- 1Plant Pathology Laboratory, School of Plant and Environmental Sciences, Alson H. Smith Jr. Agricultural Research and Extension Center, Virginia Polytechnic Institute and State University, Winchester, VA, United States
- 2Hudson Valley Research Laboratory, School of Integrative Plant Sciences, Plant Pathology and Plant-Microbe Biology Section, Cornell University, Highland, NY, United States
With the goal to develop management tools to mitigate the severity of shoot blight on pear caused by Erwinia amylovora and prevent fire blight canker development from infected shoots on pear tree wood, we evaluated five preventive treatments in inoculated experiments over 2-years. We focused on foliar spray and trunk injection applications of extract of giant knotweed, Reynoutria sachalinensis (RSE), as a plant resistance activator candidate and on comparisons to antibiotics. Five foliar applications of RSE (2.24 L/ha) on 6-year-old trees of cultivar ‘Bartlett’ resulted in 100% control of shoot blight severity and canker incidence in both years of evaluation. The RSE trunk injection in fall of the first year did not control the disease allowing 75% of shoot severity and 38.5% of canker incidence. The opposite happened in the second year, when we injected RSE in spring, providing 85.7% control of shoot blight severity and 89.8% control of canker incidence. Antibiotics oxytetracycline (OTC) and streptomycin (STM) did not provide consistent control in the second year in comparison to the first year. In the first year, both antibiotics, OTC (trunk injection and foliar spray) and STM were effective, providing 94.8%, 100% and 86.3% control of shoot blight, respectively. They also provided 100, 91 and 84% control of canker incidence, respectively. However, in the second they were not effective allowing 69 – 96% of shoot blight severity and 70 – 92% of canker incidence. Regardless of the inconsistency in the effectiveness of antibiotics, which are not recommended for shoot blight control due to risks of promoting antibiotic resistance, five preventive spray applications of RSE could be used on pear trees to prevent shoot blight and canker development. Thus, the RSE spray program we developed could serve as a valuable control tool that could help prevent sudden outbreaks of shoot blight during summer and pear tree death from fire blight cankers with no risk for pathogen resistance.
1 Introduction
Fire blight disease of pear is caused by the bacterium Erwinia amylovora (Burril; Winslow et al., 1920). It is one of the most important and enigmatic diseases of pome fruit with a wide range of hosts, including many pome fruit and ornamental plants like apple, pear, crabapple, mountain ash, shadbush, firethorn, Chaenomeles spp. and others (Van Der Zwet and Beer, 1999). The disease is more damaging on pears than apples, often leading to tree death in one season (Van Der Zwet and Beer, 1999). The life cycle of this disease is highly dependent on warm and wet conditions in spring (Steiner, 1989; Smith, 2000; Smith and Pusey, 2011; Turechek and Biggs, 2015). E. amylovora overwinters in fire blight cankers, which are necrotized patches of bark on perennial wood developed after previous year infections. In spring, the pathogen produces droplets of orange bacterial ooze that emerge on the canker. Ooze attracts vectors such as insects and birds but is also carried by wind, rain, contaminated pruning tools, and infected plant material to new infection sites (Van Der Zwet and Keil, 1979; Griffith et al., 2003; Paraschivu et al., 2021). After reaching the flower stigmas on pome fruit hosts, where it grows its populations, or surface of young shoots, rain or tissue injury facilitates infection, and the pathogen causes blossom and shoot blight. After E. amylovora invades wood tissues from infected flowers and shoots, new fire blight cankers develop on wood, thus repeating the life cycle (Aćimović et al., 2014a, Aćimović et al., 2019a). However, even though cankers have great importance in disease epidemiology, understanding the parts of E. amylovora life cycle on shoots and in fire blight cankers is still limited (Santander et al., 2022a).
Among the wide-spread pear cultivars commonly planted in key production regions, there are no varieties entirely resistant to fire blight. The commercial varieties with the most desirable fruit texture and flavor generally display high susceptibility to infection and destruction by fire blight (Van Der Zwet and Beer, 1995; Montanari et al., 2016; Mertoğlu and Evrenososglu, 2017; Paraschivu et al., 2020). In recent work, ‘Bartlett’ was classified as highly susceptible cultivar to this disease (Santander et al., 2022a), with many other popular commercial pear cultivars like ‘Bosc’, ‘Comice’, and ‘D’Anjou’ being very susceptible to extremely susceptible to fire blight. Overall, bacterial diseases on tree fruit, including fire blight, are harder to control in comparison to fungal diseases. There are much fewer market available bactericides in comparison to fungicides for control of phytopathogenic fungi. In contrast to fungal plant diseases, to control a bacterial plant disease a combination of multiple control approaches and methods is necessary (Agrios, 2005). For example, control of fire blight usually involves planting resistant cultivars, spray application of copper bactericides, antibiotics, biological materials, activators of Systemic Acquire Resistance (SAR), shoot growth retardants, and mechanical removal of infected tree parts by pruning or whole tree removal (Johnson and Temple, 2013; Johnson and Temple, 2015; Wallis and Cox, 2019; Aćimović et al., 2021; Philion and Joubert, 2021).
Pear production world-wide has been in decline for decades due to severe economic impacts and difficulties in management of fire blight and pear psylla (Psylla pyri Linnaeus) (Bonn and van der Zwet, 2000; Gaaliche et al., 2018). Fire blight can kill whole pear and young apple trees in one growing season (Breth, 2008). This disease has the potential to decimate an entire orchard rapidly (Norelli, 2003; Aćimović and Meredith, 2017). The economic losses from fire blight in the United States (U.S.) are estimated to exceed over $100 million annually (Wallis and Cox, 2020). For example, fire blight epidemic in southwest Michigan in 2000 led to over 220,000 dead trees, which accounted for $42 million in economic losses (Longstroth, 2001). An epidemic in 2016 in northern New York resulted in over $16 million in losses on apples (Aćimović et al., 2021). These devastating fire blight outbreaks are becoming more common in the past decade, which could be explained by global climate change (Coakley et al., 1999; Frumhoff et al., 2007; Hayhoe et al., 2007; Melillo et al., 2014). High temperatures, frequent rainfall events, or much earlier season infections than usual can lead to more damaging epidemics (Eastburn et al., 2011). Changes in the frequency and intensity of extreme weather events, such as flooding, high average temperature, and atmospheric carbon dioxide heighten plant susceptibility to pathogens (Dossa et al., 2015). These conditions can either improve or create conditions for developing and disseminating plant pathogenic organisms (Bebber, 2015).
Commercial pear production in the U.S. is concentrated in the Pacific Northwest, led by three states, Washington, Oregon, and California. In 2019, these three states were responsible for producing 512,540 tons of fresh pears, equivalent to $238,454 million in value, and in 2021 had an increase of over 9.7% (562,470 tons), equal to $321,562 million in value (USDA NASS, 2022). During the seasons of 2021 and 2022, the U.S. exported 156,134 million pounds of fresh pears, equivalent to $108,774 million (USDA ERS, 2022). Pears are the second most planted pome fruit in the U.S., encompassing around 45,000 A, with 75% of that production in the Pacific Northwest and a value of up to $457.1 million annually. In recent decades, high-density plantings have increased acreage for apple and pear orchards (Mass, 2008; Elkins et al., 2008). High-density orchards have many economic benefits compared to low-density orchards, including higher yields, quicker return on investments, more efficient utilization of pesticide and labor inputs, and improved fruit quality (Norelli et al., 2003). However, the combination of the costs of implementing a high-density orchard and the high susceptibility of pears to the fire blight can result in significant financial damage after a fire blight epidemic. For example, in Washington, just in 2017, pome fruit growers removed 194 acres of pear and 300 acres of apple due to fire blight favored by warm and wet spring (DuPont 2021, personal communication). The costs of removal varied from $27 to $864 per acre. Fire blight remains the top concern for growers nationally, with control costs increasing to $500 per acre per year (FPPCR - Fresh & Processed Pear Committee Research, 2021; NY ARDP, 2021; VARP - Virginia Apple Research Committee, 2021; WTFRC, 2021a). For the last 5 years, Washington Tree Fruit Research Commission (WTFRC, 2021b), Fresh & Processed Pear Committee Research (FPPCR) and Virginia Apple Research Program (VARP - Virginia Apple Research Committee, 2021) have listed the following areas as the top research priorities for industry groups in their annual request for research proposals: Fire blight, apple: 1. Build program options for growers (focus on entire season and weather events), 2. Product efficacy testing of new materials and developing SOPs for optimized efficacy (especially focusing on shoot blight control, getting to the end of the season, orchard sanitation, and new materials), 3. Management of resistance to antibiotics; Fire blight, pear: Alternative products for mid-season control and/or reduced preharvest intervals for existing products - infections becoming more chronic than occasional, need organic control options that don’t russet fruit (FPPCR - Fresh & Processed Pear Committee Research, 2021).
Nowadays, the synthetic compounds for control of fire blight are limited and the management is difficult due to the occurrence of E. amylovora strains resistant to streptomycin (McGhee and Sundin, 2011; Tancos et al., 2016a; Wallis et al., 2021). Kasugamycin (Kasumin 2L, EPA Reg. No. 66330-404, UPL Ltd. Mumbai, India) is used in few locations in the U.S.A. where streptomycin resistance in E. amylovora is reported. To control shoot blight and reduce fire blight cankers on apple trees, the plant growth regulator (PGR) prohexadione-calcium (PCA, Apogee, EPA Reg. No. 7969-188, BASF Corporation, Ludwigshafen, Germany; Kudos, EPA Reg. No. 62097-41-82917 Fine Americas Inc, Walnut Creek, CA) and the systemic acquired resistance (SAR) activator such as acibenzolar-S-methyl (ASM, Actigard, Syngenta, Basel, Switzerland) can be used (Aćimović et al., 2021). PCA inhibits the late steps of gibberellin biosynthesis, and by reducing the shoot growth and thickening the cell walls in cortical parenchyma, it reduces the incidence and severity of shoot blight (McGrath et al., 2009). SAR activators induce plant immunity synthetic pathways and result in a myriad of synthesized and accumulated defensive compounds, including antimicrobial proteins, that help the plant suppress the disease (Hammerschmidt, 2007; Aćimović and Meredith, 2017).
Aćimović et al. (2019b; 2021) showed that a high rate of PCA (247 mg/liter) applied 2 to 3 days after shoot infection reduced shoot blight severity on mature apple trees by 89.5 and 69.5%. Further, the same rate prevented E. amylovora invasion of wood from shoots (100% control), thus preventing cankers from developing on wood (Aćimović et al., 2019b; Aćimović et al., 2021). However, there are no spray materials that could provide a similar effect of reducing shoot blight and preventing cankers on pear trees. It has been reported that PCA can negatively affect pear fruit bearing by reducing fruit size on ‘Bartlett’ and return bloom on ‘Bosc’ (Smit et al., 2005; Elfving, Sugar, and Mielke, loc. cit Good Fruit Grower, 2013). Studies have shown that PCA application can increase the fruit set in apples and reduce average individual fruit weight (Sugar et al., 2004; Greene, 2008). PCA is not used in pear orchards in the US due to these side effects, including more post-harvest shoot growth flushes and lack of further research. Previously, we have shown that two early spring applications of extract of giant knotweed, Reynoutria sachalinensis (Regalia, EPA Reg. No. 84059-3, Marrone Bio Innovations, Davis, CA, U.S.A.), with a cumulative rate of 11.2 L/ha, provided 36% control of shoot blight incidence on mature apple trees of cultivar ‘Honeycrisp’ (Aćimović and Meredith, 2017). R. sachalinensis extract probably activates SAR in the host, which leads to disease reduction. However, the full potential of this extract and SAR candidate is unexplored but promising for conventional and organic apple and pear production. If effective, R. sachalinensis extract could aid in prevention of sudden outbreaks of shoot blight, which lead to development of cankers.
With the gap of no synthetic or natural compounds similar to PCA that can be used in pear for shoot blight and canker management, the objective of this study was to determine if the preventive applications of R. sachalinensis extract can reduce pear shoot blight severity and prevent the resulting canker development on pear wood, repeating the success we had on apple (Aćimović and Meredith, 2017). Our goals were to deliver the total cumulative rate of 11.2 L/ha in 5 consecutive spray applications of 2.24 L/ha on pear in spring and prove the consistency of the effects by evaluating for two years. Additionally, we wanted to compare the effect of R. sachalinensis extract to antibiotics, even though they are not recommended for managing shoot blight in commercial orchards due to higher risks of antibiotic resistance from summer applications. We hypothesized that a reduction in shoot blight severity by Regalia will slow down or stop E. amylovora invasion via shoots and thus reduce or prevent the initiation of fire blight cankers on wood. We aimed to develop a new spray program for use in conventional and organic pear production that could provide a similar effect as PCA on apple shoots and cankers and become a tool for pear fruit growers to combat shoot blight and prevent tree death from cankers.
2 Material and methods
2.1 Bacterial strain and inoculum preparation
The inoculations were performed using the American E. amylovora strain Ea273 grown overnight in Luria–Bertani broth (Bertani, 1951) using a shaker incubator set at 28°C. We prepared the inoculum in 10 mM phosphate buffered saline (PBS) solution, pH 7.4, adjusting cell suspensions to approximately 2 x 108 CFU/ml using a DEN-1 McFarland Densitometer (Grant Instruments, Shepreth, Royston, UK) and McFarland Standard calibration set 0.5-0.4 (Pro-Lab Diagnostics, Round Rock, TX, U.S.A.). Colony plate counts were performed to confirm the suspension concentrations. In 2019, colony plate counts established that we used 1.94 x 108 CFU/ml for inoculation on May 26. In 2022, colony plate counts validated that on May 11, we inoculated with a suspension concentration of 2.97 × 109 CFU/ml. Both concentrations are within the common range used for shoot blight control and canker incidence evaluations (Johnson and Temple, 2016; Aćimović et al., 2021).
2.2 Plant material and shoot inoculations
Pear orchard experiments were performed at Cornell University’s Hudson Valley Research Laboratory in Highland, NY, U.S.A., in 2019 (N41° 45 ‘ 1.594’’, W73° 58 ‘ 3.941’’) and at Virginia Tech’s Alson H. Smith Jr. Agricultural Research and Extension Center in Winchester, VA, U.S.A, in 2022 (N 39° 6’ 40”, W 78° 16’ 49”). In 2019 we used a block of 6-year-old trees of Pyrus communis cultivar ‘Bartlett’, planted with 2 m between trees and 4.5 m between rows. In 2022 we used a block of 6-year-old trees of P. communis, also cultivar ‘Bartlett’, which we planted at 3 m between trees and 5 m between rows. The soil fertilization schedule in Highland, NY, was as follows: on May 8, 2016, 40 lbs. of nitrogen, phosphorus, and potassium (NPK) 15-0-30 with B was applied to the block delivering 41.9 kg N/ha, on April 25, 2017, 50 lbs. NPK 15-0-30 with B was applied to the block, delivering 52.3 kg N/ha on May 9, 2018, 50 lbs. NPK 15-0-30 with B was applied to the block, delivering 52.3 kg N/ha, on April 16, 2019, 40 lbs. of a mixture of fertilizers yielding NPK 25-3-3 (no B) was applied to the block, delivering 69.8 kg N/ha. In Winchester, pear trees were planted in early March 2022 and were not fertilized.
In 2019 and 2022, we inoculated shoots by a stem cut inoculation method on May 26 and May 11, respectively, as per Santander et al. (2019; 2022a; 2022b) by delivering 40 μL of E. amylovora suspension in PBS per shoot, adjusted to 2 x 108 CFU/ml using the micropipette. The inoculum suspension was cooled on ice until the inoculation. A total of 10 selected shoots pear each pear tree in each treatment were inoculated. In 2019, we inoculated 15 trees, three replicates for each treatment plus three untreated replicates were also inoculated, serving as a control. In 2022 we inoculated 20 trees, four replicates for each treatment, plus four untreated tree replicates were also inoculated, serving as a control. The three and four tree replicates used as untreated controls for 2019 and 2022, respectively, were inoculated in the same way as in treatments 1 to 5 but did not receive any chemicals.
2.3 Experimental design, treatments, and application timing
An orchard experiment with spray treatments of Regalia (extract of R. sachalinensis), FireLine (oxytetracycline), FireWall (streptomycin), and one untreated control was conducted (Table 1). Also, we included two trunk injection treatments of Regalia and Arbor-OTC (oxytetracycline) to explore improving efficacy of Regalia’s as a candidate activator of Systemic Acquired Resistance. Injections were applied using a Quik-jet microinjection system (Arborjet Inc., Woburn, MA) operating at hand-generated hydraulic pressure to deliver low volumes of liquid into the tree trunk, thus enabling faster application times (Aćimović, 2014). The treatments were applied between swollen bud and fruit set growth stages, but before the inoculation with E. amylovora. Due to cold weather conditions in early spring 2022 suppressing floral bud development and the abrupt switch to warm weather in mid-spring (Supplement Figure 1), Regalia spray application at white bud stage in treatment number 3 was not conducted because the transition from green cluster to bloom was in less than 24 h due to high air temperatures. To consistently deliver the same cumulative rate of Regalia in treatment 3 (11.2 L/ha) in both experiment years, the fifth spray application of Regalia in treatment 3 was performed at the stage fruit set II and as the only application after shoot inoculation in 2022 (Table 1). In the 2019 experiment, Regalia trunk injections were performed on October 10, 2018, while for the 2022 experiment, they were conducted at bud burst in 2022. All the spray treatments were spray applied dilute to drip (935.4 L/ha) using a tractor-carrier sprayer with a brass handgun (Pak-Tank 4 × 25-gal tanks, 250 PSI, Rear’s Manufacturing, Coburg, OR) to secure good coverage with the spray solution.
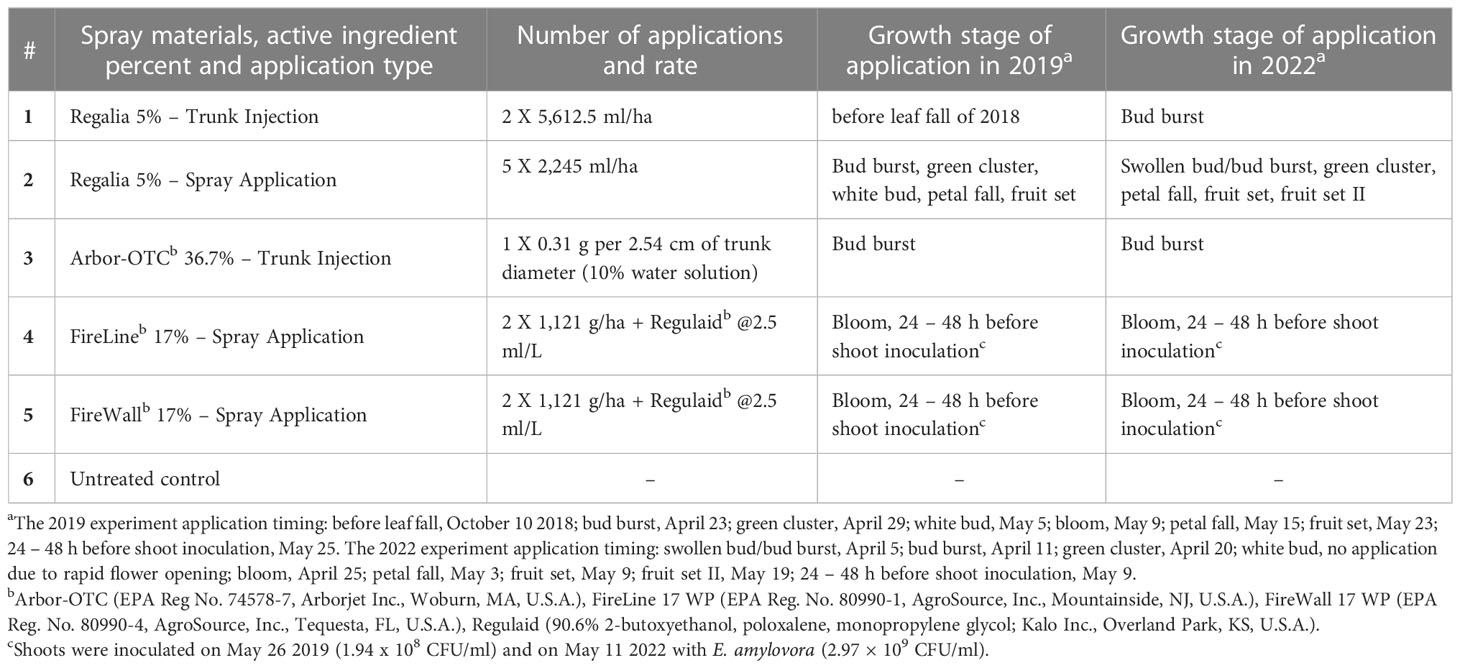
Table 1 Preventive spray and trunk-injection treatments for fire blight management evaluated in 2019 and 2022 with 5% extract of giant knotweed, R. sachalinensis (Regalia; EPA Reg. No. 84059-3), and two antibiotics, streptomycin (FireWall) and oxytetracycline (ArborOTC, FireLine) to reduce shoot blight severity and initiation of fire blight cankers on perennial wood of pear cultivar ‘Bartlett’.
2.4 Measurement of shoot blight severity, canker incidence, and canker severity
For each inoculated shoot, we calculated shoot blight severity percent by multiplying the ratio of necrotic shoot length, i.e., fire blight lesion length (cm), to the total shoot length (cm), by 100. Fire blight canker incidence, resulting from E. amylovora invasion of bark on perennial wood from the infected shoots, was calculated as the percentage of cankers that developed on wood after shoot inoculations in relation to the total number of inoculated shoots. For each canker developed on perennial pear wood from inoculated shoots, we measured canker severity, i.e., canker length (cm). In 2019 we rated the shoot blight severity (%), canker incidence (%), and canker length (in centimeters) only on June 23. In 2022, we measured the shoot blight severity, canker incidence, and canker length on the same or from the same shoots on May 31, July 1, and August 1, 2022. Mean shoot blight severity percent, mean percent of initiated cankers on perennial pear wood (canker incidence), and mean canker length were calculated from 10 shoot replicates and expressed per each replicate tree. Mean shoot blight severity, mean canker incidence percent, and mean canker length for each treatment were calculated from the three (2019) and four (2022) replicate tree means.
2.5 Statistical analysis
The treatment was considered a fixed factor. The response variables analyzed by the ANOVA on the tree as the experimental unit were shoot blight severity (as a percentage), fire blight canker incidence (as a percentage), and canker length (in centimeters). Random variables were the error associated with the tree as an experimental unit. For the 2022 data set, the ANOVA test was conducted separately for each time point for consistency of data presentation and easier comparison to the 2019 data set (P < 0.05). All data were analyzed using the MIXED procedure in SAS ver. 9.4 (SAS Institute, Cary, NC, U.S.A.).
2.5.1 Data transformation
Prior to final treatment analysis, raw data were tested for normal distribution of residuals (Shapiro-Wilk test; normal probability plot of the residuals) and equal variances of residuals (Levene’s test; box plot of residual variances, residual vs. predicted plot), which are necessary to fulfil the assumptions of ANOVA (F-test).
Shoot blight severity data in 2019 were used untransformed, as all the assumptions of ANOVA were fulfilled, including the normal distribution of residuals and equal variances of residuals. Shoot blight severity data from the first time point in 2022 were arcsine-transformed to equalize the variances of residuals, thus fulfilling all the assumptions of ANOVA. The 2022 data on shoot blight severity from the second time point were arcsine-transformed to normalize the distribution of residuals, thus fulfilling all the assumptions of ANOVA. The 2022 data on shoot blight severity from the third time point were analyzed untransformed as the original data had equal variances and normal distribution of residuals (Aćimović et al., 2021).
The 2019 data on canker incidence were square root-transformed to equalize the variances of residuals, thus fulfilling all the assumptions of ANOVA. The 2022 data on canker incidence from the first time point were log-transformed to equalize the variances of residuals, thus fulfilling all the assumptions of ANOVA. The 2022 data on canker incidence from the second time point were square root-transformed to equalize the variances of residuals, thus fulfilling all the assumptions of ANOVA. The 2022 data on canker incidence from the third time point were arcsine-transformed to normalize the distribution of residuals thus fulfilling all the assumptions of ANOVA.
The canker length data from 2019 were square root-transformed to normalize the distribution of residuals thus fulfilling all the assumptions of ANOVA. The 2022 data on canker length from the first time point were log-transformed to equalize the variances of residuals thus fulfilling all the assumptions of ANOVA. The 2022 data on canker length from the second time point were log-transformed to normalize the distribution of residuals thus fulfilling all the assumptions of ANOVA. The 2022 data on canker length from the third time point log-transformed to normalize the distribution of residuals thus fulfilling all the assumptions of ANOVA.
2.5.2 Treatment comparisons
All pairwise comparisons of treatments were performed using the LSD post-hoc test (p < 0.05) to determine the significant differences between the treatments. Means separation for one-time point in 2019 and for each time point separately in 2022 were conducted by hand-drawing lines connecting the means of similar spray treatments and assigning letters according to these lines. In 2019, each treatment mean consisted of three replicate trees, with each replicate tree mean consisting of 10 shoots per tree, and each mean shown in the form of a histogram. In 2022, each treatment mean consisted of four replicate trees, with each tree replicate mean consisting of 10 shoots per tree, and each mean shown in a form of a histogram. Error bars in the histograms represent the standard error of the mean calculated from the original raw data.
3 Results
3.1 Reduction/prevention of shoot blight severity
Shoot blight severity of the inoculated untreated control trees from both years (treatment 6) reached 80.9 and 92.4%, respectively (Figures 1, 2). In both experiment years, five spray applications of Regalia (treatment 2) consistently provided 100% control of shoot blight severity in comparison to the untreated control (treatment 6). Thus, the high cumulative rate of Regalia spray application of 11.2 L/ha provided the best season-long prevention of shoot blight severity, at 100% on average (Figures 1, 2). In 2019, Arbor-OTC trunk injection, FireLine, and FireWall spray applications (treatment 3, 4 and 5) provided 94.8%, 100%, and 86.3% shoot blight severity control, respectively, in comparison to the untreated control (Figure 1). In 2022, the same antibiotic treatments did not control shoot blight severity, as they did not significantly differ from the inoculated untreated control (Figure 2). FireWall only numerically reduced severity by 25.5%. In 2019, trunk injection of Regalia did not control the disease allowing 75% of shoot blight severity, while in 2022, it provided 85.7% control of shoot blight severity.
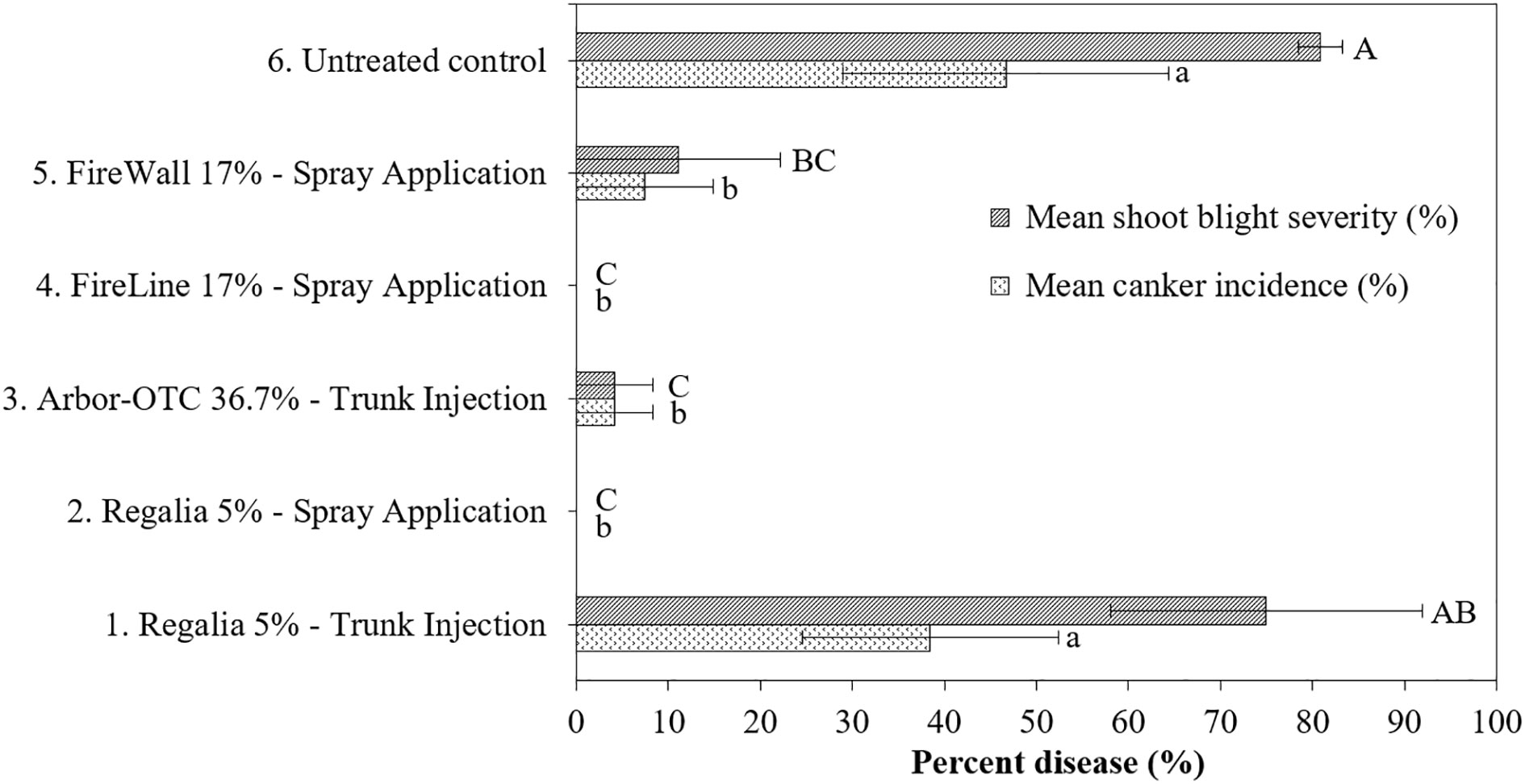
Figure 1 Shoot blight severity (Tukey test, p<0.05) and canker incidence (LSD test, p<0.05) on June 23 on pear cultivar ‘Bartlett’ after preventive spray and trunk injection treatments in 2019. Application rates are shown in Table 1. Shoots were inoculated on May 26 at 2.5- to 5-cm shoot size with E. amylovora (1.94 x 108 CFU/ml). Treatment bars of the same pattern followed by different letters are significantly different. Each mean consisted of three trees, with each tree mean consisting of 10 shoots per tree. Error bars represent the standard error of the mean.
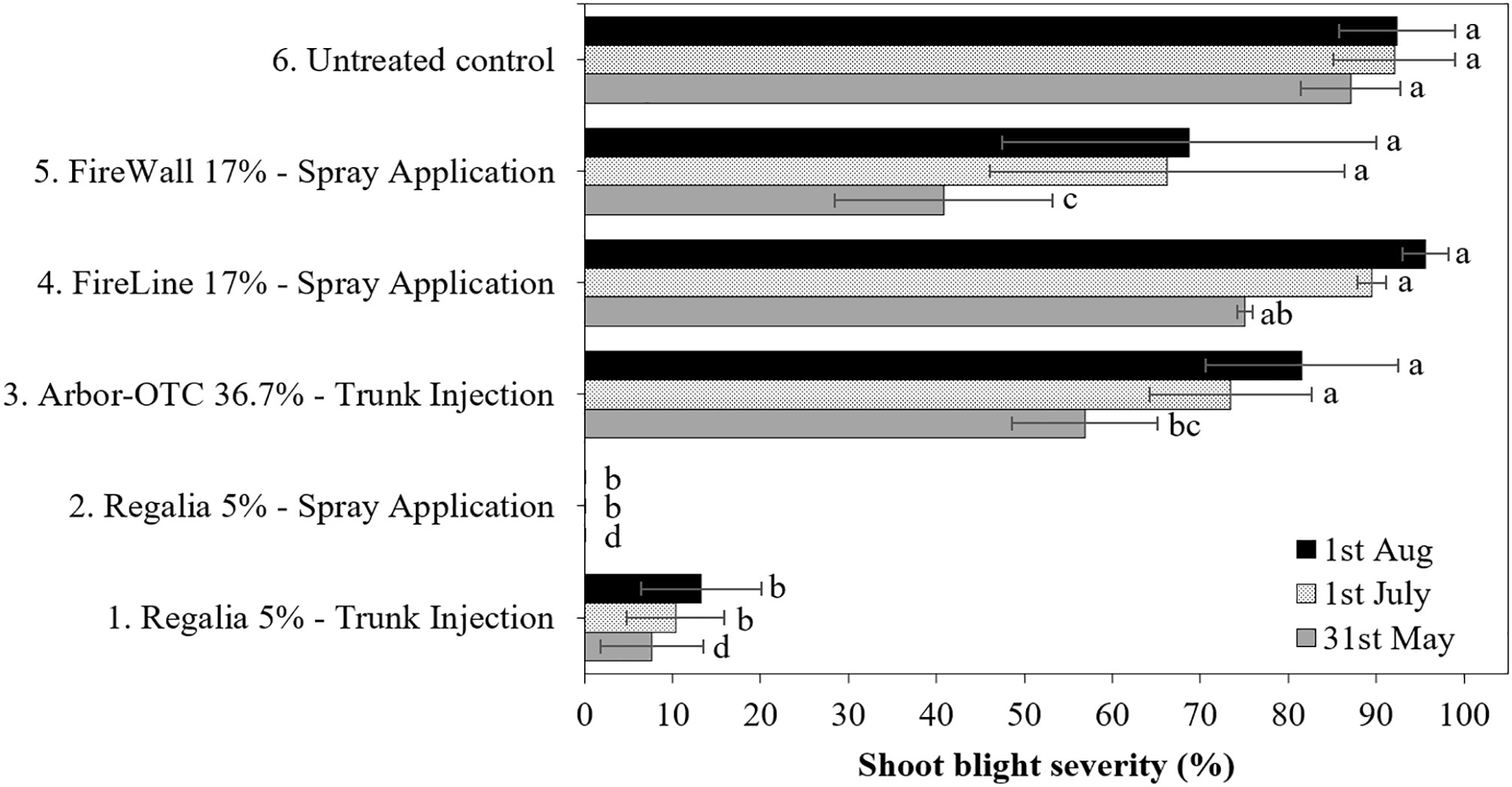
Figure 2 Shoot blight severity on pear cultivar ‘Bartlett’ after preventive spray and trunk injection treatments in 2022. Application rates are shown in Table 1. Shoots were inoculated on May 11 at 2.5- to 5-cm shoot size with E. amylovora (2.97 × 109 CFU/ml). Treatment bars of the same pattern followed by different letters are significantly different (LSD test, p<0.05). Each mean consisted of four trees, with each tree mean consisting of 10 shoots per tree. Error bars represent the standard error of the mean.
3.2 Reduction/prevention of canker incidence on perennial wood
Canker incidence in the inoculated untreated control (treatment 6) reached 46.7% and 92.6% in 2019 and 2022, respectively (Figures 1, 3). In 2019 and 2022, five spray applications of Regalia (treatment 2) provided 100% control of canker incidence in comparison to the untreated control (treatment 6). The high cumulative rate of 11.2 L/ha of spray applied Regalia provided the best season-long prevention of canker development, at 100% on average (Figures 1, 3). In 2019, Arbor-OTC trunk injection, FireLine, and Fire Wall spray applications (treatments 3, 4, and 5) provided 91%, 100%, and 84.2% control of canker incidence, respectively (Figure 1). On August 1, 2022, the same antibiotic treatments did not repeat the efficacy from 2019 in control of canker incidence as they allowed 87.5%, 92.2% and 70.0% cankers to develop and did not differ from the inoculated untreated control (Figure 3). In 2019, trunk injection of Regalia conducted in fall 2018 (treatment 1) did not prevent canker development by allowing 38.5% canker incidence on wood (Figure 1). In contrast, on August 1, 2022, the same treatment 1, although injected in spring 2022, achieved 89.8% control of canker incidence (Figure 3).
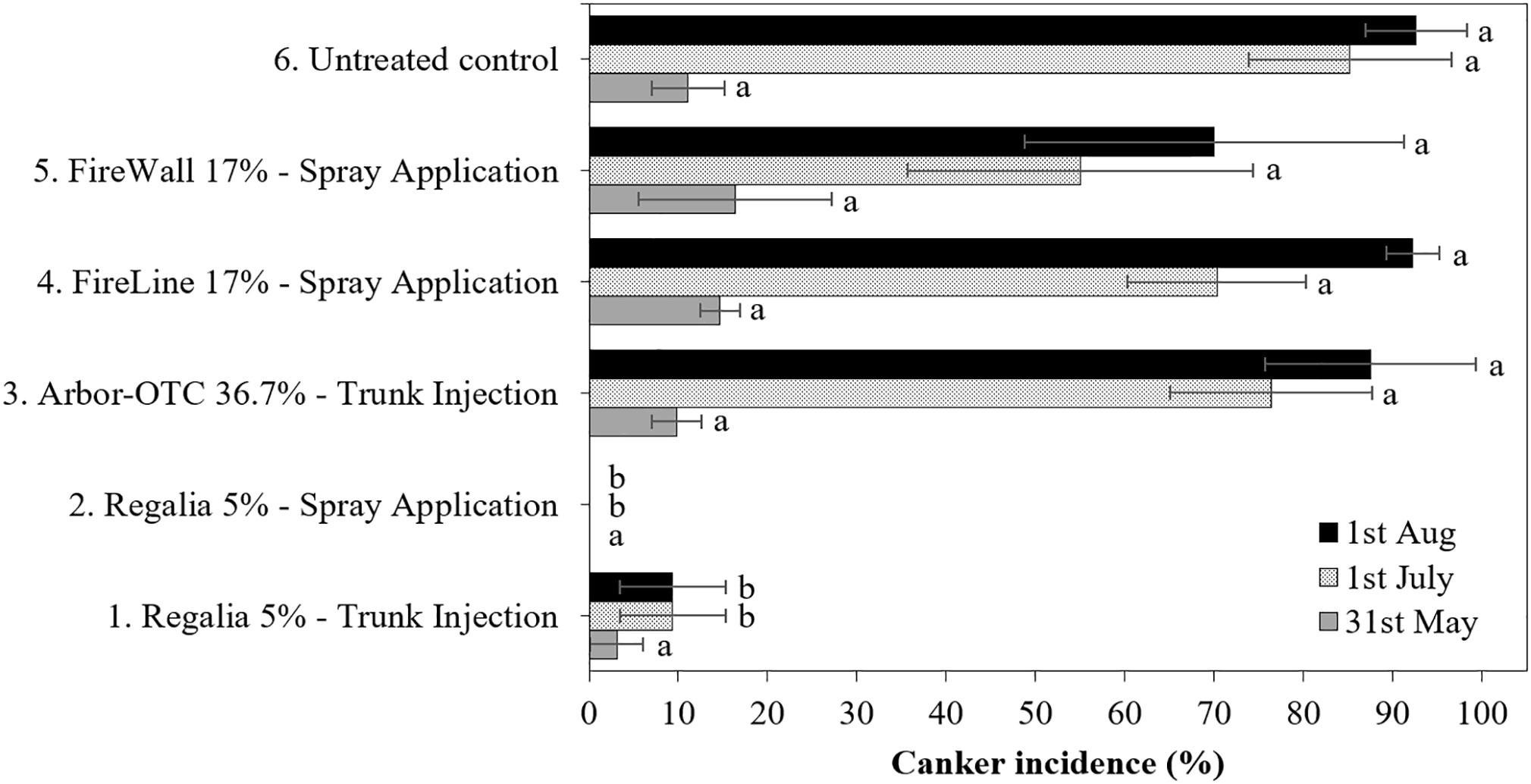
Figure 3 Canker incidence on perennial wood of pear cultivar ‘Bartlett’ from infected shoots after preventive spray and trunk injection treatments in 2022. Application rates are shown in Table 1. Shoots were inoculated on May 11 at 2.5- to 5-cm shoot size with E. amylovora (2.97 × 109 CFU/ml). Treatment lines followed by different letters are significantly different (LSD test, p<0.05). Each mean consisted of four trees, with each tree mean consisting of 10 shoots per tree. Error bars represent the standard error of the mean.
3.3 Reduction of canker length on perennial wood
Canker length in treatment 6 (inoculated untreated control) reached 2.0 cm in 2019 and 13.2 cm in 2022 (Figures 4, 5). In 2019 and 2022, Regalia spray applications (treatment 2) prevented canker expansion on perennial pear wood (100%), respectively, but in 2019 the canker length did not statistically differ from treatment 6 (inoculated untreated control) (Figures 4, 5). Similarly, FireLine spray applications in 2019 prevented canker expansion on pear wood but did not statistically differ from treatment 6 (Figure 4). In 2022, antibiotic treatments 3, 4, and 5 did not prevent canker formation on perennial pear wood by allowing 15.6 cm, 24.4 cm, and 22 cm of canker length expansion. The noticeable numerical differences in canker length between Regalia spray applications (treatment 2) and inoculated untreated control (treatment 6) in 2019 (Figure 4) were confirmed by the significant differences in 2022 (Figure 5). Consistently across both years, numerically, the most ineffective treatment was Arbor-OTC trunk injection with canker length reaching 1.8 cm in 2019 and 15.6 cm in 2022.
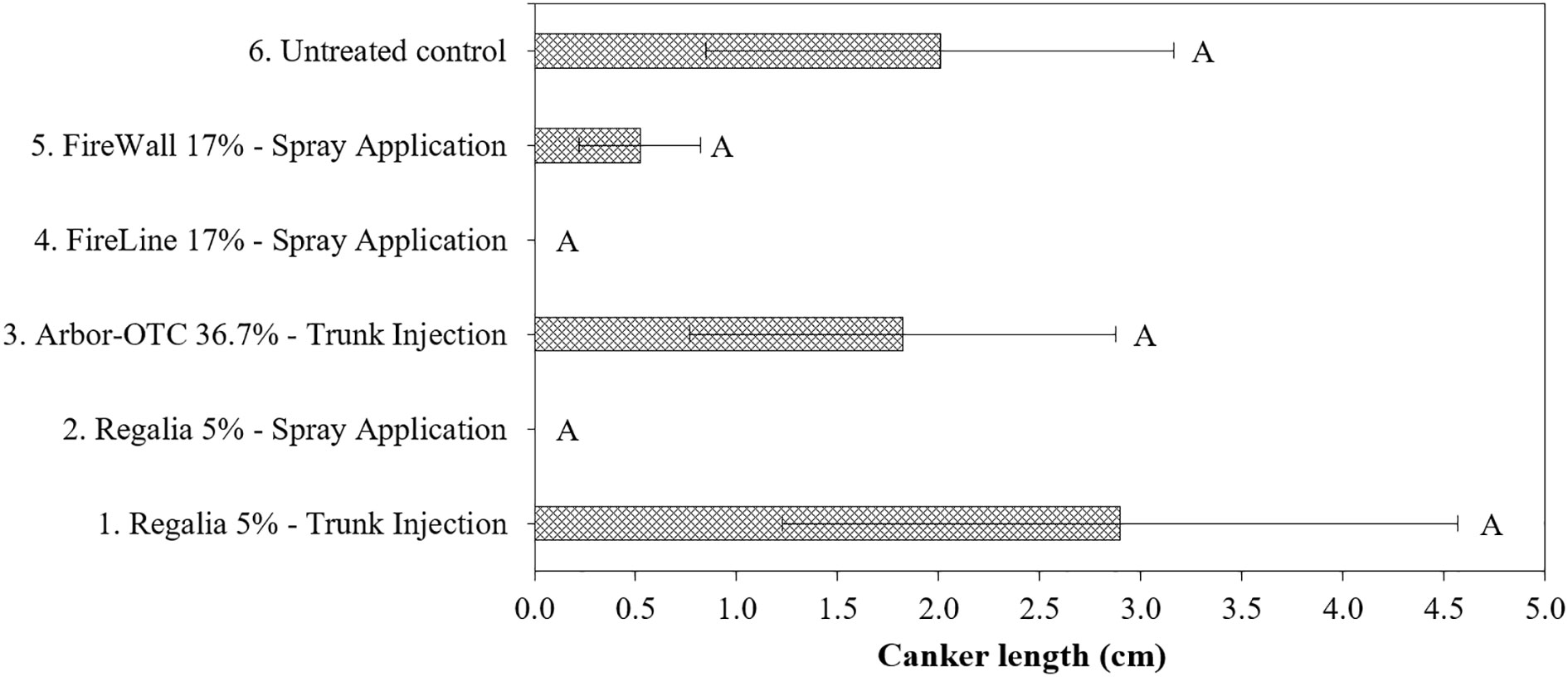
Figure 4 Length of fire blight cankers on perennial wood of pear cultivar ‘Bartlett’ developing from inoculated shoots after preventive spray and trunk injection treatments in 2019. Application rates are shown in Table 1. Shoots were inoculated on May 26 at 2.5- to 5-cm shoot size with E. amylovora (1.94 x 108 CFU/ml). Treatment bars followed by different letters are significantly different (test, p<0.05). Each mean consisted of four trees, with each tree mean consisting of 10 shoots per tree. Error bars represent the standard error of the mean.
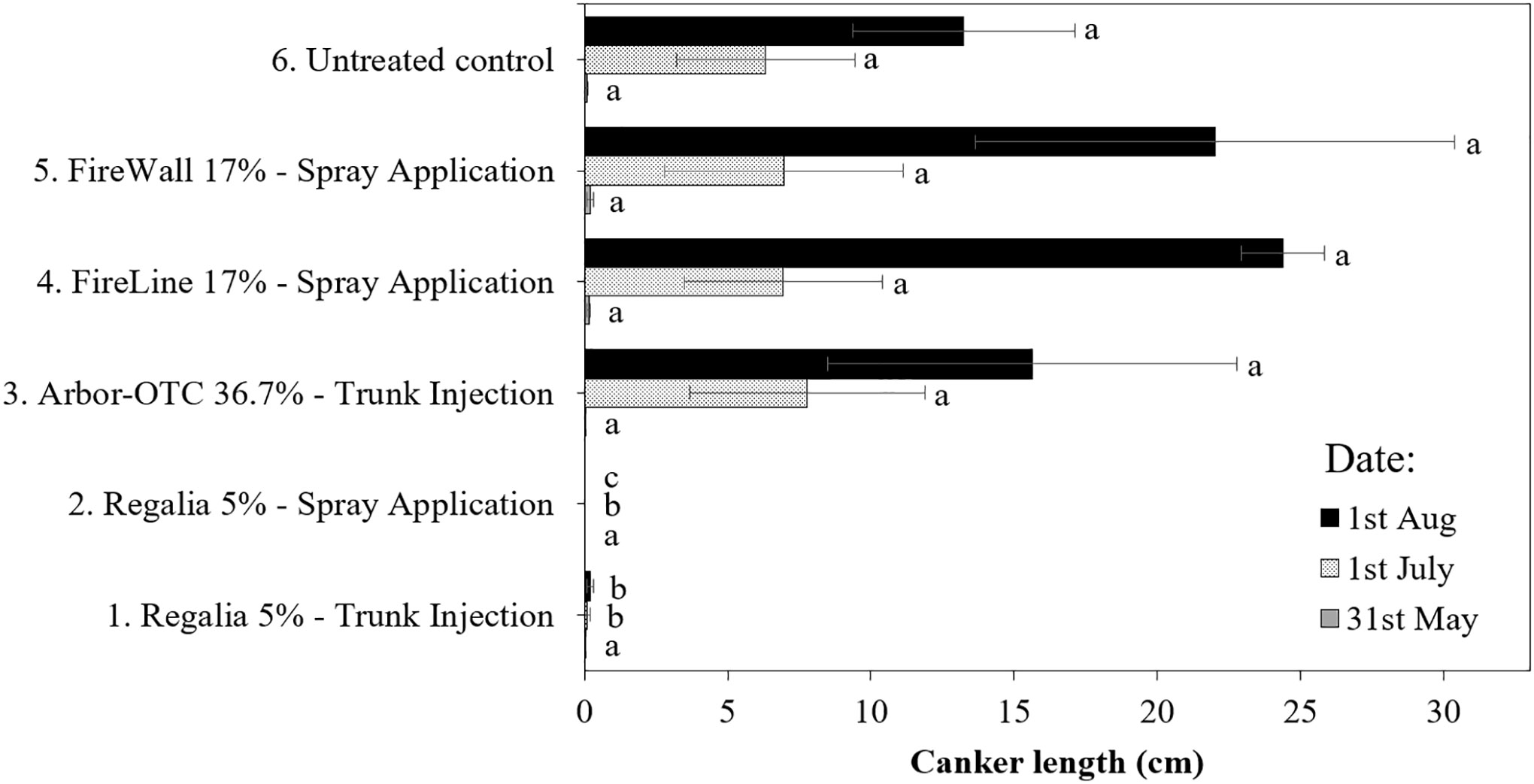
Figure 5 Length of fire blight cankers on perennial wood of pear cultivar ‘Bartlett’ from inoculated shoots after preventive spray and trunk injection treatments in 2022. Application rates are shown in Table 1. Shoots were inoculated on May 11 at 2.5- to 5-cm shoot size with E. amylovora (2.97 × 109 CFU/ml). Treatment lines followed by different letters are significantly different (LSD test, p<0.05). Each mean consisted of four trees, with each tree mean consisting of 10 shoots per tree. Error bars represent the standard error of the mean.
4 Discussion
We demonstrate in our study that preventive spray applications of Regalia reduced and prevented shoot blight severity, but more importantly Regalia also reduced and prevented fire blight canker development on perennial pear wood. Even though Regalia trunk injection in fall did not control the disease in the first experiment year, in the second experiment year, when it was injected in spring, it provided significant control of shoot blight severity and canker incidence. When fire blight cankers develop on the trunks, limbs, and rootstock, they can girdle the stem and kill branches, treetop, or whole mature or young trees in high-density pear orchards. Pear trees are much more susceptible to fire blight in comparison to apple trees (Santander et al., 2022a). Canker damage is much worse on young tree tissues of juvenile trees and cankers can serve as inoculum sources for future infections on mature trees. Therefore, we recommend through this study that five preventive spray applications of Regalia in spring are a potent option to reduce or prevent shoot blight onset and fire blight initiation on perennial pear wood.
In both experiment years, five preventive spray applications of Regalia provided the best control of shoot blight severity and canker incidence with 100% control. The canker length data supported the best control with spray applied Regalia in both years, however, in the first experiment year, the canker length did not statistically differ from the inoculated untreated control. Regalia is recommended for suppressing fire blight on pome fruit from pre-bloom to the fruit development stages. However, this plant protection material is poorly investigated for shoot blight control on pear and provides no control of shoot blight on apple (Sundin and Outwater, 2021 personal communication)1. In control of blossom blight incidence, Regalia preventive spray applications with the rate of 2,338.5 ml/ha in a tank mix with other fire blight products reduced apple blossom blight by 88% (Marrone Bio Innovations, 2022a). For blossom blight control on apples, Regalia spray applications are recommended to start at 25% bloom and re-applied when models indicate a fire blight infection period, followed by a petal fall application of the rate ranging from 4,677-9,354 ml/ha in rotation or tank mix with other products for suppression of shoot blight (Marrone Bio Innovations, 2022b). On pear cultivar ‘Bartlett’, single application of Regalia at petal fall (4,677 ml/ha) after applications of Blossom Protect (EPA Reg. No. 86174-4) plus Buffer Protect (citric acid, disodium phosphate) at 70% bloom, followed by Stargus (EPA Reg. No.: 84059-28) at full bloom, provided 39% reduction of blossom blight but was not different from untreated control (Johnson and Temple, 2021)2. In another trial on pear ‘Bartlett’, a spray program of Regalia at white bud (4,677 ml/ha), followed by Blossom Protect plus Buffer Protect, followed by Jet-Ag (EPA Reg. No. 81803-6) at full bloom, and then with Regalia applied at petal fall (4,677 ml/ha), gave 88% blossom blight control (Johnson and Temple, 2021 personal communication). After Regalia at the rate of 2,338.5 ml/ha in mix with Actigard or without it was applied at white bud, petal fall, and 10 days after petal fall on pear cultivar ‘Comice’, in alternation with Jet-Ag and Stargus at 70-80% bloom and full bloom, provided 84% and 80% blossom blight control (KC and DeShields, 2021 personal communication)3. However, there was no shoot blight control in this trial as there were no significant differences in comparison to untreated control. In our current study, five preventive spray applications of Regalia before inoculation with E. amylovora, each at a rate of 2,245 ml/ha, and cumulatively providing 11,225 ml/ha, was adequate to control shoot blight severity and canker formation. Our results indicate that the evaluated number of spray applications per growing season in the previous studies is too low to achieve desired control of shoot blight and cankers incidence. It seems that Regalia must be applied more frequently to achieve desired effect. Future research on modes of action of Regalia should determine why shoot blight incidence is controlled well on pears and not on apples. Regalia showed that it is a good alternative material for preventive control of fire blight infections on pear shoots and is an excellent OMRI-approved material for use in organic orchards (Organic Materials Review Institute, https://www.omri.org/).
Regalia trunk injection in spring significantly reduced shoot blight severity and fire blight canker development in the second experiment year with 85.7% and 90% control. This indicates that the trunk injection of this plant extract probably activated the SAR defense response in pear trees (Aćimović et al., 2015a). However, the injection in fall of the year preceding first experiment year was not effective, allowing 75% of shoot blight severity. One probable reason for this inconsistency between the two experimental years is that trunk injection in fall of 2018 was performed too far in time from the inoculation in May 2019. Regalia was probably degraded to inactive metabolites in the trunk or diluted to the non-effective levels and thus failed to control shoot blight (Aćimović et al., 2015a). In the second experiment year, high efficacy when the trunk injection was performed in spring indicated that Regalia was likely water soluble and thus had quickly translocated from the trunk into the shoots and was well distributed in the canopy (Aćimović, 2014; Aćimović et al., 2014b; Aćimović et al., 2016). Hence, it provided much better control of shoot blight and canker incidence as inoculation was done much closer in time to the injection.
In the first experiment year, FireLine provided 100% control of shoot blight severity, however in the second experiment year, the same material did not control the disease allowing 95.6% of shoot blight severity. This could be due to the extremely high photosensitivity of oxytetracycline and its degradation (Slack et al., 2021). In high-density pear plantings where the goal is to maximize light interception and increase yield (Palmer, 2008), shoots are fully exposed to light. Due to high sunlight sensitivity of oxytetracycline, it should be applied in the evening to maximize the time when this material is effective (Slack et al., 2021). Prior studies on peach leaves showed that when oxytetracycline is exposed to full natural sunlight, its residue decreased rapidly in the absence of rain, declining on average by 43.8, 77.8, and 92.1% within 1, 2, and 4 days after application, respectively (Christiano et al., 2010). After seven days of application, oxytetracycline levels were near the detection limit (Christiano et al., 2010). These results could directly support the hypothesis that FireLine was not effective in the second experiment year due to the photodegradation of the active ingredient, indicating that on the days when the spray application was made in second experiment year (April 25, May 9, 2022), the solar radiation was likely a significant factor that influenced the results. NEWA weather data from an onsite weather station in Winchester, VA, showed that the sum of solar radiation from 25 April to May 15, 2022 was 10,029 Ly in comparison to Highland, NY, with 8,850 Ly from 25 April to May 15 in 2021, and 9,254 from 25 April to May 15 in 2022 (https://newa.cornell.edu/all-weather-data-query).
In the first experiment year, FireWall provided 86.3% control of shoot blight severity, however, in the second experiment year, the same antibiotic did not control shoot blight severity. This inconsistency in effect could be due to similar, although lower streptomycin photosensitivity we hypothesized for oxytetracycline (Slack et al., 2021). Streptomycin is used for preventive control of blossom blight when fire blight prediction models indicate that flower infections can occur. It is not recommended for use when fire blight infections become visible and for control of shoot blight. The only exception are hail events when use of streptomycin is justified to prevent trauma blight infections through hail injuries (Aćimović and Meredith, 2017). Frequent post-symptom and shoot blight control applications of this antibiotic can lead to resistance of E. amylovora to streptomycin, which lead to control failures. Hence, materials with alternative modes of action like kasugamycin (Kasumin 2L, EPA Reg. No. 66330-404) or Aureobasidium pullulans DSM 14940 and DSM 14941 (Blossom Protect) are needed for controlling fire blight to offset these risks (McGhee and Sundin, 2011; Sundin et al., 2016; Johnson et al., 2022). Even though streptomycin is one of the most effective means of chemical control for fire blight, resistance risks raised concerns about its sustainability for fire blight management (Jones and Schnabel, 2000; Manulis et al., 2003; Russo et al., 2008; Tancos and Cox, 2016b).
The Arbor-OTC trunk injection in spring provided 91% control of canker incidence in the first year, but the second year did not control the cankers allowing 87.5% incidence to develop. A previous study showed that trunk-injected oxytetracycline in apples provides 60% control of blossom blight incidence (Aćimović et al., 2015b). The reduction of shoot blight severity with this antibiotic was up to 82% (Aćimović et al., 2015a). Besides the high photosensitivity of oxytetracycline, another possible reason for efficacy inconsistency in the second experiment year was that the time between the trunk injection and the inoculation with E. amylovora was insufficient for translocation of oxytetracycline and its ample distribution into the canopy. The previous study supported that time between trunk injection and inoculation might be a limiting factor for oxytetracycline to achieve spatially uniform distribution in the tree’s canopy (Aćimović, 2014).
In conclusion, we proved that Regalia can control shoot blight and fire blight cankers on pear with five preventive spray applications of 2,245 ml/ha. Our recommendation would be preventive use of Regalia as any post-symptom treatments would not result in the same control effect. Future research should evaluate if post-infection or post-symptom spray applications of Regalia could be combined with pruning removal of shoot blight and cankers to prevent further in-season fire blight infections. Even though Regalia trunk injection was inconsistent over the two years, we showed great potential of spring application to control shoot blight and cankers. More evaluations over several years are needed to confirm this effect of spring trunk injection. Trunk injection treatments could be a good option for preventive protection against fire blight in landscape Callery pears (Pyrus calleryana Decne.) and a good option for small organic or homeowner pear growers that manage a low number of trees. In these scenarios, Regalia could be alternated with a spray application of other inorganic or biocontrol products available for organic tree fruits. Streptomycin and oxytetracycline should be used only to prevent blossom blight infections. To maximize efficacy, they should be applied primarily before infections are predicted by the fire blight prediction models. Both antibiotics should be combined where resistance to streptomycin is known or replaced with kasugamycin. More research is necessary to explain why these antibiotics were inconsistent for shoot blight control in our two experiment years. It is possible that the time of the day when the applications were performed may have played the role in conjunction with photodegradation. It is recommended that antibiotics are applied to trees late in the afternoon or in the evening to secure their high efficacy by avoiding solar radiation and extending the time when these materials are effective (Slack et al., 2021).
Data availability statement
The raw data supporting the conclusions of this article will be made available by the authors, without undue reservation.
Author contributions
MCB conducted tree and shoot labelling in the field, rated disease parameters, collected the field data in 2022, and drafted the manuscript; CLM performed spray applications in 2019, assisted in collecting the data in 2019, and reviewed and edited the manuscript. BCD assisted with shoot inoculations in 2022 and reviewed and edited the manuscript. SGA originated the project ideas and questions, designed the field experiments, wrote project proposals, secured project funding, provided the necessary laboratory and field equipment and materials, supervised, and coordinated the work, mentored MCB, BCD and CLM, created graphs and tables, conducted statistical analyses of the data and reviewed, and edited the manuscript. All authors contributed to the article and approved the submitted version.
Funding
This work was supported by the Gerber Products Company, a subsidiary of Nestlé, 2018 grant program through a 1-year grant award to SGA for the project “2019 Organic Control of Fire Blight on Pears Comparing Foliar and Trunk Injection Delivery in New York”, the USDA NIFA Crop Protection and Pest Management Program grant 2022-70006-37991, proposal #2022-03444, Accession 1028841, and the SGA’s discretionary program funds and Virginia Tech faculty start-up funds.
Acknowledgments
The authors would like to thank Professor John C. Wise at Michigan State University, East Lansing, MI, Drew Afton and Julie Berens at Nestlé North America, Fremont, MI, Christopher Leffelman at Cornell's Hudson Valley Research Laboratory, Highland, NY, and Andrew Davis and Brandon Strong at Alson H. Smith Agricultural Research and Extension Center, Winchester, VA, for supporting this research in various ways and for help in planting and maintaining research plots.
Conflict of interest
The authors declare that the research was conducted in the absence of any commercial or financial relationships that could be construed as a potential conflict of interest.
The authors declare that this study received funding from Gerber Products Company. The funder was not involved in the study design, collection, analysis, interpretation of data, the writing of this article, or the decision to submit it for publication.
Publisher’s note
All claims expressed in this article are solely those of the authors and do not necessarily represent those of their affiliated organizations, or those of the publisher, the editors and the reviewers. Any product that may be evaluated in this article, or claim that may be made by its manufacturer, is not guaranteed or endorsed by the publisher.
Supplementary material
The Supplementary Material for this article can be found online at: https://www.frontiersin.org/articles/10.3389/fhort.2022.1082284/full#supplementary-material
Footnotes
- ^ Sundin, G. W., and Outwater, C. (2017). Michigan State University fire blight trial 2017 Marrone bio innovations, personal trial report. Sundin, G. W., and Outwater, C. (2018). 2018 Michigan state University fire blight trial MBI., personal trial report. Sundin, G. W., and Outwater, C. (2019). 2019 MSU shoot blight trial MBI, personal trial report. Sundin, G. W., and Outwater, C. (2021). 2021 MSU fire blight trial (MBI), personal trial report.
- ^ Johnson, K., and Temple, T. N. (2019). Non-antibiotic materials for control of pear fire blight 2019, personal trial report. Johnson, K., and Temple, T. N. (2020). Non-antibiotic materials for control of pear fire blight 2020, personal trial report.
- ^ KC, A. N., and DeShields, J. (2020). Assess the efficacy of Regalia (5%), Stargus, and Jet-Ag (alone and in program) against fire blight on pear under field conditions, personal trial report.
References
Aćimović S. G. (2014). Disease management in apples using trunk injection delivery of plant protective compounds, dissertation UMI number 3633036 (Michigan State University), 362. Available at: https://d.lib.msu.edu/etd/3195/datastream/OBJ/download/Disease_management_in_apples_using_trunk_injection_delivery_of_plant_protective_compounds.pdf.
Aćimović S. G., Balaz J. S., Aćimović D. D., Reeb P. D. (2014a). High magnitude of fire blight symptom development and canker formation from July onwards on two apple cultivars under severe natural infections. J. Plant Pathol. 96, 159–168. doi: 10.4454/JPP.V96I1.035
Aćimović S. G., VanWoerkom A. H., Reeb P. D., Vandervoort C., Garavaglia T., Cregg B. M., et al. (2014b). Spatial and temporal distribution of trunk-injected imidacloprid in apple tree canopies. Pest Manage. Sci. 70, 1751–1760. doi: 10.1002/ps.3747
Aćimović S. G., Martin D. K., Turcotte R. M., Meredith C. L., Munck I. A. (2019a). “Choosing an adequate pesticide delivery system for managing pathogens with difficult biologies: case studies on Diplodia corticola, Venturia inaequalis and Erwinia amylovora,” in Plant diseases - current threats and management trends (London, United Kingdom: IntechOpen), 133–158.
Aćimović S. G., Higgins E., Meredith C. L. (2019b). Effective post-infection programs of prohexadione-calcium for reducing shoot blight and preventing fire blight canker initiation on apple wood with cost-benefit analysis. New York. Fruit Q. 27 (2), 25–31.
Aćimović S. G., Zeng Q., McGhee G. C., Sundin G. W., Wise J. C. (2015a). Control fire blight (Erwinia amylovora) on apple trees with trunk-injected plant resistance inducers and antibiotics and assessment of induction of pathogenesis related protein genes. Front. Plant Sci. 6, 16. doi: 10.3389/fpls.2015.00016
Aćimović S. G., McGhee G. C., Sundin G. W., Wise J. C. (2015b). “Evaluation of trunk-injected bactericides and prohexadione-calcium for environmentally friendly control of fire blight (Erwinia amylovora) in apples,” in Proceedings of VII Congress on Plant Protection: Integrated Plant Protection – a Knowledge-Based Step towards Sustainable Agriculture, Forestry and Landscape Architecture, Zlatibor, Serbia, November 24-28, 2014. 129–134. Available at: https://cpb-us-e1.wpmucdn.com/blogs.cornell.edu/dist/7/7077/files/2017/03/VII_congress_on_plant_protection_proceedings_2014-11b5ixr.pdf.
Aćimović S. G., VanWoerkom A. H., Garavaglia T., Vandervoort C., Sundin G. W., Wise J. C. (2016). Seasonal and cross-seasonal timing of fungicide trunk injections on apple trees to optimize management of apple scab fungus Venturia inaequalis. Plant Dis. 100, 1606–1616. doi: 10.1094/PDIS-09-15-1061-RE
Aćimović S. G., Meredith C. L. (2017). Evaluation of newer biologicals and the SAR-activator candidate regalia in fire blight control applied by spraying or trunk injection. Fruit Q. 25 (4), 25–29.
Aćimović S. G., Meredith C. L., Santander R. D., Khodadadi F. (2021). Proof of concept for shoot blight and fire blight canker management with postinfection spray applications of prohexadione-calcium and acibenzolar-S-Methyl in apple. Plant Dis. 105, 4095–4105. doi: 10.1094/PDIS-08-20-1744-RE
Agrios G. N. (2005). Plant pathology. 5th Edition (Elsevier). Available at: https://www.sciencedirect.com/book/9780120445653/plant-pathology.
Bebber D. P. (2015). Range-expanding pests and pathogens in a warming world. Annu. Rev. Phytopathol. 53 (1), 335–356. doi: 10.1146/annurev-phyto-080614-120207
Bertani G. (1951). Studies on lysogenesis i. the mode of phage liberation by lysogenic Escherichia coli1. J. Bacteriol. 62, 293–300. doi: 10.1128/jb.62.3.293-300.1951
Bonn W. G., van der Zwet T. (2000). “Distribution and economic importance of fire blight,” in Fire blight disease and its causative agent erwinia amylovora. Ed. Vanneste J. L. (Hamilton, New Zealand: CABI), 37–53.
Coakley S. M., Scherm H., Chakraborty S. (1999). Climate change and plant disease management. Annu. Rev. Phytopathol. 37, 399–426. doi: 10.1146/annurev.phyto.37.1.399
Christiano R. S. C., Reilly C. C., Miller W. P., Scherm H. (2010). Oxytetracycline dynamics on peach leaves in relation to temperature, sunlight, and simulated rain. Plant disease 94 (10), 1213–1218.
Dossa G. S., Oliva R., Maiss E., Wydra K. (2015). High temperature enhances the resistance of cultivated African rice, Oryza glaberrima, to bacterial blight. Plant Dis. 100, 380–387. doi: 10.1094/PDIS-05-15-0536-RE
Eastburn D. M., McElrone A. J., Bilgin D. D. (2011). Influence of atmospheric and climatic change on plant-pathogen interactions. Plant Pathol. 60 (1), 54–69. doi: 10.1111/j.1365-3059.2010.02402.x
Elkins R. B., Klonsky K., DeMoura R., DeJong T. M. (2008). Economic evaluation of high density versus standard orchard configurations; case study using performance data for ‘Golden russet bosc’ pears. Acta Hort. 800, 739–746. doi: 10.17660/ActaHortic.2008.800.101
FPPCR - Fresh & Processed Pear Committee Research (2021) PNW pear research priorities for 2022, fresh and processed pear growers of Oregon & Washington. Available at: https://drive.google.com/file/d/1zoXCej8zdAUd56LKnSjEvN5r5-r0R-xp/view?usp=sharing.
Frumhoff P. C., McCarthy J. J., Melillo J. M., Moser S. C., Wuebbles D. J. (2007). “Confronting climate change in the US northeast,” in Confronting climate change in the US northeast. a report of the northeast climate impacts assessment (Cambridge, MA: Union of Concerned Scientists), 47–61.
Gaaliche B., Chehimi S., Dardouri S., Hajlaoui M. R. (2018). Health status of the pear tree following the establishment of fire blight in northern Tunisia. Int. J. Fruit Sci. 18, 85–98. doi: 10.1080/15538362.2017.1377670
Good Fruit Grower (2013). Available at: https://www.goodfruit.com/vigor-control-and-promotion-of-fruiting-of-pear-trees/.
Greene D. W. (2008). The effect of repeat annual applications of prohexadione–calcium on fruit set, return bloom, and fruit size of apples. HortScience 43 (2), 376–379. doi: 10.21273/HORTSCI.43.2.376
Griffith C. S., Sutton T. B., Peterson P. D. (2003). Fire blight: The foundation of phytobacteriology (St. Paul, MN, U. S. A.: American Phytophatological Society).
Hammerschmidt R. (2007). “Introduction: Definitions and some history,” in Induced resistance for plant defence. Eds. Walters D., Newton A., Lyon G. (Blackwell Publishing), 1–8. Available at: https://onlinelibrary.wiley.com/doi/abs/10.1002/9781118371848.ch1.
Hayhoe K., Wake C. P., Huntington T. G., Luo L., Schwartz M. D., Sheffield J., et al. (2007). Past and future changes in climate and hydrological indicators in the US northeast. Clim. Dyn. 28, 381–407. doi: 10.1007/s00382-006-0187-8
Johnson K. B., Temple T. N. (2013). Evaluation of strategies for fire blight control in organic pome fruit without antibiotics. Plant Dis. 97, 402–409. doi: 10.1094/PDIS-07-12-0638-RE
Johnson K., Temple T. N. (2015). Comparison of methods of acibenzolar-S-Methyl application for post-infection fire blight suppression in pear and apple. Plant Dis. 100, 1125–1131. doi: 10.1094/PDIS-09-15-1062-RE
Johnson K., Temple T. N. (2016). Comparison of methods of acibenzolar-s-methyl application for post-infection fire blight suppression in pear and apple. Plant Dis. 100, 1125–1131. doi: 10.1094/PDIS-09-15-1062-RE
Johnson K. B., Todd T. N., KC A., Elkins R. B. (2022). Refinement of nonantibiotic spray programs for fire blight control in organic pome fruit. Plant Dis. 106, 623–633. doi: 10.1094/PDIS-07-21-1405-RE
Jones A. L., Schnabel E. L. (2000). “The development of streptomycin-resistant strains of Erwinia amylovora,” in Fire blight disease and its causative agent Erwinia amylovora. Ed. Vanneste J. L. (Hamilton, New Zealand.: CABI), 235–251.
Longstroth M. (2001). The 2000 fire blight epidemic in southwest Michigan apple orchards. Compact. Fruit Tree 34 (1), 16–19.
Manulis S., Kleitman F., Shtienberg D., Shwartz H., Oppenheim D., Zilberstaine M., et al. (2003). Changes in the sensitivity of Erwinia amylovora populations to streptomycin and oxolinic acid in Israel. Plant Dis. 87 (6), 650–654. doi: 10.1094/PDIS.2003.87.6.650
Marrone Bio Innovations (2022a) Regalia product brochure for pome fruit. Available at: https://marronebio.com/products/regalia/.
Marrone Bio Innovations (2022b) Regalia product brochure for apples. Available at: https://marronebio.com/products/regalia/.
Mass F. (2008). Evaluation of Pyrus and quince rootstocks for high density pear orchards. Acta Hort. 800, 559–610. doi: 10.17660/ActaHortic.2008.800.80
McGhee G. C., Sundin G. W. (2011). Evaluation of kasugamycin for fire blight management, effect on nontarget bacteria, and assessment of kasugamycin resistance potential in Erwinia amylovora. Phytopathology 101, 192–204. doi: 10.1094/PHYTO-04-10-0128
McGrath M. J., Koczan J. M., Kennelly M. M., Sundin G. W. (2009). Evidence that prohexadione-calcium induces structural resistance to fire blight infection. Phytopathology 99 (5), 591–596. doi: 10.1094/PHYTO-99-5-0591
Melillo J. M., Richmond T. C., Yohe G. W. (2014). Climate change impacts in the united states: The third national climate assessment (Washington, DC: U.S. Global Change Research Program. U.S. Government Printing Office), 841. Available at: http://s3.amazonaws.com/nca2014/high/NCA3_Climate_Change_Impacts_in_the_United%20States_HighRes.pdf. doi: 10.7930/J0Z31WJ2
Mertoğlu K., Evrenososglu Y. (2017). Breeding Erwinia amylovora resistant F1 hybrid pear: selection of promising hybrid genotypes. Selçuk. Tarim. Ve. Gida. Bilimleri. Dergisi. 31 (3), 106–111. doi: 10.15316/SJAFS.2017.45
Montanari S., Prechepied L., Renault D., Frijters L., Velasco R., Horner M. (2016). A QTL detected in an interspecific pear population confers stable fire blight resistance across different environments and genetic backgrounds. Mol. Breed. 36 (4), 47. doi: 10.1007/s11032-016-0473-z
Norelli J. L. (2003). Fire blight management in the twenty-first century. Plant Dis. 87, 756–765. doi: 10.1094/PDIS.2003.87.7.756
Norelli J. L., Holleran H. T., Johnson W. C., Robinson T. L., Aldwinckle H. S. (2003). Resistance of Geneva and other apple rootstocks to Erwinia amylovora. Plant Dis. 87 (1), 26–32. doi: 10.1094/PDIS.2003.87.1.26
NY ARDP (2021) New York State Apple Research and Development Program 2021 - 2022 Priority List in Annual Request for Proposal. Additional Priorities, Category: Disease Issues. Available at: http://drive.google.com/file/d/1wkaM9CGDa7h0xcw9A1o6mMU-URPcmchB/view?usp=sharing.
Palmer J. W. (2008). Changing concepts of efficiency in orchard systems. In IX International Symposium on Integrating Canopy, Rootstock and Environmental Physiology in Orchard Systems 903 (pp. 41–49). Available at: https://www.ishs.org/ishs-article/903_1.
Paraschivu M., Ciobanu A., Cotuna O., Paraschivu M. (2020). Assessment of the bacterium Erwinia amylovora attack on several pear varieties (Pyrus communis l.) and the influence on fruits sugar content. Agric. Sci. Vet. Med. Univ. Bucharest. Sci. Papers. Ser. B. Horticult. 64 (1), 163–168.
Paraschivu M., Cotuna O., Paraschivu M., Ciobanu A., Oltenacu C. V. (2021). Infection of Erwinia amylovora on different apple varieties and the impact on fruits quality. Sci. Papers. Ser. B. Horticult. LXV (1), 219–227.
Philion V., Joubert V. (2021). Use of a growth regulator (prohexadione-Ca) and summer pruning as post symptom rescue treatments following a fire blight infection during bloom. J. Plant Pathol. 103, 163–174. doi: 10.1007/s42161-021-00805-5
Russo N. L., Burr T. J., Breth D. I., Aldwinckle H. S. (2008). Isolation of streptomycin-resistant isolates of Erwinia amylovora in new York. Plant Dis. 92 (5), 714–718. doi: 10.1094/PDIS-92-5-0714
Santander R. D., Meredith C. L., Aćimović S. G. (2019). Development of a viability digital PCR protocol for the selective detection and quantification of live Erwinia amylovora cells in cankers. Sci. Rep. 9, 11530. doi: 10.1038/s41598-019-47976-x
Santander R. D., Khodadadi F., Meredith C. L., Rađenović Ž., Clements J., Aćimović S. G. (2022a). Fire blight resistance, irrigation and conducive wet weather improve Erwinia amylovora winter survival in cankers. Front. Microbiol. 13, 1009364. doi: 10.3389/fmicb.2022.1009364
Santander R. D., Gašić K., Aćimović S. G. (2022b). “Selective quantification of Erwinia amylovora live cells in pome fruit tree cankers by viability digital PCR,” in Plant pathology: Method and protocols. methods in molecular biology, vol. 2536 . Ed. Luchi N. (New York, NY: Humana), 231–249.
Slack S., Walters K. J., Outwater C. A., Sundin G. W. (2021). Effect of kasugamycin, oxytetracycline, and streptomycin on in-orchard population dynamics of Erwinia amylovora on apple flower stigmas. Plant Dis. 105, 1843–1850. doi: 10.1094/PDIS-07-20-1469-RE
Smith T. J. (2000). Cougar blight fire blight infection risk assessment model (Washington State University). Available at: http://treefruit.wsu.edu/crop-protection/disease-management/fire-blight/cougar-blight-model/.
Smith T. J., Pusey P. L. (2011). CougarBlight 2010, a significant update of the CougarBlight fire blight infection risk model. Acta Hortic. 896, 331–336. doi: 10.17660/ActaHortic.2011.896.45
Smit M., Meintjes J. J., Jacobs G., Stassen P. J. C., Theron K. I. (2005). Shoot growth control of pear trees (Pyrus communis l.) with prohexadione-calcium. Sci. Hortic. 106 (4), 515–529. doi: 10.1016/j.scienta.2005.05.003
Steiner P. W. (1989). Predicting apple blossom infections by Erwinia amylovora using the MARYBLYT model. In V International Workshop on Fire Blight 273 (pp. 139–148). Available at: https://www.ishs.org/ishs-article/273_18.
Sugar D., Elfving D. C., Mielke E. A. (2004). Effects of prohexadione-calcium on fruit size and return bloom in pear. HortScience 39 (6), 1305–1308. doi: 10.21273/HORTSCI.39.6.1305
Sundin G. W., Castiblanco L. F., Yuan X., Zeng Q., Yang C. H. (2016). Bacterial disease management: challenges, experience, innovation and future prospects: challenges in bacterial molecular plant pathology. Mol. Plant Pathol. 17 (9), 1506–1518. doi: 10.1111/mpp.12436
Tancos K. A., Cox K. D. (2016b). Exploring diversity and origins of streptomycin-resistant Erwinia amylovora isolates in new York through CRISPR spacer arrays. Plant Dis. 100, 1307–1313. doi: 10.1094/PDIS-01-16-0088-RE
Tancos K. A., Villani S., Kuehne S., Borejsza-Wysocka E., Breth D., Carol J., et al. (2016a). Prevalence of streptomycin-resistant Erwinia amylovora in new York apple orchards. Plant Dis. 100 (4), 802–809. doi: 10.1094/PDIS-09-15-0960-RE
Turechek W. W., Biggs A. R. (2015). Maryblyt v. 7.1 for windows: an improved fire blight forecasting program for apples and pears. Plant Health Prog. 16, 16–22. doi: 10.1094/PHP-RS-14-0046
USDA, ERS - Economic Research Service (2022) Fruit and tree nut data – Export/Imports 2022. Available at: https://www.ers.usda.gov/data-products/fruit-and-tree-nuts-data/data-by-commodity/.
USDA, NASS - National Agricultural Statistics Service (2022) Noncitrus fruits and nuts 2021 summary. Available at: https://www.nass.usda.gov/Publications/Todays_Reports/reports/ncit0522.pdf.
Van der Zwet T., Beer S. V. (1995). Fire Blight - Its Nature. Prevention, and Control: A Practical Guide to Integrated Disease Management. US Department of Agriculture, Agriculture Information Bulletin (631), 97.
Van Der Zwet T., Beer S. V. (1999). Fire blight – its nature, prevention, and control: A practical guide to integrated disease management (US Department of Agriculture, Agriculture Information Bulletin No. 631 Edition slightly revised.), 97.
Van Der Zwet T., Keil H. L. (1979). Fire blight. a bacterial disease of rosaceous plants. handbook 510 (Washington, DC: United States Department of Agriculture).
VARP - Virginia Apple Research Committee (2021) Priority list for 2022 proposals. Available at: https://drive.google.com/file/d/1PQKXNgB_7jTskgQWopYhOaJH6GGjthgH/view?usp=sharing.
Wallis A. E., Cox K. D. (2019). Management of fire blight using pre-bloom application of prohexadione-calcium. Plant Dis. 104, 1048–1054. doi: 10.1094/PDIS-09-19-1948-RE
Wallis A. E., Cox K. D. (2020). Management of fire blight using pre-bloom application of prohexadione-calcium. Plant Dis. 104, 1048–1054. doi: 10.1094/PDIS-09-19-1948-RE
Wallis A., Yannuzzi I. M., Choi M. W., Spafford J., Siemon M., Ramachandran P., et al. (2021). Investigating the distribution of strains of Erwinia amylovora and streptomycin resistance in apple orchards in new York using CRISPR profiles: a six-year follow-up. Plant Dis. 105, 3554–3563. doi: 10.1094/PDIS-12-20-2585-RE
Winslow C.-E. A., Broadhurst J., Buchanan R. E., Krumwiede C., Rogers L. A., Smith G. H. (1920). The families and genera of the bacteria final report of the committee of the society of American bacteriologists on characterization and classification of bacterial types. J. Bacteriol. 5, 191–229. doi: 10.1128/jb.5.3.191-229.1920
WTFRC - Washington Tree Fruit Research Commission (2021a) Request for proposals. Available at: https://treefruitresearch.org/requests-for-proposals/.
WTFRC - Washington Tree Fruit Research Commission (2021b) Apple crop protection research priorities (2021). Available at: https://treefruitresearch.org/wp-content/uploads/2021/11/FINAL-2022-Research-Priorities-ACP_RFP.pdf.
Keywords: Erwinia amylovora, Regalia 5%, Reynoutria sachalinensis, trunk injection, oxytetracycline, streptomycin
Citation: Borba MC, Meredith CL, Dhar BC and Aćimović SG (2023) Proof of concept for management of shoot blight and fire blight cankers on pear with preventive spray applications of giant knotweed extract. Front. Hortic. 1:1082284. doi: 10.3389/fhort.2022.1082284
Received: 28 October 2022; Accepted: 19 December 2022;
Published: 16 March 2023.
Edited by:
Marcel Wenneker, Wageningen University and Research, NetherlandsReviewed by:
Radu E. Sestras, University of Agricultural Sciences and Veterinary Medicine of Cluj-Napoca, RomaniaBita Naseri, Agricultural Research, Education and Extension Organization (AREEO), Iran
Copyright © 2023 Borba, Meredith, Dhar and Aćimović. This is an open-access article distributed under the terms of the Creative Commons Attribution License (CC BY). The use, distribution or reproduction in other forums is permitted, provided the original author(s) and the copyright owner(s) are credited and that the original publication in this journal is cited, in accordance with accepted academic practice. No use, distribution or reproduction is permitted which does not comply with these terms.
*Correspondence: Srđan G. Aćimović, YWNpbW92aWNAdnQuZWR1