- 1Facultad de Ciencias Químicas, Universidad Autónoma de Chihuahua, Chihuahua, Mexico
- 2Centro de Investigación en Alimentación y Desarrollo A.C., Guaymas, Sonora, Mexico
- 3Departamento de Bioquímica-Alimentos, Facultad de Ciencias Químicas, Benemérita Universidad Autónoma de Puebla, Puebla, Mexico
Chitosan has proved to have antimicrobial properties, which can be used to protect seafood from contamination and growth of pathogens such as Vibrio cholerae. The aim of this study was to model the inhibition of Vibrio cholerae Non-01 by low and high molecular weight chitosan. Chitosan of low (LMWC) and high (HMWC) molecular weight (150 and 320 KDa respectively) was used at final concentrations of 600 and 1,000 mg/L and antimicrobial assays were carried out at temperatures of 5 and 35°C. Data were fitted to the Weibull distribution and b and n values were used to plot resistance frequency curves. Inhibition curves showed that the four treatments were effective at 5°C, with 5.5 log reduction after 312 h with the highest chitosan concentration tested, while the effect of the 600 mg/L chitosan concentration was bacteriostatic rather than bactericidal (3.5 log reduction at 388 h). The 1,000 mg/L chitosan concentration was bactericidal at 35°C (6 log reduction) at a shorter time (12 h). No differences were observed by the different molecular weight of the chitosan used. Weibull model fit adequately (R2 > 0.8908) with experimental data, the highest cellular damage occurs significantly (p < 0.05) with high chitosan concentrations regardless of molecular weight and treatment temperature. Resistance frequency plots showed a greater resistance of LMWC, with the maximum values of V. cholerae Non-01 resistance presented in the first minutes of the treatments regardless of the temperature.
Introduction
Chitosan is a cationic polysaccharide derived from chitin by a deacetylation process; chitin is a natural polymer from the exoskeletons of crustaceans, insects and certain fungi cell walls. The shrimp processing industry generates a significant amount of waste (near 50–70% of shrimp net weight), that can have the potential to be uses as a food additive, as well as a biodegradable and/or active packaging. In addition to the significant decrease in product weight during shrimp processing, the disposal and environmental management of residues, frequently represent elevated economic costs (Bagder-Elmaci et al., 2015; Avila-Sosa et al., 2016; Arancibia et al., 2017).
Chitosan is known to present antimicrobial bactericidal effects, which has been attributed to its positive charges that can interfere with the negatively-charged macromolecules residues on the microbial cell surface, causing membrane leakage (Bagder-Elmaci et al., 2015). Depending on the extraction process and origin, chitosan characteristics such as molecular weight and degree of acetylation can be different, and those properties can affect its antimicrobial characteristics (Fei Liu et al., 2001; Avadi et al., 2004). In seafood products, several studies revealed the effective antibacterial activities of chitosan against a variety of pathogenic bacteria (No et al., 2007; Benhabiles et al., 2012). The antimicrobial characteristics of chitosan can be of potential commercial exploitation, since it is safe for human consumption, and can be used to develop natural preservatives for food processing applications (Xia et al., 2011).
In the last decade, mathematical modeling applied to describe the effect of natural antimicrobials has been used as an important tool to describe growth and survival of microorganisms (Gastélum et al., 2012; Gougouli and Koutsoumanis, 2012). The aim of this study was to use modeling tools to describe the inhibition of Vibrio cholerae Non-01 by low and high molecular weight chitosan at two different temperatures, under laboratory conditions.
Materials and Methods
Bacterial Strain and Growth Conditions
Vibrio cholerae Non-01 was supplied by the Laboratorio Estatal de Salud Pública (Sonora, México). Broth subcultures were prepared by inoculating a test tube containing sterile trypticase soy broth (Bioxon, Mexico) added with 2% of NaCl (TSB 2%). After inoculation, tubes were incubated overnight at 35°C. Using the overnight growth, an inoculum with a final concentration of 1.5 × 108 cells/mL (MacFarland 0.5 standard) was prepared.
Chitosan Characterization and Solution Preparation
A commercial chitosan (Sigma Aldrich, Mexico) of low (LMWC) and high (HMWC) molecular weight was used. Molecular weight of the commercial preparations was determined by viscosity measurement, and deacetylation degree was obtained by potentiometric titration, following the methodology described by Avadi et al. (2004), with modifications proposed by Argüelles et al. (2004). An initial solution of 1% w/v of chitosan was prepared in acetic acid (1% v/v) and sterilized by UV radiation (Labconco Class II, USA). LMWC and HMWC solutions were prepared at concentrations of 600 and 1,000 mg/L.
Antimicrobial Assays
The antimicrobial effect of chitosan on V. cholerae Non-01 was first established by the determination of MIC and MBC values, according to Avadi et al. (2004). Inhibition assays were done using the methodology described by Ciafardini and Zullo (2002), at temperatures of 5 and 35°C. EM flasks (250 mL) containing 50 mL of TBS 2% and chitosan to a final concentration 600 and 1,000 mg/L were inoculated with overnight cultures of V. cholerae Non-01, with an initial concentration of 1 × 108 cells/mL (inoculum adjusted with McFarland standard). A control experiment, using TSB 2% without chitosan and inoculated with V. cholerae Non-01 was also included. Flasks were incubated under agitation (150 rpm) at the corresponding temperatures and 1-mL aliquots were taken for bacterial enumeration, which was done by decimal serial dilutions inoculated by pour plate technique in trypticase soy agar with 2% of NaCl. Plates were incubated at 35°C for 24 h and results are expressed as Log10 CFU/mL. Initial bacterial concentration was determined at the time of inoculation, as a reference for each treatment. Sampling times during the experiments varied, depending on the temperature tested. For each treatment and the control, five replicate experiments were done.
Data Modeling and Statistical Analysis
Antimicrobial assay curves were generated from experimental data by plotting log (N/N0) (where N is the number of CFU/mL at a given time and N0 is the initial number of CFU/mL) vs. time of treatment. Data were fitted to the Weibull distribution (Peleg and Shetty, 1997) from which biological parameters b and n were estimated by nonlinear regression (Kaleida Graph, Version 3.51, Synergy Software, Reading PA, USA):
where b is the inactivation rate, and n is the microorganisms' resistance to the treatments. If n is lower than 1 (concave curves) V. cholerae become more resistant to chitosan treatments having the ability to adapt to applied treatment, and if n is higher than 1 (convex curves), microorganism become more sensitive (increasingly the damaged) to treatments.
Once the values of b and n were obtained, resistance frequency curves were plotted with the following equation (Equation 2):
where tc is a measure of resistance or sensitivity of the organism and ϕ is the fraction of organisms that share at any given time (Peleg and Cole, 1998). The mode of distribution represents the treatment time in which the majority of the population dies or is inactive. The mean corresponds to the average inactivation time with its variation.
Weibull parameters were analyzed using analysis of variance (ANOVA) with Minitab 17 software (Minitab Inc., PA, USA, 2010). A p-value of 0.05 was used for deciding significant differences among averages (Tukey's test).
Results
There are several differences on the classification of chitosan based on its molecular weight and degree of acetylation. In order to prepare water-soluble hydrolysates from chitosan, several studies have reported the enzymatic and/or chemical degradation of chitosan, and identify those products as low molecular weight chitosan (Liu et al., 2015; Gohi et al., 2017). Liu et al. (2015) classified HMWC when MW is above 150 KDa and a minimum DA of 79%. For the present study, we used commercial available chitosan products, and identified them accordingly: LMWC, with a reported molecular weight range of 50-190 KDa and 75-85% deacetylation (Sigma-Aldrich, Cat No. 448869) and HMWC with a reported molecular weight range of 310–375 KDa and >75 deacetylation (Sigma-Aldrich, Cat No. 419419). In order to better characterize the material to be used, the commercial products were evaluated for the determination of molecular weight (MW) and degree of acetylation (DA), and results were in accordance to the ranges described for each chitosan type: LMWC had an average MW of 150 KDa and a DA of 17.1%, while HMWC had a MW of 320 KDa and a DA of 19.0%.
Regarding antimicrobial activity of both chitosan against Vibrio cholerae Non-01, there were differences on the bacteriostatic and bactericidal concentration. For LMWC, the effect was bactericidal, since both MIC and MBC were 700 mg/L, while for HMWC, MIC was 500 mg/L and MBC was 900 mg/L. Based on the results of MIC and MBC determinations, inhibition assays were done using 600 and 1,000 mg/L, to evaluate concentrations that were below or close to MIC and above MBC for both types of chitosan respectively.
Antimicrobial assay for LMWC and HMWC are presented in Figure 1. At 5°C it can be observed that the four treatments show favorable results with respect to antimicrobial effectiveness. V. cholerae Non-01 control curve remained constant for 240 h, and a slight increase in bacterial numbers was observed afterwards. The concentration of 600 mg/L favored the reduction of V. cholerae Non-01 up to 3.5 log cycles after 384 h; however, at that time an increase in the bacterial population was observed with both types of chitosan; however, bacterial concentration did not reach the initial concentration after 576 h of incubation (Figure 1A). No bactericidal effect was observed at 600 mg/L concentration and 35°C for neither type of chitosan (Figure 1B); during the first 12 h there was an decrease in bacterial population (1–2 Log reduction), but the bacterial population increases after this period, reaching a concentration similar or even larger than the control with no chitosan added. On the other hand, LMWC and HMWC at 1,000 mg/L, presented a bactericidal effect at both temperatures, reaching a 5.5 log reduction after 312 h of incubation at 5°C, and a similar reduction in a much shorter period (12 h) at 35°C (Figures 1A,B).
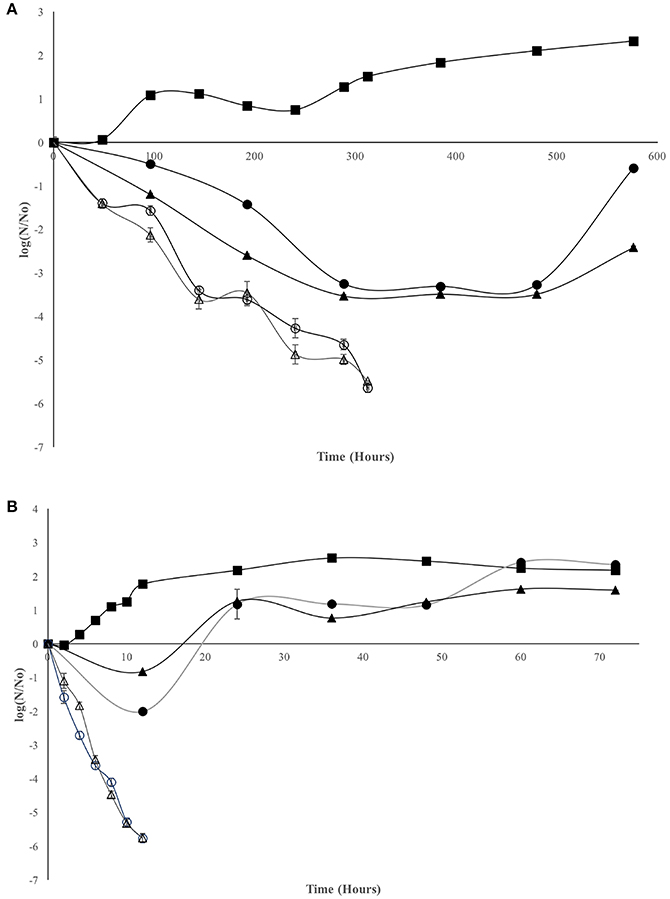
Figure 1. Effect of chitosan on Vibrio cholerae Non-01 at selected concentrations and molecular weight [Control (■), low molecular weight 600 (•) and 1,000 (○); high molecular weight 600 (▴), and 1,000 (Δ) mg/L], at different temperatures 5°C (A) and 35°C (B).
Weibull model fit adequately (R2 > 0.8908) with experimental data and the biological parameters obtained are showed in Table 1. Statistical analysis shows that for the inactivation rate (b) there were no differences among the treatments that showed inhibition, regardless of chitosan MW. The concentration of 600 mg/L of both types of chitosan at 35°C of incubation, was similar to the control experiments, with no inactivation rate (b = 0). For the n value, it can be observed that V. cholerae Non-01 was resistant to chitosan of low and high MW at 600 mg/mL incubated at 35°C, without significant differences (p > 0.05) to the control. Thus, the biggest cellular damage occurs with high chitosan concentrations (1,000 mg/L), regardless of molecular weight and treatment temperature(p < 0.05).
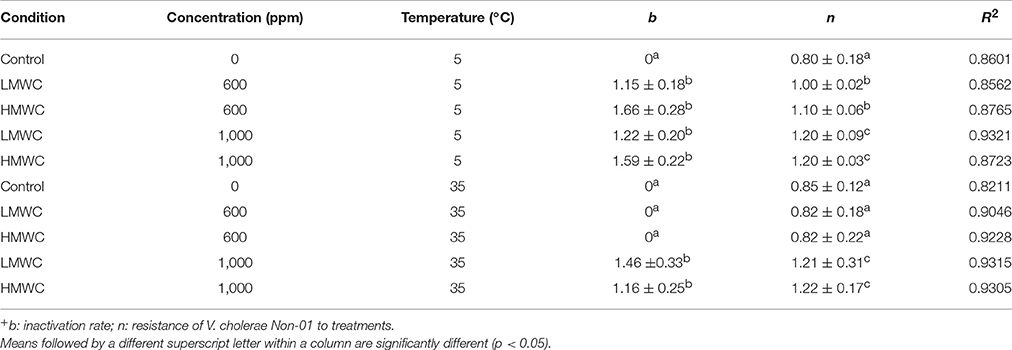
Table 1. Weibull model parameters+ (mean ± standard deviation) for V. cholerae Non-01 growth or inhibition curves subjected to selected concentrations of low or high molecular weight chitosan at different temperatures.
In order to evaluate the antimicrobial effect of LMWC and HMWC to V. cholerae Non-01, resistance frequency plots were plotted for all treatments that had n > 1 values. In Figure 2 it can be observed in Figure 2 that there is a greater resistance of V. cholerae Non-01 to LMWC, since the maximum values of resistance are presented in the first minutes of the treatments regardless of the temperature; finally, microbial resistance is close to zero at around 4 h.
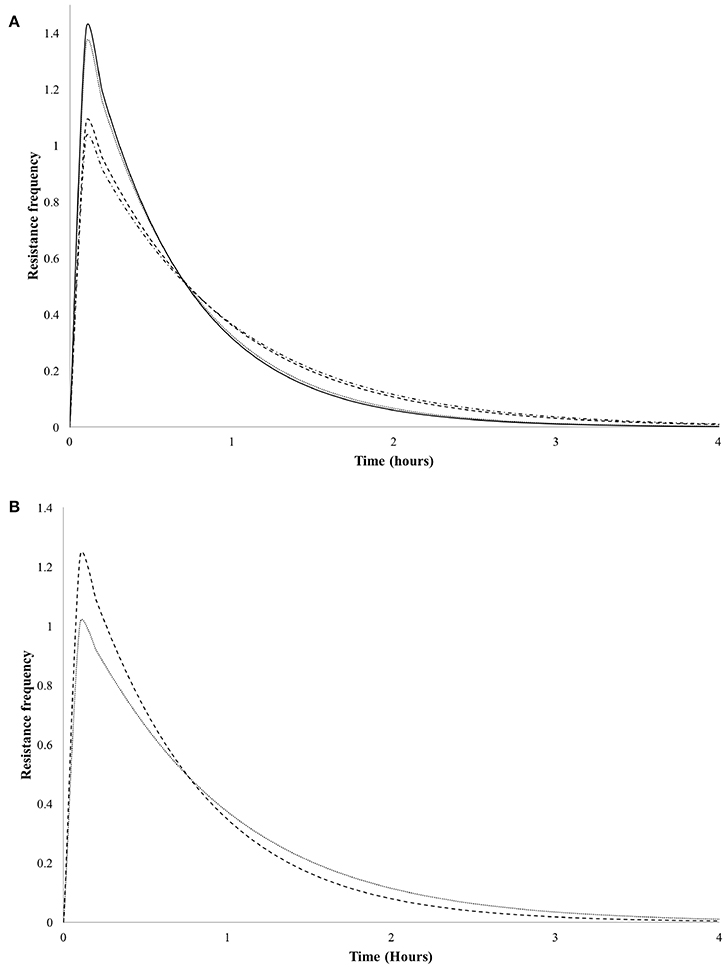
Figure 2. Resistance frequency curves of Vibrio cholerae Non-01 at selected concentrations and molecular weight [low molecular weight 600 (-•-•-) and 1,000 (----); high molecular weight 600 (—), and 1,000 (••••) mg/L] on, at different temperatures 5°C (A) and 35°C (B).
Discussion
In studies conducted at temperatures below 37°C, Zivanovic et al. (2004) reported a reduction of 7.5 log cycles of Salmonella Typhimurium (72 h, 25°C) by using a concentration of 1,000 mg/L of LMWC (150 KDa) and 15% DA; when the authors decreased the temperature (10°C), they needed more time (96 h) to reduce 7 log cycles. Similar results are reported here, since a concentration of 1,000 mg/L had a bactericidal effect on V. cholerae at 35°C in 12 h; while at lower temperature (5°C), longer time is requiered (312 h). The differences observed between the temperatures can be related to bacterial cells stress at lower temperature, as well as to the low interaction between chitosan and bacterial cells. Some authors conclude that the intermolecular aggregates formed by chitosan suspensions, reduced the number of available sites for interaction with the bacterial cell, and as a consequence, the antimicrobial effectiveness is affected (Rabea et al., 2003; Zivanovic et al., 2004).
In another study on fish filets treated with 1% chitosan and stored at 4°C, a favorable effect on two species of Vibrio (V. cholerae and V. parahaemolyticus) was observed within the first 5 days of contact, but bacterial population increased later to reach the same population than the positive control; chitosan with different molecular weight had the same effect at the same concentration (Tsai et al., 2002). A bacteriostatic effect was also observed in this study with 600 mg/L concentration of LMWC and HMWC at 5°C; a higher concentration of chitosan (1,000 mg/L) had a bactericidal effect at 312 h and the same temperature. It is important to emphasize that similar behavior was observed, even when the experimental matrix was different (fish filets vs. culture broth).
No et al. (2003), reported a reduction of up to 7.5 logarithmic cycles of V. parahaemolyticus in 24 h at 37°C, using the same chitosan concentration than in our study, but with a lower molecular weight (81 KDa). Similar inhibition concentrations were reported by Yang et al. (2005) who was able to reduce 7 log cycles of E. coli in 6 h at 37°C. Kaya et al. (2015) presented similar inhibition of V. alginolyticus with chitosan obtained from insects (cosmopolitan Orthoptera species); the effect was even better than the antibacterial activity presented by standard antibiotics. Raafat and Sahl (2009) explains that a MW higher than 100 KDa is required for chitosan to present microbial inhibition. After testing a large number of preparations, the antimicrobial activity did not differ among chitosan with MW higher than 100 KDa. According to the V. parahaemolyticus control plan established by FDA, antimicrobials should achieve 2–3 log reduction (FDA, 2011) of the pathogen; LMWC o HMWC at 1,000 mg/L concentrations, were able to reduce V. cholerae Non-01 population to nearly 6.0 log cycles.
One of the problems associated with the use of chitosan as antimicrobial in suspension, is the low water solubility and high viscosity of the polymer solutions; the production of lower molecular weight chitosan by enzymatic or chemical reactions has been proposed to overcome this problem (Gohi et al., 2017). Tsai et al. (2004) demonstrated specifically that for V. parahaemoliticus HMWC (Chitosan D90, MW 230 KDa) has a better antimicrobial action than low molecular weight hydrolysates (MW 12 KDa). They also demonstrated that further degradation of chitosan altered its antimicrobial capacity, since the mixture of chitooligosaccharide tested were not antimicrobial. Similar results were reported by Liu et al. (2015), that evaluated the effect of chitosan hydrolysates against E. coli, where a molecular weight of 88–90 KDa were the most effective. Low molecular weight, water-soluble chitosan hydrolysates can penetrate the microbial cell wall, with a different mechanism of action than the higher molecular weight polymer (Goy et al., 2009). Also, chemical modifications to increase water solubility of chitosan have proven to improve antimicrobial capacity, such as the increase in effectiveness by a chitosan oligomer (chitosan oligosaccharide lactate) when tested against fish pathogenic bacteria (Yildirim-Aksoy and Beck, 2017).
Weibull model is suitable to predict V. cholerae Non-01 inhibition by chitosan preparations, and proved to be useful for research purposes, although it is also possible to be used as a routine measurable parameter in food analysis. There are few reports that describe the antimicrobial effect of chitosan using mathematical models; Weibull biological parameters provide information that can be used to assess the response of other bacterial strains as well as to evaluate the effect of chitosan in additional laboratory conditions or in food systems.
As reported above, no significant differences (p > 0.05) of inactivation rate (b) values could be due to the interaction of chitosan with cell surface polymers, which may interfere with the microbial cellular membrane functions. The interaction can lead to release of small cellular components such as electrolytes or peptides, even without the formation of a membrane pore (Goy et al., 2009; Raafat and Sahl, 2009). A bigger cellular damage can be caused when the environmental pH is below chitosan pKa (Kong et al., 2010). It is suggested that chitosan bind to the outer membrane, which explains the loss of the barrier function (Helander et al., 2001).
Resistance frequency values could be explained by the progress of a sublethal injury to microbial cell death, due to metabolic disruption caused by chitosan adhesion to the cell surface. The transition from sublethal injury, (caused by disruption of the cell permeability barrier and leakage), to cell death that might be mediated by metabolic imbalance and impaired ionic homeostasis following chitosan challenge. Considering that chitosan binding with the cell membrane is based on electrostatic interactions, the number of cationized amines in chitosan will be related to the antimicrobial activity presented (Goy et al., 2009; Raafat and Sahl, 2009). Finally, Weibull modeling can adequately describes the antimicrobial effect of LMWC and HMWC and could be potentially used to propose control measurements of V. cholerae Non-01 contamination.
Author Contributions
MP and GN conceived and designed the experiments, MP performed the experiments, RA and GN performed statistical and modeling analysis, all authors participated in the preparation of the manuscript.
Funding
Partial financial support for this project was provided by CONACYT-CONAFOR grant CO4-2001-035. MP received and scholarship from CONACYT for her graduate studies.
Conflict of Interest Statement
The authors declare that the research was conducted in the absence of any commercial or financial relationships that could be construed as a potential conflict of interest.
Acknowledgments
Special thanks to Luisa Lorena Silva Gutiérrez, from Centro de Investigación en Alimentación y Desarrollo, A.C. Unidad Guaymas, for their support on chitosan characterization.
References
Arancibia, M. Y., Alemán, A., Calvo, M. M., López-Caballero, M. E., Montero, P., and Gómez-Guillén, M. C. (2017). Antimicrobial and antioxidant chitosan solutions enriched with active shrimp (Litopenaeus vannamei) waste materials. Food Hydrocoll. 35, 710–717. doi: 10.1016/j.foodhyd.2013.08.026
Argüelles, W., Heras Caballero, A., Acosta, N., Galed, G., Gallardo, A., and Miralles, B. (2004). “Caracterización de quitina y quitosano,” in Quitina y Quitosano: Obtención, Caracterización y Aplicaciones, eds A. Pastor, E. Agulló, C. Peniche, C. Tapia, W. Argüelles, and J. Mayorga Nakamatsu (Lima: Programa CYTED, CIAD y Pontificia Universidad Católica del Perú), 195–197.
Avadi, M. R., Sadeghi, A. M. M., Tahzibi, A., Bayati, K. H., Pouladzadeh, M., Zohuriaan-Mehr, M. J., et al. (2004). Diethylmethyl chitosan as an antimicrobial agent: synthesis, characterization and antibacterial effects. Eur. Polym. J. 40, 1355–1361. doi: 10.1016/j.eurpolymj.2004.02.015
Avila-Sosa, R., Ochoa-Velasco, C. E., Navarro-Cruz, A. R., Palou, E., and López-Malo, A. (2016). “Combinational approaches for antimicrobial packaging: chitosan and oregano oil,” in Antimicrobial Food Packaging, ed J. Barros-Velázquez (London: Academic Press), 581–588.
Bagder-Elmaci, S., Gölgser, G., Tokatk, M., Erten, H., Isci, A., and Özcelik, F. (2015). Effectiveness of chitosan against wine-related microorganisms. Anton. Leeuw. Int. J. G. 107, 675–686. doi: 10.1007/s10482-014-0362-6
Benhabiles, M. S., Salah, R., Lounici, H., Drouiche, N., Goosen, M. F., and Mameri, N. (2012). Antibacterial activity of chitin, chitosan and its oligomers prepared from shrimp shell waste. Food Hydrocoll. 29, 48–56. doi: 10.1016/j.foodhyd.2012.02.013
Ciafardini, G., and Zullo, B. A. (2002). Survival of microorganisms in extra virgin olive oil during storage. Food Microbiol. 19, 105–109. doi: 10.1006/fmic.2001.0458
FDA (2011). Chapter II Risk Assessment and Risk Management. National Shellfish Sanitation Program (NSSP) Guide for the Control of Molluscan Shellfish, 31. Available online at: http://www.fda.gov/downloads/Food/GuidanceRegulation/FederalStateFoodPrograms/UCM350344.pdf.
Fei Liu, X., Lin Guan, Y., Zhi Yang, D., Li, Z., and De Yao, K. (2001). Antibacterial action of chitosan and carboxymethylated chitosan. J. Appl. Polym. Sci. 79, 1324–1335. doi: 10.1002/1097-4628(20010214)79:7<1324::AID-APP210>3.0.CO;2-L
Gastélum, G. G., Avila-Sosa, R., López-Malo, A., and Palou, E. (2012). Listeria innocua multi-target inactivation by thermo-sonication and vanillin. Food Bioproc. Technol. 5, 665–671. doi: 10.1007/s11947-010-0334-4
Gohi, B. F. C. A., Zeng, H. Y., Pan, A. D., Han, J., and Yuan, J. (2017). pH dependence of chitosan enzymolysis. Polymers 9:174. doi: 10.3390/polym9050174
Gougouli, M., and Koutsoumanis, K. P. (2012). Modeling germination of fungal spores at constant and fluctuating temperature conditions. Int. J. Food Microbiol. 152, 153–161. doi: 10.1016/j.ijfoodmicro.2011.07.030
Goy, R. C., de Britto, D., and Assis, O. B. G. (2009). A review of the antimicrobial activity of chitosan. Polímeros Ciênc. Tecnol. 19, 241–247. doi: 10.1590/S0104-14282009000300013
Helander, I., Nurmiaho-Lassila, E. L., Ahvenainen, R., Rhoades, J., and Roller, S. (2001). Chitosan disrupts the barrier properties of the outer membrane of Gram-negative bacteria. Int. J. Food Microbiol. 71, 235–244. doi: 10.1016/S0168-1605(01)00609-2
Kaya, M., Baran, T., Asan-Ozusaglam, M., Cakmak, Y. S., Tozak, K. O., Mol, A., et al. (2015). Extraction and characterization of chitin and chitosan with antimicrobial and antioxidant activities from cosmopolitan Orthoptera species (Insecta). Biotechnol. Bioproc. E. 20, 168–179. doi: 10.1007/s12257-014-0391-z
Kong, M., Chen, X. G., Xing, K., and Park, H. J. (2010). Antimicrobial properties of chitosan and mode of action: a state of the art review. Int. J. Food Microbiol. 144, 51–63. doi: 10.1016/j.ijfoodmicro.2010.09.012
Liu, N., Sun, Y., and Zhou, D. (2015). Antimicrobial activity and mechanism of chitosan with different molecular weight. Proc. ICMTMA 2015, 166–169.
No, H. K., Lee, S. H., Park, N. Y., and Meyers, S. P. (2003). Comparison of physicochemical, binding, and antibacterial properties of chitosans prepared without and with deproteinization process. J. Agric. Food Chem. 51, 7659–7663. doi: 10.1021/jf030226w
No, H. K., Meyers, S. P., Prinyawiwatkul, W., and Xu, Z. (2007). Applications of chitosan for improvement of quality and shelf life of foods: a review. J. Food Sci. 72, 87–100. doi: 10.1111/j.1750-3841.2007.00383.x
Peleg, M., and Cole, M. B. (1998). Reinterpretation of microbial survival curves. Crit. Rev. Food Sci. Nutr. 38, 353–380. doi: 10.1080/10408699891274246
Peleg, M., and Shetty, K. (1997). Modeling microbial populations with the original and modified versions of the continuous and discrete logistic equations. Crit. Rev. Food Sci. Nutr. 37, 71–490. doi: 10.1080/10408399709527785
Raafat, D., and Sahl, H. G. (2009). Chitosan and its antimicrobial potential - a critical literature survey. Microb. Biotechnol. 2, 186–201. doi: 10.1111/j.1751-7915.2008.00080.x
Rabea, E. I., Badawy, M. E. T., Stevens, C. V., Smagghe, G., and Steurbaut, W. (2003). Chitosan as antimicrobial agent: applications and mode of action. Biomacromolecules 4, 1457–1465. doi: 10.1021/bm034130m
Tsai, G. J., Su, W. H., Chen, H. C., and Pan, C. L. (2002). Antimicrobial activity of shrimp chitin and chitosan from different treatments and applications of fish preservation. Fisheries Sci. 68, 170–177. doi: 10.1046/j.1444-2906.2002.00404.x
Tsai, G. J., Zhang, S. L., and Shieh, P. L. (2004). Antimicrobial activity of a low-molecular-weight chitosan obtained from cellulase digestion of chitosan. J. Food Protect. 67, 396–398. doi: 10.4315/0362-028X-67.2.396
Xia, W., Liu, P., Zhang, J., and Chen, J. (2011). Biological activities of chitosan and chitooligosaccharides. Food Hydrocoll. 25, 170–179. doi: 10.1016/j.foodhyd.2010.03.003
Yang, T. C., Chou, C. C., and Li, C. F. (2005). Antibacterial activity of N-alkylated disaccharide chitosan derivatives. Int. J. Food Microbiol. 97, 237–245. doi: 10.1016/S0168-1605(03)00083-7
Yildirim-Aksoy, M., and Beck, B. H. (2017). Antimicrobial activity of chitosan and a chitosan oligomer against bacterial pathogens of warmwater fish. J. Appl. Microbiol. 122, 1570–1578. doi: 10.1111/jam.13460
Keywords: V. cholerae, chitosan, Weibull distribution, inhibition modeling, inhibition frequency
Citation: Paredes-Aguilar MdlC, Avila-Sosa R and Nevárez-Moorillón GV (2017) Modeling the Inhibition of Vibrio cholerae Non-01 in Trypticase Soy Broth by Chitosan of Low and High Molecular Weight. Front. Mar. Sci. 4:341. doi: 10.3389/fmars.2017.00341
Received: 04 August 2017; Accepted: 12 October 2017;
Published: 27 October 2017.
Edited by:
Pedro Fernandes, Universidade de Lisboa, PortugalReviewed by:
Odilio Benedito Garrido Assis, Embrapa Instrumentacao, BrazilIddya Karunasagar, Nitte University, India
Copyright © 2017 Paredes-Aguilar, Avila-Sosa and Nevárez-Moorillón. This is an open-access article distributed under the terms of the Creative Commons Attribution License (CC BY). The use, distribution or reproduction in other forums is permitted, provided the original author(s) or licensor are credited and that the original publication in this journal is cited, in accordance with accepted academic practice. No use, distribution or reproduction is permitted which does not comply with these terms.
*Correspondence: Guadalupe V. Nevárez-Moorillón, vnevare@uach.mx