycp3) and four female-biased genes (sox3, gdf9, foxl2, wt1a) representing sex-related candidate genes were individually evaluated by qPCR (primer sequences in Supplementary Table S9). The results showed that except for wt1a, the overall up- or downregulation expression profiles of all four male-biased DEGs and three female-biased DEGs were consistent between qPCR and transcriptome data (Figure 4C), indicating the validity of the RNASeq data. Interestingly, two genes showed sex-specific expression with foxl2 detected only in female and dmrt1 only in male of cobia individuals by qPCR (Figure 4C).
Discussion
This study revealed previous findings (Shaffer and Nakamura, 1989; Dutney et al., 2017; Molina et al., 2018; Díaz-Muñoz et al., 2019) that female cobia grow significantly faster than male fish. Sexual size dimorphism has been observed in a number of commercially significant fish species [for review see (Mei and Gui, 2015):]. For sexual maturity of cobia, males (around 1 year old) have a lower average age than females (1.5–2 year old) (Kuang et al., 2021). In this study, the divergence in growth rate between male and female fish at 11.5 months post-hatching seems to start around the age of male maturation. The earlier maturation in males is usually associated with slower growth rates as more energy investment into reproduction (Hüssy et al., 2012). The potential mechanism for the divergence in sexual size dimorphism phenomena of cobia needs to be further investigated.
The current study represents the first comparative gonad transcriptome studies of cobia to date. The identified sexually dimorphic genes (DEGs), especially the seventy-six well documented candidate genes associated with sex determination and sex-specific gonad development may be effective indicators for cobia genotypic sex prediction; such as the DM-domain-containing genes of dmrt1, dmrt2 and dmrta2. dmrt1 is essential for the maintenance of male-specified germ cells and testes differentiation (Webster et al., 2017). In fish, dmrt1 is a master sex-determining gene in half-smooth tongue sole (Cynoglossus semilaevis) (Cui et al., 2017), and its duplicated copy of dmy was confirmed as a sex-determining gene in medaka (Oryzias latipes) (Matsuda et al., 2002; Bratuś and Słota, 2006; Herpin and Schartl, 2011). In the protandrous hermaphrodite barramundi (Lates calcarifer) (Domingos et al., 2018), higher methylation levels in the promoter region of dmrt1 in female gonads is associated with the total splicing out of DM domain in dmrt1 mRNA, indicating a seemingly ubiquitous sex related role of this transcription factor in vertebrates. In the present study, dmrt1 was highly expressed in the testes of cobia (log2FC = 8.3). The trend of its expression was quite similar to those in other fish such as tilapia (O. niloticus) (Kobayashi and Nagahama, 2009; Tao et al., 2013), rainbow trout (Cavileer et al., 2009) and blunt snout bream (Megalobrama amblycephala) (Su et al., 2015), suggesting that dmrt1 may be a key player for cobia testicular differentiation. In addition, the dmrt2 gene highly expressed in male germ cells in Chinese tongue sole (Zhu et al., 2019), was also significantly upregulated during ovary-to-testis sex reversal in the swamp eel (Sheng et al., 2014). Here, dmrt2 was dominantly expressed in male gonads (log2FC = 7.6) of cobia, indicating this gene may play a functional role in gonadal differentiation/development and germ cell maturation in the testes of cobia.
The present study also detected eight members of the SOX gene family including sox9, sox8, sox6 and sox5 highly expressed in testes, whereas the sox3, sox7, sox11 and sox17 were dominantly expressed in ovaries, suggesting that the SOX genes play complicated roles in sex differentiation in cobia. The sex-determining region Y (SRY) is a sex determining gene in humans (Maloy and Hughes, 2013), which is the key switch for individuals developing into males or females. Several other SRY-related HMG-box transcription factors were also found to be involved in the regulation of sex determination and differentiation in fish. sox3 is the male-determining factor on the Y chromosome in the fish Oryzias dancena (Takehana et al., 2014), while it was found mainly expressed in the ovaries of yellowfin seabream (Acanthopagrus latus) (Li et al., 2020). In addition, sox2 is the master sex determination gene in turbot (Martínez et al., 2019). Notably, the sox9 gene, a major transcription factor in testicular development, has been widely studied in various fish species, for example, rainbow trout and Japanese flounder (Paralichthys olivaceus) (Baron et al., 2005; Raghuveer and Senthilkumaran, 2010; Li et al., 2018).
The forkhead-box L2 (foxl2) transcription factor is considered a marker of ovarian differentiation in vertebrates including fish. As in gonochoristic fish species, foxl2 was exclusively expressed in somatic cells of developing (pre-vitellogenic) ovaries (Wang et al., 2004; Nakamoto et al., 2006; Yamaguchi et al., 2007; Nakamoto et al., 2009; Jiang et al., 2011). In protogynous hermaphrodite fish, foxl2 expression level declines from early ovarian developmental stages to the stage of transition to testes (Kobayashi et al., 2010; Hu et al., 2014a), and vice versa in protandrous species (Wu and Chang, 2009). In our study, foxl2 was predominantly expressed in females (log2FC = 4.5), suggesting it is associated with female gonad differentiation in cobia. Also, foxl2 functions by binding to the promoter region of cyp19a (P450 aromatase) activating transcription and regulating estrogen synthesis (Zhang et al., 2017). In fish, cyp19a1 plays a pivotal role in sex differentiation and ovary development by converting testosterone into estradiol, and is regarded as a reliable early marker of ovarian differentiation (Yuan et al., 2021). In the present study, cyp19a1 was found to be highly upregulated in ovaries compared to testes, exhibiting a similar expression pattern to other fish species such as Nile tilapia (Oreochromis niloticus), tiger puffer (Takifugu rubripes) and spotted scat (Scatophagus argus) (Tao et al., 2013; Jiang et al., 2017; Yan et al., 2018; He et al., 2019; Chen et al., 2021). Other CYP components are also involved in the steroid hormone biosynthesis pathway and are required for sex steroid production in various teleost species including rainbow trout, European sea bass (Dicentrachus labrax) and spotted scat (Liu et al., 2000; Socorro et al., 2007; Blázquez et al., 2017; He et al., 2019; Meng et al., 2020; Nyuji et al., 2020). Our study identified eight steroid-metabolizing enzymes with cyp1a1, cyp11b, cyp26c1 and cyp21a2 overexpressed in testes, especially the first two showing male-specific expression; while cyp19a1, cyp11a, cyp26a1 and cyp17a2 highly upregulated in the ovaries. Cyp1a1 has a role in E2 metabolism in mammals, and is suggested to be primarily responsible for E2 metabolism in zebrafish (Scornaienchi et al., 2010; Sun et al., 2018). High expression of cyp1a1 was observed in the ovaries of Japanese sardine (Nyuji et al., 2020) and stinging catfish (Chaube et al., 2021), suggesting its critical role in the complex regulation of FOM and ovulation. However, cyp1a1 is overexpressed in the testes of Olive Flounder (Paralichthys olivaceus) (Fan et al., 2014). P450 11β-hydroxylase (cyp11b), a key enzyme for the synthesis of 11-ketotestosterone (11-KT) in testes, is a potent masculinising steroid in fish species such as honeycomb groupers (Epinephelus merra) (Bhandari et al., 2006; Wang and Orban, 2007). For example, the expression of cyp11b was observed to be either male-specific or comparatively higher in the testes of European sea bass (Socorro et al., 2007), rainbow trout (Liu et al., 2000), tiger puffer (Yan et al., 2018), Spot-fin porcupinefish (Chen et al., 2021) and Japanese flounder (Meng et al., 2020). In tambaqui (C. macropomum) however, cyp1a1 was upregulated in females (Lobo et al., 2020). Therefore, the CYP members identified in this study may play potential roles in the development of gonads and participate in regulating reproductive functions (e.g., synthesis of steroid hormones) of cobia.
Moreover, two members of wnt family including wnt1 and wnt4b were also exclusively expressed in the testes of cobia. Th wnt family has been linked to testicular development and suppression of developmental pathways in ovaries (Tevosian and Manuylov, 2008). One of the best characterized components in wnt/b-catenin signaling pathway, wnt4, is involved in the mice testes determination pathway via acting with the testis-determining gene Sry to initiate proper testes differentiation. wnt4 is also known as one of the few key ovarian-determining genes in mammals (Vainio et al., 1999). This indicated that wnt4 can have a specific, but distinct role in both male and female gonad development (Jeays-Ward et al., 2004). Different from mammals, fish possess multiple subtypes of wnt4 (wnt4a1, wnt4a2 and wnt4b) due to teleost-specific whole genome duplication. The three different wnt4 subtypes were observed to be either strongly expressed in ovarian tissue or in the early stage of testes for several studied fish species including medaka (Li et al., 2012), orange-spotted grouper (Chen et al., 2015), black seabream (Wu and Chang, 2009), spotted scat (Chen et al., 2016), half-smooth tongue sole (Hu et al., 2014b), olive flounder (Chen et al., 2016) and rainbow trout (Nicol et al., 2012). These results showed that fish do not display an ovary-predominant wnt4 expression profile during early gonadal differentiation. Similar to wnt4, the wnt1 was observed in the testes of mice (Erickson et al., 1993), however, this gene is also known to act in the WNT/β-catenin signaling pathway, to the membrane of oocytes and early preimplantation embryos in mammals (Harwood et al., 2008). In fish, a previous study in lake sturgeon (Acipenser fulvescens) showed wnt1 was more highly expressed in females compared to males (Hale et al., 2010). The male-specific expression pattern of wnt1 and wnt4b presented in our study, suggests that they may be necessary to trigger testicular differentiation in male cobia, and thus could provide an important clue for gonad differentiation of the species. Therefore, further underlying functional analysis of these genes and their regulatory network should be conducted.
In addition, DEGs such as vasa, zar1, three zps, nine spatas, and piwil involving germ cell development, gametogenesis and gamete maturation, were also in the gonad of cobia in the current study. A recent publication (Ma et al., 2022) showed that the vasa gene appeared to be specifically expressed in the testes and ovaries of cobia and mainly expressed in germ cells by qPCR and chromogenic in situ hybridization (CISH) analysis. Zona pellucida plays a protective role in fish oocytes, and is important in sperm binding (Litscher and Wassarman, 2018). In the present study, zp1, zp3 and zp4 showed higher expression levels in females than in males, suggesting these genes may also play critical roles in folliculogenesis and reproduction in cobia. Overall, these identified gonocyte-specific genes may be helpful for investigating the control mechanisms during oogenesis and spermatogenesis in cobia. Furthermore, according to functional prediction and classification, the identified gonadal differentially expressed genes of cobia were significantly enriched in eleven reproductive related pathways including the Wnt signaling pathway, MAPK signaling pathway and TGF-beta signaling pathway, which have all play important roles in the development of ovarian and testicular functions. The pathway enrichment analyses for the ovary- and testis-biased genes provide insights into the molecular landscape underlying the functions of ovaries and testes. The comparison of ovarian and testicular transcriptomes offers a precious resource for further investigation of the genetic basis of sex determination, sex differentiation and sexual size dimorphism of cobia. In the future, our current study may be complemented with mRNA, miRNA and lncRNA sequencing for undifferentiated gonads and differentiated gonads of immature males and immature females to have more comprehensive studies for investigation of the sex determination and sex differentiation of the species.
Conclusion
This study compared ovary and testis transcriptomes of cobia, identifying a set of DEGs and pathways known to be involved in gonadal development, gametogenesis and physiological function. This is the first comparative gonad transcriptomic study in cobia, providing significant information to enrich the genetic resources of the species. Furthermore, the valuable information on sex-associated genes could further facilitate exploration of the molecular mechanisms of gonad development and gametogenesis in cobia. Our findings can also provide a reference for future research on the mechanism of sexual growth dimorphism and sex control breeding of monosex female cobia populations in commercial farming.
Data availability statement
The datasets presented in this study can be found in online repositories. The names of the repository/repositories and accession number(s) can be found below: https://www.ncbi.nlm.nih.gov/genbank/, PRJNA853560.
Ethics statement
Ethical review and approval was not required for the animal study because All fish samples and data reported in this study were historical datasets and preserved gonadal samples of harvested (dead) fish from commercial production destined for human consumption. The testis and ovary samples used in this study were not derived from experimentally manipulated animals. Research on harvested (dead) commercially farmed animals do not require approval from our Institutional Animal Care and Use Committee (IACUC).
Author contributions
XS: Project coordination and data analysis, wrote the first draft of the manuscript; JD: Conceived and managed the project, obtained funding and revised the manuscript; JY: Conceived the project, obtained funding and revised the manuscript; GBG: Performed sample logistics, extractions and quality controls, revised the manuscript; ZP: Performed the qPCR validation analysis; DF and JA: Carried out the growth experiment and most of the sampling in farm. All authors gave final approval for publication and agreed to be held accountable for the work performed therein.
Funding
This work was funded by the Cobia Genome Consortium established between Open Blue Sea Farms Panama S.A. (OBSF), Singapore campus of James Cook University (JCUS) and University of Chile (UoC).
Conflict of interest
Authors DF and JA were employed by Open Blue Sea Farms.
The remaining authors declare that the research was conducted in the absence of any commercial or financial relationships that could be construed as a potential conflict of interest.
The authors declare that this study received funding from Open Blue Sea Farms Panama S.A. (OBSF). The funder had the following involvement with the study in carrying out the growth experiment, most of the sampling in farm and revising manuscript.
Publisher’s note
All claims expressed in this article are solely those of the authors and do not necessarily represent those of their affiliated organizations, or those of the publisher, the editors and the reviewers. Any product that may be evaluated in this article, or claim that may be made by its manufacturer, is not guaranteed or endorsed by the publisher.
Supplementary material
The Supplementary Material for this article can be found online at: https://www.frontiersin.org/articles/10.3389/fgene.2023.1128943/full#supplementary-material
References
Anders, S., Pyl, P. T., and Huber, W. (2015). HTSeq—A Python framework to work with high-throughput sequencing data. bioinformatics 31, 166–169. doi:10.1093/bioinformatics/btu638
Barbosa, D. A., Araújo, B. C., Branco, G. S., Hilsdorf, A. W., Nunes, L. R., Moreira, R. G., et al. (2020). De novo transcriptome assembly and functional annotation of cobia (Rachycentron canadum): Molecular approach as a base to nutritional studies. bioRxiv. doi:10.1101/2020.12.04.374918
Baron, D., Houlgatte, R., Fostier, A., and Guiguen, Y. (2005). Large-scale temporal gene expression profiling during gonadal differentiation and early gametogenesis in rainbow trout. Biol. reproduction 73, 959–966. doi:10.1095/biolreprod.105.041830
Benetti, D. D., Suarez, J., Camperio, J., Hoenig, R. H., Tudela, C. E., Daugherty, Z., et al. (2021). A review on cobia, Rachycentron canadum, aquaculture. J. World Aquac. Soc. 52, 691–709. doi:10.1111/jwas.12810
Bhandari, R. K., Alam, M. A., Soyano, K., and Nakamura, M. (2006). Induction of female-to-male sex change in the honeycomb grouper (Epinephelus merra) by 11-ketotestosterone treatments. Zoological Sci. 23, 65–69. doi:10.2108/zsj.23.65
Blázquez, M., Medina, P., Crespo, B., Gómez, A., and Zanuy, S. (2017). Identification of conserved genes triggering puberty in European sea bass males (Dicentrarchus labrax) by microarray expression profiling. BMC genomics 18, 1–17. doi:10.1186/s12864-017-3823-2
Bratuś, A., and Słota, E. (2006). DMRT1/Dmrt1, the sex determining or sex differentiating gene in Vertebrata. Folia Biol. (Kraków) 54, 81–86. doi:10.3409/173491606778557563
Cao, D., Li, J., Huang, B., Zhang, J., Pan, C., Huang, J., et al. (2020). RNA-seq analysis reveals divergent adaptive response to hyper-and hypo-salinity in cobia, Rachycentron canadum. Fish physiology Biochem. 46, 1713–1727. doi:10.1007/s10695-020-00823-7
Cavileer, T., Hunter, S., Okutsu, T., Yoshizaki, G., and Nagler, J. (2009). Identification of novel genes associated with molecular sex differentiation in the embryonic gonads of rainbow trout (Oncorhynchus mykiss). Sex. Dev. 3, 214–224. doi:10.1159/000228722
Chakraborty, S. B., Mazumdar, D., Chatterji, U., and Banerjee, S. (2011). Growth of mixed-sex and monosex nile Tilapia in different culture systems. Turkish J. Fish. Aquatic Sci. 11. doi:10.4194/trjfas.2011.0117
Chaube, R., Rawat, A., Inbaraj, R., and Joy, K. (2021). Cloning and characterization of estrogen hydroxylase (cyp1a1 and cyp1b1) genes in the stinging catfish Heteropneustes fossilis and induction of mRNA expression during final oocyte maturation. Comp. Biochem. Physiology Part A Mol. Integr. Physiology 253, 110863. doi:10.1016/j.cbpa.2020.110863
Chen, H., Li, S., Xiao, L., Zhang, Y., Li, G., Liu, X., et al. (2015). Wnt4 in protogynous hermaphroditic orange-spotted grouper (Epinephelus coioides): Identification and expression. Comp. Biochem. Physiology Part B Biochem. Mol. Biol. 183, 67–74. doi:10.1016/j.cbpb.2015.01.002
Chen, J., Yinglei, L., Zhang, J., Liu, H., and Li, Y. (2016). Identification and expression analysis of two Wnt4 genes in the spotted scat (Scatophagus argus). Electron. J. Biotechnol. 19, 20–27. doi:10.1016/j.ejbt.2016.01.003
Chen, H., Li, Z., Wang, Y., Huang, H., Yang, X., Li, S., et al. (2021). Comparison of gonadal transcriptomes uncovers reproduction-related genes with sexually dimorphic expression patterns in Diodon hystrix. Animals 11, 1042. doi:10.3390/ani11041042
Chen, J., Zhu, Z., and Hu, W. (2022). Progress in research on fish sex determining genes. Water Biol. Secur. 1, 100008. doi:10.1016/j.watbs.2022.100008
Cui, Z., Liu, Y., Wang, W., Wang, Q., Zhang, N., Lin, F., et al. (2017). Genome editing reveals dmrt1 as an essential male sex-determining gene in Chinese tongue sole (Cynoglossus semilaevis). Sci. Rep. 7, 1–10. doi:10.1038/srep42213
Díaz-Muñoz, D. N., Díaz, N., Torres, O., Leiva, J. C., Palacios, H., Romero, F., et al. (2019). Culture of cobia Rachycentron canadum in a recirculation aquaculture system in northern Chile. Lat. Am. J. aquatic Res. 47, 733–742. doi:10.3856/vol47-issue5-fulltext-2
Domingos, J. A., Budd, A. M., Banh, Q. Q., Goldsbury, J. A., Zenger, K. R., and Jerry, D. R. (2018). Sex-specific dmrt1 and cyp19a1 methylation and alternative splicing in gonads of the protandrous hermaphrodite barramundi. PLoS One 13, e0204182. doi:10.1371/journal.pone.0204182
Dutney, L., Elizur, A., and Lee, P. (2017). Analysis of sexually dimorphic growth in captive reared cobia (Rachycentron canadum) and the occurrence of intersex individuals. Aquaculture 468, 348–355. doi:10.1016/j.aquaculture.2016.09.044
Erickson, R. P., Lai, L. W., and Grimes, J. (1993). Creating a conditional mutation of Wnt-1 by antisense transgenesis provides evidence that Wnt-1 is not essential for spermatogenesis. Dev. Genet. 14, 274–281. doi:10.1002/dvg.1020140405
Fan, Z., You, F., Wang, L., Weng, S., Wu, Z., Hu, J., et al. (2014). Gonadal transcriptome analysis of male and female olive flounder (Paralichthys olivaceus). BioMed Res. Int. 2014, 291067, doi:10.1155/2014/291067
FAO (2009). Fishstat plus Vers. 2.3.2000: Universal software for fishery statistical time series: Aquaculture production 1950-2007; Capture production 1950-2007. FAO Fisheries and Aquaculture Department, Fishery Information, Data and Statistics Unit.
Fu, Y., Xu, Z., Wen, B., Gao, J., and Chen, Z. (2020). Gonad-specific transcriptomes reveal differential expression of gene and miRNA between male and female of the discus fish (Symphysodon aequifasciatus). Front. Physiology 11, 754. doi:10.3389/fphys.2020.00754
Gao, D., Huang, J., Lin, G., and Lu, J. (2022). A time-course transcriptome analysis of gonads from yellow catfish (Pelteobagrus fulvidraco) reveals genes associated with gonad development. BMC genomics 23, 409–413. doi:10.1186/s12864-022-08651-0
Hale, M. C., Jackson, J. R., and Dewoody, J. A. (2010). Discovery and evaluation of candidate sex-determining genes and xenobiotics in the gonads of lake sturgeon (Acipenser fulvescens). Genetica 138, 745–756. doi:10.1007/s10709-010-9455-y
Harwood, B. N., Cross, S. K., Radford, E. E., Haac, B. E., and De Vries, W. N. (2008). Members of the WNT signaling pathways are widely expressed in mouse ovaries, oocytes, and cleavage stage embryos. Dev. Dyn. 237, 1099–1111. doi:10.1002/dvdy.21491
Hassler, W. W., and Rainville, R. V. (1975). “Techniques for hatching and rearing cobia (Rachycentron canadum) through larval and juvenile stages,” in University of North Carolina, Sea Grant Program, 1235 Burlington Laboratories, UNC-SG-75-30, Raleigh, NC (NOAA), 26.
He, F. X., Jiang, D.-N., Huang, Y.-Q., Mustapha, U. F., Yang, W., Cui, X. F., et al. (2019). Comparative transcriptome analysis of male and female gonads reveals sex-biased genes in spotted scat (Scatophagus argus). Fish physiology Biochem. 45, 1963–1980. doi:10.1007/s10695-019-00693-8
Herpin, A., and Schartl, M. (2011). Dmrt1 genes at the crossroads: A widespread and central class of sexual development factors in fish. FEBS J. 278, 1010–1019. doi:10.1111/j.1742-4658.2011.08030.x
Holt, J. G., Faulk, C. K., and Schwarz, M. H. (2007). A review of the larviculture of cobia Rachycentron canadum, a warm water marine fish. Aquaculture 268, 181–187. doi:10.1016/j.aquaculture.2007.04.039
Hu, Q., Guo, W., Gao, Y., Tang, R., and Li, D. (2014a). Molecular cloning and analysis of gonadal expression of Foxl2 in the rice-field eel Monopterus albus. Sci. Rep. 4, 6884–6887. doi:10.1038/srep06884
Hu, Q., Zhu, Y., Liu, Y., Wang, N., and Chen, S. (2014b). Cloning and characterization of wnt4a gene and evidence for positive selection in half-smooth tongue sole (Cynoglossus semilaevis). Sci. Rep. 4, 7167–7169. doi:10.1038/srep07167
Huang, B., Liu, F., Cao, D., Li, G., Lin, K., Li, J., et al. (2021). Identification and characterization of miRNAs and target genes response to hypoosmotic adaption in the cobia, Rachycentron canadum. Aquac. Res. 52, 3322–3335. doi:10.1111/are.15177
Hüssy, K., Coad, J. O., Farrell, E. D., Clausen, L. W., and Clarke, M. W. (2012). Sexual dimorphism in size, age, maturation, and growth characteristics of boarfish (Capros aper) in the Northeast Atlantic. ICES J. Mar. Sci. 69, 1729–1735. doi:10.1093/icesjms/fss156
Jacobina, U. P., Cioffi, M. D. B., Souza, L. G. R., Calado, L. L., Tavares, M., Manzella, J., et al. (2011). Chromosome mapping of repetitive sequences in Rachycentron canadum (perciformes: Rachycentridae): Implications for karyotypic evolution and perspectives for biotechnological uses. J. Biomed. Biotechnol. 2011, 1–8. doi:10.1155/2011/218231
Jeays-Ward, K., Dandonneau, M., and Swain, A. (2004). Wnt4 is required for proper male as well as female sexual development. Dev. Biol. 276, 431–440. doi:10.1016/j.ydbio.2004.08.049
Jiang, W., Yang, Y., Zhao, D., Liu, X., Duan, J., Xie, S., et al. (2011). Effects of sexual steroids on the expression of foxl2 in Gobiocypris rarus. Comp. Biochem. Physiology Part B Biochem. Mol. Biol. 160, 187–193. doi:10.1016/j.cbpb.2011.08.005
Jiang, D., Chen, J., Fan, Z., Tan, D., Zhao, J., Shi, H., et al. (2017). CRISPR/Cas9-induced disruption of wt1a and wt1b reveals their different roles in kidney and gonad development in Nile tilapia. Dev. Biol. 428, 63–73. doi:10.1016/j.ydbio.2017.05.017
Kanehisa, M., Sato, Y., Kawashima, M., Furumichi, M., and Tanabe, M. (2016). KEGG as a reference resource for gene and protein annotation. Nucleic acids Res. 44, D457–D462. doi:10.1093/nar/gkv1070
Kikuchi, K., and Hamaguchi, S. (2013). Novel sex-determining genes in fish and sex chromosome evolution. Dev. Dyn. 242, 339–353. doi:10.1002/dvdy.23927
Kim, D., Pertea, G., Trapnell, C., Pimentel, H., Kelley, R., and Salzberg, S. L. (2013). TopHat2: Accurate alignment of transcriptomes in the presence of insertions, deletions and gene fusions. Genome Biol. 14, R36–R13. doi:10.1186/gb-2013-14-4-r36
Kobayashi, T., and Nagahama, Y. (2009). Molecular aspects of gonadal differentiation in a teleost fish, the Nile tilapia. Sex. Dev. 3, 108–117. doi:10.1159/000223076
Kobayashi, Y., Horiguchi, R., Nozu, R., and Nakamura, M. (2010). Expression and localization of forkhead transcriptional factor 2 (Foxl2) in the gonads of protogynous wrasse, Halichoeres trimaculatus. Biol. sex Differ. 1, 3–9. doi:10.1186/2042-6410-1-3
Kuang, J., Chen, G., Ma, Q., Mao, F., Zhou, Q., Huang, J., et al. (2021). Histological observation on gonadal differentiation and first annual gonadal development of cobia (Rachycentron canadum). Haiyang Xuebao 43, 128. doi:10.12284/hyxb2021126
Lefebvre, L. S., and Denson, M. R. (2012). Inshore spawning of cobia (Rachycentron canadum) in South Carolina. Fish. Bull. 110, 397–412.
Levy, T., Rosen, O., Eilam, B., Azulay, D., Zohar, I., Aflalo, E. D., et al. (2017). All-female monosex culture in the freshwater prawn Macrobrachium rosenbergii–A comparative large-scale field study. Aquaculture 479, 857–862. doi:10.1016/j.aquaculture.2017.07.039
Li, J., Liu, Q., Wang, D., Zhou, L., Sakai, F., and Nagahama, Y. (2012). Molecular cloning and identification of two Wnt4 genes from the medaka (Oryzias latipes). Acta Hydrobiol. Sin. 36, 983–986. doi:10.3724/sp.j.1035.2012.00983
Li, X., Yu, H., Wang, Y., Liu, X., Liu, Y., Qu, J., et al. (2018). Roles of two Sox9 genes during gonadal development in Japanese flounder: Sex differentiation, spermatogenesis and gonadal function maintenance. Int. J. Mol. Sci. 19, 512. doi:10.3390/ijms19020512
Li, S., Lin, G., Fang, W., Huang, P., Gao, D., Huang, J., et al. (2020). Gonadal transcriptome analysis of sex-related genes in the protandrous yellowfin seabream (Acanthopagrus latus). Front. Genet. 11, 709. doi:10.3389/fgene.2020.00709
Lin, R., Wang, L., Zhao, Y., Gao, J., and Chen, Z. (2017). Gonad transcriptome of discus fish (Symphysodon haraldi) and discovery of sex-related genes. Aquac. Res. 48, 5993–6000. doi:10.1111/are.13424
Lin, X., Zhou, D., Zhang, X., Li, G., Zhang, Y., Huang, C., et al. (2021). A first insight into the gonad transcriptome of Hong Kong Catfish (Clarias fuscus). Animals 11, 1131. doi:10.3390/ani11041131
Litscher, E. S., and Wassarman, P. M. (2018). The fish egg's zona pellucida. Curr. Top. Dev. Biol. 130, 275–305. doi:10.1016/bs.ctdb.2018.01.002
Liu, S., Govoroun, M., D'cotta, H., Ricordel, M. J., Lareyre, J. J., Mcmeel, O. M., et al. (2000). Expression of cytochrome P45011β (11β-hydroxylase) gene during gonadal sex differentiation and spermatogenesis in rainbow trout, Oncorhynchus mykiss. J. steroid Biochem. Mol. Biol. 75, 291–298. doi:10.1016/s0960-0760(00)00186-2
Livak, K. J., and Schmittgen, T. D. (2001). Analysis of relative gene expression data using real-time quantitative PCR and the 2(-Delta Delta C(T)) Method. methods 25, 402–408. doi:10.1006/meth.2001.1262
Lobo, I. K. C., Do Nascimento, A. R., Yamagishi, M. E. B., Guiguen, Y., Da Silva, G. F., Severac, D., et al. (2020). Transcriptome of tambaqui (Colossoma macropomum) during gonad differentiation: Different molecular signals leading to sex identity. Genomics 112, 2478–2488. doi:10.1016/j.ygeno.2020.01.022
Ma, Q., Kuang, J., Chen, G., Zhang, J., Huang, J., Mao, F., et al. (2022). Cloning and expression profiling of the gene vasa during first annual gonadal development of cobia (Rachycentron canadum). Fishes 7, 60. doi:10.3390/fishes7020060
Maekawa, S., Wang, P. C., and Chen, S. C. (2019). Differential expression of immune-related genes in head kidney and spleen of cobia (Rachycentron canadum) having Streptococcus dysgalactiae infection. Fish shellfish Immunol. 92, 842–850. doi:10.1016/j.fsi.2019.07.009
Mao, X., Cai, T., Olyarchuk, J. G., and Wei, L. (2005). Automated genome annotation and pathway identification using the KEGG Orthology (KO) as a controlled vocabulary. Bioinformatics 21, 3787–3793. doi:10.1093/bioinformatics/bti430
Martínez, P., Robledo, D., Taboada, X., Blanco, A., Gómez-Tato, A., Álvarez-Blázquez, B., et al. (2019). Multiple evidences suggest sox2 as the main driver of a young and complex sex determining ZW/ZZ system in turbot (Scophthalmus maximus). BioRxiv, 834556. doi:10.1101/834556
Matsuda, M., Nagahama, Y., Shinomiya, A., Sato, T., Matsuda, C., Kobayashi, T., et al. (2002). DMY is a Y-specific DM-domain gene required for male development in the medaka fish. Nature 417, 559–563. doi:10.1038/nature751
Mei, J., and Gui, J. F. (2015). Genetic basis and biotechnological manipulation of sexual dimorphism and sex determination in fish. Sci. China Life Sci. 58, 124–136. doi:10.1007/s11427-014-4797-9
Meng, L., Yu, H., Ni, F., Niu, J., Liu, X., and Wang, X. (2020). Roles of two cyp11 genes in sex hormone biosynthesis in Japanese flounder (Paralichthys olivaceus). Mol. reproduction Dev. 87, 53–65. doi:10.1002/mrd.23301
Molina, W. F., Benetti, D. D., Fiorentino, J. N., Lima-Filho, P. a. D., Alencar, C. E. R. D., Costa, G. W. W. F. D., et al. (2018). Early sex shape dimorphism (SShD) in Rachycentron canadum (Linnaeus, 1766) and its applications for monosex culture. Aquaculture 495, 320–327. doi:10.1016/j.aquaculture.2018.05.056
Moniruzzaman, M., Uddin, K. B., Basak, S., Mahmud, Y., Zaher, M., and Bai, S. C. (2015). Effects of stocking density on growth, body composition, yield and economic returns of monosex tilapia (Oreochromis niloticus L.) under cage culture system in Kaptai Lake of Bangladesh. J. Aquac. Res. Dev. 6, 1. doi:10.4172/2155-9546.1000357
Nair, C. M., Salin, K. R., Raju, M. S., and Sebastian, M. (2006). Economic analysis of monosex culture of giant freshwater prawn (Macrobrachium rosenbergii de man): A case study. Aquac. Res. 37, 949–954. doi:10.1111/j.1365-2109.2006.01521.x
Nakamoto, M., Matsuda, M., Wang, D.-S., Nagahama, Y., and Shibata, N. (2006). Molecular cloning and analysis of gonadal expression of Foxl2 in the medaka, Oryzias latipes. Biochem. biophysical Res. Commun. 344, 353–361. doi:10.1016/j.bbrc.2006.03.137
Nakamoto, M., Muramatsu, S., Yoshida, S., Matsuda, M., Nagahama, Y., and Shibata, N. (2009). Gonadal sex differentiation and expression of Sox9a2, Dmrt1, and Foxl2 in Oryzias luzonensis. genesis 47, 289–299. doi:10.1002/dvg.20498
Nanda, I., Kondo, M., Hornung, U., Asakawa, S., Winkler, C., Shimizu, A., et al. (2002). A duplicated copy of DMRT1 in the sex-determining region of the Y chromosome of the medaka, Oryzias latipes. Proc. Natl. Acad. Sci. 99, 11778–11783. doi:10.1073/pnas.182314699
Nicol, B., Guerin, A., Fostier, A., and Guiguen, Y. (2012). Ovary-predominant wnt4 expression during gonadal differentiation is not conserved in the rainbow trout (Oncorhynchus mykiss). Mol. reproduction Dev. 79, 51–63. doi:10.1002/mrd.21404
Nyuji, M., Hongo, Y., Yoneda, M., and Nakamura, M. (2020). Transcriptome characterization of BPG axis and expression profiles of ovarian steroidogenesis-related genes in the Japanese sardine. BMC genomics 21, 1–18. doi:10.1186/s12864-020-07080-1
Raghuveer, K., and Senthilkumaran, B. (2010). Isolation of sox9 duplicates in catfish: Localization, differential expression pattern during gonadal development and recrudescence, and hCG-induced up-regulation of sox9 in testicular slices. Reprod. Camb. Engl. 140, 477–487. doi:10.1530/REP-10-0200
Rozen, S., and Skaletsky, H. (2000). “Primer3 on the WWW for general users and for biologist programmers,” in Bioinformatics methods and protocols (Springer), 365–386.
Shaffer, R. V., and Nakamura, E. V. (1989). Synopsis of biological data on the cobia Rachycentron canadum (Piscies: Rachycentridae). Washington, DC: U. D. o. Commerce FAO Fisheries synopsis 153: 21.
Scornaienchi, M. L., Thornton, C., Willett, K. L., and Wilson, J. Y. (2010). Cytochrome P450-mediated 17β-estradiol metabolism in zebrafish (Danio rerio). J. Endocrinol. 206, 317–325. doi:10.1677/JOE-10-0075
Sheng, Y., Chen, B., Zhang, L., Luo, M., Cheng, H., and Zhou, R. (2014). Identification of Dmrt genes and their up-regulation during gonad transformation in the swamp eel (Monopterus albus). Mol. Biol. Rep. 41, 1237–1245. doi:10.1007/s11033-013-2968-6
Socorro, S., Martins, R. S., Deloffre, L., Mylonas, C. C., and Canario, A. V. (2007). A cDNA for European sea bass (Dicentrachus labrax) 11β-hydroxylase: Gene expression during the thermosensitive period and gonadogenesis. General Comp. Endocrinol. 150, 164–173. doi:10.1016/j.ygcen.2006.07.018
Stévant, I., and Nef, S. (2019). Genetic control of gonadal sex determination and development. Trends Genet. 35, 346–358. doi:10.1016/j.tig.2019.02.004
Stieglitz, J. D., Benetti, D. D., Hoenig, R. H., Sardenberg, B., Welch, A. W., and Miralao, S. (2012). Environmentally conditioned, year-round volitional spawning of cobia (Rachycentron canadum) in broodstock maturation systems. Aquac. Res. 43, 1557–1566. doi:10.1111/j.1365-2109.2011.02961.x
Su, L., Zhou, F., Ding, Z., Gao, Z., Wen, J., Wei, W., et al. (2015). Transcriptional variants of Dmrt1 and expression of four Dmrt genes in the blunt snout bream, Megalobrama amblycephala. Gene 573, 205–215. doi:10.1016/j.gene.2015.07.044
Sun, L. X., Teng, J., Zhao, Y., Li, N., Wang, H., and Ji, X. S. (2018). Gonad transcriptome analysis of high-temperature-treated females and high-temperature-induced sex-reversed neomales in nile tilapia. Int. J. Mol. Sci. 19, 689. doi:10.3390/ijms19030689
Takehana, Y., Matsuda, M., Myosho, T., Suster, M. L., Kawakami, K., Shin, T., et al. (2014). Co-option of Sox3 as the male-determining factor on the Y chromosome in the fish Oryzias dancena. Nat. Commun. 5, 1–10. doi:10.1038/ncomms5157
Tao, W., Yuan, J., Zhou, L., Sun, L., Sun, Y., Yang, S., et al. (2013). Characterization of gonadal transcriptomes from Nile tilapia (Oreochromis niloticus) reveals differentially expressed genes. PloS one 8, e63604. doi:10.1371/journal.pone.0063604
Tevosian, S. G., and Manuylov, N. L. (2008). To β or not to β: Canonical β-catenin signaling pathway and ovarian development. Dev. Dyn. 237, 3672–3680. an official publication of the American Association of Anatomists. doi:10.1002/dvdy.21784
Tran, H. B., Lee, Y. H., Guo, J. J., and Cheng, T. C. (2018). De novo transcriptome analysis of immune response on cobia (Rachycentron canadum) infected with Photobacterium damselae subsp. piscicida revealed inhibition of complement components and involvement of MyD88-independent pathway. Fish shellfish Immunol. 77, 120–130. doi:10.1016/j.fsi.2018.03.041
Tveterås, R., Jory, D. E., and Nystoyl, R. (2019). Goal 2019: Global finfish production review and forecast. Available online: globalseafood.org/advocate/goal-2019-global-finfish-production-review-and-forecast/ (accessed onNovember16, 2022).
Vainio, S., Heikkilä, M., Kispert, A., Chin, N., and Mcmahon, A. P. (1999). Female development in mammals is regulated by Wnt-4 signalling. Nature 397, 405–409. doi:10.1038/17068
Vandesompele, J., De Preter, K., Pattyn, F., Poppe, B., Van Roy, N., De Paepe, A., et al. (2002). Accurate normalization of real-time quantitative RT-PCR data by geometric averaging of multiple internal control genes. Genome Biol. 3 (7), 1–12.
Vidotto, M., Grapputo, A., Boscari, E., Barbisan, F., Coppe, A., Grandi, G., et al. (2013). Transcriptome sequencing and de novo annotation of the critically endangered Adriatic sturgeon. BMC genomics 14, 407–416. doi:10.1186/1471-2164-14-407
Wang, X., and Orban, L. (2007). Anti-Müllerian hormone and 11β-hydroxylase show reciprocal expression to that of aromatase in the transforming gonad of zebrafish males. Dev. Dyn. 236, 1329–1338. an official publication of the American Association of Anatomists. doi:10.1002/dvdy.21129
Wang, P. P., Wang, J. H., Yan, Z. P., Hu, M., Lau, G. K., Fan, S. T., et al. (2004). Expression of hepatocyte-like phenotypes in bone marrow stromal cells after HGF induction. Biochem. biophysical Res. Commun. 320, 712–716. doi:10.1016/j.bbrc.2004.05.213
Wang, Z., Gerstein, M., and Snyder, M. (2009). RNA-seq: A revolutionary tool for transcriptomics. Nat. Rev. Genet. 10, 57–63. doi:10.1038/nrg2484
Webster, K. A., Schach, U., Ordaz, A., Steinfeld, J. S., Draper, B. W., and Siegfried, K. R. (2017). Dmrt1 is necessary for male sexual development in zebrafish. Dev. Biol. 422, 33–46. doi:10.1016/j.ydbio.2016.12.008
Wu, G. C., and Chang, C. F. (2009). Wnt4 is associated with the development of ovarian tissue in the protandrous black porgy, Acanthopagrus schlegeli. Biol. reproduction 81, 1073–1082. doi:10.1095/biolreprod.109.077362
Yamaguchi, T., Yamaguchi, S., Hirai, T., and Kitano, T. (2007). Follicle-stimulating hormone signaling and Foxl2 are involved in transcriptional regulation of aromatase gene during gonadal sex differentiation in Japanese flounder, Paralichthys olivaceus. Biochem. biophysical Res. Commun. 359, 935–940. doi:10.1016/j.bbrc.2007.05.208
Yan, H., Shen, X., Cui, X., Wu, Y., Wang, L., Zhang, L., et al. (2018). Identification of genes involved in gonadal sex differentiation and the dimorphic expression pattern in Takifugu rubripes gonad at the early stage of sex differentiation. Fish physiology Biochem. 44, 1275–1290. doi:10.1007/s10695-018-0519-8
Yano, A., Guyomard, R., Nicol, B., Jouanno, E., Quillet, E., Klopp, C., et al. (2012). An immune-related gene evolved into the master sex-determining gene in rainbow trout, Oncorhynchus mykiss. Curr. Biol. 22, 1423–1428. doi:10.1016/j.cub.2012.05.045
Young, M. D., Wakefield, M. J., Smyth, G. K., and Oshlack, A. (2010). Gene ontology analysis for RNA-seq: Accounting for selection bias. Genome Biol. 11, R14–R12. doi:10.1186/gb-2010-11-2-r14
Yuan, Z., Shen, X., Yan, H., Jiang, J., Liu, B., Zhang, L., et al. (2021). Effects of the thyroid endocrine system on gonadal sex ratios and sex-related gene expression in the pufferfish Takifugu rubripes. Front. Endocrinol. 12, 674954. doi:10.3389/fendo.2021.674954
Zhang, X., Li, M., Ma, H., Liu, X., Shi, H., Li, M., et al. (2017). Mutation of foxl2 or cyp19a1a results in female to male sex reversal in XX Nile tilapia. Endocrinology 158, 2634–2647. doi:10.1210/en.2017-00127
Keywords: cobia, gonad transcriptome, Rachycentron canadum, reproductive related pathways, sexual size dimorphism, sex-biased genes
Citation: Shen X, Yáñez JM, Bastos Gomes G, Poon ZWJ, Foster D, Alarcon JF and Domingos JA (2023) Comparative gonad transcriptome analysis in cobia (Rachycentron canadum). Front. Genet. 14:1128943. doi: 10.3389/fgene.2023.1128943
Received: 21 December 2022; Accepted: 24 March 2023;
Published: 06 April 2023.
Edited by:
Pouya Zamani, Bu-Ali Sina University, IranReviewed by:
Zhong-duo Wang, Guangdong Ocean University, ChinaXinping Zhu, Pearl River Fisheries Research Institute, Chinese Academy of Fishery Sciences, China
Copyright © 2023 Shen, Yáñez, Bastos Gomes, Poon, Foster, Alarcon and Domingos. This is an open-access article distributed under the terms of the Creative Commons Attribution License (CC BY). The use, distribution or reproduction in other forums is permitted, provided the original author(s) and the copyright owner(s) are credited and that the original publication in this journal is cited, in accordance with accepted academic practice. No use, distribution or reproduction is permitted which does not comply with these terms.
*Correspondence: Xueyan Shen, eHVleWFuLnNoZW5AamN1LmVkdS5hdQ==; Jose A. Domingos, am9zZS5kb21pbmdvczFAamN1LmVkdS5hdQ==