- Entomology Laboratory, Department of Zoology, University of Calcutta, Kolkata, West Bengal, India
Ant–plant associations are ubiquitous and highly diverse in almost all terrestrial environments, resulting in complex ecological networks. Although ant–plant mutualism is prevalent, ant-mediated pollination is uncommon, and only a few investigations have demonstrated their role in pollination. Thus, the topic of ant-mediated pollination requires revision to assess its significance in pollination biology. Ants are frequent floral visitors, but their impact on plant reproductive fitness is rarely acknowledged; nonetheless, numerous flower-visiting ants have been investigated for their involvement in promoting floral development and hybrid vigor in crops. In this study, we present a summary of the scientific literature published over the last four decades on ants’ involvement in pollination, the diversity of pollinating ants to various host plants, the ant–plant pollinating networks, and seasonal patterns of ant-mediated pollination. Ants generally forage for flowers in quest of nectar and other sustenance, and in doing so they pollinate the flowers that they encounter. This review identified the pollination networks between ants and plants at the species and family levels. Pollination is often affected by a number of aspects, including the flower’s sex, its ovary position, the inflorescence it bears, and the time of year. The available literature demonstrates that ants visit the inflorescences of the same species only to promote cross-pollination, a process known as “geitonogamy”; however, we conclude that ants may visit different inflorescences of different plants in the field. If ant pollination is the norm, there is less selection pressure to acquire self-compatibility; nonetheless, ants' cross-pollination may have caused ants to co-evolve with the pollinating flowers. This indicates that ants are more than just curious bystanders to some flowers; they act as significant pollinators.
Introduction
Nature’s beauty and grace are sleeping into the seed vessels, which is only possible through the diverse pollination strategies in plants and insects under self and cross-pollination modes (1). A floral visitor’s efficacy can be measured in terms of its contribution to plant health (2–4); similarly, the visitor’s pollination performance is also correlated to its fitness (5). The concept of the co-evolution of flowering plants and their pollinators (6, 7) originated many decades ago (8) on the basis of numerous remarkable interactions between flowers and insects that transfer pollen to floral stigma (9, 10). Flower–insect co-adaptation is an ongoing process in which the partnership is developed by reducing reproductive obstacles through pollen transfer (11). Although entomophily pollinators are diverse (12), ant-mediated pollination is less prevalent than that of other pollinating species (13). It has been reported that ants have a limited pollination ability (13). However, there might be other reasons for the lack of research on the subject; had the scenario been reversed, the probabilities would have differed.
A well-organized mutualistic relationship develops when ants engage with plants to transfer pollen (14). Plants defend against herbivores; sometimes, it may be costly to exclude mutualists, as seen in aggressive bodyguard ants, which repel hovering pollinators (15). As a result, resource allocation might cause trade-offs between plant defense and reproductive success (16). A plant’s interactions with other species may also provide a “context” for defining its costs. For example, foliar herbivory can influence floral traits (17), and natural enemies can exert selection pressure that runs counter to pollinators’ “labor” (18, 19). Plant defense can thus be accompanied by reduced competitive ability or the “deterrence” of mutualists (20). Their structures (21, 22), scents (23), and phenologies (24) aid plants in repelling natural enemies by limiting pollinator access. Bodyguard ants can indirectly defend plants by attacking growing blossoms (25, 26). Such interactions may limit conflicts between bodyguard ants and pollinators (27, 28); therefore, bodyguard ants provide opportunities to explore conflicts between plant defense and reproduction (15).
Since the frequency of ants’ visits to myrmecophilous plants is dependent on the plant’s health (29, 30), angiosperms have evolved different flower arrangements, with brilliant colors, perfumes, and enhanced nectar production. Like many other entomo-pollinators, ants are drawn to angiosperms because of their rich morphological textures and modifications (31). In most instances, ant-mediated pollination is supposed to be a low-energy system; while not using energetically costly resources to attract insect pollinators, plants have evolved specific traits for attracting ants (32). Ants as pollination vectors may be favored in habitats where they are abundant and where local vegetation supports open flowers with ground-level inflorescences (33–35). However, the pollination ability of ants can be hindered by multiple factors, including their tendency to self-groom and their small body size compared with the reproductive structures of the flowers they encounter (36). Furthermore, pollen germination may be inhibited by their metapleural gland secretions (37, 38), which may not favor pollination. Despite ants’ preference for nectar, which facilitates floral visitation, they can sometimes impede pollination by destroying flowers (39), feeding on pollen (40–42), or interfering with or repelling other pollinating vectors (43–46).
Traditional ant–plant network analysis has focused on bipartite grids that primarily address a single node of ecological interaction, such as “ant–flower,” “ant–seed,” or “ant–nectar” (47, 48). However, in ecological communities, ant–plant interactions can be multifaceted. One species of ant may interact with various plants and fulfill multiple roles, such as pollinator, disperser, protector, and neuter (49, 50). Therefore, ant–plant interactions in a particular ecosystem can be incorporated into a multi-network species interaction in a multi-trophic environment, allowing their affiliations to be quantified as a significant model for “biodiversity interaction” (50–52). These networks play a crucial role in determining the “interaction diversity” of many ecosystems, particularly those in the tropics (52–54). Therefore, their prevalence makes them one of the most significant faunas on earth (55). Ants’ diets vary according to species; some are predators, while others feed on seeds, honey, or fungus (56). They occupy most terrestrial environments, and constitute 10%–15% of the total animal biomass. The dominance of angiosperms in most terrestrial ecosystems permits various facultative and obligatory connections between plants and insects. Aside from ants’ floral nectar-gathering behavior, their visiting of extra-floral nectaries forms part of plants’ indirect anti-herbivory defense strategy since ants often attack predatory arthropods while patrolling and thus protect the plants.
Although insect–plant interactions have been extensively researched, the role of ants as pollinators has received remarkably less attention. Ant pollination is an unusual mutualistic interaction (57), yet their pollination links with plants are often defined as reciprocal mutualism. Such pollinating relationships are often regarded as hostile since they are likely to fight or interact with other heterospecific pollinators (53). However, a significant amount of mutual exchange of resources may occur through their pollination skills. The primary goals of this review are to examine the diversity of pollinating ants and recognize their potential hosts where they actively pollinate. Since plant–herbivore networks often demonstrate how insects interact with plants in specialist or generalist capacities, this review concentrates on understanding the potential efficacy of ants’ pollination performance and efficiency. Interacting networks among ants, as pollinators, for a specific or broad range of plant communities have also been investigated.
Materials and methods
Data collection
The current study investigates three key concerns and affinities between pollinating ants and the plant communities they encounter: (a) pollinating ant diversity (species and subfamilies), plant diversity (species and families), and the ant–plant network. (b) the importance of floral features (inflorescence, sexuality, and ovarian style) in pollination, and (c) pollination phenology.
Data acquisition
Data sampling
More than 100 peer-reviewed published relevant studies from the last four decades (1981–2022) were analyzed during the review. We collected data only from studies in which authors reported on “true pollination”; those which contained references to observations of “flower visits” without a depiction of “true pollination” were ignored. There were three main categories of information in the available literature:
1. An explicit mention of ant species to plant species where ant-mediated pollination was documented.
2. An explicit mention of ant-mediated pollination without stating the names of the ant or plant species.
3. An indefinite, probabilistic view of ant pollination on some plants (or ant–plant interactions in general) where both the interacting species names (ant and plant) were either mentioned or absent.
Therefore, we narrowed the data to only the most relevant and clear examples of pollination at the species and genus levels. The data used to create the visual depictions of ant–plant networks and connections were pulled from over 50 articles (Table 1). The bipartite graphs were created with R-Studio software, although additional supporting data were obtained from other scholarly works. In the literature review, studies related to ants of varying subfamilies pollinating plants across all species and families to varying degrees were gathered. The pollinated flowers’ inflorescence, floral sexuality (unisexual or bisexual), and ovarian styles (epigynous, hypogynous, or perigynous) were all documented.

Table 1 Diversity of pollinating ants with respect to pollinated plants, floral characteristics, pollinating season, and study sites.
Data weightage
We noticed that the pollinating activity of certain ant species was reported on frequently, whereas that of other species was discussed only occasionally, and that some species were studied still less frequently and hence mentioned only on rare occasions. We assigned statistical weightage to pollinating ant species according to their frequency in independent publications and rated the ants in order of high to low pollinator. However, ant species described by the same author/author-group in multiple publications (i.e., the same ant species described by the same author in separate literature) were disregarded. Likewise, multiple studies on an ant species in different times by the same author/author-group were also ignored. This was in place to avoid erroneous weightage of occurrence frequency of a particular ant. Therefore, in such cases, the pollinating frequency of that particular ant species, as per the record of the author/author group, was one. If two or more authors documented pollination on the same ant species (regardless of whether the pollinating plant was the same or different), the species weightage was powered by two or more depending on the records of the same species by different authors. Given the lack of reliable data on the frequency with which ants execute pollination, we opted for this approach. Despite the drawbacks of this method, it provides reliable indications as to which ant species are frequent pollinators (i.e., higher occurrence frequency of the same ant species by multiple authors) or rare pollinators (i.e., lower occurrence frequency of the same ant species by different authors) (34).
Data assortment
All documented pollination events were classified according to pollinating ants (species and subfamily), pollinated plants (species and family), floral features (sex, ovary position, and inflorescence), and seasonal prevalence. Myrmecophilous ants have been shown to have clear seasonal preferences, with evidence spanning from summer to spring vegetation. During data analysis, it was observed that pollination events were recorded in a mix of timeframes (month, season, and period) across the study areas (country locations). Hence, we converted the literature-cited timings (month, season, period) into five seasons (summer, rainy season, autumn, winter, and spring) based on the established meteorological season for the countries. For example, if pollination was observed in Brazil from October onwards, the period was converted into the rainy season (32) according to the Brazilian meteorological calendar. Similarly, if pollination was recorded in Nigeria from May onwards, it was converted to the rainy season (84) according to the Nigerian meteorological calendar. Pollination recorded from June onwards in India was also attributed to the rainy season. These conversions were undertaken to obtain a more global picture of pollination seasons to account for the fact that meteorological seasons do not always align with calendar months depending on a country’s geographical hemisphere. All conversions (month to season) were carried out using, where available, data from the respective country’s official meteorological websites. The data sets were entered into Microsoft Excel, compared with reference documents, and analyzed.
Data analysis
All records regarding the pollinating activity of ants on their different plants were analyzed and compared. The arbitrary interaction of each ant species with its pollinated plant(s) was estimated using data acquired at random from various literature studies. Binary data were organized in a Microsoft Excel file based on the arrangement of ant species with pollination plant species. We also investigated the interactions between pollinating plant families and ant sub-families based on our observations, and these data were structured in a similar matrix format. Throughout our analysis, ant morphospecies were treated as distinct species. Two interaction networks (or matrices) were created separately: (a) at the species level and (b) at the higher taxonomic level (family and subfamily). Data obtained from the network analysis were classified into two broad categories (1): plants’ exclusivity to ants—(i) the pollination of a single plant species by multiple ant species, (ii) the pollination of a limited number of plant species by multiple ant species, (iii) the pollination of a single plant species by limited species of ants, and (iv) the pollination of a single plant species by a single ant species—and (2) ants’ exclusivity to plants—(v) the pollination of multiple plant species by a single ant species, (vi) the pollination of a limited number of plant species by a single ant species, and (vii) the pollination of multiple plant species by multiple ant species.
The network data interpretation, construction, and metric analyses were performed in R software (version 4.0.3), utilizing the “Bipartite”, “Vegan”, and “Tiff” packages for graphical representations (90, 91). The nestedness metric based on the overlap and decreasing fill (NODF) metric network-level nestedness analysis (92) was also performed in R software. The NODF values range from 0 (completely non–nested network) to 100 (completely nested network) (92, 93). During calculation, the “H2′-fun” function performed network-level specialization (H2′), which denotes an index of “complementarity specialization” for the complete bipartite network (94). The H2′ range also varied from 0 (no specialization) to 1 (complete specialization) (91, 92). All matrices in the network analysis were non-weighted and followed a binary pattern where “0” indicates “no-pollination” and “1” indicates “pollination”.
The χ2 tests were employed to examine differences in pollinating ant subfamilies, pollinated plant families, flower sex (uni- or bisexual), floral styles (epigynous or hypogynous), and floral inflorescence varieties. The variances were graphically depicted and validated at significance levels of p = 0.05.
Result
The diversity of pollinating ants attending to their encountered plant(s), flower characteristics, and dispersion (Figure 1) (Table 2 Supplementary File 1) was prepared based on the previously published information (Table 1). A total of 70 ant species (Hymenoptera: Formicidae) from four subfamilies (Formicinae, Myrmicinae, Dolichoderinae, and Pseudomyrmecinae) were identified as pollinators of 41 plants from 23 families based on the data compiled from published records. Among the ant subfamilies, Formicinae accounts for the largest share of pollination (species, n = 34; 48.571%) based on the number of species sampled, and it was observed that those in the Myrmicinae (n = 18; 25.714%) and Dolichoderinae (n = 15; 21.428%) subfamilies pollinated with a lower intensity than Formicinae. The role of Pseudomyrmecinae ants in pollination is less established (n = 3; 4.285%). There was a significant variation of pollinating ants across subfamilies (χ2 = 2.794; df = 3; p = 0.000) (Figure 2). Furthermore, it was observed that Formicinae ants pollinated a wide range of flowers (pollinating plant species, n = 31), accounting for about 48.437% of all pollinated plants. Records for Myrmicinae (pollinating plant species, n = 15) and Dolichoderinae (pollinating plant species, n = 15) were equally substantial, indicating their comparable plant-tending behaviors (both pollinated 23.437% of all pollinated plants); however, they varied in terms of the diversity of pollinated flowers. Pseudomyrmecinae ants were observed to pollinate only a few plant species (n = 3; 4.687% of all pollinating plants). There was a highly significant difference in myrmecophilous pollination among the ant subfamilies for different plant species (χ2 = 2.475; df = 3; p = 0.000) (Figure 2). Although only 41 plant species belonging to 23 families were documented to have any myrmecophilous pollination, some plants were pollinated by more than one ant species (Table 1).
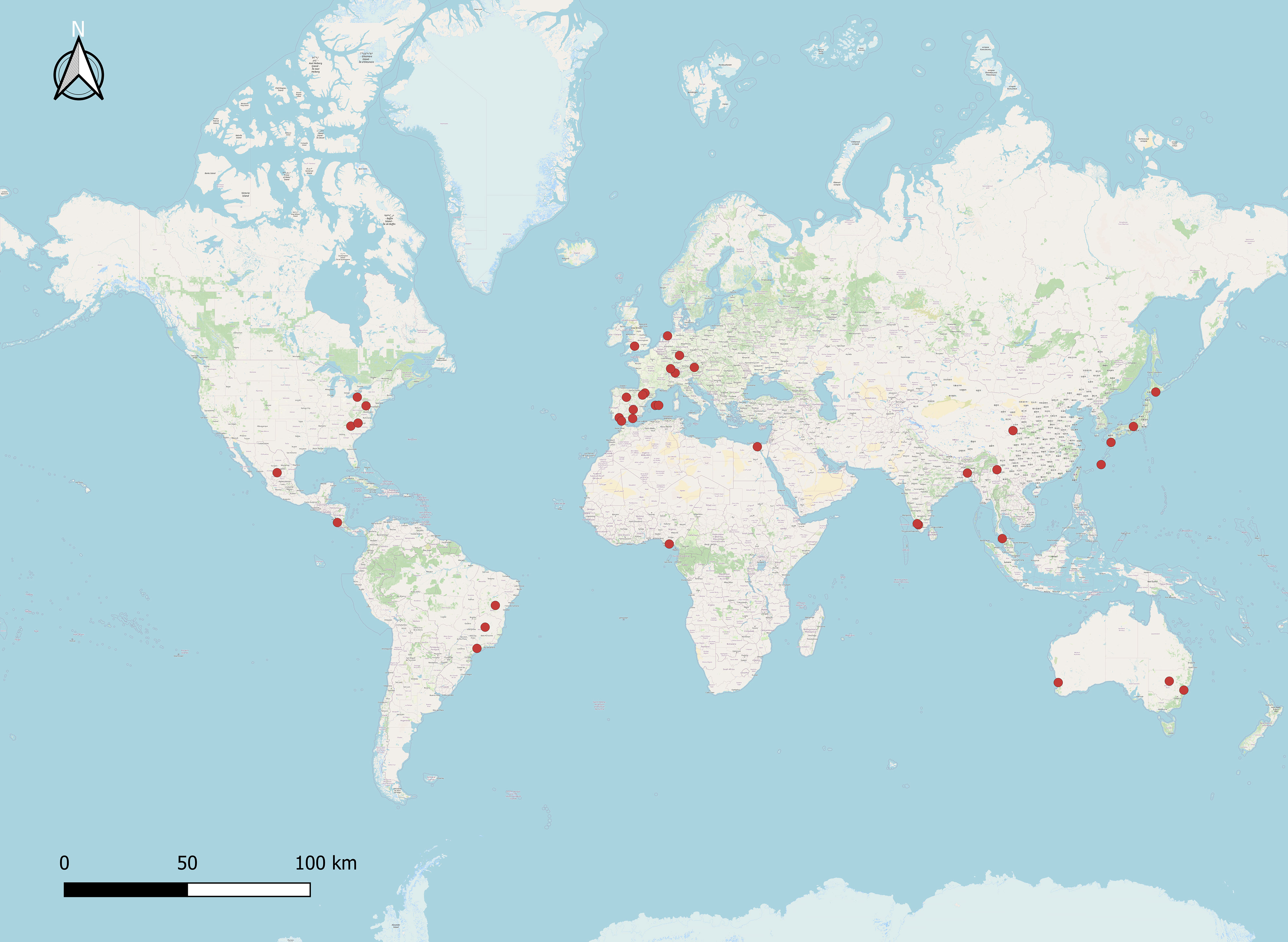
Figure 1 Global records on ant-mediated pollination studies. QGIS-employed ant pollination study sites (red dots).
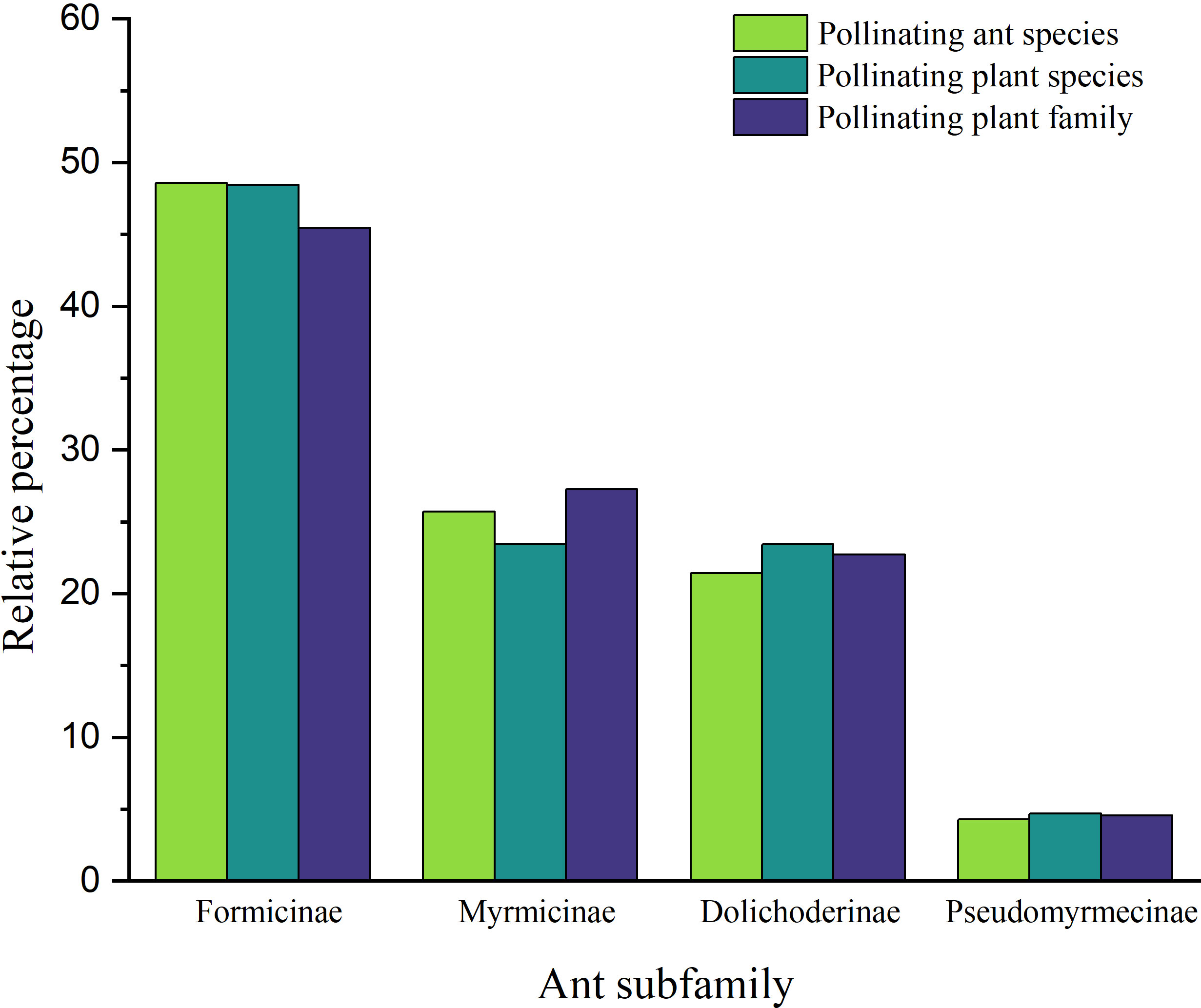
Figure 2 Overall distribution of the relative proportion of pollinating ants (species) and plants (species and family) from the studied ant subfamilies.
When plant families were considered, we found that Formicinae-pollinated plant families were the most common (n = 20; 45.454%), followed by those pollinated by Myrmicinae (n = 12; 27.272%), Dolichoderinae (n = 10; 22.727%), and Pseudomyrmecinae (n = 2; 4.545%). The result shows a statistically significant difference (χ2 = 1.491; df = 3; p = 0.001) (Figure 2) (Table 1). At least five plant families (Blandfordiaceae, Eriocaulaceae, Fabaceae, Liliaceae, and Myrtaceae) were exclusively pollinated by a single (but not the same) species of ant. Records showed that only 9 or 10 ant species have been recorded as pollinating the Apiaceae, Orchidaceae, Cytinaceae, and Euphorbiaceae families. However, only two or three ant species were reported as being possible pollinators of 14 different plant families (Figure 3) (Table 1). A significant difference was observed in the number of ant species with their respective pollinating plant families (χ2 = 5.331; df = 22; p = 0.000). This indicates that plant families are exclusive enough for ants to accomplish pollination. Furthermore, at least seven different plant families (Blandfordiaceae, Brassicaceae, Crassulaceae, Eriocaulaceae, Fabaceae, Liliaceae, and Myrtaceae) were observed to be exclusively pollinated by ants from a single subfamily. At least 11 plant families (Anacardiaceae, Arecaceae, Balanophoraceae, Caryophyllaceae, Celastraceae, Cucurbitaceae, Dioscoreaceae, Frankeniaceae, Orobanchaceae, Proteaceae, and Rosaceae) were observed to be exclusively pollinated by any two subfamilies, whereas at least five plant families (namely Amaranthaceae, Apiaceae, Cytinaceae, Euphorbiaceae, and Orchidaceae) were observed to be pollinated by any three ant subfamilies (Figure 4) (Table 1). However, there was no significant variation among the ant subfamilies that pollinated the plant families (χ2 = 2.434; df=2; p= 0.002). Although the majority of ant-pollinated flowers were hypogynous (n = 24; 58.536%) rather than epigynous (n = 17; 41.463%), no significant difference in results was observed (χ2 = 1.195; df = 1; p = 0.002) (Figure 5A) (Table 1). This indicates that angiosperms with floral parts, such as sepals, petals, and stamens, attached either to the upper part of the ovary (hence less exposed) or the lower part of the ovary (hence highly exposed), do not have any significant difference in pollination. We found that a larger proportion of bisexual flowering plants was pollinated (n = 26; 63.414%) than unisexual flowering plants (n = 15; 36.585%) (χ2 = 2.951; df = 1; p = 0.008) (Figure 5B) (Table 1). This suggests that floral sexuality may have a modest effect on pollination. In terms of inflorescence, racemose (n = 24; 58.536%), cymose (n = 6; 14.634%), and solitary (n = 4; 9.756%) flowers were the most commonly pollinated, and capitulum (n = 3; 7.317%), cyathium (n = 2; 4.878%), and panicle (n = 2; 4.878%) flowers were occasionally pollinated. When pollination success on floral inflorescences was statistically analyzed, significant variations were found (χ2 = 5.339; df = 5; p = 0.000) (Figure 5C). This finding suggests that flowering plant petal configurations significantly influence ant pollination.
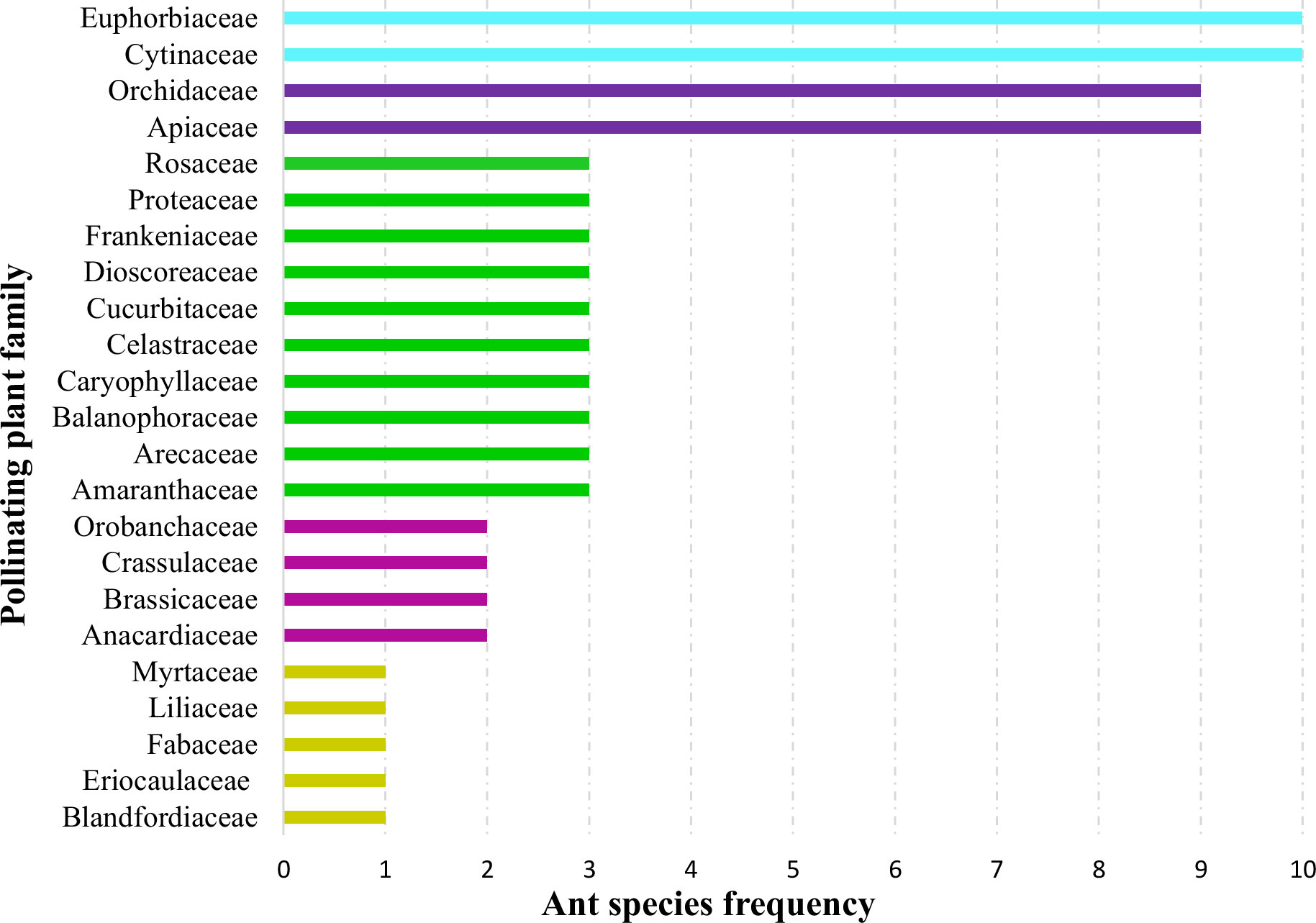
Figure 3 Distribution of pollinating ants to plant families. Each bar represents how many ant species pollinate a specific plant family. The same-color bars represent an equal number of ant species (species may vary) pollinating different plant families.
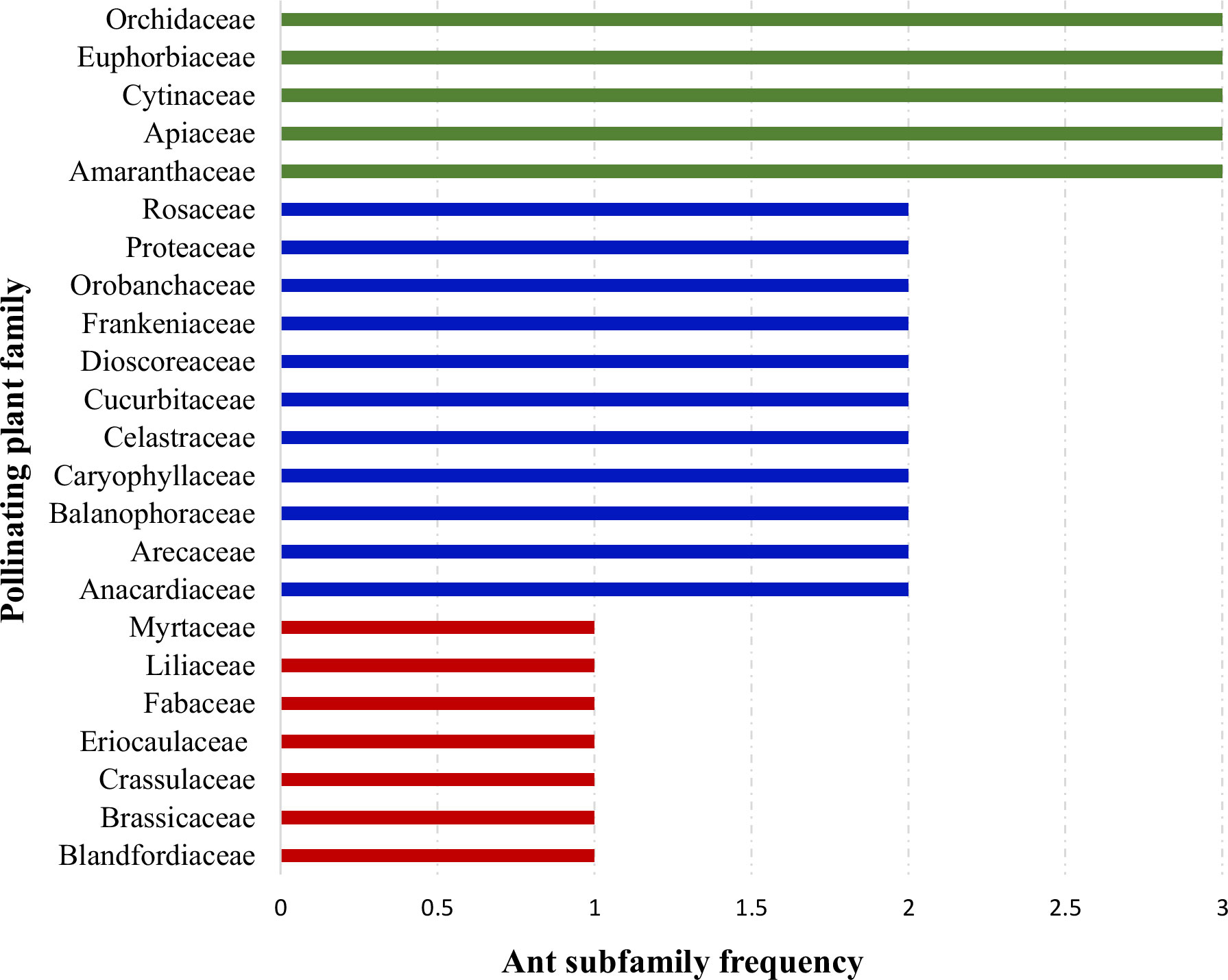
Figure 4 Distribution of pollinating ants to plant families. Each bar represents how many ant subfamilies pollinate a specific plant family. The same-color bars represent the equal number of ant subfamilies pollinating different plant families.
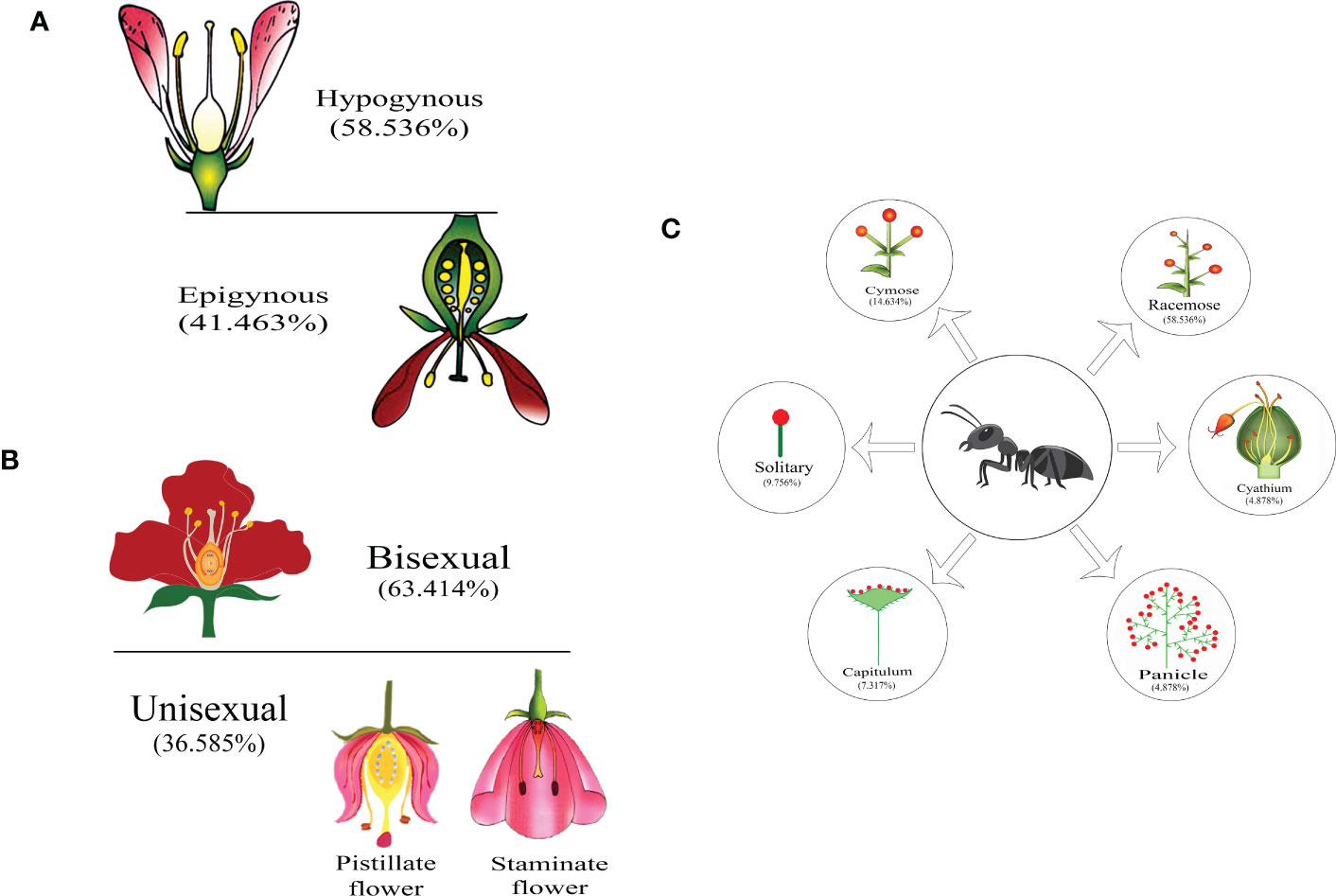
Figure 5 Myrmecophily according to floral characteristics: (A) ant pollination to flower ovary position, (B) ant pollination to flower sex, and (C) ant pollination to flower inflorescence. The magnitude of pollination is displayed in percentages for each case.
Out of the 70 pollinating ants, 56 pollinate to only one plant species, 10 pollinate to no more than two, three pollinate to no more than three, and only one pollinates to no more than four. On the other hand, among all pollinated plant species (n = 41), 19 plants were pollinated by only a single ant species, nine plants were pollinated by a maximum of any two, and nine other plants were pollinated by a maximum of any three. However, three different plant species were shown to be pollinated by four, five, or six distinct ant species. Only one plant was found to have been pollinated by up to 10 different ant species. According to the records, only Formicinae ants pollinated five plant families (Blandfordiaceae, Brassicaceae, Crassulaceae, Eriocaulaceae, and Fabaceae). Myrmicinae and Dolichoderinae were the only pollinators of at least two plant families (Liliaceae and Myrtaceae). According to the available evidence, Formicinae is both a generalist and specialist pollinator. For example, Formicinae with Pseudomyrmecinae may co-pollinate two plant families (Arecaceae and Celastraceae); Formicinae and Dolichoderinae may co-pollinate three plant families (Proteaceae, Caryophyllaceae, and Rosaceae); Formicinae and Myrmicinae may co-pollinate six plant families (Anacardiaceae, Balanophoraceae, Cucurbitaceae, Dioscoreaceae, Frankeniaceae, and Orobanchaceae); and again, Formicinae, along with Myrmicinae, and Dolichoderinae may co-pollinate five plant families (Amaranthaceae, Apiaceae, Cytinaceae, Euphorbiaceae, and Orchidaceae). The results showed that Formicinae ants were responsible for pollinating the most plant families (n = 20), followed by Myrmicinae (n = 12), Dolichoderinae (n = 10), and Pseudomyrmecinae (n = 2).
The pollinating ant–plant bipartite networks are shown in Figures 6, 7. The analysis of the bipartite interaction network between pollinating ants (upper orange nodes) and their various pollinating plants (lower green nodes) made it clear that the networks were significantly less nested (NODF = 1.797). It suggests that the network is unstable enough to account for the likelihood of substantial plant-to-ant linkages. Furthermore, the extent of specialization in this bipartite network was more significant than the average (H2′ = 0.898), indicating the existence of a specialized network between pollinating ants and the plant species they visited (Figure 6) (Table 2, Supplementary File 2). On the other hand, the bipartite network between distinct pollinating ant subfamilies (upper orange nodes) and pollinating plant families (lower green nodes) was found to be significantly nested (NODF = 55.682). This implies that the network comprises several generalist pollinating ant subfamilies interacting with different plant families. The degree of specialization (H2′ = 0.394) in such a bipartite network demonstrated the robustness of pollination interaction (Figure 7) (Table 2, Supplementary File 3).
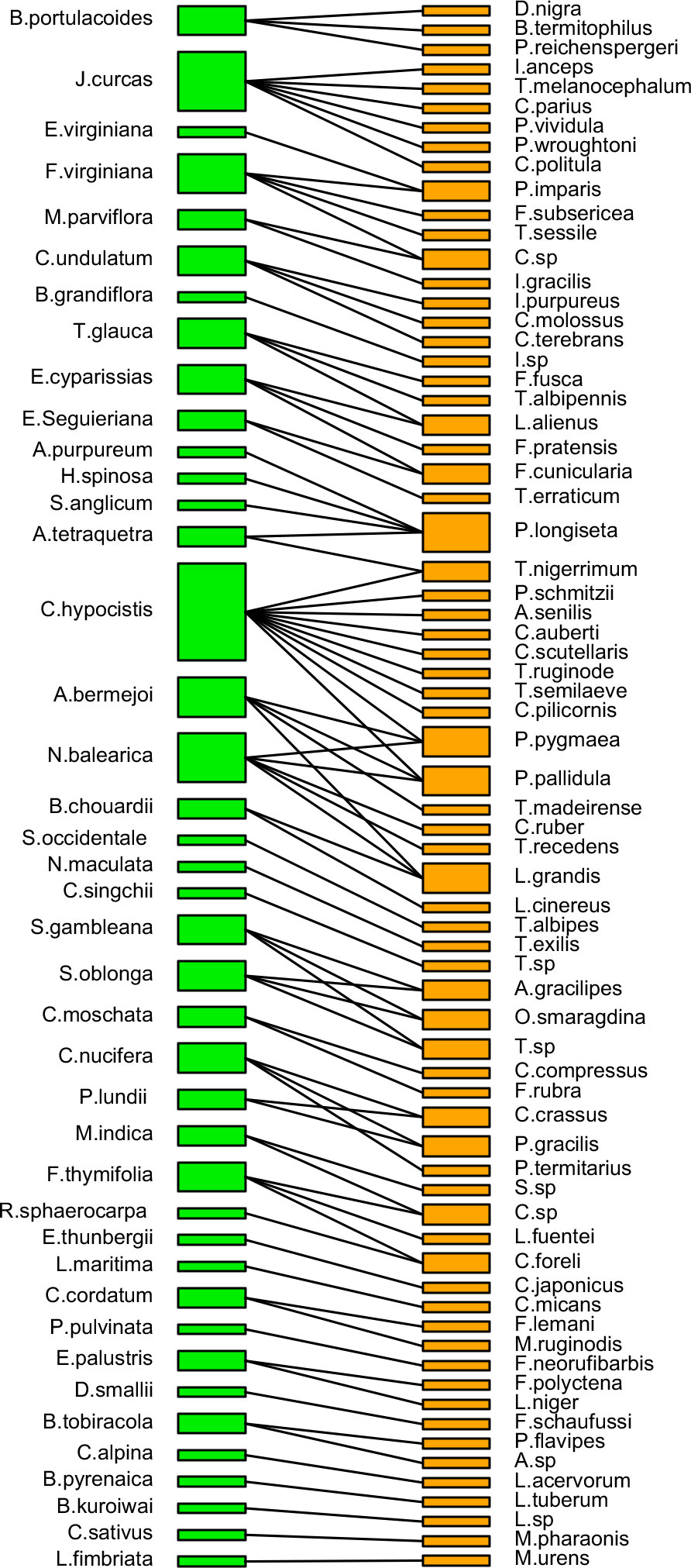
Figure 6 A bipartite network connecting pollinating ant species (upper orange nodes) and pollinated plant species (lower green nodes). The widths of the nodes represent the marginal total of pollinations made by a pollinator species or received by a plant species. There are 70 distinct ants and present 41 different plant species, displaying an interaction network based on the likelihood of pollination between the groups, which is indicated by the black line linking single or branched lines between two nodes. Thick upper nodes indicate the presence of the ant species with the highest pollination activity, whereas thin nodes indicate the presence of the ant species with the lowest (or least) pollination activity. Thick lower nodes indicate the presence of the most-pollinated plant species, whereas thin nodes indicate the presence of least-pollinated plant species.
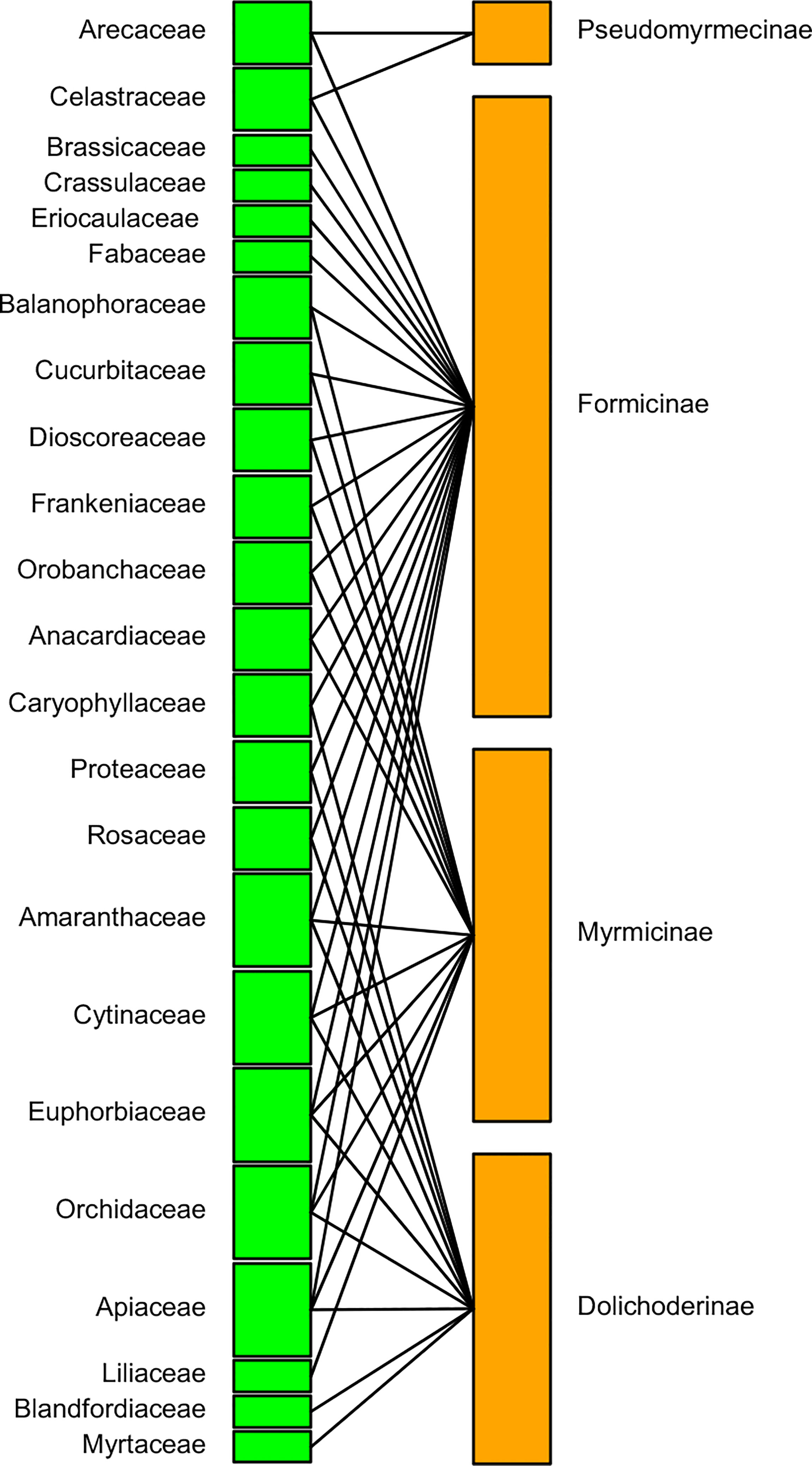
Figure 7 A bipartite network connecting pollinating ant subfamilies (upper orange nodes) to pollinated plant families (lower green nodes). The node widths show the marginal total of pollinations made by pollinator ant subfamilies or received by plant families. There are four ant subfamilies and 23 plant families, with an interaction network depending on the likelihood of pollination between the groups, which is denoted by a single or branched black line connecting two nodes. Thick upper nodes indicate the presence of the most ant subfamilies, whereas thin nodes indicate the presence of the fewer ant subfamilies. Thick lower nodes indicate the presence of most-pollinated plant families, whereas thin nodes indicate the presence of least-pollinated plant families.
According to the studied publications, pollination frequency on a single plant species or multiple plant species indicates that the endemic polygynous ant Proformica longiseta, which lives in the high mountains of Europe, is the most frequent ant-pollinator. Their pollination ability was most documented (on 14 occasions). Likewise, 11 publications have confirmed that Camponotus crassus, which lives in bromeliads, bamboo, and the cocoa plantations of The Cerrado, South America, is the second most frequent pollinator. At least seven different species of ants were reported to pollinate by only a single publication (Figure 8) (Table 2, Supplementary File 4). Figure 8 summarizes the number of publications reporting sightings of individual ant species engaged in pollination (numbers appear after ant names). Ant pollination performance varied over the year, which may be related to the success of their pollen dispersal efforts. According to our data, ants pollinated more frequently in the summer (case studies, n = 43; 30.935%) and spring (n = 38; 27.338%) than in the winter (n = 29; 20.863%) and fall (n = 18; 12.949%), but less frequently in the rainy season (n = 11; 7.913%). However, there was a statistically significant difference in ant pollination across seasons (χ2 = 2.571; df = 4; p = 0.000) (Figure 9) (Table 2, Supplementary File 5).
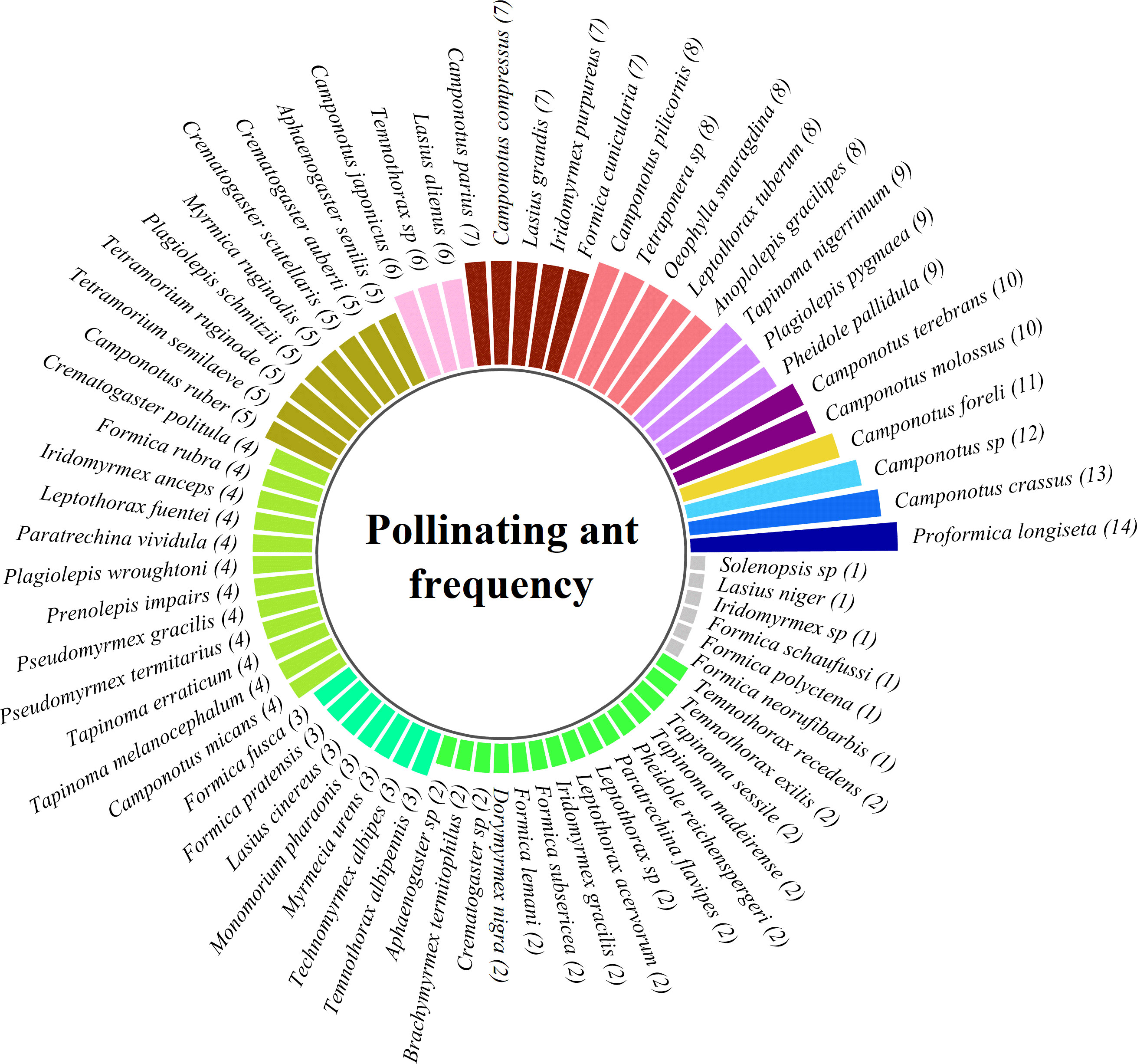
Figure 8 The pollinating ant frequency according to available records. The number after an ant species reflects how many times the species was studied in different publications by different authors. For example, “Proformica longiseta (14)” means that 14 different publications (by different authors/author groups; multiple studies on an ant species in different times by the same author/author group were ignored) recorded Proformica longiseta pollinating plants (same or different plant species).
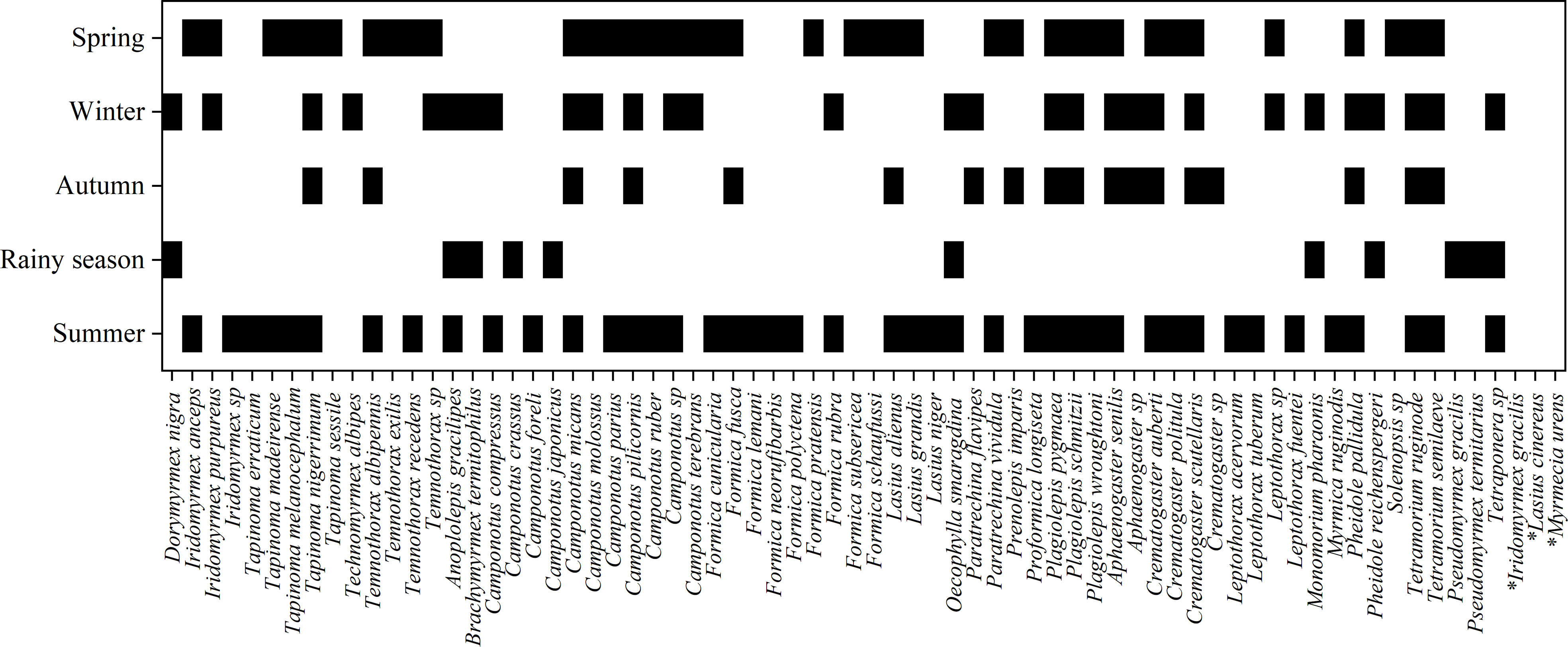
Figure 9 Ant pollination according to the seasons. The heat map illustrates the pollinating ant(s) with their pollination season(s). The black bars indicate pollination; the white bars indicate no pollination. Seasonal indications are missing in literature records for the final three species (marked with asterisks).
Discussion
The current review delves into the intricacies of ant pollination and explores data on how ants from different subfamilies perform this service for plants. Our findings reveal that when species-specific links are included, the pollination interaction network between ants and plants is less stable than when only the specificity of interactions between ant subfamilies and plant families is considered. Therefore, more generalized networks were observed at higher taxonomic levels (i.e., subfamilies or above), and more specialized networks emerged at lower taxonomic levels (i.e., species). Network ecology, in terms of stability and specialization among the involved species, has most likely been optimized in ant-to-plant pollination events over time, underscoring the vital relevance of co-evolution among the participants. It has been demonstrated that myrmecophilous ant–plant associations influence the dynamic nature of network structural integrity across various arthropod groups (95, 96). Herbivorous insects have highly specialized systems because they feed on specific plant species (97), but predatory arthropods can alternate between insect prey and floral rewards (98). Arthropods typically have a very stable and robust interaction matrix as a result of their extensive resource utilization (i.e., extensively layered network design) (99); however, in agricultural contexts, plant–pollinator networks are frequently considered as being specialized rather than nested (100). The Formicinae subfamily has the most species diversity, presumably allowing Formicinae ants to pollinate one or several plants and successfully boost pollination probability. They also exhibit higher levels of behavioral efficiency than most other groups because of their powerful mouthparts and flexible bodies, although this efficiency is not always independent of environmental stability. They are inundated with nectar during peak flowering periods, allowing them to pollinate a wide variety of flowering plants (48, 101). The presence of nectar increases the frequency of ant visits to flowers, thereby increasing the chances of pollination (101, 102). Moreover, Formicinae ants often visit the reproductive structures of foliages in quest of floral and extra-floral nectars, resulting in increased pollination. Pollination is facilitated by the visitor’s foraging skill and frequency (38, 42, 54, 101). Ants with superior foraging abilities are likely to regularly travel extensively on numerous panicles, flower sepals, and petals. For example, Camponotus ants visit floral structures more frequently than others, allowing them to pollinate more successfully (34). Thus, both shorter and more extended foraging periods may increase pollination, although longer foraging intervals may result in more pollen removal and retention on stigmas (63, 103), increasing the possibility of cross-pollination and seed germination (65). The morphology of the pollinating species, in addition to the plant parts, is essential to and promotes pollination. Ants, particularly those belonging to the genus Camponotus, have thick, hairy bodies that facilitate the passage of pollen; hence, plants may rely on ants as pollen transfer vectors, a process known as geitonogamy. Camponotus terebrans are efficient pollinators as they have physical characteristics that allow them to pollinate various flowers (57). Even when the North American winter ants Prenolepis impairs and Crematogaster sp. are present in the same flower, P. impairs is capable of pollinating plants more effectively due to its morphological advantages and integumental architectures (35, 72, 77). The Mediterranean ant Plagiolepis pygmaea is capable of adhering the anthers to its body, carrying different amounts of pollen by adhering pollen grains to its head, thorax, and gaster (35). Floral structures, particularly the openness of reproductive organs exhibited in chasmogamous flowers, greatly assist pollination (57). Ant-mediated cross-pollination occurs regularly in the chasmogamous flowers of two American beech species, Fagus grandifolia and Epifagus virginiana, because of their favorable floral features, such as their open and exposed anthers and stigmas (77).
According to myrmecophilous pollination statistics, ants from the Myrmicinae and Dolichoderinae subfamilies have less species diversity than the Formicinae subfamilies but more than the Pseudomyrmecinae subfamilies (63). As a result, Myrmicinae and Dolichoderinae were identified as the second and third most common pollinating ant subfamilies after Formicinae. Nonetheless, only a few Pseudomyrmecinae ant species have been recorded doing this job. Most ant species were found to be “generalist” pollinators, meaning that they pollinated a wide range of plants from various families, and only a few ant species exhibited “specialist” behavior and pollinated only a subset of flowering plants. Even if these results are skewed due to the content of the published sources we analyzed, Formicinae is projected to have a higher pollination frequency than others due to its greater abundance and vast distribution. Their superior pollination capacity indicates they can thrive in a broader range of environmental constraints than other subfamilies.
In contrast to Formicinae, Myrmicinae and Dolichoderinae ants interacted with a limited number of plants, indicating that they have specialized pollination hosts. Pseudomyrmecinae ants interact with plants in a more specialized manner, exhibiting the group’s more specialized pollination behavior (58, 60). Although inferring a linear relationship between an ant’s specialist or generalist behavior and the number of hosts encountered may be erroneous, we can reject the null hypothesis by assuming that, due to “positive complementarity” (104, 105), the chance of pollination is decreased when an ant visits only specific plants. Nonetheless, it appears that Myrmicinae, Dolichoderinae, and Pseudomyrmecinae ants have received less attention, resulting in fewer findings. Their poor ranking could be attributed to the fact that they might pollinate infrequently or ineffectively in the wild. Pollination deficiency in Myrmicinae and Dolichoderinae ants can be explained by their limited abundance in agricultural fields and woodlands, as the majority of the species appear to live in deserts and dunes. As a result of inefficient behavioral labor, Myrmicinae and Dolichoderinae are poor pollinators. Ants that visit plants for food are more apparent on vegetation, and are therefore capable of more effective pollination than ants that receive food through predation. Predatory Pseudomyrmecinae are rare pollinators because they prefer animal meat to plant-derived foods. They have been reported as more inclined to engage in hostile interactions with others to obtain sustenance through predation rather than gathering nectar, thereby reducing the likelihood of pollination.
Our investigation reveals that pollination success in plants varies across plant families. This could be due to beneficial plant features such as physical (color, odor, and texture) or chemical (foliar and extra-foliar nectar) cues that encourage the pollinators (69, 106–108). When ants consume nectar from flowers, they pollinate the plants without intending to do so because food resources, such as nectar, are natural ant attractants. According to our findings, ants frequently pollinate plants from four families (Euphorbiaceae, Cytinaceae, Orchidaceae, and Apiaceae) due to their appealing flower structure, color, appearance, and higher nectar production (35, 63, 66, 109). For example, ants are naturally drawn to the vibrant colors of Orchidaceae blooms (110, 111). Euphorbiaceae flowers are effectively pollinated due to their unusually elongated shapes and overall floral composition (67). The pollination interactions between ants and plants can be considered to involve negative trade-offs between species. However, such interactions are well-documented as exercises in mutualistic cooperation.
We evidenced that ants pollinate hypogynous flowers more than epigynous flowers (63). Since the ovary position is superior in hypogynous flowers, pollen rapidly clings to it, and the pollen easily moves from the stigma to the ovary via the stamen, resulting in pollination. As the ovary position is inferior in epigynous flowers, pollen transfer from the stigma to the ovary is primarily influenced by the transit distance, which is significantly shorter in hypogynous than in epigynous flowers. As a result, the ovary position of the flower is critical for insect pollination. As pollen has to travel further to reach an ovary with an inferior position, ants are assumed to be more likely to pollinate flowers with a superior ovary position. Therefore, they are assumed to have co-evolved more closely with hypogynous than epigynous flowers.
Flower sex also influences myrmecophilous pollination. According to our data, bisexual blooms exceed unisexual flowers in pollination success. As bisexual flowers have both male and female sexual organs, when ants visit these flowers they inadvertently move the distinct sexual parts of the blossoms, resulting in pollination. However, ants’ responses to male and female unisexual blooms are different. Pollinator visits to a unisexual flower do not ensure fertilization due to the separation of male and female reproductive organs. As a result, the presence of ants at unisexual flowering plants (facultative association) leads to a low rate of successful pollination (112, 113) compared with the rate for bisexual flowering plants (114).
According to our findings, one ant species may be able to pollinate as many as seven distinct types of flowering plants. The most effective pollination occurs in racemose flowers, where the blooms continuously develop along the rachis, and by traveling along the blossoms ants can pollinate efficiently. In addition to racemose, cymose inflorescences were also found to be highly pollinated by ants. Successful pollination depends on anther dehiscence (anther splitting). For instance, the longitudinal dehiscence seen in members of the Brassicaceae family allows the androecium to be fully or partially exposed and dispersed among the flowers. Solitary flowers, which have a single flower unit in the central axis, are visited by ants in the same way that racemose flowers are; however, the pollination rate is lower in solitary than in racemose flowers due to the singularity of the flowering unit. Flowers from cymose, cyathium, panicle, and capitulum inflorescences are pollinated by ants to a significantly lesser degree, likely due to their “defined” idiosyncratic flowering architectures. It has been recorded that some flower-visiting ants roam along the margins or borders of the petals but do not pollinate due to unappealing floral characteristics, including nectar (60, 114); however, if other floral features are present, ants pollinate quite efficiently (63, 65). Thus, floral morphology, particularly the number of flower components and flower structure, and animal morphology, especially ants’ exoskeletal armature, influence pollination success (115, 116, 117).
Although ant pollination occurs throughout the year, it is most widespread in the spring and summer due to ants’ increased foraging activity during the warmer months (67, 69, 70). They are frequently active in warmer regions in search of food and are therefore attracted to various flowers for pollen and nectar, ultimately pollinating them. For example, ants are highly active pollinators in Southeast Asia during the summer and spring when mango flowers extensively (65), but in the United States they are highly active during the fall, when Epifagus virginiana blooms profusely (77). However, they typically stop pollinating when it rains (36).
Conclusion
There is often a high degree of specificity in the interactions between ants and plants during pollination, although floral patterns and seasons can lead to variability in this process. In contrast to the general mutualistic networks of pollinating ants and plants, antagonistic networks among members show partner-specific interactions. The dynamics of network evolution differ between mutualistic and antagonistic communities; partner-switching is more critical to the ebb and flow of interactions in the former than in the latter, but species turnover is far more critical in the latter. However, antagonistic and mutualistic networks underwent significant ecological and topological changes, with varying consequences for pollinator and herbivore populations in agricultural settings. This review supports the view that pollinators are more agile in their engagement choices than herbivores, which are more reciprocally specialized and fix unstable affiliations. Our findings demonstrate that predictive analyses of the overall plant–ant bipartite network in a given agro-environment are crucial for guiding diversity and analyzing its various ecological benefits. More studies on the subject across diverse agroecological systems will provide insight into the optimal complexity of habitats in degraded or intensified agricultural landscapes. It will provide new opportunities for identifying diverse ants, which may have a definitive role in pollination.
The cognitive processes behind ants’ pollination instincts are obscure. Despite their high intelligence, it is reasonable to consider the possibility that ants pollinate flowers both on purpose and by coincidence while gathering food. Hence, it is essential that an ethological inquiry is undertaken to reveal the cognitive processes employed by ants as they carry out pollination tasks.
Author contributions
SD and AD conceived and designed the manuscript. SD collected and computed the data, analyzed the results, and graphically presented them. SD was primarily responsible for drafting the manuscript, and AD later revised and corrected it. Both authors finally discussed and verified the results and statistics. All authors contributed to the article and approved the submitted version.
Acknowledgments
We acknowledge the Head of the Department of Zoology, University of Calcutta, Kolkata, West Bengal, India, for the necessary laboratory facilities to execute this work. The authors are thankful to Supratim Laha, Avishek Dolai, and Krishnendu Mukherjee for the data arrangement and analysis.
Conflict of interest
The authors declare that the research was conducted in the absence of any commercial or financial relationships that could be construed as a potential conflict of interest.
Publisher’s note
All claims expressed in this article are solely those of the authors and do not necessarily represent those of their affiliated organizations, or those of the publisher, the editors and the reviewers. Any product that may be evaluated in this article, or claim that may be made by its manufacturer, is not guaranteed or endorsed by the publisher.
Supplementary material
The Supplementary Material for this article can be found online at: https://www.frontiersin.org/articles/10.3389/finsc.2023.1145761/full#supplementary-material
References
1. Howard SR, Prendergast K, Symonds MR, Shrestha M, Dyer AG. Spontaneous choices for insect-pollinated flower shapes by wild non-eusocial halictid bees. J Exp Biol (2021) 224:1–9. doi: 10.1242/jeb.242457
2. Kearns CA, Inouye DW. Techniques for pollination biologists. USA: University press of Colorado (1993).
3. Fishbein M, Venable DL. Diversity and temporal change in the effective pollinators of. Asclepias tuberosa. Ecol (1996) 77:1061–73. doi: 10.2307/2265576
4. Creux NM, Brown EA, Garner AG, Saeed S, Scher CL, Holalu SV, et al. Flower orientation influences floral temperature, pollinator visits and plant fitness. New Phytol (2021) 232:868–79. doi: 10.1111/nph.17627
5. Jacobsen DJ, Raguso RA. Lingering effects of herbivory and plant defenses on pollinators. Curr Biol (2018) 28:1164–69. doi: 10.1016/j.cub.2018.08.010
6. Hu S, Dilcher DL, Jarzen DM, Winship Taylor D. Early steps of angiosperm–pollinator co-evolution. Proc Natl Acad Sci (2008) 105:240–45. doi: 10.1073/pnas.0707989105
7. Shimizu A, Dohzono I, Nakaji M, Roff DA, Miller DG III, Osato S, et al. Fine-tuned bee-flower coevolutionary state hidden within multiple pollination interactions. Sci Rep (2014) 4:1–9. doi: 10.1038/srep03988
8. Beach JH. Pollinator foraging and the evolution of dioecy. Am Nat (1981) 118:572–77. doi: 10.1086/283851
9. Wiebes JT. Co-Evolution of figs and their insect pollinators. Annu Rev Ecol systematics (1979) 10:1–12. doi: 10.1146/annurev.es.10.110179.000245
10. Asar Y, Ho SY, Sauquet H. Early diversifications of angiosperms and their insect pollinators: were they unlinked? Trends Plant Sci (2022) 27:858–69. doi: 10.1016/j.tplants.2022.04.004
11. Macior LW. Co-Evolution of plants and animals-systematic insights from plant-insect interactions. Taxon (1971) 20:17–28. doi: 10.2307/1218530
13. Beattie AJ, Turnbull C, Knox RB, Williams EG. Ant inhibition of pollen function: a possible reason why ant pollination is rare. Am J Bot (1984) 71:421–46. doi: 10.1002/j.1537-2197.1984tb12527.x
14. Kawakita A. Evolution of obligate pollination mutualism in the tribe phyllantheae (Phyllanthaceae). Plant Species Biol (2010) 25:3–19. doi: 10.1111/j.1442-1984.2009.00266.x
15. Ness JH. A mutualism's indirect costs: the most aggressive plant bodyguards also deter pollinators. Oikos (2006) 113:506–14. doi: 10.1111/j.2006.0030-1299.14143.x
16. Strauss SY, Agrawal AA. The ecology and evolution of plant tolerance to herbivory. Trends Ecol Evol (1999) 14:179–85. doi: 10.1016/S0169-5347(98)01576-6
17. Mothershead K, Marquis RJ. Fitness impacts of herbivory through indirect effect on plant–pollinator interactions in Oenothera macrocarpa. Ecology (2000) 81:30–40. doi: 10.2307/177131
18. Gómez JM. Herbivory reduces the strength of pollinator-mediated selection in the mediterranean herb Erysimum mediohispanicum: consequences for plant specialization. Am Nat (2003) 162:242–56. doi: 10.1086/376574
19. Cariveau D, Irwin RE, Brody AK, Garcia-Mayeya LS, Von Der Ohe A. Direct and indirect effects of pollinators and seed predators to selection on plant and floral traits. Oikos (2004) 104:15–26. doi: 10.1111/j.0030-1299.2004.12641.x
20. Strauss SY, Rudgers JA, Lau JA, Irwin RE. Direct and ecological costs of resistance to herbivory. Trends Ecol Evol (2002) 17:278–85. doi: 10.1016/S0169-5347(02)02483-7
21. Galen C. Why do flowers vary? the functional ecology of variation in flower size and form within natural plant populations. Bioscience (1999) 49:631–40. doi: 10.2307/1313439
22. Agrawal AA. Overcompensation of plants in response to herbivory and the by-product benefits of mutualism. Trends Plant Sci (2000) 5:309–13. doi: 10.1016/S1360-1385(00)01679-4
23. Galen C. The effects of nectar thieving ants on seedset in floral scent morphs of Polemonium viscosum. Oikos (1983) 41:245–49. doi: 10.2307/3544271
24. Brody AK. Effects of pollinators, herbivores, and seed predators on flowering phenology. Ecology (1997) 78:1624–31. doi: 10.1890/0012-9658(1997)078[1624:EOPHAS]2.0.CO;2
25. Yu DW, Pierce NE. A castration parasite of an ant–plant mutualism. proceedings of the royal society of London. Ser B: Biol Sci (1998) 265:375–82.
26. Stanton ML, Palmer TM, Young TP, Evans A, Turner ML. Sterilization and canopy modification of a swollen thorn acacia tree by a plant-ant. Nature (1999) 401:578–81. doi: 10.1038/44119
27. Willmer P, Stone G. How aggressive ant-guards assist seed-set in acacia flowers. Nature (1997) 388:165–67. doi: 10.1038/40610
28. Raine NE, Willmer P, Stone GN. Spatial structuring and floral avoidance behavior prevent ant–pollinator conflict in a Mexican ant-acacia. Ecology (2002) 83:3086–96.
29. Waser NM, Chittka L, Price MV, Williams NM, Ollerton J. Generalization in pollination systems, and why it matters. Ecology (1996) 77:1043–60. doi: 10.2307/2265575
30. Traveset A, Sáez E. Pollination of Euphorbia dendroides by lizards and insects: spatio-temporal variation in patterns of flower visitation. Oecologia (1997) 111:241–48. doi: 10.1007/PL00008816
31. Proctor M, Yeo P, Lack A. The natural history of pollination. Harper Collins Publishers. (1996).
32. Ibarra-Isassi J, Sendoya SF. Ants as floral visitors of Blutaparon portulacoides (A. St-hil.) mears (Amaranthaceae): an ant pollination system in the Atlantic rainforest. Arthropod-Plant Interact (2016) 10:221–27. doi: 10.1007/s11829-016-9429-9
33. Hickman JC. Pollination by ants: a low-energy system. Science (1974) 184:1290–92. doi: 10.1126/science.184.4143.1290
34. Gómez JM, Zamora R, Hódar JA, García D. Experimental study of pollination by ants in mediterranean high mountain and arid habitats. Oecologia (1996) 105:236–42. doi: 10.1007/BF00328552
35. de Vega C, Arista M, Ortiz PL, Herrera CM, Talavera S. The ant-pollination system of Cytinus hypocistis (Cytinaceae), a mediterranean root holoparasite. Ann Bot (2009) 103:1065–75. doi: 10.1093/aob/mcp049
36. Del-Claro K, Rodriguez-Morales D, Calixto ES, Martins AS, Torezan-Silingardi HM. Ant pollination of Paepalanthus lundii (Eriocaulaceae) in Brazilian savanna. Ann Bot (2019) 123:1159–65. doi: 10.1093/aob/mcz021
37. Beattie AJ, Turnbull C, Hough T, Jobson S, Knox RB. The vulnerability of pollen and fungal spores to ant secretions: evidence and some evolutionary implications. Am J Bot (1985) 72:606–14. doi: 10.1002/j.1537-2197.1985.tb08315.x
38. Dutton EM, Frederickson ME. Why ant pollination is rare: new evidence and implications of the antibiotic hypothesis. Arthropod-Plant Interact (2012) 6:561–69. doi: 10.1007/s11829-012-9201-8
39. Martins C, Oliveira R, Aguiar L, Antonini Y. Pollination biology of the endangered columnar cactus Cipocereus crassisepalus: a case of close relationship between plant and pollinator. Acta Botanica Brasilica (2020) 34:177–84. doi: 10.1590/0102-33062019abb0219
40. Urbani CB, De Andrade M. Pollen eating, storing, and spitting by ants. Naturwissenschaften (1997) 84:256–58. doi: 10.1007/s11829-012-9201-8
41. Byk J, Del-Claro K. Nectar-and pollen-gathering Cephalotes ants provide no protection against herbivory: a new manipulative experiment to test ant protective capabilities. Acta Ethologica (2010) 13:33–8. doi: 10.1007/s10211-010-0071-8
42. Czechowski W, Markó B, Erős K, Csata E. Pollenivory in ants (Hymenoptera: formicidae) seems to be much more common than it was thought. Annales Zoologici (2011) 61:519–25. doi: 10.3161/000345411X603364
43. Cembrowski AR, Tan MG, Thomson JD, Frederickson ME. Ants and ant scent reduce bumblebee pollination of artificial flowers. Am Nat (2014) 183:133–39. doi: 10.1086/674101
44. Sinu PA, Sibisha V, Reshmi N, Reshmi K, Jasna T, Aswathi K, et al. Invasive ant (Anoplolepis gracilipes) disrupts pollination in pumpkin. Biol invasions (2017) 19:2599–607. doi: 10.1007/s10530-017-1470-9
45. Villamil N, Boege K, Stone GN. Ant-pollinator conflict results in pollinator deterrence but no nectar trade-offs. Front Plant Sci (2018) 9:2018.01093. doi: 10.3389/fpls.2018.01093
46. Unni AP, Mir SH, Rajesh T, Ballullaya UP, Jose T, Sinu PA. Native and invasive ants affect floral visits of pollinating honey bees in pumpkin flowers (Cucurbita maxima). Sci Rep (2021) 11:1–7. doi: 10.1038/s41598-021-83902-w
47. Gomez JM, Munoz-Pajares AJ, Abdelaziz M, Lorite J, Perfectti F. Evolution of pollination niches and floral divergence in the generalist plant Erysimum mediohispanicum. Ann Bot (2014) 113:237–49. doi: 10.1093/aob/mct186
48. Brimacombe C, Bodner K, Michalska-Smith M, Gravel D, Fortin MJ. No strong evidence that modularity, specialization or nestedness are linked to seasonal climatic variability in bipartite networks. Global Ecol Biogeography (2022) 31:2510–23. doi: 10.1111/geb.13593
49. Linhart Y. Plant pollination and dispersal. Ecol Environment Plant Sci (2014) 8:89–117. doi: 10.1007/978-1-4614-7501-9_21
50. Del-Claro K, Lange D, Torezan-Silingardi HM, Anjos DV, Calixto ES, Dáttilo W, et al. The complex ant–plant relationship within tropical ecological networks. Ecol Networks tropics (2018), 59–71. doi: 10.1007/978-3-319-68228-0_5
51. Thompson JN. Evaluating the dynamics of co-evolution among geographically structured populations. Ecology (1997) 78:1619–23. doi: 10.1890/0012-9658(1997)078[1619:ETDOCA]2.0.CO;2
52. Del-Claro K, Rico-Gray V, Torezan-Silingardi HM, Alves-Silva E, Fagundes R, Lange D, et al. Loss and gains in ant–plant interactions mediated by extra-floral nectar: fidelity, cheats, and lies. Insectes Sociaux (2016) 63:207–21. doi: 10.1007/s00040-016-0466-2
53. Rico-Gray V, Oliveira PS. The ecology and evolution of ant– plant interactions. The University of Chicago press (2007).
54. Lange D, Del-Claro K. Ant-plant interaction in a tropical savanna: may the network structure vary over time and influence on the outcomes of associations? PloS One (2014) 9:1–10. doi: 10.1371/journal.pone.0105574
55. Beattie A, Hughes L. Ant–plant interactions. Plant–animal interactions: an evolutionary approach (2002), 211–35.
57. Delnevo N, Van Etten EJ, Clemente N, Fogu L, Pavarani E, Byrne M, et al. Pollen adaptation to ant pollination: a case study from the proteaceae. Ann Bot (2020) 126:377–86. doi: 10.1016/j.biocon.2020.108824
58. Krishnasree PS, Jose PA, Subin K, Sarath TV. Reproductive biology of two threatened and highly traded medicinal plants, Salacia gambleana and Salacia oblonga, from the Western ghats of India. J Threatened Taxa (2022) 14:20859–65. doi: 10.11609/jott.6381.14.4.20859-20865
59. Nancy NP, Amin MR, Miah MRU, Miah MG. Native insect pollinators and their effect on sweet gourd production. Ann Bangladesh Agric (2019) 23:63–8. doi: 10.3329/aba.v23i1.51474
60. Souza da Conceição E, Moura JIL, de Oliveira Costa-Neto A, Delabie JHC. Choice of food resource by bees and ants (Hymenoptera: apoidea, formicidae) on coconut tree inflorescences (Cocos nucifera, arecaceae). Annales la Société entomologique France (2014) 50:6–12. doi: 10.1080/00379271.2014.933015. Taylor & Francis.
61. Sugiura N, Miyazaki S, Nagaishi S. A supplementary contribution of ants in the pollination of an orchid, Epipactis thunbergii, usually pollinated by hover flies. Plant Systematics Evol (2006) 258:17–26. doi: 10.1007/s00606-005-0391-8
62. Gómez JM. Effectiveness of ants as pollinators of Lobularia maritima: effects on main sequential fitness components of the host plant. Oecologia (2000) 122:90–7. doi: 10.1007/PL00008840
63. Luo CW, Li K, Chen XM, Huang ZY. Ants contribute significantly to the pollination of a biodiesel plant. Jatropha curcas. Environ Entomology (2012) 41:1163–68. doi: 10.1603/EN12042
64. Cursach J, Rita J. Implications of the reproductive biology of the narrow endemic Naufraga balearica (Apiaceae) for its conservation status. Plant Systematics Evol (2012) 298:581–96. doi: 10.1007/s00606-011-0568-2
65. Huda AN, Salmah MR, Hassan AA, Hamdan A, Razak MN. Pollination services of mango flower pollinators. J Insect Sci (2015) 15:1–8. doi: 10.1093/jisesa/iev090
66. Schürch S, Pfunder M, Roy BA. Effects of ants on the reproductive success of Euphorbia cyparissias and associated pathogenic rust fungi. Oikos (2000) 88:6–12. doi: 10.1034/j.1600-0706.2000.880102.x
67. Rostás M, Bollmann F, Saville D, Riedel M. Ants contribute to pollination but not to reproduction in a rare calcareous grassland forb. PeerJ (2018) 6:1–16. doi: 10.7717/peerj.4369
68. Carvalheiro LG, Barbosa ERM, Memmott J. Pollinator networks, alien species and the conservation of rare plants: Trinia glauca as a case study. J Appl Ecol (2008) 45:1419–27. doi: 10.1111/j.1365-2664.2008.01518.x
69. Schiestl FP, Glaser F. Specific ant-pollination in an alpine orchid and the role of floral scent in attracting pollinating ants. Alpine Bot (2012) 122:1–9. doi: 10.1007/s00035-011-0098-0
70. Puterbaugh MN. The roles of ants as flower visitors: experimental analysis in three alpine plant species. Oikos (1998) 83:36–46. doi: 10.2307/3546544
71. Brantjes NBM. Ant, bee and fly pollination in Epipactis palustris (L.) crantz (Orchidaceae). Acta Botanica Neerlandica (1981) 30:59–68. doi: 10.1111/j.1438-8677.1981.tb00387.x
72. Ashman TL, King EA. Are flower-visiting ants mutualists or antagonists? a study in a gynodioecious wild strawberry. Am J Bot (2005) 92:891–95. doi: 10.3732/ajb.92.5.891
73. Wyatt R. Ant-pollination of the granite outcrop endemic Diamorpha smallii (Crassulaceae). Am J Bot (1981) 68:1212–17. doi: 10.1002/j.1537-2197.1981.tb07827.x
74. García MB, Espadaler X, Olesen JM. Extreme reproduction and survival of a true cliffhanger: the endangered plant Borderea chouardii (Dioscoreaceae). Plos One (2012) 7:. doi: 10.1371/journal.pone.0044657
75. Cursach J, Rita J. Reproductive biology and reproductive output assessment in natural and introduced subpopulations of Apium bermejoi, a 'Critically endangered' endemic plant from menorca (western Mediterranean). Nordic J Bot (2012) 30:754–68. doi: 10.1111/j.1756-1051.2012.01437.x
76. Kawakita A, Kato M. Floral biology and unique pollination system of root holoparasites, Balanophora kuroiwai and b. tobiracola (Balanophoraceae). Am J Bot (2002) 89:1164–70. doi: 10.3732/ajb.89.7.1164
77. Abbate AP, Campbell JW. Parasitic beechdrops (Epifagus virginiana): a possible ant-pollinated plant. Southeastern Nat (2013) 12:661–65. doi: 10.1656/058.012.0318
78. Gómez JM, Zamora R. Generalization vs. specialization in the pollination system of Hormathophylla spinosa (Cruciferae). Ecology (1999) 80:796–805. doi: 10.1890/0012-9658(1999)080[0796:GVSITP]2.0.CO;2
79. Garcia MB, Antor RJ, Espadaler X. Ant pollination of the palaeoendemic dioecious Borderea pyrenaica (Dioscoreaceae). Plant Systematics Evol (1995) 198:17–27. doi: 10.1007/BF00985105
80. Pérez-Collazos E, Segarra-Moragues JG, Villar L, Catalán P. Ant pollination promotes spatial genetic structure in the long-lived plant Borderea pyrenaica (Dioscoreaceae). Biol J Linn Soc (2015) 116:144–55. doi: 10.7717/peerj.4369
81. Ekeke C, Ogazie CA, Agbagwa IO. Breeding biology and effect of pollinators on the fruit characteristics of cucumber (Cucumis sativus l.), cucurbitaceae. Nigerian J Bot (2018) 31:325–44.
82. Komamura R, Koyama K, Yamauch T, Konno Y, Gu L. Pollination contribution differs among insects visiting Cardiocrinum cordatum flowers. Forests (2021) 12:1–11. doi: 10.3390/f12040452
83. Peakall R, Angus CJ, Beattie AJ. The significance of ant and plant traits for ant pollination in Leporella fimbriata. Oecologia (1990) 84:457–60. doi: 10.1007/BF00328160
84. Mohsen AMA. Survey of insect mango pollinators and the pollination occurrence of mango trees in relations to fruit yields. Curr Sci Int (2019) 8:245–51.
85. Peakall R, Beattie AJ. Pollination of the orchid Microtis parviflora r. br. by flightless worker ants. Funct Ecol (1989) 3:515–22. doi: 10.2307/2389565
86. Ramsey M. Ant pollination of the perennial herb Blandfordia grandiflora (Liliaceae). Oikos (1995) 74:265–72. doi: 10.2307/3545656
87. Kuriakose G, Sinu PA, Shivanna KR. Ant pollination of Syzygium occidentale, an endemic tree species of tropical rain forests of the Western ghats, India. Arthropod-Plant Interact (2018) 12:647–55. doi: 10.1007/s11829-018-9613-1
88. García Gila J, Blasco-Aróstegui J. First report on the pollination of Neotinea maculata (Orchidaceae) by minor worker ants of the temnothorax exilis group (Hymenoptera: formicidae). Mediterranean Botany (2021) 42:1–5. doi: 10.5209/mbot.71171
89. Zhongjian L, Lijun C, Kewei L, Liqiang L, Xueyong M, Wenhui R. Chenorchis, a new orchid genus, and its eco-strategy of ant pollination. Acta Ecologica Sin (2008) 28:2433–44. doi: 10.1016/S1872-2032(08)60055-4
90. Biella P, Tommasi N, Akter A, Guzzetti L, Klecka J, Sandionigi A, et al. Foraging strategies are maintained despite workforce reduction: a multidisciplinary survey on the pollen collected by a social pollinator. PloS One (2019) 14:1–20. doi: 10.1371/journal.pone.0224037
91. Dorman M, Erell E, Vulkan A, Kloog I. Shadow: r package for geometric shadow calculations in an urban environment. R J (2019) 11:1–26. doi: 10.32614/RJ-2019-024
92. Laha S, Chatterjee S, Das A, Smith B, Basu P. Selection of non-crop plant mixes informed by arthropod-plant network analyses for multiple ecosystem services delivery towards ecological intensification of agriculture. Sustainability (2022) 14:1–13. doi: 10.3390/su14031903
93. Almeida-Neto M, Guimaraes P, Guimaraes Jr.PR, Loyola RD, Ulrich W. A consistent metric for nestedness analysis in ecological systems: reconciling concept and measurement. Oikos (2008) 117:1227–39. doi: 10.1111/j.0030-1299.2008.16644.x
94. Blüthgen N, Menzel F, Blüthgen N. Measuring specialization in species interaction networks. BMC Ecol (2006) 6:1–12.
95. Fiala B, Maschwitz U, Pong TY, Helbig AJ. Studies of a south East Asian ant-plant association: protection of macaranga trees by Crematogaster borneensis. Oecologia (1989) 79:463–70. doi: 10.1007/BF00378662
96. Goitía W, Jaffé K. Ant-plant associations in different forests in Venezuela. Neotropical Entomology (2009) 38:7–31. doi: 10.1590/S1519-566X2009000100002
97. Araújo WSD. Global patterns in the structure and robustness of plant-herbivore networks. Front Biogeography (2016) 8:1–8. doi: 10.21425/F58331053
98. Campbell AJ, Gigante Carvalheiro L, Gastauer M, Almeida-Neto M, Giannini TC. Pollinator restoration in Brazilian ecosystems relies on a small but phylogenetically-diverse set of plant families. Sci Rep (2019) 9:1–10. doi: 10.1038/s41598-019-53829-4
99. Kaiser-Bunbury CN, Muff S, Memmott J, Müller CB, Caflisch A. The robustness of pollination networks to the loss of species and interactions: a quantitative approach incorporating pollinator behaviour. Ecol Lett (2010) 13:442–52. doi: 10.1111/j.1461-0248.2009.01437.x
100. Russo L, DeBarros N, Yang S, Shea K, Mortensen D. Supporting crop pollinators with floral resources: network-based phenological matching. Ecol Evol (2013) 3:3125–40. doi: 10.1016/j.agee.2017.05
101. Hernandez-Cumplido J, Forter B, Moreira X, Heil M, Benrey B. Induced floral and extrafloral nectar production affect ant-pollinator interactions and plant fitness. Biotropica (2016) 48(3):342–8. doi: 10.1111/btp.12283
102. Dejean A, Benhamou S. Orientation and foraging movements in a patchy environment by the ant serrastruma lujae (Formicidae-myrmicinae). Behav processes (1993) 30:233–43. doi: 10.1016/0376-6357(93)90135-E
103. Horsburgh M, Semple JC, Kevan PG. Relative pollinator effectiveness of insect floral visitors to two sympatric species of wild aster: Symphyotrichum lanceolatum (Willd.) nesom and S. lateriflorum (L.) löve & löve (Asteraceae: astereae). Rhodora (2011) 113:64–86. doi: 10.3119/08-09.1
104. Losapio G, Norton Hasday E, Espadaler X, Germann C, Ortiz-Sánchez FJ, Pont A, et al. Facilitation and biodiversity jointly drive mutualistic networks. J Ecol (2021) 109:2029–37. doi: 10.1101/2020.08.25.266346
105. Koffel T, Kremer CT, Bannar-Martin K, Ernest SM, Eisenhauer N, Roscher C, et al. Modeling how community assembly alters the functioning of ecosystems. bioRxiv (2021), 2020–02. doi: 10.1101/2020.02.10.942656
106. Li P, Luo Y, Bernhardt P, Kou Y, Perner H. Pollination of Cypripedium plectrochilum (Orchidaceae) by Lasioglossum spp. (Halictidae): the roles of generalist attractants versus restrictive floral architecture. Plant Biol (2008) 10:220–30. doi: 10.1111/j.1438-8677.2007.00020.x
107. Burkle LA, Runyon JB. Floral volatiles structure plant–pollinator interactions in a diverse community across the growing season. Funct Ecol (2019) 33:2116–29. doi: 10.1111/1365-2435.13424
108. Lach L. Invasive ants: unwanted partners in ant-plant interactions? Ann Missouri Botanical Garden (2003) 90:91–108. doi: 10.2307/3298529
109. Martins DJ, Johnson SD. Hawkmoth pollination of aerangoid orchids in Kenya, with special reference to nectar sugar concentration gradients in the floral spurs. Am J Bot (2007) 94:650–59. doi: 10.3732/ajb.94.4.650
110. De LC, Rao AN, Rajeevan PK, Pathak P. Orchid improvement–an overview. J Orchid. Soc India (2014) 28:35–45.
111. Dodson CH, Dressler RL, Hills HG, Adams RM, Williams NH. Biologically active compounds in orchid fragrances: function of natural plant products in orchid flower odors and the attraction of specific pollinators are described. Science (1969) 164:1243–49. doi: 10.1126/science.164.3885.1243
112. Martins DJ. Pollination and facultative ant-association in the African leopard orchid Ansellia africana. J East Afr Natural History (2009) 98:67–77. doi: 10.2982/028.098.0105
113. Martínez-Bauer AE, Martínez GC, Murphy DJ, Burd M. Multitasking in a plant–ant interaction: how does Acacia myrtifolia manage both ants and pollinators? Oecologia (2015) . 178:461–71. doi: 10.1007/s00442-014-3215-0
114. Kawakita A, Kato M. Repeated independent evolution of obligate pollination mutualism in the phyllantheae–Epicephala association. Proc R Soc B: Biol Sci (2009) 276:417–26. doi: 10.1098/rspb.2008.1226
115. Hargreaves AL, Harder LD, Johnson SD. Consumptive emasculation: the ecological and evolutionary consequences of pollen theft. Biol Rev (2009) 84:259–76. doi: 10.1111/j.1469-185X.2008.00074.x
116. Irwin RE, Bronstein JL, Manson JS, Richardson L. Nectar robbing: ecological and evolutionary perspectives. Annu Rev ecology evolution systematics (2010) 41:271–92. doi: 10.1146/annurev.ecolsys.110308.120330
Keywords: ant pollination, myrmecophilous plant, floral characteristics, pollination network, seasonal bias
Citation: Das S and Das A (2023) Ants are more than just curious bystanders to some flowers—they act as significant pollinators. Front. Insect Sci. 3:1145761. doi: 10.3389/finsc.2023.1145761
Received: 16 January 2023; Accepted: 25 May 2023;
Published: 15 June 2023.
Edited by:
Domancar Orona-Tamayo, Centro de Innovación Aplicada en Tecnologías Competitivas (CIATEC), MexicoReviewed by:
Javaid Iqbal, King Saud University, Saudi ArabiaBożena Denisow, University of Life Sciences of Lublin, Poland
Copyright © 2023 Das and Das. This is an open-access article distributed under the terms of the Creative Commons Attribution License (CC BY). The use, distribution or reproduction in other forums is permitted, provided the original author(s) and the copyright owner(s) are credited and that the original publication in this journal is cited, in accordance with accepted academic practice. No use, distribution or reproduction is permitted which does not comply with these terms.
*Correspondence: Amlan Das, ZGFzYW1sYW5AeWFob28uY28uaW4=