Corrigendum: IgG anti-spike antibodies and surrogate neutralizing antibody levels decline faster 3 to 10 months after BNT162b2 vaccination than after SARS-CoV-2 infection in healthcare workers
- 1University Hospitals Leuven, Clinical Department of Laboratory Medicine and National Reference Center for Respiratory Pathogens, Leuven, Belgium
- 2Academic Centre of General Practice, KU Leuven, Leuven, Belgium
- 3Department of Public Health and Primary Care, KU Leuven, Leuven, Belgium
- 4Environment and Health, Department of Public Health and Primary Care, KU Leuven, Leuven, Belgium
- 5Group IDEWE, External Service for Prevention and Protection at Work, Heverlee, Belgium
- 6Laboratory of Clinical and Epidemiological Virology, Department of Microbiology, Immunology and Transplantation, Rega Institute, KU Leuven, Leuven, Belgium
- 7Department of Cardiovascular Sciences, KU Leuven, Leuven, Belgium
Background: IgG anti-spike (S) antibodies arise after SARS-CoV-2 infection as well as vaccination. Levels of IgG anti-S are linked to neutralizing antibody titers and protection against (re)infection.
Methods: We measured IgG anti-S and surrogate neutralizing antibody kinetics against Wild Type (WT) and 4 Variants of Concern (VOC) in health care workers (HCW) 3 and 10 months after natural infection (“infection”, n=83) or vaccination (2 doses of BNT162b2) with (“hybrid immunity”, n=17) or without prior SARS-CoV-2 infection (“vaccination”, n=97).
Results: The humoral immune response in the “vaccination” cohort was higher at 3 months, but lower at 10 months, compared to the “infection” cohort due to a faster decline. The “hybrid immunity” cohort had the highest antibody levels at 3 and 10 months with a slower decline compared to the “vaccination” cohort. Surrogate neutralizing antibody levels (expressed as %inhibition of ACE-2 binding) showed a linear relation with log10 of IgG anti-S against WT and four VOC. IgG anti-S corresponding to 90% inhibition ranged from 489 BAU/mL for WT to 1756 BAU/mL for Beta variant. Broad pseudoneutralization predicted live virus neutralization of Omicron BA.1 in 20 randomly selected high titer samples.
Conclusions: Hybrid immunity resulted in the strongest humoral immune response. Antibodies induced by natural infection decreased more slowly than after vaccination, resulting in higher antibody levels at 10 months compared to vaccinated HCW without prior infection. There was a linear relationship between surrogate neutralizing activity and log10 IgG anti-S for WT and 4 VOC, although some VOC showed reduced sensitivity to pseudoneutralization.
Introduction
Vaccines are an important weapon in the fight against the COVID-19 pandemic. The BNT162b2 mRNA (Pfizer) is one of the most used vaccines worldwide and proved highly effective in clinical trials as well in real-life epidemiological studies that included millions of study subjects (1, 2). This vaccine elicits a strong antibody response against the spike (S) antigen, but not against the nucleocapsid (N) antigen. Anti-N antibodies can therefore be used as a surrogate marker for natural infection after vaccination with BNT162b2. Vaccine effectiveness studies have shown a decline of protection against symptomatic infection over time, while protection against severe disease is better retained (3–5). The waning of vaccine-induced immunity has been attributed to declining anti-S antibody levels and the emergence of variants of concern (VOC). Anti-S antibody levels start to decline 2-3 months after vaccination, declining continuously up to 8 months after vaccination (6–8). A similar continuous decline has been observed for anti-S and anti-N antibodies after natural infection (9, 10).
The receptor binding domain (RBD) on the S antigen is the target of most neutralizing antibodies (Nabs), as RBD mediates viral entry through binding of the ACE2-receptor on human cells. It has been well demonstrated that the level of Nabs correlates with protective immunity (3, 11–13). Live-virus plaque reduction neutralization tests (PRNT), considered the gold standard for measuring Nabs, are cumbersome, expensive and require a biosafety level (BSL) 3 laboratory environment. Furthermore, these assays have suboptimal interlaboratory reproducibility as there is no standardized protocol that is universally used (14–16). As an alternative to PRNT, the correlation of antibodies directed against the spike RBD measured with routine laboratory assays (“binding” antibodies) could in theory be used to infer protection (12). These tests are routinely available, easier and less expensive to perform (17). As the correlation of the results of “binding” antibody assays with live-virus neutralization is not excellent, assay-dependent and changes over time after infection or vaccination, an exact Nab titer cannot be predicted based on the level of “binding” antibodies (7, 18). Measuring surrogate neutralizing antibodies (pseudoneutralization) is an intermediate way between binding antibody tests and live-virus Nab tests, giving a better approximation of Nab titers and better reproducibility, while still suitable to be performed in a routine laboratory environment (BSL-2) (15, 16).
Materials and Methods
Study Design
This study was approved by the local ethics committee at the University Hospitals Leuven (S64152). After obtaining informed consent, serum samples were collected from a cohort of health care workers (HCW) who received two doses of the BN162b2 vaccine (with a 21-day interval (20-22 days). The results of the first 4 time points up to 3 months after the first dose have been previously published (19). For this follow-up study, only participants who were sampled at both 3 and 10 months were included (114 of the 150 participants).
The study cohort included 97 participants who were COVID-19 naive when vaccinated (“vaccination” cohort) and 17 participants who had a SARS-CoV-2 infection 6 to 10 months prior to vaccination (“hybrid immunity” cohort). Infection in the 17 participants with “hybrid immunity” was confirmed by PCR or serological evidence of previous infection at baseline (positive for IgG anti-S and anti-N antibodies). Five participants in the “vaccination” cohort and 1 participant in the hybrid immunity cohort had a breakthrough infection between the 3 and 10-month time points (5 confirmed by PCR and 1 by a 3-fold or higher increase in IgG anti-S and anti-N titer). In addition, 17 participants in the “vaccination” cohort had already received a booster dose of BN162b2 before sample collection at 10 months (16 participants between 2-7 weeks and one participant 5 days before sampling). These 23 participants were excluded from all statistical analyses.
Antibody kinetics after vaccination were compared to antibody kinetics after natural infection in a cohort of HCW (“infection” cohort). This cohort consisted of HCW included in a prior arm of the same clinical study (S64152) which studied Abbott IgG anti-S and anti-N levels in HCW up to 10 months after natural infection (9). The criterion for inclusion in the current study was the availability of a sample collected at 3 months (80-100 days, median 87 days, n= 83) and a sample collected at 10 months (280-320 days, median 295 days, n=83) after positive PCR. These participants were first sampled in June-July 2020 and a second time in December 2020-January 2021, before vaccines were available for HCW in Belgium. These natural infections (March to June 2020) precede the emergence of VOC and thus correspond to “Wild Type (WT)” strains. There were no reinfections between 3 and 10 months in the infection cohort. Samples used for surrogate neutralizing antibody measurement were stored at -20°C before analysis.
Patient Population
The patient characteristics of the three cohorts are described in Table 1. The median interval in the “hybrid immunity” cohort between positive PCR and administration of the first dose of vaccine was 274 days (range 39-301 days).
Antibody Measurement
IgG antibodies against SARS-CoV-2 nucleocapsid and spike RBD were measured with the Abbott Architect (Abbott, Lake Forest Illinois) SARS-CoV-2 IgG (anti-N) and IgG II Quant (anti-S) chemiluminescence immunoassays using the manufacturer’s cut-offs for positivity of 1.4 S/CO and 50 AU/mL, respectively. IgG anti-N was only measured at baseline and at 10 months. Samples above the measuring range of 40.000 AU/mL for anti-S were further diluted 1:2 using Abbott multi-assay manual diluent according to the manufacturers’ instructions. The units of the quantitative Abbott anti-S assay (AU/mL), which uses a 6-point calibration curve, were converted to WHO units (BAU/mL) by multiplying with a factor of 0.142 according to the manufacturer’s instructions. This is not possible for the semi-quantitative Abbott anti-N assay (S/CO) which only uses a two-point calibration curve.
Surrogate Neutralizing Antibodies
Detection of surrogate neutralizing antibodies was performed using the V-PLEX ACE2 neutralization assay panel 13 (K15466U, Meso Scale Diagnostics, LCC. Rockville, USA). This panel is an extension of panel two, which was chosen by Operation Warp Speed as the basis of its standard binding assays for immunogenicity assessments in all funded Phase III clinical trials of vaccines (20). It is a competitive binding assay that quantitatively measures antibodies that block the binding of ACE2 of its cognate ligands. Neutralizing capacity is quantified by measuring light emitted from a conjugated ACE2 protein which binds non-blocked spike antigens. The more neutralizing antibodies in the patient sample, the more the binding of ACE2 to the coated spike proteins is inhibited (results are expressed in %inhibition). Samples were prediluted 1/10 according to the manufacturer’s instructions. The assay contains coated SARS-CoV-2 spike proteins of the following variants: Wild Type (Wuhan strain), Alpha (B.1.1.7), Beta (B.1.351), B.1.526.1, B.1.617, Kappa (B.1.617.1), Delta (B.1.617.2), B.1.617.3, Gamma (P.1) and zeta (P.2). We only included the WT strain and the four VOC included in the panel (Alpha, Beta, Delta and Gamma strains) for further analysis. Comparable surrogate neutralization assays have been shown to correlate well with conventional live virus neutralization assays (r = 0.93) (16).
Omicron BA.1 Virus Neutralization
Twenty serum samples with >90% inhibition at 1/10 dilution with the V-PLEX ACE2 neutralization assay against Wild Type variant (94.8 ± 1.8%) were randomly selected for live virus (Omicron BA.1 VOC) neutralization on Vero cells, as previously described (21). Live virus neutralization was performed with serial twofold dilutions of serum (1/50 to 1/1600) and results were expressed as % inhibition at 1/100 dilution or 50% Neutralizing Titer (NT50) of cytopathogenic effect.
Data Analysis
The median antibody levels (three cohorts) and %inhibition (three cohorts and 5 variants) were compared using the non-parametric rank Mann–Whitney U (MWU) test with R Studio and corrected for multiple testing using the Bonferroni method. Decline rates are reported as the median value within a cohort of individual decline rates. A p-value <0.05 was considered significant.
The log10 of the antibody titers and the log10 decline in antibody titers between 3 and 10 months were compared using the non-parametric Mann-Whitney-U test. The decline of antibody titers between 3 and 10 months was calculated by performing a simple linear regression in R studio using the log10 of the antibody titer and days post positive RT-PCR, corresponding to a one-phase exponential decay of the antibody levels.
Simple linear regression was used to calculate antibody levels (BAU/mL) correlating to 50%, 90% and 95% surrogate neutralizing inhibition levels. Spearman correlation was used to evaluate the relationship between the log10 binding antibody titer, and surrogate neutralizing inhibition levels (%), and live virus neutralization.
Surrogate neutralizing antibodies against WT and 4 VOC of the 20 samples selected for live virus neutralization were compared with One-way ANOVA with stringent FDR correction for multiple comparisons.
Results
IgG Anti-S Antibody Kinetics
All HCW in the “vaccination” and “infection” cohorts had detectable IgG anti-S antibodies within 3 months after vaccination. After 10 months, all vaccinated HCW were still seropositive compared to 92.7% of the “infection” cohort. IgG antibody levels in the “hybrid immunity” cohort were significantly higher at 3 and 10 months than in the “vaccination” and “infection” cohorts (p<0.001 for both time points, Figure 1). After 10 months, median decline of antibody levels compared to 3 months was 68%(p<0.001), 87% (p<0.001) and 49% (p<0.001) in the “hybrid immunity”, “vaccination”, and “infection” cohort, respectively. The relative decline of antibody levels was significantly faster in the “vaccination” cohort compared to the “infection” cohort and the “hybrid immunity” cohort (p<0.001 vs. both with MWU test). Figure 2A shows a simple linear regression of log10 IgG anti-S Antibody decline between 3 and 10 months in the three groups.
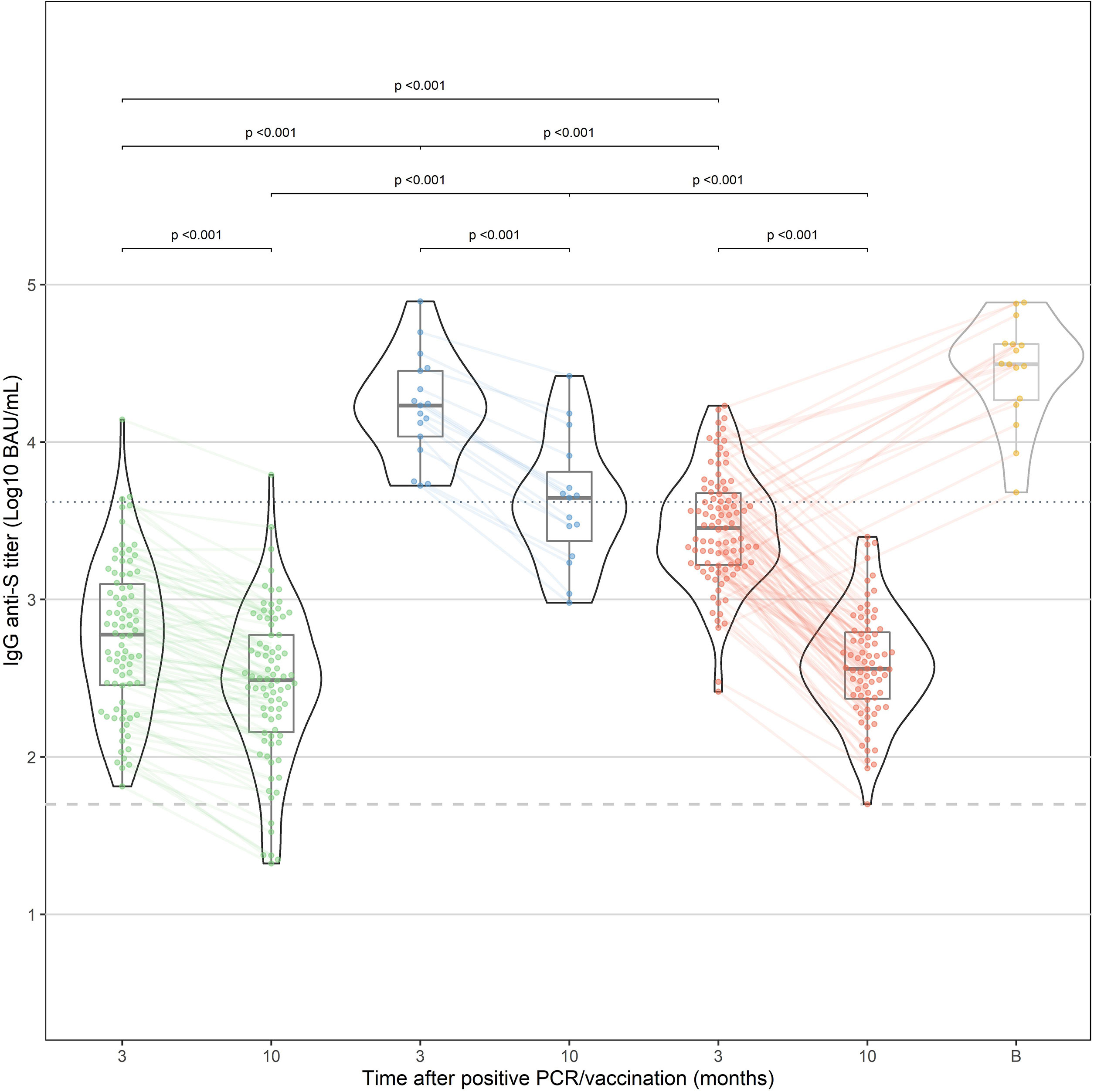
Figure 1 IgG anti-S antibody response after natural infection (“infection”, green) and after vaccination in previously infected HCW (“hybrid immunity”, blue) and naive HCW (“vaccination”, red). In addition, the results at 10 months of HCW who have received a booster dose before the 10-month time point are shown in yellow (excluded for statistical analysis). Median time after booster (shown as time point "B") was 21 days (IQR: 13.8-39.5 days).
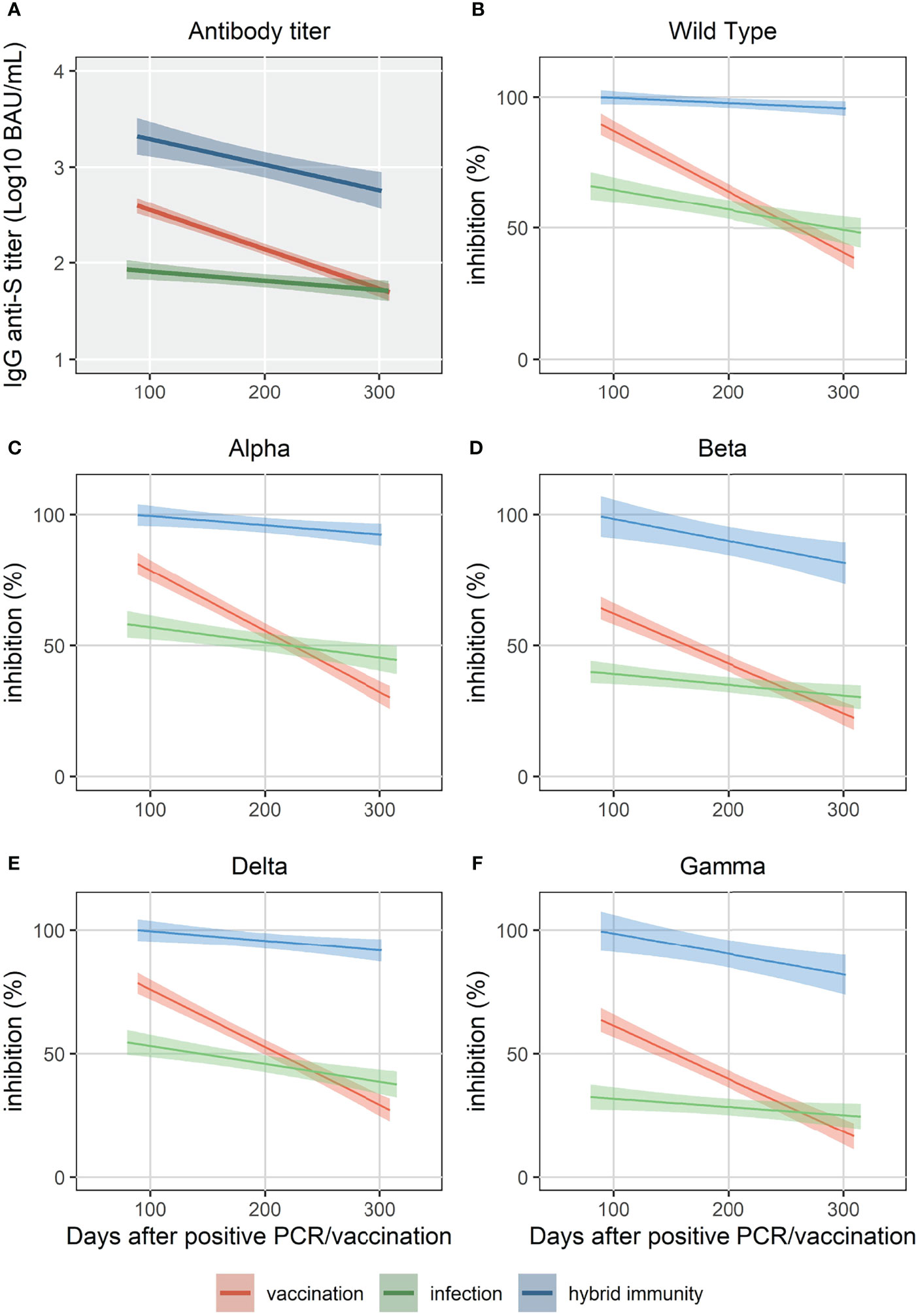
Figure 2 Simple linear regression of IgG anti-S (A) and surrogate neutralizing antibody levels (% inhibition) for WT and 4 VOC (B-F) up to 320 days.
Surrogate Neutralizing Antibody Kinetics Against Wild-Type and Four VOC
For the “vaccination” and “infection” cohort, %inhibition was significantly higher at both 3 and 10 months against the WT strain compared to Beta, Gamma and Delta VOC (p<0.05), but not compared to Alpha VOC. For the “hybrid immunity” cohort, we did not find a significant difference between WT and the four VOC at 3 and 10 months as %inhibition was close to 100% for all samples.
The “hybrid immunity” cohort showed the highest %inhibition at 3 and 10 months against all the strains compared to the “vaccination” and “infection” cohorts (p<0.001 vs. both for all strains). Median %inhibition in the “hybrid immunity “cohort was 100% for WT and the four VOC at 3 months. At 10 months, median %inhibition was 100% for WT, and between 95% (Beta) and 99% (Delta) for the four VOC.
At 3 months, %inhibition for WT and the four VOC was significantly lower in the “infection” cohort than in the “vaccination” cohort (p<0.001 for all strains, Figure 2). Ten months after vaccination, the median %inhibition decrease was 51% for WT and between 40% (Beta) and 52% (Delta) for the four VOC in the “vaccination” cohort. In the “infection” cohort, however, the median %inhibition decrease was only 16% for WT and between 7% (Beta) and 14% (Delta) for the four VOC. Due to the faster relative decrease in %inhibition in the “vaccination” cohort (p<0.001 for each strain vs. “infection” cohort, MWU-test), median %inhibition at 10 months in the “vaccination” cohort was significantly lower compared to the “infection” cohort for the four VOC (p<0.05), but not for WT (p=0.08) (Figures 3A-E).
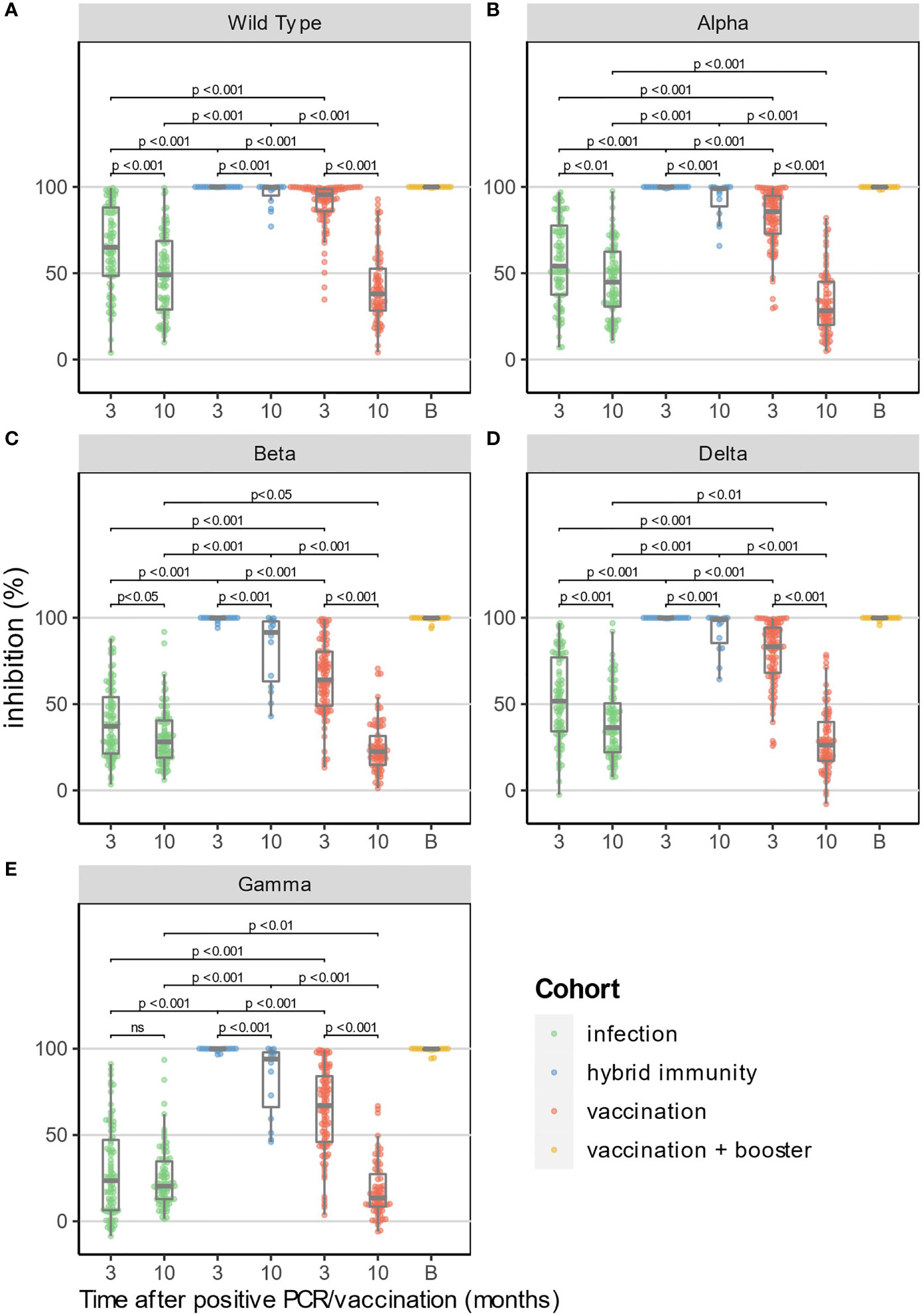
Figure 3 Surrogate neutralizing antibody levels (% inhibition) at 3 and 10 months for WT and 4 VOC (A–E). In addition, the results at 10 months of HCW who have received a booster dose before the 10-month time point are shown in yellow (excluded for statistical analysis). Median time after booster (shown as time point "B") was 21 days (IQR: 13.8-39.5 days).
Correlation Between IgG Anti-S Antibody and Surrogate Neutralizing Antibody Levels
The log10 of IgG anti-S showed a linear relation with %inhibition reaching a plateau close to 100% inhibition for WT and the four VOC (Figure 4). Given the relatively low number of individuals in our study, the three cohorts were pooled for correlation analysis. The Spearman’s rank correlation coefficient was significantly higher at 3 months than at 10 months for the four VOC and the Wild Type strain (Table 2).
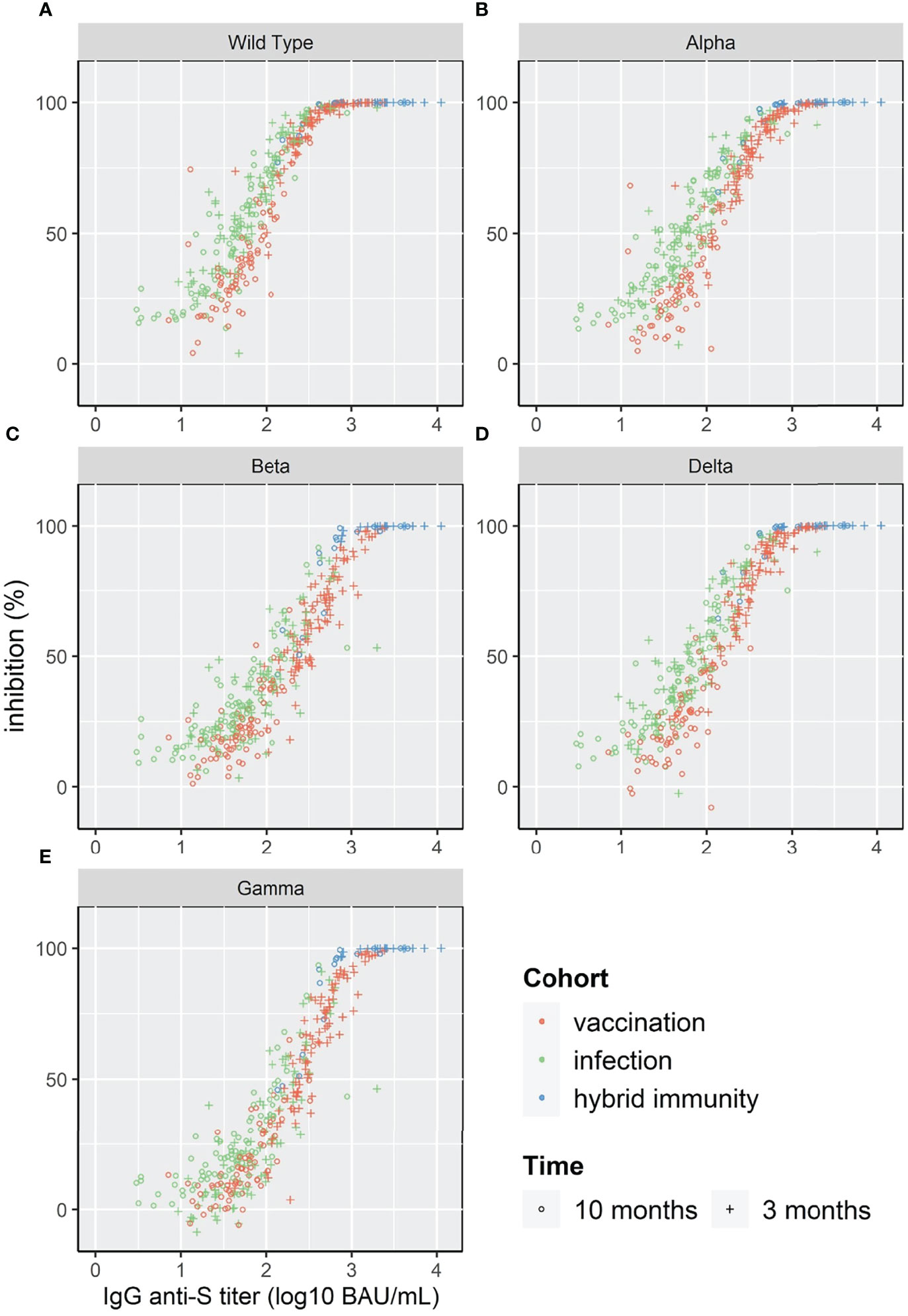
Figure 4 Scatterplot of surrogate neutralizing antibody inhibition levels (%) and IgG anti-S for WT and 4 VOC (A–E).
To compare the correlation at different antibody levels for the different strains, we calculated the IgG anti-S titer that corresponded to 50%, 90% and 95% inhibition (Table 3). The IgG anti-S titer corresponding to 90% inhibition was 489 BAU/mL for WT with an agreement of 89% (above/below cut-off) and varied between 713 BAU/mL (Alpha) and 1756 BAU/mL (Beta) for the four VOC with an agreement of ≥92%.
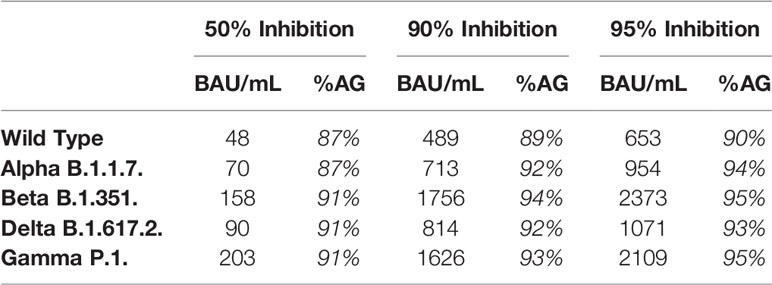
Table 3 Calculated IgG anti-S titer corresponding to 50%, 90% and 95% inhibition and % agreement (%AG, above below cut-off for antibody titer and %inhibition) for the different strains.
High Titers of Pseudoneutralization Against Alpha, Beta, Gamma and Delta VOC Predict Neutralization of Omicron BA.1
We investigated if high pseudoneutralization values against Alpha, Beta, Gamma and Delta VOC, in serum samples collected before the arrival of the Omicron variant, might predict the presence of neutralizing antibodies against the Omicron BA.1 variant in a live virus-neutralization assay in Vero cells, as previously described (21).
Of the 20 randomly selected serum samples with >90% inhibition (94.8% ± 1.8%) against WT pseudovirus, 4/20 (20%) displayed 100% Omicron neutralization at 1/100 dilution, while 13/20 (65%) showed at least 50% neutralization against Omicron (Figure 5A). We observed a gradual decrease in (pseudo)neutralization across all VOC as compared to WT (Alpha<Delta<Beta/Gamma<Omicron), in agreement with the extensively described antigenic distance among these VOC (22). Pseudoneutralization of all 5 Spike variants (1/100 dilution) was significantly correlated with Omicron BA.1 virus neutralization (Figure 5B, all p<0.05). However, a high %inhibition against Beta and Gamma VOC was the strongest predictor of Omicron neutralization, with a cutoff of 70% inhibition resulting in 84.6% sensitivity and 71.4% specificity of predicting high (>50%) Omicron neutralization (RR 2.96 95%CI[1.21-10.44], Fisher’s exact test p=0.022).
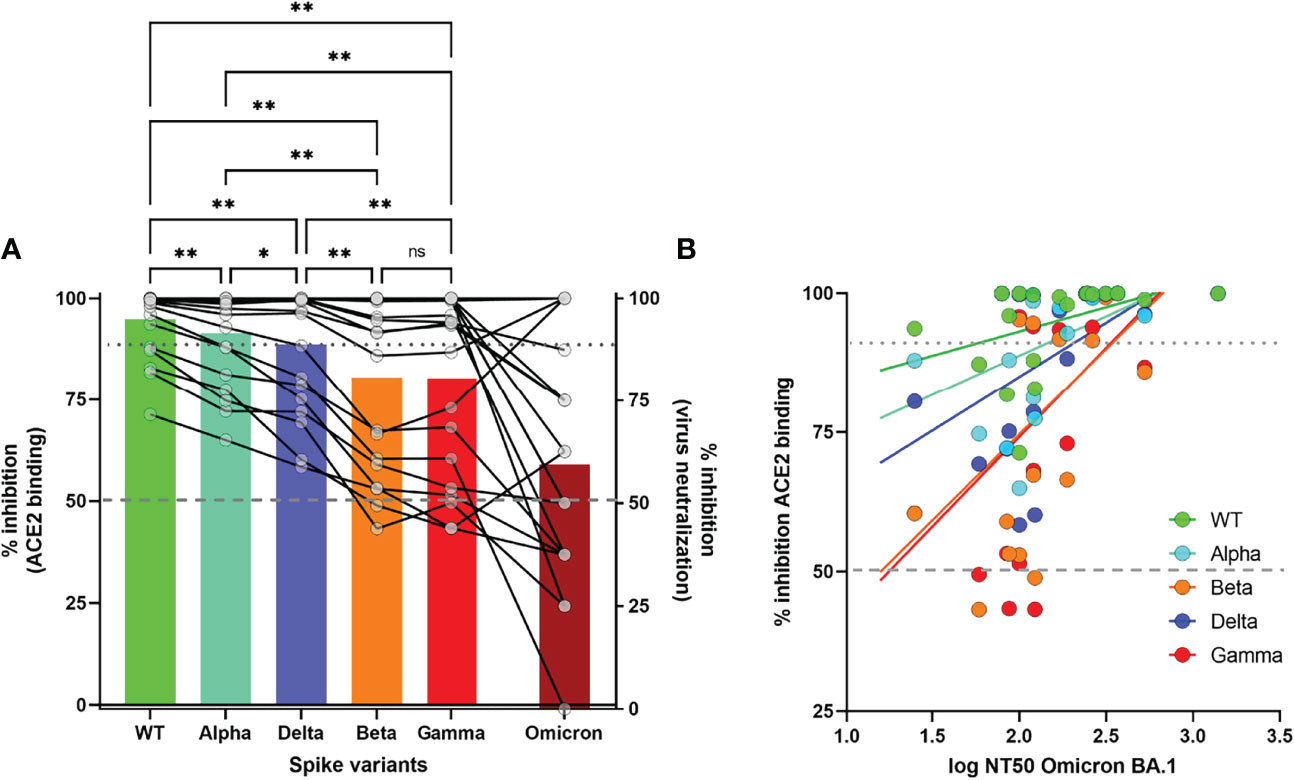
Figure 5 Correlation of surrogate neutralizing antibody levels (%inhibition) against WT and 4 VOC with virus-neutralization assay for Omicron BA.Pseudoneutralization is expressed as % inhibition of recombinant ACE2 binding for 4 VOC. Virus neutralization of Omicron is expressed as % inhibition at 1/100 dilution (Figure 5(A)) or 50% Neutralizing Titer (NT50) of cytopathogenic effect (Figure 5(B)). Statistical analysis by One-way ANOVA with stringent FDR correction for multiple comparisons (**p<0.01, *p<0.05, ns, not significant). Correlation between both assays was measured by Spearman’s Rho coefficient (p<0.05 for all 5 Spike variants). Dashed and dotted grey lines depict 50% and 90% inhibition, respectively.
Discussion
We studied the IgG anti-S and surrogate neutralizing antibody kinetics (expressed in %inhibition of ACE-2 binding), after vaccination with BNT162b2 and natural infection up to 10 months. Vaccination after prior infection (“hybrid immunity”) resulted in the highest IgG anti-S antibody levels and %inhibition and the slowest decline between 3 and 10 months. Vaccination in COVID-19 naive individuals resulted in higher IgG anti-S antibody titers and %inhibition for WT and four VOC at 3 months compared to natural infection, but not at 10 months due to a faster decline after vaccination. IgG anti-S correlated well with neutralizing activity againstWT and 4 VOC at 3 and 10 months. High titers of pseudoneutralization at 10 months (vaccinated with 2 doses BNT162b2) predicted live virus neutralization of Omicron BA.1.
IgG anti-S titers ("binding antibodies") after natural infection and vaccination correlate with (pseudo)neutralizing titers (23, 24), and neutralizing titers, in turn, correlate with protection against disease (3, 11–13). Therefore, the kinetics of IgG anti-S "binding antibodies", neutralizing antibodies and protective immunity often show a similar course in studies (1, 3, 7, 11, 12) and protection can be estimated using IgG anti-S titers (12). The exact degree of correlation depends on the assays used (e.g. total Ig vs IgG) and the threshold of protection depends on disease severity and the SARS-CoV-2 variant (3, 11, 25). Despite waning over time, residual humoral immunity may still be sufficient to protect against severe disease as lower amounts of neutralizing antibodies are required for protection against severe disease compared to mild infection (1, 3, 5, 11, 26).
We found a linear relationship between the log10 of binding IgG anti-S levels and surrogate neutralizing antibodies for WT and four VOC. Using a 1:10 dilution of the serum, 90% inhibition corresponded to IgG anti-S titers between 489 BAU/mL for WT and 1756 BAU/mL for the Beta variant. Although the shape of the relation between binding antibodies and %inhibition was similar for all variants, the level of IgG anti-S antibodies in BAU/mL required to reach 90% inhibition varied. This implies that the prediction of protective immunity is dependent on the circulating variant. When a new variant arises (such as the recent Omicron), the relationship between IgG anti-S antibodies and neutralizing capacity must be reassessed. In addition, the correlation also depends on the time after infection/vaccination. Similar to Levin et al., we found a lower Spearman’s rank correlation for WT and the four VOC at 10 months compared to at 3 months (7). Nonetheless, the correlation at 10 months was still good (ρ ≥0.79).
Waning of antibody levels after vaccination, with concomitant reductions in protective immunity, have been demonstrated in clinical trials as well as in epidemiological studies (4, 7, 27–29). In HCW, IgG anti-S antibody levels peak around 3 weeks after the second dose of BNT162b2, followed by a decline over time (19). In the phase 3 trial of BNT162b2, vaccine protection peaked between 7 days and 2 months after the second dose, followed by a progressive decline of protection (84% in the period 4-7 months after the second dose) (1). Andrews et al. showed a reduction of vaccine effectiveness of BNT162b2 of 96% early after vaccination to 66.3% at 5 months after the second dose (against the Delta variant) (4). Sheehan et al. showed immunity after vaccination with BTN162b2 already drops to around 50% after only 6 months (30).
In contrast, protection induced by “natural” infection seems to be better preserved over time, with protection rates of 85-95% against symptomatic infection up to one year after infection, even against VOC such as the Beta variant (30–32). The decline of antibody titers is also reported to be slower after natural infection compared to vaccination up to 6 months (33). Our findings of high antibody levels and surrogate neutralizing titers early after vaccination, but faster decline compared to naturally induced immunity, corroborate and extend the findings of these studies up to 10 months and against four VOC. We confirmed lower neutralizing activity against 3 VOC that contain spike mutations conferring immune escape such as the E484K (Beta and Gamma) and the L452R and T478K (Delta) (4, 34–36). The Beta and Gamma VOC show the greatest reduction in %inhibition compared to WT, corresponding to the greatest immune escape, while intermediary levels were noted for the Delta variant. High pseudoneutralization values against WT and 4 VOC at 10 months predicted the presence of neutralizing antibodies against the Omicron BA.1 with 17/20 (85%) of the samples with >90% inhibition against WT showing at least 50% neutralization against Omicron in live virus neutralization at a 1/100 dilution. High surrogate neutrolizing antibody titers against Beta and Gamma VOC, the two VOC with the highest immune escape, were the strongest predictors of Omicron neutralization.
Individuals with hybrid immunity have been shown to exhibit the highest (neutralizing) antibody levels and the best protection against reinfection (8, 37–39). This is already apparent in the first weeks after vaccination and persists up to 8 months (8, 37–39). Individuals with hybrid immunity have higher peak antibody levels and a slower decline over time up to 10 months (33, 33, 40). Our results confirm higher antibody levels and a slower decline up to 10 months in HCW with hybrid immunity. In addition, we showed that surrogate neutralizing activity was highest in the hybrid immunity cohort both at 3 and 10 months.
Taken together, our data support the administration of a booster dose within one year after initial vaccination with two doses of BNT162b2, especially in COVID-19 naive individuals where antibody levels and neutralizing capacity have waned significantly by 10 months. Booster doses have been shown to increase (neutralizing) antibody titers and concomitant protection levels, providing the rationale for the widespread booster program rollout around the world (41). The approach is further supported by the fact that immunity after vaccination with 2 doses of BNT162b2 against the recent Omicron variant showed a similar course with a peak around 65.5%, dropping to only 8.8% after more than 25 weeks in COVID-19 naive individuals while protection against reinfection was still 56.0% over 300 days after natural infection (26, 42).
A strength of our study is the use of three cohorts of HCW with comparable demographics with follow-up up to 10 months. There are also a few limitations to our study. A first limitation is the relatively small number of participants. We believe that the same participants were sampled at different time points and the comparable demographics of the different cohorts at least in part offset this limitation. A second limitation is the lack of a live-virus PRNT for WT and 4 VOC which is the golden standard technique for analyzing the neutralizing activity of serum. Nevertheless, a good correlation between pseudoneutralization and PRNT has previously been demonstrated and is supported by our data (15, 16). Finally, we did not assess cellular components of adaptive immunity which might play an important role in the persistence of protection against (severe) disease over time (43).
Conclusion
Hybrid immunity resulted in the strongest immune response at 3 and 10 months for WT and four VOC. Vaccination in COVID-19 naive HCW resulted in a stronger humoral immune response compared to natural infection at 3 months, but not at 10 months. This was due to a faster decline of IgG anti-S and surrogate neutralizing antibody levels after vaccination in COVID-19-naive HCW, compared to natural infection. There was linear relationship between log10 of binding IgG anti-S and surrogate neutralizing antibodies for WT and four VOC, although the binding antibody level corresponding to 90% inhibition varied depending on the strain.
Data Availability Statement
The raw data supporting the conclusions of this article will be made available by the authors, without undue reservation.
Ethics Statement
The studies involving human participants were reviewed and approved by The Ethics Committee Research (EC Research) of University Hospitals Leuven (UZ Leuven). The patients/participants provided their written informed consent to participate in this study.
Author Contributions
Conceptualization: JE and PV; Methodology: PV, JE, JW, and BD; Formal analysis: BD and JW; Investigation: BD, JW, BH, and SS; Resources: GP and LG; Data curation: BD, JW, PM, BH, and JE; Writing – original draft preparation: JE, BD, and PV; Writing – review and editing: JE, BD, PV, SS, GP, LG, XB, JW, and PM; Visualization: BD, JW, and JE; Supervision: PV, PM, and JW; Project administration: PV; Funding acquisition: PV. All authors contributed to the article and approved the submitted version.
Conflict of Interest
PV reports personal fees from Roche, outside the submitted work.
The remaining authors declare that the research was conducted in the absence of any commercial or financial relationships that could be construed as a potential conflict of interest.
Publisher’s Note
All claims expressed in this article are solely those of the authors and do not necessarily represent those of their affiliated organizations, or those of the publisher, the editors and the reviewers. Any product that may be evaluated in this article, or claim that may be made by its manufacturer, is not guaranteed or endorsed by the publisher.
Acknowledgments
PV is a senior clinical investigator of the FWO-Vlaanderen. We thank Myrthe Claesen and Helena Van den Black for their contribution with sample collection.
Supplementary Material
The Supplementary Material for this article can be found online at: https://www.frontiersin.org/articles/10.3389/fimmu.2022.909910/full#supplementary-material
References
1. Thomas SJ, Moreira ED, Kitchin N, Absalon J, Gurtman A, Lockhart S, et al. Safety and Efficacy of the BNT162b2 mRNA Covid-19 Vaccine Through 6 Months. New Engl J Med (2021) 385:1761–73. doi: 10.1056/NEJMoa2110345
2. Haas EJ, Angulo FJ, McLaughlin JM, Anis E, Singer SR, Khan F, et al. Impact and Effectiveness of mRNA BNT162b2 Vaccine Against SARS-CoV-2 Infections and COVID-19 Cases, Hospitalisations, and Deaths Following a Nationwide Vaccination Campaign in Israel: An Observational Study Using National Surveillance Data. Lancet (2021) 397:1819–29. doi: 10.1016/S0140-6736(21)00947-8
3. Khoury DS, Cromer D, Reynaldi A, Schlub TE, Wheatley AK, Juno JA, et al. Neutralizing Antibody Levels are Highly Predictive of Immune Protection From Symptomatic SARS-CoV-2 Infection. Nat Med (2021) 27:1–7. doi: 10.1038/s41591-021-01377-8
4. Andrews N, Tessier E, Stowe J, Gower C, Kirsebom F, Simmons R, et al. Duration of Protection Against Mild and Severe Disease by Covid-19 Vaccines. New Engl J Med (2022) 386:340–50. doi: 10.1056/nejmoa2115481
5. Collie S, Champion J, Moultrie H, Bekker L-G, Gray G. Effectiveness of BNT162b2 Vaccine Against Omicron Variant in South Africa. New Engl J Med (2022) 386:494–6. doi: 10.1056/nejmc2119270
6. Benning L, Morath C, Bartenschlager M, Reineke M, Töllner M, Nusshag C, et al. Neutralizing Antibody Activity Against the B.1.617.2 (Delta) Variant 8 Months After Two-Dose Vaccination With BNT162b2 in Health Care Workers. Clin Microbiol Infect (2022). doi: 10.1016/J.CMI.2022.01.011
7. Levin EG, Lustig Y, Cohen C, Fluss R, Indenbaum V, Amit S, et al. Waning Immune Humoral Response to BNT162b2 Covid-19 Vaccine Over 6 Months. New Engl J Med (2021) 385:e84. doi: 10.1056/nejmoa2114583
8. García-Cruces-Méndez JF, Corral-Gudino L, Del-Amo-Merino MP, Eiros-Bouza JM, Domínguez-Gil González M. SARS-CoV-2 Antibody Response Eight Months After Vaccination With mRNA Vaccines. Influence of Prior SARS-CoV-2 Exposure. Eur J Intern Med (2022) 97:113–5. doi: 10.1016/J.EJIM.2022.01.011
9. Van Elslande J, Gruwier L, Godderis L, Vermeersch P. Estimated Half-Life of SARS-CoV-2 Anti-Spike Antibodies More Than Double the Half-Life of Anti-Nucleocapsid Antibodies in Healthcare Workers. Clin Infect Dis (2021) 73(12):2366–8. doi: 10.1093/cid/ciab219
10. Van Elslande J, Oyaert M, Lorent N, Vande Weygaerde Y, Van Pottelbergh G, Godderis L, et al. Lower Persistence of Anti-Nucleocapsid Compared to Anti-Spike Antibodies Up to One Year After SARS-CoV-2 Infection. Diagn Microbiol Infect Dis (2022) 103(1):115659. doi: 10.1016/j.diagmicrobio.2022.115659
11. Cromer D, Steain M, Reynaldi A, Schlub TE, Wheatley AK, Juno JA, et al. Neutralising Antibody Titres as Predictors of Protection Against SARS-CoV-2 Variants and the Impact of Boosting: A Meta-Analysis. Lancet Microbe (2022) 3:e52–61. doi: 10.1016/S2666-5247(21)00267-6
12. Gilbert PB, Montefiori DC, McDermott AB, Fong Y, Benkeser D, Deng W, et al. Immune Correlates Analysis of the mRNA-1273 COVID-19 Vaccine Efficacy Clinical Trial. Science (2022) 375(6576). doi: 10.1126/science.abm3425
13. Bergwerk M, Gonen T, Lustig Y, Amit S, Lipsitch M, Cohen C, et al. Covid-19 Breakthrough Infections in Vaccinated Health Care Workers. New Engl J Med (2021) 385:1474–84. doi: 10.1056/nejmoa2109072
14. Wang Y. Standardised Neutralising Antibody Assays are Needed for Evaluating COVID-19 Vaccines. EBioMedicine (2021) 73:103677. doi: 10.1016/J.EBIOM.2021.103677
15. Tan CW, Chia WN, Qin X, Liu P, Chen MIC, Tiu C, et al. A SARS-CoV-2 Surrogate Virus Neutralization Test Based on Antibody-Mediated Blockage of ACE2–spike Protein–Protein Interaction. Nat Biotechnol (2020) 38(9):1073–8. doi: 10.1038/s41587-020-0631-z
16. McDade TW, Demonbreun AR, Sancilio A, Mustanski B, D’Aquila RT, McNally EM. Durability of Antibody Response to Vaccination and Surrogate Neutralization of Emerging Variants Based on SARS-CoV-2 Exposure History. Sci Rep (2021) 17325. doi: 10.1038/s41598-021-96879-3
17. Lippi G, Henry BM, Plebani M. Optimizing Effectiveness of COVID-19 Vaccination: Will Laboratory Stewardship Play a Role? Clin Chem Lab Med (2021) 59:1885–8. doi: 10.1515/CCLM-2021-0972/PDF
18. Padoan A, Bonfante F, Pagliari M, Bortolami A, Negrini D, Zuin S, et al. Analytical and Clinical Performances of Five Immunoassays for the Detection of SARS-CoV-2 Antibodies in Comparison With Neutralization Activity. EBioMedicine (2020) 62:103101. doi: 10.1016/j.ebiom.2020.103101
19. Van Elslande J, Weemaes M, Godderis L, Van Pottelbergh G, Bossuyt X, Vermeersch P. IgG Anti-Spike Antibody Levels in Healthcare Workers With and Without Prior COVID-19 Up to 3 Months After BNT162b2 Vaccination. Diagn Microbiol Infect Dis (2022) 102:115638. doi: 10.1016/j.diagmicrobio.2022.115638
20. Meso Scale Diagnostics, MSD-MULTI-SPOT Assay System V-PLEX COVID-19 ACE2 Neutralisation Kits. https://www.mesoscale.com/~/media/files/product%20inserts/v-plex%20covid-19%20serology%20assays%20insert.pdf [product insert]. [Rockville, USA]. [accessed May 31, 2022].
21. Devos T, van Thillo Q, Compernolle V, Najdovski T, Romano M, Dauby N, et al. Early High Antibody Titre Convalescent Plasma for Hospitalised COVID-19 Patients: DAWn-Plasma. Eur Respir J (2022) 2101724. doi: 10.1183/13993003.01724-2021
22. Hu Y-F, Hu J-C, Gong H-R, Danchin A, Sun R, Chu H, et al. Computation of Antigenicity Predicts SARS-CoV-2 Vaccine Breakthrough Variants. Front Immunol (2022) 13:861050/BIBTEX. doi: 10.3389/FIMMU.2022.861050/BIBTEX
23. Jääskeläinen AJ, Kuivanen S, Kekäläinen E, Ahava MJ, Loginov R, Kallio-Kokko H, et al. Performance of Six SARS-CoV-2 Immunoassays in Comparison With Microneutralisation. J Clin Virol (2020) 129:104512. doi: 10.1016/j.jcv.2020.104512
24. Legros V, Denolly S, Vogrig M, Boson B, Siret E, Rigaill J, et al. A Longitudinal Study of SARS-CoV-2-Infected Patients Reveals a High Correlation Between Neutralizing Antibodies and COVID-19 Severity. Cell Mol Immunol (2021) 18:318–27. doi: 10.1038/s41423-020-00588-2
25. Ferrari D, Clementi N, Spanò SM, Albitar-Nehme S, Ranno S, Colombini A, et al. Harmonization of Six Quantitative SARS-CoV-2 Serological Assays Using Sera of Vaccinated Subjects. Clin Chim Acta (2021) 522:144–51. doi: 10.1016/J.CCA.2021.08.024
26. Andrews N, Stowe J, Kirsebom F, Toffa S, Rickeard T, Gallagher E, et al. Covid-19 Vaccine Effectiveness Against the Omicron (B.1.1.529) Variant. New Engl J Med (2022) 386:1532–46. doi: 10.1056/nejmoa2119451
27. Goldberg Y, Mandel M, Bar-On YM, Bodenheimer O, Freedman L, Haas EJ, et al. Waning Immunity After the BNT162b2 Vaccine in Israel. New Engl J Med (2021) 385:e85. doi: 10.1056/nejmoa2114228
28. Mizrahi B, Lotan R, Kalkstein N, Peretz A, Perez G, Ben-Tov A, et al. Correlation of SARS-CoV-2-Breakthrough Infections to Time-From-Vaccine. Nat Commun (2021) 12(1):6379. doi: 10.1038/s41467-021-26672-3
29. Mazzoni A, Vanni A, Spinicci M, Lamacchia G, Kiros ST, Rocca A, et al. SARS-CoV-2 Infection and Vaccination Trigger Long-Lived B and CD4+ T Lymphocytes With Implications for Booster Strategies. J Clin Invest (2022) 132:e157990 . doi: 10.1172/JCI157990
30. Sheehan MM, Reddy AJ, Rothberg MB. Reinfection Rates Among Patients Who Previously Tested Positive for Coronavirus Disease 2019: A Retrospective Cohort Study. Clin Infect Dis (2021) 73:1882–6. doi: 10.1093/CID/CIAB234
31. Kojima N, Klausner JD. Protective Immunity After Recovery From SARS-CoV-2 Infection. Lancet Infect Dis (2022) 22:12–4. doi: 10.1016/S1473-3099(21)00676-9
32. Chemaitelly H, Bertollini R, Abu-Raddad LJ. Efficacy of Natural Immunity Against SARS-CoV-2 Reinfection With the Beta Variant. New Engl J Med (2021) 385:2585–6. doi: 10.1056/nejmc2110300
33. Wei J, Pouwels KB, Stoesser N, Matthews PC, Diamond I, Studley R, et al. Antibody Responses and Correlates of Protection in the General Population After Two Doses of the ChAdOx1 or BNT162b2 Vaccines. Nat Med (2022) 2022:1–11. doi: 10.1038/s41591-022-01721-6
34. Wall EC, Wu M, Harvey R, Kelly G, Warchal S, Sawyer C, et al. Neutralising Antibody Activity Against SARS-CoV-2 VOCs B.1.617.2 and B.1.351 by BNT162b2 Vaccination. Lancet (2021) 397:2331. doi: 10.1016/S0140-6736(21)01290-3
35. Planas D, Veyer D, Baidaliuk A, Staropoli I, Guivel-Benhassine F, Rajah MM, et al. Reduced Sensitivity of SARS-CoV-2 Variant Delta to Antibody Neutralization. Nature (2021) 2021:1–7. doi: 10.1038/s41586-021-03777-9
36. Xie X, Liu Y, Liu J, Zhang X, Zou J, Fontes-Garfias CR, et al. Neutralization of SARS-CoV-2 Spike 69/70 Deletion, E484K and N501Y Variants by BNT162b2 Vaccine-Elicited Sera. Nat Med (2021) 27:620–1. doi: 10.1038/s41591-021-01270-4
37. Ebinger JE, Fert-Bober J, Printsev I, Wu M, Sun N, Prostko JC, et al. Antibody Responses to the BNT162b2 mRNA Vaccine in Individuals Previously Infected With SARS-CoV-2. Nat Med (2021) 27:1–4. doi: 10.1038/s41591-021-01325-6
38. Bates TA, McBride SK, Leier HC, Guzman G, Lyski ZL, Schoen D, et al. Vaccination Before or After SARS-CoV-2 Infection Leads to Robust Humoral Response and Antibodies That Effectively Neutralize Variants. Sci Immunol (2022) 7:8014. doi: 10.1126/sciimmunol.abn8014
39. Abu-Raddad LJ, Chemaitelly H, Ayoub HH, Yassine HM, Benslimane FM, Al Khatib HA, et al. Association of Prior SARS-CoV-2 Infection With Risk of Breakthrough Infection Following mRNA Vaccination in Qatar. JAMA (2021) 326:1930–9. doi: 10.1001/JAMA.2021.19623
40. Hall V, Foulkes S, Insalata F, Kirwan P, Saei A, Atti A, et al. Protection Against SARS-CoV-2 After Covid-19 Vaccination and Previous Infection. New Engl J Med (2022) 386:1207–20. doi: 10.1056/nejmoa2118691
41. Barda N, Dagan N, Cohen C, Hernán MA, Lipsitch M, Kohane IS, et al. Effectiveness of a Third Dose of the BNT162b2 mRNA COVID-19 Vaccine for Preventing Severe Outcomes in Israel: An Observational Study. Lancet (2021) 398:2093–100. doi: 10.1016/S0140-6736(21)02249-2
42. Altarawneh HN, Chemaitelly H, Hasan MR, Ayoub HH, Qassim S, AlMukdad S, et al. Protection Against the Omicron Variant From Previous SARS-CoV-2 Infection. N Engl J Med volume. (2022) 386(13):1288–1290. doi: 10.1056/NEJMC2200133
Keywords: SARS-CoV-2, COVD-19 serological testing, neutralizing antibodies, vaccination, spike, IgG, immunity, COVID-19
Citation: Decru B, Van Elslande J, Steels S, Van Pottelbergh G, Godderis L, Van Holm B, Bossuyt X, Van Weyenbergh J, Maes P and Vermeersch P (2022) IgG Anti-Spike Antibodies and Surrogate Neutralizing Antibody Levels Decline Faster 3 to 10 Months After BNT162b2 Vaccination Than After SARS-CoV-2 Infection in Healthcare Workers. Front. Immunol. 13:909910. doi: 10.3389/fimmu.2022.909910
Received: 31 March 2022; Accepted: 16 May 2022;
Published: 15 June 2022.
Edited by:
Massimo Pieri, University of Rome Tor Vergata, ItalyReviewed by:
Eleonora Nicolai, University of Rome Tor Vergata, ItalyAnna Vanni, University of Florence, Italy
Copyright © 2022 Decru, Van Elslande, Steels, Van Pottelbergh, Godderis, Van Holm, Bossuyt, Van Weyenbergh, Maes and Vermeersch. This is an open-access article distributed under the terms of the Creative Commons Attribution License (CC BY). The use, distribution or reproduction in other forums is permitted, provided the original author(s) and the copyright owner(s) are credited and that the original publication in this journal is cited, in accordance with accepted academic practice. No use, distribution or reproduction is permitted which does not comply with these terms.
*Correspondence: Pieter Vermeersch, pieter.vermeersch@uzleuven.be
†These authors contributed equally to this work and share first authorship
‡These authors have contributed equally to this work and share senior authorship