- 1Centre Oceanogràfic de Balears, Instituto Español de Oceanografía, Palma, Spain
- 2Institute of Marine Sciences (ICM-CSIC), Barcelona, Spain
- 3Norwegian Institute for Water Research, Marine Biology, Oslo, Norway
- 4Ryan Institute, National University of Ireland Galway, Galway, Ireland
- 5School of biological and Marine Sciences, Plymouth University, Plymouth, UK
- 6GRID-Arendal, Arendal, Norway
- 7National Oceanography Centre, University of Southampton Waterfront Campus, Southampton, UK
- 8Centro Austral de Investigaciones Científicas-CONICET, Ushuaia, Argentina
- 9Ifremer, REM/EEP/Laboratoire Environnement Profond, Centre de Bretagne, Plouzané, France
- 10NOAA/NMFS, National Systematics Lab, Washington, DC, USA
- 11National Institute of Water and Atmospheric Research, Coasts and Oceans, Wellington, New Zealand
- 12Ifremer, RBE/HGS/Laboratoire Ressources Halieutiques d'Aquitaine, Anglet, France
Submarine canyons are major geomorphic features of continental margins around the world. Several recent multidisciplinary projects focused on the study of canyons have considerably increased our understanding of their ecological role, the goods, and services they provide to human populations, and the impacts that human activities have on their overall ecological condition. Pressures from human activities include fishing, dumping of land-based mine tailings, and oil and gas extraction. Moreover, hydrodynamic processes of canyons enhance the down-canyon transport of litter. The effects of climate change may modify the intensity of currents. This potential hydrographic change is predicted to impact the structure and functioning of canyon communities as well as affect nutrient supply to the deep-ocean ecosystem. This review not only identifies the ecological status of canyons, and current and future issues for canyon conservation, but also highlights the need for a better understanding of anthropogenic impacts on canyon ecosystems and proposes other research required to inform management measures to protect canyon ecosystems.
Introduction
As resources on land are increasingly depleted, humanity is turning to the oceans, as never before, for new sources of food and materials (Ramirez-Llodra et al., 2011). A complex and mixed interplay of impacts resulting from fisheries, oil and gas operations, mining practices, and many other anthropogenic activities, have caused unintended damage to ecosystems (Davies et al., 2007). This, in turn, may affect the supply of targeted resources, as well as impact other ecosystem services. This scenario hinders the achievement of UN Millennium Assessment goals relating to human wellbeing, including having sufficient food at all times and having a healthy physical environment (MA, 2005). Therefore, the identification and protection of key marine habitats, especially those that substantially contribute to important ecological services (e.g., nutrient cycling, carbon sequestration, fisheries, nursery grounds, and habitat support) must become a priority.
The deep sea, the largest biome on Earth, is composed of a variety of different habitats with specific biotic and abiotic characteristics (Ramirez-Llodra et al., 2010). Submarine canyons are one of these habitats. Recent novel technological developments including underwater acoustic mapping, imaging, and sampling technologies, and long-term/permanent moored or benthic observatories, have greatly contributed to our understanding of the diverse and complex hydrodynamics (Xu, 2011) and geomorphology of canyons over the last two decades (Robert et al., 2014; Quattrini et al., 2015), allowing the spatio-temporal tracking of oceanographic processes and the associated biological responses, with an integration level that grows every day (Aguzzi et al., 2012; Matabos et al., 2014). As a result of prospective surveys, we know that submarine canyons are major geomorphic features of continental margins, with more than 9000 large canyons covering 11.2% of continental slopes globally (Harris et al., 2014), with an estimated accumulated axis length of over 25,000 km (Huang et al., 2014). Canyons are characterized by steep and complex topography (Shepard and Dill, 1966; Lastras et al., 2007; Harris and Whiteway, 2011) that influences current patterns (Shepard et al., 1979; Xu, 2011) and provides a heterogeneous set of habitats, from rocky walls and outcrops to soft sediment (De Leo et al., 2014). These geomorphologic features act as preferential particle-transport routes from the productive coastal zone down continental slopes to the more stable deep seafloor (Allen and Durrieu de Madron, 2009; Puig et al., 2014).
Canyons have been described as “keystone structures” (Vetter et al., 2010) because of their role as relevant sources of goods and services to human populations. An increasing amount of data provides evidence of how canyons act benefiting and supporting fisheries (Yoklavich et al., 2000; Company et al., 2012), and enhance carbon sequestration and storage (Epping et al., 2002; Canals et al., 2006; Masson et al., 2010). Canyon habitats also provide nursery (Sardà and Cartes, 1994; Hoff, 2010; Fernandez-Arcaya et al., 2013) and refuge sites for other marine life (Tyler et al., 2009; De Leo et al., 2010; Vetter et al., 2010; Morris et al., 2013), including vulnerable marine ecosystems and essential fish-habitats such as cold-water corals and sponge fields (Schlacher et al., 2007; Huvenne et al., 2011; Davies et al., 2014). Canyons have also been shown to provide habitat for spawning females of pelagic and benthic species of commercial interest (Farrugio, 2012). Other faunal components of marine ecosystems, including mammals and marine birds, also use canyons, for example, as feeding grounds (Abelló et al., 2003; Garcia and Thomsen, 2008; Roditi-Elasar et al., 2013; Moors-Murphy, 2014). Habitat diversity and specific abiotic characteristics enhance the occurrence of high levels of biodiversity in some canyons (Vetter and Dayton, 1998; McClain and Barry, 2010; Company et al., 2012; De Leo et al., 2014). Because of this biodiversity, canyons can be a rich source of genetic resources and chemical compounds (i.e., the use of canyon organisms in biotechnological, pharmaceutical, or industrial applications, Jobstvogt et al., 2014).
The steep slopes and rocky topography have limited exploitation of seafloor resources within canyons through activities such as bottom-trawl fishing (Würtz, 2012). Consequently, many canyon areas experience lower levels of anthropogenic pressure than adjacent areas on the shelf and slope. Nevertheless, submarine canyons are increasingly subjected to different stressors, not only in relation to fishing (Company et al., 2008; Martín et al., 2008; Orejas et al., 2009; Puig et al., 2012), but also to oil and gas extraction (Harris et al., 2007). Moreover, the hydrodynamic processes of canyons enhance the transport of litter (Mordecai et al., 2011; Ramirez-Llodra et al., 2013; Tubau et al., 2015) and chemical pollutants from the shelf to deep-sea environments (Palanques et al., 2008; Koenig et al., 2013; Pham et al., 2014). Canyons have also been used as dumping areas for tailings of land-based mining (Hughes et al., 2015; Ramirez-Llodra et al., 2015). Additionally, effects of climate change may affect the physical and chemical characteristics of water masses, modifying the intensity of currents (Canals et al., 2009). These modifications may seriously impact the structure and functioning of canyon communities and have important implications for nutrient supply to the deep-ocean ecosystem (Solomon, 2007; Levin and Le Bris, 2015) as well as for carbon storage (Epping et al., 2002; Masson et al., 2010). Thus, anthropogenic threats to submarine canyons demand urgent responses to ensure sound ecosystem-based management of human resources based on robust scientific data that will guarantee both the development of local and regional economies and the long-term sustainability of ecosystems and the services they provide.
The aims of this review are to: (1) highlight current scientific knowledge concerning canyon ecosystems; (2) describe our current understanding of the role played by canyons in providing ecosystem goods and services; (3) identify the impacts to which canyons are increasingly being subjected; (4) review current frameworks and strategies for protecting canyons, and assess the extent of current canyon conservation worldwide; before finally, (5) identifying directions for future canyon research, with a focus on the conservation needs of canyons. This review is based on published literature and expert knowledge, and is the result of discussions held during the 2014 International Network for submarine Canyon Investigation and Scientific Exchange workshop (INCISE, www.incisenet.org, Huvenne and Davies, 2014).
The Ecological Role of Canyons
The interplay between canyon topography and oceanic currents has profound consequences for the diversity, functioning, and dynamics of both pelagic and benthic communities. For example, currents funneled through canyons likely enhance primary productivity (Ryan et al., 2005) and drive sediment transport and associated particle-reactive substances toward deep environments (Puig et al., 2014). Higher levels of primary productivity may lead to canyons being hotspots of faunal productivity in the deep sea (De Leo et al., 2010). The highly variable seascapes within a canyon support diverse assemblages of species that play a wide variety of ecological roles, often across small spatial scales, giving rise to enhanced biodiversity, and ecosystem function (McClain and Barry, 2010). Given their local importance, canyons represent a relevant regional source of marine biodiversity and ecosystem function (Leduc et al., 2014).
Canyon Effects on Local Circulation and Sedimentation
On many continental margins, cross-shelf exchanges of water, and particulate matter are inhibited by the presence of density fronts and associated slope currents flowing parallel to the isobaths (e.g., Font et al., 1988). Submarine canyons intercept the path of these currents, inducing a new dynamic balance, eventually enhancing non-geostrophic motions, and shelf-slope exchanges (Huthnance, 1995). Near the seafloor, alignment of the current with the direction of the canyon axis is commonly observed (Shepard et al., 1979; Puig et al., 2000). The adjustments of the current to the canyon topography produce vortex stretching and vertical motions (Klinck, 1996; Hickey, 1997). These modifications of the currents may result in local upwelling, which pumps nutrients to the euphotic zone and thus stimulates primary production (Ryan et al., 2005). Additionally, closed-circulation cells and downwelling may develop over canyons, enhancing the capacity of the canyon to trap particles transported by long-shore currents (Granata et al., 1999; Palanques et al., 2005; Allen and Durrieu de Madron, 2009). When thermohaline stratification of the water column is strong, the flow in the upper mixed layer may decouple from the underlying water levels, which interact with the rims of the canyon. In such a scenario, the current flowing above the canyon head tends to follow its path, ignoring the bottom topography, while the flow below the rim is deflected by the canyon (e.g., Palanques et al., 2005). This current flow can also induce the formation and focussing of internal waves (e.g., Hall and Carter, 2011).
Most of the particulate organic matter introduced into the marine environment by riverine inputs and coastal surface productivity, particularly the most labile fraction, is mineralized after several cycles of seafloor deposition/resuspension on the continental shelf. In contrast to this scenario, canyons act as morphological shortcuts, accelerating the transit of particles from fertile coastal and inner shelf environments toward the deep sea, thus enhancing the role of canyons as sedimentary depocentres, where enhanced oxidation and burial of organic carbon occurs (Epping et al., 2002; Masson et al., 2010). Additionally, large storm waves, hyperpycnal flows, dense shelf-water cascades, earthquakes, and other processes trigger mass failures of unstable deposits within canyon heads and on the shelf-edge areas of shelf-incising canyons (reviewed in Puig et al., 2014). Sediments (and associated organic matter) entrained in turbidity flows are exported from the canyon system for deposition on adjacent submarine fans (Talling, 2014). Thus, particle fluxes and sediment accumulation rates have been found to be much larger inside submarine canyons than in the adjacent non-dissected margin at comparable depths (Martín et al., 2006; Zúñiga et al., 2009).
Canyon Effects on Pelagic and Motile Benthic or Demersal Fauna
In the pelagic realm, the diversity, and complexity of food webs increase in response to canyon-induced upwelling of nutrients. The high level of primary production attracts pelagic-associated secondary and tertiary consumers. Abundances of megafaunal species, including a variety of demersal fishes, large pelagic predators such as tuna, swordfish, and sharks, as well as cetaceans and birds, are enhanced. All these predators are likely to be present in canyon areas for feeding and breeding, albeit intermittently in some cases (Rennie et al., 2009). For example, demersal fishes, such as macrourids and cusk (Brosme brosme), in Baltimore and Norfolk canyons (NW Atlantic), prey upon large swarms of euphausiids and amphipods as well as benthic species, such as brittle stars, which are abundant because of canyon-enhanced productivity (Ross et al., 2015). In addition, canyons may concentrate motile megafauna that leave the adjacent slope in an attempt to evade visual predators by hiding within the complex canyon topography (Farrugio, 2012). Doya et al. (2014) recorded high numbers of sablefish (Anoplopoma fimbria) along Barkley canyon walls at approximately 900m depth from the NEPTUNE Ocean Observatory, Canada. Canyon geomorphology can trap diel vertical migrants, such as hyperiid amphipods and euphausiids, when wind-generated currents push animals toward the canyon heads (Macquart-Moulin and Patriti, 1996). These trapped individuals regain their original depth position by swimming along the seabed, adopting a more nektobenthic mode of movement, in order to restart a new vertical migration cycle (Aguzzi and Company, 2010).
The accumulation of organic matter, caused by the physical and geological characteristics of some submarine canyons, promotes higher abundances, biomass, and diversity of organisms compared to the adjacent open slope (Figure 1). These conditions have been observed at eutrophic canyons along continental margins (Brodeur, 2001) as well as in those associated with oligotrophic conditions on oceanic islands (De Leo et al., 2012). Elevated sedimentation rates inside submarine canyons can favor benthic detritivores (Puig et al., 2015) and fauna capable of rapidly conveying the organic material produced in the upper water column (Bianchelli et al., 2010), thereby processing large amounts of carbon for input into the benthic food web (Vetter and Dayton, 1998; De Leo et al., 2010; van Oevelen et al., 2011). Canyons can also influence the depth distribution and population structure of particular species during the various stages of its life cycle, thus affecting the distribution of biomass and density of specific life stages. For example, in some fishes (e.g., monkfish Lophius piscatorius, hake Merluccius merluccius), larger spawning females have been more commonly observed inside submarine canyons (e.g., Petit-Rhône and Grand-Rhône) than on the adjacent open slope (Farrugio, 2012). Additionally, canyons can act as recruitment grounds for some species of fishes and crustaceans. High abundances of egg cases of an unknown species of scyliorhinid catshark were found among coral in Mississippi Canyon, Gulf of Mexico (Etnoyer and Warrenchuk, 2007). In the Gulf of Lions (NW Mediterranean), periodic dense shelf-water cascading events supply large amounts of organic material to bathyal and abyssal areas (Canals et al., 2006; Pasqual et al., 2010). Recruitment of the highly mobile deep-sea shrimp Aristeus antennatus is enhanced in years following such events (Company et al., 2012). In Blanes Canyon (NW Mediterranean), benthic, and intermediate nepheloid layers, with significant amounts of suspended sediment, are present year round. There is evidence that the juveniles of some deep-sea shrimps (Plesionika heterocarpus, P. edwardsi, P. giglioli, and P. martia) and fishes (Phycis blennoides, Mora moro, Lepidion lepidion amongst others) concentrate in the benthic intermediate nepheloid layers this canyon, which act as a nursery area for these species (Puig et al., 2001; Fernandez-Arcaya et al., 2013).
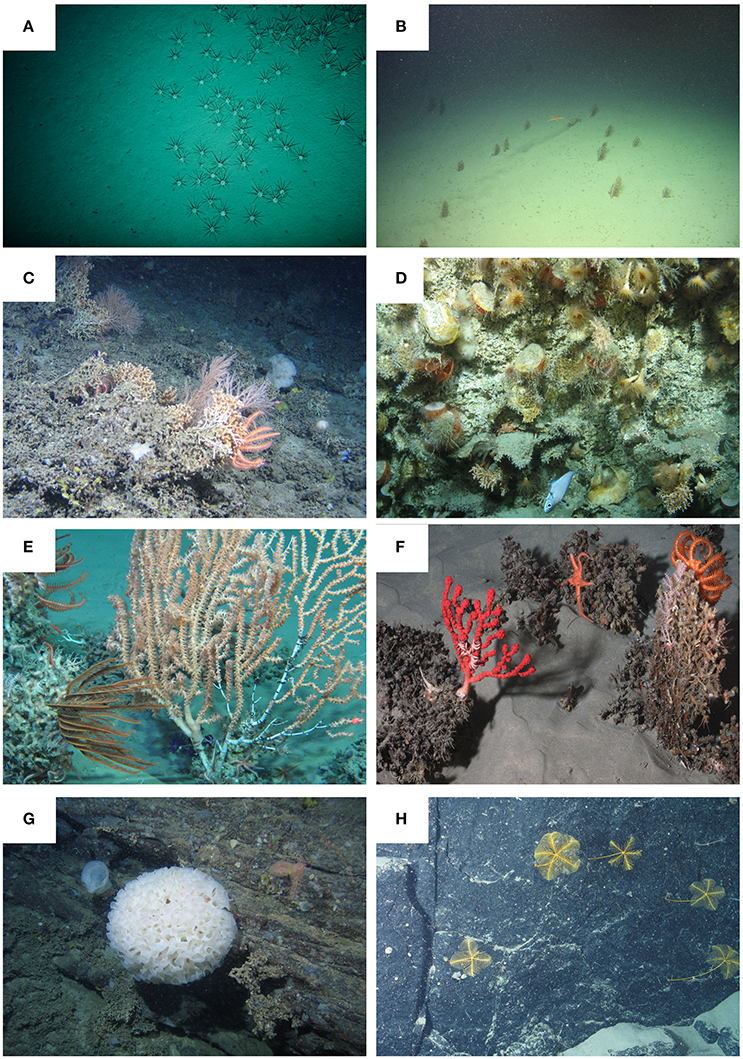
Figure 1. Images showing diversity of habitats in submarine canyons. (A) An aggregation of the echinoid Cidaris cidaris on soft sediment in a Bay of Biscay canyon (NE Atlantic). Copyright: Ifremer, Evhoe 2011. (B) Field of the sea pen Kophobelemnon at approximately 750m in Whittard canyon. Cruise CE14009. (C) Coral habitat at approximately 1500m in an unnamed canyon north of the Porcupine Bank showing a brisingid seastar, Solenosmilia coral, bamboo coral, and white and yellow sponges. Cruise CE10004. (D) Biotope at 633 to 762 m depth on a vertical wall in Whittard Canyon dominated by large limid bivalves, Acesta excavata and oyster Neopycnodonte zibrowii. The scleractinian corals Madrepora oculata and Desmophyllum are also present. Cruise CE12006 (E) Cold-water coral community at about 1800m in Whittard Canyon with bamboo coral in the foreground and Solenosmilia to the left. The crinoid is Koehlermetra and orange brisingids are top left. Cruise CE14009. (F), Cold-water coral community in a canyon in the Bay of Biscay. Dead framework of a colonial scleractinian is colonized by a Paragorgia and an unidentified gorgonian as well as many ophiuroids and a brisingid asteroid. Copyright: Ifremer, BobEco cruise 2011. (G), A hexactinellid sponge, probably the genus Farrea in an unnamed canyon north of Porcupine Bank. CE13008 cruise. (H), Stalked crinoid Anachalypsicrinus neferti on a vertical wall in a Bay of Biscay canyon. Copyright: Ifremer, BobEco cruise 2011. Images (B–E,G) taken by ROV Holland I, deployed from RV Celtic Explorer. Copyright NUI Galway / Marine Institute.
Canyon Effects on Benthic Sessile Fauna and Infauna
In the benthic realm, enhanced primary production and current regimes provide suitable ecological niches for large and abundant suspension and filter feeders, such as sponges and cold-water corals (Figure 1). Resuspension of particulate organic matter (POM), combined with a slower deposition of particulate matter descending from the euphotic zone, leads to higher levels of particulates and nutrients in the water column inside canyons (Bosley et al., 2004), resulting in enhanced primary productivity. Suspension feeders and demersal planktivores likely benefit from these high concentrations of primary producers (Vetter et al., 2010). In Whittard Canyon (Celtic Margin of the NE Atlantic), accelerated currents increase the organic matter influx and therefore the availability of food compared to less active areas on the continental slope (Morris et al., 2013; Palmas et al., 2015). In the upper region of Whittard Canyon (~700m depth), a very dense assemblage of corals and large bivalves was observed associated with a nepheloid layer that might provide a significant amount of food (Johnson et al., 2013). Furthermore, the scleractinian coral Lophelia pertusa was observed at great depth and higher densities in this canyon, than usually recorded in the NE Atlantic (Huvenne et al., 2011). The deepening of the distribution of L. pertusa could be related to downslope transport processes in canyons (Dullo et al., 2008). Likely mechanisms for food transport include hydrodynamic processes such as gravity currents and internal waves, or by the trapping effect of the canyon topography itself (Huvenne et al., 2011; Morris et al., 2013; Amaro et al., 2016). Similarly, high densities of gorgonians, pennatulids, and sponges in Pribilof and Zhemchug canyons (Bering Sea) may be supported by enhanced levels of primary productivity delivered by strong currents (Miller et al., 2012). Likewise, canyons intersecting the shelf break in East Antarctica, experience strong currents and particle fluxes and support dense communities of corals and sponges (Post et al., 2010).
Patches of detritus have been described as hotspots of food resources in canyons. These patches not only support locally high numbers of deposit feeders that benefit from the accumulation of macrophyte detritus, but also a variety of crustaceans associated with down-welling (Vetter, 1995; Okey, 1997). Overall, the presence of detritus patches in canyons provides an additional food source, contributing to higher densities, and biomass of infauna in canyon sediments than in sediments on the adjacent shelf and slope (Vetter and Dayton, 1998). Bianchelli et al. (2010) examined meiofaunal assemblages in five submarine canyons and adjacent slope habitats along Portuguese, Catalan, and Adriatic margins. Their results suggest that available food sources, including detritus, as well as topographic and hydrodynamic features of canyons, influence meiofaunal abundance and biomass.
Within canyons, the complex topography alters current regimes and therefore, sediment-transport processes, influencing the patchy distribution of large sessile megafauna. The dissymmetric distribution and abundance of corals between opposite flanks of a canyon is a common feature reported from Lacaze-Duthiers, Cassidaigne, and Cap de Creus canyons in the Mediterranean Sea (Orejas et al., 2009; Fabri et al., 2014), Guilvinec, Penmarc'h, and Whittard canyons in the NE Atlantic (De Mol et al., 2011; Morris et al., 2013; Robert et al., 2014) and The Gully in the NW Atlantic (Mortensen and Buhl-Mortensen, 2005). Similarly, distribution of corals and sponges at the heads of shelf-incising canyons seems to be related to strong currents that expose underlying bedrock in these areas (De Mol et al., 2011). At a smaller scale, steep features of exposed rock, such as vertical walls and overhangs, facilitate the settlement of the scleractinian corals L. pertusa and Madrepora oculata (Freiwald et al., 2009; Huvenne et al., 2011; Gori et al., 2013; Morris et al., 2013; Davies et al., 2014; Fabri et al., 2014).
In addition to currents and topography, substrate heterogeneity is a key factor contributing to the highly diverse faunal assemblage present in submarine canyons (De Leo et al., 2014). Submarine canyons host a wide variety of substrate types, including mud, sand, hardground, gravel, cobbles, pebbles, boulders, and rocky walls, occurring either separately or in various combinations (Baker et al., 2011, Figure 2). Most species are restricted to either hard substratum (most scleractinians, antipatharians, most gorgonians, most sponges) or soft substratum (most pennatulids, some scleractinians, some gorgonians, some sponges). For example, in Pribilof and Zhemchung canyons (Bering Sea), gorgonians, and sponges were associated with hard substrate, while pennatulids were associated with soft sediment (Miller et al., 2012). In Bari Canyon (Adriatic Sea), denser sponge aggregations were found on rocks and dead corals than in areas with heavy sedimentation rates (Bo et al., 2011). In Halibut Channel, Haddock Channel, Desbarres Canyon, and The Gully (eastern Canada margin), observed species combinations were dependent on the dominant substrate type (Hargrave et al., 2004; Mortensen and Buhl-Mortensen, 2005; Baker et al., 2011). Sponge diversity was also positively correlated with substrate heterogeneity in five canyons off the southeastern Australian margin (Schlacher et al., 2007).
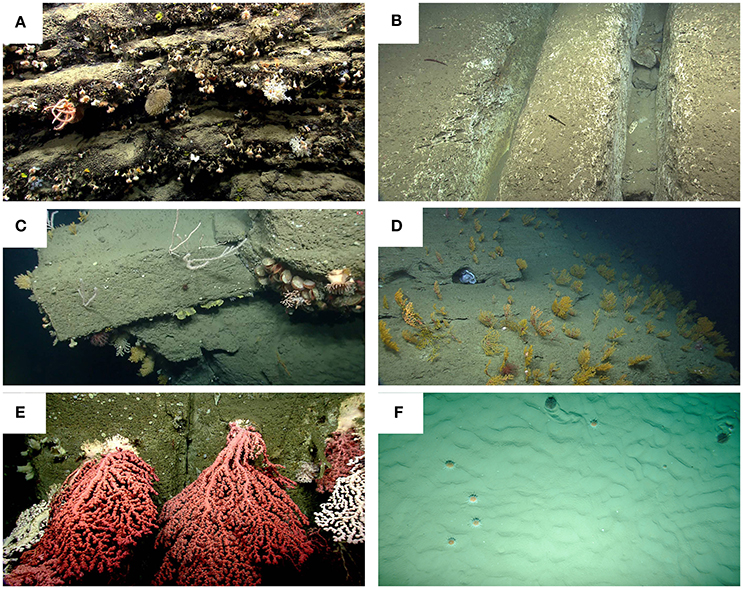
Figure 2. Examples of substrate types in submarine canyons. (A) Stony corals (predominately the solitary coral, Desmophyllum dianthus), sponges and a brisingid sea star populate the rugged, manganese-encrusted rock ledges observed on the wall of Nygren Canyon. (B) Chutes, pictured here in Lydonia Canyon, are formed by physical abrasion of the carbonate-rich rock. (C) A variety of coral species and limid bivalves colonize the edges and smooth faces of tabular blocks and eroded mudstone and siltstone in Oceanographer Canyon. (D) An extensive field of Paramuricea sp., most hosting associated invertebrates, blankets the side of Welker Canyon. Note the octopus (center photo) sheltering in a small cave. An orange, rubber work glove partially buried on the floor of an un-named minor canyon adjacent to Shallop Canyon. (E) Numerous, large colonies of Paragorgia arborea were observed on the walls of Heezen Canyon. Corals, some as large as 5 m, attached to steep, clean walls, grow into the canyon channel. Photo credit (A–E), NOAA Okeanos Explorer Program, 2013 Northeast U.S. Canyons Expedition. (F) Two unidentified echinoid species on soft sediment in a Bay of Biscay canyon (NE Atlantic). Copyright: Ifremer, Evhoe 2010.
Structure-forming corals can occur in dense patches, fields or reefs in canyons. Coral colonies can form mound-like features (bioherms) or are found attached to vertical walls, overhangs, drop stones, or any exposed hard substrata within canyons (Orejas et al., 2009; De Mol et al., 2011; Huvenne et al., 2011; Davies et al., 2014; Sánchez et al., 2014). However, patches or reefs, particularly those composed of scleractinians, often have a low density of live corals and a high amount of sediment between the colonies. In canyons, the ecosystem engineering role of cold-water corals and sponge fields has not yet been studied in detail, which contrasts with the significant data available for carbonate mounds and seamounts on the role of corals as autogenic engineers providing substrate, shelter and/or feeding place for associated species (Buhl-Mortensen et al., 2010; Santos et al., 2010). Nevertheless, there are several examples of fish and invertebrate associations with corals in canyons. In the Bering Sea, rockfishes, sculpins, poachers, and pleuronectid flounders are associated with high densities of gorgonians, pennatulids, and sponges in Zhemchug and Pribilof canyons (Miller et al., 2012). In The Gully (NW Atlantic), Mortensen and Buhl-Mortensen (2005) found a positive relationship between coral species richness and the total number of megafauna taxa, however, the abundance of fish was not correlated with the abundance of corals. Coral species richness was an important factor in explaining the variation in both fish and crustacean assemblages in northwestern Atlantic canyons (Quattrini et al., 2015). Tissot et al. (2006) hypothesized that sea pen fields on deep banks off California (E Pacific) may have an important role as refuges for small invertebrates. In the canyons off Newfoundland, however, sea pen fields did not noticeably enhance the densities and richness of megafaunal assemblages (Baker et al., 2011).
Sediment instabilities and turbidity flows give rise to disturbance regimes in canyons that can affect the dynamics of some benthic populations and communities. For example, episodic disturbance events, caused erosive flows, and sediment-mixing processes linked to current modifications induced by the canyon topography, contribute to the instability of sediments, making conditions unfavorable to many infaunal species (Romano et al., 2013). As a consequence, differences in life-history strategies are reflected in species composition of the infaunal assemblages (e.g., nematodes) in different habitat types, with, for example, opportunistic species being more abundant inside canyon systems (Gambi and Danovaro, 2016). Sediment removal from the shallower canyon regions toward the deeper margin areas can also cause a decrease in overall available nutritional material. This decrease in food availability can lead to a progressive decrease in local abundances of benthic and demersal fauna, with a subsequent decline in overall biodiversity (Pusceddu et al., 2013). Benthic communities may experience periodic cycles of disturbance, recolonization and eventual recovery of communities (McClain and Schlacher, 2015). For example, Hess et al. (2005) studied benthic foraminifera contained in a time series of samples taken in Capbreton Canyon (Bay of Biscay, NE Atlantic) after a down-slope turbidity flow event. Their results suggest that populations of foraminiferans recovered in about 6–9 months. Samples taken down-core in successive turbidity sequences contained nearly the same faunal elements as the surface assemblages. Thus, it appears that community structure of these benthic foraminifera is confined to an early stage of recolonization (Hess et al., 2005). In Nazaré Canyon (off Portugal), Paterson et al. (2011) found that frequent physical disturbance in the middle and upper sections of the canyon axis had a dramatic impact on foraminifera, with only certain species able to colonize and survive in these habitats. These two studies are testament to the influence that relatively high frequency (sub-annual) turbidity currents can have on determining benthic community structure in canyons.
Canyons as Providers of Ecosystem Services
The Millennium Ecosystem Assessment (MA, 2005) identified the conservation of ecosystems and their environmentally-sustainable use as priorities to ensure the long-term well-being of the planet. To this end, understanding ecosystem services is essential. Ecosystem goods and services (hereafter, just services) refers to the socio-economic concept that places high regard on the benefits derived from ecosystem services that sustainably support human wellbeing (Armstrong et al., 2012; Thurber et al., 2014). Consequently, the focus of conservation has shifted from the conservation and preservation of species for the sake of the species only, to the conservation of the benefits derived from ecosystem services (MA, 2005). Ecosystem services can be classified into four major categories: (1) supporting services: those functions that feed into the other services, (2) provisioning services: goods obtained directly from habitats and ecosystems, (3) regulating services: benefits obtained through the natural regulation of habitats and associated ecosystem processes, and (4) cultural services: societal benefits, for example in terms of aesthetics and education (reviewed in Armstrong et al., 2012).
Supporting Services
Supporting services have an indirect effect, both physically and temporally, on human wellbeing, as they include the ecosystem functions (e.g., nutrient cycling, habitat provision, water circulation, or resilience) on which the other services are based (Armstrong et al., 2012). Submarine canyons provide several supporting services, through their role in sustaining marine food webs (van Oevelen et al., 2011) and providing a variety of habitats, including areas for larval settlement and recruitment (Sardà and Cartes, 1994; Fernandez-Arcaya et al., 2013). Canyons facilitate the transport of nutrients from the shelf to the deep basins, affecting the overall faunal abundance and biodiversity of an area (see Section The Ecological Role of Canyons), and play a role in the maintenance of provisioning services within canyons (e.g., fisheries, see Section Provisioning Services). The role of canyons as nursery and refuge grounds is important in maintaining these provisioning services. For example, populations of the red shrimp Aristeus antennatus in NW Mediterranean canyons undergo seasonal ontogenic migrations closely related to the geomorphology of the canyons (Sardà et al., 2009). Company et al. (2008) suggest that the large augment of nutrients transported during cyclic dense shelf-water cascading events provide an increased food resource that enhances recruitment of A. antennatus. The authors suggest that these cyclic natural events help mitigate the general increasing overexploitation trend of this species observed over the last six decades. The red-shrimp fishery is extremely important for the Fishermen's Guilds in the NE Spain region. In 2014, the red-shrimp fishery generated over 14 million of Euros for Catalonia alone (Unpubl. data. Directorate of Fishing and Maritime Affaires, Government of Catalonia, Barcelona, Spain.). Figure 3 shows the relationship between A. antennatus catches and the presence of submarine canyons, providing evidence of the supporting services offered by submarine canyons.
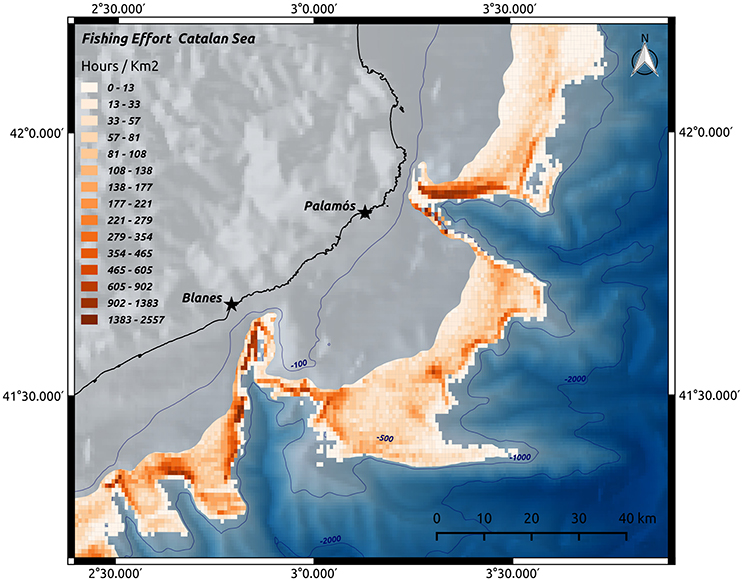
Figure 3. Spatial overlays of A. antennatus catch per unit effort (by trap fishery) on the bathymetric surface. High values are spatially associated with canyons. The trawling activity has been obtained by Vessel Monitoring System data from DeepMed Research Group©. (2006). (Biological data collection).
Regulating Services
Regulating services refer to benefits provided by natural regulatory functions of ecosystems through processes such as climate regulation, carbon sequestration, or detoxification of waste (Armstrong et al., 2012). Canyons play an important role in regulating carbon storage (Canals et al., 2006; Masson et al., 2010) and waste detoxification (Jobstvogt et al., 2014). As conduits for transport of sediment and organic matter to the deep sea, canyons contribute to the burying of carbon by taking it away from the surface layers and hence, play a role in climate regulation. Typically, biogenic particles settling on the seafloor undergo cycles of transport and re-deposition, influenced by tidal, storm, and cascading currents (de Stigter et al., 2011; Palanques et al., 2012; Sanchez-Vidal et al., 2012). Eventually, these particles reach a permanent accumulation region in zones of low hydrodynamic energy (de Haas et al., 2002). In canyons, specific hydrodynamic processes and higher particulate transport result in a significant cycling of organic carbon. This nutrient cycling service plays an important role in the gas, climate and waste regulation function, which in turn influences human health and productivity (De Groot et al., 2002). Additionally, these same transport processes can remove pollutants from shelf areas, carrying them to the deep sea where they are buried, transformed, or assimilated through processes such as bioturbation, decomposition, and sequestration (Armstrong et al., 2012). The monetary value of these services is currently outside the market system, with the exception of gas regulation ($1.3 109/year) (Costanza et al., 1997, 2014). Thus, the full monetary benefits of these regulating services have yet to be evaluated for canyon systems.
Provisioning Services
Provisioning services refer to the products obtained directly from the ecosystem, such as fish, hydrocarbons, minerals, or genetic resources. Submarine canyons can directly provide food resources through exploitation of the fish stocks and other species (e.g., crustaceans) occurring within them. However, most fisheries take place on the slopes adjacent to the canyons, where fishing is easier. In these cases, canyons can provide supporting services in the form of habitat, food, and nursery areas for commercial species (see Section Supporting services). Another provisional service of submarine canyons is as a source for cold-water corals harvested for jewelry (Foley and Armstrong, 2010). Canyons can also be a source of genetic resources. The deep sea is a particular target for biodiscovery because specific conditions (e.g., total darkness, low temperature, high pressure, and in some cases such as hydrothermal vents, very strong thermal gradients, and high concentration of metals) result in specific physiological and cellular adaptations of the fauna. These adaptations increase the likelihood of finding unique secondary metabolites that can be highly useful for commercial production of medicinally important compounds. The high “hit” rate in recent bio-discovery studies in the deep sea supports this prediction (Skropeta and Wei, 2014). Since the 1990s, almost 80% of novel marine natural products from invertebrates were derived from cnidarians and sponges (ca. 90% of the cnidarian discoveries being from octocorals; Leal et al., 2012). In a review of marine natural products, Blunt et al. (2013) emphasize that discovery of new marine-derived bioactive compounds are predominately from sponges. However, the importance of cnidarians as a source of these compounds is increasing, with an enormous unexploited resource yet to be explored (Blunt et al., 2013). Deep-sea canyons are a potentially rich source of genetic resources because they have high abundances of cnidarians and sponges (see Section Canyon effects on benthic sessile fauna and infauna). Finally, the potential monetary benefits of offshore drilling for hydrocarbons are large. Although this industry has not yet developed in submarine canyons, the oil and gas industry has started to explore options for development in these areas (see Section Oil and gas exploitation for more details).
Cultural Services
Cultural services are non-material benefits provided by the esthetic, educational, scientific, artistic, and recreational aspects of the ecosystem (Armstrong et al., 2012; Thurber et al., 2014). As part of the deep sea, the remoteness of submarine canyons and our relatively limited knowledge of their faunal communities greatly increase their interest and fascination. The mysteries largely hidden in the deep ocean fuel the imagination of civil society, including artists and scientists. Considerable amounts of funding are being invested in research to increase the understanding of canyon systems and their ecological function, followed by the promotion of awareness to promote canyon stewardship (Strang and Tran, 2010; Thurber et al., 2014). In the last 10 years, several national and international science projects fully or partially focusing on submarine canyons have been funded (e.g., the HERMES and HERMIONE projects were supported with 15.5 M euro and 8 M euro, respectively, by the European Commission seventh Framework Programme (FP7). Fascination with the deep-sea realm is not recent, as shown by the internationally renowned adventures aboard the submarine Nautilus in Twenty Thousand Leagues Under the Sea by Jules Verne (1870). However, current technology provides opportunities for civil society to explore, remotely, regions of the planet that were not accessible to most people until recently. Cabled observatories, such as Ocean Network Canada's in Barkley Canyon, provide a window for the public into the deep sea by offering online, real-time views of the canyon seabed. Similarly, ROV cruises with real-time video streaming online (e.g., as provided by NOAA Office of Exploration and Research and the Ocean Exploration Trust), help to provide this cultural service to a wide public. Through films (e.g., Blue Planet series, BBC), books (e.g., Deeper than Light by Baker et al., 2011, The Deep by Claire Nouvian), exhibitions (e.g., Deeper than Light produced by the MAR-ECO and other deep-sea Census of Marine Life projects; The Deep, produced by C. Nouvian), and online platforms (e.g., Oceans Network Canada—Learning; telepresence-coverage of expeditions on NOAA ship Okeanos Explorer), it is possible for many people to gain a better understanding and appreciation of the deep sea, with its fascinating habitats and life forms. Other “cultural” services, although not available in all canyons, are whale-watching tourism (e.g., Kaikoura Canyon, off New Zealand) and surfing competitions (Nazaré Canyon, off Portugal). The increase in awareness about the deep sea and canyon ecosystems, including their value and the current and potential impacts they face, will likely result in an increased and significant social demand for management and conservation measures. Attempts have been made to assess public perceptions of the value of deep-sea ecosystems. A choice experiment showed that the Irish public would be willing to endorse a trawling ban in all areas where corals are thought to exist, and pay a personal tax of 1 euro per year in order to protect these habitats (Wattage et al., 2011).
Human Activities Impacting Canyon Ecosystems
Five major sources of impacts are threatening submarine canyon ecosystems: fishing; oil and gas exploration and exploitation; pollution, including marine litter, chemical pollution, and submarine disposal of tailings produced by land-mine activities; ocean acidification; and climate change-related stressors (Levin and Le Bris, 2015).
Direct and Indirect Effects of Fishing
Among the human activities that can severely impact the deep-sea floor and associated biological communities, bottom trawling is arguably one of the main concerns, because of its physical impact, geographical extension, and recurrence (e.g., Jones, 1992; Benn et al., 2010; Ramirez-Llodra et al., 2011; Puig et al., 2012; Clark et al., 2015). Submarine canyons are not an exception and the enhanced presence of marine life in and around some canyons results in these ecosystems increasingly being targeted by commercial fisheries, including bottom trawling and dredging. As part of the general offshore expansion of bottom-trawling fleets during the last decades, the rims of submarine canyons, from the shelf edge down to mid-slope depths, have been increasingly targeted (Martín et al., 2014b). Large quantities of orange roughy (Hoplostethus atlanticus), black scabbardfish (Aphanopus carbo), oreos (e.g., Pseudocyttus maculatus) and various macrourid (e.g., Coryphaenoides rupestris) species have been exploited from deep-sea habitats. Deep-sea fishes tend to have intrinsically low growth rates and low fecundity, meaning that sustainable exploitation requires a very low catch rate (Norse et al., 2012; Clark et al., 2014). Furthermore, there is evidence that fishing in these ecosystems may cause substantial damage to the fragile, long-lived, sessile fauna such as structure-forming corals (Foley et al., 2011) and sponges. As a consequence, this exploitation has been compared to mining activities, because the resource is considered non-renewable on the scale of reasonable human lifespan (e.g., Clark et al., 2010; Armstrong et al., 2012).
In the NW Mediterranean Sea, an intensive fishery targeting the red shrimp Aristeus antennatus, has taken place for over six decades along the upper slope and around the deeply incised local canyons on the margin (Tobar and Sardà, 1987). The decrease of the yield per recruit, CPUE (catch per unit effort) and mean individual length over the last 20 years is probably a symptom of population changes induced by the intense exploitation (Gorelli et al., 2016). In addition to the impact on the biological communities, studies conducted in this area, within La Fonera (or Palamós) Canyon, have revealed that the trawling gear passing near and along the canyon flanks down to 800m depth significantly impacts the seafloor (Puig et al., 2012). Trawl gear produces extensive sediment resuspension (Figure 4), erosion, organic carbon impoverishment, and ultimately, results in enduring changes to seafloor morphology at the spatial scale of the entire continental margin (Puig et al., 2012; Martín et al., 2014a). Sañé et al. (2013) identified a loss in the bioavailable content of organic matter, mainly amino acids, along the trawled flanks of La Fonera Canyon, while Pusceddu et al. (2014) documented notable ecological consequences of intensive trawling. These authors found that trawling, by continuously stirring the soft sediment of seabed over the years, has led to an 80% decrease in abundance and 50% reduction in the biodiversity of meiofauna. Additionally, nematode species richness decreased by 25%, when compared to similar areas where no trawling occurs. Data also revealed that trawled sediments are impoverished (over 50% reduction) in organic matter content and have lower rates of carbon degradation (about 40%). These results suggest that continued deep-sea trawling represents a global threat to seafloor biodiversity and ecological health of submarine canyons, causing effects on their flanks similar to those resulting from agricultural plowing and human-accelerated soil erosion on land (Puig et al., 2012; Pusceddu et al., 2014). Moreover, the impacts of trawling-induced resuspension of sediments are not restricted to fishing grounds, since re-suspended sediments are advected from trawled areas toward greater depths, concentrated within nepheloid layers, and deposited into canyons through sediment-laden density flows triggered by trawling gear along steep canyon flanks (Palanques et al., 2006; Puig et al., 2012; Martín et al., 2014c). Martín et al. (2008) through radionuclide dating of a sediment core collected at 1750m depth in the axis of La Fonera Canyon (NW Mediterranean), documented a doubling of the sediment accumulation rate in the 1970s, coincident with the rapid industrialization of the local trawling fleet. Puig et al. (2015) revisited the same canyon area a decade later and confirmed the two fold increase in the sedimentation rates during the 1970s, but also suggest that the accumulation rate during the last decade could be greater than expected, approaching ~2.4 cm y−1 (compared to ~0.25 cm y−1 pre-1970s). No submarine canyon in the world has been studied as intensively as La Fonera Canyon for the effects of bottom fishing gear, but given that canyons are often targeted by fisheries, it is likely that similar and other impacts have occurred and are occurring in other canyons elsewhere in the world. In Whittard Canyon (NE Atlantic), unusual peaks in nepheloid layers with much higher concentrations of suspended particulate matter (~1–8 mg L−1) than normal (~0.075–0.5 mg L−1) have been observed (Wilson et al., 2015b). Using VMS (Vessel Monitoring System) data from fishing vessels at Whittard Canyon, these peaks were linked to trawling activity (Wilson et al., 2015a). The direct and indirect impacts of trawling can affect cold-water corals and sponge fields. These two taxonomic groups are considered highly vulnerable, because they are usually slow-growing (up to 4–10mm a year; e.g., Roberts, 2009 and references herein), long-lived and sessile, and thus very fragile and easily disturbed. Vulnerable Marine Ecosystems (VMEs), such as coral (e.g., L. pertusa) reefs and deep-sea sponge aggregations that can occur in canyons, are recognized as habitats in need of protective measures by several international organizations (e.g., OSPAR, ICES, and FAO). For VMEs threatened by trawling on continental slopes, the complex morphology of canyons might offer the last refuge (Huvenne et al., 2011). Fabri et al. (2014), for example, suggested that in the NW Mediterranean Sea, most current dense aggregations of the gorgonian Isidella elongata occur on the steep slopes of canyons unreachable to trawling. However, the full extent of the impact of trawling gear on non-target benthic fauna such as corals is unknown. Although baseline ecological data of the “pristine” ecosystem is often not available, long-term studies of certain areas and landing and discards data from fisheries provide information on the changes taking place in benthic communities. For example, Company et al. (2012) suggest that there is a canyon effect on the community structure of the benthic megafauna of Blanes Canyon (NW Mediterranean), but that differing fishing pressures targeting the red shrimp A. antennatus on the margin and in the canyon may modulate the observed patterns. Trawling effects are also implicated in inducing changes to the trophic structure of benthic communities. The results of a comparative study of the benthic community on two margins (including canyons) affected by differing fishing pressures showed a predominance of mobile-predator scavengers and decrease of suspension-, filter-, and deposit-feeders in the areas of higher trawling intensity, compared with other areas where the trawling intensity was lower (Bowden et al., 2016).
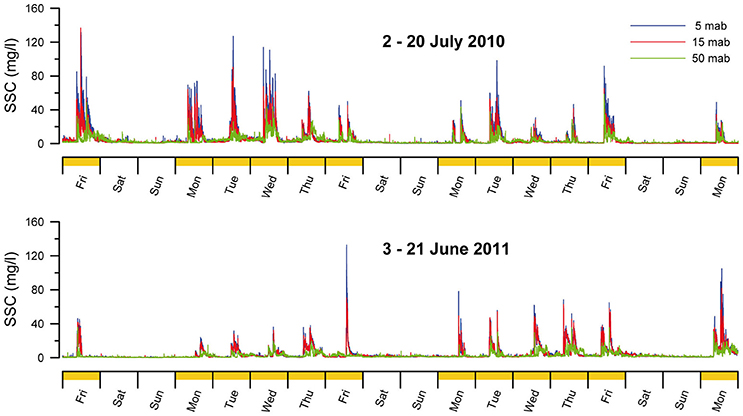
Figure 4. Time series observations of trawling-induced resuspension events recorded at the northern flank of La Fonera Canyon during two different years. Note that suspended sediment concentration (SSC) peaks measured at different heights above the sea floor (mab: meters above bottom) were observed during weekdays (that is, working days for the fishing fleet illustrated as orange bars on the x axes), but not on weekends. Plots created from data reported in Puig et al. (2012) and Martín et al. (2014c).
Longline fisheries also occur in canyon systems (e.g., sablefish Anoplopoma fimbria and groupers Epinephelus sp). Although this gear does not cause as much damage as trawling, when placed over coral habitats, the line can become entangled with the coral thus damaging the colonies during gear recovery (Orejas et al., 2009; Fabri et al., 2014). Additionally, ghost fishing is a well-reported problem related to lose gillnets or traps which continue to catch fish and damage other species.
Oil and Gas Exploitation
Submarine canyons coincide with some areas targeted by the offshore oil and gas industry for exploration and development opportunities. Examples include potential economic deposits located off West Africa (Jobe et al., 2011) and the Campos Basin located on the continental slope of Brazil, which is currently under production by PETROBRAS and other companies (Gorini et al., 1998; Day, 2002). In Australia, an assessment was made by Harris et al. (2007) of the seafloor features most characteristic of offshore oil and gas leases. Surprisingly, nearly 24% of Australian submarine canyons occur in oil and gas leases even though such leases cover only 8.7% of Australia's EEZ (Exclusive Economic Zone). Thus, it would appear that, at least for Australia, submarine canyon habitats are positively selected for with regard to oil and gas leases, in comparison to other seafloor geomorphic features.
Over the last 10 years, several studies have focused on the effects of exploratory offshore drilling in response to the growing interest by the oil and gas industry to move operations further offshore (Currie and Isaacs, 2005; Ellis et al., 2012; Bakke et al., 2013; Paine et al., 2014). This offshore shift is especially true for countries such as the United Kingdom, Norway, Italy, Malaysia, Indonesia, and Australia, which number among the leading oil-producing countries (Patin, 1999). Results from these and previous studies suggest that discharge of drilling waste is the primary environmental concern of oil and gas drilling operations. Drilling discharges are composed primarily of dense particulate solids that settle rapidly and accumulate in sediments down-current from the platform (Bakke et al., 2013). The rapid accumulation of these solids in the sediments has caused changes in benthic community composition (loss of rare species and increase in abundance of pollution tolerant species), decrease in abundances and diversity of communities and decrease in coral coverage among others (reviewed in Ellis et al., 2012). The effects of drilling discharge can also affect the biology, particularly growth and reproduction, of megafaunal species. For example, bentonite and barite particles may cause changes in reproduction and growth of scallops (Cranford, 1995). However, other studies indicate that the effects of elements incorporated in discharge are mainly a physical stress, while chemical toxicity is not always described (Bakke et al., 2013). Although studies specific to submarine canyons have not been conducted, the effects of drilling waste on benthic and demersal species is highly dependent on a number of local environmental variables (e.g., depth, current, and wave regimes, substrate type; Ellis et al., 2012). Thus, it is expected that similar or even greater effects in canyons because of the presence of VMEs (e.g., coral reefs) and potentially restricted movement of pollutants due to current regimes in canyons. Experimental and monitoring studies based on the exposure of L. pertusa to drill cuttings from oil drilling activities on the Norwegian continental shelf indicate that corals polyps tolerate well the enhanced particle deposition rates and suspended matter concentrations (Allers et al., 2013; Larsson et al., 2013; Purser, 2015). However, a small pilot experiment indicated that coral larvae might be particularly vulnerable to high particle concentrations (Larsson et al., 2013). The effect of accidental oil spills from deep offshore drilling (e.g., Deepwater Horizon oil spill in the Gulf of Mexico, White et al., 2012) could also be more severe given the physical and hydrodynamic nature of submarine canyons.
Canyons As Sinks for Marine Litter and Chemical Pollution
The London Convention (1972) and London Protocol (1996) legally banned the dumping of litter from ships. However, litter occurs in all marine habitats, from the intertidal to abyssal plains, entering the marine system from the coast (rivers, beaches) and through illegal dumping (Galgani et al., 2000; Ramirez-Llodra et al., 2011; Bergmann et al., 2015). Although dedicated and opportunistic studies on deep-sea marine litter have increased in the last decade, knowledge is still sparse. However, the data obtained to date from regional, as well as international, large-scale investigations, suggest that canyons are a major habitat for the accumulation of litter (Figure 5). The specific hydrographic patterns and increased downslope currents in submarine canyons result in canyons becoming hotspots of marine litter (Galgani et al., 1996, 2010; Mordecai et al., 2011; Ramirez-Llodra et al., 2013; Schlining et al., 2013; Pham et al., 2014; Bergmann et al., 2015; Tubau et al., 2015; Van den Beld et al., in press). A study comparing the accumulation of marine litter in different deep-sea habitats across Europe showed that litter densities in canyons were higher than in other physiographic settings, such as continental shelves, seamounts, banks, and mounds (Pham et al., 2014). In the Mediterranean, a study of marine litter on the slope, deep basin and canyons indicated that canyons preferentially accumulate light debris (e.g., plastic), which is transported downslope from the shelf in highly populated coastal regions (Ramirez-Llodra et al., 2013). In Monterey Canyon (E Pacific), heavier litter tends to accumulate in high-relief outcrops or depressions, while soft plastics accumulate in areas where structure-forming corals provide relief on the seafloor (Figure 5, Schlining et al., 2013; Van den Beld et al., in press). The effects of litter on the benthic fauna are little understood. However, impacts such as suffocation, physical damage of sessile fauna (e.g., corals, sponges, crinoids) and ghost fishing from discarded or lost fishing gear have been observed (Ramirez-Llodra et al., 2013; Pham et al., 2014; Bergmann et al., 2015). The degradation of plastics into microplastics and their transport in the water column and accumulation in the sediments provides an additional source of concern (Thompson et al., 2009; Bergmann et al., 2015). These microplastics are potential sources of toxic substances (e.g., persistent organic pollutants) and if ingested, may have lethal or sub-lethal effects on the fauna as well as pose a threat to human health through their bio-accumulation in the marine food web (Thompson et al., 2009; Lusher, 2015; Rochman, 2015).
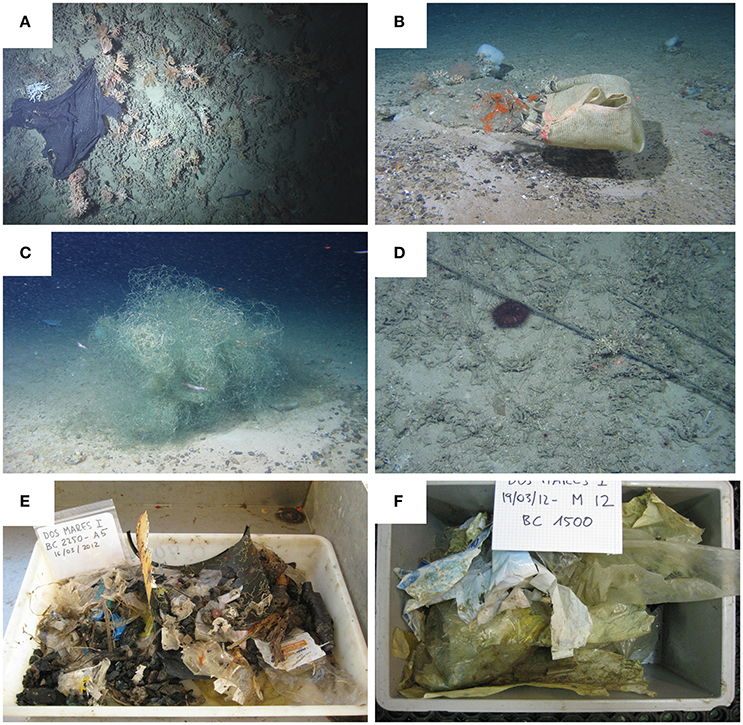
Figure 5. Images of marine litter found in different submarine canyons. (A) A plastic sheet in a cold-water coral community in a Bay of Biscay canyon. Copyright: Ifremer, BobEco cruise 2011. (B) Coral damaged by trawling and trawl lines in unnamed northern canyon at 700m. CE1004 cruise. (C) Remains of fishing gear over corals and other benthic fauna on Porcupine Bank. (D) Remains of longlines in an unnamed canyon north of the Porcupine Bank approx 1400m (NE Atlantic). CE13008 cruise. Copyright NUI Galway / Marine Institute. (E,F) Examples of marine litter collected at Blanes submarine canyon (NW Mediterranean) at 2250m (E) and 1500m (F), respectively. Copyright: ICM-CSIC, DOSMARES cruise 2013.
Investigation of chemical contamination of deep-sea ecosystems is increasing. Results show that chemicals are accumulating in deep-sea sediments, benthos, and mid-water fauna (Asante et al., 2008). The major contaminants of concern are persistent organic pollutants (POPs), toxic metals (e.g., Hg, Cd, Pb, Ni, and isotopic tracers), radioelements, pesticides, herbicides, and pharmaceuticals. The hydrodynamics of canyons favor the quick movement of labile material to the lower slope, where local benthic environments might become sinks of pollutants (Puig et al., 1999; Looser et al., 2000; Palanques et al., 2008; Castro-Jiménez et al., 2013; Cossa et al., 2014; Sanchez-Vidal et al., 2015). In the NW Mediterranean, general accumulation patterns indicate that organisms collected inside Blanes Canyon had higher concentrations of POPs than individuals collected on the adjacent open slope (Koenig et al., 2013). These inputs could be the consequence of enhanced vertical transport of hydrophobic particles associated with chemical pollutants, the dispersal of which is driven by local hydrodynamic processes that regulate flux and sediment re-suspension patterns (Koenig et al., 2013).
Canyons as Areas for Mine Tailing Disposal
Land-based mining produces large volumes of waste in the form of non-processed overburden rock and processed fine particulate tailings. The tailings (fine-fraction slurry) usually account for a very high proportion of the ore (e.g., 99% for copper and 99.99% for gold, MMSD, 2002). Some tailings can, additionally, include process chemicals (e.g., floatation or flocculation chemicals) and heavy metals, and in some cases, very sharp particles resulting from the crushing process (Lake and Hinch, 1999). Although most mines use conventional land-based damns to store tailings, there is an increasing interest in submarine tailing disposal (STD), including deep-sea tailing placement (DSTP) (reviewed in Ramirez-Llodra et al., 2015). DSTP occurs where a pipe is submerged below the mixing layer (>100m depth), and where a turbidity flow is created that transports the tailings down to the deep seafloor. The environmental impacts of DSTP can include: (1) smothering of the benthic ecosystem in the deposition area through hyper-sedimentation; (2) chemical toxicity from metals or processing chemicals; (3) modified organic matter content and porosity of sediments (grain size and angularity) that can affect feeding and recolonization; (4) formation of sediment plumes and increased turbidity which may impact pelagic organisms; and (5) risk of slope failure and re-suspension of tailings (Ramirez-Llodra et al., 2015). Currently, seven mines across the world conduct DSTP, three in Papua New Guinea, one in Indonesia, one in France, one in Greece, and one in Turkey. When considering DSTP, canyons are often proposed as preferential locations because of their natural capacity to act as conduits to deep basins. For example, the Ramu Nickel Project in Papua New Guinea discharges tailings at 150m into Basmuk Canyon, with a final deposition at 1500m (Shimmield et al., 2010). The Indonesian Batu Hijau copper and gold mine has discharged its tailings at 125 m depth into Senunu Canyon since 2000, with final deposition between 3000 and 4000m depth (Shimmield et al., 2010; Reichelt-Brushett, 2012). This DSTP is the deepest deposition of tailings in the world. In France, the alumina plants in the Marseilles area (NW Mediterranean) that process bauxite have discharged the red-mud residues produced by this process into Cassidaigne Canyon since 1967. The pipe is situated at 330m depth and the red-mud deposit extends more than 50 km from the outflow into the abyssal plain (Dauvin, 2010; Fontanier et al., 2012; Fabri et al., 2014). Excess concentrations of iron, titanium, vanadium, and chromium are recorded on the seafloor (Fontanier et al., 2012). In situ observations of sessile fauna (e.g., gorgonians) covered by the red mud show signs of tissue necrosis, which indicate that there is a negative, or even lethal, effect of the accumulation of red mud on the megafauna (Fabri et al., 2014). Nevertheless, settlement of new coral colonies has also been observed, perhaps in response to the decrease in red-mud outflow since 1988, when one company stopped discharging tailings (Fabri et al., 2014).
Governance and Management of Submarine Canyons
There is a range of legal and institutional frameworks for governing and implementing the conservation and sustainable use of marine systems. Despite the complexity of canyon management and conservation, in recent decades, some of these frameworks have been used to instigate conservation and management measures in different regions, and new frameworks are being proposed.
Legal Frameworks and Other Tools for Canyon Conservation
In 2008, the 9th meeting of the Conference of the Parties to the Convention on Biological Diversity (CBD, 2008, COP9) adopted seven scientific criteria for the identification of Ecologically or Biologically Significant Marine Areas (EBSAs) (annex I, decision IX/20); these are, (1) uniqueness or rarity, (2) special importance for life-history stages of species, (3) importance for threatened, endangered or declining species and/or habitats, (4) vulnerability, fragility, sensitivity, or slow recovery of ecosystems, (5) biological productivity, (6) biological diversity and (7) naturalness. Under these criteria and based on the specific characteristics of canyons, the CBD suggested that submarine canyons could be classified as priority areas for conservation on many continental margins areas (Davies et al., 2014). Similarly, the United Nations Food and Agricultural Organization (FAO, 2009) adopted similar criteria to identify VMEs. The VME concept has gained prominence following a resolution of the United Nations General Assembly (UNGA, resolution.61/105) to seek the protection of VMEs on the high seas and in areas of national jurisdiction (Weaver et al., 2011). Examples of VMEs include canyon habitats, as well as the coral reef and sponge aggregations that are sometimes found in canyons (FAO, 2009). These two conservation initiatives, as well as other international initiatives, are providing a platform for Regional Fisheries Management Organizations (RFMOs), Regional Seas Conventions and Action Plans (RSCAPs), and other proposals developed in the past 10 years (Jobstvogt et al., 2014) to provide protection for species and habitats that are important for sustainable marine ecosystems. The aim is to strengthen cooperative work in the development of a common roadmap under the coordination of the United Nations Environment Program (UNEP-MAP-RAC/SPA, 2010). Canyon systems, by their association with continental margins, are more often found in EEZs, where the concepts of EBSAs and VMEs are neither always nor consistently applied by nation states. Nonetheless VMEs have been identified in canyons on the Mediterranean margin (Fabri et al., 2014). In Europe, the Oslo-Paris Convention (OSPAR) from 2005 provides a legal mechanism in the northeast Atlantic area to protect several deep-sea habitats, including those submarine canyon habitats where cold-water scleractinian reefs (i.e., dominated by L. pertusa) are present (OSPAR, 2005).
Most submarine canyons are located within (EEZs) (Huang et al., 2014), which gives individual nation states the authority to control all extractive activities in their area (e.g., fisheries, oil and gas), thereby these states potentially offer protection to canyons through conservation and management measures such as Marine Protected Areas (MPAs) or fisheries management tools such as spatial/temporal closures of areas or gear restrictions. However, international agreements concerning the rights of free navigation must also be acknowledged in the development of management measures that include such area-based protection regimes. The legal framework of marine environmental protection is complex given the different levels of legislation (international, national, and regional) and varies widely depending on the countries involved (Camuffo et al., 2011). The complexity of governance depends mainly on the canyon's location. In the case of a cross border location such as Capbreton Canyon (France/Spain, Bay of Biscay) or a large area of international waters such as in the Mediterranean region, the regional disparities in governance and institutional structures make it difficult to find agreements to ensure a sustainable management of ecosystems (De Juan et al., 2012). States have no mechanisms to create globally-recognized MPAs and reserves on the high seas. Instead, there is a patchwork of bodies, including RFMO that set policies for specific areas of the ocean or specific activities (such as fishing for tuna). However, those bodies lack the legal mandate necessary to establish MPAs. Nevertheless, there are several examples of areas where progress has been made in the protection of canyon habitats (Table 1; Figure 6).
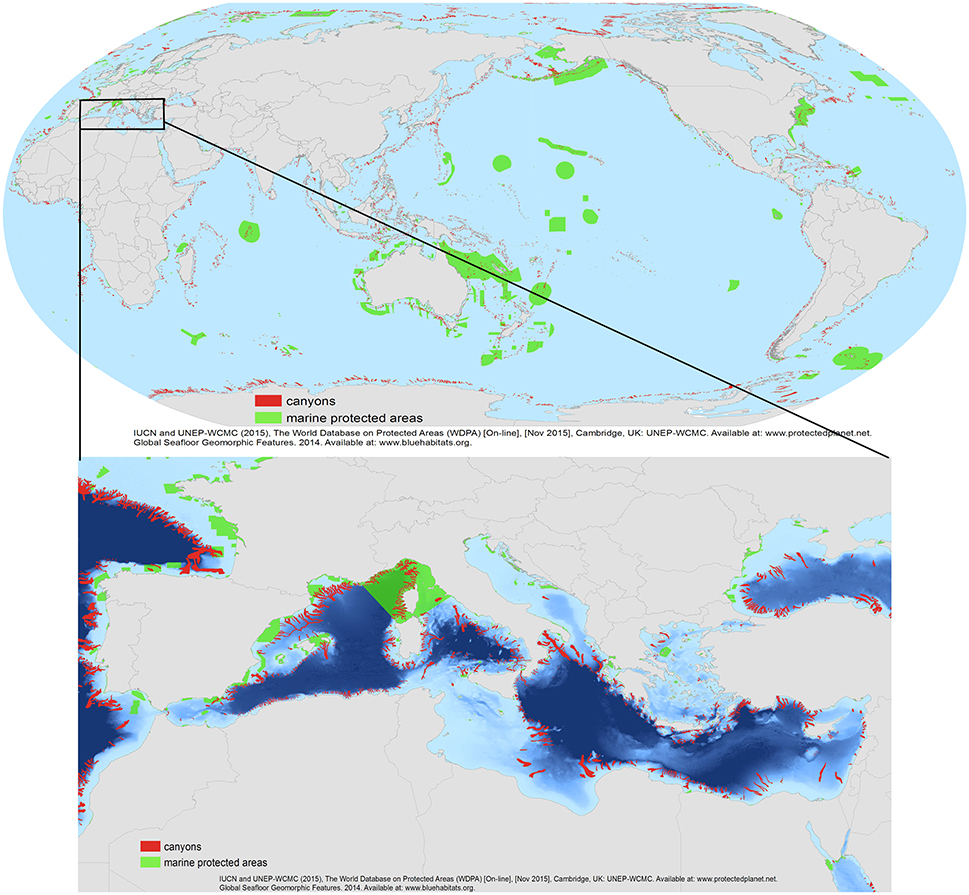
Figure 6. The global map showing submarine canyon distribution (in red) and Marine Protected Areas (MPAs) (in green). Below, a detailed map with the Mediterranean distribution of canyons in relation to MPAs. Data sources: Based on previously published data of the World Database of Protected Areas (WDPA, IUCN, and UNEP-WCMC, 2013) http://www.protectplanetocean.org/official_mpa_map and the Global Seafloor Geomorphic Features Map by (Harris et al., 2014).
Examples of Current Canyon Conservation and Management
In Canada, The Gully MPA, located 200 km offshore Nova Scotia, was created in 2004 under Canada's Ocean Act. The Gully MPA, covering an area of 2364 km2, includes three zones of protection based on conservation, management and research objectives (DFO, 2008; Westhead et al., 2012). The Gully Advisory Committee, composed of representatives from academics, industry and non-government organizations, provides advice and information to the government on the MPA and related activities (DFO, 2008; Westhead et al., 2012). In the Northeastern United States of America (USA), in addition to a network of habitat closed areas implemented by Regional Fisheries Management Councils (RFMCs), the New England Fishery Management Council (NEFMC), and Mid-Atlantic Fishery Management Council (MAFMC), added two canyon habitat closures (Oceanographer and Lydonia canyons) within Amendment 2 of the Monkfish Fishery Management Plan. These closures were implemented as a precautionary approach to prevent impacts from the development of an offshore monkfish (Lophius sp.) fishery on Essential Fish Habitats (EFH) and deep-sea canyon habitats (Packer et al., 2007; Marin and Aguilar, 2012). Another example of fisheries management decisions benefiting preservation of canyon habitats is also in the western North Atlantic, off the east coast of the USA. Conservation measures designed to protect vulnerable marine species, such as deep-sea corals that occur in canyons provide a level of protection to the canyon as a whole. The MAFMC has approved the deep-sea corals amendment to protect known or likely coral habitat while limiting negative impacts to commercial fisheries operating in the region. Magnuson-Stevens Fishery Conservation and Management Reauthorization Act (Public Law 109–479) gives the RFMCs the authority to designate zones where, and periods when, fishing may be restricted in order to protect deep-sea corals from the impacts of fishing gear. The MAFMC considered various alternatives, and two spatially overlapping coral protection zones were selected as preferred options. The broad coral zone prohibits fishing in regional waters at depths of 450m and greater, out to the limit of the EEZ. Discrete coral zones define specific areas where corals are known to occur or are highly likely to occur based on results of a habitat suitability model. The boundaries of most discrete zones outline large portions of submarine canyons. Under this amendment, all bottom-tending types of gear used in federally managed fisheries are prohibited in and around deep-sea coral habitat (i.e., canyons), with the exception of the deep-sea red crab (Chaceon quinquedens) trap fishery. This recent management recommendation in the western North Atlantic, if approved, will protect approximately 99,000 km2 of seafloor in the Mid-Atlantic region (East coast of the USA, Virginia to New York), including 15 canyons. Final approval is expected in late 2016. A similar situation can be found on the Eastern margin of the Atlantic, where Explorer and Dangeard canyons are part of a United Kingdom Marine Conservation Zone (MCZ). This conservation measure was put in place with the specific aim of protecting cold-water coral reefs, but as a result large parts of the canyons are protected.
In the Mediterranean Sea, effective governance of canyon systems requires cooperation at various levels, ranging from international conventions to regional initiatives for environmental protection (Cinnirella et al., 2014). The European Council has adopted management plans for specific Mediterranean fisheries, in areas totally or partially beyond the territorial seas (including high seas) and affecting canyon habitats. Such plans include the prohibition of fishing with trawl nets and dredges in certain areas, and at depths below 1000m (EC Regulation 1967/2006). The Regional Activity Centre for Specially Protected Areas of Mediterranean Importance (SPAMI), under the Barcelona Convention agreements, has set up the legal framework to conserve marine habitats including submarine canyons (RAC/SPA, 2010). In the French part of the Mediterranean basin, three MPAs have been created to protect cold-water corals: the “Parc Marin du Golfe du Lion” (decree 2011-1269) including Lacaze-Duthiers, Pruvot and Bourcart canyons; the “Parc National des Calanques” (decree 2012-507) including Cassidaigne Canyon with specific regulations; and the “Parc National de Port-Cros”(decree 2012-649) with extension of the adjacent bathyal seafloor (Fabri et al., 2014, Table 1). In addition, the General Fisheries Commission for the Mediterranean (GFCM) established a fishing-restricted area off the French coast in the Gulf of Lion, including Montpellier, Petit-Rhône, and Grand-Rhône canyons in 2009 for the Mediterranean (Marin and Aguilar, 2012; Fabri et al., 2014). This directive involved the regulation of certain demersal fishing gears and aimed to protect spawning aggregations and deep-sea sensitive habitats (GFCM, directive no. 33/2009/1, IUCN and UNEP-WCMC, 2016). In Spain, Protected Areas, Marine Reserves and Marine Reserves of Fishing Interest have been established, but these protected areas include only a few submarine canyons.
In 2012, the International Union for Conservation of Nature (IUCN) published a book on Mediterranean submarine canyon ecology and governance (Würtz, 2012), which was based on the conclusions of several workshops focusing on Mediterranean governance. It was recommended to Mediterranean countries that a precautionary principle be applied to the canyons under their jurisdiction, and to include canyons in national, regional and international strategies for MPAs. The increasing role of non-governmental organizations (NGOs) in calling for improvement in canyon management has resulted in investigations of the most vulnerable submarine canyons, and in some cases protection measures have been proposed (Marin and Aguilar, 2012). For example, in 2011, Oceana (i.e., nonprofit international organization focused solely on the protection of the oceans, http://eu.oceana.org/en/about-us) published a new proposal for the protection of the Mediterranean vulnerable areas called MedNet. This proposal contained 28 submarine canyons (e.g., Bejaia Canyon, Algeria; Gulf of Lion submarine canyons, France and Spain; Bari Canyon, Italy amongst others) and included a detailed review of their main characteristics and current status of conservation initiatives (Marin and Aguilar, 2012). Some of these proposed canyons (e.g., Cap de Creus Canyon and Lacaze-Duthiers Canyon) have now been classified as Sites of Community Importance (SCI) under the EU Habitats Directive.
Although progress has been made to combine both marine conservation and fisheries management, it remains fragile due to disparities in regional governance and institutional structures between countries (De Juan et al., 2012). These authors highlight the complex jurisdictional situation of international waters in the Mediterranean Sea and mention the need for cooperation between coastal states with a regional operational strategy to achieve a sustainable management of ecosystems. The case of Capbreton canyon in the Bay of Biscay (NE Atlantic) provides an example of the difficulties that cooperation must overcome. Capbreton canyon extends from French territorial waters into the EEZ of both France and Spain, and is regulated through several management measures. In the French part, these measures were first established in 1985 (Ord. n°40 1985) at the request of local French fishermen and included fishing restricted areas for gillnets, and subsequent expansion to include restriction areas for pelagic and benthic trawlers (Sanchez et al., 2013). Most of the restricted area lies within the French Territorial Sea, but part extends into the French EEZ. The French restrictions in the EEZ area, however, do not apply to foreign vessels. The extension of such an area into the EEZ raises the questions of legality, enforcement, and monitoring for scientific purposes (Sanchez et al., 2013). Additionally, the cross border location between France and Spain of Capbreton Canyon makes fisheries management even more complicated, making agreements on trans-boundary cooperation difficult. Proposals for a management plan of a “Capbreton case study” were discussed between stakeholders during European project GEPETO (http://gepetoproject.eu/), which aimed to improve future fisheries management in the south European Atlantic coast region (Uriarte et al., 2014).
Global Submarine Canyon Protection Status
A recent review of global seafloor geomorphic features, based on the analysis and interpretation of a modified version of the SRTM30_PLUS global bathymetry grid (Becker et al., 2009), included a new assessment of submarine canyons and associated geomorphic statistics (Harris et al., 2014). The database that underpins the analysis of Harris et al. (2014) provides the information needed to estimate the area of canyons within each country's EEZ as well as the area of canyon currently protected within MPAs. Here, we provide an analysis using ArcGIS ESRI tools on the proportion of canyons included in MPAs at the global scale (Figure 6; Table 1). Statistics included the area estimates for 9477 individual canyons covering a total area of 4,393,650 km2 (i.e., 1.2% of the total ocean area).
In order to estimate the area of canyons within EEZs (i.e., canyon area that can be protected within the jurisdiction of individual countries, as opposed to those located in the high seas or areas beyond national jurisdiction), global EEZ boundaries downloaded from the VLIZ database were used. In addition, a summary of global MPA boundaries was downloaded from the IUCN and UNEP-WCMC database (IUCN and UNEP-WCMC, 2016) and used to calculate the areas of canyons within MPAs. Results showed that of the overall 4,037,764.35 km2 of seafloor found in submarine canyons in EEZs (91.9% of canyon area), 13.6% of canyon areas are protected (with at least 10% of their area) within an MPA. Only 10.3% of all canyons are completely protected (100% of their area) within an MPA (Table 2, annex I).
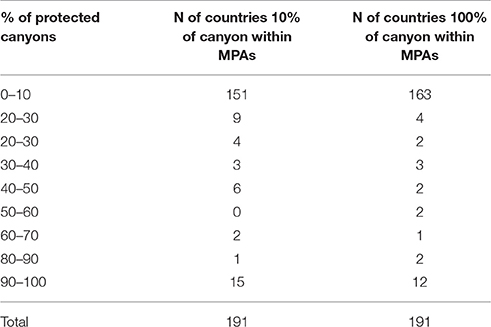
Table 2. Summarizes the total number (N) of countries/jurisdiction with at least 100 and 10% of their canyon area within MPAs (for more details see Annex 1).
This exercise identified 1956 canyons within MPAs (Table 2, annex I). Of the 191 countries/jurisdiction with submarine canyon(s) in their waters, 163 countries (83% of the total) have less than 10% of their submarine canyon(s) protected within an MPA. Only 12 countries (6.3%) have 100% of their submarine canyons covered by MPA protection. From these last 12 countries/jurisdictions, 10 are overseas territories of France and the United Kingdom (e.g., British Indian Ocean, Glorioso Island, Ile Europe, see Annex for more detail) while the other two countries (i.e., Dijibouti and the Democratic Republic of Congo) have protected the only canyon they have in their waters (Annex 1). In terms of numbers, the United States and New Zealand protected the highest number of canyons (117 and 54 respectively). Indonesia and the Philippines had the highest number of canyons within their EEZs (576 and 265 from which 20 and nine were protected respectively, Annex 1). Notably, these results only reflect those canyons protected by management provided under MPA authority. However, there are other mechanisms in place that offer other protective measures (i.e., world heritage area marine park and fisheries management areas). Yet, no standardized world database exists that includes all protected canyon areas. Therefore, some level of worldwide synthesis, although difficult to achieve, is needed to assess the conservation actions undertaken for canyon protection. The data (see detailed Figure 6 of the Mediterranean Sea) also reveal that most of the marine conservation measurements have focused on shelf ecosystems, while the vast majority of continental slope areas around the world (where submarine canyons are found) are still without any regulations to prevent them from pollution and human exploitation.
Directions for Future Canyon Research
Progress in submarine canyon research has provided considerable evidence demonstrating that canyons are seascape that host important ecosystems, and are in need of conservation. However, there are still many important scientific questions and unknowns, which hamper the development of effective conservation and management strategies for these important ecosystems.
Knowledge Gaps and Future Directions for Understanding Canyon Ecosystem Functioning and Services
Although the broad-scale physical and geological patterns and processes in submarine canyons have now been identified (e.g., the occurrence of turbidity currents, dense shelf water cascading, internal waves), their fine-scale effects and distributions are less well understood (e.g., their frequency, exact footprint, or sediment types affected). However, it is often those finer scale effects that directly influence species and communities, and a better understanding of these will lead to more robust decisions about community vulnerability, which canyon areas to prioritize for protection, and how to interpret species resilience against different types of disturbance. Small-scale effects within canyons can also influence ecosystem services, and many questions remained unanswered about these influences. For example, what is the exact effect of sediment flows and repeated resuspension on the carbon cycle within submarine canyons (as an ecosystem service)? What role does canyon geomorphology play in the determination of biodiversity and ecosystem functioning? Overall, to achieve this better resolution in our understanding, more canyons need to be studied, studies need to be long term (time-series), and studies need to be better coordinated. There is a need for more baseline surveys, using standardized methodologies and the most appropriate sampling schemes (Ayma et al., 2016). Also in general, better integration is needed across the different disciplines of submarine canyon studies, combining insights from sediment dynamics, oceanography and biogeochemistry with biological knowledge, to achieve a more holistic understanding of submarine canyon systems (e.g., Amaro et al., 2016).
Recent development of new technologies offers exciting new opportunities to answer some of the questions posed above, and to better integrate fields of study. Although the rugged topography of canyons has often limited ecological research due to restrictions of traditional sampling gear (e.g., trawling, coring), underwater technologies such as Autonomous Underwater Vehicles (AUVs), ROVs, and cabled observatories are providing new and sophisticated platforms to observe, sample and experiment in submarine canyons. Benthic multiparametric platforms endowed for oceanographic, geologic, and chemical sensors allow remote investigation of the canyon environment. When these sensors are associated with imaging and acoustic equipment, species presence can be related to particular states in the monitored environmental variables providing important data on community temporal dynamics (Aguzzi et al., 2012). In particular, key research can be to date focused on issues of relevance for canyon ecology at the largest cabled observatory network on the planet, as operated by Ocean Network Canada (ONC; http://www.oceannetworks.ca/). The North East Pacific Time-Series Experiment, (NEPTUNE) network deployed in Barkley Canyon allows to studyin situ rhythmic behavior and species tolerance in response to tidal and inertial fluxes (which has important implications on adult dispersal rates; Sbragaglia et al., 2015; Chatzievangelou et al., 2016), and to multiple climate change variables including methane, pH, pCO2, pO2, salinity, temperature, turbidity, chlorophyll-a (for primary production) (Purser et al., 2013). Such monitoring capability can be to date increased by endowing fixed networks of cabled monitoring platforms with semi-mobile rovers, tethered to the nodes, extending the spatial coverage from few m2 to few tens of m2 (Thomsen et al., 2012; Aguzzi et al., 2015). In the NW Mediterranean, the Operational Observatory of the Catalan Sea (OOCS; http://www2.ceab.csic.es/oceans/index_en.html) is a permanent pelagic buoy anchored at 200m depth at the head of Blanes Canyon (Bahamon et al., 2011). This multiparametric pelagic platform, which also hosts a meteorological station, provides important data from the surface down to approximately 50m depth on light intensity, chlorophyll, and productivity processes that influence the canyon trophic structure via atmosphere and water column (benthopelagic coupling mediated) interactions.
Addressing Knowledge Gaps for Human Impacts on Canyons
While it is becoming increasingly clear that human activities are impacting canyons, in order to set up effective mitigation strategies, the effects of different natural and anthropogenic impacts need to be better quantified. The studies that have reported on the impact of bottom trawling around La Fonera Canyon are excellent examples (e.g., Puig et al., 2012; Martín et al., 2014a,b; Pusceddu et al., 2014). But, similar work should be carried out around other canyons affected by bottom-trawling fisheries to obtain a more complete, and ideally global-scale, evaluation of the problem. In addition, the impact of other fishing techniques such as long-lining and fishing-related impacts such as ghost fishing, also need to be better quantified. Similarly, the impacts of litter, mine tailings, and chemical pollutants in canyons should be investigated on a wider scale. Should drilling for oil and gas in canyons become a reality, the potential impact of this activity will require particular consideration because of the difference between canyon habitats and the typically more open locations of current deep-water drilling activities.
Given the level of human impact already observed within some canyon systems, it would be beneficial to understand both the resistance and resilience of canyon communities. Decisions on which human activities require management measures in order to mitigate adverse impacts rely on understanding the impacts (both acute and chronic) of those activities, and the potential for affected communities to recover. There are very few data available on community recoverability in canyons. With the designation of a number of previously impacted canyons as MPAs comes a unique opportunity to monitor the recovery (or otherwise) of these systems.
Finally, climate change-related impacts require investigation. Climate change will affect submarine canyons, for example, through increases in water temperatures, change in current patterns, and ocean acidification. Using a variety of modeling approaches, the impact of future climate scenarios needs to be evaluated in order to inform management strategies for canyons. Questions to address include; what will be the effect of changing water column density on internal wave and tide generation within submarine canyons? How will canyon species change their distribution patterns in a warming ocean? And how sensitive will canyon coral communities be under a shoaling aragonite saturation horizon? Given our current limited understanding of species distribution patterns, connectivity and vulnerability within submarine canyons, these questions are challenging. However, their outcome may have important implications for conservation strategies in the longer term.
Knowledge Gaps that Influence the Effective Implementation of Conservation Policy
To enable effective conservation and ultimately management of submarine canyons, it is necessary to develop tools that allow environmental managers to make informed choices and prioritize areas in need of protection. One approach to this is through the use of a top-down classification system, where divisions can be made on the basis of useful surrogates for biological diversity (Howell, 2010). For example, biogeographical region, water mass structure, seabed topography/geomorphology, depth, and substratum (Greene et al., 1999; Allee et al., 2001; Butler et al., 2001). Figure 7 shows a complete biotope map for the SW Canyons submarine canyon system (UK), and illustrates the type of product needed by managers to make informed decisions about conservation efforts. The rational for this approach is that different physical classes represent different biological assemblages, and thus enable an assessment of the criterion of representativeness of an MPA network (Evans et al., 2015), a major criterion of assessment of network effectiveness recommended by IUCN guidelines for highly protected areas (IUCN, 1994). This approach can only be effective if the major physical drivers of biological diversity within canyon systems are understood and adequately represented by available physical data sets. Understanding the relative importance of different drivers and measuring them, is an area in which more research is needed—with a focus on comparing canyons with different physical environmental regimes in order to clarify the role of potential physical drivers in influencing differences in faunal communities and ecosystem function.
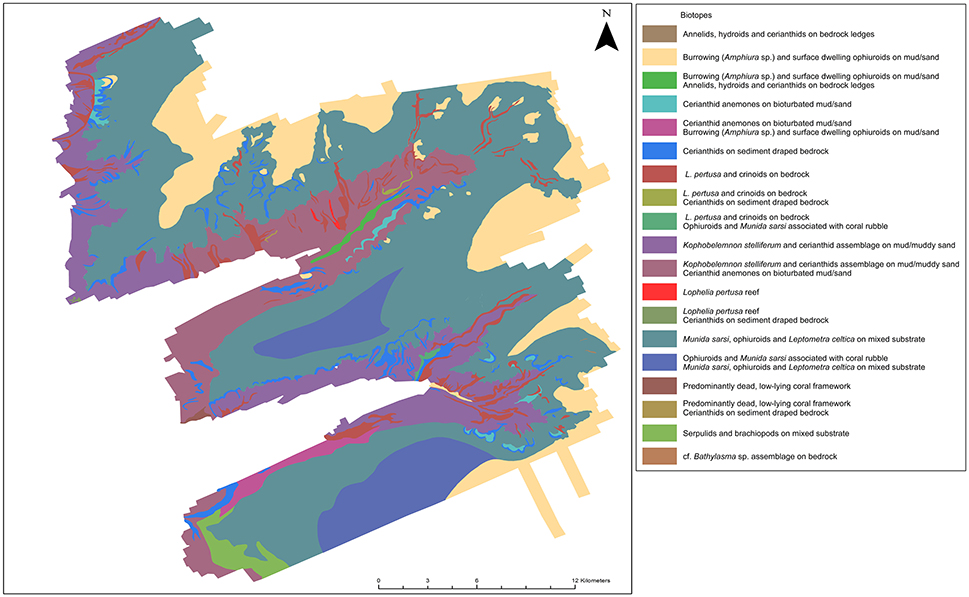
Figure 7. Full coverage biotope map for the SW Canyons submarine canyon system (UK; Davies, 2012). Polygons were produced from multibeam bathymetry, its derived layers, interpreted seabed substratum and geomorphology. Polygon color corresponds to biotopes present, which were mapped using video ground-truthing data and prediction using General Additive Models in the software R.
While top-down classifications can help design and facilitate broad-scale assessments of MPA networks, the degree to which these coarse classification systems represent finer-scale distribution of biological assemblages is still questionable (Williams et al., 2009). In addition, they do not allow the identification of areas where assemblages and species recognized as VMEs or in need of special protection measures are located. Habitat suitability modeling (also called species distribution modeling and predictive habitat mapping) offers a means to produce maps of the distribution of specific species and/or communities, within and between canyon systems. Its potential use in deep-sea conservation and management has been demonstrated in other deep-sea habitats (Piechaud et al., 2015) and across deep-sea regions (Ross and Howell, 2013). However, production of such maps again requires a robust biological classification system (where communities are considered) as well as a firm understanding of the drivers of community and species distributions. Progress in this area was made recently for canyons (Howell et al., 2010; Davies et al., 2014) but with limited spatial coverage. Further work is needed to improve habitat suitability models for canyon species, expand their number, and to cover wider geographical areas. Constructing such models will also help to improve our understanding of the links between physical drivers and biological responses in canyons.
One of the key areas in which research is lacking is an understanding of connectivity both within and between canyons systems. Knowledge of population connectivity, constituting larval dispersal, settlement, survival and successful reproduction, is key for effective marine conservation (Gaines et al., 2003) and is called for many different policy documents. Our knowledge of gene flow within and between canyon systems is very limited, but recent data suggest there may be significant barriers to dispersal between canyons for some species (Pérez-Portela et al., 2016). If barriers to gene flow exist between canyons, then this must be considered when designing spatial management strategies.
Conclusions
With continuous demand for minerals, fish and genetic resources, international interest in submarine canyons is increasing. However, many knowledge gaps remain in many regions regarding abiotic and biological processes in canyons. These issues must be addressed in order to provide the robust scientific knowledge necessary for effective management and conservation. This review demonstrates the need for further investigations to increase our understanding of community structure and ecosystem functioning within and around canyons. Future survey efforts need to incorporate rigorous and standardized sampling to obtain information regarding microhabitats, current flow, and organic matter input within the broad-scale habitat features. Such environmental data are required to better determine the mechanistic factors that affect the diversity, abundance, and distribution of fauna associated with these deep-sea habitats. There are new technologies and analytical methodologies that need to be used to efficiently and effectively provide the scientific understanding of canyons that is required.
Although we provide here the first global assessment of canyon protection, there is a need for this assessment to be augmented by an understanding of the level impacts that canyons face globally. Thus, there needs to be a compilation and synthesis of global information on impacts affecting canyons, the studies conducted to assess those impacts, and the protective and management measures taken to address these impacts. However, this information is currently sparse, non-structured and in some cases, available only in gray literature such as national or regional reports and, thus, difficult to access. This gap in global understanding of canyon impacts and management and conservation measures needs to be addressed by future studies, along with aforementioned ecological studies, if we are to achieve a global understanding of the role played by canyons in the functioning of the biosphere, the services they provide and what measures are needed to exploit their resources in an environmentally-sustainable way.
Author Contributions
UF coordinated, supervised, edited, and act as corresponding author. UF, ER, JA, ALA, JD, AD, PH, KH, VH, MM, JM, LM, MN, PP, AR, FS, and IV wrote the manuscript and helped in the revision and evaluation of the manuscript.
Conflict of Interest Statement
The authors declare that the research was conducted in the absence of any commercial or financial relationships that could be construed as a potential conflict of interest.
Acknowledgments
This paper has been prepared in the framework of the INCISE network. UF was funded by the Balearic Government post-doctoral grant 2016, co-financed by European Social Plan; VH was supported by the CODEMAP project (ERC Starting Grant no 258482) and the NERC MAREMAP programme; PP has been funded by the ABIDES project (CTM2015-65142-R); AR was supported by NIWA research project “Impact of resource use on vulnerable deep-sea communities” (MBIE contract CO1X0906). IV was funded by Ifremer and Région Bretagne. ALA is supported by Science Foundation Ireland / Marine Institute IvP award 15/IA/3100.
Supplementary Material
The Supplementary Material for this article can be found online at: http://journal.frontiersin.org/article/10.3389/fmars.2017.00005/full#supplementary-material
References
Abelló, P., Arcos, J. M., and Sola, L. G. (2003). Geographical patterns of seabird attendance to a research trawler along the Iberian Mediterranean coast. Sci. Mar. 67, 69–75. doi: 10.3989/scimar.2003.67s269
Aguzzi, J., and Company, J. (2010). Chronobiology of deep-water decapod crustaceans on continental margins. Adv. Mar. Biol. 58, 155–225. doi: 10.1016/B978-0-12-381015-1.00003-4
Aguzzi, J., Company, J. B., Costa, C., Matabos, M., Azzurro, E., Mànuel, A., et al. (2012). Challenges to the assessment of benthic populations and biodiversity as a result of rhythmic behaviour: Video solutions from cabled observatories. Oceanogr. Mar. Biol. Annu. Rev. 50, 235–286. doi: 10.1201/b12157-6
Aguzzi, J., Doya, C., Tecchio, S., De Leo, F., Azzurro, E., Costa, C., et al. (2015). Coastal observatories for monitoring of fish behaviour and their responses to environmental changes. Rev. Fish Biol. Fish. 25, 463–483. doi: 10.1007/s11160-015-9387-9
Allee, R. J., Dethier, M., Brown, D., Deegan, L., Ford, G. R., Hourigan, T. R., et al. (2001). Marine and Estuarine Ecosystem and Habitat Classification. Silver Spring, MD: NOAA Technical Memorandum. NMFS-F/SPO-43.
Allen, S., and Durrieu de Madron, X. (2009). A review of the role of submarine canyons in deep-ocean exchange with the shelf. Ocean Sci. 5, 607–620. doi: 10.5194/os-5-607-2009
Allers, E., Abed, R. M., Wehrmann, L. M., Wang, T., Larsson, A. I., Purser, A., et al. (2013). Resistance of Lophelia pertusa to coverage by sediment and petroleum drill cuttings. Mar. Pollut. Bull. 74, 132–140. doi: 10.1016/j.marpolbul.2013.07.016
Amaro, T., Huvenne, V., Allcock, A., Aslam, T., Davies, J., Danovaro, R., et al. (2016). The Whittard Canyon: A case study of submarine canyon processes. Prog. Oceanogr. 146, 38–57. doi: 10.1016/j.pocean.2016.06.003
Armstrong, C. W., Foley, N. S., Tinch, R., and van den Hove, S. (2012). Services from the deep: steps towards valuation of deep sea goods and services. Ecosyst. Services 2, 2–13. doi: 10.1016/j.ecoser.2012.07.001
Asante, K. A., Agusa, T., Mochizuki, H., Ramu, K., Inoue, S., Kubodera, T., et al. (2008). Trace elements and stable isotopes (δ13C and δ15N) in shallow and deep-water organisms from the East China Sea. Environ. Pollut. 156, 862–873. doi: 10.1016/j.envpol.2008.05.020
Ayma, A., Aguzzi, J., Canals, M., Lastras, G., Bahamon, N., Mecho, A., et al. (2016). Comparison between ROV video and Agassiz trawl methods for sampling deep water fauna of submarine canyons in the Northwestern Mediterranean Sea with observations on behavioural reactions of target species. Deep Sea Res. Part I Oceanogr. Res. Papers 114, 149–159. doi: 10.1016/j.dsr.2016.05.013
Bahamon, N., Aguzzi, J., Bernardello, R., Ahumada-Sempoal, M.-A., Puigdefabregas, J., Cateura, J., et al. (2011). The new pelagic Operational Observatory of the Catalan Sea (OOCS) for the multisensor coordinated measurement of atmospheric and oceanographic conditions. Sensors 11, 11251–11272. doi: 10.3390/s111211251
Baker, K. D., Wareham, V. E., Snelgrove, P. V., Haedrich, R. L., Fifield, D. A., Edinger, E. N., et al. (2011). Distributional patterns of deep-sea coral assemblages in three submarine canyons off Newfoundland, Canada. Mar. Ecol. Prog. Ser. 445, 235–249. doi: 10.3354/meps09448
Bakke, T., Klungsøyr, J., and Sanni, S. (2013). Environmental impacts of produced water and drilling waste discharges from the Norwegian offshore petroleum industry. Mar. Environ. Res. 92, 154–169. doi: 10.1016/j.marenvres.2013.09.012
Becker, J., Sandwell, D., Smith, W., Braud, J., Binder, B., Depner, J., et al. (2009). Global bathymetry and elevation data at 30 arc seconds resolution: SRTM30_PLUS. Mar. Geodesy. 32, 355–371. doi: 10.1080/01490410903297766
Benn, A. R., Weaver, P. P., Billet, D. S., van den Hove, S., Murdock, A. P., Doneghan, G. B., et al. (2010). Human activities on the deep seafloor in the North East Atlantic: an assessment of spatial extent. PLoS ONE 5:e12730. doi: 10.1371/journal.pone.0012730
Bianchelli, S., Gambi, C., Zeppilli, D., and Danovaro, R. (2010). Metazoan meiofauna in deep-sea canyons and adjacent open slopes: a large-scale comparison with focus on the rare taxa. Deep Sea Res. Part I Oceanogr. Res. Papers. 57, 420–433. doi: 10.1016/j.dsr.2009.12.001
Blunt, J., Copp, B., Keyzers, R., Munro, M., and Prinsep, M. (2013). Natural product reports. Nat. Prod. Rep. 39, 237–323. doi: 10.1039/C2NP20112G
Bo, M., Bertolino, M., Bavestrello, G., Canese, S., Giusti, M., Angiolillo, M., et al. (2011). “Role of deep sponge grounds in the Mediterranean Sea: a case study in southern Italy,” in Ancient Animals, New Challenges, Developments in Sponge Research, eds M. Maldonado, X. Turon, M. A. Becerro, and M. J. Uriz (Hydrobiologia: Springer), 163–177.
Bosley, K. L., Lavelle, J. W., Brodeur, R. D., Wakefield, W. W., Emmett, R. L., Baker, E. T., et al. (2004). Biological and physical processes in and around Astoria submarine Canyon, Oregon, USA. J. Mar. Syst. 50, 21–37. doi: 10.1016/j.jmarsys.2003.06.006
Bowden, D. A., Rowden, A. A., Leduc, D., Beaumont, J., and Clark, M. R. (2016). Deep-sea seabed habitats: do they support distinct mega-epifaunal communities that have different vulnerabilities to anthropogenic disturbance? Deep Sea Res. I 107, 31–47. doi: 10.1016/j.dsr.2015.10.011
Brodeur, R. D. (2001). Habitat-specific distribution of Pacific ocean perch (Sebastes alutus) in Pribilof Canyon, Bering Sea. Cont. Shelf Res. 21, 207–224. doi: 10.1016/S0278-4343(00)00083-2
Buhl-Mortensen, L., Vanreusel, A., Gooday, A. J., Levin, L. A., and Priede, I. G. (2010). Biological structures as a source of habitat heterogeneity and biodiversity on the deep ocean margins. Mar. Ecol. 31, 21–50. doi: 10.1111/j.1439-0485.2010.00359.x
Butler, A., Harris, P. T., Lyne, V., Heap, A., Passlow, V. L., Smith, R. N. P. (2001). An Interim, Draft Bioregionalisation for the Continental Slope and Deeper Waters of the South-East Marine Region of Australia. Report to The National Oceans Office, Geoscience Australia and CSIRO, Hobart, 35.
Camuffo, M., Soriani, S., and Zanetto, G. (2011). The evolution of marine protected areas (MPAs): the North Adriatic case. Manage. Environ. Qual. Int. J. 22, 59–79. doi: 10.1108/14777831111098480
Canals, M., Puig, P., de Madron, X. D., Heussner, S., Palanques, A., and Fabres, J. (2006). Flushing submarine canyons. Nature 444, 354–357. doi: 10.1038/nature05271
Canals, M., Danovaro, R., Heussner, S., Lykousis, V., Puig, P., Trincardi, F., et al. (2009). Cascades in Mediterranean submarine grand canyons. Oceanography 1, 26–43. doi: 10.5670/oceanog.2009.03
Castro-Jiménez, J., Rotllant, G., Ábalos, M., Parera, J., Dachs, J., Company, J. B., et al. (2013). Accumulation of dioxins in deep-sea crustaceans, fish and sediments from a submarine canyon (NW Mediterranean). Prog. Oceanogr. 118, 260–272. doi: 10.1016/j.pocean.2013.07.017
Chatzievangelou, D., Doya, C., Mihály, S., Sastri, A. R., Thomsen, L., and Aguzzi, J. (2016). High-frequency patterns in the abundance of benthic species near a cold-seep: an internet operated vehicle application. PLoS ONE 11:e0163808. doi: 10.1371/journal.pone.0163808
Cinnirella, S., Sardà, R., Suárez de Vivero, J. L., Brennan, R., Barausse, A., Icely, J., et al. (2014). Steps toward a shared governance response for achieving Good Environmental Status in the Mediterranean Sea. Ecol. Soci. 19:47. doi: 10.5751/ES-07065-190447
Clark, M. R., Althaus, F., Schlacher, T. A., Williams, A., Bowden, D. A., and Rowden, A. A. (2015). The impacts of deep-sea fisheries on benthic communities: a review. ICES J. Mar. Sci. 73(suppl_1): i51–i69. doi: 10.1093/icesjms/fsv123
Clark, M. R., Rowden, A. A., Schlacher, T. A., Guinotte, J., Dunstan, P. K., Williams, A., et al. (2014). Identifying Ecologically or Biologically Significant Areas (EBSA): a systematic method and its application to seamounts in the South Pacific Ocean. Ocean Coast. Manag. 91, 65–79. doi: 10.1016/j.ocecoaman.2014.01.016
Clark, M. R., Rowden, A. A., Schlacher, T., Williams, A., Consalvey, M., Stocks, K. I., et al. (2010). The ecology of seamounts: structure, function, and human impacts. Ann. Rev. Mar. Sci. 2, 253–278. doi: 10.1146/annurev-marine-120308-081109
CBD–Convention on Biological Diversity (2008). Report of the Conference of the Parties to the Convention on Biological Diversity on the Work of its Ninth Meeting in Bonn (Germany), 19–30 May 2008. UNEP/CBD/COP/9/29, 20 June 2008. Available online at: http://www.cbd.int/doc/meetings/cop/cop-09/official/cop-09-29-en.doc (14.09.2008)
Company, J. B., Puig, P., Sardà, F., Palanques, A., Latasa, M., and Scharek, R. (2008). Climate Influence on Deep Sea Populations. PLoS ONE 3:e1431. doi: 10.1371/journal.pone.0001431
Company, J. B., Ramirez-Llodra, E., Sardà, F., Puig, P., Canals, M., Calafat, A., et al. (2012). “Submarine canyons in the Catalan Sea (NW Mediterranean): megafaunal biodiversity patterns and anthropogenic threats Mediterranean Submarine Canyons: Ecology and Governance,” ed W. M. (Ed.). Gland (Switzerland) and Malaga (Spain): IUCN), 133–144.
Cossa, D., Buscail, R., Puig, P., Chiffoleau, J.-F., Radakovitch, O., Jeanty, G., et al. (2014). Origin and accumulation of trace elements in sediments of the northwestern Mediterranean margin. Chem. Geol. 380, 61–73. doi: 10.1016/j.chemgeo.2014.04.015
Costanza, R., d'Arge, R., Limburg, K., Grasso, M., de Groot, R., Faber, S., et al. (1997). The value of the world's ecosystem services and natural capital. Nature 387, 253–260. doi: 10.1038/387253a0
Costanza, R., de Groot, R., Sutton, P., van der Ploeg, S., Anderson, S. J., Kubiszewski, I., et al. (2014). Changes in the global value of ecosystem services. Glob. Environ. Change 26, 152–158. doi: 10.1016/j.gloenvcha.2014.04.002
Cranford, P. J. (1995). Relationships between food quantity and quality and absorption efficiency in sea scallops Placopecten magellanicus (Gmelin). J. Exp. Mar. Biol. Ecol. 189, 123–142. doi: 10.1016/0022-0981(95)00019-N
Currie, D. R., and Isaacs, L. R. (2005). Impact of exploratory offshore drilling on benthic communities in the Minerva gas field, Port Campbell, Australia. Mar. Environ. Res. 59, 217–233. doi: 10.1016/j.marenvres.2004.05.001
Dauvin, J. C. (2010). Towards an impact assessment of bauxite red mud waste on the knowledge of the structure and functions of bathyal ecosystems: the example of the Cassidaigne canyon (north-western Mediterranean Sea). Mar. Pollut. Bull. 60, 197–206. doi: 10.1016/j.marpolbul.2009.09.026
Davies, A. J., Roberts, J. M., and Hall-Spencer, J. (2007). Preserving deep-sea natural heritage: emerging issues in offshore conservation and management. Biol. Conserv. 138, 299–312. doi: 10.1016/j.biocon.2007.05.011
Davies, J. S. (2012). Mapping Deep-Sea Features in UK Waters for Use in Marine Protected Area Network Design. Ph.D. thesis, University of Plymouth.
Davies, J. S., Howell, K. L., Stewart, H. A., Guinan, J., and Golding, N. (2014). Defining biological assemblages (biotopes) of conservation interest in the submarine canyons of the South West Approaches (offshore United Kingdom) for use in marine habitat mapping. Deep Sea Res. II Top. Stud. Oceanogr. 104, 208–229. doi: 10.1016/j.dsr2.2014.02.001
Day, K. (2002). “Deepwater Canyon slope stability,” in Offshore Site Investigation and Geotechnics' Diversity and Sustainability'; Proceedings of an International Conference (London: Society of Underwater Technology).
De Groot, R. S., Wilson, M. A., and Boumans, R. M. (2002). A typology for the classification, description and valuation of ecosystem functions, goods and services. Ecol. Econ. 41, 393–408. doi: 10.1016/S0921-8009(02)00089-7
de Haas, H., van Weering, T. C., and de Stigter, H. (2002). Organic carbon in shelf seas: sinks or sources, processes and products. Cont. Shelf Res. 22, 691–717. doi: 10.1016/S0278-4343(01)00093-0
De Juan, S., Moranta, J., Hinz, H., Barberá, C., Ojeda-Martinez, C., Oro, D., et al. (2012). A regional network of sustainable managed areas as the way forward for the implementation of an Ecosystem-Based Fisheries Management in the Mediterranean. Ocean Coast. Manag. 65, 51–58. doi: 10.1016/j.ocecoaman.2012.04.024
De Leo, F. C., Drazen, J. C., Vetter, E. W., Rowden, A. A., and Smith, C. R. (2012). The effects of submarine canyons and the oxygen minimum zone on deep-sea fish assemblages off Hawai'i. Deep Sea Res. Part I Oceanogr. Res. Pap. 64, 54–70. doi: 10.1016/j.dsr.2012.01.014
De Leo, F. C., Smith, C. R., Rowden, A. A., Bowden, D. A., and Clark, M. R. (2010). Submarine canyons: hotspots of benthic biomass and productivity in the deep sea. Proc. R. Soc. Lond. B Biol. Sci. 277, 2783–2792. doi: 10.1098/rspb.2010.0462
De Leo, F. C., Vetter, E. W., Smith, C. R., Rowden, A. A., and McGranaghan, M. (2014). Spatial scale-dependent habitat heterogeneity influences submarine canyon macrofaunal abundance and diversity off the Main and Northwest Hawaiian Islands. Deep Sea Res. Part II Top. Stud. Oceanogr. 104, 267–290. doi: 10.1016/j.dsr2.2013.06.015
De Mol, L., Van Rooij, D., Pirlet, H., Greinert, J., Frank, N., Quemmerais, F., et al. (2011). Cold-water coral habitats in the Penmarc'h and Guilvinec Canyons (Bay of Biscay): deep-water versus shallow-water settings. Mar. Geol. 282, 40–52. doi: 10.1016/j.margeo.2010.04.011
de Stigter, H. C., Jesus, C. C., Boer, W., Richter, T. O., Costa, A., and van Weering, T. C. E. (2011). Recent sediment transportand deposition in the Lisbon-Setúbal and Cascais submarine canyons, Portuguese continental margin. Deep Sea Res. II 58, 2321–2344. doi: 10.1016/j.dsr2.2011.04.001
Doya, C., Aguzzi, J., Pardo, M., Matabos, M., Company, J., Costa, C., et al. (2014). Diel behavioral rhythms in sablefish (Anoplopoma fimbria) and other benthic species, as recorded by the Deep-sea cabled observatories in Barkley canyon (NEPTUNE-Canada). J. Mar. Syst. 130, 69–78. doi: 10.1016/j.jmarsys.2013.04.003
Dullo, W.-C., Flögel, S., and Rüggeberg, A. (2008). Cold-water coral growth in relation to the hydrography of the Celtic and Nordic European continental margin. Mar. Ecol. Prog. Ser. 371, 165–176. doi: 10.3354/meps07623
Ellis, J., Fraser, G., and Russell, J. (2012). Discharged drilling waste from oil and gas platforms and its effects on benthic communities. Mar. Ecol. Prog. Ser. 456, 285–302. doi: 10.3354/meps09622
Epping, E., van der Zee, C., Soetaert, K., and Helder, W. (2002). On the oxidation and burial of organic carbon in sediments of the Iberian margin and Nazaré Canyon (NE Atlantic). Prog. Oceanogr. 52, 399–431. doi: 10.1016/S0079-6611(02)00017-4
Etnoyer, P., and Warrenchuk, J. (2007). A catshark nursery in a deep gorgonian field in the Mississippi Canyon, Gulf of Mexico. Bull. Mar. Sci. 81, 553–559.
Evans, J. L., Peckett, F., and Howell, K. L. (2015). Combined application of biophysical habitat mapping and systematic conservation planning to assess efficiency and representativeness of the existing High Seas MPA network in the Northeast Atlantic. ICES J. Mar. Sci. 72, 1483–1497. doi: 10.1093/icesjms/fsv012
Fabri, M. C., Pedel, L., Beuck, L., Galgani, F., Hebbeln, D., and Freiwald, A. (2014). Megafauna of vulnerable marine ecosystems in French mediterranean submarine canyons: spatial distribution and anthropogenic impacts. Deep Sea Res. II Top. Stud. Oceanogr. 104, 184–207. doi: 10.1016/j.dsr2.2013.06.016
Farrugio, H. (2012). “A refugium for the spawners of exploited Mediterranean marine species: the canyons of the continental slope of the Gulf of Lion,” in Mediterranean Submarine Canyons: Ecology and Governance, ed M. Würtz (Gland; Málaga: IUCN), 45.
FAO (2009). International Guidelines for the Management of Deep-sea Fisheries in the High Seas. Rome.
Fernandez-Arcaya, U., Rotllant, G., Ramirez-Llodra, E., Recasens, L., Aguzzi, J., Flexas, M. D. M., et al. (2013). Reproductive biology and recruitment of the deep-sea fish community from the NW Mediterranean continental margin. Prog. Oceanogr. 118, 222–234. doi: 10.1016/j.pocean.2013.07.019
Foley, N. S., van Rensburg, T. M., and Armstrong, C. W. (2011). The rise and fall of the Irish orange roughy fishery: an economic analysis. Mar. Policy 35, 756–763. doi: 10.1016/j.marpol.2011.01.003
Foley, N., and Armstrong, C. W. (2010). The ecological and economic value of cold-water coral ecosystems. Ocean Coast Manage 53, 313–326. doi: 10.1016/j.ocecoaman.2010.04.009
Font, J., Salat, J., and Tintoré, J. (1988). “Permanent features of the circulation in the Catalan Sea,” in Océanographie Pélagique Méditerranéenne, eds H. J. Minas and P. Nival (Montrouge: Gauthier-Villars), 51–57.
Fontanier, C., Fabri, M. C., Buscail, R., Biscara, L., Koho, K., Reichart, G., et al. (2012). Deep-sea foraminifera from the Cassidaigne Canyon (NW Mediterranean): assessing the environmental impact of bauxite red mud disposal. Mar. Pollut. Bull. 64, 1895–1910. doi: 10.1016/j.marpolbul.2012.06.016
Freiwald, A., Beuck, L., Rüggeberg, A., Taviani, M., and Hebbeln, D. (2009). R/V Meteor Cruise M70-1 participants, 2009. The white coral community in the central Mediterranean Sea revealed by ROV surveys. Oceanography 22, 58–74. doi: 10.5670/oceanog.2009.06
Gaines, S. D., Gaylord, B., and Largier, J. L. (2003). Avoiding current oversights in marine reserve design. Ecol. Appl. 13, S32–S46. doi: 10.1890/1051-0761(2003)013[0032:ACOIMR]2.0.CO;2
Galgani, F., Fleet, D., Van Franeker, J., Katsanevakis, S., Maes, T., Mouat, J., et al. (2010). Marine Strategy Framework Directive: Task Group 10 Report Marine Litter. Office for Official Publications of the European Communities Luxembourg.
Galgani, F., Leaute, J. P., Moguedet, P., Souplet, A., Verin, Y., Carpentier, A., et al. (2000). Litter on the sea floor along European coasts. Mar. Pollut. Bull. 40, 516–527. doi: 10.1016/S0025-326X(99)00234-9
Galgani, F., Souplet, A., and Cadiou, Y. (1996). Accumulation of debris on the deep sea floor off the French Mediterranean coast. Mar. Ecol. Prog. Ser. 142, 225–234. doi: 10.3354/meps142225
Gambi, C., and Danovaro, R. (2016). Biodiversity and life strategies of deep-sea meiofauna and nematode assemblages in the Whittard Canyon (Celtic margin, NE Atlantic Ocean). Deep Sea Res. I Oceanogr. Res. Pap. 108, 13–22. doi: 10.1016/j.dsr.2015.12.001
Garcia, R., and Thomsen, L. (2008). Bioavailable organic matter in surface sediments of the Nazare canyon and adjacent slope (Western Iberian Margin. J. Mar. Syst. 74, 44–59. doi: 10.1016/j.jmarsys.2007.11.004
Gorelli, G., Sardà, F., and Company, J. B. (2016). Fishing effort increase and resource status of the deep-sea red shrimp aristeus antennatus (Risso 1816) in the Northwest Mediterranean Sea Since the 1950s. Rev. Fish. Sci. Aquac. 24, 192–202. doi: 10.1080/23308249.2015.1119799
Gori, A., Orejas, C., Madurell, T., Bramanti, L., Martins, M., Quintanilla, E., et al. (2013). Bathymetrical distribution and size structure of cold-water coral populations in the Cap de Creus and Lacaze-Duthiers canyons (northwestern Mediterranean). Biogeosciences 10, 2049–2060. doi: 10.5194/bg-10-2049-2013
Gorini, M., Maldonado, P., Silva, C., Souza, E., and Bastos, A. (1998). “Evaluation of deep water submarine hazards at Campos Basin, Brazil,” in Offshore Technology Conference.
Granata, T. C., Vidondo, B., Duarte, C. M., Satta, M. P., and Garcia, M. (1999). Hydrodynamics and particle transport associated with a submarine canyon off Blanes (Spain), NW Mediterranean Sea. Cont. Shelf Res. 19, 1249–1263. doi: 10.1016/S0278-4343(98)00118-6
Greene, H. G., Yoklavich, M. M., Starr, R. M., OŠConnell, V. M. Wakefield, W. W., Sullivan, D. E., et al. (1999). A classification scheme for deep seafloor habitats. Oceanol. Acta 22, 663–678. doi: 10.1016/S0399-1784(00)88957-4
Guinan, J., and Leahy, Y. (2010). Habitat Mapping of Geogenic Reef Offshore Ireland. Report prepared by the Marine Institute, Galway, Ireland and Geological Survey of Ireland to the Department of the Environment, Heritage and Local Government's National Parks and Wildlife Service.
Hall, R. A., and Carter, G. S. (2011). Internal tides in Monterey submarine canyon. J. Phys. Oceanogr. 41, 186–204. doi: 10.1175/2010JPO4471.1
Hargrave, B., Kostylev, V., and Hawkins, C. (2004). Benthic epifauna assemblages, biomass and respiration in The Gully region on the Scotian Shelf, NW Atlantic Ocean. Mar. Ecol. Prog. Ser. 270, 55–70. doi: 10.3354/meps270055
Harris, P. T., and Whiteway, T. (2011). Global distribution of large submarine canyons: Geomorphic differences between active and passive continental margins. Mar. Geol. 285, 69–86. doi: 10.1016/j.margeo.2011.05.008
Harris, P., Heap, A., Post, A., Whiteway, T., Potter, A., and Bradshaw, M. (2007). Marine Zone Management and the EPBC Act-How Environmental Marine Geological Information Provides Certainty for Petroleum Exploration. Canberra: APPEA Journal.
Harris, P., Macmillan-Lawler, M., Rupp, J., and Baker, E. (2014). Geomorphology of the oceans. Mar. Geol. 352, 4–24. doi: 10.1016/j.margeo.2014.01.011
Hess, S., Jorissen, F. J., Venet, V., and Abu-Zied, R. (2005). Benthic foraminiferal recovery after recent turbidite deposition in Cap Breton Canyon, Bay of Biscay. J. Foraminiferal Res. 35, 114–119. doi: 10.2113/35.2.114
Hickey, B. M. (1997). The response of a steep-sided, narrow canyon to time-variable wind forcing. J. Phys. Oceanogr. 27, 697–726. doi: 10.1175/1520-0485(1997)027<0697:TROASS>2.0.CO;2
Hoff, G. R. (2010). Identification of skate nursery habitat in the eastern Bering Sea. Mar. Ecol. Prog. Ser. 403, 243–254. doi: 10.3354/meps08424
Howell, K. L. (2010). A benthic classification system to aid in the implementation of marine protected area networks in the deep/high seas of the NE Atlantic. Biol. Conserv. 143, 1041–1056. doi: 10.1016/j.biocon.2010.02.001
Howell, K., Davies, J., and Narayanaswamy, B. (2010). Identifying deep-sea megafaunal epibenthic assemblages for use in habitat mapping and marine protected area network design. J. Mar. Biol. Assoc.U.K. 90, 33. doi: 10.1017/S0025315409991299
Huang, Z., Nichol, S. L., Harris, P. T., and Caley, M. J. (2014). Classification of submarine canyons of the Australian continental margin. Mar. Geol. 357, 362–383. doi: 10.1016/j.margeo.2014.07.007
Hughes, D. J., Shimmield, T. M., Black, K. D., and Howe, J. A. (2015). Ecological impacts of large-scale disposal of mining waste in the deep sea. Sci. Rep. 5:9985. doi: 10.1038/srep09985
Huthnance, J. M. (1995). Circulation, exchange and water masses at the ocean margin: the role of physical processes at the shelf edge. Prog. Oceanogr. 35, 353–431. doi: 10.1016/0079-6611(95)80003-C
Huvenne, V. A. I., and Davies, J. S. (2014). Towards a new and integrated approach to submarine canyon research. Deep Sea Res. Part II Top. Stud. Oceanogr. 104, 1–5. doi: 10.1016/j.dsr2.2013.09.012
Huvenne, V. A. I., Tyler, P. A., Masson, D. G., Fisher, E. H., Hauton, C., Hühnerbach, V., et al. (2011). A picture on the wall: innovative mapping reveals cold-water coral refuge in submarine canyon. PLoS ONE 6:e28755. doi: 10.1371/journal.pone.0028755
IUCN UNEP-WCMC (2016). The World Database on Protected Areas (WDPA). July 2016 version, Cambridge: UNEP-WCMC. Available online at: http://www.protectedplanet.net
Jobe, Z. R., Lowe, D. R., and Uchytil, S. J. (2011). Two fundamentally different types of submarine canyons along the continental margin of Equatorial Guinea. Mar. Petroleum Geol. 28, 843–860. doi: 10.1016/j.marpetgeo.2010.07.012
Jobstvogt, N., Townsend, M., Witte, U., and Hanley, N. (2014). How can we identify and communicate the ecological value of deep-sea ecosystem services? PLoS ONE. doi: 10.1371/journal.pone.0100646
Johnson, M. P., White, M., Wilson, A., Würzberg, L., Schwabe, E., Folch, H., et al. (2013). A vertical wall dominated by Acesta excavata and Neopycnodonte zibrowii, part of an undersampled group of deep-sea habitats. PLoS ONE 8:e79917. doi: 10.1371/journal.pone.0079917
Jones, J. (1992). Environmental impact of trawling on the seabed: a review. N.Z. J. Mar. Freshwater Res. 26, 59–67. doi: 10.1080/00288330.1992.9516500
Klinck, J. M. (1996). Circulation near submarine canyons: study. J. Geophys. Res. 101, 1211–1223. doi: 10.1029/95JC02901
Koenig, S., Fernández, P., Company, J. B., Huertas, D., and Solé, M. (2013). Are deep-sea organisms dwelling within a submarine canyon more at risk from anthropogenic contamination than those from the adjacent open slope? A case study of Blanes canyon (NW Mediterranean). Prog. Oceanogr. 118, 249–259. doi: 10.1016/j.pocean.2013.07.016
Lake, R. G., and Hinch, S. G. (1999). Acute effects of suspended sediment angularity on juvenile coho salmon (Oncorhynchus kisutch). Can. J. Fish. Aquat. Sci. 56, 862–867. doi: 10.1139/f99-024
Larsson, A. I., van Oevelen, D., Purser, A., and Thomsen, L. (2013). Tolerance to long-term exposure of suspended benthic sediments and drill cuttings in the cold-water coral Lophelia pertusa. Mar. Pollut. Bull. 70, 176–188. doi: 10.1016/j.marpolbul.2013.02.033
Lastras, G., Canals, M., Urgeles, R., Amblas, D., Ivanov, M., Droz, L., et al. (2007). A walk down the Cap de Creus canyon, Northwestern Mediterranean Sea: recent processes inferred from morphology and sediment bedforms. Mar. Geol. 246, 176–192. doi: 10.1016/j.margeo.2007.09.002
Leal, M. C., Puga, J., Serôdio, J., Gomes, N. C., and Calado, R. (2012). Trends in the discovery of new marine natural products from invertebrates over the last two decades–where and what are we bioprospecting. PLoS ONE 7:e30580. doi: 10.1371/journal.pone.0030580
Leduc, D., Rowden, A. A., Nodder, S. D., Berkenbusch, K., Probert, P. K., and Hadfield, M. G. (2014). Unusually high food availability in Kaikoura Canyon linked to distinct deep-sea nematode community. Deep Sea Res. II Top. Stud. Oceanogr. 104, 310–318. doi: 10.1016/j.dsr2.2013.06.003
Levin, L. A., and Le Bris, N. (2015). The deep ocean under climate change. Science 350, 766–768. doi: 10.1126/science.aad0126
Looser, R., Froescheis, O., Cailliet, G. M., Jarman, W. M., and Ballschmiter, K. (2000). The deep-sea as a final global sink of semivolatile persistent organic pollutants? Part II: organochlorine pesticides in surface and deep-sea dwelling fish of the North and South Atlantic and the Monterey Bay Canyon (California). Chemosphere 40, 661–670. doi: 10.1016/S0045-6535(99)00462-2
Lusher, A. (2015). “Microplastics in the marine environment: distribution, interactions and effects,” in Marine Anthropogenic Litter, eds M. Bergmann, L. Gutow, and M. Klages (Springer International Publishing), 245–307. doi: 10.1007/978-3-319-16510-3
MA (2005). “Ecosystems and human well-being: current state and trends” in Millennium Ecosystem Assessment, Global Assessment Reports (Washington, DC). Available online at: http://www.unep.org/maweb/en/Global.aspx
Macquart-Moulin, C., and Patriti, G. (1996). Accumulation of migratory micronekton crustaceans over the upper slope and submarine canyons of the northwestern Mediterranean. Deep Sea Res. Part I Oceanogr. Res. Pap. 43, 579–601. doi: 10.1016/0967-0637(96)00039-8
Marin, P., and Aguilar, R. (2012). “Mediterranean Submarine Canyons 2012: Pending Protection,” in Mediterranean Submarine Canyons: Ecology and Governance, ed W. Maurizio (Gland; Malaga: IUCN), 191–205.
Martín, J., Palanques, A., and Puig, P. (2006). Composition and variability of downward particulate matter fluxes in the Palamós submarine canyon (NW Mediterranean). J. Mar. Syst. 60, 75–97. doi: 10.1016/j.jmarsys.2005.09.010
Martín, J., Puig, P., Masqué, P., Palanques, A., and Sánchez-Gómez, A. (2014a). Impact of bottom trawling on deep-sea sediment properties along the flanks of a submarine canyon. PLoS ONE 9:e104536. doi: 10.1371/journal.pone.0104536
Martín, J., Puig, P., Palanques, A., and Giamportone, A. (2014b). Commercial bottom trawling as a driver of sediment dynamics and deep seascape evolution in the Anthropocene. Anthropocene 7, 1–15. doi: 10.1016/j.ancene.2015.01.002
Martín, J., Puig, P., Palanques, A., and Ribó, M. (2014c). Trawling-induced daily sediment resuspension in the flank of a Mediterranean submarine canyon. Deep Sea Res. II Top. Stud. Oceanogr. 104, 174–183. doi: 10.1016/j.dsr2.2013.05.036
Martín, J., Puig, P., Palanques, A., Masqué, P., and García-Orellana, J. (2008). Effect of commercial trawling on the deep sedimentation in a Mediterranean submarine canyon. Mar. Geol. 252, 150–155. doi: 10.1016/j.margeo.2008.03.012
Masson, D. G., Huvenne, V. A. I., de Stigter, H. C., Wolff, G. A., Kiriakoulakis, K., Arzola, R. G., et al. (2010). Efficient burial of carbon in a submarine canyon. Geology 38, 831–834. doi: 10.1130/G30895.1
Matabos, M., Bui, A. O., Mihály, S., Aguzzi, J., Juniper, S. K., and Ajayamohan, R. (2014). High-frequency study of epibenthic megafaunal community dynamics in Barkley Canyon: a multi-disciplinary approach using the NEPTUNE Canada network. J. Mar. Syst. 130, 56–68. doi: 10.1016/j.jmarsys.2013.05.002
McClain, C. R., and Barry, J. P. (2010). Habitat heterogeneity, disturbance, and productivity work in concert to regulate biodiversity in deep submarine canyons. Ecology 91, 964–976. doi: 10.1890/09-0087.1
McClain, C. R., and Schlacher, T. A. (2015). On some hypotheses of diversity of animal life at great depths on the sea floor. Mar. Ecol. 36, 849–872. doi: 10.1111/maec.12288
Miller, R. J., Hocevar, J., Stone, R. P., and Fedorov, D. V. (2012). Structure-forming corals and sponges and their use as fish habitat in Bering Sea submarine canyons. PLoS ONE 7:e33885. doi: 10.1371/journal.pone.0033885
Moors-Murphy, H. B. (2014). Submarine canyons as important habitat for cetaceans, with special reference to the Gully: a review. Deep Sea Res. II Top. Stud. Oceanogr. 104, 6–19. doi: 10.1016/j.dsr2.2013.12.016
Mordecai, G., Tyler, P. A., Masson, D. G., and Huvenne, V. A. I. (2011). Litter in submarine canyons off the west coast of Portugal. Deep Sea Res. II Top. Stud. Oceanogr. 58, 2489–2496. doi: 10.1016/j.dsr2.2011.08.009
Morris, K. J., Tyler, P. A., Masson, D. G., Huvenne, V. I., and Rogers, A. D. (2013). Distribution of cold-water corals in the Whittard Canyon, NE Atlantic Ocean. Deep Sea Res. II Top. Stud. Oceanogr. 92, 136–144. doi: 10.1016/j.dsr2.2013.03.036
Mortensen, P. B., and Buhl-Mortensen, L. (2005). “Deep-water corals and their habitats in The Gully, a submarine canyon off Atlantic Canada,” in Cold-Water Corals and Ecosystems, eds A. Freiwald, and M. Roberts (Springer), 247–277.
Norse, E. A., Brooke, S., Cheung, W. W. L., Clark, M. R., Ekeland, I., Froese, R., et al. (2012). Sustainability of deep-sea fisheries. Mar. Policy 36, 307–320. doi: 10.1016/j.marpol.2011.06.008
Okey, T. A. (1997). Sediment flushing observations, earthquake slumping, and benthic community changes in Monterey Canyon head. Cont. Shelf Res. 17, 877–897. doi: 10.1016/S0278-4343(96)00067-2
Orejas, C., Gori, A., Lo Iacono, C., Puig, P., Gili, J. M., and Dale, M. R. (2009). Cold-water corals in the Cap de Creus canyon, northwestern Mediterranean: spatial distribution, density and anthropogenic impact. Mar. Ecol. Prog. Ser. 397, 37–51. doi: 10.3354/meps08314
OSPAR (2005). “OSPAR Convention for the Protection of the Marine Environment of the Northeast Atlantic,” in Meeting of the Working Group on Marine Protected Areas, Species and Habitats (Bristol).
Packer, D. B., Boelke, D., Guida, V., and McGee, L. A. (2007). “State of the U.S. Deep Coral Ecosystems in the Northeastern United States Region: Maine to Cape Hatteras,” in The State of Deep Coral Ecosystems of the United States. NOAA Technical Memorandum CRCP-3 eds S. E. Lumsden, T. F. Hourigan, A. W. Bruckner and G. Dorr (Silver Spring), 365.
Paine, M. D., DeBlois, E. M., Kilgour, B. W., Tracy, E., Pocklington, P., Crowley, R. D., et al. (2014). Effects of the Terra Nova offshore oil development on benthic macro-invertebrates over 10 years of development drilling on the Grand Banks of Newfoundland, Canada. Deep Sea Res. II Top. Stud. Oceanogr. 110, 38–64. doi: 10.1016/j.dsr2.2014.10.015
Palanques, A., García-Ladona, E., Gomis, D., Martín, J., Marcos, M., Pascual, A., et al. (2005). General patterns of circulation, sediment fluxes and ecology of the Palamós (La Fonera) submarine canyon, northwestern Mediterranean. Prog. Oceanogr. 66, 89–119. doi: 10.1016/j.pocean.2004.07.016
Palanques, A., Martín, J., Puig, P., Guillén, J., Company, J., and Sard,à, F. (2006). Evidence of sediment gravity flows induced by trawling in the Palamós (Fonera) submarine canyon (northwestern Mediterranean). Deep Sea Res. I Oceanogr. Res. Pap. 53, 201–214. doi: 10.1016/j.dsr.2005.10.003
Palanques, A., Masqué, P., Puig, P., Sanchez-Cabeza, J. A., Frignani, M., and Alvisi, F. (2008). Anthropogenic trace metals in the sedimentary record of the Llobregat continental shelf and adjacent Foix Submarine Canyon (northwestern Mediterranean). Mar. Geol. 248, 213–227. doi: 10.1016/j.margeo.2007.11.001
Palanques, A., Puig, P., Durrieu de Madron, X., Sanchez-Vidal, A., Pasqual, C., Martín, J., et al. (2012). Sediment transport to the deep canyons and open-slope of the western Gulf of Lions during the 2006 intense cascading and open-sea convection period. Prog. Oceanogr. 106, 1–15. doi: 10.1016/j.pocean.2012.05.002
Palmas, F., Addis, P., Cabiddu, S., Cuccu, D., Follesa, M. C., Mura, M., et al. (2015). Distribution of spawning grounds for deep-water red srhimps in the Central western mediterranean sea. Med. Mar. Sci. 16, 117–127. doi: 10.12681/mms.859
Pasqual, C., Sanchez-Vidal, A., Zúñiga, D., Calafat, A., Canals, M., Durrieu de Madron, X., et al. (2010). Flux and composition of settling particles across the continental margin of the Gulf of Lion: the role of dense shelf water cascading. Biogeosciences 7, 217–231. doi: 10.5194/bg-7-217-2010
Paterson, G. L. J., Glover, A. G., Cunha, M. R., Neal, L., de Stigter, H. C., Kiriakoulakis, K., et al. (2011). Disturbance, productivity and diversity in deep-sea canyons: a worm's eye view. Deep Sea Res. II Top. Stud. Oceanogr. 58, 2448–2460. doi: 10.1016/j.dsr2.2011.04.008
Patin, S. (1999). The Environmental Impact of the Offshore Oil and Gas Industry. East Northport, NY: EcoMonitor Publishing.
Pérez-Portela, R., Cerro-Gálvez, E., Taboada, S., Tidu, C., Campillo-Campbell, C., Mora, J., et al. (2016). Lonely populations in the deep: genetic structure of red gorgonians at the heads of submarine canyons in the north-western Mediterranean Sea. Coral Reefs 1–14. doi: 10.1007/s00338-016-1431-2
Pham, C. K., Ramirez-Llodra, E., Alt, C. H. S., Amaro, T., Bergmann, M., Canals, M., et al. (2014). Marine litter distribution and density in european seas, from the shelves to deep basins. PLoS ONE 9:e95839. doi: 10.1371/journal.pone.0095839
Piechaud, N., Downie, A., Stewart, H. A., and Howell, K. L. (2015). The impact of modelling method selection on predicted extent and distribution of deep-sea benthic assemblages. Earth Environ. Sci. Trans. R. Soc. Edinb. 105, 251–261. doi: 10.1017/S1755691015000122
Post, A. L., O'Brien, P. E., Beaman, R. J., Riddle, M. J., and De Santis, L. (2010). Physical controls on deep water coral communities on the George V Land slope, East Antarctica. Antarct. Sci. 22, 371–378. doi: 10.1017/S0954102010000180
Puig, P., Canals, M., Company, J. B., Martin, J., Amblas, D., Lastras, G., et al. (2012). Ploughing the deep sea floor. Nature 489, 286–289. doi: 10.1038/nature11410
Puig, P., Company, J. B., Sardà, F., and Palanques, A. (2001). Responses of deep-water shrimp populations to intermediate nepheloid layer detachments on the Northwestern Mediterranean continental margin. Deep Sea Res. I 48, 2195–2207. doi: 10.1016/S0967-0637(01)00016-4
Puig, P., Martín, J., Masqué, P., and Palanques, A. (2015). Increasing sediment accumulation rates in La Fonera (Palamós) submarine canyon axis and their relationship with bottom trawling activities. Geophys. Res. Lett. 42, 8106–8113. doi: 10.1002/2015GL065052
Puig, P., Palanques, A., and Martín, J. (2014). Contemporary sediment-transport processes in submarine canyons. Ann. Rev. Mar. Sci. 6, 53–77. doi: 10.1146/annurev-marine-010213-135037
Puig, P., Palanques, A., Guillén, J., and Garcıa-Ladona, E. (2000). Deep slope currents and suspended particle fluxes in and around the Foix submarine canyon (NW Mediterranean). Deep Sea Res. I Oceanogr. Res. Pap. 47, 343–366. doi: 10.1016/S0967-0637(99)00062-X
Puig, P., Palanques, A., Sanchez-Cabeza, J. A., and Masqu,é, P. (1999). Heavy metals in particulate matter and sediments in the southern Barcelona sedimentation system (North-western Mediterranean). Mar. Chem. 63, 311–329. doi: 10.1016/S0304-4203(98)00069-3
Purser, A. (2015). A Time Series Study of Lophelia pertusa and reef megafauna responses to drill cuttings exposure on the norwegian margin. PLoS ONE 10:e0134076. doi: 10.1371/journal.pone.0134076
Purser, A., Thomsen, L., Barnes, C., Best, M., Chapman, R., Hofbauer, M., et al. (2013). Temporal and spatial benthic data collection via an internet operated Deep Sea Crawler. Methods Oceanogr. 5, 1–18. doi: 10.1016/j.mio.2013.07.001
Pusceddu, A., Bianchelli, S., Martín, J., Puig, P., Palanques, A., Masqué, P., et al. (2014). Chronic and intensive bottom trawling impairs deep-sea biodiversity and ecosystem functioning. Proc. Natl. Acad. Sci. U.S.A. 111, 8861–8866. doi: 10.1073/pnas.1405454111
Pusceddu, A., Mea, M., Canals, M., Heussner, S., Durrieu De Madron, X., Sanchez-Vidal, A., et al. (2013). Deep-sea benthic ecosystem collapse and recovery after an intense Dense Shelf Water Cascading event. Biogeosci. 10, 2659–2670. doi: 10.5194/bgd-9-17855-2012
Quattrini, A. M., Nizinski, M. S., Chaytor, J. D., Demopoulos, A. W., Roark, E. B., France, S. C., et al. (2015). Exploration of the canyon-incised continental margin of the northeastern united states reveals dynamic habitats and diverse communities. PLoS ONE 10:e0139904. doi: 10.1371/journal.pone.0139904
RAC/SPA (2010). The Mediterranean Sea Biodiversity: State of the Ecosystems, Pressures, Impacts and Future Priorities. Edited by H. Bazairi, S. Ben Haj, F. Boero, D. Cebrian, S. De Juan, A. Limam, J. Lleonart, G. Torchia, and C. Rais (Tunis: RAC/SPA), 100.
Ramirez-Llodra, E., Company, J. B., Sardà, F., and Rotllant, G. (2010). Megabenthic diversity patterns and community structure of the Blanes submarine canyon and adjacent slope in the Northwestern Mediterranean: a human overprint? Mar. Ecol. 31, 167–182. doi: 10.1111/j.1439-0485.2009.00336.x
Ramirez-Llodra, E., De Mol, B., Company, J. B., Coll, M., and Sardà, F. (2013). Effects of natural and anthropogenic processes in the distribution of marine litter in the deep Mediterranean Sea. Prog. Oceanogr. 118, 273–287. doi: 10.1016/j.pocean.2013.07.027
Ramirez-Llodra, E., Trannum, H. C., Evenset, A., Levin, L. A., Andersson, M., Finne, T. E., et al. (2015). Submarine and deep-sea mine tailing placements: a review of current practices, environmental issues, natural analogs and knowledge gaps in Norway and internationally. Mar. Pollut. Bull. 97, 13–35. doi: 10.1016/j.marpolbul.2015.05.062
Ramirez-Llodra, E., Tyler, P. A., Baker, M. C., Bergstad, O. A., Clark, M. R., Escobar, E., et al. (2011). Man and the Last Great Wilderness: Human Impact on the Deep Sea. PLoS ONE 6:e22588. doi: 10.1371/journal.pone.0022588
Reichelt-Brushett, A. J. (2012). Risk assessment and ecotoxicology: limitations and recommendations for ocean disposal of mine waste in the coral triangle. Oceanography 25, 40. doi: 10.5670/oceanog.2012.66
Rennie, S., Hanson, C., McCauley, R., Pattiaratchi, C., Burton, C., Bannister, J., et al. (2009). Physical properties and processes in the Perth Canyon, Western Australia: Links to water column production and seasonal pygmy blue whale abundance. J. Mar. Syst. 77, 21–44. doi: 10.1016/j.jmarsys.2008.11.008
Robert, K., Jones, D. O., Tyler, P. A., Van Rooij, D., and Huvenne, V. A. (2014). Finding the hotspots within a biodiversity hotspot: fine-scale biological predictions within a submarine canyon using high-resolution acoustic mapping techniques. Mar. Ecol. 36, 1256–1276. doi: 10.1111/maec.12228
Roberts, J. M. (2009). Cold-Water Corals: the Biology and Geology of Deep-Sea Coral Habitats. Cambridge: Cambridge University Press.
Rochman, C. M. (2015). “The complex mixture, fate and toxicity of chemicals associated with plastic debris in the marine environment,” in Marine Anthropogenic Litter, eds M. Bergmann, L. Gutow, and M. Klages (Springer), 117–140. doi: 10.1007/978-3-319-16510-3
Roditi-Elasar, M., Kerem, D., Angel, D. L., L., M, Steindler, L., Herut, B., Shoham-Frider, E., et al. (2013). “Akhziv Submarine Canyon: an oasis in the warming oligotrophic Levantine Basin?” in C40th CIESM, Congress Communications Webpages (Marsiglia).
Romano, C., Coenjaerts, J., Flexas, M. D. M., Zú-iga, D., Vanreusel, A., Company, J. B., et al. (2013). Spatial and temporal variability of meiobenthic density in the Blanes submarine canyon (NW Mediterranean). Prog. Oceanogr. 118, 144–158. doi: 10.1016/j.pocean.2013.07.026
Ross, R. E., and Howell, K. L. (2013). Use of predictive habitat modelling to assess the distribution and extent of the current protection of 'listed'deep-sea habitats. Diver. Distribut. 19, 433–445. doi: 10.1111/ddi.12010
Ross, S. W., Rhode, M., and Quattrini, A. M. (2015). Demersal fish distribution and habitat use within and near Baltimore and Norfolk Canyons, US middle Atlantic slope. Deep Sea Res. I Oceanogr. Res. Papers 103, 137–154. doi: 10.1016/j.dsr.2015.06.004
Ryan, J. P., Chave, F. P., and Bellingham, J. G. (2005). Physical-biological coupling in Monterey Bay, California: topographic influences on phytoplankton ecology. Mar. Ecol. Prog. Ser. 287, 23–32. doi: 10.3354/meps287023
Sañé, E., Martín, J., Puig, P., and Palanques, A. (2013). Organic biomarkers in deep-sea regions affected by bottom trawling: Pigments, fatty acids, amino acids and carbohydrates in surface sediments from the la Fonera (Palamós) Canyon, NW Mediterranean Sea. Biogeosciences 10, 8093–8108. doi: 10.5194/bg-10-8093-2013
Sánchez, F., González-Pola, C., Druet, M., García-Alegre, A., Acosta, J., Cristobo, J., et al. (2014). Habitat characterization of deep-water coral reefs in La Gaviera canyon (Avilés Canyon System, Cantabrian Sea). Deep Sea Res. II 106, 118–140. doi: 10.1016/j.dsr2.2013.12.014
Sanchez, F., Morandeau, G., Bru, N., and Lissardy, M. (2013). A restricted fishing area as a tool for fisheries management: example of the capbreton canyon, southern Bay of Biscay. Mar. Policy 42, 180–189. doi: 10.1016/j.marpol.2013.02.009
Sanchez-Vidal, A., Canals, M., Calafat, A. M., Lastras, G., Pedrosa-Pàmies, R., Menéndez, M., et al. (2012). Impacts on the deep-sea ecosystem by a severe coastal storm. PLoS ONE 7:e30395. doi: 10.1371/journal.pone.0030395
Sanchez-Vidal, A., Llorca, M., Farré, M., Canals, M., Barceló, D., Puig, P., et al. (2015). Delivery of unprecedented amounts of perfluoroalkyl substances towards the deep-sea. Sci. Total Environ. 526, 41–48. doi: 10.1016/j.scitotenv.2015.04.080
Santos, R. S., Tempera, F., and Morato, T. (2010). Background Document for Seamounts, Vol. 30. London: OSPAR Commission.
Sardà, F., and Cartes, J. (1994). Spatio-temporal variations in megabenthos abundance in three different habitats of the Catalan deep-sea (Western Mediterranean). Mar. Biol. 120, 211–219. doi: 10.1007/BF00349681
Sardà, F., Company, J., Bahamón, N., Rotllant, G., Flexas, M., Sánchez, J., et al. (2009). Relationship between environment and the occurrence of the deep-water rose shrimp Aristeus antennatus (Risso, 1816) in the Blanes submarine canyon (NW Mediterranean). Prog. Oceanogr. 82, 227–238. doi: 10.1016/j.pocean.2009.07.001
Sbragaglia, V., García, J. A., Chiesa, J. J., and Aguzzi, J. (2015). Effect of simulated tidal currents on the burrow emergence rhythms of the Norway lobster (Nephrops norvegicus). Mar. Biol. 162, 2007–2016. doi: 10.1007/s00227-015-2726-5
Schlacher, T. A., Schlacher-Hoenlinger, M. A., Williams, A., Althaus, F., Hooper, J. N., and Kloser, R. J. (2007). Richness and distribution of sponge megabenthos in continental margin canyons off southeastern Australia. Mar. Ecol. Prog. Ser. 340, 73–88. doi: 10.3354/meps340073
Schlining, K., Von Thun, S., Kuhnz, L., Schlining, B., Lundsten, L., Stout, N. J., et al. (2013). Debris in the deep: Using a 22-year video annotation database to survey marine litter in Monterey Canyon, central California, USA. Deep Sea Res. I Oceanogr. Res. Papers 79, 96–105. doi: 10.1016/j.dsr.2013.05.006
Shepard, F. P., and Dill, R. F. (1966). Submarine Canyons and other Sea Valleys. Chicago, IL: John Wiley & Sons Inc.
Shepard, F. P., Marshall, N. F., McLoughlin, P. A., and Sullivan, G. G. (1979). Currents in Submarine Canyons and Other Seavalleys. Tulsa, OK: AAPG Studies in Geology.
Shimmield, T. M., Black, K. D., Howe, J. A., Hughes, D. J., and Sherwin, T. (2010). Final Report: Independent Evaluation of Deep-Sea Mine Tailings Placement (DSTP). Final Report, PNG 295, SAMS, Oban.
Skropeta, D., and Wei, L. (2014). Recent advances in deep-sea natural products. Nat. Prod. Rep. 31, 999–1025. doi: 10.1039/C3NP70118B
Solomon, S. (2007). “Climate change 2007-the physical science basis,” in Working Group I Contribution to the Fourth Assessment Report of the IPCC (Cambridge University Press).
Talling, P. J. (2014). On the triggers, resulting flow types and frequencies of subaqueous sediment density flows in different settings. Mar. Geol. 352, 155–182. doi: 10.1016/j.margeo.2014.02.006
Thompson, R. C., Moore, C. J., vom Saal, F. S., and Swan, S. H. (2009). Plastics, the environment and human health: current consensus and future trends. Philos. Trans. R. Soc. Lond. B 364, 2153–2166. doi: 10.1098/rstb.2009.0053
Thomsen, L., Barnes, C., Best, M., Chapman, R., Pirenne, B., Thomson, R., et al. (2012). Ocean circulation promotes methane release from gas hydrate outcrops at the NEPTUNE Canada Barkley Canyon node. Geophys. Res. Lett. 39:L16605. doi: 10.1029/2012GL052462
Thurber, A. R., Sweetman, A. K., Narayanaswamy, B. E., Jones, D. O. B., Ingels, J., and Hansman, R. L. (2014). Ecosystem function and services provided by the deep sea. Biogeosciences 11, 3941–3963. doi: 10.5194/bg-11-3941-2014
Tissot, B. N., Yoklavich, M. M., Love, M. S., York, K., and Amend, M. (2006). Benthic invertebrates that form habitat on deep banks off southern California, with special reference to deep sea coral. Fish. Bull. 104, 167–181.
Tobar, R., and Sardà, F. S. (1987). Análisis de la Evolución de las Capturas de Gamba Rosada, Aristeus antennatus (Risso, 1816), en Los Últimos Decenios en Catalu-a. CENCIMAR.
Tubau, X., Canals, M., Lastras, G., Rayo, X., Rivera, J., and Amblas, D. (2015). Marine litter on the floor of deep submarine canyons of the Northwestern Mediterranean Sea: the role of hydrodynamic processes. Prog. Oceanogr. 134, 379–403. doi: 10.1016/j.pocean.2015.03.013
Tyler, P. A., Amaro, T., Arzola, R., Cunha, M. R., de Stigter, H., Gooday, A., et al. (2009). Europe's Grand Canyon: Nazaré Submarine Canyon. Oceanography 1, 52–57. doi: 10.5670/oceanog.2009.05
UNEP-MAP-RAC/SPA, (2010). “Impact of Climate Change on Marine and Coastal Biodiversity,” in The Mediterranean Sea: Current State of Knowledge. eds S. Ben Haj and A. Limam (Tunis: RAC/SPA Edit), 1–28.
Uriarte, A., Zarauz, L., Aranda, M., Santurtun, M., Iriondo, A., Berthou, P., et al. (2014). Guidelines for the Definition of Operational Management Units, Vol. 69. AZTI Report of Project GEPETO.
Van den Beld, I. M. J., Guillaumont, B., Menot, L., Bayle, C., Arnaud-Haond, S., and Bourillet, J.-F. (in press). Marine litter in submarine canyons of the Bay of Biscay. Deep Sea Res. II. doi: 10.1016/j.dsr2.2016.04.013c
van Oevelen, D., Soetaert, K., Garcia, R., de Stigter, H. C., Cunha, M. R., Pusceddu, A., et al. (2011). Canyon conditions impact carbon flows in food webs of three sections of the Nazaré canyon. Deep Sea Res. II 58, 2461–2476. doi: 10.1016/j.dsr2.2011.04.009
Vetter, E. W. (1995). Detritus-based patches of high secondary production in the nearshore benthos. Mar. Ecol. Prog. Ser. 120, 251–262. doi: 10.3354/meps120251
Vetter, E. W., and Dayton, P. K. (1998). Macrofaunal communities within and adjacent to a detritus-rich submarine canyon system. Deep Sea Res. II 45, 25–54. doi: 10.1016/S0967-0645(97)00048-9
Vetter, E. W., Smith, C. R., and De Leo, F. C. (2010). Hawaiian hotspots: enhanced megafaunal abundance and diversity in submarine canyons on the oceanic islands of Hawaii. Mar. Ecol. 31, 183–199. doi: 10.1111/j.1439-0485.2009.00351.x
Wattage, P., Glenn, H., Mardle, S., Van Rensburg, T., Grehan, A., and Foley, N. (2011). Economic value of conserving deep-sea corals in Irish waters: a choice experiment study on marine protected areas. Fish. Res. 107, 59–67. doi: 10.1016/j.fishres.2010.10.007
Weaver, P., Benn, A., Arana, P., Ardron, J., Bailey, D., Baker, K., et al (2011). The Impact of Deep-sea Fisheries and Implementation of the UNGA Resolutions 61/105 and 64/72. Report of an international scientific workshop, National Oceanography Centre, Southampton, 45. Available online at: http://hdl.handle.net/10013/epic.37995
Westhead, M. C., Fenton, D. G., Koropatnick, T. A., Macnab, P. A., and Moors, H. B. (2012). Filling the gaps one at a time: the Gully Marine Protected Area in Eastern Canada. A response to Agardy, Notarbartolo di Sciara and Christie. Mar. Policy 36, 713–715. doi: 10.1016/j.marpol.2011.10.022
White, H. K., Hsing, P.-Y., Cho, W., Shank, T. M., Cordes, E. E., Quattrini, A. M., et al. (2012). Impact of the Deepwater Horizon oil spill on a deep-water coral community in the Gulf of Mexico. Proc. Natl. Acad. Sci. U.S.A. 109, 20303–20308. doi: 10.1073/pnas.1118029109
Williams, A., Bax, N. J., Kloser, R. J., Althaus, F., Barker, B., and Keith, G. (2009). Australia's deep-water reserve network: Implications of false homogeneity for classifying abiotic surrogates of biodiversity. ICES J. Mar. Sci. 66, 214–224. doi: 10.1093/icesjms/fsn189
Wilson, A. M., Kiriakoulakis, K., Raine, R., Gerritsen, H. D., Blackbird, S., Allcock, A. L., et al. (2015a). Anthropogenic influence on sediment transport in the Whittard Canyon, NE Atlantic. Mar. Pollut. Bull. 101, 320–329. doi: 10.1016/j.marpolbul.2015.10.067
Wilson, A. M., Raine, R., Mohn, C., and White, M. (2015b). Nepheloid layer distribution in the Whittard Canyon, NE Atlantic margin. Mar. Geol. 367, 130–142. doi: 10.1016/j.margeo.2015.06.002
Xu, J. (2011). Measuring currents in submarine canyons: technological and scientific progress in the past 30 years. Geosphere 7, 868–876. doi: 10.1130/GES00640.1
Yoklavich, M. M., Greene, H. G., Cailliet, G. M., Sullivan, D. E., Lea, R. N., and Love, M. S. (2000). Habitat associations of deep-water rockfishes in a submarine canyon: an example of a natural refuge. Fish. Bull. Natl. Oceanic Atmosphere. Adm. 98, 625–641.
Keywords: submarine canyons, ecosystem service, anthropogenic impacts, conservation, management
Citation: Fernandez-Arcaya U, Ramirez-Llodra E, Aguzzi J, Allcock AL, Davies JS, Dissanayake A, Harris P, Howell K, Huvenne VAI, Macmillan-Lawler M, Martín J, Menot L, Nizinski M, Puig P, Rowden AA, Sanchez F and Van den Beld IMJ (2017) Ecological Role of Submarine Canyons and Need for Canyon Conservation: A Review. Front. Mar. Sci. 4:5. doi: 10.3389/fmars.2017.00005
Received: 03 August 2016; Accepted: 09 January 2017;
Published: 31 January 2017.
Edited by:
Ricardo Serrão Santos, University of the Azores, PortugalReviewed by:
Mustafa Yucel, Middle East Technical University, TurkeyGian Marco Luna, Consiglio Nazionale delle Ricerche (CNR), Italy
Copyright © 2017 Fernandez-Arcaya, Ramirez-Llodra, Aguzzi, Allcock, Davies, Dissanayake, Harris, Howell, Huvenne, Macmillan-Lawler, Martín, Menot, Nizinski, Puig, Rowden, Sanchez and Van den Beld. This is an open-access article distributed under the terms of the Creative Commons Attribution License (CC BY). The use, distribution or reproduction in other forums is permitted, provided the original author(s) or licensor are credited and that the original publication in this journal is cited, in accordance with accepted academic practice. No use, distribution or reproduction is permitted which does not comply with these terms.
*Correspondence: Ulla Fernandez-Arcaya, dWxsYS5mZXJuYW5kZXpAYmEuaWVvLmVz