Dynamic Interactions among Boundaries and the Expansion of Sustainable Aquaculture
- 1Centro de Estudios Avanzados en Zonas Áridas, Facultad de Ciencias del Mar, Universidad Católica del Norte, Coquimbo, Chile
- 2Bren School of Environmental Science and Management, University of California, Santa Barbara, CA, USA
- 3Departamento de Ecología, Pontificia Universidad Católica de Chile, Alameda, Chile
- 4Facultad de Ingeniería y Ciencias and Facultad de Artes Liberales, Universidad Adolfo Ibáñez, Santiago, Chile
- 5Laboratorio de Funcionamiento de Ecosistemas Acuáticos, Departamento de Sistemas Acuáticos, Facultad de Ciencias Ambientales, Universidad de Concepción, Concepcion, Chile
- 6Universidad del Desarrollo and Universidad de Concepción, Concepción, Chile
- 7Plymouth Marine Laboratory, Plymouth, UK
- 8CEFAS Lowestoft Laboratory, Suffolk, UK
Aquaculture is the fastest growing food production system in the world, generating more than half of the global seafood harvested today. These type of activities are crucial to provide key nutritional components for humanity in the future as populations worldwide are increasing and the demands for securing food resources are imperative. Multiple socio-ecological factors such as weak regulations and focus on maximizing production limit production and threaten the sustainable growth of aquaculture. We present a novel policy framework to evaluate and pursue growth in aquaculture considering four boundaries: biological productivity, environmental constraints to that productivity, policy that inhibits or promotes different kinds of aquaculture, and social preferences that determine aquaculture markets. Using a range of scenarios, we have shown that sustainable growth in aquaculture requires simultaneous consideration of all four boundaries and the potential interactions between all of these options. Our proposed conceptual framework shows that to further expand the boundaries of aquaculture production, the policy focus must remain flexible to enable the adaptation of from single-boundary approaches. Our approach takes account of the current boundaries, helping to consider the adaptive policy, which is deemed as a necessary tool for considering the dynamic interactions among boundaries, thus addressing the problem of defining the evolving limits of sustainable aquaculture.
Summary
We provide a conceptual framework of sustainable seafood aquaculture that departs from earlier approaches to sustainability based solely on regulations, technological advance or increased system efficiency. To this end we define four dimensions of the social-ecological system that supports aquaculture: (1) biological productivity, (2) environment constraints, (3) policy tools that enable or limit aquaculture, and (4) societal preferences and the current farmed seafood markets demands. Policy analyses habitually consider single boundaries, but the dynamic interaction between boundaries suggests that boundary changes can have unexpected consequences if one is not paying attention to their integrated responses. The proposed framework is a starting point for investigating how key indicators of adaptive capacity differs inside the industry on the basis of the interacting boundaries. By considering this suggested approach, then policies may be developed to increase the adaptive capacity of a globally expanding industry within defined sustainability boundaries.
Aquaculture is the fastest growing food production system in the world (Asche, 2013; Food Agriculture Organization, 2014) and a major part of the solution to provide key nutritional components to the increasing human population (Food Agriculture Organization, 2014) Aquaculture now accounts for roughly half of the world's seafood production including fish (66.6 million tons/year), algae (23.8 tons/year) and mollusks (15.1 tons/year), among other taxa such as echinoderms and crustaceans, and is bound to continue growing into the next decades (World Bank, 2013; Food Agriculture Organization, 2014). During the 2010-2013 period, 53% of the aquaculture production came from mariculture—aquaculture in the ocean's coasts and estuaries, a share that expanded by 8.3% annually in comparison with a 6.6% growth in freshwater aquaculture. This growth was attained through territorial expansion, efficiency in resource utilization and incremental technological innovations in the production process (Bostock et al., 2010; Food Agriculture Organization, 2014). The rapid expansion of intensive and extensive seafood production is taking place mostly in developing or underdeveloped countries, where regulations are weak or policy focuses solely on production and has led to a range of environmental problems, including habitat destruction, pollution, and overuse of marine resources (Primavera, 2006; Smith et al., 2010). Together, these factors threaten the sustainability, and thus future productivity of mariculture. To achieve the many goals and expectations placed on seafood aquaculture, it is necessary to improve on the current growth strategies that solely focus on production and efficiency. Furthermore, more targeted work is needed to define—with the purpose of expanding—the boundaries of sustainable aquaculture.
Here, we provide a conceptual model of seafood aquaculture that helps define the boundaries of sustainable production given the demands placed on aquaculture to feed the global human population. Sustainability is driven by four dimensions of the social-ecological system that supports aquaculture: (1) the biology of cultivated species that determines the limits and opportunities for biomass production, (2) the environment that influences the biology and production limits, (3) the policy that enables or limits different aquaculture practices, and (4) the societal preferences and demands that drive markets for farmed seafood (Figure 1A). There is broad consensus that realistic, science-based models are paramount and urgently needed to assist and guide future policy development, helping to move aquaculture beyond simple objectives (e.g., such as conservation or employment; Bostock et al., 2010). Our conceptual model provides a unique framework to identify sustainable paths for growth and its boundaries, departing from approaches to sustainability based solely on regulations, technological advance or increased system efficiency (Bostock et al., 2010; Troell et al., 2015).
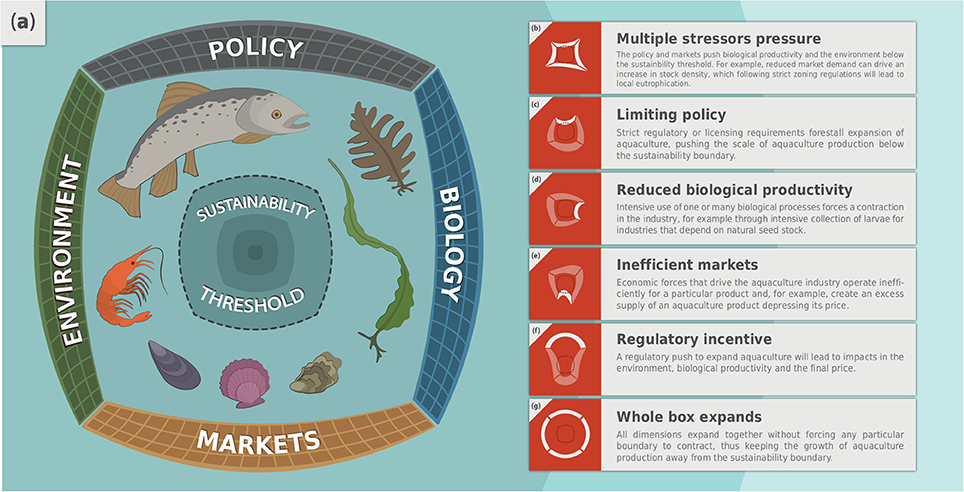
Figure 1. The illustration in (A) depicts the four dimensions of the social-ecological system that supports aquaculture and ultimately define the boundaries of sustainable production. Biology is the common traits of the cultivated species, helping to illustrate the limits and opportunities for biomass production, (2) Environment is the biophysical variability that influences the biology and overall production boundaries, (3) Policy is the regulatory framework that enables or limits different aquaculture practices, and (4) Markets are the societal preferences and demands, which drive the production of farmed seafood. The sustainability threshold, defined by the dashed line inside the four boundaries, is a part of the production space where aquaculture production will fall below the levels required to sustain the industry. The five diagrams from (B–F) provide examples of how the movement of multiple or single boundaries, either contracting or expanding aquaculture production, may interact with other boundaries, pushing them beyond the sustainability threshold.
Sustainable production of farmed seafood requires addressing each of these four dimensions individually (Figures 1C–E), but also their combined interactions (Figures 1B,G). In some cases, the location of an individual boundary drives potential aquaculture production; for example, when policy prohibits aquaculture permits (Figure 1C), recruitment failure occurs for species that use wild seed stock (Figure 1D), or markets operate inefficiently for a particular product (Figure 1E). Furthermore, when changes in one boundary may ultimately lead to affect or modify other boundaries, will often result in further delays. For example, when human activities increase the cumulative impact of multiple stressors, production may initially increase but then decrease as multiple stressors ultimately limit biological productivity (Figure 1B). Interactions occur when changes in some aspect of the social-ecological system cause or require other boundaries to contract (with increasing limitations) or expand (with innovations, Figure 1G). As Figure 1 is constructed, the area contained within the 4 boundaries can be considered to represent the total achievable production, and contractions in one or more of the four boundaries will lead to a decrease in this area and, therefore, total production assuming the other boundaries cannot adapt and expand. For example, lack of market incentives and restrictive regulations limit aquaculture production in the U.S. and the U.K., keeping sustainable production well below potential levels (Asche, 2013). Similarly, expansions in a boundary may not lead to increases in total production if other boundaries contract in response. For example, market forces and government incentives promoted the explosive growth of salmon aquaculture in Chile. The rapid expansion degraded water quality and overwhelmed sanitary barriers, which created the conditions for an outbreak of a viral disease that ultimately reduced aquaculture production (Figure 1F, Smith et al., 2010). Thus, the only way to increase the sustainable production of seafood is to simultaneously expand (or maintain) multiple boundaries in the system.
The dynamic interaction between boundaries suggests that boundary changes can have unexpected consequences if one is not paying attention to their integrated responses. A technological advancement intended to improve the biomass productivity of a species, and thus expand the biology boundary (e.g., the development and use of chemicals to control parasites or disease in cultivated species) can have a detrimental effect on the societal preferences (i.e., market) boundary, with a reduced market demand for any seafood perceived to be contaminated by such chemicals and the ensuing regulatory demands that such public perceptions may trigger (Tveterås, 2002). Similarly, increased demand of aqua-feed requires ingredients, such as small pelagic fishes of low market value or, increasingly, agricultural commodities, which creates social and environmental conflicts (Cao et al., 2015; Troell et al., 2015). If any boundary contracts too far and crosses the sustainability threshold (center of Figure 1A), then the location of other boundaries will not matter, just as if the market for aquaculture disappears or a policy prohibits aquaculture, an otherwise productive aquaculture system will not survive. Furthermore, the expansion of one boundary can have repercussions (e.g., contract) another boundary, creating the scenario where efforts to expand aquaculture become unsustainable. For example, markets could demand levels of production (biology) that imply large environmental impacts. These impacts will contract the environment boundary making it cross the sustainability threshold for the industry. Before any changes in aquaculture practice and policy are implemented, the potential impacts (direct or indirect) of these changes on each of the sustainability boundaries should be fully considered.
There are few examples where aquaculture development and expansion have addressed all boundaries in a way that allowed totally sustainable aquaculture production to increase. One good example comes from Canada, where the sustainable growth of Canadian aquaculture has occurred in response to 5-year strategic plans in place since 1999 (National Aquaculture Strategic Action Plan Initiative, 2010). These regularly updated plans are designed by the Canadian Council of Fisheries and Aquaculture Ministers, based on governance, social license and market principles, and are established after broad consultations with governmental officers, industry, First Nation tribes and other stakeholders. Between 1990 and 2002, aquaculture production quadrupled, from 40,000 to 17,000 t, and after some fluctuations it has stayed nearly constant at around 150,000 t. This growth has generated thousands of job and over $1 billion in GDP, making it the largest food commodity export (Food Agriculture Organization, 2012). Despite these successes, Canada's enormous biophysical potential remains underutilized—it produces just 0.3% of global aquaculture output—with considerable scope for sustainable growth.
The framework we propose requires one to explicitly address the adaptive capacity of a social-ecological system, a fundamental component of sustainable development in an era driven by multiple local and global drivers (Gelcich et al., 2006). Adaptive capacity is a latent characteristic that reflects aquaculture industries ability to anticipate and respond to changes, and to minimize, cope with, and recover from the consequences of change (Smit and Wandel, 2006). It is relevant to highlight that not every aquaculture development will need enhancement of the same aspects of adaptive capacit, and it is clear that some adaptation measures, such as industry re-location, can potentially create unintended and unforeseen consequences affecting social and natural systems. Those players, which hold a high adaptive capacity may be better able to convert boundary constraints into successful adaptation outcomes. Systems where technological, political, or social innovation can help expand boundaries simultaneously will promote adaptive capacit. Our conceptual framework is designed to highlight the need to develop this adaptive capacity, which is to expand the boundaries while not crossing the sustainability threshold. This follows a similar line as the “resilience space” of the 5th Assessment Report of the IPCC Working Group II, where biophysical stressors conflate with social stressors to squeeze the space for climate-resilient pathways to development (Mimura et al., 2014). In this way The proposed framework is a conceptual starting point for investigating how key indicators of adaptive capacity differ inside the industry on the basis of the interacting boundaries. In this way, policies may be developed to increase the adaptive capacity of a globally expanding industry within defined sustainability boundaries.
Growth in the production of farmed seafood requires adaptive policy tools that acknowledge and address the dynamic interactions among boundaries to sustainable aquaculture production. Growth will happen by increasing production within existing farms, through expansion into new locations or the development of new cultivated species. In any of these cases, growth will lead to environmental, social and market changes, and require institutional and scientific innovations. Policy that does not adapt to this new information and context is bound to push (or allow) systems past sustainability thresholds for at least one of the four boundaries. There is broad agreement that aquaculture has the potential to feed the world in the future (Food Agriculture Organization, 2014), yet there is great risk that this growth could occur unsustainably. By departing significantly from earlier proposals on sustainability, we focus on a full set of boundaries that interact dynamically to define the evolving limits of sustainable aquaculture. In this way, we provide a new step forward in the urgent challenge of channeling the impending expansion of mariculture around the world toward a sustainable pathway.
Author Contributions
BB, BH, SG, ML, CV, FV, and SW conceived the original idea. BB, BH, SG, and SB helped with writing and analysis.
Conflict of Interest Statement
The authors declare that the research was conducted in the absence of any commercial or financial relationships that could be construed as a potential conflict of interest.
The reviewer SA and handling Editor declared their shared affiliation, and the handling Editor states that the process nevertheless met the standards of a fair and objective review.
Acknowledgments
This work was supported by the Millennium Nucleus Center for the Study of Multiple-drivers on Marine Socio-Ecological Systems (MUSELS) funded by MINECON NC120086, SB was funded under the UK Department for the Environment, Food and Rural Affairs under the project PLACID (MF1113). We are grateful for the helpful comments from the two reviewers, The artwork of Figure 1 is by Frano Mejias.
References
Asche, F. (2013). Green Growth in Fisheries and Aquaculture. OECD Synthesis Report on Green Growth http://www.oecd.org/tad/sustainable-agriculture/48258799.pdf
Bostock, J., McAndrew, B., Richards, R., Jauncey, K., Telfer, T., Lorenzen, K., et al. (2010). Aquaculture: global status and trends. Philos. Trans. R. Soc. B. 365, 2897–2912. doi: 10.1098/rstb.2010.0170
Cao, L., Naylor, R., Henriksson, P., Leadbitter, D., Metian, M., Troell, M., et al. (2015). China's aquaculture and the world's fisheries. Science 347, 133–135. doi: 10.1126/science.1260149
Food Agriculture Organization (2012). Fishery and Aquaculture Statistics 2012. Rome: Food and Agriculture Organization.
Food Agriculture Organization (2014). The State of World Fisheries and Aquaculture 2014. Rome: Food and Agriculture Organization.
Gelcich, S., Edwards-Jones, G., Kaiser, M. J., and Castilla, J. C. (2006). Co-management policy can reduce resilience in traditionally managed marine ecosystems. Ecosystems 9, 961–966. doi: 10.1007/s10021-005-0007-8
Mimura, N., Pulwarty, R. S., Duc, D. M., Elshinnawy, I., Redsteer, M. H., Huang, H. Q., et al., (2014). “Adaptation planning and implementation,” In Climate Change 2014: Impacts, Adaptation, and Vulnerability. Part A: Global and Sectoral Aspects. Contribution of Working Group II to the Fifth Assessment Report of the Intergovernmental Panel on Climate Change, eds C. B. Field, V. R. Barros, D. J. Dokken, K. J. Mach, M. D. Mastrandrea, T. E. Bilir (Cambridge; New York, NY: Cambridge University Press), 869–898.
National Aquaculture Strategic Action Plan Initiative (2010). Fisheries and Oceans. Ottawa, ON. Available online at: http://www.dfo-mpo.gc.ca/aquaculture/lib-bib/nasapi-inpasa/index-eng.htm
Primavera, J. H. (2006). Overcoming the impacts of aquaculture in the coastal zone. Ocean Coast. Manag. 49, 531–545. doi: 10.1016/j.ocecoaman.2006.06.018
Smit, B., and Wandel, J. (2006). Adaptation, adaptive capacity and vulnerability. Glob. Environ. Change 16, 282–292. doi: 10.1016/j.gloenvcha.2006.03.008
Smith, M. D., Roheim, C. A., Crowder, L. B., Halpern, B. S., Turnipseed, M., Anderson, J. L., et al. (2010). Sustainability and global seafood. Science 327, 784–786. doi: 10.1126/science.1185345
Troell, M., Naylor, R. L., Metian, M., Beveridge, M., Tyedmers, P. H., Folke, C., et al. (2015). Does aquaculture add resilience to the global food system? Proc. Natl. Acad. Sci. U.S.A. 111, 13257–13263. doi: 10.1073/pnas.1404067111
Tveterås, S. (2002). Norwegian salmon aquaculture and sustainability: the relationship between environmental quality and industry growth. Mar. Res. Econ. 17, 121–132.
World Bank (2013). Fish to 2030: Prospects for Fisheries and Aquaculture. Agriculture and environmental services discussion paper; no. 3. Washington DC; World Bank Group. Available online at: http://documents.worldbank.org/curated/en/2013/12/18882045/fish-2030-prospects-fisheries-aquaculture.
Keywords: sustainability, aquaculture research, socioecology, molusks, fishes
Citation: Broitman BR, Halpern BS, Gelcich S, Lardies MA, Vargas CA, Vásquez-Lavín F, Widdicombe S and Birchenough SNR (2017) Dynamic Interactions among Boundaries and the Expansion of Sustainable Aquaculture. Front. Mar. Sci. 4:15. doi: 10.3389/fmars.2017.00015
Received: 03 August 2016; Accepted: 11 January 2017;
Published: 26 January 2017.
Edited by:
Romuald Lipcius, Virginia Institute of Marine Science, College of William & Mary, USAReviewed by:
Standish K. Allen Jr, Virginia Institute of Marine Science, College of William & Mary, USADoris Oliva, Universidad de Valparaiso, Chile
Copyright © 2017 Broitman, Halpern, Gelcich, Lardies, Vargas, Vásquez-Lavín, Widdicombe and Birchenough. This is an open-access article distributed under the terms of the Creative Commons Attribution License (CC BY). The use, distribution or reproduction in other forums is permitted, provided the original author(s) or licensor are credited and that the original publication in this journal is cited, in accordance with accepted academic practice. No use, distribution or reproduction is permitted which does not comply with these terms.
*Correspondence: Bernardo R. Broitman, bernardo.broitman@ceaza.cl