- 1Centre d'Etudes Biologiques de Chizé, UMR7372 CNRS-Université La Rochelle, Villiers en Bois, France
- 2National Institute of Polar Research, Tokyo, Japan
- 3School of Life and Environmental Sciences, Faculty of Science & Technology, Deakin University, Burwood, VIC, Australia
- 4Unité Biologie des Organismes et Ecosystèmes Aquatiques (BOREA, UMR 7208), Sorbonne Universités, Muséum National d'Histoire Naturelle, Université Pierre et Marie Curie, Université de Caen Basse-Normandie, CNRS, IRD, Paris, France
- 5Institut Pluridisciplinaire Hubert Curien, Département Ecologie, Physiologie et Ethologie, UMR 7178, CNRS-UDS, Strasbourg, France
In the d'Urville Sea in East Antarctica, a population of roughly 20,000 pairs of Adélie penguins of Iles des Pétrels (Terre Adélie) has experienced two massive breeding failures, with no chick surviving the 2013–14 and 2016–17 breeding seasons. In both seasons the extent of sea ice in front of the colony persisted throughout the breeding cycle of the birds. The timing of sea-ice recession differed greatly between seasons and the absence of polynya in a crucial phase of the cycle were paramount in driving these failures. The change in the icescape in front of Ile des Pétrels following the calving of the Mertz glacier in 2010, together with increase in precipitations and changes in sea-ice firmness explain this situation and are discussed in the present manuscript. To prevent additional future impacts on this colony, like competition with fisheries for instance, we strongly support a scientific research zone in the d'Urville Sea—Mertz area, one of the three zones of proposed Marine Protected Area in East Antarctica to the Commission for the Conservation of Antarctic Marine Living Resources.
Introduction
Antarctica and its surrounding ocean are at the forefront of the global environmental changes. Freshwater ice on land and sea ice are indeed strongly affected by the warming of the air and sea, and this has drastic consequences on the biotic components of the Antarctic ecosystems (Constable et al., 2014). A large number of mid-trophic level species playing pivotal roles in the food webs, rely on sea ice for their embryonic development, food and breeding, and are the main prey species of marine birds and mammals. As such, the monitoring of the biology of Antarctic meso- and top predators has been successfully used as eco-indication of environmental changes (Hindell et al., 2003).
Adélie penguins (Pygoscelis adeliae) are often considered as good indicators of environmental changes because their ecology is closely related to the state of sea ice (Ainley, 2002). Recently, satellite measurements have led to a new estimate that suggests an increase in the total number of Adélie penguins, as well as in the number of colonies across the continent (Lynch and LaRue, 2014). Using this technique and ground-based surveys, Adélie populations have been shown to be generally in decline in the Antarctic Peninsula, while other populations are stable or slightly increasing (Cimino et al., 2013; Lyver et al., 2014; Southwell et al., 2015). The use of satellite counts has contributed to the downgrading of the species on the IUCN scale from “Near Threatened” to “Least Concerned” in 2017. However, this downgrading at a continental scale may mask major local disparities and further population trajectories: stable and/or slightly increasing populations are found where sea ice has increased and the opposite where sea ice is melting. With projected continuous warming the increasing sea-ice trend is not expected to last.
In the d'Urville Sea of the East Antarctic sector, a well-studied region in terms of eco-regionalisation (Koubbi et al., 2011), the situation of Adélie penguins in Terre Adélie has rapidly deteriorated over the last 6 years. This population experienced a catastrophic breeding season with no chick surviving out of 20196 breeding pairs in the 2013–14 breeding season (Barbraud et al., 2015; Ropert-Coudert et al., 2015). This had never been recorded over the 36 years that the Adélie colony was monitored and not to such an extent in other locations around the continent. Details about the physical factors that led to this massive failure—namely sea-ice extent larger than usual and rain episodes—have been recently described for Adélie penguins (Barbraud et al., 2015; Ropert-Coudert et al., 2015). Here, we report on a second massive breeding failure for the same Adélie penguins' colony that took place in the 2016–17 season. We aim to highlight the similarities and differences in the sea-ice and meteorological conditions that could cause the two massive failures, and compare the impact these had on the foraging activity and reproductive output of the birds. Such unusual events—both in terms of size of the population affected and the increasing frequency at which they occur—could be indicative of the start of massive changes in the environment and have specific relevance to the current discussion around the establishment of a Marine Protected Area in East Antarctica, which includes the D'Urville Sea/Mertz region.
Materials and Methods
The study was conducted on Adélie penguins' colonies on Ile des Pétrels, near the Dumont d'Urville station (66°40′S, 140°01′E). Female Adélie penguins lay one or two eggs in mid-November and both parents participate to the reproductive effort by alternating foraging trips at sea, where they feed primarily on two species of krill (Euphausia superba and E. crystallorophias) and Antarctic silverfish (Pleuragramma antarctica) (Ropert-Coudert et al., 2002), with presence on the nest to keep the eggs/chicks warm and protected from predators. When the chicks are developed enough and display thermoregulatory abilities, parental trips to sea become irregular and the chicks form crèches, until they fledge in early March (Ainley, 2002).
Biological Data
Breeding success data were collected between 1993 and 2017 as part of the program 109 of the French Polar Institute (IPEV), on the colonies of Ile des Pétrels, which ranged from 10,849 to 20,957 breeding pairs over these 24 seasons. Active nests (with at least one egg) were counted in November, and the number of chicks that were still alive was counted in February. The breeding success was calculated as the number of chicks in February divided by the number of active nests in November (see Jenouvrier et al., 2006 for more details).
Foraging activity of Adélie penguins was monitored in the frame of the IPEV program 1091 through the deployment of miniaturized GPS devices (CatLog™ GPS, Catnip Technologies, USA, and AxyTrek™ GPS-accelerometry loggers, Technosmart, Italy). These devices were temporarily attached to the back feathers of the penguins using marine tape (Wilson et al., 1997) and recorded the position of the birds every 15–30 min during the long trips of the incubation phase, and every 3 min in the shorter trips of the chick-rearing phase. Location data were processed following the methods described in Widmann et al. (2015), which showed that, typically, females travel at sea for 15 days after laying the eggs, followed by the foraging trip of males that last between 10 and 15 days. The egg hatches after the return of the male. Thereafter, foraging trips become shorter, getting as short as a few hours to 3–4 days during the chick rearing phase. Foraging trip duration (days) and maximum distance (km) from the colony was calculated for each trip. Adults were weighed to the nearest 10 g at their departure from (initial body mass) and their return to their nest. The difference between these two body masses was calculated to give the body mass change for birds in incubation. Body mass changes were not calculated during chick-rearing as we could not ascertain that the adults had already fed chicks or not when recaptured at the nest and only initial body mass is thus shown. Statistical analyses were conducted in R (R Core Team, 2016). Analysis of variance with post-hoc Bonferroni multiple comparison testing was used for each stage when more than three seasons were compared.
Environmental Data
Meteorological data (monthly air temperature and direction of prevailing winds in degrees from the north) between 1956 and 2017 were downloaded from the British Antarctic Survey website (https://legacy.bas.ac.uk/met/READER/). The percentage of sea-ice cover was calculated as the percentage where sea-ice concentration was more than 15% in an area ranging from 135 and 145°E and 61 and 67°S (foraging range of Adélie penguins at Ile des Pétrels, Widmann et al., 2015) and over a period ranging from 1992 to 2017, using the data provided by the Institut Français de Recherche pour l'Exploitation de la Mer (ftp://ftp.ifremer.fr/ifremer/cersat/products/gridded/psi-concentration/data/antarctic/). Sea-ice extent was calculated as the distance (km) from the colony to the nearest open water (sea-ice concentration < 15%). A polynya was defined as a zone of open water enclosed in sea ice. Summer sea-ice characteristics (firmness) around the colony were observed several times a week by foot and using helicopter for distant sites (up to the ice edge).
Results
The breeding success varied greatly over the 1993–2017 period but it declined from 2011 onward—to the exception of the 2015–16 season which showed breeding success around 0.9—with breeding successes below 0.4 and reaching zero, or near zero success, in 2 years. Indeed, breeding success was zero for the 20196 breeding pairs in the 2013–14 season and only two chicks survived out of the 18163 breeding pairs in the 2016–17 season (Figure 1). In the remainder of the analyses we will focus on comparing the conditions between the zero success years (2013–14 and 2016–17 seasons) and the typical high breeding season of 2015–16. We chose this season because its breeding success is exceptionally high in comparison with other recent years (post 2010 and Mertz glacier calving, see Discussion) and as such causes and consequences are expected to be exacerbated (see Ropert-Coudert et al., 2009 for a similar test case). In addition, 2015–16 is the only year of high breeding success for which we have tracking data.
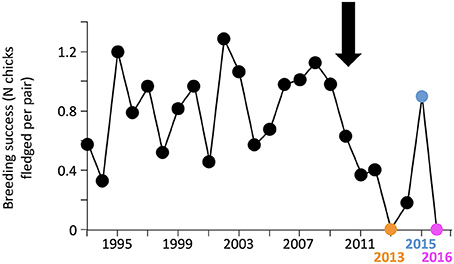
Figure 1. Reproductive success of Adélie penguins between 1994–95 and 2016–17 at Ile des Petrels, Terre Adélie. The 2 years when no (or virtually no) chicks survived are indicated by orange (2013–14) and magenta dots (2016–17). The year 2015–16 where breeding success was relatively high is indicated by a blue dot. This color code is used in the rest of the article when zero success years are compared with the high success year. The arrow indicates the collapse of the Mertz glacier (see Discussion).
The season 2013–14 showed the largest sea-ice extent and cover in our dataset (since 1992) in the beginning of the breeding season in November (Figures 2A,B). Interestingly, 2016–17 had the smallest sea-ice extent in the beginning of the breeding season but both years kept a larger than usual extent later in the summer. While it was compact in 2013–14, the sea ice in January was sherbet-like in 2016–17 and did not break for the whole summer. There was no polynya opening in vicinity of the colony in 2013–14 and 2016–17, closest opened water was 80–90 km away. In contrast, a polynya started to open in front of the colony in November 2015 and connected with the open sea by January 2016. Air temperature in the two zero years were in the upper range and 2016–17 saw the warmest November in decades (Figure 2C). Wind direction over the 3 years considered here shows a clear shift in the regime as the winds were blowing mainly from a more easterly direction than before (Figure 2D).
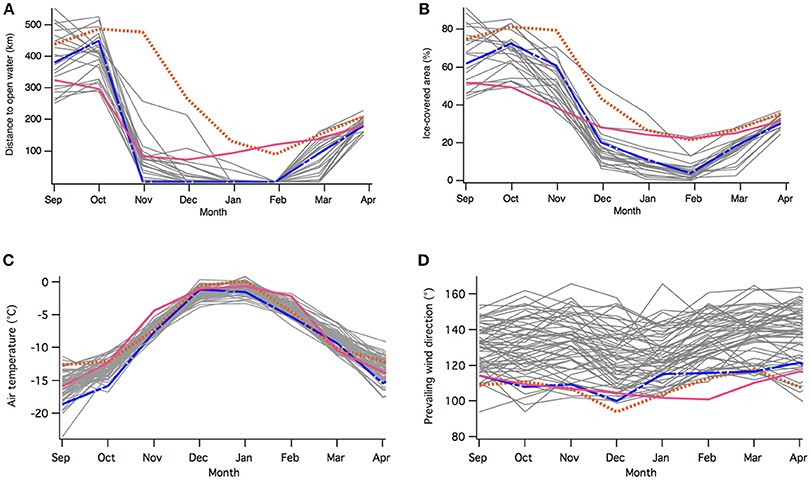
Figure 2. Monthly distance to the nearest open water from the colony (A) and the percentage of ice-covered area between 135 and 145°E, and between 61 and 67°S from 1992 to 2017 (B). Monthly average air temperature in °C (C) and direction of prevailing winds in degrees from the north (°) (D) observed at the Dumont d'Urville station for the years 1956–2017 (months on the x-axis). The years 2013–14, 2015–16, and 2016–17 are in orange, blue and magenta, respectively, while other years are in gray.
In the 2013–14 season, following the large extent of sea-ice, birds traveled further during trips of longer duration at all breeding stages (Table 1, Figure 3). In 2015–16, the maximum distances reached during incubation by females did not differ from that of the 2013–14 season but the trips were shorter in time. During incubation by males and chick rearing the distances traveled and the time spent at sea in 2015–16 were considerably less than during the 2013–14 season. During the 2016–17 season, incubating birds did not travel as far as those in the 2013–14 season but their trips were as long as in the previous zero year. Interestingly, duration and distance of foraging trips varied substantially over the 2016–17 chick-rearing phase. Birds could only have access to cracks and crevasses in the ice near the breeding colony to find food. Parents were observed provisioning chicks, and the reddish color of the feces suggested they found krill within a short radius around the colony. Yet, these resources lasted only for a few days and distances covered apparently increased again as birds stopped returning to their nests or only after protracted periods of time (up to 20 days for those recaptured).
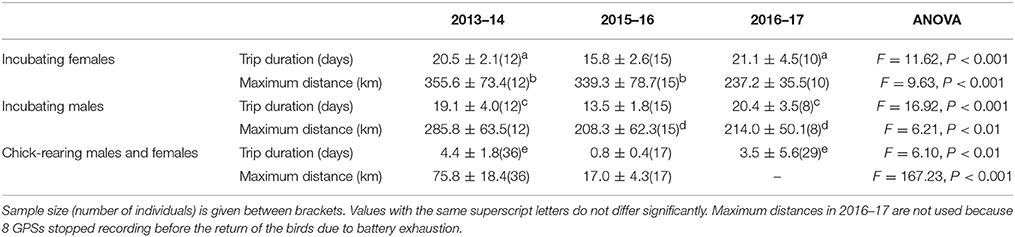
Table 1. Mean values (±SD) of durations and maximum distances reached during foraging trips of Adélie penguins, by year and by phase of the reproductive cycle.
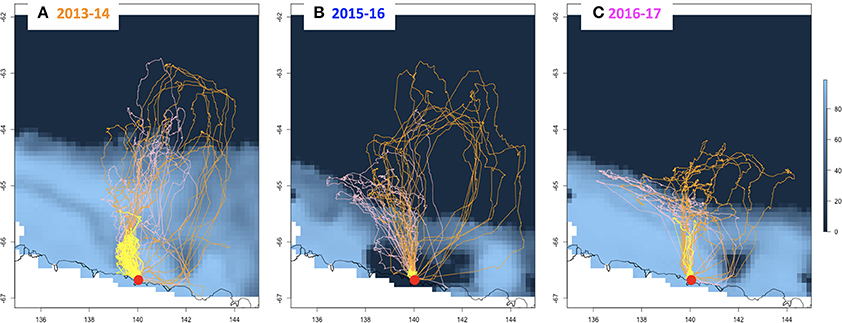
Figure 3. Foraging tracks of Adélie penguin females (orange) and males (pink) during their first incubation trips, and males and females during chick rearing (yellow) superimposed on maps of sea-ice concentration (Dec. 1st of each year) from open water (navy blue) to maximum ice concentration (lighter shades of blue), for (A) 2013–14, (B) 2015–16, and (C) 2016–17. The periphery of the continent is indicated by a black border. The colony is indicated by a red dot.
The body mass gained during foraging trips by incubating females (F = 16.7, P < 0.001, Figure 4A) and males (F = 31.0, P < 0.001, Figure 4B) was greater in the 2015–16 season compared with both the 2013–14 and 2016–17 seasons, which did not differ significantly. The initial body mass of chick-rearing birds was different among seasons: it was the heaviest in 2015–16 and the lightest in 2013-14, with 2016–17 being intermediate (F = 65.2, P < 0.001, Figure 4C).
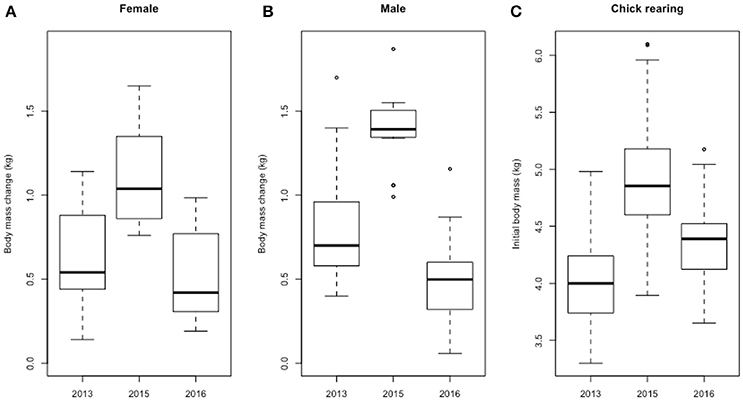
Figure 4. Body mass changes (kg) over the first incubating foraging trips of females (A) and males (B), and initial body mass of chick-rearing Adélie penguins (C), over the 3 years considered in this analysis (2013, 2015, and 2016 stands for 2013–14, 2015–16 and 2016–17, respectively).
Discussion
Sea-ice distribution contributed to shape the foraging and breeding performances of Adélie penguins, as has been reported in other locations around the Antarctic (Emmerson and Southwell, 2008). The reasons for the two catastrophic years recorded in Terre Adélie differ but in both seasons the extent of the sea ice in front of the colony was greater than in other years and, most importantly, persisted throughout the season. This abundance of sea ice could be explained by several, non-exclusive, factors. For instance, in mid-February 2010 the massive B9B iceberg coming from the Ross Sea collided with the Mertz Glacier tongue, east of Dumont d'Urville (Young et al., 2010). The expected consequences of such a massive change in the icescape included, among other things, a decrease in polynya activity (Tamura et al., 2012). As several icebergs, freed from the collision, anchored themselves in the shallow bay in front of the colony of Dumont d'Urville they created a network of “pillars” onto which sea ice could form and be retained (Massom et al., 2009). In parallel, temperatures rising from the seasonal norms in the summer also melted the icebergs' and continent's freshwater ice, which then flow toward the sea where it froze again at the contact of the −1.8°C seawater (https://www.nasa.gov/content/goddard/antarctic-sea-ice-reaches-new-record-maximum). In addition, a change in the main bearing of the winds that, instead of blowing from the mainland and detaching ice from the continent, blows now more from the East and tend to concentrate the sea ice in front of the archipelago of Pointe Géologie (Massom et al., 2009). Finally, linked to the increased temperature and change in wind regimes increased precipitation (including rain) in 2013–14 further aggravated the conditions for the Adélie penguins: besides the flooding of unprotected nests, malnourished, wet chicks with their non-waterproof plumage suffered from the cooling power of the wind leading to massive failure events (Ropert-Coudert et al., 2015).
If sea ice is the principal reason behind the failures, there are major differences in both “zero years;” one is the extent of the sea ice early in the season, and the other is the state of the sea ice, although the role of the latter deserves further investigation and repeatable measurement. The differences between the 2 years when the breeding success was zero show that it is imperative to monitor the changes in bird activity throughout the season. Here, sea-ice extent during incubation in the 2015–16 (high breeding success) was similar to that of 2016–17 but it subsequently decreased and a large polynya opened directly opposite the colony. The opening of a polynya in the vicinity of the colony has been shown to be crucial to the breeding success of the penguins from Dumont d'Urville, as it allowed parents to access food quickly and return rapidly to their nest to feed the growing chicks (Widmann et al., 2015). In other words, a very small sea-ice extent in October does not necessarily mean that the breeding success will be high, especially if, like in 2016–17, the sea ice turns into a glutinous sherbet that does not break with the offshore swell, preventing access to open water and reducing net food availability. Those are visible in the lower body mass gains during incubation in the two zero years and lower body mass of adult during chick rearing.
In the light of the above, long-term (> 10 years) and fine-scale (combining at-sea tracking of the foraging with on-land monitoring of breeding activities) observations are more than ever paramount to our understanding of the resilience of species to the growing pressure of environmental changes but also to chaotic events and their consequences (Lescroël et al., 2014). These are some of the pressing questions highlighted by the 1st Horizon Scan of the Scientific Committee on Antarctic Research (Kennicutt et al., 2014). Our long-term observatories on Adélie penguins' foraging and breeding activities contributed to the demonstration of the fragility of the populations of penguins and the ecosystem they depend upon. Recent studies in the Ross Sea highlighted the existence of an optimal sea-ice condition for Adélie populations (Ainley, 2002; Ballard et al., 2010). Here, we further suggests that this optimal may be narrower than expected, but also that the sea-ice recession timing and the characteristics of the sea ice may play a major role in shaping the foraging and breeding successes of Adélie penguins (e.g., Lescroël et al., 2014).
In summary, the Adélie penguin population of Ile des Petrels can be now considered as a population with severe environmental constraints and, based on current climatic projections of a global increase in air temperature (IPCC, 2014), this may continue for several years. Although difficult to anticipate, the absence of two generations at 3 years intervals will most certainly lead to changes in the dynamics of this population, which was increasing until now. In Terre Adélie, the success of the emperor penguin breeding is also very closely related to the extent of the fast ice attached to the Antarctic continent, partly for the same reasons than for the Adélie penguin (but on another time frame): the greater this extent, the less successful breeding, especially if no polynya forms in the vicinity of the colony (Barbraud and Weimerskirch, 2001; Massom et al., 2009; Barbraud et al., 2015). For example, in 2013, 2014, and 2016, the mortality of emperor penguin eggs and chicks was as high as 0.89, 0.97, and 0.73, respectively (number of eggs laid in May of the year that died before fledging in December of the same year, C. Barbraud, unpublished data).
Given the aforementioned environmental constraints already imposed on the populations of the archipelago of Pointe Géologie, we strongly support the planning of a Marine Protected Area in East Antarctica. Three areas are proposed by the Australian and the EU delegations to the Commission for the Conservation of Antarctic Marine Living Resources (https://www.ccamlr.org/). It includes the d'Urville Sea – Mertz, Drygalski and Mac Robertson regions. For the D'Urville Sea Mertz, there are multiple specific objectives to protect representative areas of pelagic and benthic biodiversity including essential habitats for mid-trophic level species (krill species and Antarctic silverfish) and important bird areas. In October 2017, the proponents of the East Antarctic MPA proposal indicated to CCAMLR that, for the d'Urville Mertz region, a no take zone for Antarctic krill fisheries should be proposed to at least protect the summer foraging range of the Adélie population and the emperor breeding ground of Ile des Pétrels, and to allow long term monitoring of the region until clear trends on marine birds populations are obtained. While an MPA is no solution to global environmental changes, it removes some of the additional impacts (e.g., fisheries) that could weigh on populations. Predictive modeling is a powerful conservation tool to anticipate how populations may react to expected changes (e.g., Jenouvrier et al., 2015) but such approaches require data like those presented here to be obtained in a system with no additional pressure. Protecting the waters of East Antarctica would mean the creation of a large-scale MPA in the Southern Ocean, and be the next step in realizing the initial commitment of the members of CCAMLR to establish a network of protected areas around Antarctica.
Author Contributions
YR-C, KS, CB, FA, KD, TP, and TR collected data in the field. YR-C, AK, CB, and KD analyzed the data, YR-C and AK wrote the manuscript and all authors revised it.
Funding
This study was financially supported by the French Polar Institute Paul Emile Victor (IPEV), the WWF-UK through R. Downie, the Zone Atelier Antarctique et Subantarctique – LTER France of the CNRS. This study was approved by the ethic committee of IPEV. This study is a contribution to the program SENSEI funded by the BNP Paribas Foundation.
Conflict of Interest Statement
The authors declare that the research was conducted in the absence of any commercial or financial relationships that could be construed as a potential conflict of interest.
Acknowledgments
We thank A.J.J. MacIntosh, X. Meyer, M. Pellé who participated to the fieldwork in the 2013–14 season and all the members of the logistic on site for their assistance.
References
Ainley, D. G. (2002). The Adélie Penguin: Bellwether of Climate Change. New York, NY: Columbia University Press.
Ballard, G., Dugger, K., Nur, N., and Ainley, D. (2010). Foraging strategies of Adélie penguins: adjusting body condition to cope with environmental variability. Mar. Ecol. Prog. Ser. 405, 287–302. doi: 10.3354/meps08514
Barbraud, C., Delord, K., and Weimerskirch, H. (2015). Extreme ecological response of a seabird community to unprecedented sea ice cover. R. Soc. Open Sci. 2:140456. doi: 10.1098/rsos.140456
Barbraud, C., and Weimerskirch, H. (2001). Emperor penguins and climate change. Nature 411, 183–186. doi: 10.1038/35075554
Cimino, M. A., Fraser, W. R., Irwin, A. J., and Oliver, M. J. (2013). Satellite data identify decadal trends in the quality of Pygoscelis penguin chick-rearing habitat. Glob. Change Biol. 19, 136–148. doi: 10.1111/gcb.12016
Constable, A. J., Melbourne-Thomas, J., Corney, S. P., Arrigo, K. R., Barbraud, C., Barnes, D. K., et al. (2014). Change in Southern Ocean ecosystems I: how changes in physical habitats directly affect marine biota. Glob. Chang. Biol. 20, 3004–3025. doi: 10.1111/gcb.12623
Emmerson, L., and Southwell, C. (2008). Sea ice cover and its influence on Adélie penguin reproductive performance. Ecology 89, 2096–2102. doi: 10.1890/08-0011.1
Hindell, M. A., Bradshaw, C., Harcourt, R., and Guinet, C. (2003). “Ecosystem monitoring: are seals a potential tool for monitoring change in marine systems?” in Marine Mammals. Fisheries, Tourism and Management Issues, eds N. J. Gales, and M. A. Hindell (Melbourne, VIC: CSIRO Publishing), 330–343.
IPCC (2014). Climate Change 2014: Synthesis Report. Contribution of Working Groups I, II and III to the Fifth Assessment Report of the Intergovernmental Panel on Climate Change [Core Writing Team, R. K. Pachauri and L. A. Meyer (eds.)]. IPCC, Geneva.
Jenouvrier, S., Barbraud, C., and Weimerskirch, H. (2006). Sea ice affects the population dynamics of Adélie penguins in Terre Adélie. Polar Biol. 29, 413–423. doi: 10.1007/s00300-005-0073-6
Jenouvrier, S., Péron, C., and Weimerskirch, H. (2015). Extreme climate events and individual heterogeneity shape life-history traits and population dynamics. Ecol. Monogr. 85, 605–624. doi: 10.1890/14-1834.1
Kennicutt, I. I., Chown, S. L., Cassano, J. J., Liggett, D., Massom, R., Peck, L. S., et al. (2014). Polar research: six priorities for Antarctic science. Nature 512, 23–26. doi: 10.1038/512023a
Koubbi, P., Hosie, G., Constable, A., Raymond, B., Moteki, M., Améziane, N., et al. (2011). Estimating the Biodiversity of the Shelf and Oceanic zone of the d'Urville Sea (East Antarctica) for Ecoregionalisation Using the CEAMARC (Collaborative East Antarctic Marine Census) CAML Surveys. CCAMLR WSMPA-11/A-17.
Lescroël, A., Ballard, G., Grémillet, D., Authier, M., and Ainley, D. G. (2014). Antarctic climate change: extreme events disrupt plastic phenotypic response in Adélie penguins. PLoS ONE 9:e85291. doi: 10.1371/journal.pone.0085291
Lynch, H. J., and LaRue, M. A. (2014). First global census of the Adélie Penguin. Auk 131, 457–466. doi: 10.1642/AUK-14-31.1
Lyver, P. O'., Barron, M., Barton, K. J., Ainley, D. G., Pollard, A., Gordon, S., et al. (2014). Trends in the breeding population of Adélie penguins in the Ross Sea, 1981-2012: a coincidence of climate and resource extraction effects. PLoS ONE 9:e91188. doi: 10.1371/journal.pone.0091188
Massom, R. A., Hill, K., Barbraud, C., Adams, N., Ancel, A., Emmerson, L., et al. (2009). Fast ice distribution in Adélie Land, East Antarctica: interannual variability and implications for emperor penguins Aptenodytes forsteri. Mar. Ecol. Prog. Ser. 374, 243–257. doi: 10.3354/meps07734
R Core Team (2016). R: A language and Environment for Statistical Computing. Vienna:R Foundation for Statistical Computing. Available online at: https://www.R-project.org/
Ropert-Coudert, Y., Kato, A., Bost, C.-A., Rodary, D., Sato, K., Le Maho, Y., et al. (2002). Do Adélie penguins modify their foraging behaviour in pursuit of different prey? Mar. Biol. 140, 647–652. doi: 10.1007/s00227-001-0719-z
Ropert-Coudert, Y., Kato, A., and Chiaradia, A. (2009). Impact of small-scale environmental perturbations on local marine food resources: a case study of a predator, the little penguin. Proc. R. Soc. Lond. B 276, 4105–4109. doi: 10.1098/rspb.2009.1399
Ropert-Coudert, Y., Kato, A., Meyer, X., Pellé, M., Macintosh, A. J. J., Angelier, F., et al. (2015). A complete breeding failure in an Adélie penguin colony correlates with unusual and extreme environmental events. Ecography 38, 111–113. doi: 10.1111/ecog.01182
Southwell, C., Emmerson, L., McKinlay, J., Newbery, K., Takahashi, A., Kato, A., et al. (2015). Spatially extensive standardized surveys reveal widespread, multi-decadal increase in East Antarctic Adélie penguin population. PLoS ONE 10:e0139877. doi: 10.1371/journal.pone.0139877
Tamura, T., Williams, G. D., Fraser, A. D., and Ohshima, I. K. (2012). Potential regime shift in decreased sea ice production after the Mertz Glacier calving. Nat. Commun. 3:826. doi: 10.1038/ncomms1820
Widmann, M., Kato, A., Raymond, B., et al. (2015). Habitat use and sex-specific foraging behaviour of Adélie penguins throughout the breeding season in Adélie Land, East Antarctica. Mov. Ecol. 3:30. doi: 10.1186/s40462-015-0052-7
Wilson, R. P., Pütz, K., Peters, G., et al. (1997). Long-term attachment of transmitting and recording devices to penguins and other seabirds. Wildl. Soc. Bull. 25, 101–106.
Keywords: eco-indicating species, Sea ice, breeding, foraging, marine protected areas, seabirds, extreme events
Citation: Ropert-Coudert Y, Kato A, Shiomi K, Barbraud C, Angelier F, Delord K, Poupart T, Koubbi P and Raclot T (2018) Two Recent Massive Breeding Failures in an Adélie Penguin Colony Call for the Creation of a Marine Protected Area in D'Urville Sea/Mertz. Front. Mar. Sci. 5:264. doi: 10.3389/fmars.2018.00264
Received: 11 December 2017; Accepted: 16 July 2018;
Published: 06 August 2018.
Edited by:
Anton Pieter Van de Putte, Royal Belgian Institute of Natural Sciences, BelgiumReviewed by:
Brett W. Molony, Department of Primary Industries and Regional Development of Western Australia (DPIRD), AustraliaAlastair Martin Mitri Baylis, South Atlantic Environmental Research Institute, Falkland Islands
Bruno Danis, Free University of Brussels, Belgium
Copyright © 2018 Ropert-Coudert, Kato, Shiomi, Barbraud, Angelier, Delord, Poupart, Koubbi and Raclot. This is an open-access article distributed under the terms of the Creative Commons Attribution License (CC BY). The use, distribution or reproduction in other forums is permitted, provided the original author(s) and the copyright owner(s) are credited and that the original publication in this journal is cited, in accordance with accepted academic practice. No use, distribution or reproduction is permitted which does not comply with these terms.
*Correspondence: Yan Ropert-Coudert, yan.ropert-coudert@cebc.cnrs.fr