- 1Department of Biology, University of Massachusetts Boston, Boston, MA, United States
- 2Department of Biology, Baylor University, Waco, TX, United States
- 3Anderson Cabot Center for Ocean Life, New England Aquarium, Boston, MA, United States
- 4Department of Biology, Woods Hole Oceanographic Institution, Woods Hole, MA, United States
North Atlantic right whales (Eubalaena glacialis) are highly endangered and frequently exposed to a myriad of human activities and stressors in their industrialized habitat. Entanglements in fixed fishing gear represent a particularly pervasive and often drawn-out source of anthropogenic morbidity and mortality to the species. To better understand both the physiological response to entanglement, and to determine fundamental parameters such as acquisition, duration, and severity of entanglement, we measured a suite of biogeochemical markers in the baleen of an adult female that died from a well-documented chronic entanglement in 2005 (whale Eg2301). Steroid hormones (cortisol, corticosterone, estradiol, and progesterone), thyroid hormones (triiodothyronine (T3) and thyroxine (T4)), and stable isotopes (δ13C and δ15N) were all measured in a longitudinally sampled baleen plate. This yielded an 8-year profile of foraging and migration behavior, stress response, and reproduction. Stable isotopes cycled in annual patterns that reflect the animal's north-south migration behavior and seasonally abundant zooplankton diet. A progesterone peak, lasting approximately 23 months, was associated with the single known calving event (in 2002) for this female. Estradiol, cortisol, corticosterone, T3, and T4 were also elevated, although variably so, during the progesterone peak. This whale was initially sighted with a fishing gear entanglement in September 2004, but the hormone panel suggests that the animal first interacted with the gear as early as June 2004. Elevated δ15N, T3, and T4 indicate that Eg2301 potentially experienced increased energy expenditure, significant lipid catabolism, and thermal stress approximately 3 months before the initial sighting with fishing gear. All hormones in the panel (except cortisol) were elevated above baseline by September 2004. This novel study illustrates the value of using baleen to reconstruct recent temporal profiles and as a comparative matrix in which key physiological indicators of individual whales can be used to understand the impacts of anthropogenic activity on threatened whale populations.
Introduction
North Atlantic right whales (Eubalaena glacialis Müller 1776) are highly endangered mysticete cetaceans that range in the industrialized coastal waters of the eastern United States and Canada (Kraus and Rolland, 2007; Thomas et al., 2016). Individuals are identifiable by unique patches of cornified skin on the rostrum and bonnet, which become infested by cyamid whale lice and are highly visible against a whale's black skin (Kraus et al., 1986; Figure 1A). Since the early 1980s, the New England Aquarium (Boston, MA, U.S.) has curated a photo-identification and sightings catalog for monitoring habitat use, migratory phenology, health, calving rates, and survivorship of right whales (Hamilton et al., 2007)—making them one of the most well studied wild populations on the planet. Despite significant conservation efforts, there are approximately 450 individual right whales remaining, with a trajectory of population decline (Kraus et al., 2016; Pace et al., 2017; Pettis et al., 2017a).
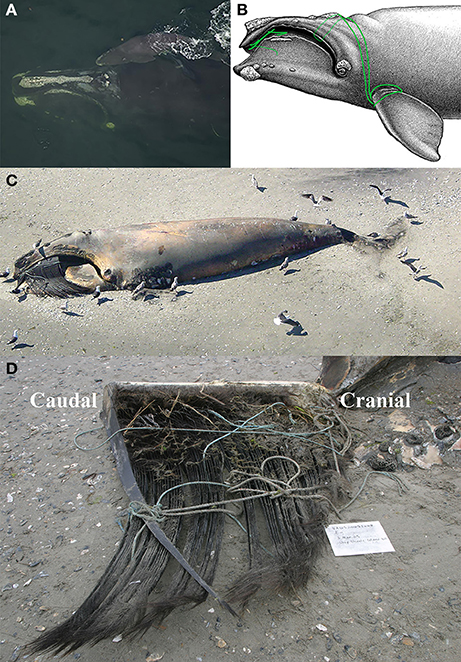
Figure 1. Field photographs and schematic of Eg2301 (A) with calf (Eg3310) in 2002, photograph: New England Aquarium under NOAA permit 14233; (B) with detail of fishing gear entanglement, illustration: Scott Landry, Center for Coastal Studies; (C) post-mortem in 2005, with portion of left baleen rack visible, photograph: U.S. Coast Guard; (D) left baleen rack, reflected to reveal the lingual baleen surface and entangling lines, photograph: Virginia Aquarium Stranding Program under NOAA permit 932-1905/MA-009526.
In recent years, fixed fishing gear entanglements have increased in frequency and severity to become the primary source of serious injury (i.e., injuries that do not result in an immediate mortality but will likely result in subsequent mortality, Moore et al., 2013) and anthropogenic mortality to right whales (Knowlton et al., 2012, 2016; van der Hoop et al., 2013; Hayes et al., 2017). Fixed fishing gear1 (herein referred to as “gear”) is stationary, anchored at least at one end and can include gillnets, long lines, pots, traps, and vertical lines and buoys. It is estimated that 12–16% of the right whale population becomes entangled in gear each year, with approximately 83% of the population showing evidence of at least one entanglement (Knowlton et al., 2012). Entanglements can become chronic when large whales survive an acute gear entanglement and carry some or all of the gear away with them, often resulting in death within 6–12 months after first detection in the field (Moore et al., 2006; Cassoff et al., 2011). These entanglements are often difficult to assign to a particular fishery or geographic location, or to track in real-time. Chronic entanglements have a variety of sub-lethal consequences including: serious [likely painful] injury (Knowlton and Kraus, 2001; Moore et al., 2005; Moore and van der Hoop, 2012; Moore, 2014), loss of body condition—from increased energetic demands due to the additional drag of attached gear and/or impaired feeding (van der Hoop et al., 2015, 2016, 2017), or compromised health and reduced fecundity (Schick et al., 2013; Robbins et al., 2015; Rolland et al., 2016; Pettis et al., 2017b). Longitudinal studies of individuals impacted by gear entanglements are especially important to ascertain the effect(s) and interactions of these potential consequences.
A retrospective biogeochemical approach can provide critical insight regarding how entanglements affect large whales, such as their impact on foraging success, migration behavior, or stress physiology during an interaction with gear. Here, we measured a panel of six hormones and stable isotopes in the baleen tissue of a 12-year old, reproductively active female right whale (catalog number Eg2301) that died from a well-documented chronic gear entanglement. Eg2301 was first seen with attached gear in September 2004, but the extent of the associated injuries suggested that she had been carrying the gear for weeks/months prior to this field sighting. The aim of this study was to validate a novel endocrine method on a well-studied wild cetacean by (i) describing the hormone and stable isotope profiles of Eg2301 during known stressors that were both anthropogenic (i.e., a chronic gear entanglement) and natural (i.e., a calving event); and (ii) approximating the time and location of Eg2301's entanglement acquisition to provide better information for future mitigation of whale-fishery interactions.
Baleen, a series of metabolically inert keratin plates in the upper jaw that comprise the filter-feeding apparatus of mysticetes, is an ideal tissue for recent retrospective, longitudinal analysis due to its growth—which is assumed to occur continuously, year round (St. Aubin et al., 1984; Werth and Potvin, 2016; Figures 1C,D). In balaenid species like right whales, adults can grow baleen plates upwards of 8 feet in length, representing approximately 10 years of tissue for retrospective analysis. Similar to mammalian hair, baleen is a cornified tissue that contains circulating endocrine compounds that have been deposited during its growth (Bryan et al., 2013; Ullmann et al., 2016; Cattet et al., 2017; Hunt et al., 2017b). For this study, we analyzed: two adrenal glucocorticoid steroids—cortisol and corticosterone—as elevations of these two hormones indicate the initiation of the vertebrate stress response via the hypothalamic-pituitary-adrenal (HPA) axis (Norris, 2006; Romero and Wingfield, 2015). Thyroid hormones—triiodothyronine (T3) and thyroxine (T4)—were analyzed as biomarkers of foraging success since these hormones are regulators of metabolic rate in mammals, and thyroid hormone status correlates with energy expenditure and body condition (reviewed in Mullur et al., 2014; McAninch and Bianco, 2015). Female gonadal steroids—progesterone and estrogen—were analyzed as indicators of pregnancy and potentially, estrous (Rolland et al., 2005; Kellar et al., 2013; Hunt et al., 2016a; Burgess et al., in press).
Additionally, we analyzed carbon (δ13C) and nitrogen (δ15N) stable isotopes in baleen, as they are well-established trophic markers of seasonal diet and foraging location in large whales (Schell and Saupe, 1993; Best and Schell, 1996; Lee et al., 2005; Hobson, 2007; Lysiak, 2008; Newsome et al., 2010; Matthews and Ferguson, 2015; Busquets-Vass et al., 2017). Animals acquire their stable isotope signatures from their diet, with predictable enrichment of both δ13C and δ15N at each trophic level (Kelly, 2000). Lysiak (2008) documented annual δ13C and δ15N oscillations in right whale baleen, which were attributed to a whale's foraging on zooplankton with disparate stable isotope signatures during annual migrations through their seasonal feeding habitats. In recent studies, stable isotopes have been used in conjunction with baleen steroid hormone analysis to establish a timeline of tissue growth—which greatly enhances the resolution at which longitudinal hormone concentrations may be interpreted (e.g., biological validations by Hunt et al., 2014, 2016a, 2017a).
Materials and Methods
Study Animal
Field observations and sighting records for whale Eg2301 were obtained from the North Atlantic Right Whale Catalog (http://rwcatalog.neaq.org/; NARWC, 2006a). Eg2301 was born in 1993, and was photographed annually in at least one of the known right whale seasonal habitats in the Gulf of Maine or southeast US (see Supplementary Material). This female whale was first observed with a calf on December 31, 2002 in the southeast US calving ground (Figure 1A), and sightings with that calf continued as she migrated northward through Gulf of Maine feeding habitats in 2003. She was last seen with her calf on September 18, 2003 in the Bay of Fundy (New Brunswick, Canada). Eg2301 was not documented again until September 6, 2004, when she was sighted on Roseway Basin (Nova Scotia, Canada) with an extensive gear entanglement, which involved rope wrapped around the left pectoral flipper and cutting across and through the blowhole, and an extensive entanglement in the mouth and baleen (Figures 1B–D). Eg2301 was last seen alive on December 8, 2004, off the North Carolina, U.S. coast. The carcass of Eg2301 was discovered on March 3, 2005 on a barrier island off the Virginia, U.S. coast (Figure 1C), approximately 6 months after the entanglement was first detected in the field. Given these observational records, Eg2301 could have been entangled for a minimum of 178 days and maximum of 532 days. A necropsy indicated a serious injury to the left pectoral flipper (a deep v-shaped laceration in the soft tissue with extensive periosteal fibro-osseous proliferation around the humerus bone), partial occlusion of the left blowhole, severe dermal abrasions, and emaciated body condition (NARWC, 2006b; Cassoff et al., 2011). A single, full-length baleen plate (with associated gingiva) was collected from the carcass for this study and stored at −20°C until analysis. Entangling rope was also collected from the carcass, but it could not be attributed to a particular fishery or location.
Sample Preparation
The baleen plate from Eg2301 was scrubbed with a plastic bristle brush and a mild shampoo [Herbal Essences] to remove sand and oils. Gingiva were flensed away to expose the unerupted base of the baleen plate. After drying at room temperature, the plate was wiped three times with 95% ethanol. We placed laboratory tape down the midline of the baleen plate, where 2 cm increments were marked for sampling, with “0” starting at the end of the wide, proximal base of the plate (i.e., the attachment point to upper jaw and newest baleen growth). To obtain higher temporal resolution of hormone data in the final year of the animal's life, we sampled the baleen plate at 1 cm intervals between 0 and 24 cm of baleen length. At each sampling point, baleen was ground into a powder using a Dremel rotary tool fitted with a tungsten carbide bit. All tools, surfaces, gloved hands, and the baleen plate were wiped with ethanol between each sampling bout to prevent cross-contamination. Given the limited amount of tissue at the distal tip of the baleen plate, hormone analysis (which requires significantly more tissue biomass—100 mg for hormone analysis vs. 1 mg for isotope analysis) was collected from 0 to 158 cm of baleen, while stable isotopes measurements were collected from the full length of the specimen (0–214 cm).
Stable Isotope Analysis
Baleen powder aliquots (1.0 ± 0.2 mg) were packaged into 4 x 6 mm tin capsules in duplicate for δ13C and δ15N analysis, using a PDZ Europa ANCA-GSL elemental analyzer interfaced with a PDZ Europa 20-20 isotope ratio mass spectrometer (Sercon Ltd., Cheshire, UK) at the University of California Davis Stable Isotope Facility. Baleen stable isotope values are reported in delta notation (δ, in parts per thousand), as the ratio of an unknown sample to an international standard (Vienna Pee-Dee Belemnite limestone for δ13C and atmospheric N2 for δ15N):
where R is a heavy-to-light isotope ratio, 13C/12C or 15N/14N. Values were normalized using reference materials with an isotopic composition that spanned that of the sample range (i.e., bovine liver, glutamic acid, and nylon 5; δ13C range: −27.72 to 37.626%0, δ15N range: −10.31 to 47.6%0) and were calibrated to NIST Standard Reference Materials (IAEA-N1, IAEA-N2, IAEA-N3, USGS-40, and USGS-41). Analytical precision, 0.05%0 for δ13C and 0.20%0 for δ15N, is based on the standard deviation of a repeated internal laboratory standard (glycine). Reference samples and standards were analyzed after every 12 baleen samples.
Data Analysis
Autoregressive (AR) models and spectral analysis were used to characterize variation and seasonality in the baleen δ13C and δ15N profiles. Linear trends were removed from each isotope time series before they were fit to high-order autoregressive (AR(p)) models, with model order p selected based on minimum Akaike information criteria (AIC). The spectral peak frequency was converted to samples per period (1/peak frequency) and then multiplied by sample interval (2 cm) to estimate the period length of each times series (after Matthews and Ferguson, 2015). All statistical analysis were performed using JMP 13.
Similar to other balaenid species, North Atlantic right whales exhibit asymptotic baleen (and body) growth, where annual tissue growth decreases with maturation (Best and Schell, 1996; Lubetkin et al., 2008; Lysiak, 2008; George et al., 2016). Given that Eg2301 was a 12-year-old whale, we expect the baleen plate to contain tissue that grew at variable annual rates across the approximately 8-year profile, such that a single estimate of the period of each isotope time series may not be the best characterization of a potentially dynamic annual growth rate. To characterize inter-annual variation in baleen growth rate, we estimated the period of each annual isotope cycle by counting the number of inclusive data points (i.e., isotope maxima to subsequent maxima) on the detrended data.
We used the stable isotope profiles to establish a timeline that indicates the calendar year of deposition for each baleen sample. The timeline begins with sample 0, which was given a date of February 2005 based on observations at necropsy, suggesting that Eg2301 was dead 1–2 weeks prior to the location of the carcass in early March 2005 (NARWC, 2006). We then worked backwards, using the estimated periods of each isotope cycle obtained from counting the number of data points per oscillation, to assign January of each previous calendar year on the baleen profiles. The field sighting record for Eg2301, which indicated migration behavior and potential times of residency in particular seasonal habitats, also informed this timeline. Protracted feeding in one area may manifest itself as a series of near-identical points in a baleen isotope record, as the whale is theoretically ingesting prey of consistent isotope signature during its residency. We noted instances of repeated sightings of Eg2301 in a particular habitat (in the same season and year) and cross-referenced these to the baleen timeline to determine if field observations matched temporally with sections of samples with similar isotope values. The sighting record also indicates that Eg2301 was first seen in the field with a new calf on December 31, 2002. This event provides an additional opportunity to ground-truth the timeline, as samples from this time period should be characterized by a precipitous decline in baleen progesterone concentration (see Hunt et al., 2016a).
Hormone Extraction
Following the extraction protocol by Hunt et al. (2014), 100 mg of baleen powder was combined with 4.0 ml of 100% methanol in a borosilicate glass tube, vortexed for 20 h at room temperature, and centrifuged for 15 min at 4,000 g. The resulting supernatant was transferred to a clean glass tube and dried down at 45°C under nitrogen in a dry-block sample evaporator. Samples were reconstituted in 1.0 ml assay buffer (catalog #X065; Arbor Assays, MI, USA) vortexed well, transferred to a cryovial, and frozen at −20°C until analysis.
Hormone Assays
Commercial enzyme immunoassay kits from Arbor Assays were used to analyze baleen progesterone (catalog #K025), 17β-estradiol (catalog #K030), cortisol (catalog #K003), corticosterone (catalog #K014), T3 (catalog #K056), and T4 (catalog #K050). Each of these assay kits has previously been biochemically validated for hormone analysis of North Atlantic right whale baleen (Hunt et al., 2016a, 2017a,b). An extensive laboratory validation study by Hunt et al. (2017b) demonstrated that all six assay antibodies exhibited reliable binding affinity to the desired hormone in right whale baleen (i.e., good parallelism; slopes of the linear portions of the binding curves of serially diluted samples and standards are not significantly different), and verified that each assay was able to distinguish a range of concentrations with acceptable mathematical accuracy (i.e., good accuracy; linear regressions of known standard dose vs. observed dose are within r2 > 0.95 and slope = 0.7–1.3).
The manufacturer's protocols were followed for analysis of all six hormones. Samples, standards, non-specific binding, and blank wells were assayed in duplicate. Any samples that fell outside 10–90% bound on the standard curve were re-assayed; samples with high hormone concentrations (percent bound < 10%) were diluted 2-fold (1:2, or up to 1:256 in some high progesterone samples) while samples with low hormone concentrations (percent bound > 90%) were concentrated within the assay wells at 2:1. Samples with > 10% coefficient of variance between duplicates were re-assayed. Results were converted to nanograms of immunoreactive hormone per gram of baleen. Baseline hormone concentrations were determined for each dataset using an iterative process excluding all points that deviate from the mean + 2 SD until no points exceed this threshold (after Brown et al., 1988). Peaks in hormone values were defined as points exceeding the overall baseline concentration + 2 SD. The corticosterone (compound B) to cortisol (compound F) ratio (i.e., B/F ratio) was calculated for all hormone samples.
Results
Stable Isotope Timeline
The Eg2301 baleen δ13C and δ15N profiles contained regular oscillations, which are hypothesized to be annual signals (Schell and Saupe, 1993; Best and Schell, 1996; Lee et al., 2005; Hobson, 2007; Newsome et al., 2010; Matthews and Ferguson, 2015; Figure 2A). Similar to another study of North Atlantic right whale baleen isotopes (Lysiak, 2008), we observed δ13C maxima in the boreal fall/winter while δ13C minima occurred in the spring. δ15N profiles were slightly out of phase with δ13C, with δ15N maxima occurring in summer/fall and minima occurring in winter (Figure 2A). Spectra from first-order autoregressive models (AR(1)) fit to the stable isotope data estimated periods of 32.4 cm (δ13C) and 29.3 cm (δ15N). The periods of each individual isotope oscillation, representing annual baleen growth rates, were estimated by counting the number of data points in each isotope cycle. Across the baleen isotope records, we observed a range of 12–17 data points per cycle ( = 15.28 ± 1.70 points, Figure 2B). This is equivalent to a mean annual growth rate of 30.56 cm yr−1. Eg2301 shows evidence of decreasing baleen growth rate, especially in the most recent years of the isotope record (i.e., 2002–2004, Figure 2) when the baleen growth rate declines to 24 cm yr−1, which is a stable growth rate reported for other adult female North Atlantic right whales (Lysiak, 2008; Hunt et al., 2016a).
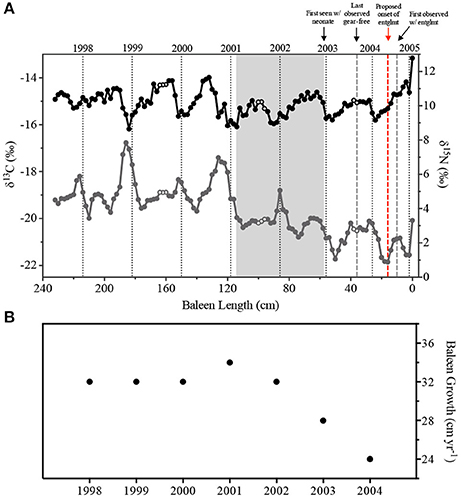
Figure 2. (A) Carbon (δ13C, gray circles) and nitrogen (δ15N, black circles) stable isotope ratios in Eg2301 baleen. Open circles represent samples matched to repeated field sightings in the Bay of Fundy (New Brunswick, Canada) in 1999 and 2001 (whale seen in July, August, and September) and 2003 (whale, with calf, seen in August and September). Vertical dotted black lines represent estimates for calendar year of baleen growth. Gray box indicates the duration of the progesterone peak associated with the single known pregnancy for Eg2301. Vertical dashed gray lines represent the minimum and maximum bounds of entanglement duration; vertical red dashed line is the revised estimate of when the entanglement was acquired. (B) Annual baleen growth rate (cm yr−1).
The two methods used to determine the period of each isotope profile, modeling and counting data points, provided similar results (period = 32.4 cm yr−1 (δ13C, via modeling), 30.56 cm yr−1 (mean annual growth rate, via counting points), or 29.3 cm yr−1 (δ15N, via modeling). Since we observed a decrease in the period of each isotope cycle across the profile (Figure 2B), we used the annual periods estimated by the counting method to build a tissue growth timeline that would best reflect the dynamic nature of the annual baleen growth rate. There were three instances of repeated field sightings of Eg2301 within a single habitat area, in the same season and same year. Eg2301 was seen in the Bay of Fundy (New Brunswick, Canada) in July/August/September of 1999 and 2001, and in August/September of 2003 (NARWC, 2006a). When these field sightings were cross-referenced with the sample timeline, we observed sections of baleen with very similar δ13C and δ15N values (open circles in Figure 2A), which suggest Eg2301's protracted feeding on zooplankton with a consistent isotopic signature during these times. The date of first sighting with a new calf (December 31, 2002) coincides with baleen samples with declining progesterone concentrations, occurring just after a sustained peak associated with gestation (see section Discussion). The sighting record of Eg2301 provides important validation for the tissue growth timeline. Given the good alignment of the timeline with these known life history and migratory events, we suggest that an error of ±1 data point (approximately 21.5–30 days) is a reasonable estimate of uncertainty for the dates of deposition assigned to each sample.
Hormone Panel
In the early years of the baleen hormone profiles for Eg2301 (i.e., between 1999 and 2001), nearly all samples fluctuate at or below baseline and corticosterone:cortisol (B/F Ratio) ratios are <1.0 (indicating that immunoreactive cortisol was measured at higher concentrations than immunoreactive corticosterone; Figure 3). A protracted progesterone peak, two orders of magnitude above baseline, was observed from early 2001 to late 2002 ( = 199.48 ng g−1, baseline = 2.249 ng g−1; Figure 3A). In 2001, the increase in progesterone coincided with three discrete estradiol spikes (Figure 3A), high cortisol ( = 9.02 ng g−1, baseline = 1.967 ng g−1) and elevated corticosterone (baseline = 4.663 ng g−1; Figure 3B), B/F ratios predominantly <1.0 (Figure 3C), and elevated but variable T3 (baseline = 0.719 ng g−1) and T4 (baseline = 0.376 ng g−1; Figure 3D). By contrast in 2002, the progesterone peak coincided with a peak in estradiol ( = 6.91 ng g−1, baseline = 2.79 ng g−1; Figure 3A), low cortisol and elevated corticosterone (Figure 3B), B/F ratios >1.0 (Figure 3C), and variable fluctuations in T4 (early 2002) and T3 (late 2002) (Figure 3D). In 2003, we observed one discrete, concomitant increase in progesterone and estradiol (Figure 3A) and several instances of elevated T3 (Figure 3D).
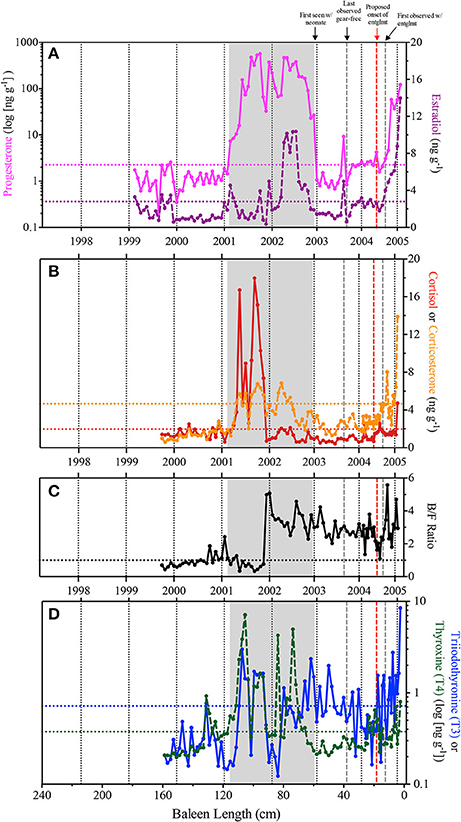
Figure 3. Observed immunoreactive hormone concentrations across the Eg2301 baleen plate. Vertical dotted black lines represent estimates for calendar year of baleen growth, as determined from stable isotope profiles. Horizontal dotted lines indicate baseline hormone levels. Gray box indicates the duration of the progesterone peak associated with the single known pregnancy for Eg2301. Vertical dashed gray lines represent the minimum and maximum bounds of entanglement duration; vertical red dashed line is the revised estimate of when the entanglement was acquired. (A) Progesterone (pink solid line—note logarithmic scale, baseline = 2.249 ng g−1) and estradiol (purple dashed line, baseline = 2.79 ng g−1); (B) Cortisol (red solid line, baseline = 1.967 ng g−1) and corticosterone (orange dashed line = 4.663 ng g−1); (C) Corticosterone:cortisol ratio (B/F Ratio), horizontal dashed line at y = 1.0 indicates equal concentrations of both hormones; (D) triiodothyronine (T3–blue solid line—note logarithmic scale, baseline = 0.719 ng g−1) and thyroxine (T4–green dashed line—note logarithmic scale, baseline = 0.376 ng g−1).
At death, in February 2005, all six hormones measured during this study were elevated above baseline (Figure 3). T3 and T4 showed variable fluctuations above baseline throughout the baleen record, but persistently increased in concentration beginning in June/July 2004 with the highest recorded concentration of T3 being the last data point on February 2005 (8.48 ng g−1; Figure 3D). By August/September 2004, corticosterone showed persistent elevations, increasing to the highest recorded concentration (13.89 ng g−1, 3-fold above baseline) by the end of the baleen record (Figure 3B). By September 2004, progesterone and estradiol rose continuously above baseline (Figure 3A). We observed three discrete spikes of high cortisol during 2004–2005, the highest concentration (4.72 ng g−1 and approximately 2-fold above baseline) occurred at the end of the hormone record (Figure 3B).
Discussion
Baleen hormone and stable isotope profiles (Figures 2, 3) showed correspondence with documented life history events for this reproductively mature, chronically entangled female right whale. A period of gestation was characterized by high baleen progesterone concentrations and contained two distinct phases, each lasting approximately 1 year: (1) low estradiol, high corticosterone-cortisol in 2001 and (2) high estradiol-corticosterone, low cortisol in 2002 (Figure 3). The end of gestation (i.e., parturition and a return to baseline progesterone values) coincides with the timing of the first field sightings of Eg2301 with a calf, on December 31, 2002. Thyroid hormones (T3 and T4) are periodically elevated across the baleen record and point to periods of short- and long-term food limitation, associated thermal stress, and increased energy expenditure. Finally, the hormone panel shows evidence of a stress response in 2004, during a period when Eg2301 was documented in the field with a chronic gear entanglement.
Gestation and Estrous
Gestation Timeline
Progesterone, as detected in several balaenid whale tissue matrices (e.g., feces, Rolland et al., 2005, 2012, 2017; blubber, Kellar et al., 2013; respiratory vapor, Burgess et al., in review; and baleen, Hunt et al., 2016a), is a robust indicator of pregnancy. Longitudinal, physiological-based estimates of mysticete gestation period, as provided by this study, are extremely limited. The protracted progesterone peak observed in the Eg2301 baleen record contains 29 data points that, according to our date of growth timeline, are equivalent to approximately 696 days (23 months). These results could indicate that North Atlantic right whale gestation is significantly longer than previously reported in other balaenids.
Based on field observations, stranding data, and whaling records, Best (1994) estimated a gestation period of 357–396 days (12–13 months) for southern right whales (Eubalaena australis). Reese et al. (2001) modeled bowhead whale (Balaena mysticetus) average gestation length at 13.9 months (predictive distribution = 12.8–15.0 months), based on observations of the reproductive tract of specimens harvested during subsistence whaling. Estimates for both species are associated with a high degree of uncertainty given the difficulty of observing small embryos within the female reproductive tract during the initial, non-linear phase of fetal growth (Best, 1994; Reese et al., 2001). Using an endocrine approach, Hunt et al. (2016a) conservatively defined gestation as “uninterrupted baleen samples with progesterone >100 ng g−1,” and detected progesterone peaks lasting 540 and 451 days (18 and 15 months, respectively) in two North Atlantic right whales. Though anecdotal, in an endocrine study of North Atlantic right whales, one adult female was sampled in the summer/fall 2004 (fecal progesterone was low) and again in summer/fall 2005 (fecal progesterone was very high), and the whale calved in the winter 2005/2006 calving season (R.M. Rolland, pers. comm., see Rolland et al., 2005). In this case, fecal progesterone was low (i.e., at or below baseline) at approximately 15–17 months prior to parturition, which puts upper bounds on the length of gestation for this particular animal. Taken together, these previous studies highlight the need for further examination of gestation length in this species, with priority to the detection and characterization of the non-linear fetal growth period in early pregnancy.
The temporal mismatch between our observed high progesterone peak and previous estimates of gestation length could potentially be explained by the timeline calculated using stable isotopes. Baleen growth rate varies with age in bowhead (Lubetkin et al., 2008) and right whales (Lysiak, 2008), where calves, juveniles, and sub-adults exhibit faster growth rates than mature adults. Changes in baleen growth rate per year, or inter-annual growth rates, were quantified in this study as the number of data points per isotope oscillation, and if significant confounding changes occurred in baleen growth rate during pregnancy, we should also see the period of annual δ13C and δ15N signals change coincidentally (i.e., the period of δ13C and δ15N oscillations should increase during a slower growth rate scenario, or decrease with a faster growth rate). We did observed inter-annual variation in Eg2301 baleen growth, with longer periods seen in older sections of baleen (Figure 2). However, during the proposed pregnancy, the 2002 cycle contained 16 data points (baleen growth rate = 32 cm yr−1) and the 2001 cycle contained 17 data points (baleen growth rate = 34 cm yr−1) (Figure 2B), suggesting that baleen growth occurred at a relatively consistent rate for the duration of the progesterone peak.
In this study, as in previous work (Schell and Saupe, 1993; Best and Schell, 1996; Lee et al., 2005; Hobson, 2007; Lysiak, 2008; Newsome et al., 2010; Aguilar et al., 2014; Matthews and Ferguson, 2015; Busquets-Vass et al., 2017), we assume that baleen grows continuously throughout the year (i.e., no intra-annual or seasonal variability in growth). Given the consistent wear patterns in baleen, such as abrasion by the tongue, intra- and extra-oral water flow, and food or sediment particles (Werth et al., 2016), there is strong selective pressure for right whales to consistently maintain their baleen given its integral role in foraging. It is possible that baleen growth rate could change during pregnancy, stress, or food limitation, as variations in the gestational growth rates of keratin-based tissues have been observed in human hair (LeBeau et al., 2011) and cow hooves (Hahn et al., 1986; Mülling et al., 1999). This may add uncertainty and error to our established timeline of baleen tissue growth. While difficult to measure directly in free-ranging cetaceans, future studies should prioritize the investigation of seasonal variability in baleen growth and wear.
Rather than being indicative of one continuous pregnancy, an alternative explanation is that the observed progesterone peak can be divided into two distinct phases: estrous and gestation. In this case, we hypothesize that gestation comprises the second half of the baleen progesterone peak. There is observational evidence for the endpoint of gestation; it ends at parturition in late 2002, when progesterone returns to baseline levels (at baleen length 58) and Eg2301 was first seen in the field with her new calf on December 31 2002; Figures 1A, 3A). However, defining the beginning phase of gestation is more tentative. Cortisol concentrations may be an adequate endocrine biomarker for the transition between estrous and gestation since a very dramatic shift was observed in that hormone between our two proposed phases of the reproductive event (Figures 3B,C). This is similar to observations of captive female Asian elephants, where a protracted cortisol peak preceded a progesterone peak in longitudinal serum samples, and indicated a transition from the follicular phase to the luteal phase (Fanson et al., 2014). Under this scenario, gestation begins in late 2001 when cortisol levels return to baseline and B/F ratios increase to >1.0 (at baleen length 88; Figures 2, 3). This provisional gestation period represents 16 baleen samples, and is equivalent to approximately 384 days (12.8 months), using our timeline of baleen growth. This corresponds well to the Best (1994) estimate of a 12–13 month gestation in southern right whales—a closely related species.
Estrous Cycling
We hypothesize that a period of estrous, a state of sexual receptivity during which a female is capable of conceiving, comprises the first half of the baleen progesterone peak (i.e., early to late 2001, from baleen length 114–90 cm; Figure 3A). During this time, corticosterone, T3, and T4 are variable but primarily elevated above baseline, estradiol is predominantly low, and cortisol is consistently high−9-fold above baseline and at the highest concentrations observed across the entire baleen record (Figure 3). Cortisol (along with other glucocorticoids, GCs) is an index of relative stress (i.e., it indicates activation of the hypothalamic-pituitary-adrenal axis), but also has a role in responding to natural states of increased energetic needs such as migration or reproduction in free-ranging cetaceans (Brann and Mahesh, 1991; Andersen, 2002; Tetsuka, 2007; Rolland et al., 2012; Trumble et al., 2013; Kellar et al., 2015; Hunt et al., 2017a). Under normal physiological conditions, short-term increases in GCs promote sexual receptivity, stimulate gonadotropins (i.e., luteinizing hormone and follicle stimulating hormone), facilitate ovulation, and ameliorate damage from inflammation (reviewed in Fanson et al., 2014). However, reproductive dysfunction or failure can occur under chronic stress and elevated GCs (Tilbrook et al., 2002). Sighting records and the right whale photographic database do not indicate any significant anthropogenic stressors (i.e., visible entangling gear, new entanglement scars, or evidence of a non-lethal vessel strike) or an overall decline in health for Eg2301 during 2001 (NARWC, 2006a; A. Knowlton, pers. comm, after Schick et al., 2013). These observations, and that Eg2301 successfully completed a full-term pregnancy in 2001–2002 suggest that the observed high cortisol values could be a component of the animal's natural reproductive cycle. This result highlights the importance of combining biomarkers of reproduction with those of stress physiology—as it could be tempting to interpret these high GCs as an indicator of a major disturbance or anthropogenic stressor in the absence of collocated progesterone and estrogen concentrations.
Estrous is poorly defined in mysticetes (Boness, 2009). However, odontocete reproductive studies show estrous cycles as spikes in urinary estrogen conjugates closely followed by luteinizing hormone surges, and cycles lasting between 30 and 41 days in Pacific white-sided dolphins (Lagenorhynchus obliquidens), bottlenose dolphins (Tursiops truncatus), and killer whales (Orcinus orca) (Robeck et al., 2004, 2005, 2009). Additionally, Robeck et al. (2004) observed sequential, natural estrous cycles within a season for one individual L. obliquidens, which entailed continuously elevated urinary progesterone and detection of a corpus luteum—with no ultrasound evidence of pregnancy—for 86 days (and 103 days in the following year). In the context of these findings, we recommend expanding the baleen hormone panel to include a suite of progesterone and estrogen conjugates or metabolites. The interactions among these hormones could provide a more detailed assessment regarding the reproductive physiology of right whales. Future studies should consider non-target analysis using high performance lipid chromatography (HPLC) to accommodate a wider range of prospective analytes.
According to the timeline of baleen growth and stable isotopes, Eg2301 was 8 years old during the proposed period of estrous, became pregnant just before age 9, and was 10 years old just after parturition. These observations are in agreement with population-wide estimates for mean age of sexual maturity (9 years) and mean age at first calving (10.1 years; Kraus et al., 2001, 2007). In addition to a period of potential estrous, a failed pregnancy, pseudopregnancy, or delayed implantation may have occurred during this time. Delayed implantation, a temporary diapause of the embryonic blastocyst, is widespread among the mammalian orders Rodentia and Carnivora, but only one known species of Cetartiodactyla (the roe deer, Capreolus capreolus) exhibits this life history strategy (Renfree and Shaw, 2000; Ptak et al., 2012). Previous studies commonly observed low estrogen concentrations during diapause and then implantation was indicated by a surge in circulating estrogens and progesterone in northern fur seals (Callorhinus ursinus, Daniel, 1981), roe deer (Aitken, 1981) and giant pandas (Ailuropoda melanoleuca, Zhang et al., 2009). If right whales undergo the delayed implantation observed in other marine mammals, our hormone panel suggests that active gestation could have begun as early June/July 2001 (when progesterone peaks) or September 2001 (when estradiol surges above baseline after protracted low levels)—meaning that the estimate of gestation period would increase to approximately 16–19 months. Future studies could investigate this further using a broader hormone panel or additional tissue matrices.
Food Limitation
Right whales feed via continuous ram filtration, which is accomplished with a complex mouth anatomy that filters prey-laden water via hydrodynamic pressure differentials across the baleen plates (Werth, 2004). The entanglement of Eg2301 included rope obstructing the mouth, with extensive, knotted wraps through the right and left baleen plates (Figures 1B–D). This gear configuration likely inhibited feeding ability and/or significantly decreased filtration efficiency, potentially leading to a prolonged fasting. As migratory capital breeders with seasonally abundant prey, right whales are well adapted to periods of food limitation, and will catabolize stored energy reserves (e.g., subdermal adipose tissue or blubber) during fasting (Lockyer, 1981). Blubber thickness measurements correspond to a whale's onboard energy balance, and fluctuate with foraging success and reproductive state (Miller et al., 2011; Irvine et al., 2017). At necropsy, the carcass of Eg2301 had extremely thin dorsal blubber (8.5 cm observed, in contrast to 13.4 ± 1.8 cm in non-entangled adult whales; NARWC, 2006b; Miller et al., 2011; van der Hoop et al., 2016). The baleen stable isotope and hormone profiles support these observations, suggesting that Eg2301 experienced severe, prolonged fasting conditions prior to death.
δ15N is an indicator of trophic position in traditional food web studies, since the heavy isotope of nitrogen (15N) is preferentially incorporated into consumer tissues from their diet, which results in a systematic enrichment in nitrogen isotope ratio (15N/14N) with each trophic step (Kelly, 2000). Thus, elevations in δ15N may correlate with dietary shifts to 15N-enriched prey, at higher trophic levels. Fasting conditions can mimic trophic enrichment, since an animal is essentially metabolizing muscle tissue during periods of fasting, thereby causing elevated tissue δ15N (Castellini and Rea, 1992; Hobson et al., 1993). Given their significant adaptations to seasonal fasting and ability to increase lipid stores, baleen whales usually do not display trophic enrichment (Lysiak, 2008; Aguilar et al., 2014; Matthews and Ferguson, 2015) with periodic food limitation and migration. Eg2301 baleen δ15N values exhibit an annual oscillating pattern similar to those observed in other North Atlantic right whales, with the exception of the last few months of the record where δ15N increases to 12.78%0, the highest value recorded in any North Atlantic right whale (Lysiak, 2008). This shift from the regular oscillations could indicate the severe depletion of blubber lipids and a heavier reliance on protein (muscle) catabolism as a means to meet ongoing energetic demands (Fuller et al., 2005; Aguilar et al., 2014).
The thyroid hormones triiodothyronine (T3) and thyroxine (T4) are important regulators of metabolic rate in mammals (Norris, 2006; Mullur et al., 2014; McAninch and Bianco, 2015). During fasting, the hypothalamic-pituitary-thyroid (HPT) axis is depressed, which decreases circulating thyroid hormones, basal metabolic rate, and energy expenditure—which could serve as a survival mechanism (Mullur et al., 2014). Contrary to this paradigm, we observed elevated concentrations of immunoreactive T3 and T4 in the baleen of Eg2301 prior to her death, when we know that her blubber layer became extremely thin and lipid depleted. Lipolysis, fat oxidation, or catabolism of the blubber lipids in fasting marine mammals serves two major roles; mobilizing stored energy (Pond, 1978; Lockyer, 1981) and generating metabolic water (Ortiz et al., 1978; Ortiz, 2001) in the absence of active feeding. Thyroid-promoted lipolysis could be a mechanism to meet osmotic and energetic demands during periods of prolonged nutritional stress. For example, captive West Indian manatees that experienced reduced food intake (i.e., a diet switch from lettuce to sea grass) exhibited increased serum T4 levels and decreased body mass (up to 17%, primarily due to loss of fat; Ortiz et al., 2000).
Blubber is a tissue that also reduces thermal conductance and is a critical adaptation to maintain thermal homeostasis (Dunkin et al., 2005; Samuel and Worthy, 2005)—a constant battle for endothermic homeotherms living in seawater. Loss of blubber thickness can correspond to a concomitant loss of thermal insulation. When under thermal stress, thyroid hormones are secreted to promote adaptive or facultative heat production in brown adipose tissue (BAT) (Silva, 2003; Norris, 2006; Mullur et al., 2014; Santini et al., 2014). In odontocete cetaceans, BAT is located at the innermost blubber layer and enveloping the entire body, exclusive of the thermal windows (Hashimoto et al., 2015). Periods of fasting could represent a negative feedback loop, where thyroid-promoted lipolysis degrades the integument for the sake of water balance and energetic homeostasis, which then necessitates elevated thyroid hormones to promote BAT-mediated thermogenesis.
The thyroid panel of Eg2301 during the entanglement period is also surprising because stress is generally thought to inhibit thyroid secretions (Eales, 1988; Norris, 2006). However, different patterns in thyroid output have been reported for some stressors that increase energetic output (e.g., exercise stress), contrary to what is seen during simple fasting (Uribe et al., 2014; Hunt et al., 2016b). For example, entangled distressed leatherback sea turtles had higher serum T4 than healthy wild individuals, presumably from the added energetic cost of carrying gear (Hunt et al., 2016b). Eg2301 would have likely expended additional energy for locomotion to compensate for the drag associated with the entangling gear (van der Hoop et al., 2013, 2015, 2016), which could be an example of exercise stress promoting thyroid function.
Acute or periodic fasting may be indicated in right whale baleen by isolated and discrete rises in thyroid hormones T3 and T4 (occurring sporadically across the baleen record) and longer-term fasting may be indicated by prolonged T3 and/or T4 elevations (occurring on three occasions: 2001, late 2002-early 2003, and mid 2004–2005; Figure 3D). In 2001, elevated T3 and T4 concentrations in the baleen coincide with the first half of the 2001–2002 progesterone peak (Figure 3). While right whales can travel significant distances, undetected, sighting records for this animal do not indicate a migration to the southeast U.S. in 2001. However, in late 2002, sighting records do indicate Eg2301 migrated approximately 1,500 km from the Gulf of Maine to the southeast US calving ground to give birth and nurse a new calf, with a subsequent return trip several months later (NARWC, 2006a). Interestingly, T3 is elevated during this period, with peaks circa November 2002 and February 2003, when Eg2301 presumably would be traveling during southbound and northbound migrations, respectively (Figure 3D). Finally, T3 elevated above baseline beginning in June 2004, 3 months prior to the detection of the entanglement in the field (Figure 3D). This suggests that Eg2301 experienced significant, chronic fasting leading to loss of blubber, and associated thermal stress over a period of approximately 9 months.
Entanglement
Entanglement Duration and Location
Eg2301 was initially observed with a gear entanglement on September 6, 2004 (minimum entanglement duration = 178 days or 6 months in Figure 3), however the baleen hormone panel suggests that the animal first interacted with gear several months earlier (mid-2004; see red line in Figure 3). Field observations of an extensive mouth/baleen entanglement plus low blubber thickness documented at necropsy support the hypothesis that Eg2301 experienced an extensive period of compromised feeding. In the hormone panel, we observed persistent T3 elevations in baleen beginning in June 2004 (NARWC, 2006b; Figures 1B,C, 3D). By September 2004, all hormones except for cortisol were elevated above baseline (Figure 3). At the September 6, 2004 field sighting (when the entanglement was first discovered), researchers noted that the skin on the left pectoral flipper appeared white underwater—suggesting that the entanglement must have already persisted long enough for the flipper tissue to become necrotic from being tightly wrapped in line (NARWC, 2006b; Figure 1B). By the end of the baleen hormone record (February 2005), cortisol also elevated above baseline and δ15N increased to a level indicating [fasting-induced] trophic enrichment (Figure 2A). Given these observations and our estimation of uncertainty of the baleen timeline, we propose that Eg2301 carried the chronic gear entanglement for a minimum of 9 (±1) months, potentially first encountering the entangling gear as early as June 2004. At that time, there are no field sighting records available for Eg2301 in late spring 2004, so we can only speculate as to her location when becoming entangled. Eg2301's baleen δ13C reaches a minima at this time, which is consistent with depleted δ13C values of zooplankton collected in the Great South Channel (southern Gulf of Maine) in May/June, as well as trends in population-wide migration behavior and baleen isotopes for other individual right whales (Hamilton et al., 2007; Lysiak, 2008). Though Eg2301 was not observed in this area in 2004, she was seen in the Great South Channel habitat in previous years (i.e., April/May/June 1999–2002 and July 2003; NARWC, 2006a). Additional isotopic markers, such as sulfur, oxygen, or deuterium (deHart and Picco, 2015; Matthews and Ferguson, 2015) could provide more spatial resolution in the baleen isotope profile to better assess the location of gear acquisition for this animal.
Stress Physiology
After the carcass of Eg2301 was recovered, researchers confirmed that entangling rope had sliced down to the left humerus bone as well as cut into the skin of the head and blowhole (NARWC, 2006b). These wounds likely caused pain and significant stress (Moore and van der Hoop, 2012). Despite these persistent injuries during the entanglement, we observed paradoxically low cortisol (June 2004—February 2005). This is unexpected since chronic stress, and thus higher cortisol concentrations, should have resulted from this entanglement, as seen in previous studies. Rolland et al. (2017) documented high cortisol in the feces of North Atlantic right whales due to chronic entanglement as well as exposure to noise from vessel traffic (Rolland et al., 2012). We suspect that either baleen is not truly reflective of circulating cortisol levels, or this could indicate insufficient adrenal gland function (i.e., hypothalamic-pituitary-adrenal axis dysfunction or “adrenal fatigue”)—the mechanisms of which are poorly understood in mammals (Edwards et al., 2011). The highest cortisol concentrations in Eg2301 baleen were observed during the proposed estrous cycle, rather than during the entanglement, suggesting that baleen cortisol may not be the best biomarker to use for studying large whale response to chronic anthropogenic stressors (Figure 3B). In contrast, corticosterone was elevated during proposed estrous, gestation, and entanglement (Figure 3B), with the highest values observed in the last 4 months of the baleen record—indicating that this hormone might be more informative when studying stress response in large whales. Furthermore, B/F ratios are consistently >1.0 beginning in 2002 (coinciding with the proposed beginning of gestation), meaning that greater concentrations of immunoreactive corticosterone were detected in these samples than immunoreactive cortisol (Figures 3B,C). Hunt et al. (2017a) noted 4-fold higher corticosterone vs. cortisol concentrations in North Atlantic right whale baleen and suggested that this species might conform to a dual GC signaling model (Koren et al., 2012), where cortisol is a better index of acute stress and corticosterone better reflects chronic stress. Though untested in this study, future hormone panels might also include the adrenal hormone aldosterone as a stress biomarker. Aldosterone was elevated in the feces of pregnant North Atlantic right whales (Burgess et al., 2017) and is detectable in right whale baleen (Hunt et al., 2017b).
In summary, we used elevations of female gonadal steroids progesterone and estradiol to identify a reproductive event in Eg2301's baleen hormone record. Beginning in September 2004, when she was first seen entangled, both of these hormones were again elevated above baseline and continued to rise until the death of Eg2301. This could be physiological evidence of a second estrous cycle or pregnancy. As capital breeders, right whales need to secure sufficient onboard energy stores in their blubber to support gestation and lactation (Irvine et al., 2017). Miller et al. (2011) observed that right whales had the thickest blubber just prior to becoming pregnant. Body condition for Eg2301 was declining during her entanglement, and would likely not meet the energetic threshold required to become pregnant or carry a fetus to term. Due to the remote location of the carcass, an internal examination was not undertaken; therefore the presence or absence of a fetus was not determined. In tandem with the visual decline in body condition during entanglement, baleen T3 and T4 concentrations showed sustained increases potentially indicating significant lipolysis or increased energy expenditure, providing another indicator to rule out a pregnancy event. Elevated progesterone in late 2004 could be attributed to the adrenal glands, which release progesterone (the parent hormone to GCs) in response to stress (reviewed in Herrera et al., 2016). This may contribute to a greater bioavailability of cortisol during the stress response, providing the body with glucose (via gluconeogenesis in the liver) and restoring homeostasis (Kudielka and Kirschbaum, 2005). This physiological response could be considered part of an unsustainable “emergency life-history stage” brought on by entanglement (Wingfield et al., 1998; van der Hoop et al., 2016) in a last ditch attempt to prolong survival. Anecdotally, in 2010–2011, increasing progesterone (R. Rolland, pers. comm.) and very high cortisol (Rolland et al., 2017) were observed in the longitudinal fecal samples of an entangled, juvenile [non-pregnant] female right whale (catalog number Eg3911).
Conclusions
The panel of biogeochemical markers from Eg2301 baleen allowed us to investigate the longitudinal physiological response of a large whale to a chronic gear entanglement in its industrialized ocean habitat. With a panel of adrenal and gonadal steroid hormones, thyroid hormones, and stable isotopes, we were able to establish a timeline of baleen tissue growth and examine the fluctuations of hormones in response to: a calving event, an approximately 3,000 km seasonal migration, prolonged periods of food limitation, and stress associated with entanglement-induced serious injury. These observations support an updated estimate of minimum entanglement duration for Eg2301 from 6 to 9 months, which enhances our understanding of the timeline of this event and provides insight into where it may have occurred. With the biological validation provided in this study, we can apply this method to future forensic studies where the cause of death of a large whale is uncertain or undetermined, or the timeline of events is not known. This novel study illustrates the value of using baleen to reconstruct recent temporal profiles and as a comparative matrix in which key physiological indicators of individual whales can be used to understand the impacts of anthropogenic activity on threatened whale populations.
Author Contributions
NL and ST conceived of the project and secured funding; ST also provided significant laboratory resources. NL is the principal author and responsible for study design, data collection, analysis and interpretation. AK contributed to the North Atlantic right whale field sightings and necropsy databases. MM conducted the necropsy, collected the baleen plate, and provided support and feedback in study design and interpretation. All authors revised this work critically and provide final approval of the version to be published.
Funding
The Woods Hole Oceanographic Institution's Ocean Life Institute and Marine Mammal Center funded this study and NL was supported by a Postdoctoral Fellowship from Baylor University.
Conflict of Interest Statement
The authors declare that the research was conducted in the absence of any commercial or financial relationships that could be construed as a potential conflict of interest.
The reviewer NMK and handling Editor declared their shared affiliation.
Acknowledgments
Right whale baleen was collected under NOAA permit 932-1905-MA-009526. We are indebted to the US Coast Guard, Sue Barco, and the Virginia Aquarium Stranding Program for facilitating sample collection at a challenging, remote necropsy site. This study was conducted through a data-sharing agreement with the North Atlantic Right Whale Consortium; we acknowledge Phillip Hamilton, the New England Aquarium, and the contributions of many researchers and institutions to this invaluable database. Joy Matthews and David Harris of the UC Davis Stable Isotope Facility ensured the meticulous analysis of our samples. Elizabeth Burgess provided critical advice in the study design and carefully reviewed early versions of this manuscript; Rebel Sanders assisted with preliminary hormone analysis. We thank two reviewers for their contributions to this manuscript.
Supplementary Material
The Supplementary Material for this article can be found online at: https://www.frontiersin.org/articles/10.3389/fmars.2018.00168/full#supplementary-material
Footnotes
References
Aguilar, A., Gimenez, J., Gomez–Campos, E., Cardona, L., and Borrell, A. (2014). d15N value does not reflect fasting in mysticetes. PLoS ONE 9:e92288. doi: 10.1371/journal.pone.0092288
Aitken, R. J. (1981). Aspects of delayed implantation in the roe deer (Capreolus capreolus). J. Reprod. Fertil. Suppl. 29, 83–95.
Andersen, C. (2002). Possible new mechanism of cortisol action in female reproductive organs: physiological implications of the free hormone hypothesis. J. Endocrinol. 173, 211–217. doi: 10.1677/joe.0.1730211
Best, P. B. (1994). Seasonality of reproduction and length of gestation in southern right whales, Eubalaena australis. J. Zool. 232, 175–189. doi: 10.1111/j.1469-7998.1994.tb01567.x
Best, P. B., and Schell, D. M. (1996). Stable isotopes in southern right whale (Eubalaena australis) baleen as indicators of seasonal movements, feeding and growth. Mar. Biol. 124, 483–494. doi: 10.1007/BF00351030
Boness, D. J. (2009). “Estrus and estrous behavior,” in Encyclopedia of Marine Mammals, 2nd Edn., eds W. F. Perrin, B. Wursig, and J. G. M. Thewissen (Burlington, MA: Elsevier Academic Press), 392–396.
Brann, D.W., and Mahesh, V. (1991). Role of corticosteroids in female reproduction. FASEB J. 5, 2691–2698. doi: 10.1096/fj.1530-6860
Brown, J. L., Goodrowe, K. L., Simmons, L. G., Armstrong, D. L., and Wildt, D. E. (1988). Evaluation of the pituitary-gonadal response to GnRH and adrenal status in the leopard (Panthera pardus japonensis) and tiger (Panthera tigris). J. Reprod. Fertil. 82, 227–236. doi: 10.1530/jrf.0.0820227
Bryan, H. M., Darimont, C. T., Paquet, P. C., Wynne-Edwards, K. E., and Smits, J. E. G. (2013). Stress and reproductive hormones in grizzly bears reflect nutritional benefits and social consequences of a salmon foraging niche. PLoS ONE 8:e80537. doi: 10.1371/journal.pone.0080537
Burgess, E. A., Hunt, K. E., Kraus, S. D., and Rolland, R. M. (in press). Quantifying hormones in exhaled breath for physiological assessment of large whales at sea. Sci. Rep.
Burgess, E. A., Hunt, K. E., Rolland, R. M., and Kraus, S. D. (2017). Adrenal responses of large whales: integrating fecal aldosterone as a complementary biomarker. Gen. Comp. Endocrinol. 252, 103–110. doi: 10.1016/j.ygcen.2017.07.026
Busquets-Vass, G., Newsome, S. D., Calambokidis, J., Serra-Valente, G., Jacobsen, J. K., Aguíñiga-Garcia, S., et al. (2017). Estimating blue whale skin isotopic incorporation rates and baleen growth rates: implications for assessing diet and movement patterns in mysticetes. PLoS ONE 12:e0177880. doi: 10.1371/journal.pone.0177880
Cassoff, R. M., Moore, K. M., McLellan, W. A., Barco, S. G., Rotstein, D. S., and Moore, M. J. (2011). Lethal entanglement in baleen whales. Dis. Aquat. Org. 96, 175–185. doi: 10.3354/dao02385
Castellini, M. A., and Rea, L. D. (1992). The biochemistry of natural fasting at its limits. Experientia 48, 575–582. doi: 10.1007/BF01920242
Cattet, M., Stenhouse, G. B., Janz, D. M., Kapronczai, L., Erlenbach, J., Jansen, H. T., et al. (2017). The quantification of reproductive hormones in the hair of captive adult brown bears and their application as indicators of sex and reproductive state. Conserv. Physiol. 5:cox032. doi: 10.1093/conphys/cox032
Daniel, J. C. (1981). Delayed implantation in the northern fur seal (Callorhinus ursinus) and other pinnipeds. J. Reprod. Fertil. Suppl. 29, 35–50.
deHart, P. A. P., and Picco, C. M. (2015). Stable oxygen and hydrogen isotope analyses of bowhead whale baleen as biochemical recorders of migration and arctic environmental change. Polar Sci. 9, 235–248. doi: 10.1016/j.polar.2015.03.002
Dunkin, R. C., McLellan, W. A., Blum, J. E., and Pabst, D. A. (2005). The ontogenetic changes in the thermal properties of Atlantic bottlenose dolphin blubber (Tursiops truncatus). J. Exp. Biol. 208, 1469–1480. doi: 10.1242/jeb.01559
Eales, J. G. (1988). The influence of nutritional state on thyrid function in various vertebrates. Am. Zool. 28, 351–362.
Edwards, L. D., Heyman, A. H., and Swidan, S. (2011). Hypocortisolism: an evidence-based review. Integr. Med. 10, 30–37.
Fanson, K. V., Keeley, T., and Fanson, B. G. (2014). Cyclic changes in cortisol across the estrous cycle in parous and nulliparous Asian elephants. Endocr. Connect. 3, 57–66. doi: 10.1530/EC-14-0025
Fuller, B. T., Fuller, J. L., Sage, N. E., Harris, D. A., O'Connell, T. C., and Hedges, R. E. (2005). Nitrogen balance and d15N: why you're not what you eat during nutritional stress. Rapid Commun. Mass Spectrom. 19, 2497–2506. doi: 10.1002/rcm.2090
George, J. C., Stimmelmayr, R., Suydam, R., Usip, S., Givens, G., Sformo, T., et al. (2016). Severe bone loss as part of the life history strategy of bowhead whales. PLoS ONE 11:e0156753. doi: 10.1371/journal.pone.0156753
Hahn, M. V., McDaniel, B. T., and Wiik, J. C. (1986). Rates of hoof growth and wear in Holstein cattle. J. Dairy Sci. 69, 2148–2156. doi: 10.3168/jds.S0022-0302(86)80647-6
Hamilton, P. K., Knowlton, A. R., and Marx, M. K. (2007). “Right whales tell their own stories: the photo-identification catalog,” in The Urban Whale, eds S. D. Kraus and R. M. Rolland (Cambridge, MA: MIT Press), 200–231.
Hashimoto, O., Ohtsuki, H., Kakizaki, T., Amou, K., Sato, R., Doi, S., et al. (2015). Brown adipose tissue in cetacean blubber. PLoS ONE 10:e0116734. doi: 10.1371/journal.pone.0116734
Hayes, S. A., Josephson, E., Maze-Foley, K., and Rosel, P. E. (2017). US Atlantic and Gulf of Mexico. Marine Mammal Stock Assessments – 2016. Woods Hole, MA: NOAA Technical Memorandum NMFS-NE-241.
Herrera, A. Y., Nielsen, S. E., and Mather, M. (2016). Stress-induced increases in progesterone and cortisol in naturally cycling women. Neurobiol. Stress. 3, 96–104. doi: 10.1016/j.ynstr.2016.02.006
Hobson, K. A. (2007). “Isotopic tracking of migrant wildlife,” in Stable Isotopes in Ecology and Environmental Science, eds R. Michener and K. Lajtha (Oxford: Blackwell Publishing), 155–175.
Hobson, K. A., Alisauskas, R. T., and Clark, R. G. (1993). Stable-nitrogen isotope enrichment in avian tissues due to fasting and nutritional stress: implications for isotopic analyses of diet. Condor 95, 388–394. doi: 10.2307/1369361
Hunt, K. E., Innis, C. J., Merigo, C. M., and Rolland, R. M. (2016b). Endocrine responses to diverse stressors of capture, entanglement and stranding in leatherback turtles (Dermochelys coriacea). Conserv. Physiol. 4:cow022. doi: 10.1093/conphys/cow022
Hunt, K. E., Lysiak, N. S., Moore, M., and Rolland, R. M. (2017a). Multi-year longitudinal profiles of cortisol and corticosterone recovered from baleen of North Atlantic right whales (Eubalaena glacialis). Gen. Comp. Endocrinol. 254, 50–59. doi: 10.1016/j.ygcen.2017.09.009
Hunt, K. E., Lysiak, N. S., Moore, M. J., and Rolland, R. M. (2016a). Longitudinal progesterone profiles from baleen of female North Atlantic right whales (Eubalaena glacialis) match recent calving history. Conserv. Physiol. 4:cow014. doi: 10.1093/conphys/cow014
Hunt, K. E., Lysiak, N. S., Robbins, J., Moore, M. J., Seton, R. E., Torres, L., et al. (2017b). Multiple steroid and thyroid hormones detected in baleen from eight whale species. Conserv. Physiol. 5:cox061. doi: 10.1093/conphys/cox061
Hunt, K. E., Stimmelmayr, R., George, C., Hanns, C., Suydam, R., Brower, H., et al. (2014). Baleen hormones: a novel tool for retrospective assessment of stress and reproduction in bowhead whales (Balaena mysticetus). Conserv. Physiol. 2:cou030. doi: 10.1093/conphys/cou030
Irvine, L. G., Thums, M., Hanson, C. E., McMahon, C. R., and Hindell, M. A. (2017). Quantifying the energy stores of captial breeding humpback whales and income breeding sperm whales using historical whaling records. R. Soc. Open Sci. 4:160290. doi: 10.1098/rsos.160290
Kellar, N. M., Catelani, K. N., Robbins, M. N., Trego, M. L., Allen, C. D., Danil, K., et al. (2015). Blubber cortisol: a potential tool for assessing stress response in free-ranging dolphins without effects due to sampling. PLoS ONE 10:e0115257. doi: 10.1371/journal.pone.011525
Kellar, N. M., Keliher, J., Trego, M. L., Catelani, K. N., Hanns, C., George, J. C., et al. (2013). Variation of bowhead whale progesterone and concentrations across demographic groups and sample matrices. Endang. Species Res. 22, 61–72. doi: 10.3354/esr00537
Kelly, J. F. (2000). Stable isotopes of carbon and nitrogen in the study of avian and mammalian trophic ecology. Can. J. Zool. 78, 1–27. doi: 10.1139/z99-165
Knowlton, A. R., and Kraus, S. D. (2001). Mortality and serious injury of Northern right whales (Eubalaena glacialis) in the western North Atlantic Ocean. J. Cetacean Res. Manag. 2(Spec. Issue), 193–208.
Knowlton, A. R., Hamilton, P. K., Marx, M. K., Pettis, H. M., and Kraus, S. D. (2012). Monitoring North Atlantic right whale Eubalaena glacialis entanglement rates: a 30 yr retrospective. Mar. Ecol. Prog. Ser. 466, 293–302. doi: 10.3354/meps09923
Knowlton, A. R., Robbins, J., Landry, S., McKenna, H. A., Kraus, S. D., and Werner, T. B. (2016). Effects of fishing rope strength on the severity of large whale entanglements. Conserv. Biol. 30, 318–328. doi: 10.1111/cobi.12590
Koren, L., Whiteside, D., Fahlman, S., Ruckstuhl, K., Kutz, S., Checkley, S., et al. (2012). Cortisol and corticosterone independence in cortisol-dominant wildlife. Gen. Comp. Endocrinol. 177, 113–119. doi: 10.1016/j.ygcen.2012.02.020
Kraus, S. D., Hamilton, P. K., Kenney, R. D., Knowlton, A. R., and Slay, C. K. (2001). Status and trends in reproduction of the North Atlantic right whale. J. Cetacean Res. Manag. 2, 231–236.
Kraus, S. D., Kenney, R. D., Mayo, C. A., McLellan, W. A., Moore, M. J., and Nowacek, D. P. (2016). Recent scientific publications cast doubt on North Atlantic right whale future. Front. Mar. Sci. 3:137. doi: 10.3389/fmars.2016.00137
Kraus, S. D., Moore, K. E., Price, C. E., Crone, M. J., Watkins, W. A., Winn, H. E., et al. (1986). The use of photographs to identify North Atlantic right whales (Eubalaena glacialis). Rep. Int. Whaling Comm. 10(Spec Issue), 145–151.
Kraus, S. D., Pace, R. M., and Frasier, T. R. (2007). “High investment, low return: the strange case of reproduction in Eubalaena glacialis,” in The Urban Whale, eds S. D. Kraus and R. M. Rolland (Cambridge, MA: MIT Press), 172–199.
Kudielka, B.M., and Kirschbaum, C. (2005). Sex differences in HPA axis responses to stress: a review. Biol. Psychol. 69, 113–132. doi: 10.1016/j.biopsycho.2004.11.009
LeBeau, M. A., Montgomery, M. A., and Brewer, J. D. (2011). The role of variations in growth rate and sample collection on interpreting results of segmental analyses of hair. Forensic Sci. Int. 210, 110–116. doi: 10.1016/j.forsciint.2011.02.015
Lee, S. H., Schell, D. M., McDonald, T. L., and Richardson, W. J. (2005). Regional and seasonal feeding by bowhead whales Balaena mysticetus as indicated by stable isotope ratios. Mar. Ecol. Prog. Ser. 285, 271–287. doi: 10.3354/meps285271
Lockyer, C. (1981). Growth and energy budgets of large baleen whales from the Southern Hemisphere. FAO Fish. Ser. 3, 379–487.
Lubetkin, S. C., Zeh, J. E., Rosa, C., and George, J. C. (2008). Age estimation for young bowhead whales (Balaena mysticetus) using annual baleen growth increments. Can. J. Zool. 86, 525–538. doi: 10.1139/Z08-028
Lysiak, N. S. (2008). Investigating the Migration and Foraging Ecology of North Atlantic Right Whales with Stable Isotope Geochemistry of Baleen and Zooplankton. Dissertation, Boston University, Boston, MA.
Matthews, C. J. D., and Ferguson, S. H. (2015). Seasonal foraging behavior of Eastern Canada-West Greenland bowhead whales: an assessment of isotopic cycles along baleen. Mar. Ecol. Prog. Ser. 522, 269–286. doi: 10.3354/meps11145
McAninch, E. A., and Bianco, A. C. (2015). Thyroid hormone signaling in energy homeostasis and energy metabolism. Ann. N.Y. Acad. Sci. 1311, 77–87. doi: 10.1111/nyas.12374
Miller, C. A., Reeb, D., Best, P. B., Knowlton, A. R., Brown, M. W., and Moore, M. J. (2011). Blubber thickness in right whales Eubalaena glacialis and Eubalaena australis related with reproduction, life history status and prey abundance. Mar. Ecol. Prog. Ser. 438, 267–283. doi: 10.3354/meps09174
Moore, M. J. (2014). How we all kill whales. ICES J. Mar. Sci. 71, 760–763. doi: 10.1093/icesjms/fsu008
Moore, M. J., and van der Hoop, J. (2012). The painful side of trap and fixed net fisheries: chronic entanglement of large whales. J. Mar. Biol. doi: 10.1155/2012/230653
Moore, M. J., Bogomolni, A., Bowman, R., Hamilton, P. K., Harry, C. T., Knowlton, A. R., et al. (2006). “Fatally entangled right whales can die extremely slowly,” in Proceedings of the Oceans 2006 (Boston, MA: IEEE). 1–3.
Moore, M. J., Knowlton, A. R., Kraus, S. D., McLellan, W. A., and Bonde, R. K. (2005). Morphometry, gross morphology and available histopathology in North Atlantic right whale (Eubalaena glacialis) mortalities (1970-2002). J. Cetacean Res. Manag. 6, 199–214.
Moore, M. J., van der Hoop, J.V., Barco, S. G., Costidis, A. M., Gulland, F. M., Jepson, P. D., et al. (2013). Criteria and case definitions for serious injury and death of pinnipeds and cetaceans caused by anthropogenic trauma. Dis. Aquat. Org. 103, 229–264. doi: 10.3354/dao02566
Mülling, C. K., Bragulla, H. H., Reese, S., Budras, K.-D., and Steinberg, W. (1999). How structures in bovine hoof epidermis are influenced by nutritional factors. Anat. Histol. Embryol. 28, 103–108. doi: 10.1046/j.1439-0264.1999.00180.x
Mullur, R., Liu, Y.-Y., and Bren, G. A. (2014). Thyroid hormone regulation of metabolism. Physiol. Rev. 94, 355–382. doi: 10.1152/physrev.00030.2013
NARWC (2006a). North Atlantic Right Whale Consortium Identification and Sightings Database. Available online at: www.narwc.org
NARWC (2006b). North Atlantic Right Whale Consortium Necropsy Database. Available online at: www.narwc.org.
Newsome, S. D., Clementz, M. T., and Koch, P. L. (2010). Using stable isotope biogeochemistry to study marine mammal ecology. Mar. Mam. Sci. 26, 509–572. doi: 10.1111/j.1748-7692.2009.00354.x
Ortiz, C. L., Costa, D., and Le Bouef, B. J. (1978). Water and energy flux in elephant seal pups fasting under natural conditions. Physiol. Zool. 51, 166–178.
Ortiz, R. M., MacKenzie, D. S., and Worthy, G. A. (2000). Thyroid hormone concentrations in captive and free-ranging West Indian manatees (Trichechus manatus). J. Exp. Biol. 203, 3631–3637.
Pace, R. M., Corkeron, P. J., and Kraus, S. D. (2017). State-space mark-recapture estimates reveal a recent decline in abundance of North Atlantic right whales. Ecol. Evol. 7, 8730–8741. doi: 10.1002/ece3.3406
Pettis, H. M., Pace, R. M., Schick, R. S., and Hamilton, P. K. (2017a). North Atlantic Right Whale Consortium Annual Report Card. Report to the North Atlantic Right Whale Consortium.
Pettis, H. M., Rolland, R. M., Hamilton, P. K., Knowlton, A. R., Burgess, E. A., and Kraus, S. D. (2017b). Body condition changes arising from natural factors and fishing gear entanglements in North Atlantic right whales Eubalaena glacialis. Endang. Species Res. 32, 237–249. doi: 10.3354/esr00800
Pond, C. M. (1978). Morphological aspects and the ecological and mechanical consequences of fat deposition in wild vertebrates. Annu. Rev. Ecol. Syst. 9, 519–570.
Ptak, G. E., Tacconi, E., Czernik, M., Toschi, P., Modlinski, J. A., and Loi, P. (2012). Embryonic diapause is conserved across mammals. PLoS ONE 7:e33027. doi: 10.1371/journal.pone.0033027
Reese, C. S., Calvin, J. A., George, J. C., and Tarpley, R. J. (2001). Estimation of fetal growth and gestation in bowhead whales. J. Am. Stat. Assoc. 96, 915–923. doi: 10.1198/016214501753209031
Renfree, M. B., and Shaw, G. (2000). Diapause. Annu. Rev. Physiol. 62, 353–375. doi: 10.1146/annurev.physiol.62.1.353
Robbins, J., Knowlton, A. R., and Landry, S. (2015). Apparent survival of North Atlantic right whales after entanglement in fishing gear. Biol. Conserv. 191, 421–427. doi: 10.1016/j.biocon.2015.07.023
Robeck, T. R., Steinman, K. J., Gearhart, S., Reidarson, T. R., McBain, J.F., and Monfort, S. L. (2004). Reproductive physiology and development of artificial insemination technology in killer whales (Orcinus orca). Biol. Reprod. 71, 650–660. doi: 10.1095/biolreprod.104.027961
Robeck, T. R., Steinman, K. J., Ramirez, K., Greenwell, M., Van Bonn, W., Yoshioka, M., et al. (2009). Seasonality, estrous cycle characterization, estrus synchronization, semen cryopreservation and artificial insemination in the Pacific white-sided dolphin (Lagenorhynchus obliquidens). Reproduction 138, 391–405. doi: 10.1530/REP-08-0528
Robeck, T. R., Steinman, K. J., Yoshioka, M., Jensen, E., O'Brien, J. K., Katsumata, E., et al. (2005). Estrous cycle characterization and artificial insemination using frozen-thawed spermatozoa in the bottlenose dolphin (Tursiops truncatus). Reproduction 129, 659–674. doi: 10.1530/rep.1.00516
Rolland, R. M., Hunt, K. E., Kraus, S. D., and Wasser, S. K. (2005). Assessing reproductive status of right whales (Eubalaena glacialis) using fecal hormone metabolites. Gen. Comp. Endocrinol. 142, 308–317. doi: 10.1016/j.ygcen.2005.02.002
Rolland, R. M., McLellan, W. A., Moore, M. J., Harms, C. A., Burgess, E. A., and Hunt, K. E. (2017). Fecal glucocorticoids and anthropogenic injury and mortality in North Atlantic right whales Eubalaena glacialis. Endang. Species Res. 34, 417–429. doi: 10.3354/esr00866
Rolland, R. M., Parks, S. E., Hunt, K. E., Castellote, M., Corkeron, P. J., Nowacek, D. P., et al. (2012). Evidence that ship noise increases stress in right whales. Proc. R. Soc. Lond. B Biol. Sci. 279, 2363–2368. doi: 10.1098/rspb.2011.2429
Rolland, R. M., Schick, R. S., Pettis, H. M., Knowlton, A. R., Hamilton, P. K., Clark, J. S., et al. (2016). Health of North Atlantic right whales Eubalaena glacialis over three decades: from individual health to demographic and population health trends. Mar. Ecol. Prog. Ser. 542, 265–282. doi: 10.3354/meps11547
Romero, L. M., and Wingfield, J. C. (2015). Tempests, Poxes, Predators, and People: Stress in Wild Animals and how They Cope. New York, NY. Oxford University Press.
Samuel, A. M., and Worthy, G. A. (2005). Variability in fatty acid composition of bottlenose dolphin (Tursiops truncatus) blubber as a function of body site, season, and reproductive state. Can. J. Zool. 82, 1933–1942. doi: 10.1139/z05-001
Santini, F., Marzullo, P., Rotondi, M., Ceccarini, G., Pagano, L., Ippolito, S., et al. (2014). Mechanisms in endocrinology: the crosstalk between thyroid gland and adipose tissue: signal integration in health and disease. Eur. J. Endocrinol. 17, R137–R152. doi: 10.1530/EJE-14-0067
Schell, D. M., and Saupe, S. M. (1993). “Feeding and growth as indicated by stable isotopes,” in The Bowhead Whale, eds J. J. Burns, J. J. Montague, and C. J. Cowles (Lawrence, KS: The Society of Marine Mammalogy), 491–506.
Schick, R. S., Kraus, S. D., Rolland, R. M., Knowlton, A. R., Hamilton, P. K., Pettis, H. M., et al. (2013). Using hierarchical bayes to understand movement, health, and survival in the endangered North Atlantic right whale. PLoS ONE 8:e64166. doi: 10.1371/journal.pone.0064166
Silva, J. E. (2003). The thermogenic effect of thyroid hormone and its clinical implications. Ann. Intern. Med. 139, 205–213. doi: 10.7326/0003-4819-139-3-200308050-00010
St. Aubin, D. J., Stinson, R. H., and Geraci, J. R. (1984). Aspects of the structure and composition of baleen, and some possible effects of exposure to petroleum hydrocarbons. Can. J. Zool. 62, 193–198. doi: 10.1139/z84-032
Tetsuka, M. (2007). Actions of glucocorticoid and their regulatory mechanisms in the ovary. Anim. Sci. J. 78, 112–120. doi: 10.1111/j.1740-0929.2007.00414.x
Thomas, P. O., Reeves, R. R., and Brownell, R. L. (2016). Status of the world baleen whales. Mar. Mam. Sci. 32, 682–734. doi: 10.1111/mms.12281
Tilbrook, A. J., Turner, A. I., and Clarke, I. J. (2002). Stress and reproduction: central mechanisms and sex differences in non-rodent species. Stress 5, 83–100. doi: 10.1080/10253890290027912
Trumble, S. J., Robinson, E. M., Berman-Kowalewski, M., Potter, C. W., and Usenko, S. (2013). Blue whale earplug reveals lifetime contaminant exposure and hormone profiles. Proc. Natl. Acad. Sci. U.S.A. 110, 16922–16926. doi: 10.1073/pnas.1311418110
Ullmann, E., Barthel, A., Petrowski, K., Stalder, T., Kirschbaum, C., and Bornstein, S. R. (2016). Pilot study of adrenal steroid hormones in hair as an indicator of chronic mental and physical stress. Sci. Rep. 6:25842. doi: 10.1038/srep25842
Uribe, R., Jaimes-Hoy, L., Ramirez-Martinez, C., Garcia-Vasquez, A., Romero, F., Cisneros, M., et al. (2014). Voluntary exercise adapts the hypothalamus-pituitary-thyroid axis in male rates. Endocrinol. 155, 2020–2030. doi: 10.1210/en.2013-1724
van der Hoop, J. M., Corkeron, P., Henry, A. G., Knowlton, A. R., and Moore, M. J. (2016). Predicting lethal entanglements as a consequence of drag from fishing gear. Mar. Pollut. Bull. 115, 91–104. doi: 10.1016/j.marpolbul.2016.11.060
van der Hoop, J. M., Corkeron, P., Kenney, J., Landry, S., Morin, D., Smith, J., et al. (2015). Drag from fishing gear entangling North Atlantic right whales. Mar. Mam. Sci. 32, 619–642. doi: 10.1111/mms.12292
van der Hoop, J. M., Moore, M. J., Barco, S. G., Cole, T. V., Daoust, P. Y., Henry, A. G., et al. (2013). Assessment of management to mitigate anthropogenic effects on large whales. Conserv. Biol. 27, 121–133. doi: 10.1111/j.1523-1739.2012.01934.x
van der Hoop, J., Corkeron, P., and Moore, M. (2017). Entanglement is a costly life-history stage in large whales. Ecol. Evol. 7, 92–106. doi: 10.1002/ece3.2615
Werth, A. J. (2004). Models of hydrodynamic flow in the bowhead whale filter feeding apparatus. J. Exp. Biol. 207, 3569–3580. doi: 10.1242/jeb.01202
Werth, A. J., and Potvin, J. (2016). Baleen hydrodynamics and morphology of cross-flow filtration in balaenid whale suspension feeding. PLoS ONE 11:e0150106. doi: 10.1371/journal.pone.0150106
Werth, A. J., Straley, J. M., and Shadwick, R. E. (2016). Baleen wear reveals intraoral water flow patterns of mysticete filter feeding. J. Morphol. 277, 453–471. doi: 10.1002/jmor.20510
Wingfield, J. C., Maney, D. L., Breuner, C. W., Jacobs, J. D., Lynn, S., Ramenofsky, M., et al. (1998). Ecological bases of hormone-behavior interactions: the “emergency life history stage.” Am. Zool. 38, 191–206. doi: 10.1093/icb/38.1.191
Keywords: baleen, steroid hormones, thyroid hormones, stable isotopes, entantlement, right whale
Citation: Lysiak NSJ, Trumble SJ, Knowlton AR and Moore MJ (2018) Characterizing the Duration and Severity of Fishing Gear Entanglement on a North Atlantic Right Whale (Eubalaena glacialis) Using Stable Isotopes, Steroid and Thyroid Hormones in Baleen. Front. Mar. Sci. 5:168. doi: 10.3389/fmars.2018.00168
Received: 16 December 2017; Accepted: 26 April 2018;
Published: 15 May 2018.
Edited by:
Lisa Marie Komoroske, National Oceanic and Atmospheric Administration (NOAA), United StatesReviewed by:
Rebecca Ruth McIntosh, Phillip Island Nature Parks, AustraliaNicholas Marc Kellar, Southwest Fisheries Science Centre, National Oceanic and Atmospheric Administration (NOAA), United States
Copyright © 2018 Lysiak, Trumble, Knowlton and Moore. This is an open-access article distributed under the terms of the Creative Commons Attribution License (CC BY). The use, distribution or reproduction in other forums is permitted, provided the original author(s) and the copyright owner are credited and that the original publication in this journal is cited, in accordance with accepted academic practice. No use, distribution or reproduction is permitted which does not comply with these terms.
*Correspondence: Nadine S. J. Lysiak, bmFkaW5lLmx5c2lha0BnbWFpbC5jb20=