- 1Anhui Province Key Laboratory of Embryo Development and Reproductive Regulation, Anhui Province Key Laboratory of Environmental Hormone and Reproduction, School of Biological and Food Engineering, Fuyang Normal University, Fuyang, China
- 2Shaanxi Key Laboratory of Molecular Biology for Agriculture, College of Animal Science and Technology, Northwest A&F University, Yangling, Shaanxi, China
Introduction: The number of litters is an important reproductive trait, which is one of the main indicators reflecting the production level and economic benefit of the pig farm. As an important reproductive organ of female mammals, the ovary is controlled by a complex transcription network of coding and non-coding genes to undergo a series of biological processes during each estrus cycle, thereby regulating the reproductive capacity of the sow. However, these molecular regulation mechanisms affecting sow litter size are still unclear.
Methods: Regarding the non-coding molecular regulatory mechanisms in ovarian function with smaller and larger litter size (SLS and LLS), we investigated the expression profile of lncRNA in pig SLS and LLS samples. Total RNAs from porcine ovaries were used to construct libraries using Ribo-Zero RNA sequencing method.
Results: Here we profiled the expression of lncRNA in porcine ovaries with SLS and LLS, and identified a total of 3,556 lncRNA candidates, of which 96 were upregulated lncRNA and 206 were downregulated lncRNA when comparing LLS to SLS. In addition, a competitive endogenous RNA (ceRNA) network was constructed, and it was found that lncRNAs LOC100513133 and LOC102168075 may serve as ceRNAs containing potential binding sites for miR-26b, let-7g and miR-125b.
Discussion: These results demonstrate that lncRNAs may play roles in modulating porcine litter size.
Introduction
The number of litters is an important reproductive trait and one of the main indicators reflecting the production level and economic benefits of pig farms (Vázquez-Gómez et al., 2020; Zheng et al., 2020). The increasing number of litters and shorter delivery intervals to obtain economic benefits has always been a global goal for sow feeding (Hidalgo et al., 2020; Schneider et al., 2015). As an important reproductive organ of female mammals, the ovary is controlled by a complex transcription network of coding and non-coding genes (Pan et al., 2014; Yin et al., 2020; Du et al., 2019).
Non-coding RNA, for example, long non-coding RNAs (lncRNAs) are involved in ovarian function regulatory network and are important competing endogenous RNAs (ce RNA) (Zhang et al., 2018; Chen, 2016). LncRNA plays an indispensable role in the process of transcription and post-transcriptional regulation of gene activity through cis and trans regulation (Liao et al., 2020; Liu B. et al., 2020; Meng et al., 2020), such as functioning as a molecular scaffold in the assembly of transcription complexes (Ponzio et al., 2017; Hou et al., 2017), or regulating RNA-protein interactions and alternative splicing (Liu S. et al., 2020; Meng et al., 2020), or acting as a microRNA (miRNA) sponge and relieving miRNA inhibition of post-transcriptional expression of their target genes (Wei et al., 2018). In the nucleus, X-inactive-specific transcript (Xist) is strictly localized within chromosome boundary and triggers chromosome remodeling to achieve stable silencing with relatively few genes remaining active (Zhang et al., 2019). Non-coding RNA SRA was found to be immunoprecipitated with MyoD, thereby affecting muscle differentiation (Hubé et al., 2011). In the cytoplasm, lncMD had been demonstrated to function as competing endogenous RNAs (ceRNAs) to bind miRNAs and regulate cattle myoblast differentiation (Sun et al., 2016).
With the rapid development of high-throughput sequencing technology and bioinformatics, lncRNAs have been found in various tissues of different species, and the dynamic expression patterns of lncRNAs under different developmental stages and physiological conditions have been revealed. They emerge as key regulators of major biological processes impacting proliferation, differentiation, and apoptosis in every branch of life, including ovarian function (Wang et al., 2019). Anna found that some differentially expressed lncRNAs were involved in spermatogenesis in pig testis tissues by transcriptome analysis (Esteve-Codina et al., 2011). Ran performed RNA sequencing (RNA-seq) analysis of pig mature and immature testicular tissues (Ran et al., 2016). Wang performed RNA-seq on endometrial tissues of pigs during pregnancy and non-pregnancy, and identified a total of 34 lncRNAs with significant differences in expression (Wang et al., 2017). In this study, we characterized lncRNA expression profiles in six sow ovaries with smaller and larger litter size (SLS and LLS) using Ribo-Zero RNA-seq method combined with bioinformatics tools, thus uncovered novel molecules in the regulatory network of swine reproduction.
Materials and methods
Sample preparation
In China, PRRS and ring disease are one of the most important factors affecting litter size. Our experiment is to guide the pig breeding industry, so we chose sows without these two diseases. In addition, the sows we selected are of the same age, body condition, and feed with the same nutritional level. Most importantly, they have no history of reproductive diseases. We collected the litter size records of the Dabai Landrace binary cross sows from the Dahua breeding farm (Guangxi Hanshiwei Food Co., Ltd., from 2016 to 2018, a total of 8,557 litters), and used SPSS25.0 to perform a significance test on these data. After normal distribution conversion and inspection, it is found that the total litter size (12.9 ± 2.17) approximately obeys the normal distribution. The critical value of 15% right tail probability is 14.7 heads per litter, and the critical value of 15% left tail probability is 9.3 heads per litter. Ovarian tissues of pig under follicles of the same diameter were taken when static reflex occurred in pigs of the same parity.
Animal welfare and research plan were approved by Animal Care Committee of the Guangxi University. The ovaries of sows (Large White × Danish Landrace binary hybrid pig) were collected from a commercial pig farm (Nanning, Guangxi, China) 4 days after the fourth delivery. Six ovary tissues were chosen to perform RNA-Seq from pig with small (SLS, 8.48 ± 0.53/litter) and large (LLS, 16.19 ± 0.43/litter) litter size after the fourth delivery, respectively. The sample was were obtained and snap-frozen in liquid nitrogen.
Library preparation and illumina sequencing
We subjected the extracted RNA from tissue samples of six pig ovaries (three pig ovaries from pigs with small litter size; three pig ovaries from pigs with large litter size) to agarose gel electrophoresis, and then RNA concentrations were quantified using Agilent 2100 Bioanalyzer and NanoDrop spectrophotometer. Ribosomal RNA was removed using Ribo-Zero™ rRNA Removal Kit (Epicentre, Madison, WI, United States). Library preparation and sequencing analysis were described as previous study (Shen et al., 2019). The raw data files were cleaned up for quality control through Trim Galore and then mapped to the pig reference genome (Sscrofa 11.1) from UCSC (Kim et al., 2013). CuffLinks were used to linear transcripts assembly and abundance assessment, then we identified reads from fusion transcripts that did not match the linear RNA sequence. The lncRNAs ≤100 kb in length, with at least two support sequences, and sequences with no more than two mismatches were retained for further analysis.
Gene ontology and pathway analysis
Gene Ontology analysis (GO; http://www.geneontology.org) was used to explore potential functions of differentially expressed mRNA and lncRNA. GO terminology analyzes information about biological processes involving genes, and cellular components or metabolic pathways, and highlights molecular functions. In addition, we also performed the Kyoto Encyclopedia of Genes and Genomes pathway analysis (KEGG; http://www.kegg.jp), using DAVID to gain insight into the interaction and reaction network of differentially expressed lncRNA molecules. The -log10 P value represents a significant enrichment of a given GO term or KEGG pathway between upregulated and downregulated entities.
CeRNA network construction of lnc RNA
Bnd lncRNA sequences, an interaction regulation network was constructed between mRNA-miRNA-lncRNA. The RNAhybrid (https://bibiserv.cebitec.uni-bielefeld.de/rnahybrid/) and TargetScan (http://www.targetscan.org/) were used to predict the interactions of miRNA-mRNA and miRNA-circRNA. The correlation between the expression levels of lncRNA and miRNA was calculated with SPSS Pearson correlation assay.
CDNA synthesis, and real-time qPCR of lnc RNA
Invitrogen Trizol reagent (Thermo, MA, United States) was used to extract total RNA from porcine ovarian tissue, and PrimeScript™ RT kit (TaKaRa, Dalian, China) with gDNA eraser was used to reverse transcription of total RNA while removing genomic DNA. Real-time quantitative PCR (qPCR; 95°C 30 s, 95°C 5 s, 60°C 30 s for 40 cycles, following 70°C 10 min for elongation) of lnc RNA was performed using SYBR Green kit (TaKaRa, Dalian, China) in triplicate on a Bio-Rad CF96 system (Bio-Rad, United States), and the data was normalized using β-actin. The primers are listed in Supplementary Table S1.
Statistical analyses
The data showed mean ± SEM. P-values were calculated by a Student’s t-test. P < 0.05 was considered as significant difference.
Results
Ribo-zero RNA-Seq of pig ovary
In this study, we selected six ovarian samples to perform Ribo-Zero RNA-Seq from pig with small (SLS, 8.48 ± 0.53/litter) and large (LLS, 16.19 ± 0.43/litter) litter size after the fourth delivery, respectively (Supplementary Table S2). The significance of large litter size was higher than that of small litter size (P < 0.001). We used Pfam and Cpat databases to filter, and analyzed the protein coding ability predictions of new lncRNAs through CPC and CNCI tools, and identified 3,556 potential lncRNA transcripts (Figure 1A; Supplementary Table S3). As shown in Figure 1B, Pearson correlation analysis showed that there is a good correlation between SLS and LLS ovarian samples. We obtained 75–83 and 78–85 million unique mapped clean reads from the SLS and LLS sample libraries (Table 1), and found that 69.7% of the reads are located in the exon and sequence coding for aminoacids in protein (CDS) regions, while the distribution in the non-coding (intergenic, intronic, UTR, transcription initiation site (TSS), and transcription end site (TES) regions markedly reduced (30.3%; Figure 1C).
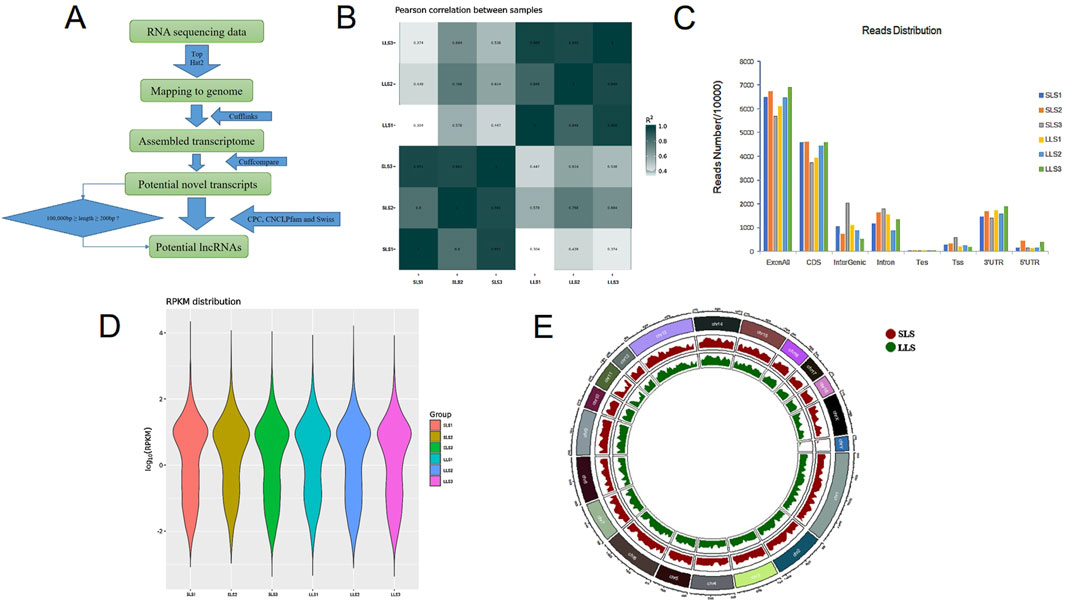
Figure 1. Analysis of ovarian transcriptome sequencing in large and small litter size pigs. (A) Identification process of lncRNAs in this study. (B) Principal component analysis (PCA) was used to analyze the pearson correlation between small litter size (SLS) and large litter size (LLS) of transcriptome data, and the two groups could be distinguished. n = 3. (C) The distribution of reads data in different regions of the genome. The SLS and LLS mean small litter size and large litter size, respectively. (D) The distribution of RNA expression (RPKM) in each sample. The SLS and LLS mean small litter size and large litter size, respectively. (E) The distribution of reads data on each chromosome in pigs. Red and green represent chromosomes of small litter size and large litter size of pig, respectively.
As shown in Figure 1D, the average expression level of the identified RNA including lncRNA and mRNA is 12.97 (FPKM), showing a lower expression level. In addition, we found that every chromosome could produce lncRNA, and the longer the chromosome, the more likely it is to produce lncRNA (Figure 1E).
Differentially expressed mRNAs
In this study, 16,428 genes were detected (Supplementary Table S4), and 2,392 unigenes were significantly differently expressed at the LLS and SLS ovarian samples (P < 0.05; Figure 2A); all differentially expressed genes (DEGs) were shown in Supplementary Table S5. In order to verify the accuracy of RNA-seq, we randomly selected 8 genes (4 upregulated genes and 4 downregulated genes) for qPCR detection, and compared the qPCR data with the sequencing data (Table 2). It can be found that the qPCR results of the selected DEGs are basically similar to the RNA-seq results, indicating that the sequencing data has high accuracy.
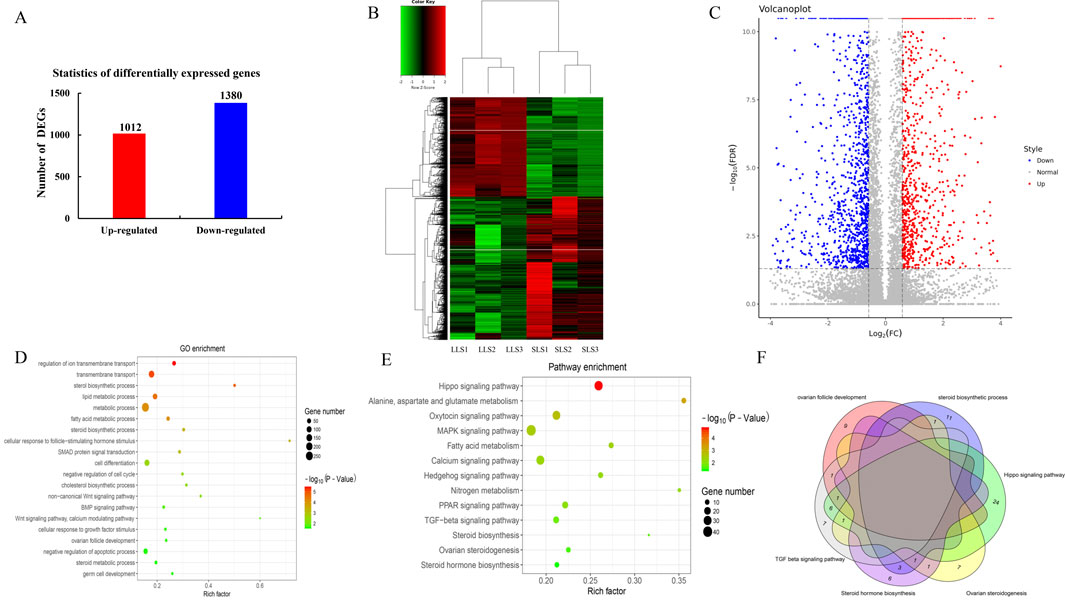
Figure 2. Analysis of differentially expressed genes and functional enrichment in ovarian transcriptome. (A) There were 1,012 upregulated genes and 1,380 downregulated genes in differentially expressed genes. (B) Heat map of differentially expressed genes between small litter size and large litter size. DEG expression levels are represented as FPKM-normalized log2-transformed counts. (C) Volcano map of differentially expressed genes, where red dots indicate upregulated genes, blue dots indicate downregulated genes, and gray dots indicate genes with no significant difference. (D, E) GO and KEGG signal pathway enrichment analysis of differentially expressed genes. The size of the dots represents the number of genes enriched, and the color of the dots represents the P value. GO, Gene Ontology; KEGG, Kyoto Encyclopedia of Genes and Genomes. (F) GO and KEGG analyses were combined and shown as Venn diagram. GO, Gene Ontology; KEGG, Kyoto Encyclopedia of Genes and Genomes.
As shown in Figure 2B, the cluster heat map of DEGs and the Pearson correlation in each sample were displayed. To further explore the potential functions of DEGs, we used volcano plots to compare their transcript expression abundance in SLS and LLS ovarian samples (Figure 2C).
To explore the possible biological functions of DEGs identified in SLS and LLS porcine ovarian tissues, GO analysis was performed (Figure 2D). The results showed that these DEGs were significantly enriched by 20 biological processes, including steroid biosynthesis process, lipid metabolism process, ovarian follicle development, granular cell differentiation, negative regulation of apoptosis process, and cell response to follicle stimulating hormone stimulation.
In order to better understand the molecular regulation of DEGs, we performed KEGG pathway analysis (Figure 2E). It showed that a total of 50 pathways are enriched, among which the most significant is the hippo signaling pathway (path: 04390), with 32 annotation genes. From the three enrichment pathways of steroid biosynthesis (path: 00100), ovarian steroid production (path: 04913) and steroid hormone biosynthesis (path: 00140), it could be seen that the synthesis and metabolism of steroids play an important role in the ovaries of pigs. In order to accurately obtain the key genes that may affect the pig litter size, we combined GO analysis and KEGG pathway analysis. The results showed that 15 genes may be candidate genes affecting piglet size traits. Among them, INHBA, BMP4 are related to ovarian follicle development, CAPN5 and FOXL2 are related to the regulation of granulosa cell differentiation; CYP21A2, STAR, HSD17B2, HSD17B8 and HSD17B12 are involved in steroid biosynthesis; In addition, the key genes TGFBR1 and TGFB3 in TGF signaling pathway are also significantly different (Figure 2F). It is worth noting that TGF signaling pathway is highly related to the regulation of reproductive performance.
Differentially expressed lncRNAs
From the fold change and the adjusted level of significance, a total of 302 differentially expressed lncRNAs were screened (P < 0.05; Figure 3A; Supplementary Table S6). We found that 206 lncRNAs were downregulated and 96 lncRNAs were upregulated (Figure 3B), and LLS group (FPKM = 346.38) had an obvious trend of high expression of lncRNA when comparing SLS group (FPKM = 256.71). It is worth mentioning that ALAS1 (FPKM = 32,089.67) and LOC100513133 (FPKM = 10,768) have the highest expression levels among all upregulated and downregulated lncRNAs, respectively. In order to better understand the expression changes and potential functions of lncRNA, a volcano plot is shown in Figure 3C.
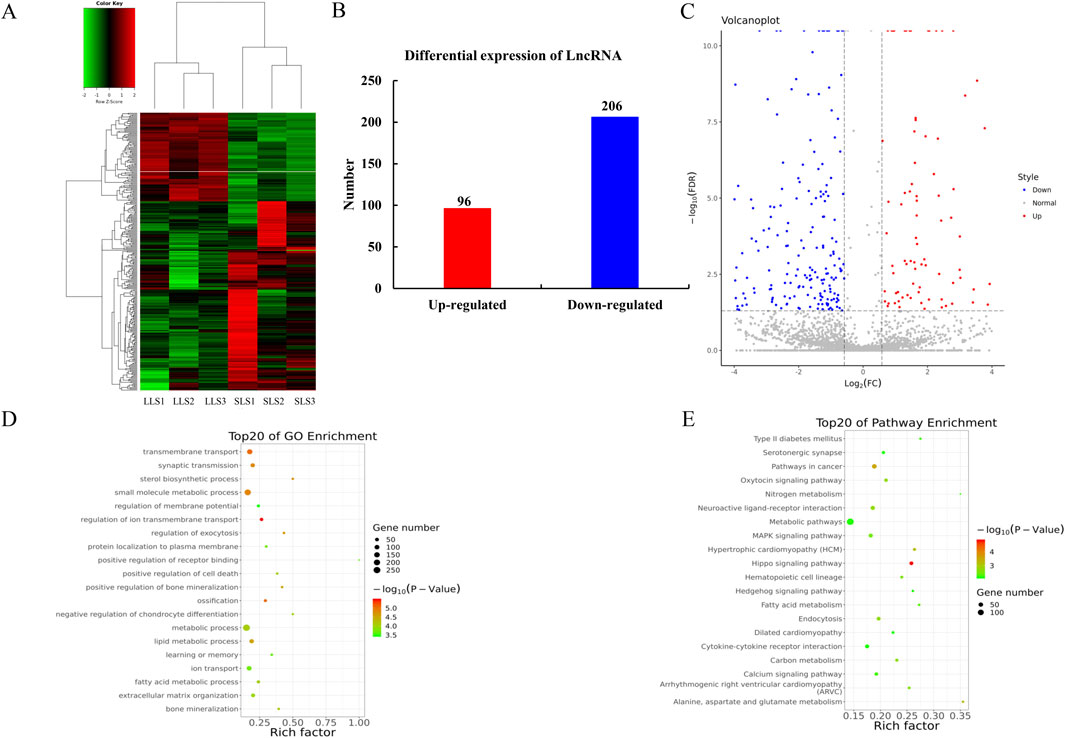
Figure 3. Differential expression and enrichment analysis of lncRNAs in ovarian transcriptome. (A) A heat map was drawn for 302 differentially expressed lncRNAs. DEG expression levels are represented as FPKM-normalized log2-transformed counts. (B) Among 302 differentially expressed lncRNAs, 97 were upregulated and 205 were downregulated. The SLS and LLS mean small litter size and large litter size, respectively. (C) In the volcano map of lncRNAs, red dots indicate upregulated lncRNAs, blue dots indicate downregulated lncRNAs, and gray dots indicate lncRNAs with no significant difference. (D, E) Go and KEGG enrichment analysis of differentially expressed lncRNAs target genes was performed. The results showed that the top 20 were significantly enriched. GO, Gene Ontology; KEGG, Kyoto Encyclopedia of Genes and Genomes.
LncRNA regulates the transcription and translation of coding genes in cis and trans regulation: when the role of lncRNA is limited to the same chromosome (for example, acting as a molecular scaffold), it performs cis regulation; when it affects gene expression on other chromosomes, trans regulation plays a role (for example, acting as a miRNA sponge). As shown in Figures 3D, E, the first 20 enriched GO terms and KEGG pathways are shown. Interestingly, metabolic processes in both GO and KEGG analyses had the most cis and trans’ target gene clusters, indicating that metabolic processes may play an important role in ovarian function.
In order to verify the accuracy of sequencing data, we randomly selected 12 genes (6 upregulated lncRNAs and 6 downregulated lncRNAs) for qPCR detection, and compared the qPCR data with the sequencing data (Figure 4; Supplementary Table S7). It can be found that the qPCR results of the selected differentially expressed lncRNAs are basically similar to the RNA-seq results, indicating that the lncRNA-Seq data are highly accuracy.
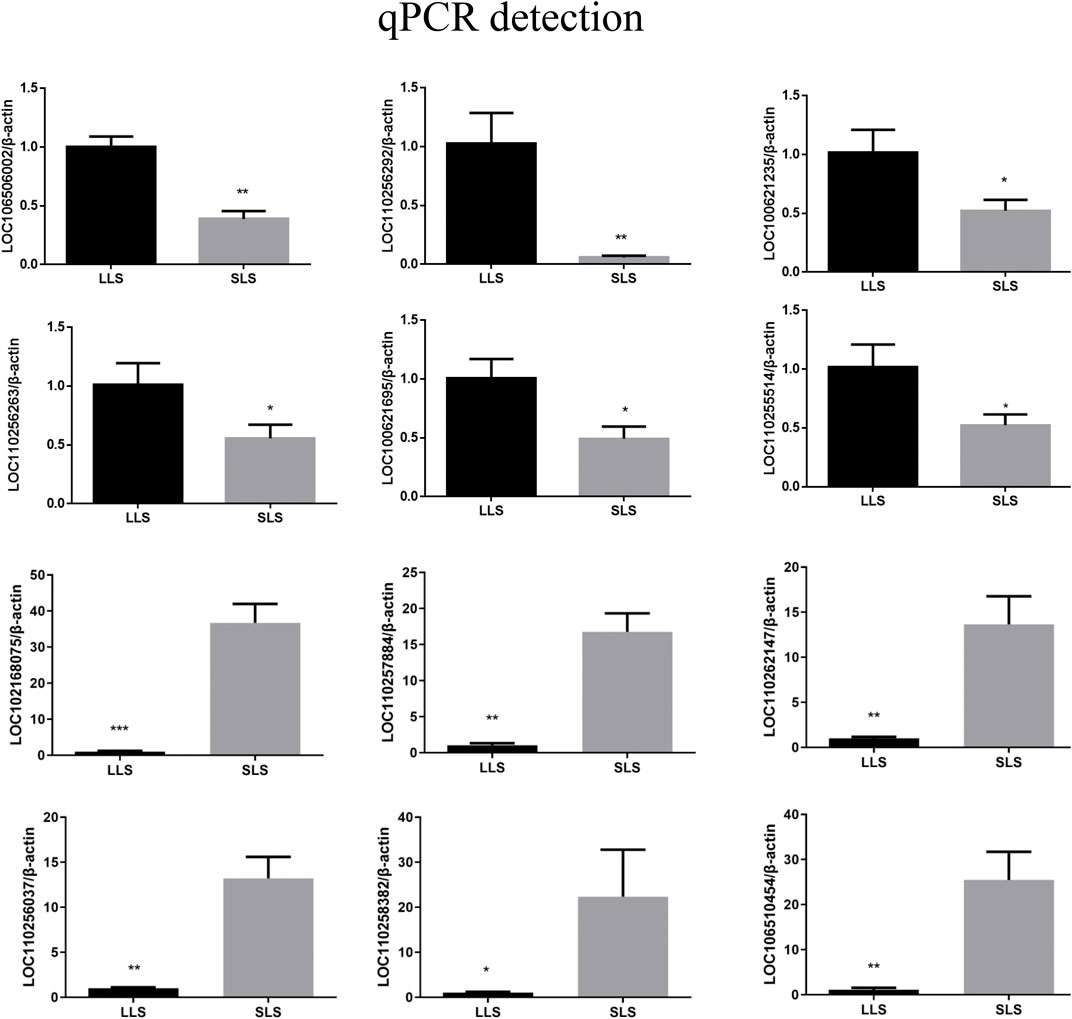
Figure 4. The expression level of lncRNAs was verified by qPCR assay. The transcriptomes differentially expressed lncRNAs, which were verified by qPCR. The internal reference gene was β-actin, with three biological replicates in each group. The SLS and LLS mean small litter size and large litter size, respectively.
CeRNAs networks
lncRNA can act as a molecular sponge of miRNA to sequester miRNA from its target gene, that is, miRNA is the common target of lncRNA and mRNA. We selected 9 miRNAs, 9 lncRNAs and 342 mRNAs related to ovarian development to construct an mRNA-miRNA-lncRNA (ceRNA) network (Supplementary Figure S1). For example, LOC100513133 and LOC102168075 have multiple binding sites for microRNAs related to ovarian development, such as miR-26b (Liu et al., 2016; Lin et al., 2012), let-7g (Zhou et al., 2016; Cao et al., 2015a) and miR-125b (Zhang D. et al., 2020; Yao et al., 2018; Yao et al., 2019). This ceRNA network may provide valuable information for ovarian function.
Discussion
The next-generation sequencing shows that endogenous lncRNA is usually expressed in a variety of pig tissues, including ovaries, in a spatio-temporal specific manner. It was found that some differentially expressed lncRNAs were involved in spermatogenesis in pig testis tissues by transcriptome analysis. In addition, during pregnancy and non-pregnancy, a total of 34 lncRNAs were differentially expressed in pig endometrial tissues and involved in various biological processes (Wang et al., 2017). In this study, 16,428 genes were detected, and 2,392 genes were significantly differently expressed at the SLS and LLS ovarian samples. GO and KEGG analysis showed that 15 genes may be candidate genes affecting piglet size traits. Moreover, 302 lncRNAs were significantly differently expressed in SLS and LLS ovarian samples. ALAS1 and LOC100513133 showed the highest expression level of all upregulated and downregulated lncRNAs, revealing that they may has the potential to perform important functions on modulating porcine litter size.
Studies have shown that LncRNA regulates gene expression during the growth and development of different tissues in mammals, including ovaries. According to its position in the cell, lncRNA usually has a variety of regulatory mechanisms. In the cell nucleus, lncRNA can act as a molecular scaffold to recruit transcriptase or transcription factors to mediate the transcription of coding genes. In the cytoplasm, lncRNA acts as a ceRNA to competitively bind to miRNA, thereby reducing the inhibitory effect of miRNA on target genes (Sun, et al., 2016). As an important reproductive organ of female mammals, the ovary is controlled by a complex transcription network of coding and non-coding genes to undergo a series of biological processes during each estrus cycle, thereby regulating the reproductive capacity of the sow. However, these molecular regulation mechanisms affecting sow litter size are still unclear. In this study, abundant lncRNAs were differentially expressed in the SLS and LLS ovary tissue, revealing lncRNAs to have specific roles in ovarian function but not by-product of mRNA. Moreover, an mRNA-miRNA-lncRNA network was constructed according to the competitive endogenous RNA mechanism. The lncRNA LOC100513133 and LOC102168075 could be as ceRNAs containing potential binding sites for miR-26b, let-7g and miR-125b (Cao, et al. 2015b; Zhang, et al. 2020b). Previous studies have shown miR-125b is potential regulatory factor for the development of polycystic ovarian syndrome and miR-26b has a protective effect on ovarian granulosa cells (Xuan et al., 2024; Liu S. et al., 2020). Therefore, our next step is to explore the role of lncRNA in pig ovarian function.
Conclusion
This study is to map the expression of mRNA and lncRNA expression in porcine ovaries with smaller and larger litter size. Thousands of lncRNAs were identified, and several of them showed very different abundances in ovary tissues. We further constructed ceRNA network, and found that LOC100513133 and LOC102168075 might act as ceRNA. Our research has increased the understanding of the genetic mechanism of pig ovarian function and may provide new molecular target for the precise breeding of pig in China.
Data availability statement
The RNA-seq data of porcine ovary have been uploaded to the GEO database of NCBI and the data ID is GSE136592.
Ethics statement
The animal studies were approved by Institutional Animal Care and Use Committee at the Fuyang Normal University. The studies were conducted in accordance with the local legislation and institutional requirements. Written informed consent was obtained from the owners for the participation of their animals in this study.
Author contributions
SD: Data curation, Writing – original draft. FL: Data curation, Writing – original draft. LM: Data curation, Writing – review and editing. SC: Data curation, Writing – review and editing. HS: Data curation, Writing – review and editing. YQ: Data curation, Writing – review and editing. CH: Data curation, Writing – review and editing. SG: Data curation, Writing – review and editing. JL: Data curation, Writing – review and editing. SS: Data curation, Writing – review and editing. YL: Investigation, Writing – review and editing, Writing – original draft. GX: Investigation, Writing – review and editing, Writing – original draft.
Funding
The author(s) declare that financial support was received for the research and/or publication of this article. This work was supported by the Anhui Natural Science Foundation Project (2208085MC76), Key Projects of Scientific Research Plan of Colleges and Universities of Anhui Province (2022AH051345 and 2023AH050427), The University Synergy Innovation Program of Anhui Province (GXXT-2023-044), The Horizontal Cooperation Projects of Fuyang Normal University (HX2023023000 and HX2024039000), and The Open Project of Anhui Province Key Laboratory of Embryo Development and Reproductive Regulation (FSKFKT024D).
Conflict of interest
The authors declare that the research was conducted in the absence of any commercial or financial relationships that could be construed as a potential conflict of interest.
Publisher’s note
All claims expressed in this article are solely those of the authors and do not necessarily represent those of their affiliated organizations, or those of the publisher, the editors and the reviewers. Any product that may be evaluated in this article, or claim that may be made by its manufacturer, is not guaranteed or endorsed by the publisher.
Supplementary material
The Supplementary Material for this article can be found online at: https://www.frontiersin.org/articles/10.3389/fgene.2025.1498076/full#supplementary-material
SUPPLEMENTARY FIGURE S1 | mRNA-miRNA-lncRNA interaction network. The green circle represents the downregulated mRNA, the red circle represents the upregulated mRNA, the blue V-shape represents the downregulated miRNA, the pink V-shape represents the upregulated miRNA, the purple diamond represents the downregulated lncRNA, and the orange diamond represents the upregulated lncRNA.
References
Cao, R., Wu, W., Zhou, X., Liu, K., Li, B., Huang, X., et al. (2015a). Let-7g induces granulosa cell apoptosis by targeting map3k1 in the porcine ovary. Int. J. Biochem. Cell Biol. 68, 148–157. doi:10.1016/j.biocel.2015.08.011
Cao, R., Wu, W. J., Zhou, X. L., Xiao, P., Wang, Y., and Liu, H. L. (2015b). Expression and preliminary functional profiling of the let-7 family during porcine ovary follicle atresia. Mol. Cells 38, 304–311. doi:10.14348/molcells.2015.2122
Chen, L. L. (2016). Linking long noncoding rna localization and function. Trends Biochem. Sci. 41, 761–772. doi:10.1016/j.tibs.2016.07.003
Du, X., Li, Q., Cao, Q., Wang, S., Liu, H., and Li, Q. (2019). Integrated analysis of mirna-mrna interaction network in porcine granulosa cells undergoing oxidative stress. Oxid. Med. Cell Longev. 2019, 1041583. doi:10.1155/2019/1041583
Esteve-Codina, A., Kofler, R., Palmieri, N., Bussotti, G., Notredame, C., and Perez-Enciso, M. (2011). Exploring the gonad transcriptome of two extreme male pigs with rna-seq. BMC Genomics 12, 552. doi:10.1186/1471-2164-12-552
Hidalgo, J., Tsuruta, S., Lourenco, D., Masuda, Y., Huang, Y., Gray, K. A., et al. (2020). Changes in genetic parameters for fitness and growth traits in pigs under genomic selection. J. Anim. Sci. 98, skaa032. doi:10.1093/jas/skaa032
Hou, J., Long, H., Zhou, C., Zheng, S., Wu, H., Guo, T., et al. (2017). Long noncoding rna braveheart promotes cardiogenic differentiation of mesenchymal stem cells in vitro. Stem Cell Res. Ther. 8, 4. doi:10.1186/s13287-016-0454-5
Hubé, F., Velasco, G., Rollin, J., Furling, D., and Francastel, C. (2011). Steroid receptor rna activator protein binds to and counteracts sra rna-mediated activation of myod and muscle differentiation. Nucleic Acids Res. 39, 513–525. doi:10.1093/nar/gkq833
Kim, D., Pertea, G., Trapnell, C., Pimentel, H., Kelley, R., and Salzberg, S. L. (2013). Tophat2: accurate alignment of transcriptomes in the presence of insertions, deletions and gene fusions. Genome Biol. 14, R36. doi:10.1186/gb-2013-14-4-r36
Liao, K., Qian, Z., Zhang, S., Chen, B., Li, Z., Huang, R., et al. (2020). The lgmn pseudogene promotes tumor progression by acting as a mir-495-3p sponge in glioblastoma. Cancer Lett. 490, 111–123. doi:10.1016/j.canlet.2020.07.012
Lin, F., Li, R., Pan, Z. X., Zhou, B., Yu, D. B., Wang, X. G., et al. (2012). Mir-26b promotes granulosa cell apoptosis by targeting atm during follicular atresia in porcine ovary. PloS One 7, e38640. doi:10.1371/journal.pone.0038640
Liu, B., Luo, C., Lin, H., Ji, X., Zhang, E., and Li, X. (2020a). Long noncoding RNA XIST acts as a ceRNA of miR-362-5p to suppress breast cancer progression. Cancer Biother Radiopharm. 36, 456–466. doi:10.1089/cbr.2019.3481
Liu, J., Tu, F., Yao, W., Li, X., Xie, Z., Liu, H., et al. (2016). Conserved mir-26b enhances ovarian granulosa cell apoptosis through has2-ha-cd44-caspase-3 pathway by targeting has2. Sci. Rep. 6, 21197. doi:10.1038/srep21197
Liu, S., Li, L., Li, M., and Zhang, J. (2020b). Effect of miR-26b-5p on cis-diamine dichloroplatinum-induced ovarian granulosa cell injury by targeting MAP3K9. Vitro Cell Dev. Biol. Anim. 56, 213–221. doi:10.1007/s11626-020-00439-4
Meng, Q., Li, Z., Pan, J., and Sun, X. (2020). Long noncoding rna duxap8 regulates proliferation and apoptosis of ovarian cancer cells via targeting mir-590-5p. Hum. Cell 33, 1240–1251. doi:10.1007/s13577-020-00398-8
Pan, L., Gong, W., Zhou, Y., Li, X., Yu, J., and Hu, S. (2014). A comprehensive transcriptomic analysis of infant and adult mouse ovary. Genomics,Proteomics and Bioinforma. 12, 239–248. doi:10.1016/j.gpb.2014.08.002
Ponzio, G., Rezzonico, R., Bourget, I., Allan, R., Nottet, N., Popa, A., et al. (2017). A new long noncoding rna (lncrna) is induced in cutaneous squamous cell carcinoma and down-regulates several anticancer and cell differentiation genes in mouse. J. Biol. Chem. 292, 12483–12495. doi:10.1074/jbc.M117.776260
Ran, M., Chen, B., Li, Z., Wu, M., Liu, X., He, C., et al. (2016). Systematic identification of long noncoding rnas in immature and mature porcine testes. Biol. Reprod. 94, 77. doi:10.1095/biolreprod.115.136911
Schneider, J. F., Miles, J. R., Brown-Brandl, T. M., Nienaber, J. A., Rohrer, G. A., and Vallet, J. L. (2015). Genomewide association analysis for average birth interval and stillbirth in swine. J. Anim. Sci. 93, 529–540. doi:10.2527/jas.2014-7899
Shen, X., Liu, Z., Cao, X., He, H., Han, S., Chen, Y., et al. (2019). Circular rna profiling identified an abundant circular RNA circtmtc1 that inhibits chicken skeletal muscle satellite cell differentiation by sponging mir-128-3p. Int. Biol. Sci. 15, 2265–2281. doi:10.7150/ijbs.36412
Sun, X., Li, M., Sun, Y., Cai, H., Lan, X., Huang, Y., et al. (2016). The developmental transcriptome sequencing of bovine skeletal muscle reveals a long noncoding rna, lncmd, promotes muscle differentiation by sponging mir-125b. Biochim. Biophys. Acta 1863, 2835–2845. doi:10.1016/j.bbamcr.2016.08.014
Vázquez-Gómez, M., Garcia-Contreras, C., Pesantez-Pacheco, J. L., Torres-Rovira, L., Heras-Molina, A., Astiz, S., et al. (2020). Differential effects of litter size and within-litter birthweight on postnatal traits of fatty pigs. Animals 10, 870. doi:10.3390/ani10050870
Wang, Y., Hu, T., Wu, L., Liu, X., Xue, S., and Lei, M. (2017). Identification of non-coding and coding RNAs in porcine endometrium. Genomics 109, 43–50. doi:10.1016/j.ygeno.2016.11.007
Wang, Z., Yang, Y., Li, S., Li, K., and Tang, Z. (2019). Analysis and comparison of long non-coding rnas expressed in the ovaries of meishan and yorkshire pigs. Anim. Genet. 50, 660–669. doi:10.1111/age.12849
Wei, C., Wu, M., Wang, C., Liu, R., Zhao, H., Yang, L., et al. (2018). Long noncoding rna lnc-semt modulates igf2 expression by sponging mir-125b to promote sheep muscle development and growth. Cell Physiol. Biochem. 49, 447–462. doi:10.1159/000492979
Xuan, F., Jin, R., Zhou, W., Ye, Y., Ren, Y., Lu, J., et al. (2024). LncRNA SNHG12 promotes cell proliferation and inhibits apoptosis of granulosa cells in polycystic ovarian syndrome by sponging miR-129 and miR-125b. J. Ovarian Res. 17, 72. doi:10.1186/s13048-024-01392-6
Yao, Y., Niu, J., Sizhu, S., Li, B., Chen, Y., Li, R., et al. (2018). Microrna-125b regulates apoptosis by targeting bone morphogenetic protein receptor 1b in yak granulosa cells. DNA Cell Biol. 37, 878–887. doi:10.1089/dna.2018.4354
Yao, Y., Reheman, A., Xu, Y., and Li, Q. (2019). Mir-125b contributes to ovarian granulosa cell apoptosis through targeting bmpr1b, a major gene for sheep prolificacy. Reprod. Sci. 26, 295–305. doi:10.1177/1933719118770544
Yin, Z., Lian, L., Zhu, F., Zhang, Z. H., Hincke, M., Yang, N., et al. (2020). The transcriptome landscapes of ovary and three oviduct segments during chicken (gallus gallus) egg formation. Genomics 112, 243–251. doi:10.1016/j.ygeno.2019.02.003
Zhang, D., Tang, H. Y., Tan, L., and Zhao, D. M. (2020a). Malat1 is involved in the pathophysiological process of pcos by modulating tgfβ signaling in granulosa cells. Mol. Cell Endocrinol. 499, 110589. doi:10.1016/j.mce.2019.110589
Zhang, G., Lan, Y., Xie, A., Shi, J., Zhao, H., Xu, L., et al. (2019). Comprehensive analysis of long noncoding rna (lncrna)-chromatin interactions reveals lncrna functions dependent on binding diverse regulatory elements. J. Biol. Chem. 294, 15613–15622. doi:10.1074/jbc.RA119.008732
Zhang, X., Xiao, H., Zhang, X., E, Q., Gong, X., Li, T., et al. (2020b). Decreased microrna-125b-5p disrupts follicle steroidogenesis through targeting pak3/erk1/2 signalling in mouse preantral follicles. Metabolism 107, 154241. doi:10.1016/j.metabol.2020.154241
Zhang, Y., Tao, Y., and Liao, Q. (2018). Long noncoding rna: a crosslink in biological regulatory network. Brief. Bioinform 19, 930–945. doi:10.1093/bib/bbx042
Zheng, X., Zhao, P., Yang, K., Ning, C., Wang, H., Zhou, L., et al. (2020). CNV analysis of Meishan pig by next-generation sequencing and effects of AHR gene CNV on pig reproductive traits. J. Anim. Sci. Biotechnol. 11, 42. doi:10.1186/s40104-020-00442-5
Keywords: lncRNA, pig, ovary, litter size, ceRNA
Citation: Duan S, Li F, Meng L, Cheng S, Shi H, Qu Y, He C, Gao S, Li J, Sun S, Liu Y and Xu G (2025) Discovery of differentially expressed lncRNAs in porcine ovaries with smaller and bigger litter size. Front. Genet. 16:1498076. doi: 10.3389/fgene.2025.1498076
Received: 18 September 2024; Accepted: 03 April 2025;
Published: 16 April 2025.
Edited by:
Xiaoxue Zhang, Gansu Agricultural University, ChinaReviewed by:
Hui Li, Guangxi University, ChinaNing Song, Anhui Agricultural University, China
Sui Shiyan, Dali University, China
Copyright © 2025 Duan, Li, Meng, Cheng, Shi, Qu, He, Gao, Li, Sun, Liu and Xu. This is an open-access article distributed under the terms of the Creative Commons Attribution License (CC BY). The use, distribution or reproduction in other forums is permitted, provided the original author(s) and the copyright owner(s) are credited and that the original publication in this journal is cited, in accordance with accepted academic practice. No use, distribution or reproduction is permitted which does not comply with these terms.
*Correspondence: Yong Liu, bGl1eW9uZ0BmeW51LmVkdS5jbg==; Gaoxiao Xu, eGd4MTM4QDEyNi5jb20=
†These authors have contributed equally to this work and share first authorship