- 1Department of Bioengineering, Institute for Bioengineering and Biosciences, Instituto Superior Técnico, Universidade de Lisboa, Lisboa, Portugal
- 2Centre for Ecology, Evolution and Environmental Changes, Faculdade de Ciências, Universidade de Lisboa, Lisboa, Portugal
The colorful carotenoid pigments are known as biological active compounds that have beneficial effects on the metabolism of animals and humans. Carotenoids provide protection against several stressors, including UV radiation, reactive oxygen species and free radicals, have important roles in vision, and act as precursors of transcription regulators and in the immune system. Studies on human nutrition point to the relevance of consuming functional foods instead of supplementing the human diet with the desired nutrients. This stresses the importance of obtaining dietary items with high quality nutritional value for human consumption. The various biological roles of carotenoids in aquatic animals ascribes them a major role in aquaculture where they are routinely added to ensure the development and health of fish such as salmon, trout and red porgy, and of shellfish like shrimp and lobster. In aquaculture, it is widely recognized that fish larvae dramatically increase their survival rate when reared on live feeds (e.g., rotifers, Artemia sp. and copepods) containing carotenoids. Ultimately, pigmentation provided by carotenoids is one of the relevant quality attributes of the aquatic animal for consumer acceptability and market value. Appropriate feeds for aquaculture are thus required to provide the carotenoids necessary for the desired pigmentation. In this review, we discuss the role of carotenoids in aquatic food webs, both in the wild and in aquaculture, and its relevance for human health.
Introduction
Carotenoids are synthesized by photosynthetic algae and plants, fungi and bacteria while other organisms must obtain the necessary carotenoids either directly from the diet, or modify the dietary carotenoid precursors through metabolic reactions. In autotrophs, carotenoids act mainly as photoprotectors related to the photosynthetic process, while other organisms in the food web benefit from their protection against ultraviolet (UV) radiation, their antioxidant properties against reactive oxygen species (ROS) and free radicals (Maoka, 2011; Caramujo et al., 2012), their role in vision, as precursors of transcription regulators, and in the immune system (Bendich and Olson, 1989; von Schantz et al., 1999; Hill and Johnson, 2012; Anbazahan et al., 2014). In vertebrates, body coloration play important roles in camouflage, communication, ecological interactions and speciation (Sefc et al., 2014). In fish, carotenoid-based body coloration is influenced by diet and body condition and has been positively related to mating success and social dominance (Maan and Sefc, 2013). In bacteria, carotenoids have also been linked to tolerance and adaptation to several stressful conditions that include salinity, radioactive compounds, pH and temperature (Antón et al., 2000; Asker et al., 2007).
The main feature of the carotenoid molecule is the long conjugated double-bond system with the ability to absorb light in the 400–500 nm visible region of the electromagnetic spectrum (Goodwin, 1980). This polyene chain ascribes the characteristic yellow, orange, and red color to carotenoids, and is responsible for their main roles in plants and algae where they act as: accessory light-harvesting pigments, participating in the collection of light energy and its transfer to chlorophyll for photosynthesis; and as photo-protectors of chlorophyll dissipating the excessive energy used in photosynthesis and inhibiting the formation of ROS (Krinsky, 1968; Cogdell, 1978). The chemical structure of the carotenoids plays an important role in their oxygen scavenge properties (Britton, 1995). Carotenoids such as lycopene (Ψ-carotene) and ζ-carotene are acyclic, whilst others like γ- and δ-carotene have a six-carbon ring at one end, or one ring at each end of the molecule such as α- and β-carotene (Figure 1). These hydrocarbon carotenoids have oxygenated derivatives, or xanthophylls, where the hydroxy, keto, epoxy, or aldehyde oxygen-containing groups are responsible for their polarity, solubility and overall chemical properties. In nature, the most thermodynamically stable configuration found in carotenoids is in general the all-E, but the Z-isomers have also been found in small amounts in raw foods and in increased values in processed foods (Rodriguez-Amaya, 2015). The chemical structure of carotenoids regulates not only their antioxidant activity, but also modulates other dietary properties such as their release from the food matrix, uptake by intestinal mucosal cells, tissue accumulation and metabolism (Cadenas and Packer, 1996). Humans favor the absorption of high polarity xanthophylls, such as lutein, zeaxanthin, astaxanthin and fucoxanthin over other hydrocarbon carotenoids (Britton, 1995).
The Mediterranean diet, which was inscribed on the UNESCO's Representative List of the Intangible Cultural Heritage of Humanity in 2013, is known to offer considerable health benefits when compared to other western diets. This diet can be characterized by four essential dietary indicators: high monounsaturated:saturated fatty acid ratio (1.6–2.0); high intake of fibers (41–62 g person−1 day−1); high antioxidant levels (3,500–5,300 trolox equivalent person−1 day−1); and high phytosterols intake (370–555 mg person−1 day−1) (Saura-Calixto and Goñi, 2009). The Mediterranean diet also includes considerable amounts of fish and shellfish, rich in polyunsaturated fatty acids (PUFA). Both observational and clinical studies have shown the benefits of a diet rich in fish containing ω-3 PUFA, such as eicosapentaenoic acid (EPA) and docosahexaenoic acid (DHA) in reducing cardiovascular and neurologic diseases (Mozaffarian and Rimm, 2006; Barros et al., 2014). Ingestion of fatty acids in the optimal ω-3:ω-6 PUFA ratio in addition to the consumption of marine antioxidant carotenoids, could lead to better cognitive and neuromotor health since antioxidants may reduce the oxidative stress caused by oxidized derivatives of PUFA which react with nervous cells (Barros et al., 2014).
Fish supply for human consumption is increasing at an annual rate of ca. 3.2%, with 42% of the 158 million tons consumed in 2012 being supplied by aquaculture (FAO, 2014). According to FAO, aquaculture production in that year reached 90.4 million tons, including 66.6 million tons of fish and 23.8 million tons of aquatic algae, worth respectively 137.7 billion and 6.4 billion dollars. The health driven increase in fish consumption (from 9.9 kg per capita in 1960s to 19.2 kg in 2012, FAO, 2014) has been accompanied by an increased intake of antioxidant compounds such as carotenoids. The carotenoid market attained 1.5 billion dollars in 2014 and is expected to grow at an annual growth rate of 3.9% until 2019 (BCC_research, 2015). Ensuring that aquaculture supplies fish and shellfish containing the suitable amount of PUFA and carotenoids for human nutrition will be of high importance in the future as the reported health benefits of carotenoids increase.
In the present review we discuss the functions and sources of carotenoids for aquatic organisms and the relevance of carotenoids to human health. We highlight carotenoid importance for aquaculture and suggest the best forms of its incorporation into diets used in aquaculture to achieve the desired coloration of gonads, muscle and integument.
Relevance of Carotenoids to Human Health
The importance of the antioxidant properties of carotenoids to human health derives from their potential to reduce the oxidative stress linked to various ROS related disorders, including various types of cancer, neurological and cardiovascular diseases (Voutilainen et al., 2006; Fiedor and Burda, 2014). Carotenoids (especially α-carotene, β-carotene and β-cryptoxanthin) are also important precursors of vitamin A which is essential for normal growth and development, immune system function, and vision. Since carotenoids have the ability to absorb light in the visible range of the spectrum, carotenoids like lutein and zeaxanthin efficiently absorb blue light reducing the amount that reaches the critical visual structures and thus protect the eye from light-induced oxidative damage (Krinsky et al., 2003). Carotenoids appear to have a role in facilitating intercellular communication by increasing the expression of the gene encoding a connexin protein which forms pores in cell membranes (Bertram, 1999; Livny et al., 2002). Cells communicate through these pores by exchanging small molecules.
Several studies have shown a direct correlation between a human diet rich in fruit, berries and vegetables containing high levels of carotenoids, and higher levels of serum carotenoids and lower risk of disease including several forms of cancer, cardiovascular and degenerative diseases (Virtamo et al., 2003; Wang et al., 2013; Fiedor and Burda, 2014). The ascribed health benefits of these natural foods cannot be unequivocally associated to the intake of carotenoids alone, since other antioxidant compounds present in the foods are ingested, such as vitamins, folate, and phenolic compounds (Virtamo et al., 2003; Voutilainen et al., 2006). Epidemiological evidence shows that people who ingest more dietary carotenoids exhibit a reduced risk for cancer, yet results from intervention trials indicate that the supplemental β-carotene does not prevent cardiovascular diseases or cancer (Chatterjee et al., 2012; Karppi et al., 2012; Wang et al., 2013), and may enhance lung cancer incidence and mortality among smokers (Omenn et al., 1996; Russell, 2002; Virtamo et al., 2003). The inefficient use of β-carotene as chemopreventive agent has been tentatively linked to cell-specific effects of the carotenoid in in vitro cultures: absence of effect on gap junctional intercellular communication (GJIC), Cx43 expression and growth of non-transformed or neoplastic murine lung epithelial cells, and enhanced GJIC, Cx43 expression and reduced growth of murine fibroblasts (Banoub et al., 1996). Nevertheless, these overall null or negative effects of this carotenoid supplementation may, on the one hand, result from the depletion in ROS caused by a high concentration of oxygen scavengers. ROS must be kept in balance in the human body where they have several regulatory functions and act as regulatory mediators in signaling processes and gene expression (Palmer and Paulson, 1997; Dröge, 2002), as anti-tumorigenic agents (Storz, 2005), and have anti-inflammatory and immunoregulatory roles (Hultqvist et al., 2009). On the other hand, β-carotene may serve either as an antioxidant or as a prooxidant, depending on its intrinsic properties as well as on the redox potential of the biological environment in which it acts (Palozza et al., 2003). Additionally, studies on animals have shown that high doses of β-carotene result in lung cancer, with or without smoke exposure, although the pathology was worse in the former case (Russell, 2004). This is apparently caused by an induction of the activity of the cytochrome P450 (CYP), resulting in lower retinoic acid levels in tissue and diminished retinoid signaling which, by a cascade of events, leads to tumor cell proliferation.
It is noteworthy that all these studies where carotenoid supplements had no effect on health or were correlated to an increase in the number of tumor cases were performed by using β-carotene as dietary supplement. Clinical trials using carotenoid supplements other than β-carotene are conspicuously scarce in the scientific literature and their results are largely inconclusive. Lycopene serum or tissue content has been shown to be inversely associated with the risk of atherosclerosis (Klipstein-Grobusch et al., 2000) and of prostate cancer (Lu et al., 2001) yet, it is still not determined whether tomatoes or lycopene alone are responsible for this health benefit (Giovannucci et al., 1995; Story et al., 2010). Similarly, cataract formation and macular degeneration were significantly reduced by dietary intake of fruits and vegetables rich in lutein and zeaxanthin (Brown et al., 1999; Johnson et al., 2000; SanGiovanni and Neuringer, 2012). Lutein and zeaxanthin which are selectively accumulated in the retina, and are particularly abundant in the macula, seem to offer protection against age-related macular degeneration (AMD) (Snodderly, 1995). Similarly to trials with lycopene, serum lutein concentration increased after 1 month in lutein supplementation of the diet, yet no significant changes in visual acuity or visual field tests in persons aged 60 years and older were observed (Rosenthal et al., 2006; Huang et al., 2008). Astaxanthin, a carotenoid reported by in vitro studies to have free radical antioxidant activity several fold higher than that of β-carotene and α-tocopherol (Kurashige et al., 1990) has been tested as a dietary supplement in some studies. Astaxanthin has been reported to prevent the progression of diabetic nephropathy mainly through the ROS scavenging effect in mitochondria of mesangial cells (Manabe et al., 2008) and to decrease oxidative stress and inflammation and enhance immune response (Park et al., 2010; Miyachi et al., 2015). Extract of the green alga Haematococcus pluvialis rich in astaxanthin inhibited the growth of human colon cancer cells by arresting cell cycle progression and promoting apoptosis (Palozza et al., 2009). It has been noted that the bioavailability of astaxanthin as well as other carotenoids was increased with the supplement of lipid based formulations (Odeberg et al., 2003). The generality of trials, so far, point to health advantages in using carotenoids not as dietary supplements but rather in the form of functional foods that “are consumed as part of a normal diet and deliver one or more active ingredients (that have physiologic effects and may enhance health) within the food matrix” (Zeisel, 1999).
Carotenoids in the Marine Environment
Importance of Light Radiation for Carotenoid Production and Accumulation in Food Webs
Carotenoids are synthesized by photosynthetic organisms, bacteria and fungi, whilst in general, animals must obtain the necessary carotenoids either directly from the diet or modify the dietary carotenoid precursors through metabolic reactions to fit their requirements. These metabolic modifications are mainly oxidation, reduction, cleavage of double bonds or epoxy bonds, and translation of double bonds (Matsuno, 1989). The physiological functions of carotenoids in photo-autotrophs are related to the photosynthetic process. Carotenoids participate in the collection of light energy (photosynthetic active radiation, PAR, λ = 400–700 nm) and its transfer to chlorophyll for photosynthesis, and act as photo-protectors by both dissipating the excessive energy that could damage the cholophyll molecule, and inhibiting the formation of ROS (Mimuro and Katoh, 1991; Demmig-Adams et al., 1996). In heterotrophic microorganisms, carotenoid production is not as essential as in photoautotrophic microbes which need protection from direct light (Goodwin, 1980), yet carotenoids are widely distributed in extremophiles. In these microorganisms, carotenoids are often accumulated in membranes where they contribute to its stabilization under extreme conditions (Yokoyama et al., 1995). In bacteria, carotenoids have been linked to tolerance and adaptation to several stressful conditions including salinity, radioactive compounds, pollutants, pH, temperature and UV radiation. Although present in several autotrophs, the major source of carotenoids to marine food webs is considered to be from photosynthetic organisms.
UV radiation induces photochemical degradation of dissolved organic matter and can ultimately damage the DNA of several marine organisms including bacteria, cyanobacteria and phyto- and zooplankton (Tedetti and Sempéré, 2006). The effects of this radiation are modulated by (i) its penetration in the water column (Table 1), (ii) the duration of exposure, (iii) the vulnerability and repair mechanisms presented by the organisms (Mora et al., 2005; Tedetti and Sempéré, 2006). The depth at which e.g., UV-B radiation is reduced to 10% of the surface value (Z10%) ranges from 17 m in open ocean to 3 m in coastal waters, according to water's optical properties which are a function of turbidity, dissolved organic matter (DOM), phytoplankton abundance and suspended particles (Tedetti and Sempéré, 2006). Coastal areas and shallow waters close to the continental shelf have, in general, lower transparency than open ocean water due to inorganic suspended matter and dissolved organic compounds. In Northern European coastal waters, UV radiation Z10% (λ = 310 nm) varies from 0.08 to 10.4 m (Aas and Højerslev, 2001).
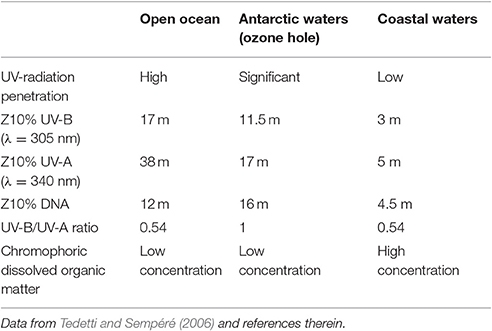
Table 1. UV-radiation (UV) characteristics in open ocean, Antarctic and coastal waters: 10% irradiance depth (Z10%) for UV-A, UV-B, and DNA damage effective dose, UV-B/UV-A ratio offers an indication of the concentration of stratospheric ozone and colored dissolved organic matter, known to hamper the penetration of radiation.
Aquaculture facilities built close to sea shore should take local UV radiation values and water turbidity into account. Although the direct effects of exposure to UV radiation are likely minimal within the context of all the other environmental factors that cause very high levels of egg and larval mortality in marine organisms, studies have shown that UV-induced egg mortality could be as high as 32.5% for the planktonic copepod Calanus finmarchicus and considerably less (1.2%) for the Atlantic cod Gadus morhua in the waters of the estuary and Gulf of St. Lawrence, Canada (Browman et al., 2000). Other direct effects of UV exposure on fish eggs or larvae include malformation (Dong et al., 2007), retarded growth (Jokinen et al., 2008), lesion of skin, eyes, and brain (Blazer et al., 1997; McFadzen et al., 2000), and weakened immune system (Markkula et al., 2005, 2006). These pernicious effects may be higher for fish larvae that are often present in surface waters since UV-B (λ = 280–320 nm) exposure induces DNA damage in a variety of fish larvae such as Atlantic cod, northern anchovy Englausis mordax, icefish Cephalus aceratus and Japanese medaka Oryzias latipes (see Fukunishi et al., 2012 for references). UV-B exposure is also likely to weaken fish larvae by inducing physiological stress and damage as suggested by a study that observed a decrease in cod larval escape response to predators, which can increase the impact of predation on fish recruitment success (Fukunishi et al., 2012). The extent of UV induced damage is largely modulated by the presence of competent photoprotection by e.g., carotenoids, mycosporine-like amino acids (MAAs) and other pigments, and photorepair systems.
Carotenoid pigments are known to offer protection from UV radiation to zooplankton crustaceans in transparent lakes (Hairston, 1976). However, colored pigments also make the animal more conspicuous, increasing the risk of being captured by visual predators, such as pelagic fish and fish larval stages. In fact, the carotenoid content in marine pelagic copepods is much lower (by an order of magnitude) than in freshwater species, especially in those under low visual predation pressure from fish (Table 2) while the content of other natural sunscreen compounds such as the transparent MAAs is similar (Hylander et al., 2014). It has been estimated that zooplankton mortality rates due to predation may reach 10% per day in the ocean (Kiørboe and Hirst, 2008; Hylander et al., 2014) and fish predation on marine pelagic copepods may be responsible for more than 69% of the mortality rate of adult males (Hirst et al., 2010). Therefore, a preference for the non-pigmented MAAs over carotenoid pigments to provide protection against UV radiation without increasing visual predation risk may be an adaptation of wild marine copepods to avoid predation. The plasticity of this preference was evidenced in a study by Hylander and Hansson, where it was shown that the carotenoid content level of zooplankton increased ca. 30% when zooplankton were exposed to UV radiation in the absence of predator cues (Hylander and Hansson, 2013).
In fish, protection from UV radiation is largely provided by pigments other than carotenoids (Armstrong et al., 2000). Integument pigments of fish are stored in a variety of pigment cell types or chromatophores, generally classified into six categories based on their hue: melanophores (black or brown, containing melanin), iridophores (iridescent, containing mainly purines), xanthophores (yellow, containing pteridines, and carotenoids), erythrophores (red containing pteridines and carotenoids), leucophores (white, containing mainly purines), and cyanophores (blue, containing undefined pigments) (Goodwin, 1980; Fujii, 1993, 2000; Kodric-Brown, 1998). Based on pigment absorbance spectra of chromatophores, it is assumed that leucophores and melanophores provide most of the UV photoprotection to fish since pteridines and melanin have strong absorbances in the UV region of the spectrum. Within the xanthophores and erythrophores, pteridines are assumed to offer somewhat greater UV protection to fish than carotenoids, since pteridines absorb more light than carotenoids in the UV portion of the spectrum (Armstrong et al., 2000). MAAs are also taken up by fish, they are accumulated in UV-radiation sensitive tissues such as the eye lenses (Mason et al., 1998).
Carotenoid Pigments in the Plant-Animal Interface
Many aquatic animals deposit carotenoids obtained mainly from photo-autotrophs (phytoplankton and microalgae) in their gonads, carapaces, muscle, and integuments. These carotenoids are either directly accumulated without modification or are converted into other carotenoids prior to deposition in tissues. Certain animals (e.g., crustaceans) exhibit little accumulation of dietary carotenoids while others (e.g., echinoderms and molluscs) present tissues that largely reflect their dietary intake (Figure 2).
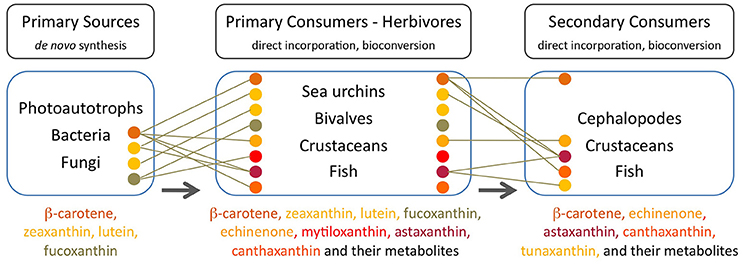
Figure 2. Major carotenoids present in trophic compartments of the marine food web. Each carotenoid is indicated by a colored circle corresponding to the name in the ordered list at the bottom of each compartment. Lines indicate the major source of each carotenoid in consumers. Arrows indicate the direction of carotenoid transfer and metabolic transformation through the food web, from primary sources at its base to the higher trophic levels (Goodwin, 1984; Maoka, 2011 and references therein).
In sea urchins (echinoderm), the pigment profile of the gut wall largely reflects the dietary intake of algae while the gonads do not reflect the composition of dietary carotenoids. Sea urchins that feed on Laminaria species present an abundance of absorbed lutein, β-carotene, fucoxanthin (degraded to e.g., fuxocanthinol) and some metabolized derivatives e.g., echinenone or isozeaxanthin (Symonds et al., 2007). When feeding on Rhodophycea algae (i.e., lacking fucoxanthin), the predominant pigments in the gut wall of sea urchins are chlorophyll-a, lutein and zeaxanthin (Haug et al., 2003). Echinenone is the primary carotenoid in the gonads of edible sea urchins (Matsuno and Tsushima, 2001) and its content is limited by the availability, uptake and bioconversion of β,β-carotene from natural dietary sources (Tsushima, 2007; Hagen et al., 2008). Symonds et al. (2007) proposed two alteranative routes of conversion of all-trans β-carotene into 9′-cis-echinenone noting that neither the all-trans nor 9′-cis forms of echinenone occur in the natural diet of wild Paracentrotus lividus and the presence of both forms of echinenone in the gut wall demonstrates their formation in situ via the metabolism of other dietary carotenoids. The levels of the 9′-cis form of echinenone is typically 10-fold greater than the all-trans form in the gonad (Symonds et al., 2007).
The gonadas of sea urchins exhibit a pattern of seasonal carotenoid depletion and replenishment in females and carotenoid retention in males (Hagen et al., 2008). Echinenone is preferentially incorporated into the eggs and therefore female urchins need to replenish their echinenone content on an annual/seasonal basis. Male sea urchins may not require annual carotenoid replenishment because sperm does not contain carotenoids and overall carotenoid content in male gonads tends to be seasonally stable. When males reach a saturation threshold for echinenone either the uptake or bioconversion of β,β-carotene is curtailed (Hagen et al., 2008). High-quality sea urchin gonads have a uniform yellowish-orange color which is a reflection of its carotenoid content, and substandard color is a direct result of carotenoid levels being either too low, leading to paleness or too high, leading to dark miscoloration (i.e., dark red/brown). Paleness is the primary problem in gonad enhancement cultivation and occurs when gonad growth is higher than carotenoid deposition (Plank et al., 2002). Since sea urchins cannot synthesize carotenoids de novo, paleness results from an insufficient supply of dietary carotenoids (Pearce et al., 2003; Shpigel et al., 2006). Difficulties to improve gonad color partly result from the fact that carotenoid deposition is complicated by the bioconversion of dietary β,β-carotene to echinenone (Tsushima, 2007). A protein with a carotenoid-binding function, echinenone-binding protein (EBP) has been isolated and characterized from the gonad of the New Zealand sea urchin Evechinus chloroticus (Pilbrow et al., 2014). Secondary structure prediction and amino acid sequence homology analyses, led the authors to suggest that this EBP is a member of the fatty acid-binding proteins (FABPs) family which again stresses a connection between carotenoids and lipids in animal tissues.
Filter feeding molluscs, such as bivalves, accumulate carotenoids obtained both directly from their dietary microalgae (e.g., fucoxanthin, diatoxanthin, diadinoxanthin, and alloxanthin) or after modification through metabolic reactions (Liaaen-Jensen, 1998; Maoka and Akimoto, 2008). Metabolites derived from fucoxanthin like mytiloxanthin or crasssostreaxanthin are widely distributed in marine mussels and oysters (Matsuno, 1989; Liaaen-Jensen, 1998; Maoka and Akimoto, 2008). In contrast to the carotenoid composition of bivalves, astaxanthin and its esters originating from zooplankton are the major carotenoids in species of cephalopodes like octopus and cuttlefish (Maoka et al., 1989). Herbivorous crustacean zooplankton are an important entrance point of astaxanthin in the wider marine food web, mainly through predation by higher heterotrophs. Astaxanthin is the main pigment in the body and eggs of crustaceans and depletion of astaxanthin at the basis of the food web may decrease antioxidant protection at higher trophic levels and subject predatory fish to oxidative stress (van Nieuwerburgh et al., 2005).
Crustaceans such as copepods and shrimp can rapidly produce astaxanthin after absorption of precursors from dietary phytoplankton (mainly β-carotene and some zeaxanthin; Goodwin, 1980; Matsuno, 1989, 2001) after digestion, and little accumulation of astaxanthin precursors is observed in their body tissues (Dall et al., 1995; van Nieuwerburgh et al., 2005). Free astaxanthin can then be esterified into mono- or diesters by attachment of one or two fatty acids, respectively. Astaxanthin esters are the main astaxanthin forms accumulated in the tissues of zooplankton and probably protect storage lipids (Sommer et al., 2006) whilst free astaxanthin may be incorporated into cell membranes where it protects against lipid peroxidation and helps to maintain membrane structure (McNulty et al., 2007). In the exoskeleton of crustaceans, astaxanthin exists as carotenoproteins such as crustacyanin, and exhibits purple, blue to blue-black (e.g., lobster Homarus gammarus), and yellow colors. These colors have direct implications for the fitness and survival of many crustaceans via camouflage or mate selection (Wade et al., 2009; Ferrari et al., 2012).
Astaxanthin esters, in addition to their photoprotector properties and ability to quench singlet oxygen (Kobayashi and Sakamoto, 1999), of being particularly strong scavengers of free radicals preventing the peroxidation of PUFA, may also be used as metabolic energy source (Ringelberg, 1980), even if they only contribute to ca. 2% of the total lipid content of copepods (Hairston, 1979). During the rapid combustion of lipids in upwardly migrating copepods, astaxanthin may even act as physiological “replacement” of oxygen as an electron acceptor (Łotocka et al., 2004). Recently, Schneider et al. related carotenoid accumulation in copepods to lipid metabolism and reproduction rather than to UV-protection (Schneider et al., 2016). The authors observed that the seasonal variability in total astaxanthin content of the copepods was characterized by two periods of net loss that coincided with peaks of egg production in spring and summer leading to minimum astaxanthin content in fall when the highest predation pressure by young-of-the-year fish was observed. The study results suggest that accumulation of astaxanthin in copepods is strongly related to lipid metabolism but not to UVR-photoprotection, and that seasonal changes of fatty acids and carotenoids are connected to the reproduction cycle. Such suggestions agree with previous observations that report that the most important function of astaxanthin in copepods is that of an antioxidant for protecting lipids from peroxidation (Bandaranayake and Gentien, 1982; Terao, 1989).
Herbivorous fish may derive their pigments directly from autotrophs (Choat and Clements, 1998) and freshwater Cyprinidae fish can synthesize (3S,3′S)-astaxanthin from zeaxanthin by oxidative metabolic conversion (Liaaen-Jensen, 1998; Schiedt, 1998; Matsuno, 2001). Nevertheless, fish generally feed on a variety of food items and obtain carotenoids from their heterotrophic prey.
Carotenoids in Fish
Many fish species accumulate carotenoids in their integuments and gonads, and members of the Salmonidae family have the ability to accumulate astaxanthin in muscle. Astaxanthin is widely distributed in both marine and freshwater fish while tunaxanthin, responsible for the bright yellow color in the fins and skin, is widely distributed in fish belonging to the order Perciformes (Maoka, 2011). In Perciformes, tunaxanthin is metabolized from astaxanthin via zeaxanthin (Schiedt, 1998; Matsuno, 2001). Other carotenoids are present in particular groups of fish or as minor carotenoids (see Maoka, 2011). Unlike the freshwater Cyprinidae fish which can synthesize astaxanthin from zeaxanthin, Perciformes and Salmonidae lack the ability to synthesize astaxanthin from other carotenoids and must directly obtain it from their prey (Liaaen-Jensen, 1998; Schiedt, 1998; Matsuno, 2001).
In the integument of fish, carotenoid pigments responsible for bright red, orange and yellow hues are stored in the yellow xanthophores or red erythrophores (Goodwin, 1980; Kodric-Brown, 1998). The predominant carotenoid in the integument of fish is a characteristic of the species and the most common are tunaxanthin (yellow), lutein (greenish-yellow), β-carotene (orange), α- and β-doradexanthins (yellow), zeaxanthin (yellow-orange), canthaxanthin (orange-red), astaxanthin (pink-red), echinenone (red), and taraxanthin (yellow). Integumentary carotenoids may contribute to some photoprotection, yet they are mainly used for camouflage and signaling, especially as breeding color. Males of sockeye salmon, Oncorhynchus nerka, in experiments in the wild were observed to preferentially select and spawn with artificial female models with a red hue, the predominant color of females (Foote et al., 2004). Cichlid female fish may use the carotenoid coloration to evaluate the health and vigor of their prospective mates and pigmented tissues can thus be important traits in speciation in wild populations (Boughman, 2002). Carotenoid based skin coloration is an example of an honest signal of mate quality since carotenoids ascribe health benefits to vertebrates as precursors for vitamin A and transcription regulators, antioxidants, free-radical scavengers, immune-system stimulants and cancer inhibitors, and are mobilized from muscle to ovaries which suggest a function in reproduction (Kodric-Brown and Brown, 1984; Ando et al., 1986).
Aquatic animals conspicuously accumulate carotenoids in their gonads which is assumed to be essential for their reproduction and the successful development of their eggs and early larval stages. Astaxanthin supplementation in cultured salmon and red sea bream increased ovary development, fertilization, hatching and larval growth (Torrissen and Christiansen, 1995). In the case of the sea urchin, supplementation with β-carotene, which was metabolized to echinenone, also increased reproduction and the survival of larvae (Tsushima et al., 1997).
Astaxanthin as the Main Carotenoid in Marine Organisms
The red pigment astaxanthin is the most abundant carotenoid in marine organisms such as zooplankton, shrimp, and salmonoid fish (Table 3), providing pigmentation and antioxidant protection (Cadenas and Packer, 1996; Maoka, 2011). Astaxanthin, like other carotenoids, is not produced by de novo synthesis by aquatic heterotrophs that must either acquire it directly through their diet or modify the dietary carotenoid precursors through metabolic reactions to fit their requirements. In the wild, Perciformes and Salmonidae obtain astaxanthin from dietary crustacean zooplankton and the particular dietary requirements of each fish type must be taken into account to maintain the desired characteristics under culture conditions. The retention of dietary carotenoids by muscle tissues in salmonoids varies between 2 and 22%, but no beneficial effect may be attained by simply increasing astanxanthin concentration in dry extruded fish feed above 60–70 mg/kg (Torrissen, 1996; Rajasingh et al., 2006). However, by increasing the lipid level in the diet, carotenoid retention could be increased leading to higher astaxanthin concentrations in fish muscle.
Astaxanthin is the main pigment in the body and eggs of herbivorous crustacean zooplankton which are an important entrance point of this carotenoid in the wider marine food web, mainly through predation of higher heterotrophs. Depletion of astaxanthin at the basis of the food web may decrease antioxidant protection at higher trophic levels and subject predatory fish to oxidative stress (van Nieuwerburgh et al., 2005). Curiously, low accumulation of astaxanthin with increasing food availability was observed with the calanoid copepod Acartia bifilosa, suggesting a food-dependent decrease in assimilation efficiency (Holeton et al., 2009). This could have ecological relevance during eutrophication, which occurs in many coastal areas: phytoplankton blooms may favor copepod egg production and population growth but at the expense of the astaxanthin content in each copepod, thus affecting zooplanktivores such as fish. Astaxanthin is usually esterified with fatty acids probably to protect storage lipids (Sommer et al., 2006) whilst free astaxanthin may be incorporated into cell membranes where it protects against lipid peroxidation and helps to maintain membrane structure (McNulty et al., 2007). These properties are important to maintain fish fitness.
Carotenoids in Aquaculture
Color in fish is a quality criterion used by consumers to assess the nutritive value, health, freshness and taste of salmonids, crustaceans and other farmed fish. The market value of e.g., salmon, red porgy or red sea bream is dependent on the color of the fish as well as the exoskeleton and muscular ephitelium of shrimps, lobster and other crustacean carapaces and molluskan gonads. In aquaculture, managing the external characteristics that give added value to cultured fish is therefore of paramount importance.
In wild salmonids, carotenoid levels may range from 26 to 39 mg/kg for sockeye salmon (Oncorhynchus nerka) to 8–9 mg/kg for chinook salmon (O. tshawytscha) and more than 3 mg/kg in rainbow trout (O. mykiss), depending on preys and environmental conditions (Meyers, 1994). In the European Union, canthaxanthin is currently used alone or together with astaxanthin up to a concentration of 25 mg per kg of feed for salmonids (EFSA, 2007). In terms of organoleptic properties for commercial market, a minimum concentration of carotenoids (canthaxanthin or astaxanthin) of 8 mg/kg flesh is required for salmonids and a maximum of 13.7 mg/kg is permitted (European Commission, 2002).
It is recognized that carotenoid retention in fish is promoted when the carotenoids are provided together with lipids. Contrary to proteins and carbohydrates, lipids seem to significantly affect pigmentation (Bjerkeng, 2000). This results from the fact that, as hydrophobic compounds, carotenoids are not easily solubilized in the aqueous environment of the gastrointestinal track of fish, and association to lipids may facilitate digestion, absorption, and transport mechanisms (Castenmiller and West, 1998). When rainbow trout was grown using pigments from Chlorella vulgaris or commercially available pigments at 15 and 20% of lipids, pigment concentration in the muscle was higher when fed on the high-fat diet (Gouveia et al., 1998). The difference in deposition and retention of carotenoids in muscle between the two lipid diets increased with time: deposition increased 10–20% at week 3 and 30–40% at week 6 whilst retention increased 10–15 and 30% after the same period.
When astaxanthin from the micro-algae H. pluvialis (at a concentration 32 mg/kg) and synthetic asthaxantin were fed to rainbow trout using fish oil or olive oil, the fish presented higher serum concentrations of astaxanthin when fed on synthetic carotenoid and olive oil (Choubert et al., 2006). Muscle astaxanthin levels were lower for fish fed with algae after 6 weeks and no significant differences were observed between trouts fed on olive or fish oil. Nevertheless, astaxanthin retention in muscle was higher in fish fed on the diet with combined synthetic astaxanthin and olive oil than on fish eating synthetic astaxanthin and fish oil.
One of the biggest problems in aquaculture is related to the feeding of fish during the first larval stages when their incomplete developments results in the inability to digest some food and their small size hampers eating and swallowing of large food particles (Rønnestad et al., 2013). Appropriate feeding protocols for each species should be planned, including the amount of food offered and the periodicity of feeding, as well as the optimum temporal sequence for different prey types during development when live feed is provided (Rønnestad et al., 2013).
Live Feeds for Aquaculture
Live food has several advantages over formulated larval food, including the stimulation of the natural instinct of larvae for catching preys and of not polluting the water of tanks when not completely consumed (Hertrampf and Piedad-Pascual, 2000). Among the organisms used for feed are those belonging to phytoplankton and zooplankton.
Since the end of the nineteenth century, France, Canada, USA and UK have been using hatcheries to propagate commercially interesting species such as cod (G. morhua), haddock (Melanogrammus aeglefinus), turbot (Scophthalmus maximus), winter flounder (Pleuronectes americanus), and lobster (Homarus sp.) (Bengtson, 2007). However, they were released into the ocean during the prolarva stage, due to the lack of convenient live feed for their postlarval stages, resulting in very high mortality rates. Two major developments in the 1930s were of paramount importance to the development of modern aquaculture: the discovery that the nauplii of Artemia could feed both freshwater and marine larval fish (Seale, 1933); and the research on the culture of kuruma prawn, Penaeus japonicus, in Japan (Mock, 1972). Since the dormant cysts of Artemia can be stored for long periods in cans, requiring only 24 h of incubation before hatching, this makes them one of the most convenient, easy to produce, live food for aquaculture, although nutritional quality variability may be quite high geographically and temporally (Bengtson et al., 1991), especially at the level of essential highly unsaturated fatty acids (Merchie, 1996). Curiously, the carotenoid content of Artemia varies during the life cycle: hydration of the cysts causes a reversible all-trans to cis-canthaxanthin conversion; after hatching of cysts, cis-canthaxanthin is gradually isomerized to the all-trans isomer; the content in the cis-isomer decreases to nearly zero in Artemia fed on algae but reappears in females at an advanced stage of the reproductive cycle, with the all-trans-isomer, and its precursors echinenone and β-carotene, remaining throughout the life cycle (Nelis et al., 1988). It was hypothesized, by the authors of the study, that the cis-isomer may possess the stereochemical conformation required to provide a “lock” on certain proteins in the embryo so that they are not digested. After hatching, this lock would have to be removed to allow their consumption by the nauplius. The cis-isomer, once dissociated from the protein would spontaneously be converted to the more stable all-trans form. Nevertheless, further studies are necessary to fully elucidate the role of both isomers during the life cycle of Artemia.
Since marine fish and crustacean require PUFA for growth and survival, rotifers and Artemia nauplii used to feed larval fish are usually enriched with yeast and algae supplemented with PUFA and xanthophylls. The heterotrophic marine protists thraustochytrids have been successfully used as source of docosahexaenoic acid (DHA) and other PUFA, as well as carotenoids, thus reducing the enrichment steps (Raghukumar, 2008). Screening for the best producing marine protist strains has resulted in the selection of strains that produce at least 50% of the biomass as lipids, of which at least 25% as DHA, and a yield of at least 5 g/L (Raghukumar, 2008) and, by improving the extraction of carotenoids, yields of 29–30.5 μg/g have been reached (Armenta et al., 2006).
The lutein content of the rotifer Brachionus sp. could be increased by feeding the individuals with lutein-rich freeze-dried cells of the freshwater microalga Muriellopsis sp. in seawater (van Bergeijk et al., 2013). Curiously, the lutein content of the rotifers was higher when the enrichment periods lasted only 2–2.5 h than during 13.5–24 h periods. The lutein content (0.06–0.16 mg/g) of the rotifers was low when compared to the lutein content of Muriellopsis sp. (ca. 3 mg/g) but it was in the range of natural copepods, providing adequate concentration for marine fish larvae. Although rotifers are not the natural diet of marine fish larvae, as opposed to copepods, they are well ingested by larvae and easily cultivated at high density and low cost, being commonly used as copepods substitutes for first feeding of marine fish larvae (Lubzens and Zmora, 2007; Hamre, 2016). However, the nutrient composition of commercially available rotifer diets varies significantly, which results in large variation in the nutritional composition of rotifers fed to marine fish larvae in industrial aquaculture (Hamre, 2016). When comparing the nutrient profiles of Brachionus sp. diets used in commercial marine fish hatcheries, raging from culture to enrichments diets, Hamre (2016) found that the composition in fatty acids and vitamins C and E are, in general, appropriate but the concentration of vitamin A, iodide and selenium could vary significantly (Hamre, 2016).
The Schizochytrium sp. strain KH105, producing both the polyunsaturated DHA and EPA, and the carotenoids canthaxanthin and astaxanthin, was successfully incorporated by rotifers and Artemia nauplii (Yamasaki et al., 2007). The carotenoid content in the body of rotifers increased 35% at a maximum KH105 cell concentration of 400 ppm for 24 h, whilst in Artemia nauplii the canthanxanthin content increased from 0.70 to 1.19 mg/g when KH105 was supplied at 500 rpm.
The size of rotifers and easy culturing makes them an ideal prey for fish larvae although its insufficient nutritional quality directs the search for more suitable alternatives in aquaculture. Studies at experimental scale have shown that the survival of Eugerres brasilianus was more than double when the larvae were fed on copepods (12.5%) than on rotifers (6%) (Molejón and Alvarez-Lajonchère, 2003). At pilot scale, the larvae survival rate reached 30–50% when feeding on copepods and the mean harvest density was of 6.1 juveniles/L, whilst in the control tank where no copepods were provided, larval survival reached 12–18% and the mean harvest density was of 5.1 juvenile/L. The study suggests that pelagic copepods with short life cycles and high growth rate such as Oithona oculata could be cultured at high-yields and low cost, allowing the production of stress-resistant larvae. Copepods contain less essential amino acids (30–32%) than rotifers (43%) in the free amino acid fraction, but present higher content of important fatty acids such as DHA (23–32% in copepods; 7% in rotifers) and higher DHA/EPA ratio (1.35–1.63 in copepods; 0.54 in rotifers) (Drillet et al., 2006). Marine copepods are also known to contain enzymes like amylases, proteases, exonucleases and esterases, which are mostly lacking in earlier fish larval stages and are essential to improve prey digestibility, and thus fish larval development and survival (Munilla-Moran et al., 1990; Schipp et al., 1999; Perumal et al., 2015).
Future Perspectives and Considerations
So far, clinical trials on the effects of carotenoids on human health have been focused almost exclusively on β-carotene. Studies that test various carotenoids to fully understand the consequence of carotenoid supplementation on human health are necessary. The effect of various carotenoids on the immune and endocrine systems, their metabolism, mechanism of action, and the interaction of carotenoids in vivo should be elucidated.
It is also of utmost importance for the aquaculture industry to understand and control several parameters that have been found to influence the development and pigmentation of fish, such as: digestibility of carotenoids, which is apparently influenced by feed intake (Rørvik et al., 2010) and temperature (Ginés et al., 2004); carotenoid retention by tissue, which depends on fish species; metabolic turnover of carotenoids and physiological effects of carotenoid intake during the growth stages of the breeding species. Nutritional formulations should consider possible effects not only on pigmentation but also on the innate immune system and on muscle, skeleton and neural development of fish. Increasing concerns and awareness of issues related to health and wellness by human consumers increase the demand of correct nutritional attributes, sensory properties and correct fish pigmentation. Producers of fish in aquaculture facilities must take such factors into consideration while bearing in mind all the practicalities of fish production, processing and harvesting conditions.
Author Contributions
The manuscript was written, revised and approved for publication by both authors.
Conflict of Interest Statement
The authors declare that the research was conducted in the absence of any commercial or financial relationships that could be construed as a potential conflict of interest.
Acknowledgments
CdC acknowledges Fundação para a Ciência e a Tecnologia, I.P. (FCT), Portugal, for financial support under program “FCT Investigator 2013” (IF/01203/2013/CP1163/CT0002). The work was partially funded by national funds through FCT in the frame of the projects UID/BIA/00329/2013 and UID/BIO/04565/2013.
References
Aas, E., and Højerslev, N. K. (2001). Attenuation of ultraviolet irradiance in North European coastal waters. Oceanologia 43, 139–168.
Anbazahan, S. M., Mari, L. S., Yogeshwari, G., Jagruthi, C., Thirumurugan, R., Arockiaraj, J., et al. (2014). Immune response and disease resistance of carotenoids supplementation diet in Cyprinus carpio against Aeromonas hydrophila. Fish Shellfish Immunol. 40, 9–13. doi: 10.1016/j.fsi.2014.06.011
Ando, S., Takeyama, T., and Hatano, M. (1986). Transport associated with serum vitellogenin of carotenoid in chum salmon (Oncorhynchus keta). Agric. Biol. Chem. 50, 557–563. doi: 10.1080/00021369.1986.10867435
Antón, J., Rosselló-Mora, R., Rodríguez-Valera, F., and Amann, R. (2000). Extremely halophilic bacteria in crystallizer ponds from solar salterns. Appl. Environ. Microbiol. 66, 3052–3057. doi: 10.1128/aem.66.7.3052-3057.2000
Armenta, R. E., Burja, A., Radianingtyas, H., and Barrow, C. J. (2006). Critical assessment of various techniques for the extraction of carotenoids and co-enzyme Q10 from the Thraustochytrid strain ONC-T18. J. Agric. Food. Chem. 54, 9752–9758. doi: 10.1021/jf061260o
Armstrong, T. N., Cronin, T. W., and Bradley, B. P. (2000). Microspectrophotometric analysis of intact chromatophores of the Japanese medaka, Oryzias latipes. Pigm. Cell Res. 13, 116–119. doi: 10.1034/j.1600-0749.2000.130210.x
Asker, D., Beppu, T., and Ueda, K. (2007). Unique diversity of carotenoid-producing bacteria isolated from Misasa, a radioactive site in Japan. Appl. Microbiol. Biotechnol. 77, 383–392. doi: 10.1007/s00253-007-1157-8
Bandaranayake, W. M., and Gentien, P. (1982). Carotenoids of Temora turbinata, Centropages furcatus, Undinula vulgaris and Euchaeta russelli. Comp. Biochem. Physiol. B Biochem. Mol. Biol. 72, 409–414. doi: 10.1016/0305-0491(82)90219-X
Banoub, R. W., Fernstrom, M., and Ruch, R. J. (1996). Lack of growth inhibition or enhancement of gap junctional intercellular communication and connexin43 expression by β-carotene in murine lung epithelial cells in vitro. Cancer Lett. 108, 35–40. doi: 10.1016/S0304-3835(96)04367-4
Barros, M. P., Poppe, S. C., and Bondan, E. F. (2014). Neuroprotective properties of the marine carotenoid astaxanthin and omega-3 fatty acids, and perspectives for the natural combination of both in krill oil. Nutrients 6, 1293–1317. doi: 10.3390/nu6031293
Bengtson, D. A. (2007). “Status of marine aquaculture in relation to live prey: past, present and future,” in Live Feeds in Marine Aquaculture, eds J. G. Støttrup and L. A. McEvoy (Oxford: Blackwell Science Ltd), 1–16.
Bengtson, D. A., Léger, P., and Sorgeloos, P. (1991). “Chapter 11. Use of Artemia as a food source for aquaculture,” in Artemia Biology, eds R. A. Browne, P. Sorgeloos, and C. N. A. Trotman (Boca Raton, Fl: CRC Press, Inc.), 255–285.
Bertram, J. S. (1999). Carotenoids and gene regulation. Nutr. Rev. 57, 182–191. doi: 10.1111/j.1753-4887.1999.tb06941.x
Bjerkeng, B. (2000). “Carotenoid pigmentation of salmonid fishes - recent progress,” in Avances en Nutrición Acuícola V. 2000. Memorias del Quinto Simposio Internacional de Nutrición y Tecnología de Alimentos, eds L. E. Cruz Suárez, D. Ricque Marie, M. Tapia Salazar, M. A. Olvera Novoa, and R. Civera Cerecedo (Mérida Yucatán; Monterrey: Universidad Autónoma de Nuevo León), 71–89.
Blazer, V. S., Fabacher, D. L., Little, E. E., Ewing, M. S., and Kocan, K. M. (1997). Effects of Ultraviolet-B radiation on fish: histologic comparison of a UVB-sensitive and a UVB-tolerant species. J. Aquat. Anim. Health 9, 132–143.
Boughman, J. W. (2002). How sensory drive can promote speciation. Trends Ecol. Evol. 17, 571–577. doi: 10.1016/S0169-5347(02)02595-8
Britton, G. (1995). Structure and properties of carotenoids in relation to function. FASEB J. 9, 1551–1558.
Browman, H. I., Rodriguez, C. A., Béland, F., Cullen, J. J., Davis, R. F., Kouwenberg, J. H. M., et al. (2000). Impact of ultraviolet radiation on marine crustacean zooplankton and ichthyoplankton: a synthesis of results from the estuary and Gulf of St. Lawrence, Canada. Mar. Ecol. Prog. Ser. 199, 293–311. doi: 10.3354/meps199293
Brown, L., Rimm, E. B., Seddon, J. M., Giovannucci, E. L., Chasan-Taber, L., Spiegelman, D., et al. (1999). A prospective study of carotenoid intake and risk of cataract extraction in US men. Am. J. Clin. Nutr. 70, 517–524.
Caramujo, M.-J., de Carvalho, C. C., Silva, S. J., and Carman, K. R. (2012). Dietary carotenoids regulate astaxanthin content of copepods and modulate their susceptibility to UV light and copper toxicity. Mar. Drugs 10, 998–1018. doi: 10.3390/md10050998
Castenmiller, J. J., and West, C. E. (1998). Bioavailability and bioconversion of carotenoids. Annu. Rev. Nutr. 18, 19–38. doi: 10.1146/annurev.nutr.18.1.19
Chatterjee, M., Roy, K., Janarthan, M., and Das, S. (2012). Biological activity of carotenoids: its implications in cancer risk and prevention. Curr. Pharm. Biotechnol. 13, 180–190. doi: 10.2174/138920112798868683
Choat, J. H., and Clements, K. D. (1998). Vertebrate herbivores in marine and terrestrial environments: a nutritional ecology perspective. Annu. Rev. Ecol. Syst. 29, 375–403. doi: 10.1146/annurev.ecolsys.29.1.375
Choubert, G., Mendes-Pinto, M. M., and Morais, R. (2006). Pigmenting efficacy of astaxanthin fed to rainbow trout Oncorhynchus mykiss: effect of dietary astaxanthin and lipid sources. Aquaculture 257, 429–436. doi: 10.1016/j.aquaculture.2006.02.055
Cogdell, R. J. (1978). Carotenoids in photosynthesis. Philos. Trans. R. Soc. B Biol. Sci. 284, 569–579. doi: 10.1098/rstb.1978.0090
Dall, W., Smith, D. M., and Moore, L. E. (1995). Carotenoids in the tiger prawn Penaeus esculentus during ovarian maturation. Mar. Biol. 123, 435–441. doi: 10.1007/bf00349222
Demmig-Adams, B., Gilmore, A. M., and Adams, W. W. (1996). Carotenoids 3: in vivo function of carotenoids in higher plants. FASEB J. 10, 403–412.
Dong, Q., Svoboda, K., Tiersch, T. R., and Monroe, W. T. (2007). Photobiological effects of UVA and UVB light in zebrafish embryos: evidence for a competent photorepair system. J. Photochem. Photobiol. B Biol. 88, 137–146. doi: 10.1016/j.jphotobiol.2007.07.002
Drillet, G., Jørgensen, N. O. G., Sørensen, T. F., Ramløv, H., and Hansen, B. W. (2006). Biochemical and technical observations supporting the use of copepods as live feed organisms in marine larviculture. Aquacult. Res. 37, 756–772. doi: 10.1111/j.1365-2109.2006.01489.x
Dröge, W. (2002). Free radicals in the physiological control of cell function. Physiol. Rev. 82, 47–95. doi: 10.1152/physrev.00018.2001
EFSA (2007). Opinion of the Scientific Panel on additives and products or substances used in animal feed (FEEDAP) on the Maximum Residue Limits for canthaxanthin in foodstuffs coming from animals fed with canthaxanthin used as a feed additive in accordance with Council Directive 70/524/EEC. EFSA J. 507, 1–19. doi: 10.2903/j.efsa.2007.507
European Commission (2002). Opinion of the Scientific Committee on Animal Nutrition on the Use of Canthaxanthin in Feedingstuffs for Salmon and Trout, Laying Hens, and Other Poultry. Brussels.
Ferrari, M., Folli, C., Pincolini, E., McClintock, T. S., Rössle, M., Berni, R., et al. (2012). Structural characterization of recombinant crustacyanin subunits from the lobster Homarus americanus. Acta Crystallogr. Sect. F Struct. Biol. Cryst. Commun. 68(Pt 8), 846–853. doi: 10.1107/s1744309112026103
Fiedor, J., and Burda, K. (2014). Potential role of carotenoids as antioxidants in human health and disease. Nutrients 6, 466–488. doi: 10.3390/nu6020466
Foote, C. J., Brown, G. S., and Hawryshyn, C. W. (2004). Female colour and male choice in sockeye salmon: implications for the phenotypic convergence of anadromous and nonanadromous morphs. Anim. Behav. 67, 69–83. doi: 10.1016/j.anbehav.2003.02.004
Fujii, R. (1993). Cytophysiology of fish chromatophores. Int. Rev. Cytol. 143, 191–255. doi: 10.1016/S0074-7696(08)61876-8
Fujii, R. (2000). The regulation of motile activity in fish chromatophores. Pigm. Cell Res. 13, 300–319. doi: 10.1034/j.1600-0749.2000.130502.x
Fukunishi, Y., Browman, H. I., Durif, C. M., Bjelland, R. M., and Skiftesvik, A. B. (2012). Effect of sub-lethal exposure to ultraviolet radiation on the escape performance of Atlantic cod larvae (Gadus morhua). PLoS ONE 7:e35554. doi: 10.1371/journal.pone.0035554
Gaillard, M., Juillet, C., Cézilly, F., and Perrot-Minnot, M.-J. (2004). Carotenoids of two freshwater amphipod species (Gammarus pulex and G. roeseli) and their common acanthocephalan parasite Polymorphus minutus. Comp. Biochem. Physiol. B Biochem. Mol. Biol. 139, 129–136. doi: 10.1016/j.cbpc.2004.07.001
Ginés, R., Valdimarsdottir, T., Sveinsdottir, K., and Thorarensen, H. (2004). Effects of rearing temperature and strain on sensory characteristics, texture, colour and fat of Arctic charr (Salvelinus alpinus). Food Qual. Prefer. 15, 177–185. doi: 10.1016/S0950-3293(03)00056-9
Giovannucci, E., Ascherio, A., Rimm, E. B., Stampfer, M. J., Colditz, G. A., and Willett, W. C. (1995). Intake of carotenoids and retino in relation to risk of prostate cancer. J. Natl. Cancer Inst. 87, 1767–1776. doi: 10.1093/jnci/87.23.1767
Gouveia, L., Choubert, G., Gomes, E., Rema, P., and Empis, J. (1998). Use of Chlorella vulgaris as a carotenoid source for rainbow trout: effect of dietary lipid content on pigmentation, digestibility and retention in the muscle tissue. Aquac. Int. 6, 269–279. doi: 10.1023/a:1009251714573
Hagen, N. T., Jørgensen, I., and Egeland, E. S. (2008). Sex-specific seasonal variation in the carotenoid content of sea urchin gonads. Aquat. Biol. 3, 227–235. doi: 10.3354/ab00084
Hairston, N. C. (1976). Photoprotection by carotenoid pigments in the copepod Diaptomus nevadensis. Proc. Natl. Acad. Sci. U.S.A. 73, 971–974.
Hairston, N. G. (1979). The adaptive significance of color polymorphism in two species of Diaptomus (Copepoda). Limnol. Oceanogr. 24, 15–37. doi: 10.4319/lo.1979.24.1.0015
Hamre, K. (2016). Nutrient profiles of rotifers (Brachionus sp.) and rotifer diets from four different marine fish hatcheries. Aquaculture 450, 136–142. doi: 10.1016/j.aquaculture.2015.07.016
Haug, E., Guillou, M., Connan, S., Goulard, F., and Diouris, M. (2003). HPLC analysis of algal pigments to define diet of sea urchins. J. Mar. Biol. Assoc. U.K. 83, 571–573. doi: 10.1017/S0025315403007495h
Hertrampf, J. W., and Piedad-Pascual, F. (2000). “Live Foods,” in Handbook on Ingredients for Aquaculture Feeds (Dordrecht: Springer), 241–254.
Hill, G. E., and Johnson, J. D. (2012). The vitamin A–redox hypothesis: a biochemical basis for honest signaling via carotenoid pigmentation. Amer. Nat. 180, E127–E150. doi: 10.1086/667861
Hirst, A. G., Bonnet, D., Conway, D. V. P., and Kiørboe, T. (2010). Does predation controls adult sex ratios and longevities in marine pelagic copepods? Limnol. Oceanogr. 55, 2193–2206. doi: 10.4319/lo.2010.55.5.2193
Holeton, C., Lindell, K., Holmborn, T., Hogfors, H., and Gorokhova, E. (2009). Decreased astaxanthin at high feeding rates in the calanoid copepod Acartia bifilosa. J. Plankton Res. 31, 661–668. doi: 10.1093/plankt/fbp016
Huang, L. L., Coleman, H. R., Kim, J., de Monasterio, F., Wong, W. T., Schleicher, R. L., et al. (2008). Oral supplementation of lutein/zeaxanthin and omega-3 long chain polyunsaturated fatty acids in persons aged 60 years or older, with or without AMD. Invest. Ophthalmol. Visual Sci. 49, 3864–3869. doi: 10.1167/iovs.07-1420
Hultqvist, M., Olsson, L. M., Gelderman, K. A., and Holmdahl, R. (2009). The protective role of ROS in autoimmune disease. Trends Immunol. 30, 201–208. doi: 10.1016/j.it.2009.03.004
Hylander, S., Grenvald, J. C., and Kiørboe, T. (2014). Fitness costs and benefits of ultraviolet radiation exposure in marine pelagic copepods. Funct. Ecol. 28, 149–158. doi: 10.1111/1365-2435.12159
Hylander, S., and Hansson, L.-A. (2013). Vertical distribution and pigmentation of Antarctic zooplankton determined by a blend of UV radiation, predation and food availability. Aquat. Ecol. 47, 467–480. doi: 10.1007/s10452-013-9459-7
Johnson, E. J., Hammond, B. R., Yeum, K.-J., Qin, J., Wang, X. D., Castaneda, C., et al. (2000). Relation among serum and tissue concentrations of lutein and zeaxanthin and macular pigment density. Am. J. Clin. Nutr. 71, 1555–1562.
Jokinen, I. E., Markkula, E. S., Salo, H. M., Kuhn, P., Nikoskelainen, S., Arts, M. T., et al. (2008). Exposure to increased ambient ultraviolet B radiation has negative effects on growth, condition and immune function of juvenile Atlantic salmon (Salmo salar). Photochem. Photobiol. 84, 1265–1271. doi: 10.1111/j.1751-1097.2008.00358.x
Karppi, J., Kurl, S., Laukkanen, J. A., and Kauhanen, J. (2012). Serum beta-carotene in relation to risk of prostate cancer: the Kuopio ischaemic heart disease risk factor study. Nutr. Cancer 64, 361–367. doi: 10.1080/01635581.2012.658949
Kiørboe, T., and Hirst, A. G. (2008). Optimal development time in pelagic copepods. Mar. Ecol. Prog. Ser. 367, 15–22. doi: 10.3354/meps07572
Klipstein-Grobusch, K., Launer, L. J., Geleijnse, J. M., Boeing, H., Hofman, A., and Witteman, J. C. (2000). Serum carotenoids and atherosclerosis. Atherosclerosis 148, 49–56. doi: 10.1016/s0021-9150(99)00221-x
Kobayashi, M., and Sakamoto, Y. (1999). Singlet oxygen quenching ability of astaxanthin esters from the green alga Haematococcus pluvialis. Biotechnol. Lett. 21, 265–269. doi: 10.1023/a:1005445927433
Kodric-Brown, A. (1998). Sexual dichromatism and temporary color changes in the reproduction of fishes. Am. Zool. 38, 70.
Kodric-Brown, A., and Brown, J. H. (1984). Truth in advertising: the kinds of traits favored by sexual selection. Amer. Nat. 124, 309–323.
Krinsky, N. I. (1968). “The protective function of carotenoid pigments,” in Photophysiology, ed A. C. Giese (New York, NY: Academic Press), 123–195.
Krinsky, N. I., Landrum, J. T., and Bone, R. A. (2003). Biologic mechanisms of the protective role of lutein and zeaxanthin in the eye. Annu. Rev. Nutr. 23, 171–201. doi: 10.1146/annurev.nutr.23.011702.073307
Kurashige, M., Okimasu, E., Inoue, M., and Utsumi, K. (1990). Inhibition of oxidative injury of biological-membrane by astaxanthin. Physiol. Chem. Phys. Med. NMR 22, 27–38.
Latscha, T. (1989). “The role of astaxanthin in shrimp pigmentation,” in Advances in Tropical Aquaculture, Workshop at Tahiti (Tahiti: Archimer).
Liaaen-Jensen, S. (1998). “Carotenoids in Food Chain,” in Carotenoids: Biosynthesis and Metabolism, eds G. Britton, S. Liaaen-Jensen, and H. Pfander (Basel: Birkhäuser), 359–371.
Livny, O., Kaplan, I., Reifen, R., Polak-Charcon, S., Madar, Z., and Schwartz, B. (2002). Lycopene inhibits proliferation and enhances gap-junction communication of KB-1 human oral tumor cells. J. Nutr. 132, 3754–3759.
Łotocka, M., Styczyńska-Jurewicz, E., and Błędzki, L. A. (2004). Changes in carotenoid composition in different developmental stages of copepods: Pseudocalanus acuspes Giesbrecht and Acartia spp. J. Plankton Res. 26, 159–166. doi: 10.1093/plankt/fbh021
Lu, Q.-Y., Hung, J.-C., Heber, D., Go, V. L., Reuter, V. E., Cordon-Cardo, C., et al. (2001). Inverse associations between plasma lycopene and other carotenoids and prostate cancer. Cancer Epidemiol. Biomarkers Prev. 10, 749–756.
Lubzens, E., and Zmora, O. (2007). “Production and nutritional value of rotifers,” in Live Feeds in Marine Aquaculture, eds J. G. Støttrup and L. A. McEvoy (Oxford: Blackwell Science Ltd.), 17–64.
Maan, M. E., and Sefc, K. M. (2013). Colour variation in cichlid fish: developmental mechanisms, selective pressures and evolutionary consequences. Semin. Cell Dev. Biol. 24, 516–528. doi: 10.1016/j.semcdb.2013.05.003
Manabe, E., Handa, O., Naito, Y., Mizushima, K., Akagiri, S., Adachi, S., et al. (2008). Astaxanthin protects mesangial cells from hyperglycemia-induced oxidative signaling. J. Cell. Biochem. 103, 1925–1937. doi: 10.1002/jcb.21583
Maoka, T., and Akimoto, N. (2008). Carotenoids and their fatty acid esters of spiny lobster Panulirus japonicus. J. Oleo. Sci. 57, 145–152. doi: 10.5650/jos.57.145
Maoka, T., Katsuyama, M., Kaneko, N., and Matsuno, T. (1985). Stereochemical investigation of carotenoids in the Antartic krill Euphasia superba. Bull. Jpn. Soc. Sci. Fish 51, 1671–1673.
Maoka, T., Yokoi, S., and Matsuno, T. (1989). Comparative biochemical studies of carotenoids in nine species of cephalopoda. Comp. Biochem. Physiol. B Biochem. Mol. Biol. 92, 247–250. doi: 10.1016/0305-0491(89)90273-3
Markkula, S. E., Salo, H. M., Immonen, A. K., and Jokinen, E. I. (2005). Effects of short- and long-term ultraviolet B irradiation on the immune system of the common carp (Cyprinus carpio)†. Photochem. Photobiol. 81, 595–602. doi: 10.1111/j.1751-1097.2005.tb00231.x
Markkula, S. E., Salo, H. M., Rikalainen, A.-K., and Jokinen, E. I. (2006). Different sensitivity of carp (Cyprinus carpio) and rainbow trout (Oncorhynchus mykiss) to the immunomodulatory effects of UVB irradiation. Fish Shellfish Immunol. 21, 70–79. doi: 10.1016/j.fsi.2005.10.007
Mason, D. S., Schafer, F., Shick, J. M., and Dunlap, W. C. (1998). Ultraviolet radiation-absorbing mycosporine-like amino acids (MAAs) are acquired from their diet by medaka fish (Oryzias latipes) but not by SKH-1 hairless mice. Comp. Biochem. Physiol. A Mol. Integr. Physiol. 120, 587–598. doi: 10.1016/S1095-6433(98)10069-7
Matsuno, T. (1989). “Animal Carotenoids,” in Carotenoids: Chemistry and Biology, eds N. I. Krinsky, M. M. Mathews-Roth, and R. F. Taylor (Boston, MA: Springer), 59–74.
Matsuno, T. (2001). Aquatic animal carotenoids. Fish. Sci. 67, 771–783. doi: 10.1046/j.1444-2906.2001.00323.x
Matsuno, T., and Tsushima, M. (2001). “Carotenoids in sea urchins,” in Developments in Aquaculture and Fisheries Science, ed. M. L. John (Amsterdam: Elsevier), 115–138.
McFadzen, I., Baynes, S., Hallam, J., Beesley, A., and Lowe, D. (2000). Histopathology of the skin of UV-B irradiated sole (Solea solea) and turbot (Scophthalmus maximus) larvae. Mar. Environ. Res. 50, 273–277. doi: 10.1016/S0141-1136(00)00085-4
McNulty, H. P., Byun, J., Lockwood, S. F., Jacob, R. F., and Mason, R. P. (2007). Differential effects of carotenoids on lipid peroxidation due to membrane interactions: X-ray diffraction analysis. Biochim. Biophys. Acta Biomembr. 1768, 167–174. doi: 10.1016/j.bbamem.2006.09.010
Merchie, G. (1996). “4.3. Use of nauplii and meta-nauplii,” in Manual on the production and use of live food for aquaculture. FAO Fisheries Technical Paper No. 361, eds P. Lavens and P. Sorgeloos (Rome: FAO), 295.
Meyers, S. P. (1994). Developments in world aquaculture, feed formulations and role of carotenoids. Pure Appl. Chem. 66, 1069–1076. doi: 10.1351/pac199466051069
Mimuro, M., and Katoh, T. (1991). Carotenoids in photosynthesis - Absorption, transfer and dissipation of light energy. Pure Appl. Chem. 63, 123–130. doi: 10.1351/pac199163010123
Miyachi, M., Matsuno, T., Asano, K., and Mataga, I. (2015). Anti-inflammatory effects of astaxanthin in the human gingival keratinocyte line NDUSD-1. J. Clin. Biochem. Nutr. 56, 171–178. doi: 10.3164/jcbn.14-109
Mock, C. R. (1972). The Culture of Penaeus japonicus in Japan. Proc. Annu. Meet. World Maric. Soc. 3, 285–286. doi: 10.1111/j.1749-7345.1972.tb00069.x
Molejón, O. G. H., and Alvarez-Lajonchère, L. (2003). Culture experiments with Oithona oculata Farran, 1913 (Copepoda: Cyclopoida), and its advantages as food for marine fish larvae. Aquaculture 219, 471–483. doi: 10.1016/S0044-8486(02)00644-0
Mora, S. D., Demers, S., and Vernet, M. (2005). The Effects of UV Radiation in the Marine Environment. Cambridge: Cambridge University Press.
Mozaffarian, D., and Rimm, E. B. (2006). Fish intake, contaminants, and human health: evaluating the risks and the benefits. JAMA 296, 1885–1899. doi: 10.1001/jama.296.15.1885
Munilla-Moran, R., Stark, J. R., and Barbour, A. (1990). The role of exogenous enzymes in digestion in cultured turbot larvae (Scophthalmus maximus L.). Aquaculture 88, 337–350. doi: 10.1016/0044-8486(90)90159-K
Nelis, H. J., Lavens, P., Van Steenberge, M. M., Sorgeloos, P., Criel, G. R., and De Leenheer, A. P. (1988). Qualitative and quantitative changes in the carotenoids during development of the brine shrimp Artemia. J. Lipid. Res. 29, 491–499.
Odeberg, J. M., Lignell, Å., Pettersson, A., and Höglund, P. (2003). Oral bioavailability of the antioxidant astaxanthin in humans is enhanced by incorporation of lipid based formulations. Eur. J. Pharm. Sci. 19, 299–304. doi: 10.1016/S0928-0987(03)00135-0
Omenn, G. S., Goodman, G. E., Thornquist, M. D., Balmes, J., Cullen, M. R., Glass, A., et al. (1996). Effects of a combination of beta carotene and vitamin A on lung cancer and cardiovascular disease. N. Engl. J. Med. 334, 1150–1155. doi: 10.1056/NEJM199605023341802
Palmer, H. J., and Paulson, K. E. (1997). Reactive oxygen species and antioxidants in signal transduction and gene expression. Nutr. Rev. 55, 353–361. doi: 10.1111/j.1753-4887.1997.tb01561.x
Palozza, P., Serini, S., Di Nicuolo, F., Piccioni, E., and Calviello, G. (2003). Prooxidant effects of β-carotene in cultured cells. Mol. Aspects Med. 24, 353–362. doi: 10.1016/S0098-2997(03)00031-1
Palozza, P., Torelli, C., Boninsegna, A., Simone, R., Catalano, A., Mele, M. C., et al. (2009). Growth-inhibitory effects of the astaxanthin-rich alga Haematococcus pluvialis in human colon cancer cells. Cancer Lett. 283, 108–117. doi: 10.1016/j.canlet.2009.03.031
Park, J. S., Chyun, J. H., Kim, Y. K., Line, L. L., and Chew, B. P. (2010). Astaxanthin decreased oxidative stress and inflammation and enhanced immune response in humans. Nutr. Metab. 7:18. doi: 10.1186/1743-7075-7-18
Pearce, C. M., Daggett, T. L., and Robinson, S. M. C. (2003). Effects of starch type, macroalgal meal source, and β-carotene on gonad yield and quality of the green sea urchin, Strongylocentrotus droebachiensis (Müller), fed prepared diets. J. Shellfish Res. 22, 505–519.
Perumal, S., Ananth, S., Nandakumar, R., Jayalakshmi, T., Kaviyarasan, M., and Pachiappan, P. (2015). “Intensive indoor and outdoor pilot-scale culture of marine copepods,” in Advances in Marine and Brackishwater Aquaculture, eds S. Perumal, A. R. Thirunavukkarasu, and P. Pachiappan (New Delhi: Springer), 33–42.
Pilbrow, J., Sabherwal, M., Garama, D., and Carne, A. (2014). A novel fatty acid-binding protein-like carotenoid-binding protein from the gonad of the New Zealand sea urchin Evechinus chloroticus. PLoS ONE 9:e106465. doi: 10.1371/journal.pone.0106465
Plank, L. R., Lawrence, J. M., Lawrence, A. L., and Olvera, R. M. (2002). The effect of dietary carotenoids on gonad production and carotenoid profiles in the sea urchin Lytechinus variegatus. J. World Aquacult. Soc. 33, 127–137. doi: 10.1111/j.1749-7345.2002.tb00487.x
Raghukumar, S. (2008). Thraustochytrid marine protists: production of PUFAs and other emerging technologies. Mar. Biotechnol. 10, 631–640. doi: 10.1007/s10126-008-9135-4
Rajasingh, H., Øyehaug, L., Våge, D. I., and Omholt, S. W. (2006). Carotenoid dynamics in Atlantic salmon. BMC Biol. 4:10. doi: 10.1186/1741-7007-4-10
Rautio, M., Bonilla, S., and Vincent, W. F. (2009). UV photoprotectants in arctic zooplankton. Aquat. Biol. 7, 93–105. doi: 10.3354/ab00184
Ringelberg, J. (1980). Aspects of red pigmentation in zooplankton, especially copepods. Am. Soc. Limnol. Oceanogr. Spec. Symp. 3, 91–97.
Rodriguez-Amaya, D. B. (2015). “Nomenclature, structures, and physical and chemical properties,” in Food Carotenoids: Chemistry, Biology, and Technology (Chichester: John Wiley & Sons, Ltd.), 1–23.
Rønnestad, I., Yúfera, M., Ueberschär, B., Ribeiro, L., Sæle, Ø., and Boglione, C. (2013). Feeding behaviour and digestive physiology in larval fish: current knowledge, and gaps and bottlenecks in research. Rev. Aquacult. 5, S59–S98. doi: 10.1111/raq.12010
Rørvik, K. A., Ytrestøyl, T., Lundberg, E., Jakobsen, F. A., Jakobsen, A. A., and Bjerkeng, B. (2010). How apparent digestibility of carotenoids, macronutrients, and minerals are differently affected by ration level in Atlantic salmon, Salmo salar. J. Appl. Aquacult. 22, 123–139. doi: 10.1080/10454431003736227
Rosenthal, J. M., Kim, J., de Monastario, F., Thompson, D. J. S., Bone, R. A., Landrum, J. T., et al. (2006). Dose-ranging study of lutein supplementation in persons aged 60 years or older. Invest. Ophthalmol. Visual Sci. 47, 5227–5233. doi: 10.1167/iovs.05-1513
Russell, R. M. (2002). Beta-carotene and lung cancer. Pure Appl. Chem. 74, 1461–1467. doi: 10.1351/pac200274081461
Russell, R. M. (2004). The enigma of β-carotene in carcinogenesis: what can be learned from animal studies. J. Nutr. 134, 262S–268S.
Sachindra, N. M., Bhaskar, N., and Mahendrakar, N. S. (2005). Carotenoids in crabs from marine and fresh waters of India. LWT Food Sci. Technol. 38, 221–225. doi: 10.1016/j.lwt.2004.06.003
SanGiovanni, J. P., and Neuringer, M. (2012). The putative role of lutein and zeaxanthin as protective agents against age-related macular degeneration: promise of molecular genetics for guiding mechanistic and translational research in the field. Am. J. Clin. Nutr. 96, 1223S–1233S. doi: 10.3945/ajcn.112.038240
Saura-Calixto, F., and Goñi, I. (2009). Definition of the Mediterranean diet based on bioactive compounds. Crit. Rev. Food Sci. Nutr. 49, 145–152. doi: 10.1080/10408390701764732
Schiedt, K. (1998). Absorption and Metabolism of Carotenoids in Birds, Fish and Crustaceans. Basel: Birkhäuser.
Schipp, G. R., Bosmans, J. M. P., and Marshall, A. J. (1999). A method for hatchery culture of tropical calanoid copepods, Acartia spp. Aquaculture 174, 81–88. doi: 10.1016/S0044-8486(98)00508-0
Schneider, T., Grosbois, G., Vincent, W. F., and Rautio, M. (2016). Carotenoid accumulation in copepods is related to lipid metabolism and reproduction rather than to UV-protection. Limnol. Oceanogr. 61, 1201–1213. doi: 10.1002/lno.10283
Seale, A. (1933). The brine shrimp (Artemia) as a satisfactory live food for fishes. Trans. Am. Fish. Soc. 63, 129–130.
Sefc, K. M., Brown, A. C., and Clotfelter, E. D. (2014). Carotenoid-based coloration in cichlid fishes. Comp. Biochem. Physiol. A Mol. Integr. Physiol. 173, 42–51. doi: 10.1016/j.cbpa.2014.03.006
Shpigel, M., Schlosser, S. C., Ben-Amotz, A., Lawrence, A. L., and Lawrence, J. M. (2006). Effects of dietary carotenoid on the gut and the gonad of the sea urchin Paracentrotus lividus. Aquaculture 261, 1269–1280. doi: 10.1016/j.aquaculture.2006.08.029
Snodderly, D. M. (1995). Evidence for protection against age-related macular degeneration by carotenoids and antioxidant vitamins. Am. J. Clin. Nutr. 62, 1448S–1461S.
Sommaruga, R. (2010). Preferential accumulation of carotenoids rather than of mycosporine-like amino acids in copepods from high altitude Himalayan lakes. Hydrobiologia 648, 143–156. doi: 10.1007/s10750-010-0141-y
Sommer, F., Agurto, C., Henriksen, P., and Kiørboe, T. (2006). Astaxanthin in the calanoid copepod Calanus helgolandicus: dynamics of esterification and vertical distribution in the German Bight, North Sea. Mar. Ecol. Prog. Ser. 319, 167–173. doi: 10.3354/meps319167
Story, E. N., Kopec, R. E., Schwartz, S. J., and Harris, G. K. (2010). An update on the health effects of tomato lycopene. Annu. Rev. Food Sci. Technol. 1, 189–210. doi: 10.1146/annurev.food.102308.124120
Storz, P. (2005). Reactive oxygen species in tumour progression. Front. Biosci. 10, 1881–1896. doi: 10.2741/1667
Suhnel, S., Lagreze, F., Ferreira, J. F., Campestrini, L. H., and Maraschin, M. (2009). Carotenoid extraction from the gonad of the scallop Nodipecten nodosus (Linnaeus, 1758) (Bivalvia: Pectinidae). Braz. J. Biol. 69, 209–215. doi: 10.1590/S1519-69842009000100028
Symonds, R. C., Kelly, M. S., Caris-Veyrat, C., and Young, A. J. (2007). Carotenoids in the sea urchin Paracentrotus lividus: occurrence of 9′-cis-echinenone as the dominant carotenoid in gonad colour determination. Comp. Biochem. Physiol. B Biochem. Mol. Biol. 148, 432–444. doi: 10.1016/j.cbpb.2007.07.012
Tanaka, Y., and Katayama, T. (1979). Carotenoids in wild and cultured, red sea bream, Pagrus major Temminck & Schlegel and prawn, Penaeus japonicus Bate. Mem. Fac. Fish Kagoshima Univ. 28, 1–7.
Tedetti, M., and Sempéré, R. (2006). Penetration of ultraviolet radiation in the marine environment. A review. Photochem. Photobiol. 82, 389–397. doi: 10.1562/2005-11-09-ir-733
Terao, J. (1989). Antioxidant activity of β-carotene-related carotenoids in solution. Lipids 24, 659–661. doi: 10.1007/bf02535085
Thoen, H. H., Johnsen, G., and Berge, J. (2011). Pigmentation and spectral absorbance in the deep-sea arctic amphipods Eurythenes gryllus and Anonyx sp. Polar Biol 34, 83–93. doi: 10.1007/s00300-010-0861-5
Torrissen, O. (1996). “Effective use of carotenoids for salmon flesh pigmentation,” in Eastern Nutrition Conference (Ottawa, ON: Canadian Feed Industry Association).
Torrissen, O. J., and Christiansen, R. (1995). Requirements for carotenoids in fish diets. J. Appl. Ichthyol. 11, 225–230. doi: 10.1111/j.1439-0426.1995.tb00022.x
Tsushima, M. (2007). “Carotenoids in sea urchins,” in Developments in Aquaculture and Fisheries Science, ed M. L. John (Amsterdam: Elsevier), 159–166.
Tsushima, M., Kawakami, T., Mine, M., and Matsuno, T. (1997). The role of carotenoids in the development of the sea urchin Pseudocentrotus depressus. Invertebr. Reprod. Dev. 32, 149–153. doi: 10.1080/07924259.1997.9672616
van Bergeijk, S. A., Salmeron, J. A. P., Perez, A. I. L., Moreno, J., Canavate, J. P., and Garcia-Gonzalez, M. (2013). Lutein enrichment of the rotifer Brachionus sp using freeze-dried Muriellopsis sp cells. Aquacult. Res. 44, 1724–1730. doi: 10.1111/j.1365-2109.2012.03178.x
van Nieuwerburgh, L., Wänstrand, I., Liu, J., and Snoeijs, P. (2005). Astaxanthin production in marine pelagic copepods grazing on two different phytoplankton diets. J. Sea Res. 53, 147–160. doi: 10.1016/j.seares.2004.07.003
Virtamo, J., Pietinen, P., Huttunen, J. K., Korhonen, P., Malila, N., Virtanen, M. J., et al. (2003). Incidence of cancer and mortality following α-tocopherol and β-carotene supplementation: a postintervention follow-up. JAMA 290, 476–485. doi: 10.1001/jama.290.4.476
von Schantz, T., Bensch, S., Grahn, M., Hasselquist, D., and Wittzell, H. (1999). Good genes, oxidative stress and condition–dependent sexual signals. Proc. R. Soc. Lond. B. Biol. Sci. 266, 1–12. doi: 10.1098/rspb.1999.0597
Voutilainen, S., Nurmi, T., Mursu, J., and Rissanen, T. H. (2006). Carotenoids and cardiovascular health. Am. J. Clin. Nutr. 83, 1265–1271.
Wade, N. M., Tollenaere, A., Hall, M. R., and Degnan, B. M. (2009). Evolution of a novel carotenoid-binding protein responsible for crustacean shell color. Mol. Biol. Evol. 26, 1851–1864. doi: 10.1093/molbev/msp092
Wang, Y., Chun, O. K., and Song, W. O. (2013). Plasma and dietary antioxidant status as cardiovascular disease risk factors: a review of human studies. Nutrients 5, 2969–3004. doi: 10.3390/nu5082969
Yamasaki, T., Aki, T., Mori, Y., Yamamoto, T., Shinozaki, M., Kawamoto, S., et al. (2007). Nutritional enrichment of larval fish feed with thraustochytrid producing polyunsaturated fatty acids and xanthophylls. J. Biosci. Bioeng. 104, 200–206. doi: 10.1263/jbb.104.200
Yanar, Y., Çelik, M., and Yanar, M. (2004). Seasonal changes in total carotenoid contents of wild marine shrimps (Penaeus semisulcatus and Metapenaeus monoceros) inhabiting the eastern Mediterranean. Food Chem. 88, 267–269. doi: 10.1016/j.foodchem.2004.01.037
Yokoyama, A., Sandmann, G., Hoshino, T., Adachi, K., Sakai, M., and Shizuri, Y. (1995). Thermozeaxanthins, new carotenoid-glycoside-esters from thermophilic eubacterium Thermus thermophilus. Tetrahedron Lett. 36, 4901–4904. doi: 10.1016/0040-4039(95)00881-C
Ytrestøyl, T., Coral-Hinostroza, G., Hatlen, B., Robb, D. H. F., and Bjerkeng, B. (2004). Carotenoid and lipid content in muscle of Atlantic salmon, Salmo salar, transferred to seawater as 0+ or 1+ smolts. Comp. Biochem. Physiol. B Biochem. Mol. Biol. 138, 29–40. doi: 10.1016/j.cbpc.2004.01.011
Keywords: astaxanthin, β-carotene, antioxidant, UV radiation, food webs, aquaculture, fisheries
Citation: de Carvalho CCCR and Caramujo MJ (2017) Carotenoids in Aquatic Ecosystems and Aquaculture: A Colorful Business with Implications for Human Health. Front. Mar. Sci. 4:93. doi: 10.3389/fmars.2017.00093
Received: 07 January 2017; Accepted: 16 March 2017;
Published: 03 April 2017.
Edited by:
Antonio Trincone, Istituto di Chimica Biomolecolare, Consiglio Nazionale delle Ricerche, ItalyReviewed by:
Tiziano Verri, University of Salento, ItalyFrancisco Javier Alarcón, University of Almería, Spain
Brett Marc Macey, Department of Agriculture, Forestry and Fisheries, South Africa
Copyright © 2017 de Carvalho and Caramujo. This is an open-access article distributed under the terms of the Creative Commons Attribution License (CC BY). The use, distribution or reproduction in other forums is permitted, provided the original author(s) or licensor are credited and that the original publication in this journal is cited, in accordance with accepted academic practice. No use, distribution or reproduction is permitted which does not comply with these terms.
*Correspondence: Carla C. C. R. de Carvalho, Y2NhcnZhbGhvQHRlY25pY28udWxpc2JvYS5wdA==