- 1Department of Pathology, The First Hospital of China Medical University, Shenyang, Liaoning, China
- 2Department of Pathology, College of Basic Medical Sciences, China Medical University, Shenyang, Liaoning, China
- 3Department of Pathology, Sichuan Cancer Hospital & Institute, Sichuan Cancer Center, School of Medicine, University of Electronic Science and Technology of China, Chengdu, Sichuan, China
Differentiated embryo-chondrocyte expressed gene 2 (DEC2) is a member of the basic helix-loop-helix (bHLH) subfamily of transcription factors. DEC2 is implicated in tumor immunotherapy, immune system function regulation, and autoimmune diseases. DEC2 enhances Th2 cell differentiation by regulating the IL-2 and IL-4 signaling pathways and mediates the growth of B-1a cells, thereby promoting the occurrence and development of inflammatory responses. In this study, we review the reported roles of DEC2, including the regulation of immune cell differentiation and cytokine production in various cells in humans, and discuss its potential in treating autoimmune diseases and tumors.
1 Introduction
DEC2, also known as BHLHE41 (class E basic helix-loop-helix protein 41)/BHLHB3 (Class B basic helix-loop-helix protein 3), is a bHLH repressor transcription factor (1), whose sequence is similar to that of BHLHE40 (class E basic helix-loop-helix protein 40)/DEC1 (Differentiated embryo-chondrocyte expressed gene 1)/STRA-13 (stimulated with retinoic acid 13). It is encoded by DEC2, located on the human chromosome 12p11.23-p12.1 and comprises 482 amino acid residues with a molecular weight of 50.5 kDa (2). DEC2 is classified as a transcriptional repressor based on its domain and transcriptional properties and shares a high degree of sequence homology with other bHLH transcriptional repressor subfamily members (2). The bHLH family of transcription suppressors performs diverse transcriptional repression functions involving various mechanisms (3, 4). DEC2 achieves transcriptional repression of the target gene promoter activity via DNA binding (5).
Recent studies have demonstrated the crucial role of DEC2 in regulating circadian rhythms, immune homeostasis, cell differentiation, regeneration, and metabolism (6–8). DEC2 regulates the circadian rhythm by mediating E-box-dependent transcriptional repression through various mechanisms (9). DEC2 also participates in several pathways with diverse functions that help regulate the biological behavior of immune cells and tumors. For example, DEC2 regulates the self-renewal of B-1a cells (10). In cervical cancer, DEC2 inhibits the epithelial–mesenchymal transition (EMT) and tumor metastasis through the Notch signaling pathway (11). In gastric cancer, DEC2 inhibits EMT-related metastasis by inactivating several pathways, including the ERK/NF-κB (12) and PI3K/Akt pathways (13). In addition, DEC2 regulates the differentiation of Th2 cells (7) and the self-renewal of alveolar macrophages (AMs) (14). DEC2 is also closely associated with tumors, with its transcription level serving as a marker of cancer progression (12, 13, 15–18).
Despite the complex structure and diverse immune functions of DEC2, its role in the immune system remains inadequately understood. Therefore, additional investigations are necessary to ascertain DEC2’s potential in diagnosing and treating autoimmune diseases, as well as identifying novel targets for tumor treatment. Exploring the role and potential of DEC2 in diagnosing and treating autoimmune diseases offers avenues for identifying new therapeutic directions in oncology.
2 DEC2 and immune cells
2.1 DEC2 and T cells
Naive CD4+ T cells possess the ability to undergo differentiation into distinct Th cell subsets, including Th1, Th2, and Th17 cells, based on their cytokine expression profiles and lineage-specific transcription factors (12, 13). Specifically, Th2 cells are characterized by the expression of transcription factors such as GATA-3 and type 2 cytokines, including IL-4 (19). During immune responses, the Th2 cell population can significantly expand, serving as a crucial, albeit not exclusive, source of IL-4 (15).The expression of DEC2 is mediated by the IL-4/Stat6 pathway and can be further enhanced by ICOS or IL-25 (20). DEC2 expression is necessary to maintain the continuous differentiation of Th2 cells in later stages (7). Dec2-deficient mice exhibit significant defects in Th2 immune response, and T cell-specific DEC2 transgenic mice are more prone to developing allergic airway inflammation (21). DEC2 deficiency has notable consequences on Th2 cells, leading to a substantial reduction in type 2 cytokine levels. This deficiency also results in diminished expression of key transcription factors, including GATA-3 and JunB (7).. Consequently, DEC2 is preferentially expressed in Th2 cells and plays a crucial role in their differentiation.
There is an autoregulatory cycle between JunB, GATA-3, and DEC2 (21). DEC2 can positively regulate GATA-3 and JunB; that is, the deficiency in type 2 cytokine expression can be alleviated by JunB or GATA-3 expression (21). In addition, DEC2 enhances the sensitivity of Th2 cells to IL-2 by enhancing CD25 expression in a STAT6-dependent manner (7). Therefore, DEC2 promotes Th2 differentiation by activating JunB and GATA-3 expression and promoting early IL-4 production. GATA-3 further regulates DEC2 expression, forming a cycle involving JunB, GATA-3, and DEC2 (21) (Figure 1).
IL-2 promotes IL-4 expression and T helper (Th) 2 cell differentiation via various mechanisms (19). IL-2, a 15.5 kDa cytokine secreted by antigen-activated T cells, plays a vital role in lymphocyte activation and differentiation (22). It is an essential growth factor for T cells and is associated with the initiation of immune responses (23). For example, IL-2 enhances the cytolytic activity of NK cells and tumor-infiltrating lymphocytes, promotes immunoglobulin production in activated B cells, and maintains the homeostatic proliferation of regulatory T cells (Tregs) (22). In addition, IL-2 acts on the innate lymphoid cells, regulates the differentiation of effector T cells, and affects memory T cells, effector T cells, and monocytes (22). DEC2 enhances the IL-2R-mediated signaling pathway and promotes Th2 differentiation (7).
DEC2 induced by IL-4 signaling may help maintain high levels of IL-2R, thus facilitating Th2 cell activation at later stages (7). Therefore, DEC2 is critical in regulating the IL-2 signaling pathway and exerts a synergistic effect with IL-4 to promote Th2 cell differentiation (Figure 2).
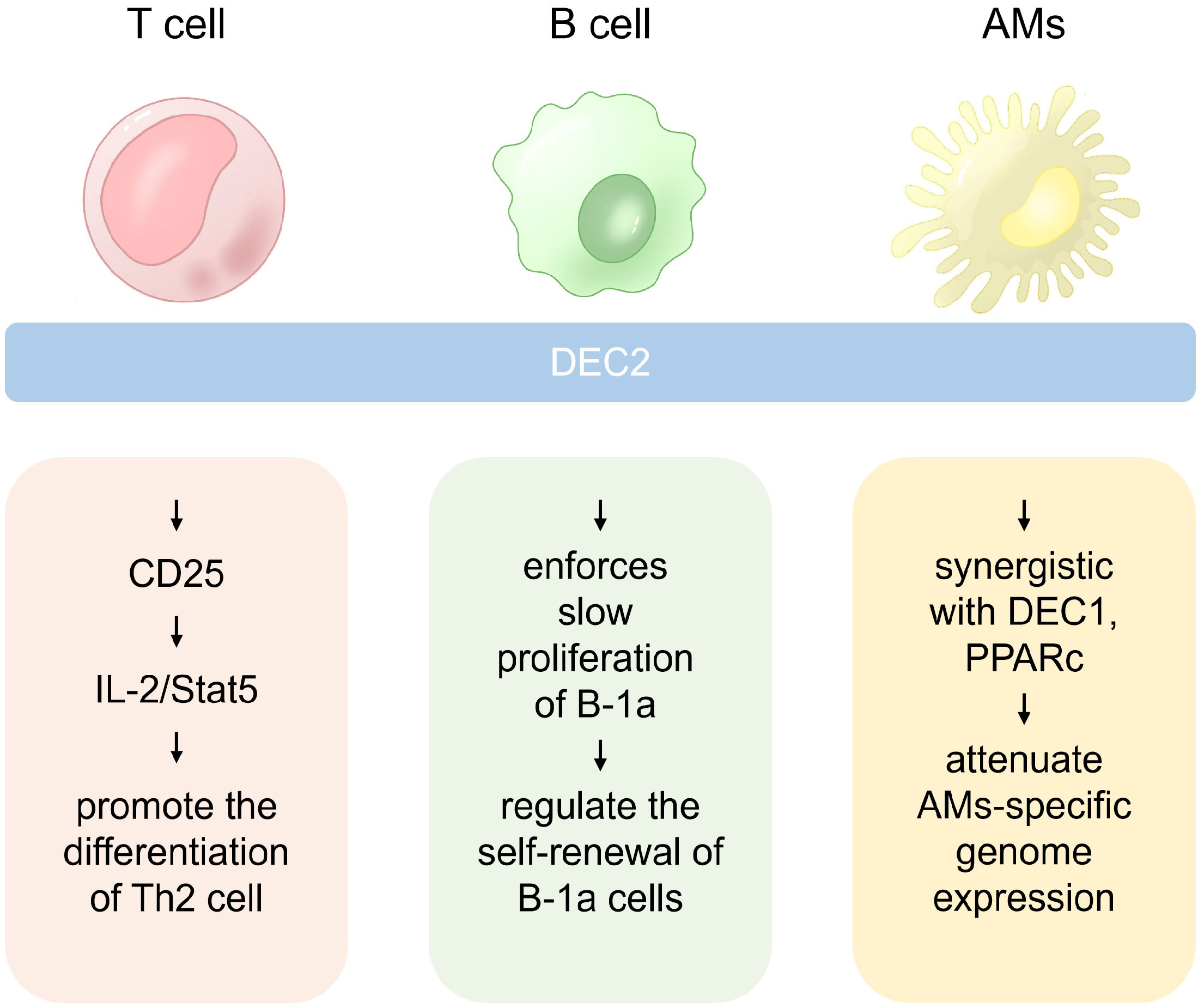
Figure 2 The role of DEC2 in different immune cells. In Th2 cells, DEC2 is pivotal for differentiation, while in B-1a cells, it governs self-renewal by enhancing responsiveness to pro-survival cytokines. Additionally, DEC2 is a critical regulator of alveolar macrophage (AM) self-renewal.
Blockers of the above pathway, such as Rademikibart (24, 25), AK120 (26) and other IL-4Rα antagonists that bind to IL-4Rα, or Black Ginseng Extract (27) can effectively block the IL-4/Stat6 pathway and are indicated for the treatment of Th2-associated inflammatory diseases.
2.2 B cells
The B-1 cell population is an innate B lymphocyte subset first identified over 30 years ago (28). These cells, which are found in the peritoneal and pleural spaces, omentum, and spleen, are considered the first line of defense against pathogens and are involved in maintaining homeostasis in the internal environment (29). B-1 cells protect from infection, regulate Tregs during the initiation of pathogen-specific immunity, and participate in autoimmune diseases (30).
B cells can be categorized as B-1 or B-2 based on their characteristic surface molecular phenotype, and the main subsets of B-1 cells are divided into CD5+ B-1a cells and CD5− B-1b cells (31). DEC2 is crucial in regulating B-1a cell development, self-renewal, and B cell receptor (BCR) repertoire (10, 32). The regulation of DEC2 in B1-a cells can be categorized into organ, cellular, and molecular levels. DEC2 is highly expressed in B-1a and B-1b lymphocytes, lung macrophages, and microglia (33). During B-cell development, DEC2 is expressed at low levels in pre-B, immature B, and plasma cells of the bone marrow and transitional B cells of the spleen (10). DEC2 exhibits elevated expression levels in immature B cells found in fetal and neonatal livers when contrasted with its expression in adult bone marrow. This heightened expression is correlated with an increased tendency for precursor cell production in fetuses and neonates (10). DEC2 is highly expressed in postnatal and adult B-1 cells and is upregulated during cell development.
B-1a cell numbers were significantly reduced in DEC2−/− and DEC1−/− DEC2−/− double-knockout (DKO) mice, highlighting the critical role of DEC2 in B-1a cell differentiation and stabilization (10). Mutated B-1a cells develop abnormal cell phenotypes, accompanied by a notable alteration in the BCR repertoire. This is illustrated by the absence of the PTC-specific VH12/Vκ4 BCR (10). At the molecular level, DEC2 coordinates with DEC1 to mediate cell cycle arrest by directly inhibiting cell cycle regulators, including E2F transcription factors (10). Additionally, DEC2 also controls the self-renewal of B-1a cells by rendering B-1a cells receptive to pro-survival cytokine signaling (10, 21).
DEC2 also limits the number of B-1a cells in human adulthood (10, 34). Thus, DEC2 controls B-1a cells in multiple ways by regulating their development, BCR repertoire, and self-renewal (Figure 2).
2.3 Other immune cells
Macrophages are the first line of defense against pathogens (35). Most alveolar macrophages (AMs) differentiate after exposure to granulocyte-macrophage colony-stimulating factor (GM-CSF) produced by alveolar epithelial cells (36). When cells are exposed to GM-CSF and TGFβ, it leads to the activation of PPARc expression. This activation of PPARc expression, in turn, initiates the transcription of RNA essential for the function of AMs. The intricate process involves collaboration with other transcription factors, such as BACH1, BACH2, and CEBPb (37).
DEC2 is a regulator of AMs that perform homeostatic functions in the alveoli (14). DEC2 and DEC1 attenuate the expression of certain genes described below. Genes that comprise an “AM signature”, such as Epcam and Acaa1b, were upregulated in DEC2/DEC1-deficient AMs (14). However, the macrophage population with “a non-AM signature” showed the upregulation of widely expressed genes, including genes encoding the complement component C1q (C1qa, C1qb, and C1qc), transcription factor MafB (Mafb), cholesterol carrier apolipoprotein E (ApoE), and chemokine-like receptor 1 (Cnklr1) (14). Therefore, DEC2 emerges as a crucial regulator in AM self-renewal, serving as the guardian of characteristic genes. Its involvement in the control of tissue-specific functions of macrophages suggests likely interactions with other transcription factors (Figure 2).
Macrophages serve as crucial innate immune defenders within tissues, particularly in combating lipopolysaccharide (LPS)-induced periodontal inflammation (38). Activation of macrophages triggers the release of proinflammatory cytokines, including interleukin (IL)-1β. LPS can activate caspase-11 and stimulate the secretion of IL-1β, which subsequently triggers pyroptosis. He et al. (39) reported that DEC2 overexpression reduced IL-1β expression in Porphyromonas gingivalis LPS-induced macrophages. Consequently, DEC2 deficiency in periodontal macrophages aggravated P. gingivalis LPS-induced periodontal inflammation and pyroptosis.
3 DEC2 in immunoregulation and tumor therapy
In the tumor microenvironment, cancer cells evade host immunity through several pathways. One of the most critical components of this pathway is the immunosuppressive co-signal (immune checkpoint) mediated by programmed death receptor 1 (PD-1) and its ligand, PD-L1 (40, 41). PD-L1 binds to the PD-1 receptor expressed on T cells and tumor-associated macrophages (TAM) and blocks antitumor activity by inducing their apoptosis (42, 43). Notably, therapeutic interventions targeting these immune checkpoints, specifically PD-1 and PD-L1, have received regulatory approval for the treatment of specific malignancies (44, 45).
In this immunologically dynamic context, Tsuruta et al. reported (46) that DEC2 exhibits diurnal fluctuations in its expression within tumor-associated macrophages (TAMs), exerting periodic suppression on NF-κB-induced transactivation of the Pdcd1 gene in RAW264.7 cells. This cyclic inhibition may contribute to the diurnal expression pattern of PD-1 in TAMs. Li et al. (12) demonstrated that DEC2 could also inhibit tumor proliferation and metastasis by regulating the NF-κB pathway in gastric cancer.
4 DEC2 and autoimmune diseases
Autoimmune diseases, such as systemic lupus erythematosus (SLE) and rheumatoid arthritis (RA), typically involve multiple tissues and organs (47, 48). DEC2 plays a crucial role in the pathogenesis and treatment of autoimmune diseases.
RA is a common chronic inflammatory joint disease in which IL-1β is a critical pathogenic factor (49). DEC2 is closely associated with RA etiology. DEC2 increases IL-1β expression in Th2 cells and is abundantly expressed in the RA synovium (50). DEC2 directly regulates IL-1β expression in HEK293 cells and primary human fibroblasts (50). The mRNA and protein expression of DEC2 is increased in synovial fibroblasts in an NF-κB -dependent manner under the influence of TNF-α (50).
SLE is a chronic inflammatory autoimmune disease characterized by the production of large amounts of heterogeneous autoantibodies against self-antigens (51); its most dangerous clinical manifestation is lupus nephritis. Imaizumi et al. reported the possibility of a DEC2-mediated IFN-β/RIG-I/CCL5 negative feedback loop (52), which they hypothesize that may play a role in controlling renal inflammation and antiviral immune responses, leading to inflammatory kidney diseases, such as lupus nephritis. However, it is unclear whether the negative feedback loop has a pro-inflammatory or anti-inflammatory function in the pathogenesis of inflammatory kidney disease and whether it is beneficial or harmful to the host. Qi et al. (53) confirmed that miR-16 reduces the possibility of renal tissue dysplasia and glomerular injury in Fcgr2b−/− mice by downregulating DEC2 and inhibiting thylakoid cell proliferation.
5 Perspectives and conclusion
DEC2 plays vital roles in circadian and non-circadian regulation, such as cell differentiation, regeneration, and maintenance of immune homeostasis. DEC2 is involved in regulating various immune cells. In Th2 cells, DEC2 promotes production through the IL-4/Stat6 pathway. It also enhances the IL-2R-mediated signaling pathway and promotes Th2 differentiation in a Stat6-dependent manner. DEC2 promotes development, self-renewal, and BCR repertoire formation in B-1a cells at various levels. The expression of DEC2 is markedly elevated in certain lymphocytes, lung macrophages, microglia, as well as immature B cells in the fetal and neonatal liver, whereas it is comparatively lower in pre-B cells, immature B cells, adult bone marrow plasma cells, and spleen migratory B cells. DEC2 exhibits the capability to inhibit the periodicity of B-1a cell cycle, regulate their self-renewal, and maintain the balance between B-1 and B-2 cell. DEC2 can also participate in the regulation of tissue-specific functions of AMs through interaction with other transcription factors. In periodontal tissue macrophages, DEC2 deficiency exacerbates periodontal inflammation and pyroptosis. In tumor immunity, DEC2 inhibits tumor growth and migration by regulating cell cycle proteins, epithelial–mesenchymal transition, and hypoxia-inducible factors (12, 15, 18, 54, 55). In autoimmune diseases, such as RA and SLE, DEC2 expression is abnormal and promotes the production of pathogenic factors such as IL-1β. Research on DEC2 has provided novel insights into the treatment of tumors and autoimmune diseases. However, owing to the complex structure of DEC2 and the large number of regulatory factors and signaling pathways involved, most current studies on its function are at the in vitro level. Further investigation is required to elucidate its mechanisms of immune regulation in vivo.
Author contributions
YJL: Writing – original draft. YM: Writing – original draft. WZ: Writing – original draft. YL: Writing – original draft. JH: Writing – original draft. NT: Writing – original draft. DZ: Writing – review & editing.
Funding
The author(s) declare that financial support was received for the research, authorship, and/or publication of this article. This work was supported by the Natural Science Foundation of Liaoning Province (grant No. 2021-BS-106 to DZ), the National Natural Science Foundation of China (grant No. 81672302 to DZ, No. 81302312 to YL), General Project of Liaoning Provincial Education Department Foundation (grant No. LK201638 to DZ), the Natural Science Foundation of Liaoning Province (grant No. 20170540989 to YL), the Scientific Research Funds project of ​ Education Department of Liaoning Province (grant no. JC2019027 to YL), the Scientific Research Funds project of Science and Technology Department of Sichuan Province (grant no. 21YYJC1616 to YL), and the Scientific Research Project of Sichuan Province (grant no. S2002 to YL).
Conflict of interest
The authors declare that the research was conducted in the absence of any commercial or financial relationships that could be construed as a potential conflict of interest.
Publisher’s note
All claims expressed in this article are solely those of the authors and do not necessarily represent those of their affiliated organizations, or those of the publisher, the editors and the reviewers. Any product that may be evaluated in this article, or claim that may be made by its manufacturer, is not guaranteed or endorsed by the publisher.
References
1. Rossner MJ, Dörr J, Gass P, Schwab MH, Nave KA. SHARPs: mammalian enhancer-of-split- and hairy-related proteins coupled to neuronal stimulation. Mol Cell Neurosci. (1997) 9:460–75. doi: 10.1006/mcne.1997.0640
2. Fujimoto K, Shen M, Noshiro M, Matsubara K, Shingu S, Honda K, et al. Molecular cloning and characterization of DEC2, a new member of basic helix-loop-helix proteins. Biochem Biophys Res Commun. (2001) 280:164–71. doi: 10.1006/bbrc.2000.4133
3. Murre C, Bain G, van Dijk MA, Engel I, Furnari BA, Massari ME, et al. Structure and function of helix-loop-helix proteins. Biochim Biophys Acta. (1994) 1218:129–35. doi: 10.1016/0167-4781(94)90001-9
4. Edwards HE, Gorelick DA. The evolution and structure/function of bHLH-PAS transcription factor family. Biochem Soc Trans. (2022) 50:1227–43. doi: 10.1042/BST20211225
5. Li Y, Xie M, Song X, Gragen S, Sachdeva K, Wan Y, et al. DEC1 negatively regulates the expression of DEC2 through binding to the E-box in the proximal promoter. J Biol Chem. (2003) 278:16899–907. doi: 10.1074/jbc.M300596200
6. Ow JR, Tan YH, Jin Y, Bahirvani AG, Taneja R. Stra13 and Sharp-1, the non-grouchy regulators of development and disease. Curr Top Dev Biol. (2014) 110:317–38. doi: 10.1016/B978-0-12-405943-6.00009-9
7. Liu Z, Li Z, Mao K, Zou J, Wang Y, Tao Z, et al. Dec2 promotes Th2 cell differentiation by enhancing IL-2R signaling. J Immunol. (2009) 183:6320–9. doi: 10.4049/jimmunol.0900975
8. Honma S, Kawamoto T, Takagi Y, Fujimoto K, Sato F, Noshiro M, et al. Dec1 and Dec2 are regulators of the mammalian molecular clock. Nature. (2002) 419:841–4. doi: 10.1038/nature01123
9. Fujimoto K, Hamaguchi H, Hashiba T, Nakamura T, Kawamoto T, Sato F, et al. Transcriptional repression by the basic helix-loop-helix protein Dec2: multiple mechanisms through E-box elements. Int J Mol Med. (2007) 19:925–32. doi: 10.3892/ijmm
10. Kreslavsky T, Vilagos B, Tagoh H, Poliakova DK, Schwickert TA, Wöhner M, et al. Essential role for the transcription factor Bhlhe41 in regulating the development, self-renewal and BCR repertoire of B-1a cells. Nat Immunol. (2017) 18:442–55. doi: 10.1038/ni.3694
11. Liao Y, He X, Qiu H, Che Q, Wang F, Lu W, et al. Suppression of the epithelial-mesenchymal transition by SHARP1 is linked to the NOTCH1 signaling pathway in metastasis of endometrial cancer. BMC Cancer. (2014) 14:487. doi: 10.1186/1471-2407-14-487
12. Li P, Jia Y-F, Ma X-L, Zheng Y, Kong Y, Zhang Y, et al. DEC2 suppresses tumor proliferation and metastasis by regulating ERK/NF-κB pathway in gastric cancer. Am J Cancer Res. (2016) 6:1741–57.
13. Wu Y, Sato H, Suzuki T, Yoshizawa T, Morohashi S, Seino H, et al. Involvement of c-Myc in the proliferation of MCF-7 human breast cancer cells induced by bHLH transcription factor DEC2. Int J Mol Med. (2015) 35:815–20. doi: 10.3892/ijmm.2014.2042
14. Rauschmeier R, Gustafsson C, Reinhardt A, A-Gonzalez N, Tortola L, Cansever D, et al. Bhlhe40 and Bhlhe41 transcription factors regulate alveolar macrophage self-renewal and identity. EMBO J. (2019) 38:e101233. doi: 10.15252/embj.2018101233
15. Furukawa T, Mimami K, Nagata T, Yamamoto M, Sato M, Tanimoto A. Approach to functions of BHLHE41/DEC2 in non-small lung cancer development. Int J Mol Sci. (2023) 24:11731. doi: 10.3390/ijms241411731
16. Asanoma K, Liu G, Yamane T, Miyanari Y, Takao T, Yagi H, et al. Regulation of the mechanism of TWIST1 transcription by BHLHE40 and BHLHE41 in cancer cells. Mol Cell Biol. (2015) 35:4096–109. doi: 10.1128/MCB.00678-15
17. Fang W, Li Q, Wang M, Zheng M, Xu H. DEC2 serves as potential tumor suppressor in breast carcinoma. Dis Markers. (2020) 2020:6053154. doi: 10.1155/2020/6053154
18. Liu Q, Wu Y, Yoshizawa T, Yan X, Morohashi S, Seino H, et al. Basic helix-loop-helix transcription factor DEC2 functions as an anti-apoptotic factor during paclitaxel-induced apoptosis in human prostate cancer cells. Int J Mol Med. (2016) 38:1727–33. doi: 10.3892/ijmm.2016.2798
19. Zhu J. T helper cell differentiation, heterogeneity, and plasticity. Cold Spring Harb Perspect Biol. (2018) 10:a030338. doi: 10.1101/cshperspect.a030338
20. Ho I-C, Miaw S-C. Regulation of IL-4 expression in immunity and diseases. Adv Exp Med Biol. (2016) 941:31–77. doi: 10.1007/978-94-024-0921-5_3
21. Yang XO, Angkasekwinai P, Zhu J, Peng J, Liu Z, Nurieva R, et al. Requirement for the basic helix-loop-helix transcription factor Dec2 in initial TH2 lineage commitment. Nat Immunol. (2009) 10:1260–6. doi: 10.1038/ni.1821
22. Mitra S, Leonard WJ. Biology of IL-2 and its therapeutic modulation: Mechanisms and strategies. J Leukoc Biol. (2018) 103:643–55. doi: 10.1002/JLB.2RI0717-278R
23. Abbas AK, Trotta E R, Simeonov D, Marson A, Bluestone JA. Revisiting IL-2: Biology and therapeutic prospects. Sci Immunol. (2018) 3:eaat1482. doi: 10.1126/sciimmunol.aat1482
24. Silverberg JI, Strober B, Feinstein B, Xu J, Guttman-Yassky E, Simpson EL, et al. Efficacy and safety of rademikibart (CBP-201), a next-generation monoclonal antibody targeting IL-4Rα, in adults with moderate-to-severe atopic dermatitis: a phase 2 randomized trial (CBP-201-WW001). J Allergy Clin Immunol. (2023) S0091-6749(23)02546-0. doi: 10.1016/j.jaci.2023.11.924
25. Zhang L, Ding Y, Wang Q, Pan W, Wei Z, Smith PA, et al. Preclinical immunological characterization of rademikibart (CBP-201), a next-generation human monoclonal antibody targeting IL-4Rα, for the treatment of Th2 inflammatory diseases. Sci Rep. (2023) 13:12411. doi: 10.1038/s41598-023-39311-2
26. Wynne CJ, Cole A, Lemech C, Wang G, Zhang Y, Chen B, et al. Safety, pharmacokinetics and preliminary efficacy of IL4-Rα Monoclonal antibody AK120 in both healthy and atopic dermatitis subjects: A phase I, randomized, two-part, double-blind, placebo-controlled, dose-escalation, first-in-human clinical study. Dermatol Ther (Heidelb). (2023) 13:2357–73. doi: 10.1007/s13555-023-01010-1
27. Song YN, Lee J-W, Ryu HW, Lee JK, Oh ES, Kim D-Y, et al. Black ginseng extract exerts potentially anti-asthmatic activity by inhibiting the protein kinase Cθ-mediated IL-4/STAT6 signaling pathway. Int J Mol Sci. (2023) 24:11970. doi: 10.3390/ijms241511970
28. Kreslavsky T, Wong JB, Fischer M, Skok JA, Busslinger M. Control of B-1a cell development by instructive BCR signaling. Curr Opin Immunol. (2018) 51:24–31. doi: 10.1016/j.coi.2018.01.001
29. Alugupalli KR, Gerstein RM. Divide and conquer: division of labor by B-1 B cells. Immunity. (2005) 23:1–2. doi: 10.1016/j.immuni.2005.07.001
30. Hayakawa K, Hardy RR, Parks DR, Herzenberg LA. The “Ly-1 B” cell subpopulation in normal immunodefective, and autoimmune mice. J Exp Med. (1983) 157:202–18. doi: 10.1084/jem.157.1.202
31. Baumgarth N. The double life of a B-1 cell: self-reactivity selects for protective effector functions. Nat Rev Immunol. (2011) 11:34–46. doi: 10.1038/nri2901
32. Samten B. Regulation of B-1a cells: another novel function of the basic helix-loop-helix transcriptional regulator BHLHE41. Cell Mol Immunol. (2017) 14:802–4. doi: 10.1038/cmi.2017.75
33. Montecino-Rodriguez E, Dorshkind K. B-1 B cell development in the fetus and adult. Immunity. (2012) 36:13–21. doi: 10.1016/j.immuni.2011.11.017
34. Hong H, Lee J, Park G-Y, Kim S, Park J, Park JS, et al. Postnatal regulation of B-1a cell development and survival by the CIC-PER2-BHLHE41 axis. Cell Rep. (2022) 38:110386. doi: 10.1016/j.celrep.2022.110386
35. Ruitenberg EJ. Immunological defence mechanisms and inflammation. Tijdschr Diergeneeskd. (1977) 102:1073–83.
36. Gschwend J, Sherman SPM, Ridder F, Feng X, Liang H-E, Locksley RM, et al. Alveolar macrophages rely on GM-CSF from alveolar epithelial type 2 cells before and after birth. J Exp Med. (2021) 218:e20210745. doi: 10.1084/jem.20210745
37. Schneider C, Nobs SP, Kurrer M, Rehrauer H, Thiele C, Kopf M. Induction of the nuclear receptor PPAR-γ by the cytokine GM-CSF is critical for the differentiation of fetal monocytes into alveolar macrophages. Nat Immunol. (2014) 15:1026–37. doi: 10.1038/ni.3005
38. Davies LC, Jenkins SJ, Allen JE, Taylor PR. Tissue-resident macrophages. Nat Immunol. (2013) 14:986–95. doi: 10.1038/ni.2705
39. He D, Li X, Zhang F, Wang C, Liu Y, Bhawal UK, et al. Dec2 inhibits macrophage pyroptosis to promote periodontal homeostasis. J Periodontal Implant Sci. (2022) 52:28–38. doi: 10.5051/jpis.2101380069
40. Iwai Y, Ishida M, Tanaka Y, Okazaki T, Honjo T, Minato N. Involvement of PD-L1 on tumor cells in the escape from host immune system and tumor immunotherapy by PD-L1 blockade. Proc Natl Acad Sci U S A. (2002) 99:12293–7. doi: 10.1073/pnas.192461099
41. Topalian SL, Taube JM, Anders RA, Pardoll DM. Mechanism-driven biomarkers to guide immune checkpoint blockade in cancer therapy. Nat Rev Cancer. (2016) 16:275–87. doi: 10.1038/nrc.2016.36
42. Wherry EJ, Kurachi M. Molecular and cellular insights into T cell exhaustion. Nat Rev Immunol. (2015) 15:486–99. doi: 10.1038/nri3862
43. Ishida Y, Agata Y, Shibahara K, Honjo T. Induced expression of PD-1, a novel member of the immunoglobulin gene superfamily, upon programmed cell death. EMBO J. (1992) 11:3887–95. doi: 10.1002/embj.1992.11.issue-11
44. Mahoney KM, Freeman GJ, McDermott DF. The next immune-checkpoint inhibitors: PD-1/PD-L1 blockade in melanoma. Clin Ther. (2015) 37:764–82. doi: 10.1016/j.clinthera.2015.02.018
45. Tolba MF, Omar HA. Immunotherapy, an evolving approach for the management of triple negative breast cancer: Converting non-responders to responders. Crit Rev Oncol Hematol. (2018) 122:202–7. doi: 10.1016/j.critrevonc.2018.01.005
46. Tsuruta A, Shiiba Y, Matsunaga N, Fujimoto M, Yoshida Y, Koyanagi S, et al. Diurnal expression of PD-1 on tumor-associated macrophages underlies the dosing time-dependent antitumor effects of the PD-1/PD-L1 inhibitor BMS-1 in B16/BL6 melanoma-bearing mice. Mol Cancer Res. (2022) 20:972–82. doi: 10.1158/1541-7786.MCR-21-0786
47. Rose J. Autoimmune connective tissue diseases: systemic lupus erythematosus and rheumatoid arthritis. Immunol Allergy Clin North Am. (2023) 43:613–25. doi: 10.1016/j.iac.2022.10.006
48. Xiao ZX, Miller JS, Zheng SG. An updated advance of autoantibodies in autoimmune diseases. Autoimmun Rev. (2021) 20:102743. doi: 10.1016/j.autrev.2020.102743
50. Olkkonen J, Kouri V-P, Hynninen J, Konttinen YT, Mandelin J. Differentially expressed in chondrocytes 2 (DEC2) increases the expression of IL-1β and is abundantly present in synovial membrane in rheumatoid arthritis. PloS One. (2015) 10:e0145279. doi: 10.1371/journal.pone.0145279
51. Qi S, Chen Q, Xu D, Xie N, Dai Y. Clinical application of protein biomarkers in lupus erythematosus and lupus nephritis. Lupus. (2018) 27:1582–90. doi: 10.1177/0961203318773643
52. Imaizumi T, Sato F, Tanaka H, Matsumiya T, Yoshida H, Yashiro-Aizawa T, et al. Basic-helix-loop-helix transcription factor DEC2 constitutes negative feedback loop in IFN-β-mediated inflammatory responses in human mesangial cells. Immunol Lett. (2011) 136:37–43. doi: 10.1016/j.imlet.2010.11.009
53. Qi H, Cao Q, Liu Q. MicroRNA-16 directly binds to DEC2 and inactivates the TLR4 signaling pathway to inhibit lupus nephritis-induced kidney tissue hyperplasia and mesangial cell proliferation. Int Immunopharmacol. (2020) 88:106859. doi: 10.1016/j.intimp.2020.106859
54. Liu Q, Wu Y, Seino H, Haga T, Yoshizawa T, Morohashi S, et al. Correlation between DEC1/DEC2 and epithelial−mesenchymal transition in human prostate cancer PC−3 cells. Mol Med Rep. (2018) 18:3859–65. doi: 10.3892/mmr
Keywords: DEC2, DEC1, Bhlhe41, immunity, immune cells
Citation: Li Y, Ma Y, Liu Y, Tang N, Zhang W, Huo J and Zhang D (2024) Role of differentiated embryo-chondrocyte expressed gene 2 in immunity. Front. Immunol. 15:1335473. doi: 10.3389/fimmu.2024.1335473
Received: 09 November 2023; Accepted: 29 February 2024;
Published: 12 March 2024.
Edited by:
Stephen Robert Daley, Queensland University of Technology, AustraliaReviewed by:
Yoontae Lee, Pohang University of Science and Technology, Republic of KoreaUjjal Bhawal, Nihon University, Japan
Copyright © 2024 Li, Ma, Liu, Tang, Zhang, Huo and Zhang. This is an open-access article distributed under the terms of the Creative Commons Attribution License (CC BY). The use, distribution or reproduction in other forums is permitted, provided the original author(s) and the copyright owner(s) are credited and that the original publication in this journal is cited, in accordance with accepted academic practice. No use, distribution or reproduction is permitted which does not comply with these terms.
*Correspondence: Di Zhang, zhangdi_new@hotmail.com
†These authors have contributed equally to this work