- Department of Biochemistry & Biomedicine, the School of Life Sciences, University of Sussex, Brighton, United Kingdom
It has been acknowledged that more women suffer from adverse effects of drugs than men globally. A group of drugs targeting serotonin [5-hydroxytryptamine] (5-HT) binding G-protein-coupled receptors (GPCRs) have been reported to preferentially affect women more than men, causing adverse effects such as breast cancer and infertility. 5-HT GPCR-targeted drugs in the central nervous system (CNS) manage psychiatric conditions, such as depression or bipolar and in the peripheral nervous system (PNS) treat migraines. Physiological characteristics such as specific types of hormones, higher body fat density and smaller body mass in women result in disparities in pharmacodynamics of drugs, thus explaining sex-related differences in the observed adverse effects. In this review, we discuss the side effects of drugs targeting 5-HT GPCRs based on serotonin's roles in the CNS and PNS. We have systematically reviewed adverse effects of drugs targeting 5-HT GPCR using information from the Food and Drug Administration and European Medicines Agency. Further information on drug side effects and receptor targets was acquired from the SIDER and DrugBank databases, respectively. These drugs bind to 5-HT GPCRs in the CNS, namely the brain, and PNS such as breasts, ovaries and testes, potentially causing side effects within these areas. Oestrogen affects both the biosynthesis of 5-HT and the densities of 5-HT GPCRs in given tissues and cells. 5-HT GPCR-targeting drugs perturb this process. This is likely a reason why women are experiencing more adverse effects than men due to their periodic increase and the relatively high concentrations of oestrogen in women and, thus a greater incidence of the oestrogen-mediated 5-HT system interference. In addition, women have a lower concentration of serotonin relative to men and also have a relatively faster rate of serotonin metabolism which might be contributing to the former. We discuss potential approaches that could mitigate at least some of the adverse effects experienced by women taking the 5-HT GPCR-targeting drugs.
Introduction
Serotonin [5-hydroxytryptamine] (5-HT) receptors belong to the rhodopsin class A family of G-protein-coupled receptors (GPCRs) (1) and are implicated in processes such as aggression, emesis, mood, and cognition (2, 3). There are fourteen 5-HT-binding receptors encoded by the human genome, of which one is an ion channel and the remaining thirteen are GPCRs. The GPCRs are grouped into six subtypes (5-HT1, 5-HT2, 5-HT4, 5-HT5, 5-HT6 and 5-HT7,receptors) based on their function and structure. 5-HT2, 5-HT4, 5-HT6 and 5-HT7 are excitatory receptors, while 5-HT1 and 5-HT5 receptors are inhibitory (4, 5). 5-HT1, 5-HT2, and 5-HT5 subtypes have further isoforms, such as 5-HT1a, 5-HT1b, 5-HT1d, 5-HT1e, 5-HT1f, 5-HT2a, 5-HT2b, 5-HT2c, 5-HT5a, and 5-HT5b. 5-HT5b is a pseudogene in humans (Table 1). 5-HT GPCRs are present in both the central nervous system (CNS) and peripheral nervous system (PNS) and have a distinct short N-terminal extracellular domain that binds to serotonin to induce a cellular response (1).
Serotonin is a type of catechol amine that serves as a neurotransmitter in the CNS as well as a hormone, regulating many fertility- and postnatal-morphogenesis of mammary glands, important for reproduction. Serotonin circulates in the cardiovascular system mostly stored in platelets (2). In the gut, serotonin is synthesised from an essential amino acid, tryptophan by tryptophan hydroxylase (TPH), which produces 5-hydroxytryptophan. Aromatic l-amino acid decarboxylase converts 5-hydroxytryptophan into serotonin (5, 6). It has been reported that women metabolise serotonin at a greater rate than men (7). In addition, men have a higher rate of serotonin synthesis than women (8). On average, serotonin levels are generally found to be higher in men than in women by approximately 50%. Moreover, there is a change in serotonin concentration over the 28-day periodic cycle in women (7), which poses a serious challenge in drug administration.
5-HT GPCR-targeting drugs generally have a complementary structure to their target receptor binding sites found in the CNS and PNS. 5-HT GPCR-targeting drugs act as agonists or antagonists. Excitatory-type 5-HT GPCRs are activated by agonists and inhibited by antagonists. Inhibitory-type 5-HT GPCRs inhibit a given pathway when bound to 5-HT in a negative feedback loop and therefore, agonists will replace such activity by 5-HT in promoting negative feedback, while antagonists will block the inhibitory pathway. 5-HT GPCR-targeting drugs generally have a complementary structure to its target receptor binding sites found in the CNS and PNS, where antagonists intended to block excitatory-type 5-HT GPCRs, could also bind to inhibitory-type 5-HT GPCRs thereby potentially competing with 5-HT and prevent the normal inhibitory pathway function. Similarly, agonists that activate excitatory-type 5-HT GPCRs may activate the inhibitory-type 5-HT GPCRs that further enhance the inhibitory pathway. When the intended target is an inhibitory 5-HT GPCR, drug treatment could also affect the excitatory-type 5-HT GPCRs. In both cases, excess activation of excitatory and inhibitory type 5-HT GPCRs could result in adverse effects (9).
The pharmaceuticals are intended to elicit their desired response in the PNS or CNS. However, there have been a few reports indicating that those remaining in PNS perturb the normal function of 5-HT GPCRs in systems such as serotonin-regulated milk production in breasts, muscle contraction in ovaries and sperm production in testis causing adverse effects within these organs (10). Side effects reported amongst those drugs targeting 5-HT GPCRs include libido decrease/increase, headache, abdominal pain and/or infertility (Table 2 and Supplementary Table S2). Some 5-HT GPCR-targeted drugs cause severe adverse effects, such as hyperprolactinemia, which is also seen more often in women than men (11).
Current drug trial regimes test primarily men throughout the clinical trial phases. A review in 2018 reported that 72% of randomized controlled trials testing efficacy of drugs did not describe sex specific analysis (12). Despite the data being available, many of the studies are not reporting gender specific data. In addition, medics and researchers across the world have suggested that more women should be involved in clinical trials to evaluate and adjust the dosage and timing of drug administration for women (10, 12). Moreover, hormonal cycles and their dynamic changes at the time of any drug administration are the factors that influence sex differences' side effects (10). Clearly, a great deal of consideration is still required to understand how sexual hormones, such as oestrogen, androgen and testosterone, mediate sex-based differences in pharmacodynamics.
In this review, we will discuss the propensity of neurological pharmaceuticals targeting 5-HT GPCR to interfere with the normal function of serotonin in the CNS and PNS. The roles of sexual hormones, oestrogen, androgen and testosterone in the 5-HT system were analysed to understand how targeting 5-HT GPCRs could perturb or hinder the regulatory effect of sexual hormones on 5-HT biosynthesis alter the expression of 5-HT GPCRs that potentially contribute to side effects. Furthermore, we will discuss how the internal physiological system in women is more vulnerable to neurological pharmaceuticals, which increases the chance of side effects (Supplementary Table S1).
Serotonin in the CNS and PNS
According to Human Protein Atlas (https://www.proteinatlas.org), all thirteen 5-HT GPCRs are expressed in the human brain (13). In the CNS, serotonin is produced in the cell bodies of serotonergic neurons found in the raphe nuclei from where serotonin is stored and released. These serotonergic neurons send signals toward ascending projections that terminate in the midbrain, limbic, cortical and hindbrain regions in a defined manner (14). Serotonin binds to 5-HT GPCRs in all regions of the brain. Individual neurons may express more than one type of 5-HT GPCR and serotonergic receptors can function on their own or in concert with other subtypes. For example, 5-HT2c and 5-HT1a receptors together modulate anxiety, while 5-HT2c receptor expressed on its own mediate appetite, locomotion and reward in neuronal cells.
Dysfunction of the serotonergic system is implicated in the pathogenesis of neurological and psychiatric disorders (14). Examples of disorders treated by 5-HT GPCR-targeted drugs include schizophrenia, generalized anxiety disorder (GAD), obsessive-compulsive disorder (OCD), major depressive disorder (MDD), premenstrual dysphoric disorder (PMDD), migraine, dravet syndrome, anti-heart burn and menopausal symptoms (Table 2). The average age of onset of psychiatric disorders on the above list lies between 18 and 25. It is widely accepted that the average age of the onset of GAD and OCD is approximately 30 years old, menopause is usually between 42 and 52 years of age, whereas that of dravet syndrome is 1–18 months (15–17). Elevated levels of serotonin in the brain could result in schizophrenia. Thus, treatment methods for this condition is to compensate for the hyper activities of both inhibitory and excitatory 5-HT GPCRs (Table 2). Conversely, GAD, OCD, MDD, PMDD, migraine, dravet syndrome and menopausal symptoms are caused by reduced serotonin levels in the brain. These disorders require mitigation of reduced activities of excitatory and inhibitory 5-HT GPCRs (Table 2). Bipolar patients can experience either an elevation or decrease in serotonin depending on whether they are experiencing a manic or depressive episode, respectively (18).
Serotonin has been shown to increase prolactin, a peptide hormone synthesized by lactotrophs in the anterior pituitary and is essential for lactation, breast development, pregnancy, sexual intercourse, ovarian functioning, regulation of progesterone-secreting structures and inhibition of hypothalamic gonadotropin-releasing hormone (19). Prolactin concentration is generally lower in men compared to women and it is at the highest in women during pregnancy (20). In mammals, intravenous administration of serotonin results in increased prolactin secretion (19, 21). Serotonin induces the release of prolactin releasing factors (PRFs), such as oxytocin and vasoactive intestinal peptide from the hypothalamus. PRFs influence lactotrophs in the anterior pituitary gland to release prolactin (19, 21).
Serotonin can cause vasoconstriction or vasodilation through either inhibitory (5-HT1) or excitatory (5-HT2) 5-HT GPCRs, respectively (22). In the extracerebral carotid arteries, serotonin binds to serotonergic receptors (5-HT1b and 5-HT1d) causing contraction of vascular smooth muscle cells and vasoconstriction of blood vessels. Migraines occur when there is a reduction in serotonin that leads to vasodilation of intracranial extracerebral blood vessels. When blood vessels dilate, the trigeminovascular system triggers the release of vasodilators, such as calcitonin gene-related peptide (CGRP), which further exacerbates pain responses. The reduction of oestrogen during menstruation and the late-luteal phase of the menstrual cycle can in-turn reduce serotonin concentration in the cardiovascular system and is potentially a cause of migraines in some women during their menstrual cycle (23).
Synergistic and antagonistic effect of sexual hormones and serotonin in the brain
There is evidence of significant interactions between sexual hormones and serotonin biosynthesis enzymes such as TPH in the CNS. There are 2 forms of TPH encoded by the human genome. TPH1 is localised both in the brain and body, whereas TPH2 is primarily expressed in the brain (13). Studies using primates and mammals showed there is a positive correlation between the accumulation of oestradiol and progesterone and the TPH enzyme (24). Results using oestrogen receptor (ER)α and ERβ KO mice showed that ERβ transcription factor is translocated to the nucleus and binds to the TPH1 promoter when oestrogen levels increase and this leads to an accumulation of TPH1 (25). In an in vitro system in the presence of oestrogen, ERβ was also shown to upregulate TPH2 by binding to the oestrogen response element in the promoter (Figure 1) (26).
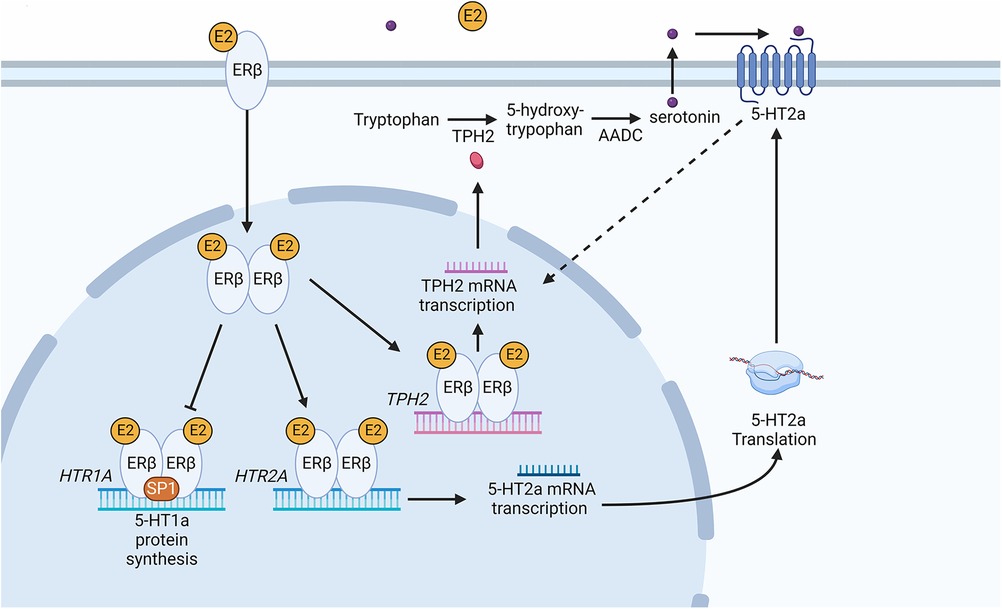
Figure 1. Schematic diagram of the serotonin synthesis pathway following an increase in oestradiol in the peripheral nervous tissue. The increase in E2 leads to the activation of ERβ causing it to dimerize. Dimerized ERβ interacts with SP1 to inhibit HTR1A receptor transcription. Secondly, ERβ causes transcription of the HTR2A receptor gene, increasing 5-HT2a receptor protein in the cell. An increase in 5-HT2a results in an increase in tryptophan hydroxylase 2 (TPH2) mRNA and subsequently TPH2 protein. The dotted line represents an indirect induction of TPH2 by 5HT2a. Dimerized ERβ directly induces TPH2 transcription leading to TPH2 protein synthesis. TPH2 catalyses the conversion of tryptophan into 5-hydroxytryptophan, which is then converted into serotonin (5-hydroxytryptamine) via aromatic l-amino acid decarboxylase (AADC). Serotonin binding with 5-HT2a leads to positive feedback increasing TPH2 and resulting in serotonin production. Created with BioRender.com.
Sexual hormones were also shown to regulate serotonin receptor isoforms 5-HT2a and 5-HT1a. In the case of 5-HT2a receptor, the effect of female hormones was synergistic (27), whereas the hormones act antagonistically to the accumulation of 5-HT1a receptors (Figure 2). Studies of post-menopausal women between the ages 45–55, receiving 17β-oestradiol (E2) hormonal replacement therapy for 14 weeks, followed by a maximum of 6 weeks of E2 and micronized progesterone have shown there is a positive correlation between an increase in oestrogen level and serotonin binding to the 5-HT2a receptor (28). PET scanning of the frontal cortex, to visualize [18F] altanserin labelled 5-HT2a receptor, indicated that in the presence of E2 and progesterone, 5-HT2a receptor expression increases (28). An increase in oestrogen concentration in the hypothalamus of rhesus macaque monkeys caused a decrease in radiolabelled [3H]-8-OH-DPAT binding to the 5-HT1a receptor and HTR1A gene expression (29). Safe (2001) has shown in an in vitro system that an increase in E2 initiated a decrease in HTR1A expression by causing the trimer complex formation of the transcription factor, specificity protein 1 (Sp1), and homodimer ERβ. The trimer complex is translocated to the nucleus to inhibit HTR1A transcription via binding to the ER binding element region of HTR1A (Figure 1). It was proposed that the binding of these transcription factors recruit unknown proteins and repress HTR1A expression when oestrogen concentrations increase (30). Conversely, HTR1A expression increases when oestrogen decreases. A study, using COS-1 cells transiently co-transfected with HTR1A promoter fused to a reporter gene and an expression vector encoding ERα or ERβ, suggested that activation of ERα results in HTR1A expression. The AF-1 domain specific to ERα receptor allows it to interact with the nuclear factor kappa-light-chain-enhancer of activated B cells (NF-kB) and this interaction is essential for the activation of HTR1A promoter (31).
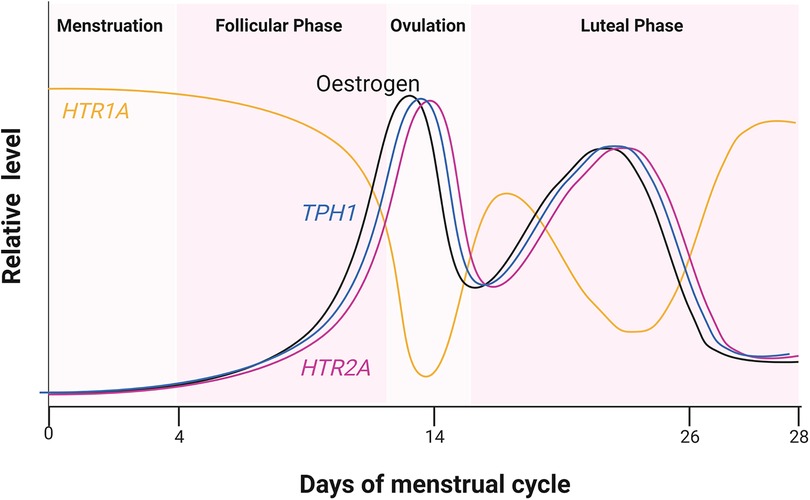
Figure 2. Relative hormone level during the four estrus phases. Cycling of oestrogen levels during the menstrual cycle was compared to the expression of HTR2A and HTR1A encoding 5-HT2a and 5-HT1a receptors, respectively. Oestrogen receptor (ER) also regulates TPH1 encoding one of the serotonin biosynthesis genes. Created with BioRender.com.
These results clearly indicated that oestrogen plays a major role in 5-HT1a and 5-HT2a receptor regulation in women. The following studies showed that the small amount of oestrogen in men also plays a role in 5-HT2 receptor expression. Autoradiography showed that radiolabelled 5-HT2a receptor density was reduced in the frontal, piriform and cingulate cortex, the nucleus accumbens and olfactory tubercle of male Wistar rats upon castration (32). An increase in the number of radio-labelled 5-HT2a receptors was observed only when oestradiol benzoate (EB) or testosterone propionate (TP) were present and not with dihydrotestosterone (DHT). It was speculated that this increase in 5-HT2a receptor density was due to an endogenous aromatase converting TP to E2. The authors concluded that only oestrogen converted from testosterone in vivo increases 5-HT2a receptor mRNA accumulation and 5-HT2a GPCR protein levels (32).
Serotonin and serotonergic GPCRs in women
All 13 serotonergic GPCRs are also present in the PNS. However, the localization of each is not identical between women and men (Table 1). Human Protein Atlas shows that 5-HT1, 5-HT2, 5-HT5 and 5-HT7 receptor mRNA and protein are present in the breasts of women but they are not detected in that of men (13). Serotonin is known to be synthesised and secreted from the mammary glands in female breasts (6). The development of mammary glands and milk production in humans requires a concerted sequence of events. Prolactin released from the pituitary gland in the CNS induces serotonin production in the breast tissue. There is a negative feedback loop between prolactin and serotonin. Prolactin promotes milk production while serotonin inhibits it in humans. Matsuda et al. (2004) and Stull et al. (2007) showed in mice that prolactin stimulates the expression of serotonin biosynthesis genes, TPH1 and aromatic l-amino acid decarboxylase. Both genes were detected in the breast tissues at many developmental stages, particularly during nulliparous, milk filling, pregnancy, postpartum and involuting. Breastfeeding triggers a reflex secretion of prolactin from the pituitary gland in the CNS and the expression of serotonin biosynthesis genes forms part of a negative-feedback system, induced in response to stimulation by prolactin during milk filling (6). Histological analysis of mammary gland cultures of pregnant mice showed the addition of serotonin in the presence of prolactin results in the collapse of alveolar clusters (6). It has been shown that binding of serotonin to the 5-HT7 receptor leads to a decline in key scaffolding proteins forming tight junctions between epithelia resulting in the leakage of lactose to the basolateral side of the cell that represses milk production from the epithelial cells (33).
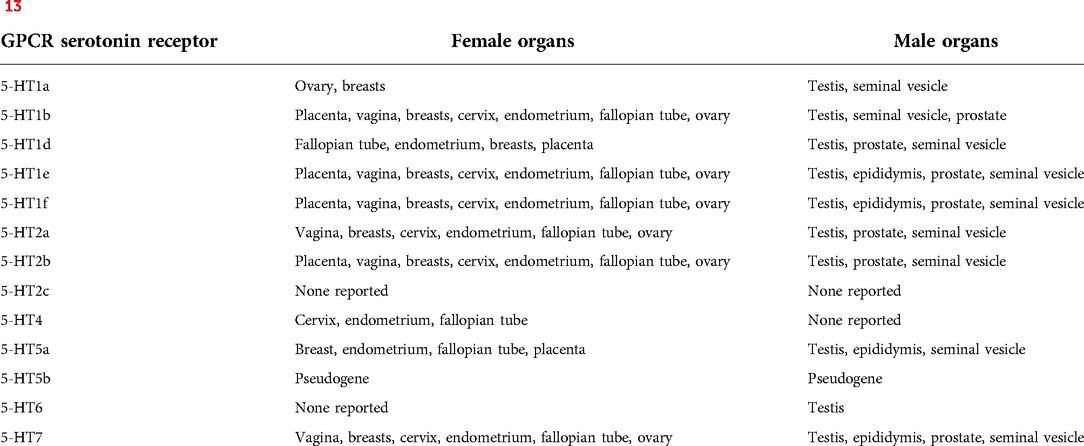
Table 1. Serotonin receptor expression in sex organs. GPCR 5-HT receptors expressed in female and Male organs according to Human Protein Atlas (13).
Studies also showed that while prolactin promotes it, serotonin suppresses expression of the β-casein gene that encodes a milk protein found in mammalian milk, which is a bioactive peptide essential for suckling by infants (34). Western blot analysis indicated that with elevated levels of prolactin, there is an increase in β-casein mRNA in MCF-12A human mammary epithelial cell (35, 36). Prolactin binds to the receptors found in the mammary epithelial cell, which triggers activation and transcription of β-casein. An increase in locally produced serotonin in the mammary gland has been shown to decrease β-Casein in a concentration-dependent manner. It was concluded that serotonin attenuates β-Casein accumulation (6, 36). Taken together, breast tissues receive autocrine as well as paracrine serotonergic signals essential for the maintenance of milk homeostasis (Figure 3).
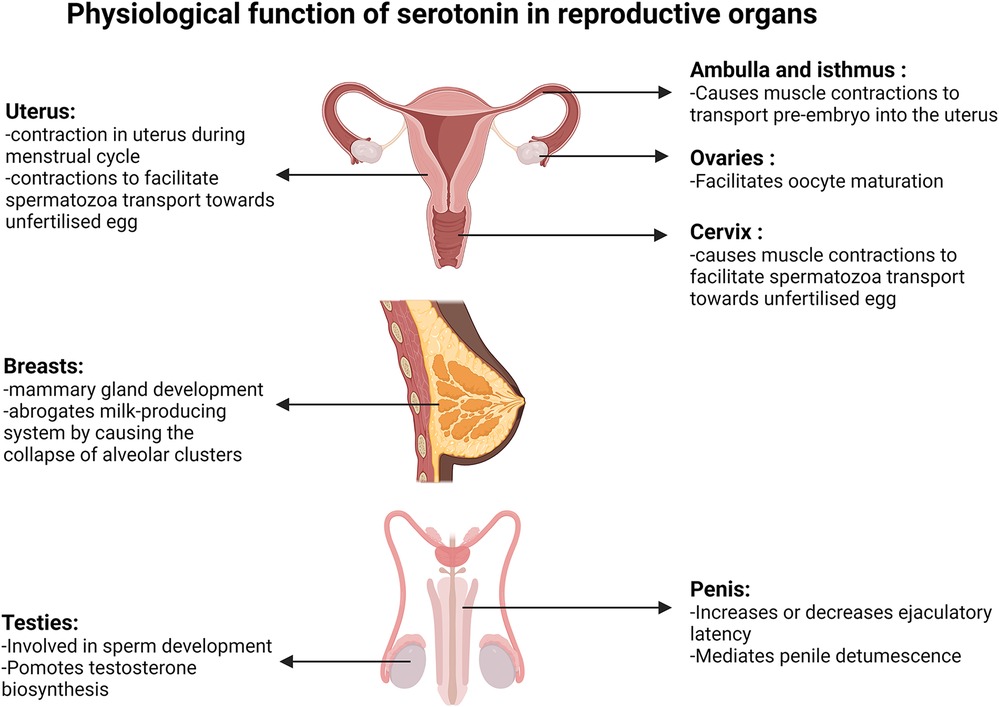
Figure 3. Physiological function of serotonin in reproductive organs. Diagrams present the function of serotonin in the uterus, fallopian tube, ovaries, cervix, breasts, testis and penis. Created with BioRender.com.
Serotonin and oestrogen act synergistically in uterus and cervix contractions. Oestrogen concentration is at its lowest during menstruation, then gradually increases during the follicular phase that culminates between days 12 and 14 and peaks again during the mid to early luteal phase (Figure 2). Reports from a non-invasive ultrasound study in women revealed that the frequency of uterine contraction increases during the late follicular phase and declines throughout the luteal phase (37). Additionally, serotonin was shown to enhance uterine and cervix peristaltic contraction during the early phases of the menstrual cycle when oestrogen is elevated (38). The absence of uterine contractions may lead to endometriosis, dysmenorrhea or prevention of oocyte migration from the ovaries to the uterus, which all potentially lead to infertility (37). It has been demonstrated that serotonin enhances the contractions initiated by oestrogen during the migration of sperm towards the oviduct, likely contributing to the chances of egg fertilization (Figure 3). Hysterosalpingoscintigraphy, intrauterine pressure and oestrogen measurement showed that, by imitating sperm entrance into the posterior vaginal fornix, oestradiol concentration and thereby uterine contractions increase (39). It was also shown that E2 perfusion causes uterine peristalsis with a cervico-fundal direction (40). Oestrogen and serotonin synergistically mediate contractions in the uterus and cervix (38) and elevated levels of serotonin and 5-HT2a receptors were postulated to influence uterine contractions (41). 5-HT2 receptors coupling to the Gαq-protein family, stimulated by serotonin, have been shown to cause activation of the phospholipase C/intracellular calcium pathway, ultimately resulting in uterine muscle contraction in rats (42). Oviduct motility is important for pre-embryo and gamete transportation (Figure 3). Radioligand binding assay showed that serotonin binding to 5-HT7 receptors in muscles of the isthmus and ampulla, found in the oviduct, caused muscle relaxation (43). Serotonin has been shown to bind to 5-HT2a, 5-HT2b and 5-HT7 receptors in the ovaries and through receptor-mediated actions on Ca2+ and cAMP, control mammalian follicular growth and oocyte meiotic maturation (44).
Serotonin and serotonergic GPCRs in men
Effects of serotonin on fertility are not confined to women and there is evidence that it also influences male reproductive performance. In male testicles, serotonin is involved in normal sperm development and testosterone biosynthesis (Figure 3). Inhibiting serotonin synthesis causes a decrease in follicle-stimulating hormone (FSH) and testosterone concentration in blood samples. Using a membrane integrity assay, caspase activity measurement, and flow cytometry tests it was shown that in the absence of serotonin, the number of viable sperm decreases, the sperm membrane integrity declines and there is an increase in abnormal sperm production (45).
Serotonin binding to 5-HT GPCRs can increase or decrease ejaculatory latency (Figure 3). Intraurethral infusion of serotonin showed that serotonin decreases the threshold urethral perfusion pressure and increases the number of ejaculation reflexes (46). Studies in rats and rhesus monkeys showed that 5-HT1c and 5-HT1d receptor agonists inhibit ejaculation in a dose dependent manner. Conversely, serotonin facilitates male ejaculation by interacting with 5-HT1a and 5-HT2 receptors, while administration of 5-HT2 receptor antagonists inhibits male ejaculation in mice (46, 47).
Side effect of drugs targeting serotonin 5-HT GPCRs
Studies have shown that 40% of men and 60% of women treated with antipsychotics had a prolactin level above the upper limit of the normal range (15–25 µg/L) (11). Hyperprolactinemia is unlikely a symptom of psychiatric disorders as the level of prolactin found in healthy adults and patients with psychiatric conditions prior to drug administration are comparable (11). It is suggested that neurological drugs targeting 5-HT GPCRs in the pituitary gland increase prolactin in both women and men often to above the normal levels and is therefore, one of the most common side effects caused by those drugs. Drugs that are agonists to excitatory-type 5-HT GPCR, such as 5-HT2a, mimic serotonin, which results in prolactin secretion from lactotroph cells in breast tissue into the bloodstream. Antagonist drugs to inhibitory-type 5-HT GPCR, for example 5-HT1a, remove prolactin inhibitory pathways and thus prolactin levels remain elevated in the bloodstream (48). The 5-HT GPCR antagonists risperidone, olanzapine and paliperidone, and the agonist ziprasidone (Table 2) are all reported to cause hyperprolactinemia. Side effects linked to hyperprolactinemia are decreased fertility, headaches, reduced spermatogenesis, galactorrhoea, amenorrhea and androgen deficiency, leading to erectile dysfunction and decrease in libido (60, 61). Hyperprolactinemia, caused by antipsychotics, may be further exacerbated in women as the result of a higher baseline prolactin level compared to that of men. Pregnant women may experience hyperprolactinemia from even higher levels of prolactin secreted by the immune system, uterus, placenta and mammary glands compared to those who are not pregnant (20). Serotonin interacts with 5-HT GPCRs, triggering prolactin synthesis in the breast tissue. Consequently, breast development, lactation and pain may occur in breasts (21). Drugs such as trazodone hydrochloride and citalopram hydrobromide cause lactation, breast enlargement and pain (Supplementary Table S2) and it is possible that these drugs mimic serotonin to cause these effects.
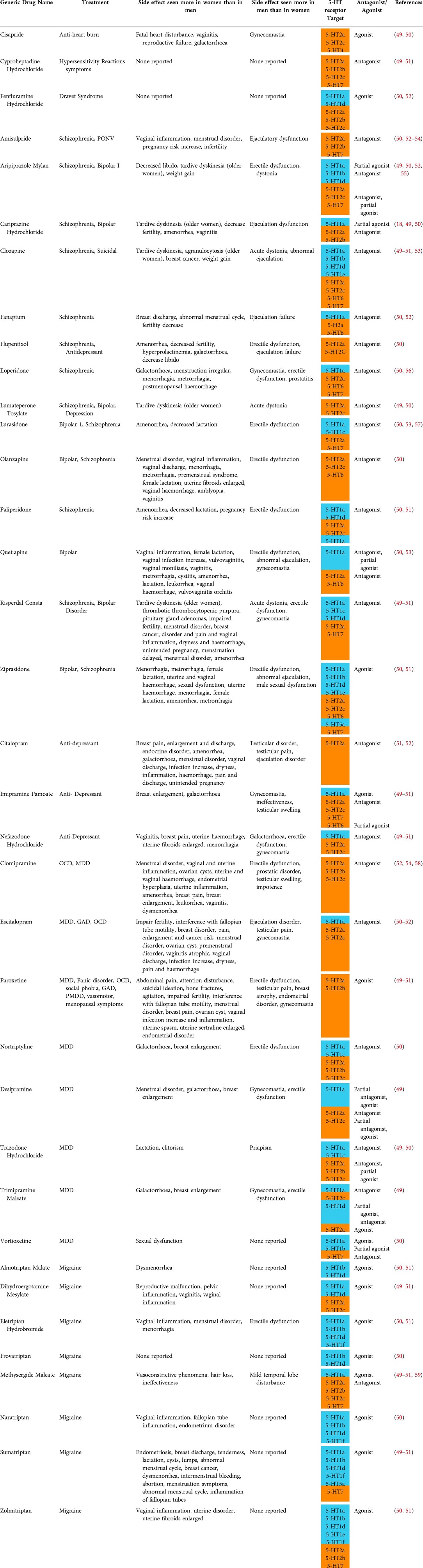
Table 2. Drugs used to treat psychiatric and neurological conditions that target serotonin receptors and their side effects in women and men. Data obtained from EMA and FDA drug approving bodies, SIDER and DrugBank. Abbreviations: MDD, multiple personality disorder; OCD, obsessive compulsive disorder; GAD, generalized anxiety disorder; PMDD, premenstrual dysphoric disorder; SAD, social anxiety disorder; PD, panic disorder; 5-HT, serotonin. Orange coloured cells represent excitatory receptors and blue represents inhibitory receptors.
Benign breast tumour is another common side effect of 5-HT GPCR-targeted drugs predominantly seen in women since prolactin promotes breast cell division (62) and prolonged hyperprolactinemia increases the risks of breast cancer. In cancer cells, constitutive prolactin activity promotes cell proliferation, cell motility, angiogenesis, and metastasis (63). Risperidone, sumatriptan, zolmitriptan and clozapine have both been shown to increase the risks of breast cancers (Supplementary Table S2). A cohort study showed breast cancer patients administered SSRIs have a 27% increase in mortality, showing that some antipsychotics contribute to breast cancer progression (64). Microarray analyses using a cancer cell line and immunohistochemistry using breast tumour tissues extracted from patients showed elevated accumulation of serotonin biosynthesis enzyme genes and proteins, respectively. In addition, HTR7, HTR1D and HTR2B genes encoding 5-HT GPCRs were also found upregulated in these samples (65). Serotonin is generally thought to promote the disease progression of breast cancer. The shift in expression of 5-HT GPCRs is believed to promote malignant pathways, such as cell proliferation and inhibition of cell cycle arrest. The above mentioned drugs identified to increase the risks of breast cancer (Supplementary Table S2) may be targeting the 5-HT7 receptor known to drive p38 mitogen-activated protein kinase (p38 MAPK) pathways that increases the chances of transforming breast cells to cancer cells (66).
Menstrual irregularities and infertility in women may also result from taking 5-HT GPCR-targeted drugs. A study using female mice receiving continuous infusions of prolactin to induce hyperprolactinemia showed prolonged elevated prolactin caused abrogation of the oestrous cycle and a decrease in FSH, luteinizing hormone (LH) and ovum lutea, resulting in anovulation (67). Without LH regulating the menstrual cycle and FSH causing female egg development, ovulation and menstruation do not take place, leading to infertility. As shown in Table 2 and Supplementary Table S2, side effects such as hyperprolactinemia and menstrual irregularities and/or infertility have been reported for drugs such as ziprasidone, olanzapine and risperidone. Taken together with the experimental results in mice, these drugs may cause menstrual irregularities and infertility as a result of hyperprolactinemia in women.
The effects of paroxetine and escitalopram oxalate have been tested on isolated isthmus and ampulla of 20 postmenopausal women who underwent hysterectomy (68). The tension of the isolated preparations was recorded by an isometric transducer and the effects of both drugs were recorded over time. The results showed that both paroxetine and escitalopram oxalate cause spontaneous contractions in the fallopian tube that may interfere with their natural function (68). A prolonged and uncontrolled fallopian tube spasm is associated with a decrease in fertility because it prevents sperm from being retained in the oviduct. Thus, the sperm cannot successfully fertilise the ovum (69). Although this is just one report, the above observation may explain the female reproductive impairment reported in women from taking paroxetine and escitalopram oxalate (Table 2).
A systematic literature review demonstrated that SSRIs altering sperm cell morphology cause a reduction in the number of viable sperm and, as a result, decrease male fertility (65). Hyperprolactinemia in male mice has been shown to inhibit the release of testosterone, which resulted in erectile dysfunction. Drugs, such as aripiprazole, flupentixol, risperidone and olanzapine have been reported to cause both hyperprolactinemia and erectile dysfunction (Table 2 and Supplementary Table S2).
Interpretation
Across the board, the estimated number of adverse side effect cases reported in women is 1.5 to 1.7-fold greater than in men (65), which is consistent with the data published by Martin et al. (1998) (5). Thus, the ratio of women experiencing more adverse effects from medications has remained consistent over twenty years. In this review, we have investigated side effect reports, packet notes, and experimental results using laboratory animals and humans concerning drugs targeting serotonin 5-HT GPCRs and critically analysed the mechanism of the side effects in women and men (70).
Serotonin stored in the platelets in the cardiovascular system remains in the PNS, while serotonin in the CNS remains in the brain in the normal healthy body due to the blood-brain barrier (2). Here, we propose that those neurological drugs targeting 5-HT GPCRs in the CNS may interact with 5-HT GPCRs in the PNS and cause side effects within these areas. Expression patterns of 5-HT GPCRs in the PNS are localised in sexual organs and are, therefore, different between women and men. Due to the essential role of 5-HT GPCRs in sexual organs in humans, any disturbance of serotonin concentration in the PNS impacts the normal function of the respective sexual organs.
In both women and men, oestrogen regulates the expression of 5-HT GPCRs and serotonin biosynthesis (Figure 1). The promoters of the TPH1 and TPH2 genes have an ER binding element, and therefore oestrogen, and not androgen or testosterone, triggers serotonin biosynthesis (25, 26). When the oestrogen level is high, ER binding to the promoter of HTR2A results in upregulation of the 5-HT2a receptor. When oestrogen is elevated, ERß binds to the HTR1A promoter to inhibit transcription, thereby reducing the number of 5-HT1a receptors, whereas a decrease in oestrogen allows ERα binding to the HTR1A promoter, resulting in an increase of 5-HT1a receptors. A reduction of oestrogen results in a reduction of 5-HT2a receptors (28, 30). Compared to women, men produce lower levels of oestrogen and their oestrogen levels fluctuate less due to the absence of a menstrual cycle. Thus, it is assumed that oestrogen influences 5-HT GPCRs to a greater extent in women than in men. Studies have shown that serotonin biosynthesis and signalling pathways are affected preferentially by oestrogen, while there is very little evidence for male hormones such as androgen and testosterone having the same interaction. As a result, excitatory 5-HT GPCRs, such as 5-HT2 receptor types, can be hyper-activated when the oestrogen concentration is high (27, 32).
Results suggest neurological drugs interfere with the regulation of 5-HT GPCRs by oestrogen. A reduction and thus a potentially lower than anticipated level of serotonin concentration in the cardiovascular system leads to vasodilation of the extracerebral carotid arteries. This would increase the volume of blood flow in these arteries and increase pressure on the surrounding tissues in the centre of the brain causing pain as a result. The 5-HT1a receptor is known to induce vasoconstriction in the presence of the expected concentration of serotonin. There is a trough in serotonin concentration coinciding with the decrease in oestrogen during the menstrual cycle (Figure 2). This may explain why there are more women suffering from migraines and headaches that coincide with the time when oestrogen is at its lowest, i.e., prior to and during menstruation. Drugs treating bipolar disorder and schizophrenia are intended to act as an antagonist, targeting 5-HT2 receptors in the CNS. However, they may also bind to 5-HT1 receptors and could prevent vasoconstriction in the extracerebral carotid arteries if they remain in the PNS. Such antagonists, if given to women during pre-menstruation when oestrogen levels are at their lowest and 5-HT1a receptor expression is at its highest (Figure 2) during pre-menstruation, could cause severe and potentially debilitating headaches. This is because an inhibition of 5-HT1 receptors by 5-HT GPCR antagonists would result in vasodilation. One suggestion to be considered is to reduce the dosage or end administration of 5-HT GPCR antagonists to women who are bipolar or schizophrenic during phases of the menstrual cycle when oestrogen is at its lowest.
Although drugs for the treatment of dravets syndrome and other conditions, such as bipolar and schizophrenia, target 5-HT GPCR, those used to treat the former do not have a large number of reported side effect cases (Supplementary Table S2). Dravets syndrome, in most cases, is diagnosed and treated in infants, whereas schizophrenia and bipolar are diagnosed and given to patients post puberty (15, 17). Thus, drugs for dravets syndrome are less likely to interfere with the oestrogen-mediated serotonin system to the same extent as in sexually matured and adult patients treated with other 5-HT GPCR drugs. This could explain why erectile dysfunction, menstrual irregularities, lactation or breast pain are not commonly reported side effects of drugs treating dravet syndrome patients, while it is for those taking antipsychotics. The impact of drugs targeting 5-HT GPCRs on sexual hormones could be a major cause of reported side effects.
The selective serotonin reuptake inhibitor, fluvoxamine, known to increase serotonin concentrations in the brain, results in a decrease in β-casein expression in breast cells (MCF-12A) (71). These results suggest that agonists to excitatory 5-HT GPCRs, which artificially promote the downstream G-protein signalling, are likely to be suppressing production of the milk protein, β-casein and thereby preventing milk production. Women who breastfeed and take antipsychotics, such as aripiprazole, could experience a delayed activation of milk secretion. We noticed that not all packet notes of antipsychotics have this warning. However, as all antipsychotics could affect milk secretion, clinicians could inform those breastfeeding and pregnant patients of the potential for delay and reduction in milk production.
In a study conducted by the Institute of Psychiatry, sexual dysfunction experienced by patients taking antipsychotics is more commonly seen in women than in men (15.2: 3.7 ratio between women and men, respectively n = 103) (71). The two major consequences affecting women and men taking 5-HT targeted drugs are the changing number of 5-HT GPCRs affected by a given oestrogen concentration and hyperprolactinemia. Infertility caused by 5-HT targeted drugs may be experienced more in women than in men due to the changing levels of oestrogen during the menstrual cycle. The risk of infertility may be heightened in women when oestrogen concentrations are low and thus excitatory 5-HT GPCRs decrease, causing reduced muscle contractions in the fallopian tube, uterus, and cervix, essential for fertility. During this time, agonists to inhibitory 5-HT GPCRs or antagonists to excitatory 5-HT GPCRs may further inhibit serotonin's influence on fertility. Hyperprolactinemia in women will affect ovule production and ovulation and manifest in men as a decrease in sperm production. Patients taking 5-HT GPCR-targeting drugs should be informed of potential infertility. Hyperprolactinemia and elevated 5-HT1d, 5-HT2b and 5-HT7 receptor activity are both linked to a higher risk of developing breast cancer due to their positive role in cell proliferation (63, 66). One of the most common side effects of 5-HT GPCR-targeting drugs is hyperprolactinemia (Supplementary Table S2). Due to the chances of infertility and developing cancer, blood prolactin levels could be monitored.
Women are subject to cycles of dynamic change in concentrations of serotonin compared to men through oestrogen hormonal activation of serotonin biosynthesis (Figure 1). Thus, when oestrogen is at its highest during ovulation until the mid-luteal phase, the endogenous serotonin concentration is expected to be relatively high. In order to reduce the side effect, agonists to excitatory 5-HT receptors or antagonists to inhibitory 5-HT receptors could be administered during the menstrual period when the oestrogen concentration is at its lowest.
Serotonin metabolism differs between men and women. Animal testing of most drugs has been conducted preferentially on male animals due to their consistency and the relatively low deviation in the results. Given that the 5-HT GPCR-targeting drugs are likely metabolised slower in women than in men, the absorption, distribution, metabolism and excretion (ADME) of each drug needs to be tested using female animals. Generally, women have a lower biological concentration of serotonin. Therefore, we should consider administering lower doses of 5-HT GPCR-targeting drugs to women.
In conclusion, more female mouse models can be used during phase II of drug development when testing for those targeting 5-HT GPCRs. Secondly, blood prolactin concentration can be monitored following administration of 5-HT GPCR-targeting drugs in both women and men. Lastly, we could take into consideration the timing of 5-HT GPCR-targeting drug administration in relation to cycling oestrogen due to the menstrual cycle in women.
Author contributions
IMJ and HO both contributed in conceiving the design of investigation and to the writing of this manuscript. All authors contributed to the article and approved the submitted version.
Acknowledgments
We would like to thank Lisa Jamu and Dr Styn Jamu for reading and editing the manuscript. We’d like to thank Professor Jeffery Hill for critically reading the manuscript.
Conflict of interest
The authors declare that the research was conducted in the absence of any commercial or financial relationships that could be construed as a potential conflict of interest.
Publisher's note
All claims expressed in this article are solely those of the authors and do not necessarily represent those of their affiliated organizations, or those of the publisher, the editors and the reviewers. Any product that may be evaluated in this article, or claim that may be made by its manufacturer, is not guaranteed or endorsed by the publisher.
Supplementary material
The Supplementary Material for this article can be found online at: https://www.frontiersin.org/articles/10.3389/fgwh.2022.1012463/full#supplementary-material.
References
1. McCorvy JD, Roth BL. Structure and function of serotonin G protein-coupled receptors. Pharmacol Ther. (2015) 150:129–42. doi: 10.1016/j.pharmthera.2015.01.009
2. Berger M, Gray JA, Roth BL. The expanded biology of serotonin. Annu Rev Med. (2009) 60:355–66. doi: 10.1146/annurev.med.60.042307.110802
3. Howes OD, Wheeler MJ, Pilowsky LS, Landau S, Murray RM, Smith S. Sexual function and gonadal hormones in patients taking antipsychotic treatment for schizophrenia or schizoaffective disorder. J Clin Psychiatry. (2007) 68(3):361–7. doi: 10.4088/JCP.v68n0302
4. Nichols DE, Nichols CD. Serotonin receptors. Chem Rev. (2008) 108(5):1614–41. doi: 10.1021/cr078224o
5. Sizemore TR, Dacks AM. Serotonergic modulation differentially targets distinct network elements within the antennal lobe of drosophila melanogaster. Sci Rep. (2016) 6:37119. doi: 10.1038/srep37119
6. Matsuda M, Imaoka T, Vomachka AJ, Gudelsky GA, Hou Z, Mistry M, et al. Serotonin regulates mammary gland development via an autocrine-paracrine loop. Dev Cell. (2004) 6(2):193–203. doi: 10.1016/S1534-5807(04)00022-X
7. Young SN, Gauthier S, Anderson GM, Purdy WC. Tryptophan, 5-hydroxyindoleacetic acid and indoleacetic acid in human cerebrospinal fluid: interrelationships and the influence of age, sex, epilepsy and anticonvulsant drugs. J Neurol Neurosurg Psychiatry. (1980) 43(5):438–45. doi: 10.1136/jnnp.43.5.438
8. Nishizawa S, Benkelfat C, Young SN, Leyton M, Mzengeza S, de Montigny C, et al. Differences between males and females in rates of serotonin synthesis in human brain. Proc Natl Acad Sci U S A. (1997) 94(10):5308–13. doi: 10.1073/pnas.94.10.5308
9. Hauser AS, Chavali S, Masuho I, Jahn LJ, Martemyanov KA, Gloriam DE, et al. Pharmacogenomics of GPCR drug targets. Cell. (2018) 172(1-2):41–54.e19. doi: 10.1016/j.cell.2017.11.033
10. Zucker I, Prendergast BJ. Sex differences in pharmacokinetics predict adverse drug reactions in women. Biol Sex Differ. (2020) 11(1):32. doi: 10.1186/s13293-020-00308-5
11. Haddad PM, Wieck A. Antipsychotic-induced hyperprolactinaemia: mechanisms, clinical features and management. Drugs. (2004) 64(20):2291–314. doi: 10.2165/00003495-200464200-00003
12. Geller SE, Koch AR, Roesch P, Filut A, Hallgren E, Carnes M. The more things change, the more they stay the same: a study to evaluate compliance with inclusion and assessment of women and minorities in randomized controlled trials. Acad Med. (2018) 93(4):630–5. doi: 10.1097/ACM.0000000000002027
13. Uhlén M, Fagerberg L, Hallström BM, Lindskog C, Oksvold P, Mardinoglu A, et al. Tissue-based map of the human proteome. Science. (2015) 347(6220):1260419. doi: 10.1126/science.1260419
14. Cao L, Hu R, Xu T, Zhang ZN, Li W, Lu J. Characterization of induced pluripotent stem cell-derived human serotonergic neurons. Front Cell Neurosci. (2017) 11:131. doi: 10.3389/fncel.2017.00131
15. Chen C, Fang F, Wang X, Lv J, Wang X, Jin H. Phenotypic and genotypic characteristics of SCN1A associated seizure diseases. Front Mol Neurosci. (2022) 15:821012. doi: 10.3389/fnmol.2022.821012
16. Herson M, Kulkarni J. Hormonal agents for the treatment of depression associated with the menopause. Drugs Aging. (2022). doi: 10.1007/s40266-022-00962-x.35908135
17. Pedersen CB, Mors O, Bertelsen A, Waltoft BL, Agerbo E, McGrath JJ, et al. A comprehensive nationwide study of the incidence rate and lifetime risk for treated mental disorders. JAMA Psychiatry. (2014) 71(5):573–81. doi: 10.1001/jamapsychiatry.2014.16
18. Culpepper L, Vieta E, Kelly DL, Patel MD, Szatmári B, Hankinson A, et al. Minimal effects of cariprazine on prolactin levels in bipolar disorder and schizophrenia. Neuropsychiatr Dis Treat. (2022) 18:995–1011. doi: 10.2147/NDT.S348143
19. Garthwaite TL, Hagen TC. Evidence that serotonin stimulates a prolactin-releasing factor in the rat. Neuroendocrinology. (1979) 29(3):215–20. doi: 10.1159/000122925
20. Thapa S, Bhusal K. Hyperprolactinemia. StatPearls. Treasure Island (FL): StatPearls Publishing Copyright © 2022, StatPearls Publishing LLC (2022).
21. Balsa JA, Sánchez-Franco F, Pazos F, Lara JI, Lorenzo MJ, Maldonado G, et al. Direct action of serotonin on prolactin, growth hormone, corticotropin and luteinizing hormone release in cocultures of anterior and posterior pituitary lobes: autocrine and/or paracrine action of vasoactive intestinal peptide. Neuroendocrinology. (1998) 68(5):326–33. doi: 10.1159/000054381
22. Calama E, Fernández MM, Morán A, Martín ML, San Román L. Vasodilator and vasoconstrictor responses induced by 5-hydroxytryptamine in the in situ blood autoperfused hindquarters of the anaesthetized rat. Naunyn Schmiedebergs Arch Pharmacol. (2002) 366(2):110–6. doi: 10.1007/s00210-002-0579-1
23. Hamel E. Serotonin and migraine: biology and clinical implications. Cephalalgia. (2007) 27(11):1293–300. doi: 10.1111/j.1468-2982.2007.01476.x
24. Bethea CL, Mirkes SJ, Shively CA, Adams MR. Steroid regulation of tryptophan hydroxylase protein in the dorsal raphe of macaques. Biol Psychiatry. (2000) 47(6):562–76. doi: 10.1016/S0006-3223(99)00156-0
25. Gundlah C, Alves SE, Clark JA, Pai LY, Schaeffer JM, Rohrer SP. Estrogen receptor-beta regulates tryptophan hydroxylase-1 expression in the murine midbrain raphe. Biol Psychiatry. (2005) 57(8):938–42. doi: 10.1016/j.biopsych.2005.01.014
26. Hiroi R, Handa RJ. Estrogen receptor-β regulates human tryptophan hydroxylase-2 through an estrogen response element in the 5′ untranslated region. J Neurochem. (2013) 127(4):487–95. doi: 10.1111/jnc.12401
27. Cyr M, Bossé R, Di Paolo T. Gonadal hormones modulate 5-hydroxytryptamine2A receptors: emphasis on the rat frontal cortex. Neuroscience. (1998) 83(3):829–36. doi: 10.1016/S0306-4522(97)00445-4
28. Moses EL, Drevets WC, Smith G, Mathis CA, Kalro BN, Butters MA, et al. Effects of estradiol and progesterone administration on human serotonin 2A receptor binding: a PET study. Biol Psychiatry. (2000) 48(8):854–60. doi: 10.1016/S0006-3223(00)00967-7
29. Lu NZ, Bethea CL. Ovarian steroid regulation of 5-HT1A receptor binding and G protein activation in female monkeys. Neuropsychopharmacology. (2002) 27(1):12–24. doi: 10.1016/S0893-133X(01)00423-7
30. Safe S. Transcriptional activation of genes by 17 beta-estradiol through estrogen receptor-Sp1 interactions. Vitam Horm. (2001) 62:231–52. doi: 10.1016/S0083-6729(01)62006-5
31. Wissink S, van der Burg B, Katzenellenbogen BS, van der Saag PT. Synergistic activation of the serotonin-1A receptor by nuclear factor-kappa B and estrogen. Mol Endocrinol. (2001) 15(4):543–52. doi: 10.1210/mend.15.4.0629
32. Sumner BE, Fink G. Testosterone as well as estrogen increases serotonin2A receptor mRNA and binding site densities in the Male rat brain. Brain Res Mol Brain Res. (1998) 59(2):205–14. doi: 10.1016/S0169-328X(98)00148-X
33. Stull MA, Pai V, Vomachka AJ, Marshall AM, Jacob GA, Horseman ND. Mammary gland homeostasis employs serotonergic regulation of epithelial tight junctions. Proc Natl Acad Sci U S A. (2007) 104(42):16708–13. doi: 10.1073/pnas.0708136104
34. Kolb AF, Huber RC, Lillico SG, Carlisle A, Robinson CJ, Neil C, et al. Milk lacking α-casein leads to permanent reduction in body size in mice. PLoS One. (2011) 6(7):e21775. doi: 10.1371/journal.pone.0021775
35. Chiba T, Kimura S, Takahashi K, Morimoto Y, Maeda T, Sanbe A, et al. Serotonin regulates β-casein expression via 5-HT7 receptors in human mammary epithelial MCF-12A cells. Biol Pharm Bull. (2015) 38(3):448–53. doi: 10.1248/bpb.b14-00723
36. Chiba T, Kimura S, Takahashi K, Morimoto Y, Sanbe A, Ueda H, et al. Serotonin suppresses β-casein expression via inhibition of the signal transducer and activator of transcription 5 (STAT5) protein phosphorylation in human mammary epithelial cells MCF-12A. Biol Pharm Bull. (2014) 37(8):1336–40. doi: 10.1248/bpb.b14-00273
37. Bulletti C, de Ziegler D, Polli V, Diotallevi L, Del Ferro E, Flamigni C. Uterine contractility during the menstrual cycle. Hum Reprod. (2000) 15(Suppl 1):81–9. doi: 10.1093/humrep/15.suppl_1.81
38. Oropeza MV, Ponce Monter H, Reynoso Isla M, Campos MG. The ovarian and cervical regions of the rat uterus display a different contractile response to serotonin and prostaglandin F2alpha. I. The estrous cycle. Life Sci. (2000) 66(25):Pl345–51. doi: 10.1016/S0024-3205(00)80010-7
39. Kissler S, Siebzehnruebl E, Kohl J, Mueller A, Hamscho N, Gaetje R, et al. Uterine contractility and directed sperm transport assessed by hysterosalpingoscintigraphy (HSSG) and intrauterine pressure (IUP) measurement. Acta Obstet Gynecol Scand. (2004) 83(4):369–74. doi: 10.1111/j.0001-6349.2004.00412.x
40. Mueller A, Siemer J, Schreiner S, Koesztner H, Hoffmann I, Binder H, et al. Role of estrogen and progesterone in the regulation of uterine peristalsis: results from perfused non-pregnant swine uteri. Hum Reprod. (2006) 21(7):1863–8. doi: 10.1093/humrep/del056
41. Minosyan TY, Lu R, Eghbali M, Toro L, Stefani E. Increased 5-HT contractile response in late pregnant rat myometrium is associated with a higher density of 5-HT2A receptors. J Physiol. (2007) 581(Pt 1):91–7. doi: 10.1113/jphysiol.2007.129726
42. Cordeaux Y, Pasupathy D, Bacon J, Charnock-Jones DS, Smith GC. Characterization of serotonin receptors in pregnant human myometrium. J Pharmacol Exp Ther. (2009) 328(3):682–91. doi: 10.1124/jpet.108.143040
43. Inoue M, Kitazawa T, Cao J, Taneike T. 5-HT7 receptor-mediated relaxation of the oviduct in nonpregnant proestrus pigs. Eur J Pharmacol. (2003) 461(2-3):207–18. doi: 10.1016/S0014-2999(03)01312-8
44. Amireault P, Dube F. Intracellular cAMP and calcium signaling by serotonin in mouse cumulus-oocyte complexes. Mol Pharmacol. (2005) 68(6):1678–87. doi: 10.1124/mol.104.010124
45. Díaz-Ramos J, Flores-Flores M, Ayala ME, Aragón-Martínez A. Impaired serotonin communication during juvenile development in rats diminishes adult sperm quality. Syst Biol Reprod Med. (2018) 64(5):340–7. doi: 10.1080/19396368.2018.1472825
46. Ishigami T, Yoshioka K, Karicheti V, Marson L. A role for peripheral 5-HT2 receptors in serotonin-induced facilitation of the expulsion phase of ejaculation in Male rats. J Sex Med. (2013) 10(11):2688–702. doi: 10.1111/jsm.12306
47. Pomerantz SM, Hepner BC, Wertz JM. 5-HT1A And 5-HT1C/1D receptor agonists produce reciprocal effects on Male sexual behavior of rhesus monkeys. Eur J Pharmacol. (1993) 243(3):227–34. doi: 10.1016/0014-2999(93)90179-L
48. Chaiseha Y, Kang SW, Leclerc B, Kosonsiriluk S, Sartsoongnoen N, El Halawani ME. Serotonin receptor subtypes influence prolactin secretion in the Turkey. Gen Comp Endocrinol. (2010) 165(1):170–5. doi: 10.1016/j.ygcen.2009.06.018
49. Administration USFaD. Drugs@FDA: FDA-Approved Drugs. Available at: https://www.accessdata.fda.gov/scripts/cder/daf/index.cfm
50. Wishart DS, Knox C, Guo AC, Shrivastava S, Hassanali M, Stothard P, et al. Drugbank: a comprehensive resource for in silico drug discovery and exploration. Nucleic Acids Res. (2006) 34(Database issue):D668–72. doi: 10.1093/nar/gkj067
51. Kuhn M, Letunic I, Jensen LJ, Bork P. The SIDER database of drugs and side effects. Nucleic Acids Res. (2016) 44(D1):D1075–9. doi: 10.1093/nar/gkv1075
53. Stroup TS, Gray N. Management of common adverse effects of antipsychotic medications. World Psychiatry. (2018) 17(3):341–56. doi: 10.1002/wps.20567
54. Nagapurkar U, Andrade C. Ejaculatory dysfunction associated with low dose amisulpride. Indian J Psychiatry. (2013) 55(1):97. doi: 10.4103/0019-5545.105530
55. Calabrese JR, Sanchez R, Jin N, Amatniek J, Cox K, Johnson B, et al. Efficacy and safety of aripiprazole once-monthly in the maintenance treatment of bipolar I disorder: a double-blind, placebo-controlled, 52-week randomized withdrawal study. J Clin Psychiatry. (2017) 78(3):324–31. doi: 10.4088/JCP.16m11201
56. Dutta A, Barua S, Dan A, Chakraborty K, Mandal M. Iloperidone-induced galactorrhea in a middle-aged female. Indian J Psychol Med. (2012) 34(4):396–8. doi: 10.4103/0253-7176.108233
57. Javed A, Arthur H, Curtis L, Hansen L, Pappa S. Practical guidance on the use of lurasidone for the treatment of adults with schizophrenia. Neurol Ther. (2019) 8(2):215–30. doi: 10.1007/s40120-019-0138-z
58. Uguz F, Sahingoz M, Kose SA, Ozbebit O, Sengul C, Selvi Y, et al. Antidepressants and menstruation disorders in women: a cross-sectional study in three centers. Gen Hosp Psychiatry. (2012) 34(5):529–33. doi: 10.1016/j.genhosppsych.2012.03.014
59. Curran DA, Lance JW. Clinical trial of methysergide and other preparations in the management of migraine. J Neurol Neurosurg Psychiatry. (1964) 27(5):463–9. doi: 10.1136/jnnp.27.5.463
60. Al-Chalabi M, Bass AN, Alsalman I. Physiology, prolactin. StatPearls. Treasure Island (FL): StatPearls Publishing Copyright © 2021, StatPearls Publishing LLC (2021).
61. Peuskens J. Risperidone in the treatment of patients with chronic schizophrenia: a multi-national, multi-centre, double-blind, parallel-group study versus haloperidol. Risperidone study group. Br J Psychiatry. (1995) 166(6):712–26; discussion 727–33. doi: 10.1192/bjp.166.6.712
62. Van Coppenolle F, Skryma R, Ouadid-Ahidouch H, Slomianny C, Roudbaraki M, Delcourt P, et al. Prolactin stimulates cell proliferation through a long form of prolactin receptor and K+ channel activation. Biochem J. (2004) 377(Pt 3):569–78. doi: 10.1042/bj20030859
63. Clevenger CV, Furth PA, Hankinson SE, Schuler LA. The role of prolactin in mammary carcinoma. Endocr Rev. (2003) 24(1):1–27. doi: 10.1210/er.2001-0036
64. Busby J, Mills K, Zhang SD, Liberante FG, Cardwell CR. Selective serotonin reuptake inhibitor use and breast cancer survival: a population-based cohort study. Breast Cancer Res. (2018) 20(1):4. doi: 10.1186/s13058-017-0928-0
65. Milosavljević JZ, Milosavljević MN, Arsenijević PS, Milentijević MN, Stefanović SM. The effects of selective serotonin reuptake inhibitors on Male and female fertility: a brief literature review. Int J Psychiatry Clin Pract. (2022) 26(1):43–9. doi: 10.1080/13651501.2021.1872647
66. Pai VP, Marshall AM, Hernandez LL, Buckley AR, Horseman ND. Altered serotonin physiology in human breast cancers favors paradoxical growth and cell survival. Breast Cancer Res. (2009) 11(6):R81. doi: 10.1186/bcr2448
67. Sonigo C, Bouilly J, Carré N, Tolle V, Caraty A, Tello J, et al. Hyperprolactinemia-induced ovarian acyclicity is reversed by kisspeptin administration. J Clin Invest. (2012) 122(10):3791–5. doi: 10.1172/JCI63937
68. Milosavljević MN, Janković SV, Janković SM, Djurić J, Protrka Z, Arsenijević S, et al. Effects of selective serotonin reuptake inhibitors on motility of isolated fallopian tube. Clin Exp Pharmacol Physiol. (2019) 46(8):780–7. doi: 10.1111/1440-1681.13118
69. Lyons RA, Saridogan E, Djahanbakhch O. The reproductive significance of human fallopian tube cilia. Hum Reprod Update. (2006) 12(4):363–72. doi: 10.1093/humupd/dml012
70. Martin RM, Biswas PN, Freemantle SN, Pearce GL, Mann RD. Age and sex distribution of suspected adverse drug reactions to newly marketed drugs in general practice in England: analysis of 48 cohort studies. Br J Clin Pharmacol. (1998) 46(5):505–11. doi: 10.1046/j.1365-2125.1998.00817.x
Keywords: serotonin 5-HT, GPCR (G protein coupled receptor), CNS—central nervous system, PNS—peripheral nervous system, tryptophane hydroxyrase (TPH), oestrogen (E) 2, prolactin
Citation: Jamu IM and Okamoto H (2022) Recent advances in understanding adverse effects associated with drugs targeting the serotonin receptor, 5-HT GPCR. Front. Glob. Womens Health 3:1012463. doi: 10.3389/fgwh.2022.1012463
Received: 5 August 2022; Accepted: 14 November 2022;
Published: 8 December 2022.
Edited by:
Sanne AE Peters, University of Oxford, United KingdomReviewed by:
Deborah Rudin, University Hospital of Basel, SwitzerlandKevin James Cummings, University of Missouri, United States
© 2022 Jamu and Okamoto. This is an open-access article distributed under the terms of the Creative Commons Attribution License (CC BY). The use, distribution or reproduction in other forums is permitted, provided the original author(s) and the copyright owner(s) are credited and that the original publication in this journal is cited, in accordance with accepted academic practice. No use, distribution or reproduction is permitted which does not comply with these terms.
*Correspondence: Haruko Okamoto h.okamoto@sussex.ac.uk
Specialty Section: This article was submitted to Sex and Gender Differences in Disease, a section of the journal Frontiers in Global Women's Health