- Marine Science Institute, University of California, Santa Barbara, Santa Barbara, CA, United States
Shipwrecks can have significant localized effects when grounded on shallow coral reefs. These effects are not limited to the immediate physical damage, but can have wide-spread and lasting impacts due to alteration of the chemical makeup of the surrounding water column. This can subsequently impact the growth of benthic organisms, often leading to phase shifts and high levels of mortality of corals in the vicinity of the wreck. At Palmyra atoll, the grounding of a longline fishing vessel on the shallow reef terrace is associated with a phase shift to the corallimorph, Rhodactis howesii. In 2013, a wreck removal effort initiated by the US Department of Fish and Wildlife resulted in the successful extraction and disposal of the wreckage, after which the density and percent cover of R. howesii in the immediate vicinity of the wreck site dropped precipitously. Here, we document the response of the fish community to the wreck removal and localized decline in R. howesii. We show that the biomass of scarid parrotfishes and acanthurid surgeonfishes and unicornfishes (primarily herbivores) increased after the removal of the wreck, while biomass of chaetodontid butterflyfishes (primarily invertivores, many species are known to feed on coelenterate polyps) declined over the study period. The density of small scarids and acanthurids also increased, but only after a few years post removal. Overall these results indicate that Palmyra’s unfished herbivore population has rapidly responded to the removal of the wreck and associated decrease in corallimorph cover, can maintain high levels of grazing where space is made available for colonization of early successional algae species, and may have the potential to facilitate reef recovery.
Introduction
Ship groundings can have many significant deleterious effects on coral reefs. In addition to a number of consequences from the physical impact itself (Precht et al., 2001; Riegl, 2001), ship material has been shown to have wider-reaching impacts due to processes that alter species composition and competitive dynamics within benthic assemblages. Shipwrecks have the potential to carry non-native species that may subsequently colonize the natural reef (Marshall and Edgar, 2003), though there is limited documentation for this and often exotic species in marine environments are not traced to their exact point of origin. However, there is convincing evidence that wrecks can cause changes in water chemistry resulting from metals and contaminants that leach over time from the wreck debris. This alteration of water chemistry can facilitate certain benthic species, particularly species whose growth is limited by specific metals such as iron. Often, these opportunistic species then begin to outcompete corals and other benthic organisms for space, resulting in wreck-associated benthic phase shifts where the enriched species (e.g., fleshy algae, cyanobacteria, or sessile invertebrates) become dominant (Kelly et al., 2012). Some hypothesize that benthic phase shifts on coral reefs may be extremely difficult or even in some cases impossible to reverse, due to hysteresis in these systems favoring processes that prevent the successful reestablishment of corals once another benthic group becomes established (Knowlton, 1992; Norström et al., 2009; Hughes et al., 2010). Studies that forecast rates of reef recovery after a ship wreck estimate that it could take many decades to centuries for corals to recover to their pre-disturbance state (Precht et al., 2001; Riegl, 2001). Recovery potential is likely to be context-dependent, and will vary based on the initial benthic community state, restoration effort and timing, the prevalence and intensity of additional ecological stressors, and the abundance and diversity of consumers in the system that can prevent or reverse a phase shift.
Herbivorous fish populations can show remarkable capacity to respond to disturbances that alter benthic composition and resource availability. Russ et al. (2015) questioned the prevailing paradigm that herbivores prevent phase shifts via a top–down control of the benthos through grazing, and promoted the concept that the benthos controls the herbivores in a bottom–up manner through resource distribution, while leaving room for the idea that these two concepts are not mutually exclusive. There have been some clear examples of both bottom–up and top–down processes at work in a complementary fashion, with the herbivores responding to the benthos and the benthos responding in turn to the herbivores. In Mo’orea, French Polynesia, a large-scale coral mortality event resulting from a combination of crown-of-thorn seastar outbreak and a cyclone resulted in a 90% decline in coral cover. Following these events the biomass of scarids tripled which prevented a wholesale transition to a macroalgal state (Adam et al., 2011; Holbrook et al., 2016). This resulted in increased coral recruitment and partial coral recovery within a relatively short time frame (Han et al., 2016). Other examples also show that coral reef fish populations can respond rapidly to disturbances that alter benthic assemblages and influence the abundance and distribution of preferred food resources (Lindahl et al., 2001; Russ and Leahy, 2017; Hempson et al., 2018). At Rose Atoll, in the US territory of American Samoa, iron enrichment from a shipwreck facilitated an invasion of cyanobacteria and macroalgae that eventually overgrew and killed the dominate reef building crustose coralline algae (CCA) and corals over a substantial area of reef. Concern about degradation of the reef leading to a breach and subsequent erosion of lagoonal islands (which constitute vital nesting ground for sea turtles and seabirds) led to a removal effort which was completed in 2010 (Pendleton, 2012). Monitoring of fish communities showed that the abundance and biomass of herbivorous reef fishes, particularly that of surgeonfishes and unicornfishes, was far higher in the vicinity of the wreck than in other areas of the atoll, which was attributed to the bloom of cyanobacteria and algae (Schroeder et al., 2008). In recent years, since debris removal has been completed and metallic sources of enrichment have been eliminated, invasive algae and cyanobacteria have declined and corals have started to recover (Kenyon et al., 2010; Pendleton, 2012). These examples show a promising level of resilience in these coral reef systems, both to natural (Mo’orea) and anthropogenic (Rose Atoll) disturbances and in both inhabited and fished (Mo’orea) and remote and protected (Rose Atoll) systems.
The current study was conducted at Palmyra atoll, a remote coral atoll and US National Wildlife Refuge in the central Pacific, where a shipwreck on the shallow reef was associated with a benthic phase shift to the corallimorph, Rhodactis howesii, which proliferated and overgrew corals across a large expanse of reef (Work et al., 2008, 2018). The US Fish and Wildlife Service removed the wreck in 2013, in an effort to eliminate the point sources of metallic pollutants thought to potentially be facilitating the growth of the corallimorph. In the years since the removal of the wreck, the density and percent cover of the corallimorph in the immediate vicinity of the wreck site has declined (Work et al., 2018). Here we document the response of the fish community to this transition in the benthic assemblage. Specifically, we show that the abundance and biomass of herbivorous fish taxa increased substantially in the few years following the wreck removal and that there was an overall shift in fish community structure over the study period. We hypothesize that this result is related to decreases in corallimorph cover which opened up space for the colonization and growth of the food sources of these species.
Materials and Methods
Study Site
Palmyra atoll (5°53′N 162°5′W) is a remote, low-lying, coral atoll in the Northern Line Islands, and sits roughly 1600 km south of the main Hawaiian Islands (Figure 1A). Palmyra has no indigenous population and has had relatively low levels of inhabitance and visitation throughout its history, apart from US naval occupation during World War II when the islands housed several thousand soldiers. Though brief, the military occupation left lasting impacts on Palmyra. Among other activities, the military alteration to the atoll included the dredging of a channel into the western lagoon, and construction of a causeway that separated two lagoons (Dawson, 1959; Collen et al., 2009). This activity severely altered the flow of water throughout the atoll, creating strong flow from the lagoons to the reef terraces and forereef (Collen et al., 2009). Since the military occupation during the 1940’s, Palmyra has remained largely uninhabited, apart from limited private yacht traffic. Palmyra was transferred from private ownership to become a US Fish and Wildlife Refuge in 2001 and since that time there has been no extractive fishing permitted on Palmyra’s reefs. Consequently, Palmyra hosts relatively high biomass of species that are exploited in inhabited regions, such as apex predators (DeMartini et al., 2008; Sandin et al., 2008; Bradley et al., 2017), and herbivorous fishes (Edwards et al., 2014).
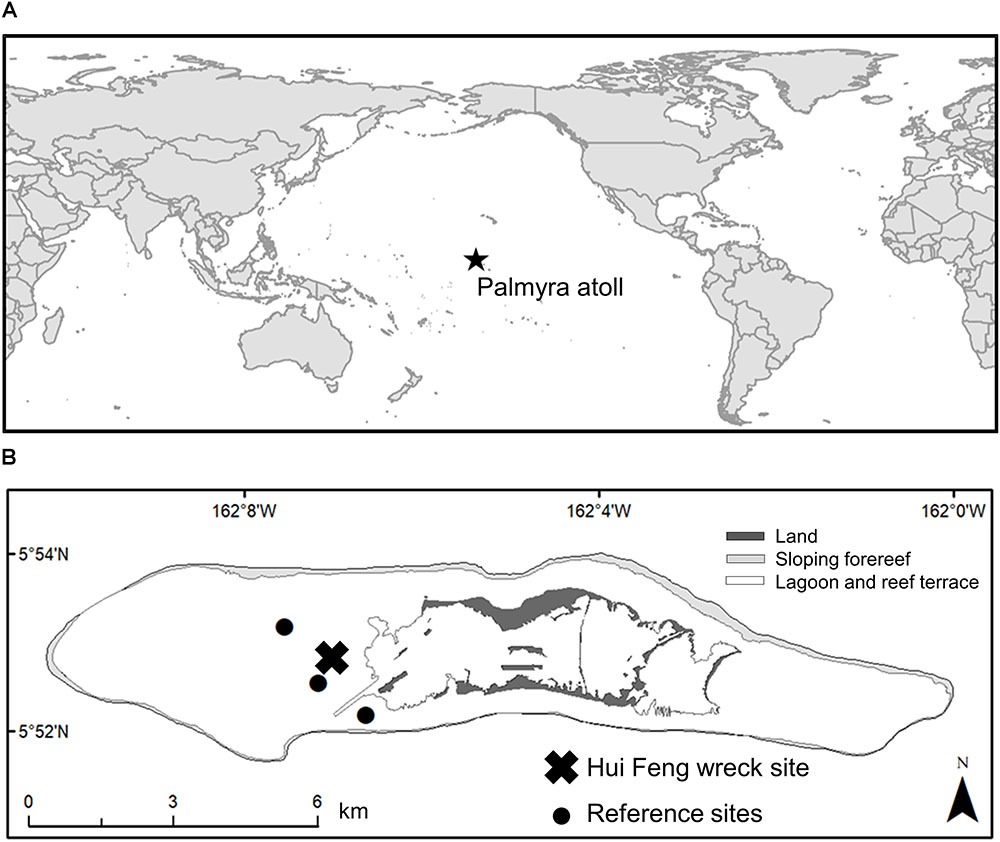
FIGURE 1. (A) Location of Palmyra atoll in the Pacific Ocean, (B) location of Hui Feng wreck site and reference survey sites on the shallow western terrace and backreef at Palmyra.
Shipwreck Site
The Hui Feng was a 37 m Taiwanese longlining fishing vessel constructed of steel, concrete, and wood that wrecked on the shallow reef (∼6 m) of Palmyra’s western terrace in 1991 (Figures 1B, 2A). It is believed that the vessel was wrecked intentionally, with the objective of securing political asylum for its crew members. The spread of a corallimorph, Rhodactis howesii (Figure 2B), is associated with the shipwreck, though it is believed that it may have been naturally present at Palmyra in low abundance prior to the wreck (Work et al., 2008, 2018). The exact mechanism for its facilitation by the wreck is not yet well understood. Corallimorphs have been shown to respond positively to disturbance (Chadwick-Furman and Spiegel, 2000; Muhando et al., 2002) and it has also been proposed that the ship material may be leaching into the surrounding water column and facilitating growth via iron enrichment, yet evidence for this has been inconclusive (Carter, 2014). Work et al. (2008) tracked the proliferation of the corallimorph, noting that it was present but in low abundance in 2004 but was beginning to expand by the following year. By 2007, they had documented corallimorph colonies as far as 1100 m from the wreck and the affected area covered approximately 1 km2. At that time, the average density of corallimorphs in the immediate vicinity of the wreck was 288 individuals per square meter, and rare to absent across the rest of the reefs of the atoll, except for near a few man-made boat moorings (Work et al., 2008). By 2011 the affected area had grown to 3.5 km2, primarily spreading to the NW and SE (Work et al., 2018). Corallimorphs can reproduce asexually (Chadwick-Furman and Spiegel, 2000), enabling them to quickly overgrow and smother living corals as well as physically harm and kill them with stinging nematocysts (Langmead and Chadwick-Furman, 1999; Work et al., 2008), hence there is a high potential for mass coral mortality resulting from a proliferation of corallimorph. There is strong evidence for these negative effects of corallimorph on the shallow coral reef at Palmyra (Work et al., 2008, 2018).
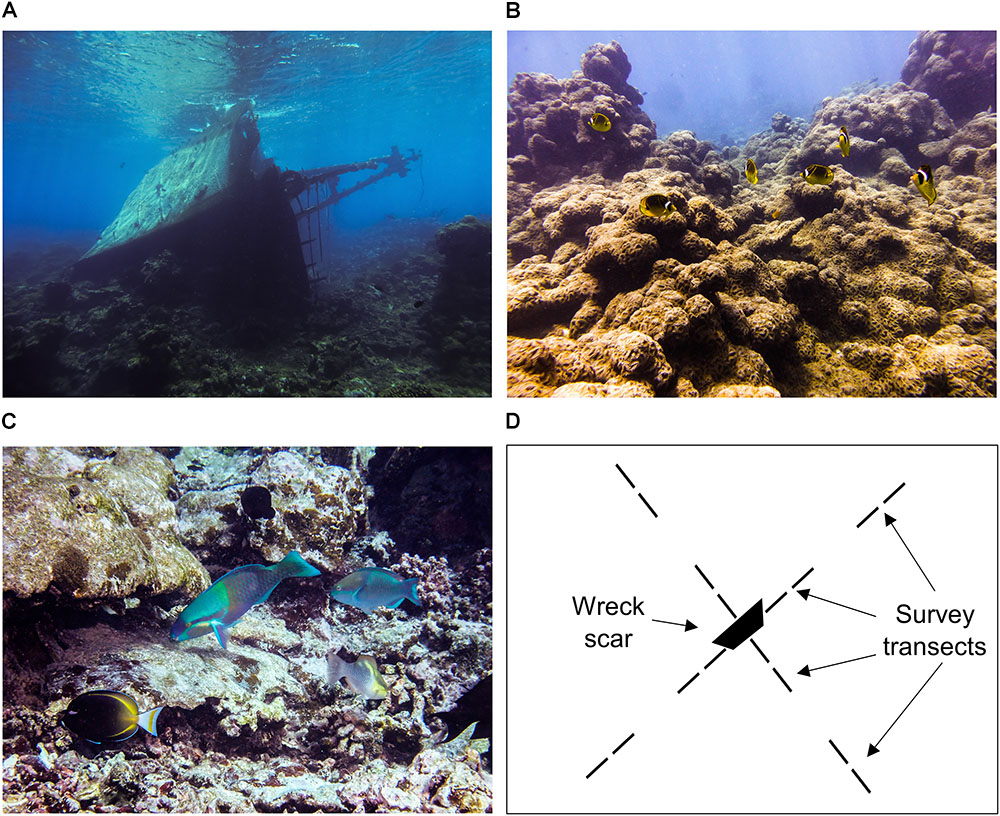
FIGURE 2. (A) Image of the wreck in 2013 prior to removal, (B) image from the reef near the wreck in 2013 showing characteristically high (nearly 100%) cover of corallimorph, (C) image from the reef near the wreck site in 2017 showing mixed algal assemblage (CCA, turfing algae, macroalgae) with low corallimorph cover, and (D) diagram depicting transect layout at the wreck site (each transect is 25 m in length, outer transects originate 100 m from the center of the wreck site).
In 2013, the US Department of Fish and Wildlife initiated a removal effort to extract the shipwreck from the reef. Over the course of 56 days, more than 180 metric tons of debris was carefully removed from the wreck site. The site was declared debris-free on December 31, 2013. Monitoring conducted since the removal has shown that while the corallimorph has continued to spread spatially across Palmyra and is now present at high densities at sites far from the wreck site, the density and percent cover in the immediate vicinity of the wreck site has declined and colonies have become more fragmented in space (Work et al., 2018). This newly available bare substrate has been colonized by a variety of benthic groups such as CCA, turfing algae, and macroalgae (Figure 2C). Experimental plots where corallimorph was killed were colonized by CCA and after 15 months they contained small Pocilloporids, indicating the potential for coral to recover after a decline in corallimorph cover (Work et al., 2018).
Fish Surveys
We conducted annual surveys of the fish community in the area adjacent to the shipwreck site from 2013 to 2017 (the 2013 survey was done prior to the removal of the wreck) which consisted of n = 16 belt transects, 25 m in length, radiating out from the site of the shipwreck (Figure 2D). In each intercardinal direction (NE, NW, SE, SW) we surveyed four transects, with two transects located immediately adjacent to the wreck and two transects originating roughly 100 m from the center of the wreck. Along each transect, an observer on SCUBA identified to species, counted, and sized to the nearest centimeter all fishes greater than or equal to 20 cm in a 4 m swath extending to the surface. The observer then swam back along the transect counting all fishes less than 20 cm on a 2 m swath extending to the surface (Friedlander et al., 2014; Davis et al., 2017). Cracks and crevices were searched for cryptic species. In 2015, 2016, and 2017 the observer also estimated the percent cover of corallimorphs on the benthos within the transect area. This was a visual estimate, completed on an additional swim down the transect line after completion of the 2 m fish transect. In 2013, 2014, and 2017 we surveyed additional sites, more distant (ca. 300–1200 m) from the wreck site and epicenter of the corallimorph invasion. Transects surveyed per site ranged from 4 to 9. These sites will be hereafter referred to as “reference sites.” Annual surveys were conducted in the mid-late summer (late August/early September) each year.
Density, Biomass, and Diversity Calculations
We calculated biomass for surveyed fishes using the length–weight parameters (W = aTLb, where parameters a and b are species-specific constants, TL is total length [cm], and W is weight [g]) assembled by the NOAA Coral Reef Ecosystem Program from FishBase (Froese and Pauly, 2009; Heenan et al., 2014). Row-specific biomass and density were calculated by dividing the row biomass and number of individuals by the area surveyed according to the size of the individual fish (100 m2 for fishes 20 cm or larger, 50 m2 for fishes less than 20 cm) and those values were pooled across species or family to attain transect level biomass density and density values for various taxonomic groupings. Total fish biomass was calculated excluding sharks and jacks for all analyses, because these are large, mobile predators and their biomass values may disproportionately contribute to overall biomass, though due to their mobility they are not necessarily associated with any given site, habitat, or time point. Species richness and Shannon diversity index were calculated for each transect using the ‘vegan’ package in R (Oksanen et al., 2007; R Core Team, 2013.
Statistical Analysis
We used ANOVAs to test for the effect of year on corallimorph percent cover, biomass of all fishes (excluding sharks and jacks), scarids, acanthurids and chaetodontids, density of scarid and acanthurid recruits, species richness, and Shannon diversity. We performed post hoc Tukey honest significant difference tests for all models that showed significant variation across years. We square root transformed density and biomass values prior to analysis to improve residual spread and normality. This analysis was performed using JMP Pro 13.
To compare fish biomass values between the wreck site and reference sites we ran one-way ANOVAs using the mean biomass value from each transect at the wreck and the reference sites for each year that surveys were conducted. Reference sites were surveyed in 2013, 2014, and 2017 so only those were the years compared. We square root transformed biomass values prior to analysis to improve residual spread and normality.
To examine multivariate differences in the composition of fish assemblages through time, we used transect-level species densities to perform a non-metric multidimensional scaling (nMDS) analysis. We used a Bray-Curtis similarity matrix and square root transformed species density values prior to analysis. We used PERMANOVA tests with the single factor of ‘Year’ to statistically test for differences in fish communities over time. This analysis was done using PRIMER-e v.7.
Results
Corallimorph cover at the Hui Feng wreck site declined over the last three years of the study period from a mean of 36.9% (±6.7% SE) in 2015 to a mean of 8.6% (±4.8% SE) in 2017 on survey transects at the Hui Feng wreck site (Figure 3 and Table 1a).
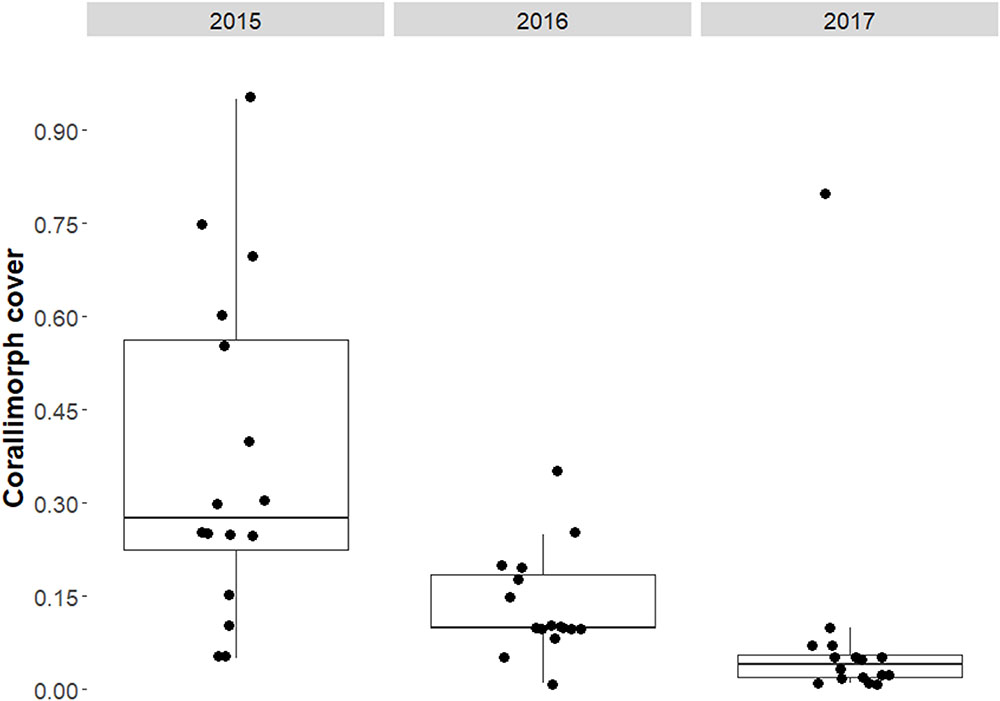
FIGURE 3. Box and whiskers plot of the proportional cover of corallimorph on transects around the shipwreck site from 2015 to 2017.
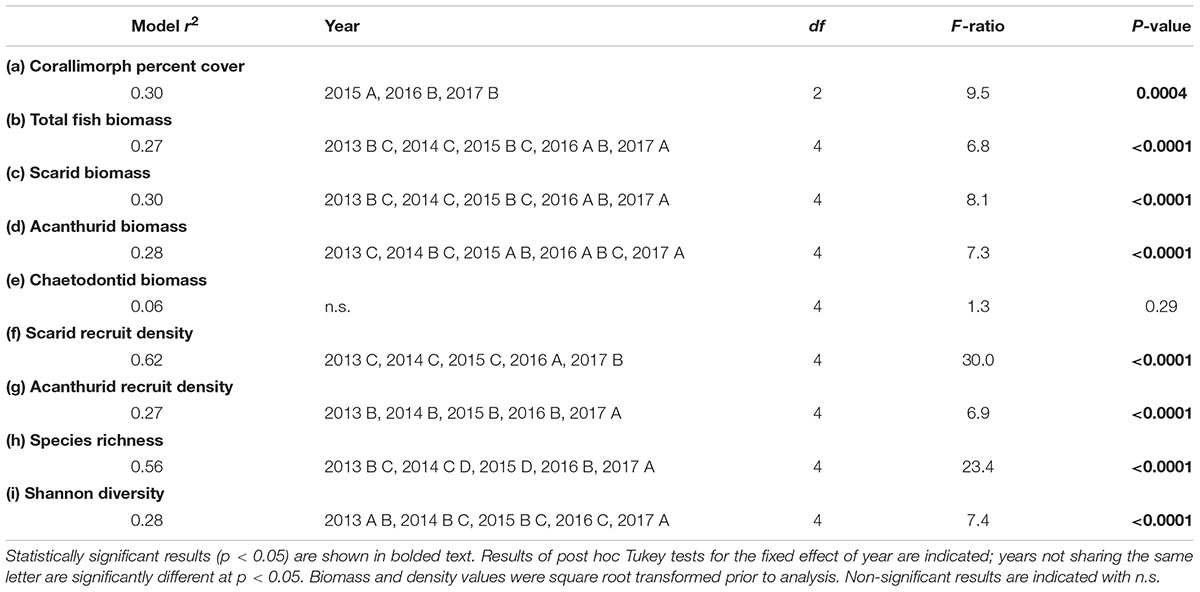
TABLE 1. Results of ANOVAs testing the effect of year on (a) corallimorph cover, (b) total fish biomass, (c) scarid biomass, (d) acanthurid biomass, (e) chaetodontid biomass, (f) scarid recruit density, (g) acanthurid recruit density, (h) species richness, and (i) Shannon diversity at the site of the Hui Feng wreck.
Some fish families showed strong temporal trends between 2013 and 2017 (Figure 4 and Table 1). Overall, fish biomass increased and this was driven primarily by herbivores, with less of a response by the other trophic groups. Total fish (Figure 4A and Table 1b), scarid parrotfishes (Family Labridae; tribe Scarini, Figure 4B and Table 1c), and acanthurids (Family Acanthuridae, includes surgeonfish, unicornfish, and bristletooth, Figure 4C and Table 1d) biomass increased significantly, while biomass of chaetodontid butterflyfishes (Family Chaetodontidae, Figure 4D and Table 1e) declined, though the temporal trend was not statistically significant. Trends in fish biomass near the wreck site relative to biomass at distant reference sites varied by family. In 2013 and 2014, total fish biomass near the wreck site was not significantly different from reference sites, but in 2017 biomass near the wreck was significantly higher (Table 2a). Similarly, in 2013 and 2014 scarid biomass did not differ between the wreck site and reference sites but in 2017 it differed strongly, and was nearly three times higher at the wreck site than at reference sites (Table 2b). The trend in total biomass was largely driven by this large increase in scarid biomass. Acanthurids also increased in biomass at the wreck sites over the study period, however, their pattern of change relative to the reference sites differed from the scarids. In 2013 and 2014 acanthurid biomass was significantly depressed at the wreck sites relative to reference sites (roughly three times higher at the reference sites than at the wreck site in 2013) but by 2017 mean biomass at the wreck and reference sites was not significantly different (Table 2c). Chaetodontids declined in abundance near the wreck site throughout the study period. In 2013 and 2014 chaetodontid biomass was significantly higher (roughly four and a half and seven and a half times higher respectively) at the wreck site than at the reference sites. In 2017 chaetodontid biomass was still significantly higher at the wreck site from the reference sites but the magnitude of the difference has decreased (Figure 4D and Table 2d). Density of recruit sized (<10 cm) scarids and acanthurids increased in the last years of the study period (Figure 5 and Tables 1f,g). Overall there was variation in species richness and diversity over the study period with richness increasing slightly over the last years of the study period but there was no strong temporal trend in diversity (Figure 6 and Tables 1h,i).
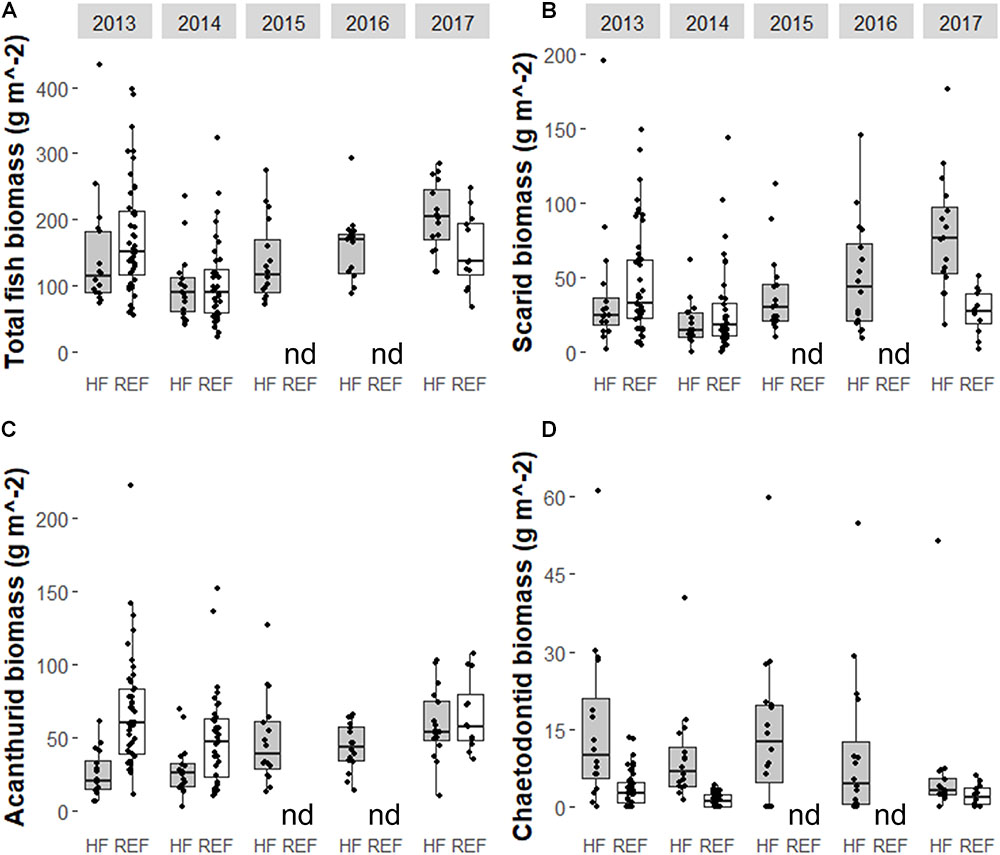
FIGURE 4. Box and whiskers plot of the biomass of (A) all fishes (excluding sharks and jacks), (B) scarids, (C) acanthurids, and (D) chaetodontids on transects surrounding the site of the Hui Feng shipwreck (shown in gray and noted on the x-axis with ‘HF’) and at reference sites (shown in white and noted on the x-axis with ‘REF’) on the reef terrace and backreef at Palmyra Atoll from 2013 to 2017. Note that y-axis varies between panels. Reference sites were not surveyed in 2015 or 2016 (no data indicated by ‘nd’). The wreck was removed between the 2013 and 2014 surveys.
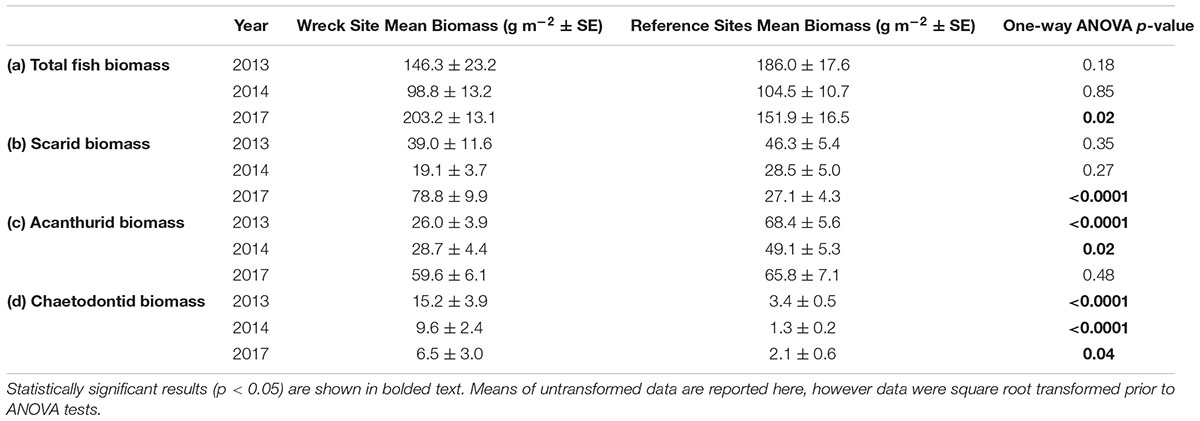
TABLE 2. Results of one-way ANOVAs comparing the mean transect-level biomass values from the wreck site to reference sites in 2013, 2014, and 2017 for (a) total fish (excluding sharks and jacks), (b) scarids, (c) acanthurids, and (d) chaetodontids.
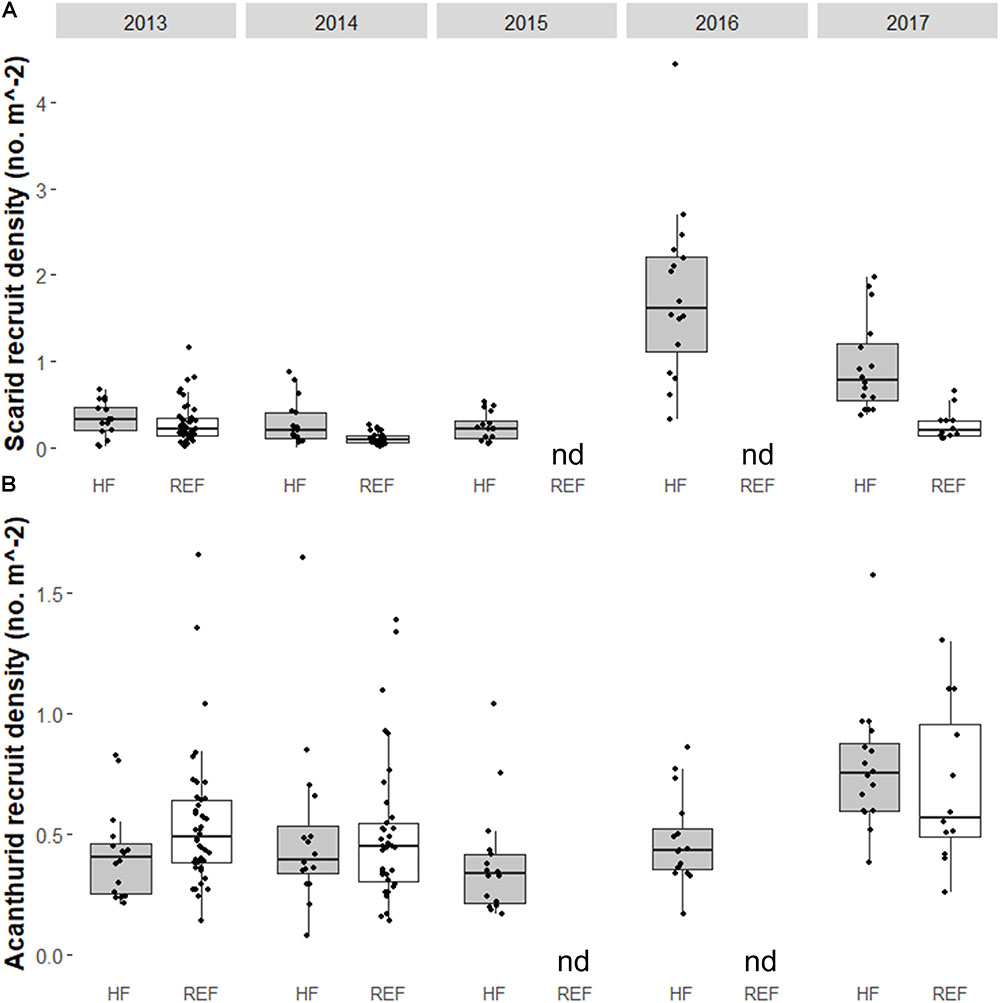
FIGURE 5. Box and whiskers plot of the density of recruit (total length < 10 cm) (A) scarids and (B) acanthurids at the Hui Feng wreck site (shown in gray and noted on the x-axis with ‘HF’) and at reference sites (shown in white and noted on the x-axis with ‘REF’) on the reef terrace and backreef at Palmyra Atoll from 2013 to 2017. Note that y-axis varies between panels. Reference sites were not surveyed in 2015 or 2016 (no data indicated by ‘nd’). The wreck was removed between the 2013 and 2014 surveys.
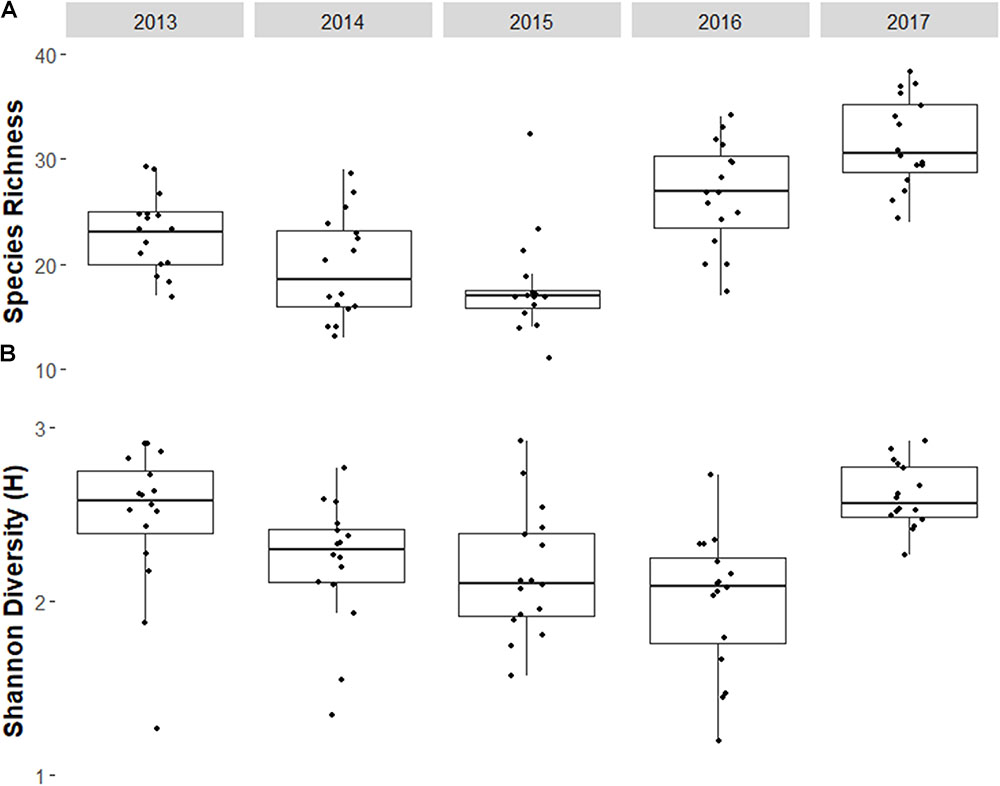
FIGURE 6. Box and whiskers plot of (A) Species richness per transect, (B) and Shannon Diversity Index per transect around the Hui Feng wreck site. The wreck was removed between the 2013 and 2014 surveys.
An nMDS plot shows some separation between the first three years and the last two years of the study period, indicating a shift in species composition and community structure in the area around the wreck site (Figure 7). The PERMANOVA showed significant variation in the fish community across years (Table 3a) with significant variation between all years except 2013 – 2014 and 2014 – 2015 (Table 3b). Percent similarity between years ranged from 38.7 (2015 – 2016) to 50.5 (2016 – 2017, Table 3c).
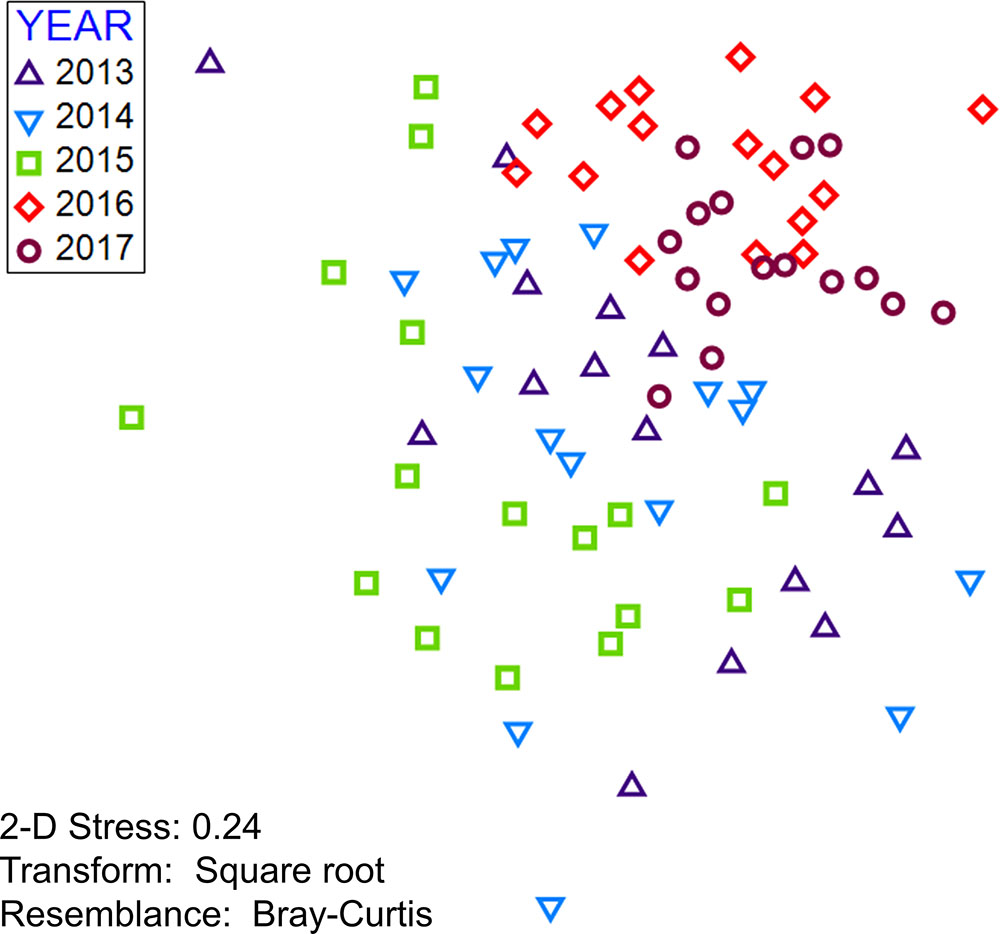
FIGURE 7. Multidimensional scaling plot of fish community around the Hui Feng wreck site using density by species, colored by year. Each point on the plot represents an individual transect in multidimensional space. Data were square root transformed prior to analysis and a Bray-Curtis similarity matrix was used.
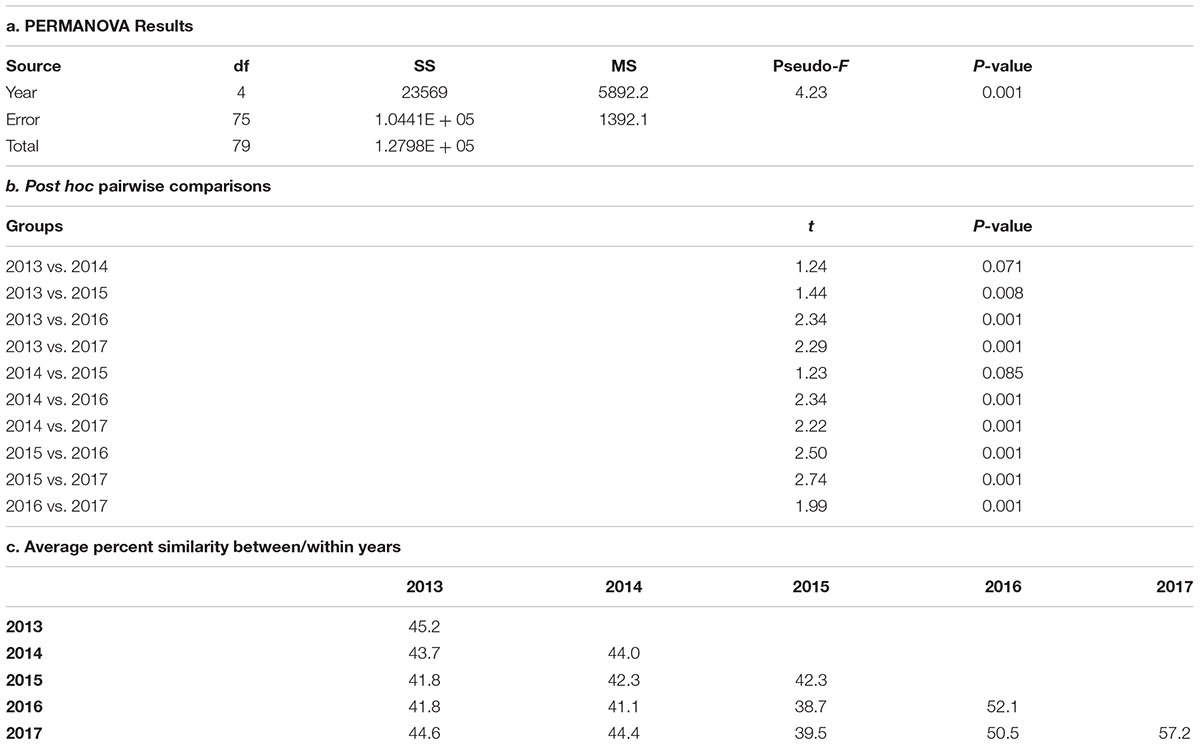
TABLE 3. Results of a PERMANOVA testing differences in fish community structure across 5 years at the site of the Hui Feng shipwreck.
Discussion
After the Hui Feng shipwreck was removed from the shallow reef of Palmyra atoll in 2013, there was a decline in the density and percent cover of the corallimorpharian, Rhodactis howesii, from the reef surrounding the wreck site (Work et al., 2018). Here we show that there was a corresponding shift in fish community composition, and in particular that the biomass of grazing scarids and acanthurids increased while the biomass of chaetodontids declined, especially directly adjacent to the wreck site. Similar patterns were not observed at reference sites in nearby areas of the reef terrace and backreef, indicating that this was a localized effect which we hypothesize to be in response to changes in benthic resources.
This current study documents a two-part transition in the fish community that appears to be shaped by changes in the benthic assemblage through time. First, abundant corallimorphs seemed to stimulate an increase in chaetodontids. While we don’t have data prior to the corallimorph invasion to compare chaetodontid abundance before to after the phase shift, comparisons with reference sites show highly elevated chaetodontid abundance in the initial part of the study period while corallimorph density was very high, and declining abundance in recent years as corallimorph is receding. Interestingly, chaetodontids are often used as indicators coral reef health, since many species are specialists or obligate consumers of scleractinian coral polyps (Russ and Leahy, 2017). However, in this case chaetodontid biomass was high in the degraded state with high corallimorph cover and very low coral cover. Chaetodontid biomass at the wreck site is dominated by the raccoon butterflyfish, Chaetodon lunula, in all years of the study period. This species has been shown to be a dietary generalist relative to other chaetodontids (Pratchett, 2005, 2007), and has been observed to feed on corallimorph polyps in Palmyra (Work et al., 2018, and authors’ personal observation).
The secondary response occurred within the grazer community, once the wreck was removed and corallimorph density began to decline. The reduction in cover of corallimorph opened up bare space which could then be colonize by early successional benthic species, and this corresponded with increases in scarid and acanthurid biomass at the wreck site. This result is consistent with other studies that have linked increases in grazer biomass to increases in bare substrate that are open for colonization by algae and cyanobacteria (Schroeder et al., 2008; Adam et al., 2011; Han et al., 2016; Holbrook et al., 2016). The most notable increases in biomass and abundance were observed in the excavating parrotfishes Chlorurus microrhinos and C. sordidus which are both common at Palmyra. Recent evidence suggests that large, excavating parrotfish species preferentially target early successional cyanobacterial protein sources to sustain their rapid growth and frequent reproduction (Clements et al., 2017) which is consistent with their increases in areas recently cleared of corallimorph. There were also increases, to a lesser magnitude, in the abundance of smaller scraping parrotfishes of the genus Scarus. Within the acanthurids, the most prominent increase was in the common bristletooth, Ctenochaetus striatus. This species has been classified as a detritivore but it has been shown that they also consume a substantial amount of turfing algae (Marshell and Mumby, 2012). Grazing fishes can be critical for maintaining low levels of benthic algae after a disturbances that decreases coral cover. By keeping algae cropped, grazers facilitate reef recovery by maintaining bare space and CCA, which constitute settlement substrate for corals (Belliveau and Paul, 2002; Mumby et al., 2006; Hughes et al., 2007; Smith et al., 2010; Han et al., 2016). When grazers are excluded, macroalgae can proliferate and suppress rates of coral recruitment, retarding reef recovery (Kuffner et al., 2006; Hughes et al., 2007).
In the time frame since the removal of the shipwreck at Palmyra, there has not been a noticeable recovery of corals in the affected area. However, with slow growth rates and variable recruitment rates of corals, it may take several years to detect a change in coral cover, especially since coral cover had been so severely depressed locally by the corallimorph. Future work will track benthic succession and coral recruitment on the reef surrounding the wreck with experimentally manipulated levels of herbivory exposure, and continued monitoring will track long-term changes in the benthic and fish communities.
Though costly and logistically challenging, particularly in remote locations, removal of ship wreckage and other point sources of metallic pollutants can have promising restorative effects on shallow coral reefs, even in cases where removals occur long after occurrence of the grounding. These effects may be even stronger in remote and “pristine” environments, where intact fish communities have a high capacity to respond to even dramatic shifts in benthic assemblages. The current study provides an example of a rapid and strong response of an unfished fish assemblage to a benthic phase shift likely triggered by management action. This and other work demonstrating the potential for herbivorous fishes to prevent and reverse macroalgal phase shifts provide an optimistic view of the recovery potential for Palmyra’s reefs.
Author Contributions
JC conceived of the study. All authors conducted the study and provided editorial advice. KD and JC performed the analysis. KD wrote the manuscript.
Funding
This work is funded by the Marisla Foundation, award number 1-16-058/1. This is publication number 145 from the Palmyra Atoll Research Consortium.
Conflict of Interest Statement
The authors declare that the research was conducted in the absence of any commercial or financial relationships that could be construed as a potential conflict of interest.
Acknowledgments
We would like to thank the Palmyra Atoll National Wildlife Refuge, U.S. Fish and Wildlife Service, Department of the Interior and The Nature Conservancy for permitting and facilitating this work. Thank you to the Palmyra Atoll Research Consortium for scientific collaboration. Thanks to Jacob Eurich and Scott Clark for assisting with data collection.
References
Adam, T. C., Schmitt, R. J., Holbrook, S. J., Brooks, A. J., Edmunds, P. J., Carpenter, R. C., et al. (2011). Herbivory, connectivity, and ecosystem resilience: response of a coral reef to a large-scale perturbation. PLoS One 6:23717. doi: 10.1371/journal.pone.0023717
Belliveau, S. A., and Paul, V. J. (2002). Effects of herbivory and nutrients on the early colonization of crustose coralline and fleshy algae. Mar. Ecol. Progr. Ser. 232, 105–114. doi: 10.3354/meps232105
Bradley, D., Conklin, E., Papastamatiou, Y. P., Mccauley, D. J., Pollock, K., Pollock, A., et al. (2017). Resetting predator baselines in coral reef ecosystems. Sci. Rep. 7:43131. doi: 10.1038/srep43131
Carter, A. L. (2014). Invasion Mechanisms of the Corallimorph, Rhodactis howesii, at Palmyra Atoll. San Diego, CA: University of California, San Diego.
Chadwick-Furman, N. E., and Spiegel, M. (2000). Abundance and clonal replication in the tropical corallimorpharian Rhodactis rhodostoma. Invertebr. Biol. 119, 351–360. doi: 10.1111/j.1744-7410.2000.tb00103.x
Clements, K. D., German, D. P., Piché, J., Tribollet, A., and Choat, J. H. (2017). Integrating ecological roles and trophic diversification on coral reefs: multiple lines of evidence identify parrotfishes as microphages. Biol. J. Linnean Soc. 120, 729–751. doi: 10.1111/bij.12914
Collen, J., Garton, D., and Gardner, J. (2009). Shoreline changes and sediment redistribution at Palmyra Atoll (Equatorial Pacific Ocean): 1874–present. J. Coastal Res. 25, 711–722. doi: 10.2112/08-1007.1
Davis, K., Carlson, P. M., Bradley, D., Warner, R. R., and Caselle, J. E. (2017). Predation risk influences feeding rates but competition structures space use for a common Pacific parrotfish. Oecologia 184, 139–149. doi: 10.1007/s00442-017-3857-9
Dawson, E. (1959). Changes in Palmyra Atoll and its vegettion through the activities of man, 1913-1958. Pac. Natural. 1, 1–52.
DeMartini, E. E., Friedlander, A. M., Sandin, S. A., and Sala, E. (2008). Differences in fish-assemblage structure between fished and unfished atolls in the northern Line Islands, central Pacific. Mar. Ecol. Progr. Ser. 365, 199–215. doi: 10.3354/meps07501
Edwards, C. B., Friedlander, A., Green, A., Hardt, M., Sala, E., Sweatman, H., et al. (2014). Global assessment of the status of coral reef herbivorous fishes: evidence for fishing effects. Proc. R. Soc. Lond. B Biol. Sci. 281, 20131835. doi: 10.1098/rspb.2013.1835
Friedlander, A. M., Caselle, J. E., Ballesteros, E., Brown, E. K., Turchik, A., and Sala, E. (2014). The real bounty: marine biodiversity in the pitcairn islands. PLoS One 9:e100142. doi: 10.1371/journal.pone.0100142
Froese, R., and Pauly, D. (2009). FishBase: A Global Information System on Fishes. Manila: International Center for Living Acquatic Resources Management.
Han, X., Adam, T. C., Schmitt, R. J., Brooks, A. J., and Holbrook, S. J. (2016). Response of herbivore functional groups to sequential perturbations in Moorea, French Polynesia. Coral Reefs 35, 999–1009. doi: 10.1007/s00338-016-1423-2
Heenan, A., Ayotte, P., Gray, A., Lino, K., Mccoy, K., Zamzow, J., et al. (2014). Ecological Monitoring 2012–2013: Reef Fishes and Benthic Habitats of the Main Hawaiian Islands, American Samoa, and Pacific Remote Island Areas. PIFSC Data Report DR-14-003. Honolulu, HI: Pacific Islands Fisheries Science Center. doi: 10.7289/V55H7D81
Hempson, T. N., Graham, N. A. J., Macneil, M. A., Hoey, A. S., and Wilson, S. K. (2018). Ecosystem regime shifts disrupt trophic structure. Ecol. Appl. 28, 191–200. doi: 10.1002/eap.1639
Holbrook, S. J., Schmitt, R. J., Adam, T. C., and Brooks, A. J. (2016). Coral reef resilience, tipping points and the strength of herbivory. Sci. Rep. 6:35817. doi: 10.1038/srep35817
Hughes, T. P., Graham, N. A., Jackson, J. B., Mumby, P. J., and Steneck, R. S. (2010). Rising to the challenge of sustaining coral reef resilience. Trends Ecol. Evol. 25, 633–642. doi: 10.1016/j.tree.2010.07.011
Hughes, T. P., Rodrigues, M. J., Bellwood, D. R., Ceccarelli, D., Hoegh-Guldberg, O., Mccook, L., et al. (2007). Phase shifts, herbivory, and the resilience of coral reefs to climate change. Curr. Biol. 17, 360–365. doi: 10.1016/j.cub.2006.12.049
Kelly, L. W., Barott, K. L., Dinsdale, E., Friedlander, A. M., Nosrat, B., Obura, D., et al. (2012). Black reefs: iron-induced phase shifts on coral reefs. ISME J. 6:638. doi: 10.1038/ismej.2011.114
Kenyon, J. C., Maragos, J. E., and Cooper, S. (2010). Characterization of coral communities at Rose Atoll, American Samoa. Atoll Res. Bull. 586, 1–28. doi: 10.5479/si.00775630.586.1
Knowlton, N. (1992). Thresholds and multiple stable states in coral reef community dynamics. Am. Zool. 32, 674–682. doi: 10.1093/icb/32.6.674
Kuffner, I. B., Walters, L. J., Becerro, M. A., Paul, V. J., Ritson-Williams, R., and Beach, K. S. (2006). Inhibition of coral recruitment by macroalgae and cyanobacteria. Mar. Ecol. Progr. Ser. 323, 107–117. doi: 10.3354/meps323107
Langmead, O., and Chadwick-Furman, N. E. (1999). Marginal tentacles of the corallimorpharian Rhodactis rhodostoma. 2. Induced development and long-term effects on coral competitors. Mar. Biol. 134, 491–500. doi: 10.1007/s002270050565
Lindahl, U., Öhman, M. C., and Schelten, C. K. (2001). The 1997/1998 Mass mortality of corals: effects on fish communities on a Tanzanian coral reef. Mar. Pollut. Bull. 42, 127–131. doi: 10.1016/S0025-326X(00)00167-3
Marshall, P. A., and Edgar, G. J. (2003). The effect of the Jessica grounding on subtidal invertebrate and plant communities at the Galápagos wreck site. Mar. Pollut. Bull. 47, 284–295. doi: 10.1016/S0025-326X(03)00157-7
Marshell, A., and Mumby, P. J. (2012). Revisiting the functional roles of the surgeonfish Acanthurus nigrofuscus and Ctenochaetus striatus. Coral Reefs 31, 1093–1101. doi: 10.1007/s00338-012-0931-y
Muhando, C. A., Kuguru, B. L., Wagner, G. M., Mbije, N. E., and Öhman, M. C. (2002). Environmental effects on the distribution of corallimorpharians in Tanzania. J. Hum. Environ. 31, 558–561. doi: 10.1579/0044-7447-31.7.558
Mumby, P. J., Dahlgren, C. P., Harborne, A. R., Kappel, C. V., Micheli, F., Brumbaugh, D. R., et al. (2006). Fishing, trophic cascades, and the process of grazing on coral reefs. Science 311, 98–101. doi: 10.1126/science.1121129
Norström, A. V., Nyström, M., Lokrantz, J., and Folke, C. (2009). Alternative states on coral reefs: beyond coral–macroalgal phase shifts. Mar. Ecol. Progr. Ser. 376, 295–306. doi: 10.3354/meps07815
Oksanen, J., Kindt, R., Legendre, P., O’hara, B., Stevens, M. H. H., Oksanen, M. J., et al. (2007). The vegan package. Commun. Ecol. Package 10, 631–637.
Pendleton, F. (2012). Rose Atoll 1993 Shipwreck Restoration Status Report. Pago Pago, American Samoa: US Fish and Wildlife Service Report.
Pratchett, M. S. (2005). Dietary overlap among coral-feeding butterflyfishes (Chaetodontidae) at Lizard Island, northern Great Barrier Reef. Mar. Biol. 148, 373–382. doi: 10.1007/s00227-005-0084-4
Pratchett, M. S. (2007). Dietary selection by coral-feeding butterflyfishes (Chaetodontidae) on the Great Barrier Reef, Australia. Raffles Bull. Zool. 14, 171–176.
Precht, W. F., Aronson, R. B., and Swanson, D. W. (2001). Improving scientific decision-making in the restoration of ship-grounding sites on coral reefs. Bull. Mar. Sci. 69, 1001–1012.
R Core Team (2013). R: A language and Environment for Statistical Computing. Vienna: The R Foundation for Statistical Computing.
Riegl, B. (2001). Degradation of reef structure, coral and fish communities in the Red Sea by ship groundings and dynamite fisheries. Bull. Mar. Sci. 69, 595–611.
Russ, G. R., and Leahy, S. M. (2017). Rapid decline and decadal-scale recovery of corals and Chaetodon butterflyfish on Philippine coral reefs. Mar. Biol. 164:29. doi: 10.1007/s00227-016-3056-y
Russ, G. R., Questel, S. L. A., Rizzari, J. R., and Alcala, A. C. (2015). The parrotfish-coral relationship: refuting the ubiquity of a prevailing paradigm. Mar. Biol. 162, 2029–2045. doi: 10.1007/s00227-015-2728-3
Sandin, S. A., Smith, J. E., Demartini, E. E., Dinsdale, E. A., Donner, S. D., Friedlander, A. M., et al. (2008). Baselines and degradation of coral reefs in the Northern Line Islands. PLoS One 3:e1548. doi: 10.1371/journal.pone.0001548
Schroeder, R. E., Green, A. L., Demartini, E. E., and Kenyon, J. C. (2008). Long-term effects of a ship-grounding on coral reef fish assemblages at Rose Atoll, American Samoa. Bull. Mar. Sci. 82, 345–364.
Smith, J. E., Hunter, C. L., and Smith, C. M. (2010). The effects of top–down versus bottom–up control on benthic coral reef community structure. Oecologia 163, 497–507. doi: 10.1007/s00442-009-1546-z
Work, T. M., Aeby, G. S., and Maragos, J. E. (2008). Phase shift from a coral to a corallimorph-dominated reef associated with a shipwreck on Palmyra Atoll. PLoS One 3:2989. doi: 10.1371/journal.pone.0002989
Keywords: Palmyra atoll, shipwreck, reef restoration, reef resilience, herbivory, corallimorph
Citation: Davis K, Carlson PM and Caselle JE (2018) Herbivorous Fish Populations Respond Positively to a Shipwreck Removal and Associated Alteration of Benthic Habitat. Front. Mar. Sci. 5:406. doi: 10.3389/fmars.2018.00406
Received: 20 August 2018; Accepted: 15 October 2018;
Published: 06 November 2018.
Edited by:
Hajime Kayanne, The University of Tokyo, JapanReviewed by:
Carlos Eduardo Leite Ferreira, Universidade Federal Fluminense, BrazilDouglas Fenner, Independent Researcher, Pago Pago, American Samoa
Copyright © 2018 Davis, Carlson and Caselle. This is an open-access article distributed under the terms of the Creative Commons Attribution License (CC BY). The use, distribution or reproduction in other forums is permitted, provided the original author(s) and the copyright owner(s) are credited and that the original publication in this journal is cited, in accordance with accepted academic practice. No use, distribution or reproduction is permitted which does not comply with these terms.
*Correspondence: Kathryn Davis, a2F0aWVkYXZpc0B1Y3NiLmVkdQ==