- 1Ifremer, REM/EEP/LEP, Centre de Bretagne, Plouzané, France
- 2Ifremer, REM, Centre de Bretagne, Plouzané, France
- 3Ifremer, UMR MARBEC, Station de Sète, Sète, France
- 4LDC_CONSULT, Bayonne, France
The topographical and hydrological complexity of submarine canyons, coupled with high substratum heterogeneity, make them ideal environments for cold-water coral (CWC) habitats. These habitats, including reefs, are thought to provide important functions for many organisms. The canyons incising the continental slope of the Bay of Biscay have distinct morphological differences from the north to the south. CWCs have been reported from this basin in the late nineteenth century; however, little is known about their present-day distribution, diversity and environmental drivers in the canyons. In this study, the characteristics and distribution of CWC habitats in the submarine canyons of the Bay of Biscay are investigated. Twenty-four canyons and three locations between adjacent canyons were sampled using a Remotely Operated Vehicle (ROV) or a towed camera system. Acquired images were annotated for habitat type (using the CoralFISH classification system), substrate cover and coral identification. Furthermore, the influence of hydrological factors and geomorphology on the CWC distribution was investigated. Eleven coral habitats, formed by 62 morphotypes of scleractinians, gorgonians, antipatharians and seapens, inhabiting hard and/or soft substrate, were observed. The distribution patterns were heterogenous at regional and local scales; the south Bay of Biscay and the southeastern flank favored soft substrate habitats. Biogenic and hard substrate habitats supported higher coral diversities than soft substrate habitats and had similar species compositions. A higher coral species turnover characterized soft substrate habitats. Substrate type was the most important driver of the patterns in both distribution and composition. Observations of coral reefs on steeper areas in the canyons and coral rubble on flatter areas on the interfluve/upper slope support the hypothesis that canyons serve as refuges, being less accessible to trawling, although natural causes may also contribute to the explanation of this distribution pattern. The results of this study fed into a proposal of a Natura 2000 network in the Bay of Biscay where management plans are rare.
Introduction
Submarine canyons incise many continental shelves and slopes around the world (Harris and Whiteway, 2011). These physiographical features have a complex, heterogeneous topography that creates specific hydrological processes, such as accelerated (bottom) currents, internal waves and dense shelf water cascading (De Leo et al., 2010; Harris and Whiteway, 2011). These processes have an influence on the sediment accumulation within canyons and are thought to transport organic matter from the continental shelf to the deep sea and to increase the suspended particulate matter concentration (De Leo et al., 2010; Harris and Whiteway, 2011). Internal waves enhance the mixing of water masses and the release of nutrients which in turn favor the development of plankton (Pingree and Mardell, 1985; Huthnance, 1995; Khripounoff et al., 2014). This primary production will be transported into the canyons, increasing the amount and quality of food (Huthnance, 1995; Amaro et al., 2015, 2016).
Because of the heterogeneous topography, including exposed hard and steep substrate, and the hydrological patterns, submarine canyons are hypothesized as biodiversity hotspots of cold-water corals (CWCs) (Mortensen and Buhl-Mortensen, 2005; White et al., 2005; Orejas et al., 2009; De Leo et al., 2010), compared to adjacent slope areas (Vetter et al., 2010; Cunha et al., 2011). CWCs are defined organisms belonging to the cnidarian classes Anthozoa and Hydrozoa that produce either calcium carbonate or black, horn-like, proteinaceous skeleton elements (Cairns, 2007). The orders that meet this definition are Scleractinia (stony corals), Alcyonacea (soft corals, including gorgonians), Antipatharia (black corals), Pennatulacea (seapens) as well as the hydrozoan family Stylasteridae (hydrocorals) (Cairns, 2007). Most of these CWCs need hard substrate to settle, with the exception of most seapens, some scleractinians (predominantly solitary) and some gorgonian species (Roberts et al., 2009). CWCs are filter-feeders that rely on currents to deliver food particles (Wagner et al., 2012) and vertical migration of zooplankton (Carlier et al., 2009; Mienis et al., 2012; Wagner et al., 2012; Hebbeln et al., 2014).
The scleractinians Lophelia pertusa and Madrepora oculata can form reefs (Roberts et al., 2006), which function as refuges, feeding areas and nurseries for many species, including commercially important fish (Roberts et al., 2006). CWC reefs are also linked to a high biodiversity (Freiwald et al., 2004; Roberts et al., 2009). While antipatharians, gorgonians and seapens cannot form reefs, they can occur in dense aggregations (coral gardens) and may provide similar functions as reefs (Roberts et al., 2006; Buhl-Mortensen et al., 2010; Baillon et al., 2012), for example, rockfish association with gorgonians in canyons of the Bering Sea (Miller et al., 2012).
Deep-water scleractinians occur mainly between 50 and 1,000 m water depth and in water temperatures of 4°–10°C (Roberts et al., 2006), occurring in a narrow density envelope of sigma-theta 27.35–27.65 kg/m3 as proposed by Dullo et al. (2008) for the North-East Atlantic that is linked to trophic inputs. Antipatharians and seapens can occur much deeper, even greater than 6,000 m (Williams, 2011; Wagner et al., 2012). The occurrence, abundance and diversity of CWC species and/or habitat were influenced by temperature, salinity, water density, currents and trophic input (e.g., Roberts et al., 2006; Dullo et al., 2008; Yesson et al., 2012, in press; Mohn et al., 2014; Robert et al., 2015). Terrain parameters can be extracted from multibeam bathymetry and can serve as useful surrogates for habitat mapping. Slope, rugosity and Bathymetric Position Index (BPI) are examples of parameters measuring canyon topography and are known to have an influence on the presence of CWC species/habitats (e.g., Howell et al., 2011; Yesson et al., 2012; Robert et al., 2015).
CWCs are long-lived, have slow growth rates, form important structural habitats and are vulnerable to human activities, such as the fishing and oil and gas industries (Roberts et al., 2006; Ramirez-Llodra et al., 2011). Thus, CWC habitats meet the criteria of both VMEs (Vulnerable Marine Ecosystems; FAO) and EBSAs (Ecologically or Biologically Significant Marine Areas; CBD). As such, CWCs and their habitats have been listed as threatened or endangered by international organizations (OSPAR, ICES, Habitats Directives). Canyons are seen as natural refuges for these CWC habitats as well as other habitats, such as oyster banks (Van Rooij et al., 2010; Huvenne et al., 2011; Fernandez-Arcaya et al., 2017). Mapping and understanding the distribution of CWC habitats is, therefore, needed for conservation management along European margins. As a result of the importance of this part of the NE Atlantic as an integral sector or transition zone of this margin (Reveillaud et al., 2008; De Mol et al., 2011), the Bay of Biscay could potentially play a large role in persistence of those ecosystems on a global scale and, thus, potentially important for the connectivity between regions.
Habitat maps are becoming increasingly used in marine management and conservation. Areas selected for marine management and conservation can have different spatial scales, ranging from a particular zone or geographical feature, e.g., canyons or carbonate mounds, to an Exclusive Economic Zone (EEZ) of a country or areas as large as the North-east Atlantic. Classification systems aid in creating comprehensive, detailed and integrative habitat maps. An important advantage of classification systems is that they permit the use of a standardized terminology and habitat type over a large region. Classification systems may have different information or concepts, e.g., region, seascape, and biotope, depending on the goal of the marine management plan (Costello, 2009). A hierarchical system allows mapping of habitats at different scales, using a variety of data, depending on availability (e.g., different resolutions, quality, etc.); and can be adjusted to the needs and goals of the user (Costello, 2009).
A classification system comprising coral biota was developed during the EC FP7-funded project CoralFISH (Davies et al., in press). This classification system enables the comparison of coral habitats between regions in the NE Atlantic and Mediterranean using standardized terms and methods. It is a hierarchical system including biotopes (or habitats) formed by the dominant coral group(s)/species, dominant substrate type and potential geoforms, such as boulders and vertical walls (Davies et al., in press).
The continental margin of the Bay of Biscay, studied here, is incised by more than a 100 canyons and are organized into drainage basins (Bourillet et al., 2003). Eight drainage basins occur from the Goban Spur to the Capbreton Canyon (Bourillet et al., 2006). Scleractinians were first reported in the Bay of Biscay in the late nineteenth century (e.g., Roule, 1896). However, the first maps of scleractinian occurrences were produced in the following century: Joubin (1922) used fishermen reports on scleractinians causing damage to demersal trawls and Le Danois (1948) mapped coral reefs along the continental slope of the Bay. Despite this early discovery, only a few CWC studies had been undertaken in the French part of the Bay of Biscay (Zibrowius, 1980; Reveillaud et al., 2008; De Mol et al., 2011) and the largest part of this basin still remains unexplored.
Here, we report investigations of the presence of CWC habitats in the submarine canyons of the Bay of Biscay based on surveys using a Remotely Operated Vehicle (ROV) and a towed camera system. The CoralFISH coral biota classification system was used to delimit coral habitats. The goals of this study were: (i) to identify coral habitats in the canyons of the Bay of Biscay, (ii) to identify coral species and their abundances, densities and diversities within these habitats and their compositions, (iii) to investigate the distribution of coral habitats in the Bay of Biscay, and (iv) to explore the influence of other environmental factors (temperature, water density, depth, derivatives of the bathymetry) and geomorphology on the presence and distribution of coral habitats.
Materials and Methods
Study Site
Margin Morphology
The Bay of Biscay is part of the North-East Atlantic Ocean, located west of France and north of Spain. It is a passive margin containing three parts; the Celtic, Armorican and Aquitaine margins (Zaragosi et al., 2000). Within this study, the Bay of Biscay was defined using geological boundaries (see Bourillet et al., 2006) therefore including all three margins, as the Celtic margin is part of the Celtic Sea according to hydrographical terms.
The morphology of the Celtic and the Armorican margins (Figure 1), limited by the Goban Spur in the north and the Conti spur in the south, is characterized by spurs and canyons (Bourillet et al., 2006). The continental shelf is wide, up to 200 km for the Armorican margin and more than 250 km for the Celtic margin (Bourillet et al., 2006). These two margins can be divided into three zones based on the geomorphology of the continental margins, the influences of sedimentation and hydrodynamic regimes (Figure 1): (i) the Celtic and northern Armorican margin, (ii) the central Armorican margin, and (iii) the southern Armorican margin.
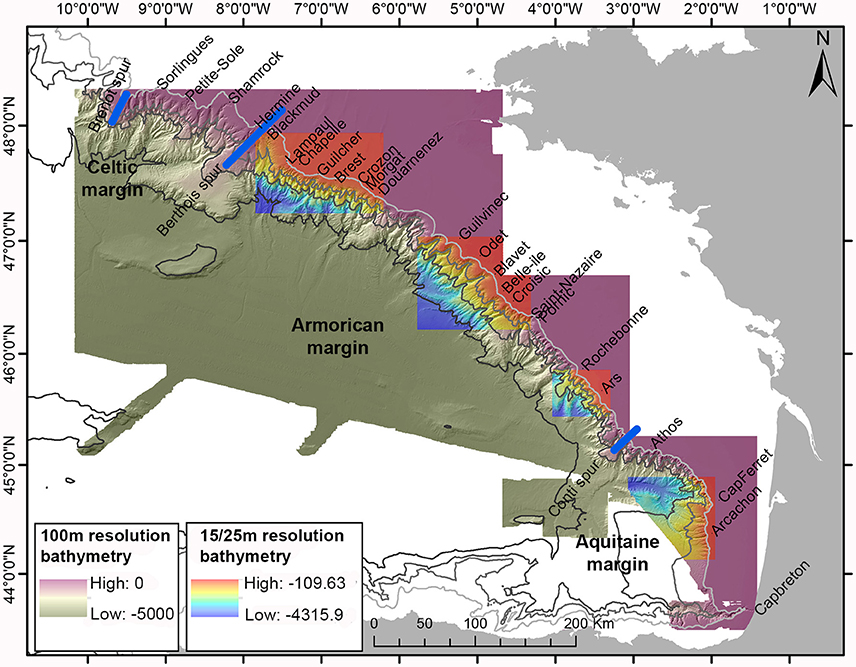
Figure 1. A map of the submarine canyons incising the continental slope of the Bay of Biscay. The bathymetry is available at a 100 m resolution for the whole Bay of Biscay (green-purple) and at a 15/25 m resolution for four boxes representing each zone (rainbow-colored) from Bourillet et al. (2012). The blue lines indicate the Brenot, Berthois and Conti spurs, dividing the margin into the Celtic, Armorican and Aquitaine margins.
The morphology of the first zone—the Celtic and northern Armorican margins—is complex and comprises some shelf-incising submarine canyons (Figure 1). These canyons contain several morphological features, such as cliffs, mainly formed in the head of the canyons by regressive erosion from the bottom to the top of the canyon (Bourillet et al., 2010). There are three main deep-sea drainage systems: (i) the Petite Sole drainage basin on the Celtic margin, comprising the canyons from Sorlingues to Hermine, (ii) the Chapelle drainage basin, comprising the canyons from Blackmud to Guilcher, (Bourillet and Lericolais, 2003) and, (iii) the West Brittany drainage basin, comprising the canyons from Brest to Douarnenez (Zaragosi et al., 2000, 2001). Zone 1 is under the influence of tidal currents of the English Channel (Zaragosi et al., 2001) and is linked with the drainage basins of rivers, such as the Seine, via the Channel paleo-river (Bourillet et al., 2003).
The second zone is the central part of the Armorican margin (Figure 1). This zone includes an alternation of large and narrow canyons. The large canyons, considered to be formed first, cut the continental shelf, while the heads of the narrow canyons, formed during a later stage, are located halfway down the slope (Bourillet et al., 2010). Two drainage systems are found within this part of the Armorican shelf: (i) the South Brittany drainage system, including the canyons from Audierne, south of Douarnenez Canyon, to Blavet Canyons (Zaragosi et al., 2001), and (ii) the Gascogne drainage system from the Belle-île to Yeu Canyons (also including Croisic, Saint-Nazaire and Pornic Canyons) (Bourillet et al., 2006). These canyons are not under the influence of the English Channel any longer and sediment is transported from the continental shelf to these canyons (Zaragosi et al., 2001).
The third zone comprises the southern part of the Armorican margin (Figure 1). Even though canyons still reach the continental shelf in this part of the Bay of Biscay, they are smoother than those on the Celtic and northern Armorican margins. The flanks of the canyons in this zone are regular and sedimentary, while the thalwegs are continuous with sloping banks. Cliffs are either scarce or not present in this zone (Bourillet et al., 2010). The Rochebonne drainage basin, comprising the canyons from Sables d'Olonnes, north of Rochebonne Canyon, to Oleron Canyon, north of the Conti Spur, is the only drainage system within this zone (Bourillet et al., 2006) and includes Rochebonne and Ars Canyons.
The third margin of the Bay of Biscay, the Aquitaine margin, from the Conti Spur to Capbreton Canyon, can be considered as a fourth zone of the Bay of Biscay (Figure 1). The continental shelf is narrow, with only 70 km from the shore (Bourillet et al., 2006). The continental slope of this margin is a “tectonic-dominated” margin instead of a “canyon-dominated” slope (Bourillet et al., 2006). The dominant relief, the Landes plateau, is surrounded by two majors canyons: Cap-Ferret and Capbreton. The “gouf de Capbreton” is an exceptional example of a shelf-incising canyon with a head close to the beach and a very gentle along-slope profile (Cirac et al., 2001; Bourillet et al., 2007). The flanks of the canyons on this margin have a weak slope and they do not present steps or cliffs. The Cap-Ferret and Arcachon thalwegs are broader than those of other canyons, north of this zone/margin (Bourillet, 2010; De Chambure et al., 2013).
Water Masses and Currents
In the Bay of Biscay several water masses with different origins and densities can be found (de Madron et al., 1999; van Aken, 2000a,b): (i) the Eastern North Atlantic Water (ENAW), (ii) the Mediterranean Outflow Water (MOW), (iii) the Labrador Sea Water (LSW), and (iv) the Northeast Atlantic Deep Water (NEADW). The ENAW is usually found between 200 and 600 m water depth. It originates from the Labrador Current and may contain a significant amount of Antarctic Intermediate Water (AAIW) transported to the NE Atlantic by the Gulf stream-North Atlantic Current. The more saline and denser MOW is deeper and generally flows between 700 and 1,300 m water depth. This water mass is more pronounced in the south of the Bay of Biscay and becomes less noticeable in the north of the Bay of Biscay toward Porcupine Seabight. The third water mass is the LSW and reaches to ~2,000 m depth. It is usually mixed with the MOW by internal waves near the continental slope and therefore this water mass is less apparent. The fourth and last layer is the NEADW originating from multiple water masses, including MOW and LSW. It occurs approximately between 2,000 and 2,600 m water depth.
There are several currents and other hydrological processes occurring in the Bay of Biscay that modify the temperature, salinity and density of the water masses. The slope current runs along the continental slope of the Bay of Biscay. It arrives in this basin via the Portuguese and Spanish continental slopes (Pingree and Le Cann, 1989, 1992; Koutsikopoulos and Le Cann, 1996) and is thought to be a density driven current formed by the MOW (Pingree and Le Cann, 1989). Even though the slope current is influenced by the complex morphology of the continental slope, it moves dominantly northwards/polewards (Pingree and Le Cann, 1989, 1992; Koutsikopoulos and Le Cann, 1996).
Other important processes in canyons of the Bay of Biscay are tidal currents and internal waves (Koutsikopoulos and Le Cann, 1996; Mulder et al., 2012). Tidal currents can have speeds up to 1 m/s (de Madron et al., 1999; Mulder et al., 2012; Khripounoff et al., 2014) and are weaker in the southern region of the Bay of Biscay (lower than 45°N) (Koutsikopoulos and Le Cann, 1996). These currents follow a semi-diurnal tidal frequency (e.g., Pingree and Le Cann, 1989; Koutsikopoulos and Le Cann, 1996; de Madron et al., 1999; Mulder et al., 2012) and can extend deep into the canyons (Pichon et al., 2013). Internal waves cause the resuspension of sediment and create nepheloid layers, as observed in Cap-Ferret Canyon on the Aquitaine margin (de Madron et al., 1999).
Currents have an influence on the primary production or on other biological traits within the Bay of Biscay. Tidal currents and upwelling are thought to promote water mixing and the release of nutrients. This creates a favorable environment for phytoplankton growth, thus enhancing primary production (Pingree and Mardell, 1985; Huthnance, 1995). Tidal currents and internal waves can transport the primary production into canyons, thus providing food for filter-feeders such as CWCs (Huthnance, 1995; Amaro et al., 2015, 2016).
Data Collection
Data were collected during seven cruises on the R/V Pourquoi Pas? the R/V Le Suroît and the R/V Thalassa between 2009 and 2012 (Table 1). The BobGeo, BobGeo 2 (Bourillet, 2009, 2010) and BobEco (Arnaud-Haond, 2011; Arnaud-Haond and Grehan, 2011) cruises were performed under the European FP7-funded project CoralFISH. This project “assesses the interaction between CWCs, fish and fisheries, in order to develop monitoring and predictive modeling tools for ecosystem based management in the deep waters of Europe and beyond” (http://eu-fp7-coralfish.net/). The main objectives of these cruises were the exploration for, and study of geological features and/or marine ecosystems, with a specific focus on scleractinian coral habitats in the canyons of the Bay of Biscay. The Evhoe cruises were resource surveys targeting the evaluation of fish stocks in the Bay of Biscay for multiple utilizations, e.g., stock evaluation models. Data acquired using a towed camera system (see below) on these cruises were utilized to explore the benthic communities in canyons.
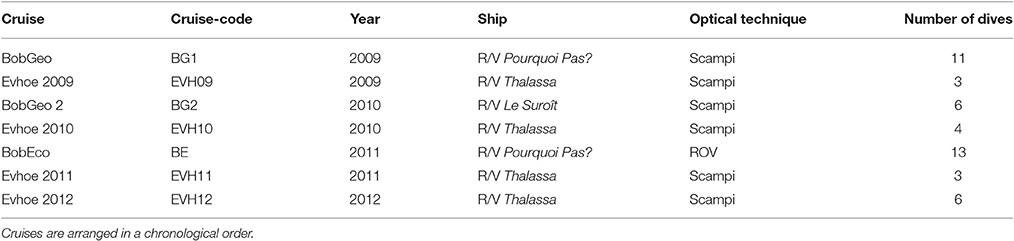
Table 1. Dive information including cruise name, year, ship, the optical technique that is used and the number of dives analyzed during this study.
In total, 46 dives using an ROV or a towed camera were undertaken: 43 dives within 24 canyons and 3 additional dives on interfluves or on the upper slope between adjacent canyons (Table 2). The latter dives were difficult to assign to either one of these two canyons; the name of both canyons were therefore used and they were, thus, treated separately (interfluve: dive BE_480 between Morgat and Douarnenez; upper slope: dive BG2_05 between Odet and Guilvinec and dive BG1_08 between Odet and Blavet Canyons; Table 2). Most canyons were named, except for the canyon north of Éperon Ostrea; to simplify the reference to this particular canyon, the term/name “La Chapelle” was used, named after “le haut-fond de La Chapelle,” an area of sand waves on the continental shelf near the head of this canyon.
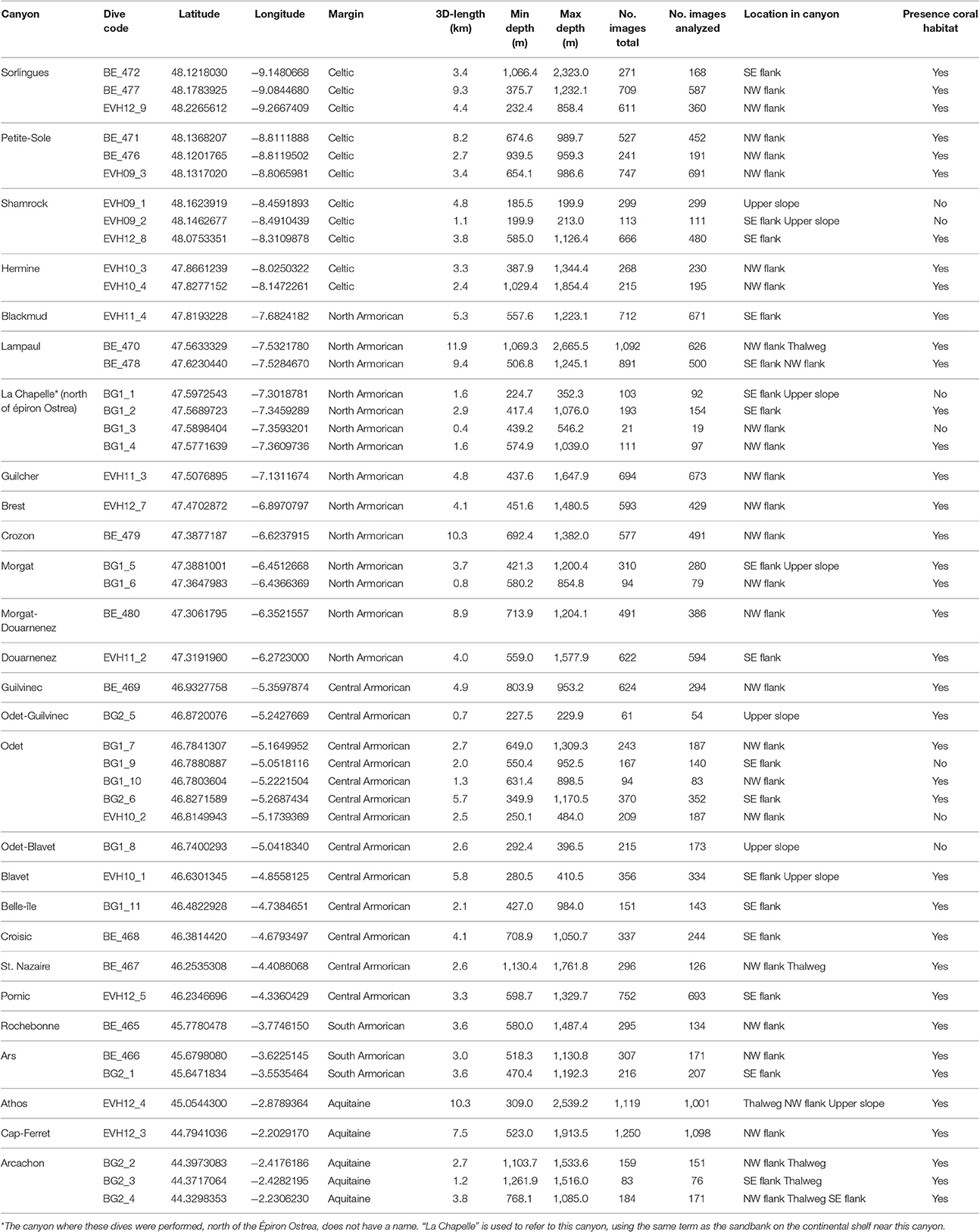
Table 2. Description of the dives used to collect the image footage, including the margin of the Bay of Biscay, the number of images (total and analyzed), the location within the canyon (NW flank, north-western flank; SE flank, south-eastern flank) and the presence of coral habitat.
A towed camera system, Scampi, was used to collect images during 33 dives performed on six cruises included in this study (Table 1). The frame was fitted with a Nikon D700 stills camera directed vertically downwards. The camera was towed ~2–3 m above the seafloor and photos were manually taken at intervals of ~10–90 s.
During the BobEco cruise, image footage for 13 dives was acquired using the ROV Victor6000 (Table 1). The ROV was equipped with multiple cameras, of which the vertically directed video camera was used within this study (Sony FCB-H11). Frame-grabs from the videos were taken at 1-min intervals using the ADELIE annotation software (Ifremer, www.ifremer.fr/adelie) to allow comparison between the ROV image footage with photos taken by the Scampi system; both frame-grabs and photos are called “images” hereafter.
Metadata, such as the navigation data and the timecodes of the videos/photos were extracted using ADELIE. A USBL system (Ultra-Short BaseLine system) was used for accurate positioning of the vehicles, but was unavailable for all Evhoe-cruises, so the ship navigation was used for these dives.
Image Analysis
Each image was subjected to a quality control prior to analysis, based on three criteria: (i) altitude; images were included if they were taken between 1 and 5 m from the seafloor, (ii) image quality; poor quality images due to sediment clouds obscuring the image, the image being out of focus or taken in low light-conditions, were removed before analysis, and (iii) vehicle movement; images were excluded during stationary phases (ROV only).
Images that passed the quality control were annotated for (i) habitat type, (ii) substrate cover (subset; see below), and (iii) fauna (subset; see below).
Habitat Type
Habitat type was visually assigned for images based on the coral biota classification system created within the CoralFISH project (Davies et al., in press). This classification system was created as a tool to standardize habitat observations across the CoralFISH regions in the North-east Atlantic and Mediterranean. The definition of a habitat given by this classification was an area “where a coherent suite of conspicuous epibenthic organisms, including CWCs, extending throughout a minimum estimated area of 25 m2 as observed by underwater cameras.” Coral biotopes (or habitats) were based on the dominant coral species or group of species, the presence of scleractinian framework, usually L. pertusa and M. oculata, and the substrate type. Large geological features, such as vertical walls and boulders, were also included in the classification.
The CoralFISH coral biota classification system is a hierarchical system consisting of three levels (Davies et al., in press): level 1, the broadest level, includes the dominant of coral type(s) and substrate type, whereas level 3, the most detailed level, includes the coral species constructing the habitat, the substrate type and possible geological features as well as conspicuous non-coral species. Due to the large variation and thus the high number of level 3 habitats over a large study area, only the first level of this classification was used (Biotope level 1).
Substrate Cover and Fauna
Substrate cover and fauna were analyzed on a subset (2,350 images) of the 4,191 images on which CWC habitats were observed (see Section Results). The subset was created by selecting images at an interval of ~1 min from the beginning of the dive. As the images acquired using the Scampi system were taken manually and at irregular intervals, images taken 15 s before or after the 1-min interval were considered for the subset.
Substrate cover, including colonial scleractinians, was measured using a 100 point grid, which was placed over the subset images using the software COVER (C. Carré, Ifremer; see Gomes-Pereira et al., 2016). Substrate types were assigned to each point and a relative substrate percentage cover of the visible part of the image was calculated for each subset image.
For each subset image, fauna were enumerated and identified to the lowest taxonomic level possible. It is difficult to identify deep-sea species to species level from images, because of a lack of associated identifications based on morphological characteristics or genetics. Therefore, they were identified as morphotypes and given an Operational Taxonomic Unit (OTU). To aid identification, a species catalog was used (Howell and Davies, 2010: http://www.marlin.ac.uk/deep-sea-species-image-catalogue/) and updated as part of a collaborative project between Plymouth University, Ifremer and NOAA. For this current study, the focus was coral morphotypes. Where possible, species were identified and/or confirmed by coral taxonomists using voucher-specimen collected during the BobEco cruise (see Acknowledgments).
For the reef-forming scleractinian species L. pertusa, M. oculata, and Solenosmilia variabilis percentage cover was measured using the same method as substrate cover. These three species were not included in any result concerning the abundances of individual organisms. However, they were included in a number of analyses (see Section Statistical Analysis). In many instances, the resolution of images did not allow for discrimination between L. pertusa and M. oculata, therefore were treated as one morphotype, since previous literature has shown that these species nearly systematically co-occur in the Bay of Biscay (De Mol et al., 2011; Arnaud-Haond et al., in press).
Habitat Segments
In order to assess the length of CWC habitats, their taxonomic composition and environmental characteristics, habitat segments were defined as all the adjacent images showing the same habitat, until another habitat was assigned to an image. The lengths (hereafter “linear”) of both habitat segments and dives were measured in 3D using ArcGIS (Table 2).
Environmental Data
Bathymetry and Derivatives
A digital Terrain Model (DTM) was available for the whole Bay of Biscay at a resolution of 100 m (Figure 1). DTMs were also available for four boxes (bob-boxes) at a higher resolution of 15 or 25 m (Bourillet et al., 2012), representing each of the four zones of the Bay of Biscay (Figure 1; Section Margin Morphology). The boxes Bob-1 and Bob-2 have a surface of ~10,000 km2 and are located on the northern and central part of the Armorican margin, respectively. The third box, Bob-3, has a surface of ~1,900 km2 and represents the southern part of the Armorican margin. The forth box, Bob-4, with a surface of ~7,000 km2, is located on the Aquitaine margin. The resolutions of Bob-1, Bob-3, and Bob-4 are 15 m, however, the resolution of the Bob-2 bathymetry is lower (25 m), due to poor weather conditions during the time of acquisition. The bathymetry for the dives on the Celtic margin and Athos Canyon (Aquitaine margin) was only available at the lower (100 m) resolution. For the consistency across the whole dataset, depth-values were extracted from the lower (100 m) bathymetry resolution. This resolution was also used to measure the 3D-length of the dives and habitat segments.
Terrain derivatives were extracted from the bathymetry using the ArcGIS extension Benthic Terrain Modeler v. 3.0 (Wright et al., 2012). Slope, direction (northness or cosAspect, eastness or sinAspect), curvature (general, plan and profile), Surface to Planar, BPI and a measure of rugosity (VRM = Vector Ruggedness Measure) were calculated. Neighborhood sizes of ~200, 300, and 500 m were chosen for the fine scale BPI and 1 and 1.5 km for the broad scale BPI. For the VRM, similar neighborhood sizes (except 200 m) were used.
Geomorphology
Geomorphological classes were produced for the entire Bay of Biscay, using bathymetry data, its derivatives, such as slope, canyon network extraction as well as expert interpretation (De Chambure et al., 2013). The geomorphological classes are available on the same resolutions as the DTMs and mapped by Bourillet et al. (2012) and are using the Coastal and Marine Ecological Classification Standard (CMECS) code (Madden et al., 2008). A total of 21 classes have been created on the higher (15/25 m) resolution for the bob-boxes and 15 classes on the lower (100 m) resolution for the whole Bay of Biscay.
One of 15 geomorphological classes at a 100 m resolution was attributed to each image for the whole dataset in ArcGIS 10.2 and one of 21 classes at a 15/25 m resolution for each image in the bob-boxes. Due to the uneven and sometimes low number of images per geomorphological class, classes were merged for statistical robustness according to three different criteria: (i) Morphology with three attributes: Canyon, Interfluve and Upper slope, (ii) Slope, with four intervals depending on the resolution: at 15 m resolution: <10°, 10–20°, 20–40°, and >40°; at 100 m resolution: <10°, 10–15°, 15–25°, and >25°, and (iii) Location, with two attributes: northwestern and southeastern flank of a canyon/interfluve (Table 2).
Temperature, Salinity, and Water Density
Seawater potential temperature and salinity data were publically available (Copernicus Marine Environment Monitoring Service: http://marine.copernicus.eu/) at a resolution of 0.083° latitude (~10 km). These variables were available for different depth layers (from 0 to 5,500 m depth). The monthly means from 15/12/2009 to 15/12/2011, available at the lowest depth layer as possible, were used to calculate mean potential temperature (in Kelvin) and salinity (in PSU). A geotiff (geo-referenced image) was created for both variables and a value extracted for each image of the data set in ArcGIS. Temperature values were transformed to °C.
The seawater density can describe the mixing of water masses. The potential density anomaly (sigma-theta or σΘ), using the potential temperature instead of in situ measured data, was calculated according to Dullo et al. (2008).
A raster of the sigma-theta was calculated using the rasters of the potential temperature and salinity data in the software program R. A value was extracted for each image using ArcGIS from a geotiff.
Statistical Analysis
Coral Community and Composition
Several metrics—abundance, density and diversity—were used to characterize the habitats and the engineering coral species. Mean coral densities per habitat type and per segment were calculated. The density was expressed per image, instead of per linear transect or surface measure for three reasons: (i) only a subset of images were analyzed for fauna which makes it difficult to give densities per linear meter, (ii) no lasers were available on both optical techniques, and (iii) the altimeter on the Scampi frame was not reliable enough to give good estimations of area of images, complicating the expression of densities by surface measure. A spearman correlation was calculated to investigate whether segment length and mean densities (in individuals/image) per segment were correlated.
Rarefaction analysis (individual based) was used to compare differences in richness of corals between habitats. The Hurlbert's index (Hurlbert, 1971) is given as a diversity index, comparing the diversity of the habitats using a random sample size equal to the smallest number of individuals observed in one of these habitat, and thus, limiting the influence of unequal sample sizes. The three scleractinians measured as percentage cover were excluded from these analyses.
A Spearman correlation tested the relationship between the mean percentage of scleractinian cover and the total abundances of other coral types, i.e., antipatharians, gorgonians and seapens, per habitat segment.
Principal Component Analyses (PCA) were computed to investigate the (dis)similarities in species composition of the different habitats. Two PCAs were performed: (i) a PCA on a covariance matrix using the Hellinger-transformed abundances of each morphotype per habitat, excluding the reef-forming scleractinians L. pertusa, M. oculata, and S. variabilis, and (ii) a PCA on a correlation matrix using the raw total abundances of morphotypes and the mean percentage scleractinian cover per habitat. The later included the three previous mentioned scleractinians. The Hellinger-transformation gives a low weight to the morphotypes with a high abundance (Legendre and Gallagher, 2001).
Habitat Distribution and Environmental Characteristics
Coral habitats were mapped in the canyons surveyed in the Bay of Biscay. The proportion of each coral habitat per canyon was used to describe the distribution of these habitats per canyon.
A PCA was computed to investigate the (dis)similarities in habitat types observed in the canyons using the Hellinger-transformed total linear (i.e., lengths) of each coral habitat (Legendre and Gallagher, 2001).
A specific principal component analysis with respect to instrumental variables, the Between-Class Analysis (BCA; Doledec and Chessel, 1987), was used to investigate if the habitats were characterized by different environmental settings. The BCA is a multivariate analysis which partitions and maximizes the variance between groups of one qualitative variable. A matrix of environmental variables (including latitude, temperature, sigma-theta, slope, northness, eastness, general, plan and profile curvature, Surface to Planar, BPI, and VRM) per habitat segment was used as the response variable and the habitats (all habitats separately or categorized into biogenic, hard substrate and soft substrate habitats) were used for partitioning. This analysis aims to discriminate the segments based on their environmental conditions and how much of this variation is explained by the habitats.
Chi-square tests were used to test for differences in coral distribution according to the three qualitative variables: morphology, slope and location. Chi-square tests were undertaken using the image data, rather than habitat segments because they involve the geomorphological classes (see Section Environmental Data) and habitat segments can cross geomorphological classes.
All analyses were performed using the open source software R. The R packages “ade4” (Dray and Dufour, 2007) and “vegan” (Oksanen et al., 2016) were used for the diversity measurements, PCAs and BCA.
Results
Of the 14,874 analyzed images, coral habitats were recorded on 4,191 images, of which 2,350 images were selected for substrate cover measurements and species identification (“subset” images).
Substrate and Scleractinian Coral Framework Cover
Seven geological substrate types were encountered and divided into two main categories: (i) hard substrate, consisting of hardground/bedrock, hardground/bedrock covered by a (thin) layer of soft sediment, consolidated mud, boulders, pebbles and/or cobbles, and (ii) soft substrate, consisting of mud/sand and/or gravel.
Four biogenic substrate types were observed: live scleractinian framework, dead scleractinian framework, scleractinian coral rubble, and shell debris. Percentages of these categories were summed to obtain the percentage of total framework (live and dead) and total scleractinian cover (live and dead framework and rubble). Scleractinian framework and rubble included the reef-forming species (L. pertusa, M. oculata, and S. variabilis) as well as another colonial scleractinian Enallopsammia rostrata. E. rostrata occurred mostly, if not only, on vertical features, such as steps or walls, but did not form reefs or dense structures like the reef-forming scleractinians do. However, the cover of E. rostrata was included in the total scleractinian framework and the total cover, because of the three-dimensional structures they can form compared to solitary scleractinians that are usually only a few centimeters high.
Up to four substrate types were recorded for an image, although the seafloor was covered by either two or three substrates on most images.
Coral Habitats and Species
Eleven different coral habitats were observed (Figures 2A–J) using the CoralFISH classification: coral reef, coral rubble, colonial scleractinians on hard substrate, solitary scleractinians on hard substrate, antipatharians or gorgonians on hard substrate, mixed corals on hard substrate, colonial scleractinians on soft substrate, solitary corals on soft substrate, gorgonians on soft substrate, seapens on soft substrate and mixed corals on soft substrate. Hereafter, hard and soft substrate will be abbreviated as “HS” and “SS,” respectively, and will be used in combination with the structuring coral type to indicate the habitat. A description of each habitat is given in the (Supplementary Data S1) including their linear, their coral species composition and environmental settings.
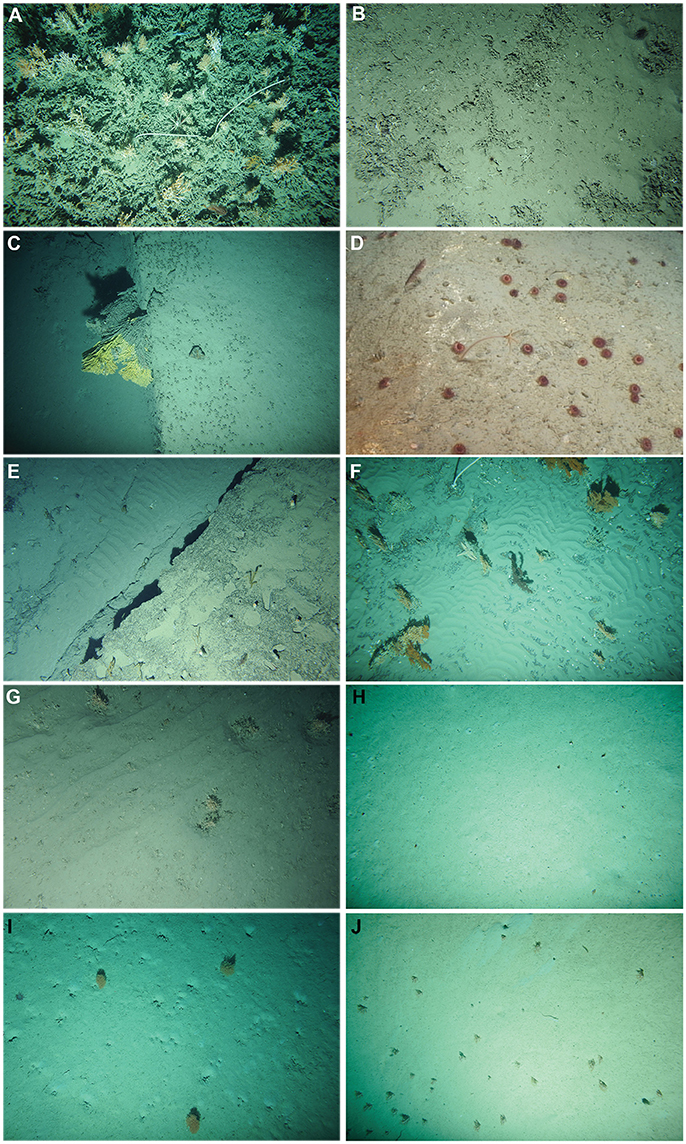
Figure 2. Example images of the coral habitats of the Bay of Biscay: (A) Coral reef (BobGeo 2009), (B) Coral rubble (Evhoe 2012), (C) Colonial scleractinians on hard substrate (Evhoe 2011), (D) Solitary scleractinians on hard substrate (BobEco 2011), (E) Antipatharians or gorgonians on hard substrate (Evhoe 2012), (F) Mixed corals on hard substrate (Evhoe 2009), (G) Colonial scleractinians on soft substrate (Evhoe 2012), (H) Solitary scleractinians on soft substrate (Evhoe 2011), (I) gorgonians on soft substrate (BobGeo 2009) and (J) Seapens on soft substrate (Evhoe 2010). Mixed corals on soft substrate is formed by the same species as (I,J); therefore, a representative image of this habitat is not added. Copyright of all images in this figure: Ifremer. Ifremer provided permission for reproduction.
The coral habitats were observed in total linears (or length; see Section Habitat Segments) from as little as 6 m to more than 10 km (Table 3). The most common habitat was coral rubble, with a linear of 18.1 km, equivalent to 10.1% of the total observed linear; followed by coral reef (linear: 10.7 km; 6.0% of the total observed linear). Both rubble and reef habitats were also observed on the highest number of segments (162 and 106 respectively). Seapens SS was the third most observed habitat (linear: 6.7 km; 3.8% of the total observed linear) and thereby the most common soft substrate habitat. Mixed corals SS was the habitat on soft substrate that was observed the least (linear: 365 m; 0.2% of the total observed linear) and the “solitary scleractinians HS” habitat was observed only once, with a linear of 6.0 m (less than 0.01% of the total observed linear). Due to this small contribution, this latter habitat was excluded from any analyses as none of the three images of this habitat was selected for the “subset” image dataset (Table 3).
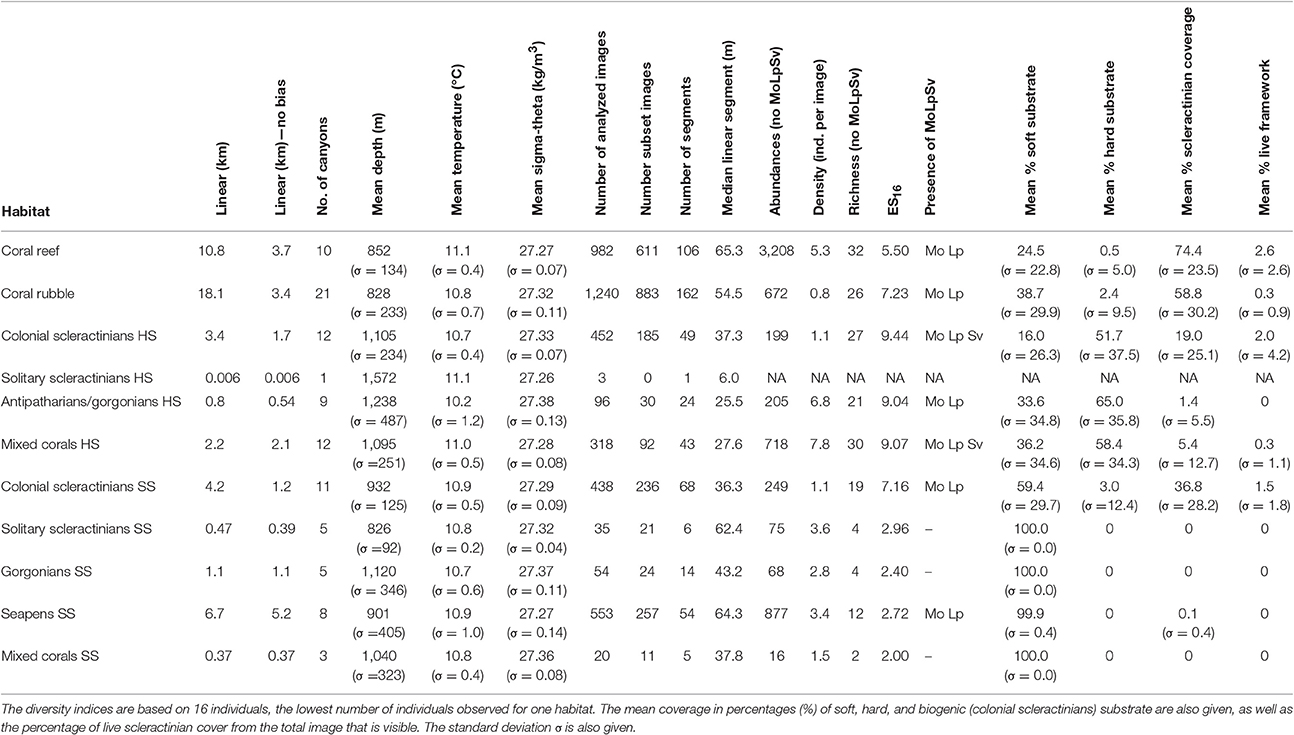
Table 3. Information of the different coral habitats in the Bay of Biscay including the linear (with and without BobEco cruise, which had a sampling bias toward scleractinian corals); the environmental factors (mean and standard deviation σ); the abundances, densities, species richness, and diversity indices without Madrepora oculata (Mo), Lophelia pertusa (Lp), and Solenosmilia variabilis (Sv); the presence of these scleractinians, and the percent cover of substrate types.
Even though rubble and reef habitats had the longest total linear, these are mainly caused by a higher sample size and a few segments that were much longer than most segments. The median segment length of coral reef (65.3 m) was similar to the median segment lengths of seapens SS (64.3 m) and solitary scleractinians SS (62.4 m) (Table 3). Coral rubble has a smaller median segment (54.5 m; Table 3).
The Coral Assemblages
Community structure
A total of 6,287 individual corals were observed belonging to 59 coral morphotypes (Tables 3, 4). Including the three reef-forming scleractinian corals—L. pertusa, M. oculata, and S. variabilis—of which coverage was measured, instead of abundances, the total added up to 62 morphotypes. Thirty-four of these morphotypes were identified down to at least genus level. The most abundant morphotypes that could be counted, comprising together 53% of the coral individuals were the antipatharian Leiopathes spp. (2,089 individuals; 33.2%), the primnoid Narella versluysi (677 individuals; 10.8%) and the seapen Kophobelemnon cf. stelliferum (581 individuals; 9.2%) (Table 4). The soft coral suborder Alcyoniina (Alcyonacea) was observed the least with 17 individuals (0.3% of the total observed coral individuals) (Table 4).
The abundances, densities (individuals per image) and species richness varied according to the coral habitat types (Table 3). Abundance patterns between habitats did not strictly follow their sample size patterns. In particular, the most common habitat, coral rubble, had a low number of corals (672 individuals) relative to coral reef (3,208 individuals). Overall, the mean coral density within habitat segments did not correlate with the linear of the segments (R = −0.003, p = 0.955), suggesting that the size of the habitats did not influence the aggregation of corals. Mixed corals HS, antipatharians/gorgonians HS and coral reef achieved the top three highest coral densities (7.8, 6.8, and 5.3 individuals/image respectively). The highest densities on soft substrate habitats were half of those on hard substrate (solitary scleractinians SS: 3.6 individuals/image; seapens SS: 3.4 individuals/image). Similarly, the total number of morphotypes on hard substrate and biogenic habitats ranged from 21 to 32, of which coral reef and mixed coral HS are the most species rich (32 and 30 morphotypes respectively). The total number of morphotypes on soft substrate habitats ranged from 2 to 19, of which colonial scleractinians SS and seapens SS are the most species rich (19 and 12 morphotypes respectively).
Species accumulation curves showed that, with the exception of coral reef, seapens SS and mixed corals HS, habitats were undersampled as no curve reached an asymptote (Figure 3). Comparison of diversity values, even with indices limiting sampling biases, should, therefore, be taken cautiously. Some patterns, however, still stand out. The diversity of the soft substrate habitats formed by solitary scleractinians, gorgonians, seapens and a mix of these corals was very low compared to the coral habitats on hard substrate and the biogenic habitats, in line with the density and richness patterns (Figure 3; Table 3). A noticeable exception is the colonial scleractinians SS habitat, which diversity was similar to that of biogenic habitats. The two best characterized habitats, coral reef and seapens SS, shared a low equitability, as shown by the slope of the species accumulation curves. In the coral reef habitat, three morphotypes and a cluster of species were highly abundant, contributing to 84% of total abundance: the antipatharian Leiopathes spp. (1,923 individuals), the antipatharian Stichopathes gravieri (293 individuals) and the gorgonian N. versluysi (238 individuals) and unidentified antipatharians (212 individuals). For the seapens SS habitat, K. cf. stelliferum and Distichoptilum gracile contributed to 95% of the total abundance.
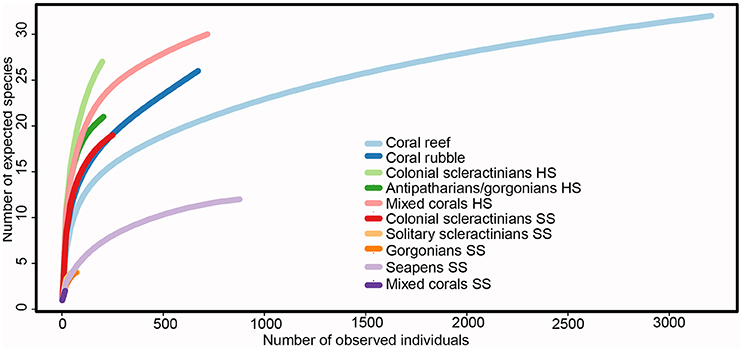
Figure 3. Rarefaction curves of the coral habitats, excluding the reef-forming scleractinians M. oculata, L. pertusa, and S. variabilis. The number of species (y-axis) is based on the number of individuals (x-axis) in each habitat. Each color represents a different coral habitat.
In general, soft substrate habitats had a lower coral abundance, density and diversity than those of biogenic and hard substrate coral habitats. Exceptions are the coral abundance on seapens SS that is relatively high and the diversity on colonial scleractinians SS that is similar to that of biogenic habitats.
Community composition
Two PCAs were used to explore variations in coral community composition between habitats. In the co-variance PCA (Figure 4A), the relative abundances of the species are considered but for this reason, reef-forming corals were excluded. In the correlation PCA (Figure 4B), all species were included and characterized by either their abundances or percent cover, but data were normalized.
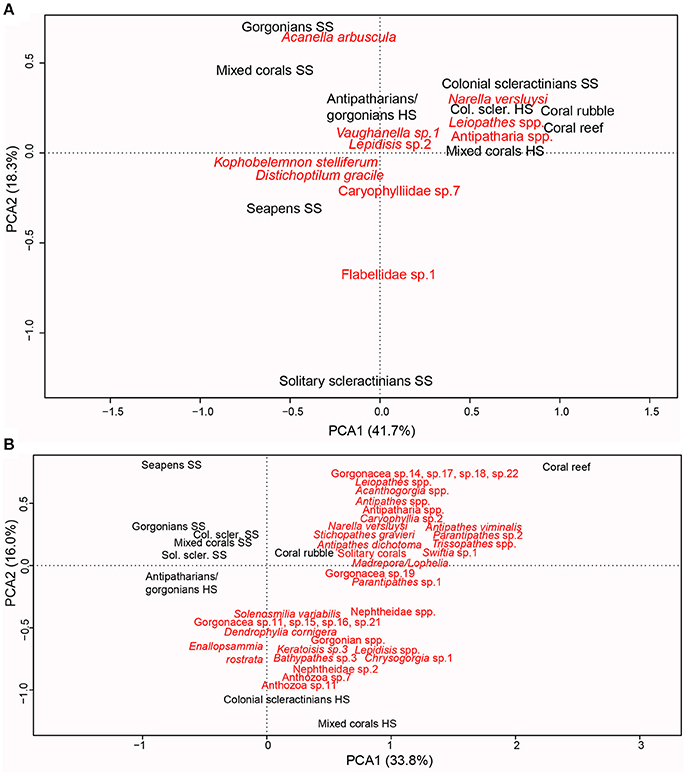
Figure 4. Biplot ordination of the coral habitats (in black) and coral taxa (in red) in the two first axis of (A) a covariance PCA computed on Hellinger-transformed abundances of non-reef forming coral taxa per habitat. For clarity only those species contributing to at least 5% of the variance along one axis are represented. (B) A correlation PCA computed on raw abundances of non-reef forming corals or percent cover of reef-forming corals (M. oculata, L. pertusa, and S. variabilis). For clarity, only those species contributing for at least 3% of the variance along one axis are represented. Col. scler. HS, Colonial scleractinians HS; Col. scler. SS, Colonial scleractinians SS; Sol. scler. SS, Solitary scleractinians SS.
Without considering the reef-forming scleractinians, the coral composition discriminates hard substrate or biogenic habitats from soft substrate habitats along the first axis of the covariance PCA, explaining 42% of the variance. The only exception to this pattern is colonial scleractinian SS, which clusters with the hard substrate and biogenic habitats. This cluster of hard substrate, biogenic and colonial scleractinians SS habitats is dominated by the gorgonian N. versluysi as well as Leiopathes spp. and other unidentified antipatharians. The second axis of the PCA, explaining 18% of the variance in coral composition, discriminated the different soft substrate habitats, with gorgonian SS and mixed corals SS mainly dominated by the gorgonian Acanella arbuscula, seapens SS dominated by K. cf. stelliferum and D. gracile and solitary scleractinians SS dominated by a caryophyllid and a flabellid.
By adding the percent cover of colonial scleractinians in a correlation PCA, the coral composition further discriminates the habitats dominated by L. pertusa/M. oculata that form biogenic habitats (coral reef and coral rubble) and colonial scleractinians SS, from the habitats dominated by S. variabilis on hard substrate (colonial scleractinians HS). The former cluster of biogenic habitats is characterized by the occurrence of a mix of gorgonians and antipatharians while the later cluster of hard substrate habitats is colonized mainly by gorgonians. The only antipatharian (Bathypathes sp. 3), characterizing hard substrate habitats, was observed once. The morphotype Anthozoa sp. 7, either a gorgonian or an antipatharian, and a soft coral from the suborder Alcyoniina (Nephtheidae sp. 2) also characterizes hard substrate habitats. Solitary corals dominated biogenic reefs, on the other hand.
The abundances of antipatharians, gorgonians and seapens were significantly correlated with scleractinian coral cover (Figure 5). The abundance of antipatharians was positively correlated with each of the scleractinian cover measurements (live and dead framework, coral rubble, total framework and total coral cover), but most strongly with the total cover of framework (r = 0.315, p ≤ 0.001). Of the three coral cover measurement separately, antipatharians correlated mostly with live coral framework (r = 0.3, p ≤ 0.001) and the least with coral rubble (r = 0.148, p ≤ 0.001). The abundance of gorgonians on hard substrate was also positively, but weakly, correlated with live and dead coral framework (r = 0.162, p ≤ 0.001 and r = 0.074, p ≤ 0.001, respectively). A significant correlation was found between the abundances of antipatharians and gorgonians on hard substrate (r = 0.176, p ≤ 0.001).
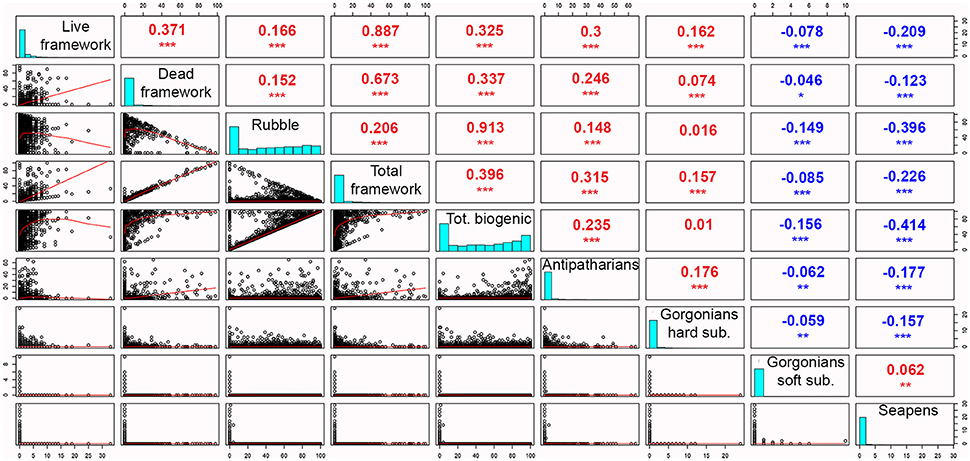
Figure 5. Correlation matrix between scleractinian coral cover and the abundances of antipatharians, gorgonians on hard substrate, gorgonians on soft substrate and seapens. Lower matrix: correlation plots, upper matrix: Spearman coefficient and significance of the test (p-value: * ≤ 0.05; ** ≤ 0.01, *** ≤ 0.001), diagonal data: distribution for each variable.
The abundance of seapens were negatively correlated with each of the measurements of scleractinian coral cover as well as with antipatharians and gorgonians on hard substrate (−0.123 ≤ r ≤ −0.414; p = 0.001). The correlation of the abundance of gorgonians on soft substrate was negative with coral rubble and total cover (r = −0.149 and −0.156, p = 0.001) but close to zero with the three different framework measures and antipatharian/gorgonian abundances.
Distribution of Coral Habitats
Coral habitats were observed in all 24 canyons of the Bay of Biscay that were surveyed during this study and on 39 out of 46 dives analyzed here (Tables 2, 5). On the upper slope between Odet and Blavet Canyons, no coral habitats were observed.
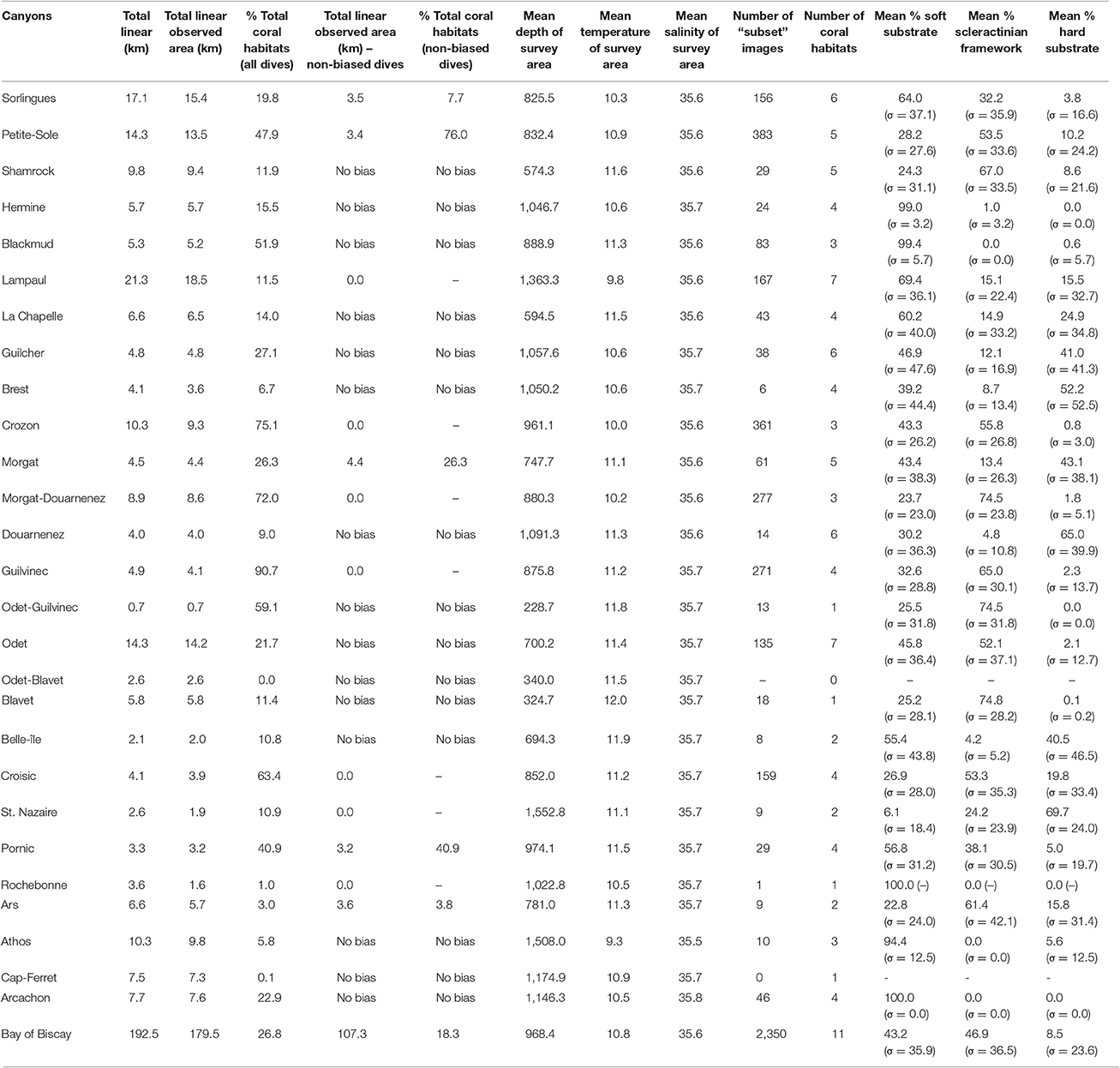
Table 5. A description of the canyons including the linear of observed area, the percentage of coral habitat taken from the observed area linear (both biased and non-biased for scleractinians), the environmental settings of the canyons and the mean substrate cover (in percentages; standard deviation σ between brackets).
In most canyons, at least four different coral habitats were observed (55.6% of the canyons), up to a maximum of seven habitats in Lampaul and Odet Canyons (Figure 6, Tables 3, 5). Coral rubble was the most common (21 canyons) and mixed corals SS was the least common habitat observed (3 canyons). Reefs and colonial scleractinians on hard and soft substrate were always associated with coral rubble at the scale of individual canyons, except in Blackmud Canyon, where a small proportion of colonial scleractinians HS—scleractinians on a vertical wall—were observed (less than 0.1% of the total coral linear in this canyon), but no coral rubble. Coral rubble, on the other hand, was also observed in canyons where no live scleractinian habitats were encountered.
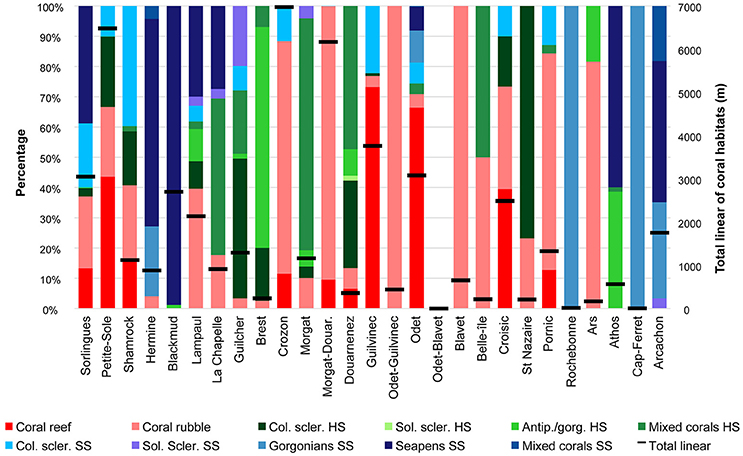
Figure 6. The distribution of the coral habitats in the submarine canyons of the Bay of Biscay. The stacked barplots show the proportion of each coral habitat (primary y-axis) in the canyons (x-axis) of the Bay of Biscay taken from the total linear of coral habitats within that canyon that is indicated by the black lines (secondary y-axis). The canyons are arranged from the most northern canyon (Sorlingues; on the left of the x-axis) to the most southern canyon (Arcachon; on the right of the x-axis) that are investigated in this study. Col. scler, Colonial scleractinians; Sol. scler, Solitary scleractinians; Antip./gorg., Antipatharians/gorgonians.
Overall, habitats formed by colonial scleractinians were absent in the southern part of the Bay of Biscay (Figure 6). One exception was the coral rubble habitat in Ars Canyon (linear = 173.7 m; 81.6% of the coral linear in that canyon). The Aquitaine margin was dominated by coral habitats on soft substrate (total linear = 2.1 km, corresponding to 90% of the coral habitat linear on this margin) (Figure 6).
A PCA was used to investigate (dis)similarities in habitat composition between canyons (Figure 7; Table 5). The first axis (explaining 31% of the variance) discriminates canyons dominated by hard substrate and/or biogenic habitats (including colonial scleractinians SS) from canyons dominated by soft sediment habitats. The three surveyed canyons incising the Aquitaine margin belonged to this second group. The second axis (explaining 19% of the variance) discriminates canyons dominated by habitats formed by L. pertusa/M. oculata from canyons dominated by other coral species on hard substrate. The PCA thus showed that the associations of coral habitats tend to characterize three groups of canyons: (i) canyons dominated by soft substrate habitats formed by other corals than reef-forming scleractinians, (ii) canyons dominated by reef, rubble and colonial scleractinians on soft substrate, and (iii) canyons dominated by hard substrate habitats.
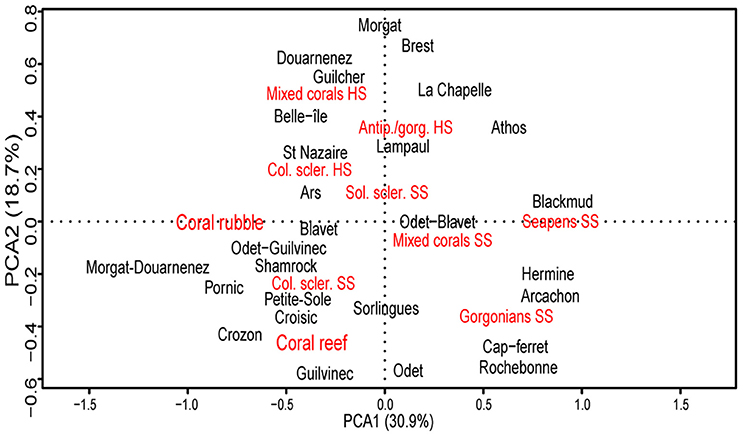
Figure 7. Biplot ordination of canyons (in black) and coral habitats (in red) in the first two axis of a covariance PCA computed on the Hellinger-transformed linear of coral habitats per canyon. The Solitary scleractinians HS habitat was removed prior to analysis. Antip./gorg., Antipatharians/gorgonians; Col. scler., Colonial scleractinians; Sol. scler., Solitary scleractinians.
Oceanographic and Geomorphological Settings
The coral habitats were observed mostly between 600 and 1,200 m water depth (Figure 8A; Table 3). Coral rubble was the shallowest (228 m; Odet-Guilvinec) and antipatharians/gorgonians HS the deepest (2,348 m; Athos) coral habitat. Seapens SS had the widest depth range of over 2,000 m (234–2,305 m water depth), while the narrowest depth range was 332 m (solitary scleractinians SS: from 752 to 1,085 m water depth).
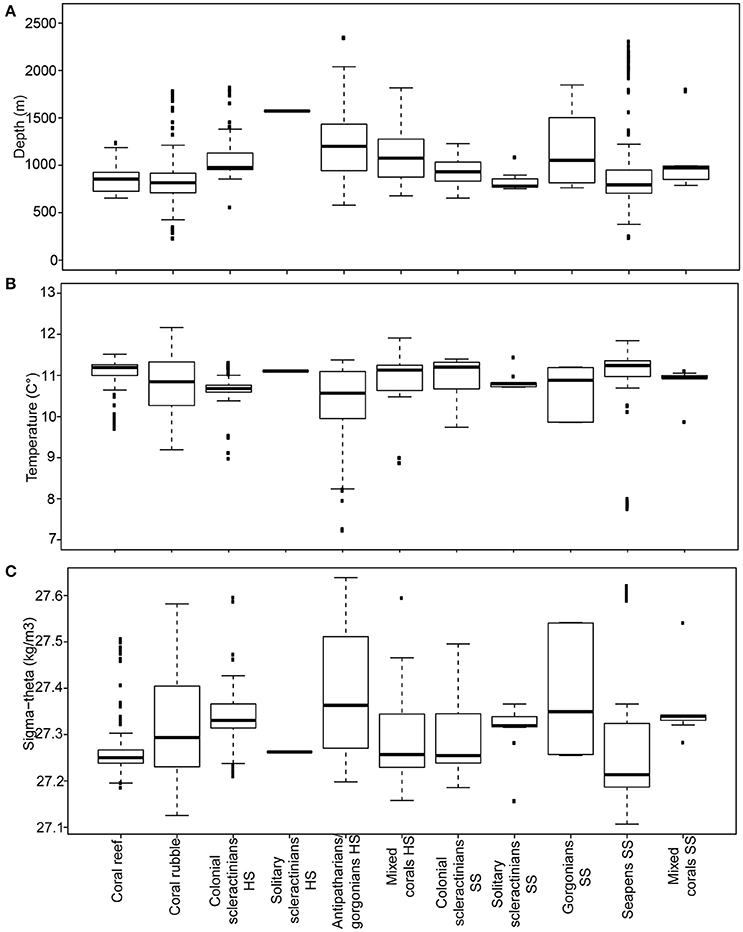
Figure 8. Boxplots of (A) depth, (B) temperature, (C) water density (sigma-theta) for the 11 coral habitats observed in the Bay of Biscay.
Temperatures ranged from 7 to 12°C, with a mean temperature of 10.8°C (Figure 8B) and sigma-theta ranged from 27.11 to 27.64 kg/m3 (Figure 8C). Patterns of variations in temperature and water density between habitats were similar to the depth patterns.
BCAs investigated the relationship between coral habitats and habitat type and the oceanographic characteristics as well as the derivatives of the bathymetry to assess if these environmental setting vary among habitats (Table 5). Environmental multivariates were significantly different between habitats and habitat type (p = 0.001). The coral habitats, however, explained only 9.1% of the variance in environmental settings. Habitat type (hard substrate, soft substrate and biogenic substrate that included colonial scleractinians SS in this analysis) explained even less (5.3%).
The influence of geomorphology was assessed at a macro-scale by comparing the habitat distribution with the expert-supervised classification of geomorphological features. Coral habitats were observed on 12 of 15 classes on the 100 m resolution, while on the higher (15/25 m) resolution these habitats were observed on 18 of 20 geomorphological classes (Supplementary Data S2). At both resolutions, the majority of images of coral habitats were located on canyon or interfluve flanks, compared to other geomorphological classes.
The occurrences of coral habitats on the broadest scale of morphology—canyon, interfluves and upper-slope—differed from a random distribution on both resolutions (high res.: χ2 = 1,076, df = 18, p < 0.001, Figure 9A; low res.: χ2 = 289.2, df = 18, p < 0.001). At both resolutions, and for most coral habitats, occurrences were more frequent in the canyons than on the interfluves and the upper slope. The only consistent exception was coral rubble that was more frequent on the interfluves/upper slope than in the canyons. The seapens SS habitat was also more frequent on the interfluves according to the low bathymetric resolution but this pattern was not consistent at the high resolution (data not shown). The location on the northwestern or southeastern flanks of canyons and interfluves also had a significant influence on the occurrences of coral habitats (high res.: χ2 = 511.89, df = 10, p < 0.001, Figure 9B; low res.: χ2 = 598.71, df = 9, p < 0.001). At both resolutions, the occurrences of most coral habitats were more frequent on the northwestern flank than on the southeastern flank. The seapens SS and mixed corals HS habitats were coherent exceptions, with more occurrences on the southeastern flank. Finally, the influence of slope on the coral habitats was also investigated. At both high and low resolution, slope had a significant influence on the distribution of coral habitats (high res.: χ2 = 595.84, df = 27, p < 0.001, Figure 9C; low res.: χ2 = 1,140.9, df = 27, p < 0.001). The slope, however, mainly influenced hard substrate and biogenic habitats while its influence was low on soft substrate habitats. Furthermore, the distribution of coral rubble was highly skewed toward smoother slopes compared to all other habitats. These two patterns were consistent at both resolutions. The colonial scleractinians HS and seapens SS habitats were also more frequently observed on smoother slopes (<10°), but on only one of the bathymetrical resolutions, respectively the low or high resolution (data not shown).
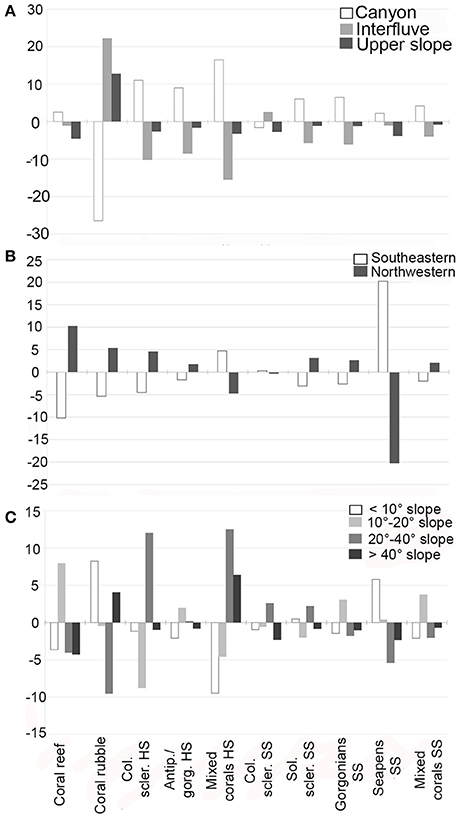
Figure 9. Standard residuals of chi-square tests of the frequency of images of each habitat on (A) the different canyon morphologies, (B) the southeastern and northwestern flank of the canyon/interfluves, and (C) the four slope intervals of the canyon/interfluves flank. If the residuals are less than −2, the observed frequency is less than the expected frequency according to a random distribution. If the residual is greater than 2, the observed frequency is greater than the expected frequency. The lower (< −2) or higher (>2) the residual value is, the stronger is the contribution of this category to the observed distribution. Col. scler. HS, Colonial scleractinians HS; Antip./Gorg. HS, Antipatharians/gorgonians HS; Col. scler. SS, Colonial scleractinians SS; Sol. Scler. SS, Solitary scleractinians SS.
Discussion
This study greatly increases the knowledge of coral habitats in the Bay of Biscay including a large number of canyons and a high diversity of coral habitats representing a total linear of nearly 50 km. Thus far, there have been few studies within the Bay of Biscay. They mainly focused on scleractinian species (Joubin, 1922; Zibrowius, 1980; Reveillaud et al., 2008) and facies (Le Danois, 1948; De Mol et al., 2011; Sanchez et al., 2014) or coral habitats and assemblages, in Whittard, Dangeard or Explorer Canyons (Howell et al., 2011; Huvenne et al., 2011; Morris et al., 2013; Davies et al., 2014; Robert et al., 2015).
Le Danois (1948) described both scleractinian and sand/mud facies on the continental margin of the Bay of Biscay as one of the first studies in this basin. He observed (i) aggregations of the seapens K. stelliferum, Umbellula spp., and Pennatula spp. emerging from muddy bottoms between 500 and 1,000 m depth, particularly in the north and south of the basin, (ii) scleractinian facies formed by the reef-forming species L. pertusa, M. oculata, and S. variabilis on the Celtic and Armorican margins, and (iii) several gorgonians, antipatharians and solitary scleractinians that were associated with this scleractinian facies including some species that are also observed in the present study, e.g., N. versluysi and Antipathes dichotoma. The present study included canyons that were not visited by Le Danois (1948) and habitats were seen in situ on the image footage. However, it was not possible to precisely compare distribution patterns with the present study because of low positioning accuracy (before GPS) in Le Danois (1948)'s study.
Influence of Substrate Type
Distribution of Coral Habitats at Regional and Canyon Scales
The distribution of CWC habitats is heterogeneous in the canyons of the Bay of Biscay. The majority of canyons in this study hosts four or more and up to seven, coral habitats in the same canyon. The heterogeneity in the distribution of coral habitats seems to be largely driven by the substratum type, both at the scale of the Bay of Biscay and at the scale of canyons; the most important patterns being (i) the absence of live scleractinian habitats in canyons on the southern Armorican margin and the Aquitaine margin and (ii) the dominance of habitats on soft substrate in canyons incising the Aquitaine margin.
At the canyon scale, canyons can be divided into three groups based on their dominant substrate type, depending on their coral habitat composition. The canyon grouping matches with the mean percentage of substrate cover within each group (soft, hard, and/or scleractinians).
In general, the canyons in the southern part of the Bay of Biscay are smoother and more sedimentary without geomorphological features known for their hard substrate when compared with canyons in the northern or central parts of the Bay of Biscay which present falls and cliffs (Bourillet et al., 2010). The canyons on the Aquitaine margin also seem to have a different sedimentation regime, because of their shorter distance to the shore (Mulder et al., 2012; Schmidt et al., 2014), similar to Nazaré Canyon (de Stigter et al., 2007). The southern canyons, e.g., Cap-Ferret and Capbreton Canyons exhibit higher sedimentation rates and more recent sediment input than canyons on the Armorican margin (Mulder et al., 2012; Schmidt et al., 2014). This sediment was not being remobilized due to the low internal wave energy and lower current speeds in the canyons of the Aquitaine margin (Koutsikopoulos and Le Cann, 1996; Mulder et al., 2012), preventing erosion and, therefore, the exposure of hard substrate. This erosion is observed in Blackmud, Audierne and Guilvinec Canyons on the northern and central Armorican margin (Mulder et al., 2012; Khripounoff et al., 2014). Such a regime of higher sedimentation toward the southern Bay of Biscay likely explains both the quasi absence of scleractinian habitats and the dominance of soft substrate habitats on the southern Armorican margin and the Aquitaine margin.
Variations in sedimentation regime at canyon scale may also account for some of the variability in habitat composition and explain the statistically significant differences observed in the distribution of scleractinian and seapen habitats between the flanks of canyons. The Blackmud Canyon provides a good example. Due to its location on the Armorican margin, elevated currents and lack of recent sedimentation (Mulder et al., 2012), this canyon was a good candidate for scleractinian habitats. However, only the southeastern flank could have been explored, which turned out to be dominated by seapens on soft substrate.
This asymmetry in canyons—one eroded flank and one sedimentary flank—has been previously observed in the Bay of Biscay (Van Rooij et al., 2010; De Mol et al., 2011; Huvenne et al., 2011; Sanchez et al., 2014), in the Mediterranean (Orejas et al., 2009; Fabri et al., 2014) and off Canada (Mortensen and Buhl-Mortensen, 2005). The observed asymmetry is due to a dominated current, e.g., a westerly current in Cassidaigne Canyon, or dense shelf water cascading in Cap de Creus and Lacaze-Duthiers Canyons (Orejas et al., 2009; Fabri et al., 2014). In the Bay of Biscay, the slope current flows polewards along the slope (Pingree and Le Cann, 1989, 1992; Koutsikopoulos and Le Cann, 1996) in a dominant southeastern—northwestern direction, eroding the northwestern flank from canyons and exposing hard substrate on this flank. This important current in the Bay of Biscay has therefore been suggested to favor the development of species needing hard substratum on the northwestern flank compared to the more sedimentary southeastern flank (Van Rooij et al., 2010). In the present study, however, the occurrence of mixed corals on hard substrate on the sedimentary southeastern flank contradicts previous observations and hypothesis. This habitat occurred more often on steep areas with slopes of more than 20°, suggesting that this apparent exception may be due to the steep topography itself or the accelerated currents it forms preventing deposition of sediment. This local prevention of sediment deposition may potentially lead to the occasional exposure of hard substrate on the southeastern flank of canyons.
To summarize, the distribution patterns of coral habitats—a dominance of soft substrate habitats in canyons of the Aquitaine margin and an absence of live scleractinian habitats in the southern Bay of Biscay—can be related to the substrate heterogeneity, influenced by differences in morphology and hydrology, at the scale of the Bay of Biscay. Coral habitat distribution also varies at a canyon scale, due to an asymmetry of hydrological regimes within canyons, leading to eroded northwestern flanks that enhance the colonization by scleractinians and more sedimentary southeastern flanks favoring soft sediment habitats.
Variability of Coral Assemblages among Substrate Type
Coral habitats in the Bay of Biscay are dominated by the three reef-forming scleractinians as well as the antipatharian Leiopathes spp., the gorgonian N. versluysi and the seapen K. cf. stelliferum. Coral assemblages, however, are much more diverse than these few dominant species. A total of 62 coral morphotypes were identified in this study. The composition of coral assemblages and the correlations between coral densities or cover all indicated a clear dichotomy between soft sediment dominated habitats and hard substrate/biogenic habitats.
Corals are usually specialized in either hard or soft substrate. Seapens are adapted to live in soft sediment by a peduncle which anchors the colony in the sediment (Williams, 1995). Most antipatharians, colonial scleractinians and most gorgonians need hard substrate to settle (Roberts et al., 2009; Wagner et al., 2012). Members of the gorgonian family Isididae, e.g., A. arbuscula, can also occur in soft sediment due to a root-like holdfast (e.g., Mortensen and Buhl-Mortensen, 2005; Wienberg et al., 2009).
The biogenic and hard substrate habitats share coral morphotypes and clustered together in the PCA excluding the three reef-forming scleractinians. The coral assemblage of colonial scleractinians on soft substrate habitat also clustered with these habitats, despite the dominance of soft sediment in this habitat. Coral species preferring hard substrate could settle on the scleractinian framework emerging from the soft sediment. These corals, therefore, can be present in a habitat with a non-optimal substrate cover, as was observed in Nora Canyon in the Mediterranean Sea (Taviani et al., in press). With the addition of the reef-forming scleractinians in the analysis, the biogenic reef assemblages differed from those on hard substratum; in reef, L. pertusa/M. oculata colonies are present, while S. variabilis is absent. Additionally, the reef composition is characterized by different gorgonian morphotypes than that of hard substrate habitats and it also includes antipatharians and solitary corals, that are almost absent in hard substrate habitats.
While the biogenic and hard substrate habitats shared some species, the coral assemblages of the soft substrate habitats (excluding colonial scleractinians) differ more from each other as shown by the PCA (Figure 4A), and thus, show a higher species turnover. The alpha-diversity of these habitats is also generally lower than that of the coral assemblages of biogenic and hard substrate habitats. The mixed corals on soft substrate habitat is characterized by the gorgonian A. arbuscula, also characterizing gorgonians on soft substrate, but it also includes the seapen K. cf. stelliferum, characterizing the seapens habitat. The few segments of this mixed coral habitat are surrounded by either gorgonian or seapen habitat, what could suggest that the mixed coral habitat may function as a “transition” zone between the gorgonian or seapen habitats. Even though these three habitats share K. cf. stelliferum and A. arbuscula, the PCA does separate the seapen habitat from the other two soft sediment habitats, due to other seapen species, e.g., D. gracile, which were exclusively seen in the seapens habitat.
Influence of Internal Tides and Geomorphology on Coral Habitats
Hydrodynamics, such as downwelling and tidal currents, that may be influenced by the local seafloor topography, are important for the food transport and supply to CWCs, as shown in the Mingulay Reef Complex (Davies et al., 2009) and Rockall Bank (Soetaert et al., 2016). The Bay of Biscay is characterized by peculiar hydrodynamics mainly due to the numerous canyons and the steep continental slope. Tidal currents strengthened along the canyon seafloor and internal waves on the upper part of the slope favor exchanges between deep and superficial water masses and are reported in several canyons in this basin (Pingree and Mardell, 1985; de Madron et al., 1999; Mulder et al., 2012; Pichon et al., 2013; Khripounoff et al., 2014). Measurements from Guilvinec canyon, for example, show internal waves with vertical speeds enhancing the renewal of water with considerable daily variations in temperature, salinity and oxygen (Khripounoff et al., 2014). Thus, it increases the amount of suspended material, a potential food for CWCs, that could move to water depths up to 2,800 m (Pichon et al., 2013), corresponding to the observed maximum water depth of antipatharians/gorgonians on hard substrate habitat in this study. Coral habitats can also be related to benthic nepheloid layers, as observed in Cap-Ferret Canyon (de Madron et al., 1999) including a layer at the same depth (1,850 m) as gorgonians on soft substrate habitat.
The presence of coral habitats in this study indicates that corals are able to form habitats (with a minimum size of 25 m2 according to the CoralFISH definition) on hard or soft bottoms within the canyons of the Bay of Biscay, but the environmental factors available at the resolution in this study did not discriminate habitats. Several habitat suitability models have predicted that the canyons are indeed (highly) suitable for octocorals (Yesson et al., 2012), antipatharians (Yesson et al., in press) and colonial scleractinians (Davies and Guinotte, 2011), but temperature, salinity, water density, slope, rugosity and bathymetric position index (BPI) came out as important factors in these models controlling the distribution (Davies and Guinotte, 2011; Howell et al., 2011; Yesson et al., 2012, in press; Robert et al., 2015). Most “habitat” suitability models were, however, devoted to predict occurrences of the coral (sub)order or species only and the results may not be the same if the suitability of habitats is being predicted (Howell et al., 2011), similarly to the model of Robert et al. (2015) predicting abundances, species richness and diversity.
Nevertheless, with an estimated height between 10 and 60 cm emerging from the sediment of which the majority is dead, scleractinian reefs in the Bay of Biscay appear to be lower than those described in other areas along the NE Atlantic margins; scleractinian reefs along the coast of Norway are very high, between 2 and 33 m, and the outermost part is dominated by live L. pertusa colonies (Mortensen et al., 2001; Flogel et al., 2014) and carbonate mounds off Ireland, built up by dead scleractinian framework and sediment, can also reach up to several hundreds of meters high, covered by coral rubble on the flanks and live L. pertusa and/or M. oculata colonies (~0.75–1 m in height) on the summit (Huvenne et al., 2005, 2007; Wheeler et al., 2005, 2007). The area covered by the reefs in the Bay of Biscay also appears smaller. The minimum size of an individual Norwegian reef is ~50 m in diameter, while the largest measures ~500 m (Mortensen et al., 2001). The live scleractinian reef covering the summits of carbonate mounds off Ireland can approach 500 m in size (Huvenne et al., 2005). Approximately three quarters of the reef segments in our study are smaller than 100 m in linear and almost 15% is smaller than 25 m (26% if all coral habitats are considered). Therefore, although CWCs find suitable conditions to form habitats on hard or soft bottom in the canyons of the Bay of Biscay, scleractinian corals do not inhabit their optimal conditions here, as observed in northern continental margins of the NE Atlantic. This may be due to high sedimentation rates (that may be too high for prestige scleractinian reefs) in canyons, a potential difference in hydrology, the steepness of the topography and/or differences in food supply and quality. Nevertheless, the diversity of CWC habitats and species identify the Bay of Biscay as an essential section or transition zone for coral habitats between the north European margin and the Mediterranean, as it is suggested by Reveillaud et al. (2008) and De Mol et al. (2011).
There is a high overlap between the predicted suitable areas of these coral orders/species, including the canyons for all corals (Davies and Guinotte, 2011; Yesson et al., 2012, in press). This pattern is emphasized by the present study as the environmental factors (e.g., temperature), as well as the derivatives of the 100 m resolution bathymetry do not discriminate a specific environment for each coral habitat. In other words, CWC habitats in the Bay of Biscay share similar environmental settings, at the resolution at which these environmental factors are available. The size of coral habitats, of which most segments measured less than 100 m, compared to the resolution of the environmental factors, ranging from 100 m to 0.083° latitude (~10 km) easily explain the difficulty to discriminate particular environmental settings for the different coral habitats. The observed pattern also points out the limits of habitat suitability models which were based on a rather low resolution of oceanographic parameters (0.04°–1° latitude) (Davies et al., 2008; Davies and Guinotte, 2011; Yesson et al., 2012). Limited resolution understandably causes uncertainties in habitat mapping and suitability models (Davies and Guinotte, 2011; Rengstorf et al., 2012) whereby some local features, such as individual coral habitats, could be missed. The bathymetry is often available in high(er) resolutions for a mosaic of better studied areas associated with specific cruises. A comparison of models using different resolutions of bathymetrical data resulted into significant changes in the predictive habitat suitability maps, and a minimum resolution of 250 m was determined as necessary to identify individual coral mounds (Rengstorf et al., 2012). In our study, the slope classes (of the geomorphological classes with a 15/25 m resolution) resulted in differential coral habitat distribution; live coral habitats tend to occur on steeper areas (>10°) and coral rubble on flatter areas (<10°), an influence that appeared to be stronger for hard substrate and biogenic habitats than for soft substrate habitats.
These results suggest the importance of high resolution environmental datasets that allow to study the link between environment and habitat. Habitat maps with high resolution data, thus predicting specific habitat distributions, could feed into marine spatial planning plans.
Advantages and Limitations of a Habitat Classification System
The use of a classification system is useful for habitat mapping and therefore interesting for conservation initiatives such as OSPAR (Oslo-Paris Convention) and ICES (the International Council for the Exploration of the Sea). A classification system permits the use of a standardized habitat description over large areas. The CoralFISH classification system was developed to deliver standardized terminology across the CoralFISH regions, especially for marine management purposes (Davies et al., in press). It encompasses both species and their environment, a scale suggested to be most accurate and ecological relevant for spatial planning and conservation (Costello, 2009). Using a classification system to analyze images is less time-consuming (at least four times) than a detailed analysis to the species level. Besides, the risk of misidentification is lower at a habitat level than at species level. However, a classification system also has limitations. Firstly, the diversity associated with habitats, i.e., here 62 morphotypes, is masked by the use of only the structuring species. Second, a classification system can skew conservation efforts by assigning a higher weight to similar habitats. For example, in the present case, five hard substrate/biogenic habitats and one soft substrate habitat with similar compositions could be considered as one management unit, whereas the four soft substrate habitats with different species compositions should be considered as four different units. Third, several international organizations favor their preservation of certain habitats, including corals and sponges, but have different definitions of a habitat that cannot be all captured in one classification. The CoralFISH classification is close to the classification of FAO Vulnerable Marine Ecosystems (ICES) but is difficult to adapt to the definitions of “reefs” used by the EC Habitats Directive that considers all hard substrate, whether colonized or not (European Commission, 2013). Fourth, the habitats are a priori assumptions or hypotheses about the association of biology and physiography and therefore cannot be analyzed a posteriori to test the reliability of these hypotheses, i.e., the link between biology and geology. And finally, the habitat scale focuses on an aggregation of structuring individuals on a certain surface unit, but exclude the isolated occurrences or aggregations smaller than this unit, thus, it does not reflect the realized distribution of coral species.
Classification systems are, therefore, useful for conservation because they provide information about habitat distribution rapidly compared to analyses at the species level, yet they limit the ability to understand the biology and ecology of species.
Conservation
Threats and Canyons as Refuges for CWC Habitats
The submarine canyons in the Bay of Biscay host a large range of coral habitats, making them an important target for marine management and conservation. Coral habitats are threatened by human activities of which litter and fisheries are the main impacts in the Bay of Biscay.
Litter, including lost fishing gear, is largely present in the canyons of the Bay of Biscay (Galgani et al., 1995; van den Beld et al., in press). Corals, boulders and other features forming relief on the seafloor are important structures that can trap litter (e.g., Galgani et al., 2000) as shown by the presence of more litter items in areas with a seafloor relief formed by geological or biological features than without a relief (Watters et al., 2010; Bergmann and Klages, 2012; Schlining et al., 2013; van den Beld et al., in press).
Bottom trawling is probably one of the largest anthropogenic threats to CWCs (Fosså et al., 2002; Hall-Spencer et al., 2002; Benn et al., 2010) and this fishing activity is increasing on the rims of canyons (Martín et al., 2014b). Trawl gear can damage CWC communities by reducing or changing coral abundances, diversity and community or the removal of structuring species (reviewed by Clark et al., 2016), as shown for coral reefs of the NE Atlantic (Hall-Spencer et al., 2002) and Solenosmilia thickets on Australian seamounts (Althaus et al., 2009). In addition to damages caused by physical contact with trawl gear, trawling can have an indirect impact on CWCs. It causes extensive sediment resuspension in the water column that is transported further down the canyon and it changes the seafloor relief by smoothening canyon flanks through repeatedly scraping off the seafloor resulting in homogeneous slopes and low rugosity (Puig et al., 2012; Martín et al., 2014a). These effects can also have an impact on CWCs and associated community.
Canyons are less accessible for trawling gears than the interfluves and upper slope due to their steep and complex topography and, therefore, may function as a natural refuge for CWCs (Huvenne et al., 2011; Fernandez-Arcaya et al., 2017). The results of this present study support this hypothesis. Most (live) habitats, including coral reef, occurred more frequently in the canyons than on the interfluves or upper slope. A consistent exception was coral rubble that was more frequent on the interfluves and upper slope than in the canyon. Furthermore, live coral habitats are more frequently observed on steeper areas, hardly accessible to trawling, while coral rubble is more often observed on flatter areas. Similarly to the last result, the highest number of corals in Whittard Canyon has been observed on areas with steep slopes (Morris et al., 2013).
Impact by fisheries may have an influence on the fields of rubble on shallow areas, such as the upper slope, and the presence of live corals in canyons. Previous evidence from the 1920s show that fishermen trawling around and on the continental slope of the Bay of Biscay, had caught L. pertusa and M. oculata in their nets (Joubin, 1922).
Natural causes also influence the distribution of coral species and habitats. Live corals, such as scleractinians, may prefer steep topography over flatter areas, which could be related to for example accelerated currents. Furthermore, environmental changes over time, such as sea temperature elevation since the Last Glacial Maximum, can cause the death and breakdown of CWCs. In Guilvinec and Penmarc'h Canyons, age measurements suggested that the dead scleractinian corals, occurring in shallower waters (200–300 m), are older (~7–8 ka) than the live corals deeper (600–700 m) in the canyon (~1–2 ka) (De Mol et al., 2011). The authors suggested that both trawling and natural events could cause these dead corals.
Besides coral rubble, the seapen habitat is also observed in shallow waters (from 234 m water depth), on areas with a slope value less than 20° and is more common on interfluves than in the canyon (100 m res.). Some seapen species can retract completely within the sediment, as has been observed for Protoptilum carpenteri (Packer et al., 2007; Baker et al., 2012) and K. stelliferum, Pennatula phosphorea, and Virgularia mirabilis (De Clippele et al., 2015). This may suggest that some seapen species are resilient to trawling compared to other corals. It may be also possible that seapens (re)colonize an area more rapidly than colonial scleractinians. However, it may also be possible that trawling does not take place as shallow as certain seapens, as it is suggested for Funiculina quadrangularis (not able to withdraw in the sediment) occurring at 240 m water depth in Mediterranean canyons (Fabri et al., 2014).
In summary, our results support the hypothesis that canyons may function as natural refuges for coral habitats. Trawling may cause the observed distribution of live habitats favoring steep slopes, but the influence of natural causes cannot be excluded. Seapens exhibit specific features that may make them more resilient to trawling and may explain their similar distribution to coral rubble, compared to other coral habitats.
Conservation in the French Bay of Biscay
Submarine canyons are “hotspots” for coral habitats and could serve as natural refuges for certain coral habitats. However, conservation measures are rare in the Bay of Biscay. Until the present day, there are two measures on the Aquitaine margin. A fishing restricted area is in place around Capbreton Canyon since 1985, prohibiting certain types of fishing around this canyon (Sanchez et al., 2013). Under the EC Birds Directive, a Special Protection Area (SPA) has been designated at the head of Cap-Ferret Canyon, but does not include management measures of relevance for benthic habitats.
This study fed into a proposal to define sectors for a network of Natura 2000 sites to protect reef habitats under the Habitats Directive (MNHN-SPN and GIS-Posidonie, 2014). The designation of a Natura 2000 network comprising submarine canyons is a step forward in the protection of deep-sea habitats in the French Atlantic.
The Natura 2000 management measures will, however, not apply to soft sediment coral habitats, because this type of habitat does not fall under the Habitats Directives. Although the diversity was low, the (possible) unique species compositions may make them potential candidates for protection. The OSPAR commission does have “Seapen and burrowing megafauna communities” and “Coral gardens” listed as threatened and/or declining habitats (OSPAR, 2008) recognizing its potential vulnerability to anthropogenic impact, but most of the seapen communities remain unprotected by any form of legislation. Seapens can have an important value for humans, supported by the presence of burrows made by e.g., the commercially important crustacean Nephrops norvegicus (langoustine) (OSPAR, 2010) and associations of fish larvae, e.g., Sebastes spp., with seapens (Baillon et al., 2012).
Conclusions
This study included 24 canyons and the results reported here, thus, largely increases the understanding of the distribution of CWC habitats in submarine canyons of the Bay of Biscay. A general regional pattern can be suggested, with a dominance of soft substrate coral habitats and an absence of live scleractinian habitats in the south. Results support the importance of the substrate type on the habitat distribution at different spatial scales, with coral assemblages mostly differentiated in hard/biogenic vs. soft substrate coral communities. The latter one harbors a lower coral diversity and distinct coral compositions between habitats. The overlap of environmental conditions associated with distinct coral habitats can be due to the resolution of the habitats and environmental factors. However, it may also be possible that some discriminating differences exist that would be caused by features that could not be included in this study, e.g., current speed and exposition to current. The geomorphological classes may also provide a good indication of the kind of environment favoring coral habitat development, if data are available with a high enough resolution. Provided this link would be better understood, such classes would help inform management plans, with a less detailed and time-consuming image collection and analysis required than a species level analysis. This study may also open doors for potential management for soft substrate coral habitats, each of which appeared to be structured by a different morphotype.
Author Contributions
Collection of the biological data: IV, BG, JD, SA, and JB. Design of image analysis protocol: IV, JD, BG, and LM. Image analysis: IV. Statistical analysis: IV and LM. Collection/analysis/interpretation of bathymetry and geomorphological classes: JB and LC. Wrote the paper: IV, LM, and KO. Critically reviewed the paper: JB, SA, LC, JD, and BG. Chief-scientists of cruises: SA and JB.
Funding
IV was funded by Ifremer and Région Bretagne. The cruises BobGeo, BobGeo 2 and BobEco were part of the FP7 EU project CoralFISH (grant agreement no. 213144) and supported by the French Oceanographic Fleet.
Conflict of Interest Statement
The authors declare that the research was conducted in the absence of any commercial or financial relationships that could be construed as a potential conflict of interest.
Acknowledgments
We would like to thank captains, crew, chief-scientists and scientific teams during the BobGeo, BobGeo 2, BobEco, and Evhoe cruises (2009-2012). We would like to thank Jean-Pierre Brulport (Ifremer) for collecting the imagery data on the Evhoe cruises. Christophe Bayle and Julie Tourolle (Ifremer) are thanked because of their help relating ArcGIS and the cleaning of the navigation. We thank Paul Gatti (Ifremer) for the r-script used for calculating the water density using the temperature and salinity data. Last, but not least, we thank the experts on coral taxonomy for their (confirmations of) identifications of the different coral groups. Dr. Andreia Braga-Henriques (University of Azores, Portugal) and Dr. Tina Molodtsova (P. P. Shirshov Institute of Oceanology, Russia) came to Ifremer to identify gorgonians and antipatharians/Alcyoniina, respectively. Dr. Stephen Cairns (Smithsonian Institution, Washington DC, USA; scleractinians), Dr. Less Watling (University of Hawaii, HI, USA; gorgonians), Dr. Gary Williams (California Academy of Sciences, CA, USA; pennatulids), Dr. Dennis Opresko (Smithsonian Institution, Washington DC, USA; antipatharians), and Dr. Tina Molodtsova (P. P. Shirshov Institute of Oceanology, Russia; antipatharians) confirmed identifications or identified specimens during the Coral identification workshop held prior to the 5th International Symposium on Deep-Sea Corals in Amsterdam, the Netherlands. We also would like to thank the two reviewers for their remarks, comments and suggestions, that helped us to improve this manuscript. Most of the work was done in the FP7 EU project CoralFISH (grant agreement no. 213144) with input in the last phase of writing from the H2020 EU project ATLAS (grant agreement no. 678760).
Supplementary Material
The Supplementary Material for this article can be found online at: http://journal.frontiersin.org/article/10.3389/fmars.2017.00118/full#supplementary-material
References
Althaus, F., Williams, A., Schlacher, T. A., Kloser, R. J., Green, M. A., Barker, B. A., et al. (2009). Impacts of bottom trawling on deep-coral ecosystems of seamounts are long-lasting. Mar. Ecol. Prog. Ser. 397, 279–294. doi: 10.3354/meps08248
Amaro, T., de Stigter, H., Lavaleye, M., and Duineveld, G. (2015). Organic matter enrichment in the Whittard Channel; its origin and possible effects on benthic megafauna. Deep Sea Res. I Oceanogr. Res. Pap. 102, 90–100. doi: 10.1016/j.dsr.2015.04.014
Amaro, T., Huvenne, V. A. I., Allcock, A. L., Aslam, T., Davies, J. S., Danovaro, R., et al. (2016). The Whittard Canyon - A case study of submarine canyon processes. Prog. Oceanogr. 146, 38–57. doi: 10.1016/j.pocean.2016.06.003
Arnaud-Haond, S., Van den Beld, I., Becheler, R., Orejas, C., Menot, L., Frank, N., et al. (in press). Two “pillars” of cold-water coral reefs along Atlantic European margins: prevalent association of Madrepora oculata with Lophelia pertusa, from reef to colony scale. Deep Sea Res. II Top. Stud. Oceanogr. doi: 10.1016/j.dsr2.2015.07.013
Baillon, S., Hamel, J.-F., Wareham, V. E., and Mercier, A. (2012). Deep cold-water corals as nurseries for fish larvae. Front. Ecol. Environ. 10, 351–356. doi: 10.1890/120022
Baker, K. D., Wareham, V. E., Snelgrove, P. V. R., Haedrich, R. L., Fifield, D. A., Edinger, E. N., et al. (2012). Distributional patterns of deep-sea coral assemblages in three submarine canyons off Newfoundland, Canada. Mar. Ecol. Prog. Ser. 445, 235–249. doi: 10.3354/meps09448
Benn, A. R., Weaver, P. P., Billet, D. S. M., van den Hove, S., Murdock, A. P., Doneghan, G. B., et al. (2010). Human activities on the deep seafloor in the North East Atlantic: an assessment of spatial extent. PLoS ONE 5:e12730. doi: 10.1371/journal.pone.0012730
Bergmann, M., and Klages, M. (2012). Increase of litter at the Arctic deep-sea observatory HAUSGARTEN. Mar. Pollut. Bull. 64, 2734–2741. doi: 10.1016/j.marpolbul.2012.09.018
Bourillet, J.-F. C., Augris, C., Cirac, P., Mazé, J.-P., Normand, A., Loubrieu, B., et al. (2007). Le canyon de Capbreton. Cartes Morpho-Bathymétriques, ed Quae collection Atlas et cartes, 3 Edn. Brest: Ifremer; Université Bordeaux 1 (Coed.).
Bourillet, J. F., de Chambure, L., and Loubrieu, B. (2012). Sur les traces des coraux d'eau froide du golfe de Gascogne: 8 cartes bathymorphologiques et géomorphologiques au 1/100.000/Cold Water Corals of the Bay of Biscay: 8 Bathymorphological and Geomorphological Maps at 1:100,000. Paris: Editions Quae.
Bourillet, J.-F., and Lericolais, G. (2003). “Morphology and seismic stratigraphy of the Manche paleoriver system, Western Approaches,” in European Margin Sediment Dynamics, eds J. Mienert and P. Weaver (Berlin; Heidelberg: Springer), 229–232.
Bourillet, J. F., Reynaud, J. Y., Baltzer, A., and Zaragosi, S. (2003). The “Fleuve Manche”: the submarine sedimentary features from the outer shelf to the deep-sea fans. J. Quarter. Sci. 18, 261–282. doi: 10.1002/jqs.757
Bourillet, J.-F., Schmitt, T., Savini, A., Guillaumont, B., and Arnaud-Haond, S. (2010). “Geomorphological characterisation of cold-water corals habitats (Bay of Biscay, NE Atlantic),” in Geohab 2010, 4-7 May, ed G. Lamarche (Niwa), 52.
Bourillet, J. F., Zaragosi, S., and Mulder, T. (2006). The French Atlantic margin and deep-sea submarine systems. Geo-Mar. Lett. 26, 311–315. doi: 10.1007/s00367-006-0042-2
Buhl-Mortensen, L., Vanreusel, A., Gooday, A. J., Levin, L. A., Priede, I. G., Buhl-Mortensen, P., et al. (2010). Biological structures as a source of habitat heterogeneity and biodiversity on the deep ocean margins. Mar. Ecol. 31, 21–50. doi: 10.1111/j.1439-0485.2010.00359.x
Cairns, S. D. (2007). Deep-water corals: an overview with special reference to diversity and distribution of deep-water scleractinian corals. Bull. Mar. Sci. 81, 311–322.
Carlier, A., Le Guilloux, E., Olu, K., Sarrazin, J., Mastrototaro, F., Taviani, M., et al. (2009). Trophic relationships in a deep Mediterranean cold-water coral bank (Santa Maria di Leuca, Ionian Sea). Mar. Ecol. Prog. Ser. 397, 125–137. doi: 10.3354/meps08361
Cirac, P., Bourillet, J.-F., Griboulard, R., Normand, A., Mulder, T., and Itsas, L. É. (2001). Le canyon de Capbreton: nouvelles approches morphostructurales et morphosédimentaires. Premiers résultats de la campagne Itsas. Comptes Rendus Acad. Sci. IIA Earth Planet. Sci. 332, 447–455. doi: 10.1016/S1251-8050(01)01557-9
Clark, M. R., Althaus, F., Schlacher, T. A., Williams, A., Bowden, D. A., and Rowden, A. A. (2016). The impacts of deep-sea fisheries on benthic communities: a review. ICES J. Mar. Sci. 73, 51–69. doi: 10.1093/icesjms/fsv123
Costello, M. J. (2009). Distinguishing marine habitat classification concepts for ecological data management. Mar. Ecol. Prog. Ser. 397, 253–268. doi: 10.3354/meps08317
Cunha, M. R., Paterson, G. L. J., Amaro, T., Blackbird, S., de Stigter, H. C., Ferreira, C., et al. (2011). Biodiversity of macrofaunal assemblages from three Portuguese submarine canyons (NE Atlantic). Deep Sea Res. II Top. Stud. Oceanogr. 58, 2433–2447. doi: 10.1016/j.dsr2.2011.04.007
Davies, A. J., Duineveld, G. C. A., Lavaleye, M. S. S., Bergman, M. J. N., van Haren, H., and Roberts, J. M. (2009). Downwelling and deep-water bottom currents as food supply mechanisms to the cold-water coral Lophelia pertusa (Scleractinia) at the Mingulay Reef Complex. Limnol. Oceanogr. 54, 620–629. doi: 10.4319/lo.2009.54.2.0620
Davies, A. J., and Guinotte, J. M. (2011). Global habitat suitability for framework-forming cold-water corals. PLoS ONE 6:e18483. doi: 10.1371/journal.pone.0018483
Davies, A. J., Wisshak, M., Orr, J. C., and Roberts, J. M. (2008). Predicting suitable habitat for the cold-water coral Lophelia pertusa (Scleractinia). Deep Sea Res. I Oceanogr. Res. Pap. 55, 1048–1062. doi: 10.1016/j.dsr.2008.04.010
Davies, J. S., Guillaumont, B., Tempera, F., Vertino, A., Beuck, L., Ólafsdóttir, S. H., et al. (in press). A new classification scheme of European cold-water coral habitats: implications for ecosystem-based management of the deep sea. Deep Sea Res. II Top. Stud. Oceanogr. doi: 10.1016/j.dsr2.2017.04.014
Davies, J. S., Howell, K. L., Stewart, H. A., Guinan, J., and Golding, N. (2014). Defining biological assemblages (biotopes) of conservation interest in the submarine canyons of the South West Approaches (offshore United Kingdom) for use in marine habitat mapping. Deep Sea Res. II Top. Stud. Oceanogr. 104, 208–229. doi: 10.1016/j.dsr2.2014.02.001
De Chambure, L., Bourillet, J.-F., and Bartel, C. (2013). Geomorphological Classification in Bay of Biscay. Morpho-Sedimentary Mapping of the Seabed in Selected Areas. France: Ifremer.
De Clippele, L. H., Buhl-Mortensen, P., and Buhl-Mortensen, L. (2015). Fauna associated with cold water gorgonians and sea pens. Cont. Shelf Res. 105, 67–78. doi: 10.1016/j.csr.2015.06.007
De Leo, F. C., Smith, C. R., Rowden, A. A., Bowden, D. A., and Clark, M. R. (2010). Submarine canyons: hotspots of benthic biomass and productivity in the deep sea. Proc. R. Soc. B Biol. Sci. 277, 2783–2792. doi: 10.1098/rspb.2010.0462
de Madron, X. D., Castaing, P., Nyffeler, F., and Courp, T. (1999). Slope transport of suspended particulate matter on the Aquitanian margin of the Bay of Biscay. Deep Sea Res. II Top. Stud. Oceanogr. 46, 2003–2027. doi: 10.1016/S0967-0645(99)00053-3
De Mol, L., Van Rooij, D., Pirlet, H., Greinert, J., Frank, N., Quemmerais, F., et al. (2011). Cold-water coral habitats in the Penmarc'h and Guilvinec Canyons (Bay of Biscay): deep-water versus shallow-water settings. Mar. Geol. 282, 40–52. doi: 10.1016/j.margeo.2010.04.011
de Stigter, H. C., Boer, W., de Jesus Mendes, P. A., Jesus, C. C., Thomsen, L., van den Bergh, G. D., et al. (2007). Recent sediment transport and deposition in the Nazaré Canyon, Portuguese continental margin. Mar. Geol. 246, 144–164. doi: 10.1016/j.margeo.2007.04.011
Doledec, S., and Chessel, D. (1987). Rythmes saisonniers et composantes stationnelles en milieu aquatique I - description d'un plan d'observations complet par projection de variables. Acta Oecol. Oecol. Gen. 8, 403–426.
Dray, S., and Dufour, A. B. (2007). The ade4 package: implementing the duality diagram for ecologists. J. Stat. Softw. 22, 1–20. doi: 10.18637/jss.v022.i04
Dullo, W. C., Flogel, S., and Ruggeberg, A. (2008). Cold-water coral growth in relation to the hydrography of the Celtic and Nordic European continental margin. Mar. Ecol. Prog. Ser. 371, 165–176. doi: 10.3354/meps07623
European Commission, D. E. (2013). Interpretation Manual of European Union Habitats - EUR28. Brussels.
Fabri, M. C., Pedel, L., Beuck, L., Galgani, F., Hebbeln, D., and Freiwald, A. (2014). Megafauna of vulnerable marine ecosystems in French Mediterranean submarine canyons: spatial distribution and anthropogenic impacts. Deep Sea Res. II Top. Stud. Oceanogr. 104, 184–207. doi: 10.1016/j.dsr2.2013.06.016
Fernandez-Arcaya, U., Ramirez-Llodra, E., Aguzzi, J., Allcock, A. L., Davies, J. S., Dissanayake, A., et al. (2017). Ecological role of submarine canyons and need for canyon conservation: a review. Front. Mar. Sci. 4:5. doi: 10.3389/fmars.2017.00005
Flogel, S., Dullo, W. C., Pfannkuche, O., Kiriakoulakis, K., and Ruggeberg, A. (2014). Geochemical and physical constraints for the occurrence of living cold-water corals. Deep Sea Res. II Top. Stud. Oceanogr. 99, 19–26. doi: 10.1016/j.dsr2.2013.06.006
Fosså, J. H., Mortensen, P. B., and Furevik, D. M. (2002). The deep-water coral Lophelia pertusa in Norwegian waters: distribution and fishery impacts. Hydrobiologia 471, 1–12. doi: 10.1023/A:1016504430684
Freiwald, A., Fosså, J. H., Grehan, A., Koslow, J. A., and Roberts, J. M. (2004). Cold-Water Coral Reefs. Cambridge: UNEP-WCMC.
Galgani, F., Burgeot, T., Bocquene, G., Vincent, F., Leaute, J. P., Labastie, J., et al. (1995). Distribution and abundance of debris on the continental shelf of the Bay of Biscay and in Seine Bay. Mar. Pollut. Bull. 30, 58–62. doi: 10.1016/0025-326X(94)00101-E
Galgani, F., Leaute, J. P., Moguedet, P., Souplet, A., Verin, Y., Carpentier, A., et al. (2000). Litter on the sea floor along European coasts. Mar. Pollut. Bull. 40, 516–527. doi: 10.1016/S0025-326X(99)00234-9
Gomes-Pereira, J. N., Auger, V., Beisiegel, K., Benjamin, R., Bergmann, M., Bowden, D., et al. (2016). Current and future trends in marine image annotation software. Prog. Oceanogr. 149, 106–120. doi: 10.1016/j.pocean.2016.07.005
Hall-Spencer, J., Allain, V., and Foss,å, J. H. (2002). Trawling damage to Northeast Atlantic ancient coral reefs. Proc. R. Soc. B Biol. Sci. 269, 507–511. doi: 10.1098/rspb.2001.1910
Harris, P. T., and Whiteway, T. (2011). Global distribution of large submarine canyons: geomorphic differences between active and passive continental margins. Mar. Geol. 285, 69–86. doi: 10.1016/j.margeo.2011.05.008
Hebbeln, D., Wienberg, C., Wintersteller, P., Freiwald, A., Becker, M., Beuck, L., et al. (2014). Environmental forcing of the Campeche cold-water coral province, southern Gulf of Mexico. Biogeosciences 11, 1799–1815. doi: 10.5194/bg-11-1799-2014
Howell, K. L., and Davies, J. S. (2010). Deep-Sea Species Image Catalogue. Plymouth: Marine Biology and Ecology Research Centre, Marine Institute at the University of Plymouth.
Howell, K. L., Holt, R., Endrino, I. P., and Stewart, H. (2011). When the species is also a habitat: comparing the predictively modelled distributions of Lophelia pertusa and the reef habitat it forms. Biol. Conserv. 144, 2656–2665. doi: 10.1016/j.biocon.2011.07.025
Hurlbert, S. H. (1971). The nonconcept of species diversity: a critique and alternative parameters. Ecology 52, 577–586. doi: 10.2307/1934145
Huthnance, J. M. (1995). Circulation, exchange and water masses at the ocean margin: the role of physical processes at the shelf edge. Prog. Oceanogr. 35, 353–431. doi: 10.1016/0079-6611(95)80003-C
Huvenne, V. A. I., Bailey, W. R., Shannon, P. M., Naeth, J., di Primio, R., Henriet, J. P., et al. (2007). The Magellan mound province in the Porcupine Basin. Int. J. Earth Sci. 96, 85–101. doi: 10.1007/s00531-005-0494-z
Huvenne, V. A. I., Beyer, A., de Haas, H., Dekindt, K., Henriet, J.-P., Kozachenko, M., et al. (2005). “The seabed appearance of different coral bank provinces in the Porcupine Seabight, NE Atlantic: results from sidescan sonar and ROV seabed mapping,” in Cold-Water Corals and Ecosystems, eds A. Freiwald and J. M. Roberts (Berlin; Heidelberg: Springer), 535–569.
Huvenne, V. A. I., Tyler, P. A., Masson, D. G., Fisher, E. H., Hauton, C., Huhnerbach, V., et al. (2011). A picture on the wall: innovative mapping reveals cold-water coral refuge in submarine canyon. PLoS ONE 6:e28755. doi: 10.1371/journal.pone.0028755
Joubin, M. L. (1922). Les Coraux de mer Profonde Nuisibles aux Chalutiers. Paris: Ed Blondel La Rougery.
Khripounoff, A., Caprais, J.-C., Le Bruchec, J., Rodier, P., Noel, P., and Cathalot, C. (2014). Deep cold-water coral ecosystems in the Brittany submarine canyons (Northeast Atlantic): hydrodynamics, particle supply, respiration, and carbon cycling. Limnol. Oceanogr. 59, 87–98. doi: 10.4319/lo.2014.59.1.0087
Koutsikopoulos, C., and Le Cann, B. (1996). Physical processes and hydrological structures related to the Bay of Biscay anchovy. Sci. Mar. 60, 9–19.
Le Danois, E. (1948). Les Profondeurs de la mer, Trente ans de Recherches sur la Faune Sous-Marine au Large des Côtes de France. Paris: Payot.
Legendre, P., and Gallagher, E. D. (2001). Ecologically meaningful transformations for ordination of species data. Oecologia 129, 271–280. doi: 10.1007/s004420100716
Madden, C., Goodin, K., Allee, B., Finkbeiner, M., and Bamford, D. (2008). Coastal and Marine Ecological Classification Standard. Arlington, TX: NOAA and NatureServe.
Martín, J., Puig, P., Masque, P., Palanques, A., and Sanchez-Gomez, A. (2014a). Impact of bottom trawling on deep-sea sediment properties along the flanks of a submarine canyon. PLoS ONE 9:e104536. doi: 10.1371/journal.pone.0104536
Martín, J., Puig, P., Palanques, A., and Giamportone, A. (2014b). Commercial bottom trawling as a driver of sediment dynamics and deep seascape evolution in the Anthropocene. Anthropocene 7, 1–15. doi: 10.1016/j.ancene.2015.01.002
Mienis, F., Duineveld, G. C. A., Davies, A. J., Ross, S. W., Seim, H., Bane, J., et al. (2012). The influence of near-bed hydrodynamic conditions on cold-water corals in the Viosca Knoll area, Gulf of Mexico. Deep Sea Res. I Oceanogr. Res. Pap. 60, 32–45. doi: 10.1016/j.dsr.2011.10.007
Miller, R. J., Hocevar, J., Stone, R. P., and Fedorov, D. V. (2012). Structure-forming corals and sponges and their use as fish habitat in Bering Sea submarine canyons. PLoS ONE 7:e33885. doi: 10.1371/journal.pone.0033885
Mohn, C., Rengstorf, A., White, M., Duineveld, G., Mienis, F., Soetaert, K., et al. (2014). Linking benthic hydrodynamics and cold-water coral occurrences: a high-resolution model study at three cold-water coral provinces in the NE Atlantic. Prog. Oceanogr. 122, 92–104. doi: 10.1016/j.pocean.2013.12.003
Morris, K. J., Tyler, P. A., Masson, D. G., Huvenne, V. I. A., and Rogers, A. D. (2013). Distribution of cold-water corals in the Whittard Canyon, NE Atlantic Ocean. Deep Sea Res. II Top. Stud. Oceanogr. 92, 136–144. doi: 10.1016/j.dsr2.2013.03.036
Mortensen, P. B., and Buhl-Mortensen, L. (2005). “Deep-water corals and their habitats in The Gully, a submarine canyon off Atlantic Canada,” in Cold-Water Corals and Ecosystems, eds A. Freiwald and J. M. Roberts (Berlin; Heidelberg: Springer), 247–277.
Mortensen, P. B., Hovland, M. T., Fosså, J. H., and Furevik, D. M. (2001). Distribution, abundance and size of Lophelia pertusa coral reefs in mid-Norway in relation to seabed characteristics. J. Mar. Biol. Assoc. UK 81, 581–597. doi: 10.1017/S002531540100426X
Mulder, T., Zaragosi, S., Garlan, T., Mavel, J., Cremer, M., Sottolichio, A., et al. (2012). Present deep-submarine canyons activity in the Bay of Biscay (NE Atlantic). Mar. Geol. 295, 113–127. doi: 10.1016/j.margeo.2011.12.005
Oksanen, J., Blanchet, F. G., Kindt, R., Legendre, P., Minchin, P. R., O'Hara, R. B., et al. (2016). Vegan: Community Ecology Package. R package version 2.3-5. Availabe online at: https://CRAN.R-project.org/package=vegan
Orejas, C., Gori, A., Lo Iacono, C., Puig, P., Gili, J.-M., and Dale, M. R. T. (2009). Cold-water corals in the Cap de Creus Canyon, northwestern Mediterranean: spatial distribution, density and anthropogenic impact. Mar. Ecol. Prog. Ser. 397, 37–51. doi: 10.3354/meps08314
OSPAR (2008). OSPAR List of Threatened and/or Declining Species and Habitats. Agreement 2008-6. London.
OSPAR (2010). Background Document for Seapen and Burrowing Megafauna Communities, ed A. Curd. London: OSPAR Commission.
Packer, D. B., Boelke, D., Guida, V., and McGee, L. A. (2007). “State of deep coral ecosystems in the northeastern US region: Maine to Cape Hatteras,” in The State of Deep Coral Ecosystems Of the United States, eds S. E. Lumsden, T. F. Hourigan, A. W. Bruckner, and G. Dorr (Silver Spring, MD: NOAA), 195–232.
Pichon, A., Morel, Y., Baraille, R., and Quaresma, L. S. (2013). Internal tide interactions in the Bay of Biscay: observations and modelling. J. Mar. Syst. 109, S26–S44. doi: 10.1016/j.jmarsys.2011.07.003
Pingree, R. D., and Le Cann, B. (1989). Celtic and Armorican slope and shelf residual currents. Prog. Oceanogr. 23, 303–338. doi: 10.1016/0079-6611(89)90003-7
Pingree, R. D., and Le Cann, B. (1992). Three anticyclonic slope water oceanic eDDIES (SWODDIES) in the southern Bay of Biscay in 1990. Deep Sea Res. I Oceanogr. Res. Pap. 39, 1147–1175. doi: 10.1016/0198-0149(92)90062-X
Pingree, R. D., and Mardell, G. T. (1985). Solitary internal waves in the Celtic Sea. Prog. Oceanogr. 14, 431–441. doi: 10.1016/0079-6611(85)90021-7
Puig, P., Canals, M., Company, J. B., Martín, J., Amblas, D., Lastras, G., et al. (2012). Ploughing the deep sea floor. Nature 489, 286–290. doi: 10.1038/nature11410
Ramirez-Llodra, E., Tyler, P. A., Baker, M. C., Bergstad, O. A., Clark, M. R., Escobar, E., et al. (2011). Man and the last great wilderness: human impact on the deep sea. PLoS ONE 6:e22588. doi: 10.1371/journal.pone.0022588
Rengstorf, A. M., Grehan, A., Yesson, C., and Brown, C. (2012). Towards high-resolution habitat suitability modeling of vulnerable marine ecosystems in the deep-sea: resolving terrain attribute dependencies. Mar. Geodesy 35, 343–361. doi: 10.1080/01490419.2012.699020
Reveillaud, J., Freiwald, A., Van Rooij, D., Le Guilloux, E., Altuna, A., Foubert, A., et al. (2008). The distribution of scleractinian corals in the Bay of Biscay, NE Atlantic. Facies 54, 317–331. doi: 10.1007/s10347-008-0138-4
Robert, K., Jones, D. O. B., Tyler, P. A., Van Rooij, D., and Huvenne, V. A. I. (2015). Finding the hotspots within a biodiversity hotspot: fine-scale biological predictions within a submarine canyon using high-resolution acoustic mapping techniques. Mar. Ecol. 36, 1256–1276. doi: 10.1111/maec.12228
Roberts, J. M., Wheeler, A., Freiwald, A., and Cairns, S. (2009). Cold-Water Corals. The Biology and Geology of Deep-Sea Coral Habitats. New York, NY: Cambridge University Press.
Roberts, J. M., Wheeler, A. J., and Freiwald, A. (2006). Reefs of the deep: the biology and geology of cold-water coral ecosystems. Science 312, 543–547. doi: 10.1126/science.1119861
Roule, L. (1896). “Coelentérés,” in Résultats Scientifiques de la Campagne du “Caudan” Dans le Golfe de Gascogne: août-septembre 1895, ed R. Kœhler (Lyon: Annales de l'Université de Lyon), 299–323.
Sanchez, F., Gonzalez-Pola, C., Druet, M., Garcia-Alegre, A., Acosta, J., Cristobo, J., et al. (2014). Habitat characterization of deep-water coral reefs in La Gaviera Canyon (Aviles Canyon System, Cantabrian Sea). Deep Sea Res. II Top. Stud. Oceanogr. 106, 118–140. doi: 10.1016/j.dsr2.2013.12.014
Sanchez, F., Morandeau, G., Bru, N., and Lissardy, M. (2013). A restricted fishing area as a tool for fisheries management: example of the Capbreton Canyon, southern Bay of Biscay. Mar. Policy 42, 180–189. doi: 10.1016/j.marpol.2013.02.009
Schlining, K., von Thun, S., Kuhnz, L., Schlining, B., Lundsten, L., Stout, N. J., et al. (2013). Debris in the deep: using a 22-year video annotation database to survey marine litter in Monterey Canyon, central California, USA. Deep Sea Res. I Oceanogr. Res. Pap. 79, 96–105. doi: 10.1016/j.dsr.2013.05.006
Schmidt, S., Howa, H., Diallo, A., Martin, J., Cremer, M., Duros, P., et al. (2014). Recent sediment transport and deposition in the Cap-Ferret Canyon, South-East margin of Bay of Biscay. Deep Sea Res. II Top. Stud. Oceanogr. 104, 134–144. doi: 10.1016/j.dsr2.2013.06.004
Soetaert, K., Mohn, C., Rengstorf, A., Grehan, A., and van Oevelen, D. (2016). Ecosystem engineering creates a direct nutritional link between 600-m deep cold-water coral mounds and surface productivity. Sci. Rep. 6:35057. doi: 10.1038/srep35057
MNHN-SPN and GIS-Posidonie (2014). Méthodologie et Recommandations pour l'Extension du Réseau Natura 2000 Au-delà de la mer Territoriale pour l'Habitat récifs (1170): Région Biogéographique Marine Atlantique. Rapport SPN 2014.
Taviani, M., Angeletti, L., Canese, S., Cannas, R., Cardone, F., Cau, A., et al. (in press). The “Sardinian cold-water coral province” in the context of the Mediterranean coral ecosystems. Deep Sea Res. II Top. Studi. Oceanog. doi: 10.1016/j.dsr2.2015.12.008
van Aken, H. M. (2000a). The hydrography of the mid-latitude Northeast Atlantic Ocean I: the deep water masses. Deep Sea Res. I Oceanogr. Res. Pap. 47, 757–788. doi: 10.1016/S0967-0637(99)00092-8
van Aken, H. M. (2000b). The hydrography of the mid-latitude Northeast Atlantic Ocean II: the intermediate water masses. Deep Sea Res. I Oceanogr. Res. Pap. 47, 789–824. doi: 10.1016/S0967-0637(99)00112-0
van den Beld, I. M. J., Guillaumont, B., Menot, L., Bayle, C., Arnaud-Haond, S., and Bourillet, J.-F. (in press). Marine litter in submarine canyons of the Bay of Biscay. Deep Sea Res. II Top. Stud. Oceanogr. doi: 10.1016/j.dsr2.2016.04.013
Van Rooij, D., De Mol, L., Le Guilloux, E., Wisshak, M., Huvenne, V. A. I., Moeremans, R., et al. (2010). Environmental setting of deep-water oysters in the Bay of Biscay. Deep Sea Res. I Oceanogr. Res. Pap. 57, 1561–1572. doi: 10.1016/j.dsr.2010.09.002
Vetter, E. W., Smith, C. R., and De Leo, F. C. (2010). Hawaiian hotspots: enhanced megafaunal abundance and diversity in submarine canyons on the oceanic islands of Hawaii. Mar. Ecol. 31, 183–199. doi: 10.1111/j.1439-0485.2009.00351.x
Wagner, D., Luck, D. G., and Toonen, R. J. (2012). “Chapter two - the biology and ecology of black corals (Cnidaria: Anthozoa: Hexacorallia: Antipatharia),” in Advances in Marine Biology, Vol. 63, ed M. Lesser (Academic Press), 67–132.
Watters, D. L., Yoklavich, M. M., Love, M. S., and Schroeder, D. M. (2010). Assessing marine debris in deep seafloor habitats off California. Mar. Pollut. Bull. 60, 131–138. doi: 10.1016/j.marpolbul.2009.08.019
Wheeler, A. J., Beyer, A., Freiwald, A., de Haas, H., Huvenne, V. A. I., Kozachenko, M., et al. (2007). Morphology and environment of cold-water coral carbonate mounds on the NW European margin. Int. J. Earth Sci. 96, 37–56. doi: 10.1007/s00531-006-0130-6
Wheeler, A. J., Kozachenko, M., Beyer, A., Foubert, A., Huvenne, V. A. I., Klages, M., et al. (2005). “Sedimentary processes and carbonate mounds in the Belgica Mound province, Porcupine Seabight, NE Atlantic,” in Cold-water Corals and Ecosystems, eds A. Freiwald and J.M. Roberts (Berlin; Heidelberg: Springer), 571–603.
White, M., Mohn, C., de Stigter, H., and Mottram, G. (2005). “Deep-water coral development as a function of hydrodynamics and surface productivity around the submarine banks of the Rockall Trough, NE Atlantic,” in Cold-Water Corals and Ecosystems, eds A. Freiwald and J. M. Roberts (Berlin; Heidelberg: Springer), 503–514.
Wienberg, C., Hebbeln, D., Fink, H. G., Mienis, F., Dorschel, B., Vertino, A., et al. (2009). Scleractinian cold-water corals in the Gulf of Cádiz — first clues about their spatial and temporal distribution. Deep Sea Res. I Oceanogr. Res. Pap. 56, 1873–1893. doi: 10.1016/j.dsr.2009.05.016
Williams, G. C. (1995). Living genera of sea pens (Coelenterata, Octocorallia, Pennatulacea): illustrated key and synopses. Zool. J. Linn. Soc. 113, 93–140. doi: 10.1111/j.1096-3642.1995.tb00929.x
Williams, G. C. (2011). The global diversity of sea pens (Cnidaria: Octocorallia: Pennatulacea). PLoS ONE 6:e22747. doi: 10.1371/journal.pone.0022747
Wright, D. J., Pendleton, M., Boulware, J., Walbridge, S., Gerlt, B., Eslinger, D., et al. (2012). ArcGISBenthic Terrain Modeler (BTM), v. 3.0. Environmental Systems Research Institute, NOAA Coastal Services Center, Massachusetts Office of Coastal Zone Management. Available online at: http://esriurl.com/5754
Yesson, C., Bedford, F., Rogers, A. D., and Taylor, M. L. (in press). The global distribution of deep-water Antipatharia habitat. Deep Sea Res. II Top. Studi. Oceanogr. doi: 10.1016/j.dsr2.2015.12.004
Yesson, C., Taylor, M. L., Tittensor, D. P., Davies, A. J., Guinotte, J., Baco, A., et al. (2012). Global habitat suitability of cold-water octocorals. J. Biogeogr. 39, 1278–1292. doi: 10.1111/j.1365-2699.2011.02681.x
Zaragosi, S., Auffret, G. A., Faugères, J. C., Garlan, T., Pujol, C., and Cortijo, E. (2000). Physiography and recent sediment distribution of the Celtic deep-sea fan, Bay of Biscay. Mar. Geol. 169, 207–237. doi: 10.1016/S0025-3227(00)00054-2
Keywords: cold-water corals, habitats, submarine canyons, Bay of Biscay, NE Atlantic, ROV, towed camera, distribution
Citation: van den Beld IMJ, Bourillet J-F, Arnaud-Haond S, de Chambure L, Davies JS, Guillaumont B, Olu K and Menot L (2017) Cold-Water Coral Habitats in Submarine Canyons of the Bay of Biscay. Front. Mar. Sci. 4:118. doi: 10.3389/fmars.2017.00118
Received: 11 January 2017; Accepted: 12 April 2017;
Published: 16 May 2017.
Edited by:
Ricardo Serrão Santos, University of the Azores, PortugalReviewed by:
Murray Roberts, University of Edinburgh, UKTina Molodtsova, Shirshov Institute of Oceanology, Russia
Copyright © 2017 van den Beld, Bourillet, Arnaud-Haond, de Chambure, Davies, Guillaumont, Olu and Menot. This is an open-access article distributed under the terms of the Creative Commons Attribution License (CC BY). The use, distribution or reproduction in other forums is permitted, provided the original author(s) or licensor are credited and that the original publication in this journal is cited, in accordance with accepted academic practice. No use, distribution or reproduction is permitted which does not comply with these terms.
*Correspondence: Inge M. J. van den Beld, SW5nZS52YW4uZGVuLkJlbGRAaWZyZW1lci5mcg==
†Present Address: Jaime S. Davies, Marine Biology and Ecology Research Centre, Plymouth University, Plymouth, UK