- Department of Biology, Lamont-Doherty Earth Observatory, Columbia University, Palisades, NY, United States
Considerable attention has been given to the roles of the carbon and phosphate cycles in aquatic environments, but less attention has been given to an experimental analysis of the coupling of the C and P cycles in freshwater and marine ecosystems. Using laboratory microcosm experiments, prepared with natural pond-water microbial communities, evidence is presented for the coupling of dissolved organic C with microbial production of alkaline phosphatase driving the phosphorus cycle in freshwater microbial communities. The effects of glucose C-supplementation in microcosm microbial communities (including bacteria and heterotrophic nanoflagellates) on gains in microbial C-content and alkaline phosphatase activity (APA) were estimated in relation to control microcosms without C-supplementation. The C-supplementation increased total microbial APA (pmol min−1 μg−1 bacterial C) in the C-supplemented treatment (6.5 ± 0.6) compared to the non-supplemented cultures (5.1 ± 1.7). Microbial-bound APA in the C-supplemented treatment was particularly enhanced (4.4 ± 0.9) compared to control cultures (1.3 ± 0.8), but the amount of free (soluble) APA in the aquatic phase was less compared to the controls (n = 5, p < 0.001). Alkaline phosphatase activity was highly correlated (r = 0.97) with bacterial densities in the C-supplemented cultures, further supporting the hypothesis that C-supplementation can increase phosphorus remineralization through elevated production of microbial alkaline phosphatase. This laboratory-based, experimental study suggests that additional research on the coupling of the C and P cycles in freshwater and marine environments may yield productive insights into the finer details of the roles of these two biogeochemical cycles in aquatic microbial community dynamics.
Introduction
There is considerable evidence that eukaryotic microbial communities serve a significant role in fertility of aquatic and terrestrial environments (e.g., Bloem et al., 1989; Adl, 2003; Anderson, 2014b), particularly the contribution of heterotrophic protists such as nanoflagellates that prey on bacteria and promote remineralization of mineral and carbon compounds (e.g., Cole et al., 1977; Clarholm, 1989; Caron, 1994; Selph et al., 2003; Anderson, 2012). Some of this effect can be attributed to nutrient release during phagotrophic feeding, especially in the microbial loop (e.g., Güde, 1985; Caron, 1994). Further contributions come from the death and lysis of bacteria (Renn, 1937) and by stimulation of bacterial hydrolytic enzymes mediating remineralization of inorganic nutrients such as phosphorus and nitrogen (Hadas et al., 1992; Ferrier-Pagès and Rassoulzadegan, 1994). In heterotrophic microbial communities, organic carbon compounds are a major source of nutrients for bacteria at the base of the food web (e.g., Ducklow, 2000; Anderson and McGuire, 2013). Heterotrophic nanoflagellates (HNAN) are often abundant major predators on bacteria, and are important links in the trophic hierarchy between bacteria and higher biota such as invertebrates (Caron et al., 1985; Anderson, 2012); therefore, the abundance and C-content of HNAN are analyzed in addition to bacteria. Moreover, because there is a focus in this study on C enrichment, and the C and P biogeochemical cycles in microbial communities, the alkaline phosphatase activity (APA) is normalized to bacterial C-biomass, expressed as APA units μg−1 bacterial C-content.
Although there is substantial published research on the role of organic C in microbial community dynamics, there is less research on the coupling of organic C with the phosphorus cycle, including production of microbial phosphatases, a potentially significant source of phosphate remineralization, especially in aquatic environments (Gibson et al., 1996; Dyhrman et al., 2007; Duhamel and Moutin, 2009; Ruttenberg and Dyhrman, 2012; Duhamel et al., 2014). Because of the potential threats from global warming, considerable research has been done on the coupling of nitrogen and carbon cycles, largely in relation to the effect of nitrogen on primary production, thus enhancing CO2 sequestration (Esser et al., 2011; Zaehle, 2013). However, there is a relative lack of ecological experimental research on the potential role of organic C in driving the phosphorus cycle as mediated by microbial organo-phosphohydrolytic enzymes within the context of a community level analysis. A complex network of interactions link microbial communities to the biogeochemical changes of C and P (e.g., Anderson et al., 2018), including the role of increased atmospheric C as CO2 that drives primary production, thus producing more soluble organic nutrients in aquatic ecosystems. Soluble nutrients potentially enhance heterotrophic microbial production, including release of enzymes such as alkaline phosphatases that convert organic phosphates into free phosphate available to support further growth of primary producers, and micro-heterotrophs. Understanding the dynamics and inter-relations of these processes can yield deeper insights into aquatic microplankton interactions.
This is a report of the effects of dissolved organic C (DOC) enrichment on alkaline phosphatase activity in freshwater heterotrophic microbial communities with a goal of estimating the role of DOC in phosphorus remineralization. The major research questions addressed are as follows: (1) What is the effect of emending freshwater microbial cultures with glucose-C on the relative gain in densities and C-content of bacteria and heterotrophic nanoflagellates? (2) To what extent does enrichment with glucose-C affect the alkaline phosphatase activity in these cultures? (3) Is there experimental evidence of coupling of the C cycle and phosphorus cycle in aquatic microbial communities?
Materials and Methods
Laboratory Cultures
Laboratory cultures of microbial communities were established using a standard source of pond water distributed by Carolina Biological (Burlington, NC), a filtered and pasteurized water obtained from a local freshwater pond at Burlington, NC. To establish a sufficiently robust microbial community in laboratory cultures, the pond water was inoculated with a suspension of dark, rich sediment obtained from the margin of a freshwater pond on the Lamont-Doherty Earth Observatory Campus (41.004247, - 73.911677). A sample of the surface sediment (0.1 g) was added to 5 ml of the Carolina pond water in a 15-ml plastic conical centrifuge tube and thoroughly suspended using a vortex mixer. One milliliter of the suspension was added to 100 ml of Carolina pond water to be used for the experimental laboratory cultures. Fifty milliliter of this prepared pond water was distributed into each of two sterile 250 ml Falcon culture flasks (Thermo Fisher Scientific, Waltham, MA). One flask served as a control and the other flask served as a C-enrichment culture, emended with reagent grade glucose at a final concentration of 4 mg glucose-C ml−1 water. The cultures were maintained in the dark at 20°C (a reasonable growth temperature in a temperate pond) in a constant temperature incubator (Percival Scientific, Inc., Perry, IA) for 6 days before analysis; assuming the glucose remained labile. Experiments were replicated 5 times during 2 weeks. Because a different 0.1 g portion of sediment was used for each replication, the initial composition of the inoculating biota varied. At 6 days, the final biomass varied sufficiently to permit correlation of enzyme activity with densities.
Microbial Densities and C-Content
After 6-d incubation, 5 ml of thoroughly suspended culture were withdrawn from each of the control and treatment flasks and fixed by addition of pure glutaraldehyde (Ladd Research, Williston, VT), final concentration of 3% (v/v). Bacterial and heterotrophic flagellate densities and C-content were assessed at 1000x magnification by UV epifluorescent microscopy (Anderson et al., 2001). During microscopic enumeration of bacteria and HNAN, they were sized within 0.5 μm using an ocular reticule, deemed sufficiently accurate for the purposes of this research. While counting cocci, the diameter of representative individuals was measured and used in the formula for a sphere to estimate the volume. Likewise, the major and minor axes of bacilli were measured and used in the formula for a prolate spheroid to estimate the cell volume. The length and width of HNANs was assessed during counting and converted to volume using the formula for a prolate spheroid. The C-content of each group of microbes was estimated using a size-based regression equation relating C-content to cell volume (125.3 fg C μm−3) as previously published by Pelegri et al. (1999). Densities of the microbes and their biomasses were expressed as number ml−1 and μg ml−1, respectively, and reported as means ± SE based on data from the five replicate experiments. The Pelegri et al. coefficient for conversion of volume to C-content was deemed to be adequate for both bacteria and HNAN, because the HNAN are relatively small in the range of 2–3 μm. Thus the differences in size ranges are not likely to cause a major bias in C-content estimates, at least within the precision needed for the limited comparisons made in this study.
Alkaline Phosphatase Activity Assays
Alkaline phosphatase activity in the control and treatment cultures was assayed using a Sigma APO100 colorimetric kit with paranitrophenylphosphate (pNPP) substrate as specified by the supplier (Sigma-Aldrich, St. Louis, MO). Blank cuvettes were prepared by adding 980 μL of buffer (1.0 M Diethanolamine and 0.50 mM Mg Cl2, pH 9.8). Test cuvettes were prepared by adding 20 μL pNPP substrate solution and 960 μL of the buffer. The cuvettes were equilibrated to 37°C before adding 20 μL of the microcosm test sample to the test and blank cuvettes, respectively. The contents of the cuvettes were each immediately mixed by inversion and incubated for 30 min. The absorbance of the test sample vs. the blank was measured at a wavelength of 405 nm using a Beckman DU dual beam spectrophotometer (Beckman, Indianapolis, IN). Triplicate measurements were made for each assay and a mean value was calculated. Total enzyme activity in the control and treatment cultures was obtained using unaltered aliquots of samples from each condition. Free activity (non-bacterial bound) was assayed by passing samples of the cultures through 0.22 μm pore-sized filters (EMD Millipore Corp., Billerica, MA) before enzyme analysis. The enzyme assays were done immediately after sample aliquots were taken from the microcosms and also immediately after filtration through the micropore filters. Bacterial-bound activity was estimated by subtraction of the free activity from the total activity. Enzyme activity was expressed as μmol min−1 ml−1 of culture, or converted to pmol min−1 μg−1 of bacterial C in samples from each culture condition. The enzyme activities were converted from μmol min−1 ml−1 to pmol min−1 μg−1 C, by first converting the volume in ml to μg-C based on the estimated C ml−1 for the density of bacteria in the sample. The alkaline phosphatase activity was converted from μmol min−1 to pmol min−1 by dividing it by 106, the number of pmols in a μmol. APA activity was normalized to only the bacterial C-content, because the HNAN C-content was relatively negligible; i.e., as much as two orders of magnitude less. Therefore, for purposes of approximation suitable for this analysis, the APA was normalized only to bacterial C-content. Bacteria can be good proxies for microbial contributions to some ecologically relevant measurements, such as correlation with total soil respiration in some ecosystems (e.g., Anderson, 2014a). Moreover, expressing APA units in terms of bacterial C-content is consistent with prior studies in our research program.
Statistical Analyses
Correlation analyses were obtained using StatPlus® (AnalystSoft, Inc., Alexandria, VA) for Microsoft Excel (Microsoft Corp., Redmond, WA), Student's t-test (GraphPad Software, La Jolla, CA) was used to assess the statistical significance of differences (p ≤ 0.05) in mean data for the experimental and control samples, because it is an appropriate analysis to use for data from two groups. Additionally, the change in value (gain) for each of the two means was also reported.
Results
Question 1: C Enhancement Effects on Microbial Densities and C-Content
What is the effect of emending freshwater microbial cultures with glucose-C on the relative gain in densities and C-content of bacteria and heterotrophic nanoflagellates? Mean densities and C-content of bacteria and heterotrophic nanoflagellates, including gains resulting from C enrichment (experimental treatment values minus control values), are presented in Table 1. As would be expected, the data for heterotrophic nanoflagellates (higher up the trophic hierarchy) were substantially lower than for the bacteria at the base of the food chain. The relative gains in density for the two groups of microbes expressed as percentage increase of the C-supplemented cultures relative to the controls are: bacteria (189%), and HNAN (192%). With respect to the relative gains in C-content, the corresponding proportional increases expressed as percentages are: bacteria (195%), and HNAN (188%).
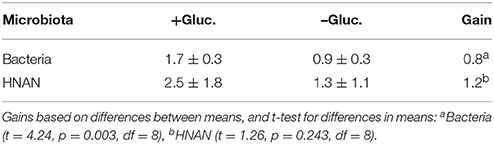
Table 1. Mean ± SE densities (no. ml−1) of bacteria (×109), heterotrophic nanoflagellates (HNAN) (×106) in glucose-supplemented (+Gluc.) and non-supplemented (–Gluc.) cultures, and the gain expressed as the mean difference between the two conditions.
Question 2: C Enhancement of Alkaline Phosphatase Activity
To what extent does enrichment with glucose-C affect the alkaline phosphatase activity in these cultures? Mean data for alkaline phosphatase activities (total, free, and bacterial-bound) for the C-enriched and control cultures are presented in Table 3. The amount of enzyme activity was larger in the C-enriched cultures compared to the control cultures. The gain for the total enzyme activity, normalized to bacterial C, was 1.4 pmol min−1 μg−1 bacterial C. For free activity, there was a relatively greater amount in the control than the treatment culture with a difference of −1.7. The relative gain in bacterial-bound activity, obtained by algebraic subtraction of the mean free value (−1.7) from the total (1.4), was 3.1 (Table 3). The gain in bound APA is larger than the estimated total gain, because the free enzyme activity was lower in the control culture than the C-enriched culture. This indicates that C-enrichment tended to increase the bacterial-bound enzyme activity relative to that of the controls. It is important to note that these values are gains (difference values), hence it is possible for the estimate of the gain in bacterial-bound activity to be greater than the gain in total activity. The linear correlation of the enzyme activity with bacterial densities was r = 0.97 (p < 0.001, N = 5). Thus, bacterial densities accounted for ~94% of the variance in APA based on the square of the correlation coefficient.
Question 3: Coupling of the C and Phosphorus Cycles
Is there evidence of coupling of the C cycle and phosphorus cycle in aquatic microbial communities? Based on the foregoing data, evidence indicates that soluble organic C enrichment in the aquatic microbial communities not only increases the C-content of the microbiota, but secondarily also increases the alkaline phosphatase activity (particularly bacterial-bound APA), thus potentially driving phosphorus remineralization due to organo-phosphate hydrolysis. As reported above, in this experimental preparation, the total APA enzyme activity increased by a factor of 1.4 for every μg of glucose-C added, when compared to the control; and there was a substantial increase in bacterial-bound APA activity. Based on the statistical analyses in Tables 1–3, the increase in bacterial abundance and bacterial-bound APA in the C-enriched treatments are statistically significant (p < 0.05).
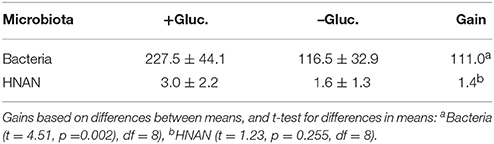
Table 2. Mean ± SE carbon content (μg ml−1) of bacteria and heterotrophic nanoflagellates (HNAN) in glucose-supplemented (+Gluc.) and non-supplemented (–Gluc.) cultures, and the gain in C expressed as the mean difference between the two conditions.
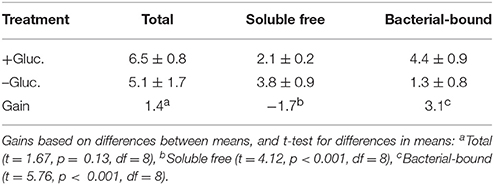
Table 3. Mean ± SE of total, free, and bacterial-bound alkaline phosphatase activity (pmol min−1 μg−1 bacterial C) in glucose-supplemented (+Gluc.) and non-supplemented (–Gluc.) cultures, and the gain expressed as the mean difference between the two conditions.
Discussion
Summary of Conclusions
Glucose enrichment of the freshwater culture medium produced a gain of 111 μg bacterial C ml−1 of culture medium. This represents ~3% of the amount of glucose-C added ml−1 (i.e., 4.0 mg ml−1). A positive correlation between alkaline phosphatase activity and bacterial densities was found accounting for ~94% of the variance in the enzyme activity, estimated from the square of the correlation coefficient. Based on this experiment, for every μg of C added above that in the control culture, the microcosm alkaline phosphatase activity (pmol min−1 μg−1 bacterial C) increased by a factor of 1.4. Moreover, C-enrichment tends to increase the amount of bacterial-bound alkaline phosphatase, compared to that in control cultures lacking C-enrichment. In this study, the APA was normalized to estimated total bacterial C biomass, because the focus was on the C-budget and relevance of the APA to the C-cycle. However, this does not provide an estimate of the APA relative to the standing stock of metabolically active bacteria. Such estimates can be achieved by using fluorescent staining techniques that permit microscopic identification of the bacteria exhibiting surface APA activity. Some of these methods are discussed more fully in Section Relationships to prior research.
In this study, glucose was used as the sole C source. Glucose is commonly used in experimental microbe ecological research as representative of smaller, soluble organic nutrients (e.g., Hanson and Snyder, 1980; Shiah and Ducklow, 1994; Frost and Elser, 2002; Meon and Amon, 2004; Valliéres et al., 2008). Moreover, phytoplankton can be a significant source of glucose, in natural aquatic systems (e.g., Fogg, 1971; Moshiri et al., 1979). However, further research using a broader range of organic nutrients is needed to more fully document the effects of soluble C-enrichment on community structure and the production of APA, as well as the fate of soluble P produced as a result of organic phosphohydrolysis. Among other aspects, the molecular size and chemical composition of the organic matter, as well as its state (dissolved or particulate), needs to be examined in relation to the role of microbial communities in the aquatic P cycle.
Both the quality (kind) and quantity of soluble organic matter in natural aquatic ecosystems affect the composition and activity of microbial communities (e.g., Pérez and Sommaruga, 2006; Apple and del Giorgio, 2007). Although the use of a single C source in this study provided initial, experimental evidence of coupling of the C and P cycles, further studies with a broader composition of soluble organic matter may contribute greater predictability of the microbial community activity relative to the natural environment. Moreover microcosm, and other “bottle,” experiments can introduce artifacts during laboratory incubation. Among other factors, the composition and activity of the prokaryote community may change during incubation, especially with longer durations such as days (e.g., Massana et al., 2001), especially if predator-prey relationships are not typical of the natural environment. In this study, heterotrophic nanoflagellate predators were present, and their densities and estimated C-biomass were included in addition to estimates for the bacteria (Tables 1, 2).
Relationships to Prior Research
These data support the hypothesis of close coupling of the carbon and phosphorus biogeochemical cycles in freshwater aquatic microbial communities and provides a quantitative estimate of the likely gain in phosphorus remineralization that can occur due to soluble organic C enrichment. Organic C derived potentially from primary production, from decaying biota, or from allochthonous sources, can enhance microbial alkaline phosphatase production and thus increase phosphorus remineralization by organo-phosphate hydrolysis. Based on the evidence in this research, it appears that C-enrichment produces less free enzyme and more bacterial-bound enzyme compared to control conditions without C-enrichment. This has also been reported in studies of organic C enrichment in natural environments (e.g., Chróst et al., 1989). It is not immediately clear why this is the case. However, further research is needed to better document changes in the cellular physiology of the microbes, particularly examining the localization of the APA on the bacterial surface, perhaps by using modern fluorescent labeling techniques (e.g., Giepmans et al., 2006). Moreover, additional insights may be obtained by transcriptome analyses to determine if there are changes temporally in the transcription of alkaline phosphatase mRNA and other proteins of biogeochemical significance (e.g., Mark et al., 2005). This avenue of research may be enhanced by examining the effects of different levels of aquatic C enrichment on microbial APA, and possible variations in its transcription.
Although prior research has documented the role of phagotrophic predation in the release of organo-phosphohydrolases, thus promoting phosphorus remineralization, the data presented here augments these prior findings by adding experimental evidence of C-based increase in APA production within the context of as somewhat broader community-based analysis, including major bacterial predators (e.g., HNAN). Free phosphate is typically assimilated much more rapidly by bacteria than heterotrophic eukaryotic microbes and bacteria may be one of the more important contributors to the phosphorous cycle in marine ecosystems (Duhamel and Moutin, 2009; Popendorf and Duhamel, 2015). Moreover, among lake microplankton, bacteria may account for as much as 94% of the APA (Chróst et al., 1984). Overall, the increased available phosphorus due to C enrichment as observed in the research reported here may enhance bacterial growth, thus providing a positive forcing function on growth of bacterial predators higher up the food web. In some marine environments, however, bacterial remineralization of soluble phosphates may exceed the immediate needs of the bacteria, thus making the excess P directly available to drive primary production of phytoplankton, and consequently increased biomass of their predators (White et al., 2012).
The research reported here expands our knowledge base of the dynamic role of organic C, beyond its role as a nutrient source, to include a link to increased alkaline phosphatase activity, thus driving phosphorus remineralization in freshwater heterotrophic microbial communities. This is one of the first experimental microcosm studies to provide a quantitative estimate of the C-supplementation effect on APA in microbial communities. It is consistent with prior published research in the natural environment showing that dissolved organic C has significant contributory effects on activity of bacterial alkaline phosphatase and primary productivity in freshwater and marine ecosystems (e.g., Stewart and Wetzel, 1982; Chróst et al., 1989; Boyer et al., 2006). Moreover, similar results of C-enrichment effects on APA were recently reported for laboratory microcosm studies using organic-rich arctic soil microbial communities (Anderson et al., 2018). Elucidation of the finer ecological implications will require additional research on how environmental variables interact with the organic C enhancement effect, including variations in temperature, oxygen concentration, organic and mineral nutrient concentrations (particularly organo-phosphate substrates and available free phosphate), and interactions of the heterotrophic microbiota with photosynthetic protists in the aquatic environment. Further comparative microcosm, and field-based studies, across a continuum of different terrestrial and aquatic ecosystems (e.g., coastal and oceanic), correlating organic C concentrations with microbial alkaline phosphatase activity, are needed to provide further evidence of the coupling of carbon and phosphate cycles in the natural environment as suggested by this laboratory experimental study.
Author Contributions
The author confirms being the sole contributor of this work and approved it for publication.
Conflict of Interest Statement
The author declares that the research was conducted in the absence of any commercial or financial relationships that could be construed as a potential conflict of interest.
Acknowledgments
This is Lamont-Doherty Earth Observatory Contribution number 8179.
References
Anderson, O. R. (2012). The role of bacterial-based protist communities in aquatic and soil ecosystems and the carbon biogeochemical cycle, with emphasis on naked amoebae. Acta Protozool. 51, 209–221. doi: 10.4467/16890027AP.12.017.0763
Anderson, O. R. (2014a). Bacterial and heterotrophic nanoflagellate densities and C-biomass estimates along an Alaskan tundra transect with prediction of respiratory CO2 efflux. J. Eukaryot. Microbiol. 61, 11–16. doi: 10.1111/jeu.12081
Anderson, O. R. (2014b). “The role of soil microbial communities in soil carbon processes and the biogeochemical carbon cycle” in Soil Carbon: Types, Management Practices and Environmental Benefits, ed A. Margit (New York, NY: Nova Publishers), 1–50.
Anderson, O. R., Gorrell, T., Bergen, A., Kruzansky, R., and Levandowsky, M. (2001). Naked amoebas and bacteria in an oil-impacted salt marsh community. Microb. Ecol. 42, 474–481. doi: 10.1007/s00248-001-0008-x
Anderson, O. R., Juhl, A. R., and Bock, N. (2018). Effects of organic carbon enrichment on respiration rates, phosphatase activities, and abundance of heterotrophic bacteria and protists in organic-rich Arctic and mineral-rich temperate soil samples. Polar Biol. 41, 11–24. doi: 10.1007/s00300-017-2166-4
Anderson, O. R., and McGuire, K. (2013). C-biomass of bacteria, fungi, and protozoan communities in arctic tundra soil, including some trophic relationships. Acta Protozool. 52, 217–227. doi: 10.4467/16890027AP.14.002.1439
Apple, J. K., and del Giorgio, P. A. (2007). Organic substrate quality as the link between bacterioplankton carbon demand and growth efficiency in a temperate salt-marsh estuary. ISME J. 1, 729–742. doi: 10.1038/ismej.2007.86
Bloem, J., Albert, C., Bär-Gillissen, M.-J. B., Berman, T., and Cappenberg, T. E. (1989). Nutrient cycling through phytoplankton bacteria and protozoa in selectively filtered Lake Vechten Netherlands water. J. Plankton Res. 11, 119–131.
Boyer, J. N., Dailey, S. K., Gibson, P. J., Rogers, M. T., and Mir-Gonzalez, D. (2006). The role of dissolved organic matter bioavailability in promoting phytoplankton blooms in Florida Bay. Hydrobiologia 569, 71–85. doi: 10.1007/s10750-006-0123-2
Caron, D. A. (1994). Inorganic nutrients, bacteria, and the microbial loop. Microb. Ecol. 28, 295–298.
Caron, D. A., Goldman, J. C., Andersen, O. K., and Dennett, M. R. (1985). Nutrient cycling in a microflagellate food chain II. Population dynamics and carbon cycling. Mar. Ecol. Prog. Ser. 24, 243–254.
Chróst, R. J., Münster, U., Rai, H., Albrecht, D., Witzel, P. K., and Overbeck, J. (1989). Photosynthetic production and exoenzymatic degradation of organic matter in the euphotic zone of a eutrophic lake. J. Plankton Res. 11, 223–242.
Chróst, R. J., Waldemar, S., and Halemejko, G. Z. (1984). Longterm studies on alkaline phosphatase activity (APA) in a lake with fish-aquaculture in relation to lake eutrophication and phosphorus cycle. Arch. Hydrobiol. Suppl. 70, 1–32.
Clarholm, M. (1989). Effects of plant-bacterial-amoebal interactions on plant uptake of nitrogen under field conditions. Biol. Fertil. Soils 8, 373–378.
Cole, C. V., Elliottt, E. T., Hunt, H. W., and Coleman, D. C. (1977). Trophic interactions in soils as they affect energy and nutrient dynamics. V. Phosphorus transformations. Microbial. Ecol. 4, 381–387.
Ducklow, H. (2000). “Bacterial production and biomass in the oceans.” in Microbial Ecology of the Oceans, ed D. L. Kirchman (Wilmington, DE: Wiley-Liss, Inc.), 85–120.
Duhamel, S., Björkman, K. M., Doggett, J. K., and Karl, D. M. (2014). Microbial response to enhanced phosphorus cycling in the North Pacific Subtropical Gyre. Mar. Ecol. Prog. Ser. 504, 43–58. doi: 10.3354/meps10757
Duhamel, S., and Moutin, T. (2009). Carbon and phosphate incorporation rates of microbial assemblages in contrasting environments in the Southeast Pacific. Mar. Ecol. Prog. Ser. 375, 53–64. doi: 10.3354/meps07765
Dyhrman, S. T., Ammerman, J. W., and Van Mooy, B. A. S. (2007). Microbes and the marine phosphorus cycle. Oceanography 20, 110–116. doi: 10.5670/oceanog.2007.54
Esser, G., Kattge, J., and Sakalli, A. (2011). Feedback of carbon and nitrogen cycles enhances carbon sequestration in the terrestrial biosphere. Glob. Change Biol. 17, 819–842. doi: 10.1111/j.1365-2486.2010.02261.x
Ferrier-Pagès, C., and Rassoulzadegan, F. (1994). Seasonal impact of the microzooplankton on pico- and nanoplankton growth rates in the northwest Mediterranean Sea. Mar. Ecol Prog. Ser. 108, 283–294.
Fogg, G. E. (1971). Extracellular products of algae in freshwater. Arch. Hydrobiol, Ergebn. Limnol. 5, 1–25.
Frost, P. C., and Elser, J. J. (2002). Effects of light and nutrients on the net accumulation and elemental composition of epilithon in boreal lakes. Freshw. Biol. 47, 173–183. doi: 10.1046/j.1365-2427.2002.00796.x
Gibson, C. E., Foy, R. H., and Bailey-Watts, A. E. (1996). An analysis of the total phosphorus cycle in some temperate lakes: the response to enrichment. Freshw. Biol. 35, 525–532. doi: 10.1111/j.1365-2427.1996.tb01766.x
Giepmans, B. N., Adams, S. R., Ellisman, M. H., and Tsien, R. Y. (2006). The fluorescent toolbox for assessing protein location and function. Science 312, 217–224. doi: 10.1126/science.1124618
Güde, H. (1985). Influence of phagotrophic processes on the regeneration of nutrients in two-stage continuous culture systems. Microbial. Ecol. 11, 193–204.
Hadas, O., Pinkas, R., and Wynne, D. (1992). Nitrate reductase activity, ammonium regeneration, and orthophosphate uptake in protozoa isolated from Lake Kinneret, Israel. Microbial. Ecol. 23, 107–115.
Hanson, R. B., and Snyder, J. (1980). Glucose exchanges in a salt marsh estuary: biological activity and chemical measurements. Limnol. Oceanogr. 25, 633–642.
Mark, G. L., Dow, J. M., Kiely, P. D., Higgins, H., Haynes, J., Baysse, C., et al. (2005). Transcriptome profiling of bacterial responses to root exudates identifies genes involved in microbe-plant interactions. Proc. Natl. Acad. Sci. U.S.A 102, 17454–17459. doi: 10.1073/pnas.0506407102
Massana, R., Pedrós-Alió, C., Casamayor, E. O., and Gasol, J. M. (2001). Changes in marine bacterioplankton phylogenetic composition during incubations designed to measure biogeochemically significant parameters. Limnol. Oceanogr. 46, 1181–1188. doi: 10.4319/lo.2001.46.5.1181
Meon, B., and Amon, R. M. W. (2004). Heterotrophic bacterial activity and fluxes of dissolved free amino acids and glucose in the Arctic rivers Ob, Yenisei and the adjacent Kara Sea. Aquat. Microb. Ecol. 37, 121–135. doi: 10.3354/ame037121
Moshiri, G. A., Crumpton, W. G., and Aumen, N. G. (1979). Dissolved glucose in a bayou estuary, possible sources and utilization by bacteria. Hydrobiologia 62, 71–74.
Pelegri, S. P., Dolan, J., and Rassoulzadegan, F. (1999). Use of high temperature catalytic oxidation (HTCO) to measure carbon content of microorganisms. Aquat. Microb. Ecol. 16, 273–280.
Pérez, M. T., and Sommaruga, R. (2006). Differential effect of algal- and soil-derived dissolved organic matter on alpine lake bacterial community composition and activity. Limnol. Oceanogr. 51, 2527–2537. doi: 10.4319/lo.2006.51.6.2527
Popendorf, K. J., and Duhamel, S. (2015). Variable phosphorus uptake rates and application across microbial groups in the oligotrophic Gulf of Mexico. Environ. Microbiol. 17, 3992–4006. doi: 10.1111/1462-2920.12932
Ruttenberg, K. C., and Dyhrman, S. T. (2012). Dissolved organic phosphorus production during simulated phytoplankton blooms in a coastal upwelling system. Front. Microbiol. 3:274. doi: 10.3389/fmicb.2012.00274
Selph, K. E., Landry, M. R., and Laws, E. A. (2003). Heterotrophic nanoflagellate enhancement of bacterial growth through nutrient remineralization in chemostat culture. Aquat. Microb. Ecol. 32, 23–37. doi: 10.3354/ame032023
Shiah, F.-K., and Ducklow, H. W. (1994). Temperature and substrate regulation of bacterial abundance, production and specific growth rate in Chesapeake Bay, USA. Mar. Ecol. Prog. Ser. 103, 297–308.
Stewart, A. J., and Wetzel, R. G. (1982). Influence of dissolved humic materials on carbon assimilation and alkaline phosphatase activity in natural algal-bacterial assemblages. Freshw. Biol. 12, 369–380.
Valliéres, C., Retamal, L., Ramlal, P., Osburn, C. L., and Vincent, W. F. (2008). Bacterial production and microbial food web structure in a large arctic river and the coastal Arctic Ocean. J. Marine Syst. 74, 756–773. doi: 10.1016/j.jmarsys.2007.12.002
White, A. E., Watkins-Brandt, K. S., Engle, M. A., Burkhardt, B., and Paytan, A. (2012). Characterization of the rate and temperature sensitivities of bacterial remineralization of dissolved organic phosphorus compounds by natural populations. Front. Microbiol. 3:276. doi: 10.3389/fmicb.2012.00276
Keywords: aquatic alkaline phosphatase, bacterial C biomass, biogeochemical cycles, heterotrophic nanoflagellate C biomass, microbial food webs, phosphorus biomineralization, planktonic microbial communities
Citation: Anderson OR (2018) Evidence for Coupling of the Carbon and Phosphorus Biogeochemical Cycles in Freshwater Microbial Communities. Front. Mar. Sci. 5:20. doi: 10.3389/fmars.2018.00020
Received: 11 November 2017; Accepted: 16 January 2018;
Published: 05 February 2018.
Edited by:
Christian Lønborg, Australian Institute of Marine Science, AustraliaReviewed by:
L. Antonio Cuevas, EULA-Chile, Universidad de Concepcion, ChileRenata Zaccone, Istituto per l'Ambiente Marino Costiero (CNR), Italy
Casey Michael Godwin, University of Michigan, United States
Copyright © 2018 Anderson. This is an open-access article distributed under the terms of the Creative Commons Attribution License (CC BY). The use, distribution or reproduction in other forums is permitted, provided the original author(s) and the copyright owner are credited and that the original publication in this journal is cited, in accordance with accepted academic practice. No use, distribution or reproduction is permitted which does not comply with these terms.
*Correspondence: O. Roger Anderson, b3JhQGxkZW8uY29sdW1iaWEuZWR1