- 1Department of Chemistry, Life Sciences and Environmental Sustainability, University of Parma, Parma, Italy
- 2Marine Research Institute, University of Klaipeda, Klaipeda, Lithuania
- 3Optical Remote Sensing Group, CNR-IREA, Milan, Italy
- 4Department of Life Sciences and Biotechnology, University of Ferrara, Ferrara, Italy
- 5Department of Biology and Center for Environmental Studies, Virginia Commonwealth University, Richmond, VA, United States
The Curonian Lagoon is Europe’s largest lagoon and one of the most seriously impacted by harmful blooms of cyanobacteria. Intensive studies over the past 20 years have allowed us to identify the major drivers determining the composition and spatial extent of hyperblooms in this system. We summarize and discuss the main outcomes of these studies and provide an updated, conceptual scheme of the multiple interactions between climatic and hydrologic factors, and their influence on internal and external processes that promote cyanobacterial blooms. Retrospective analysis of remote sensed images demonstrated the variability of blooms in terms of timing, extension and intensity, suggesting that they occur only under specific circumstances. Monthly analysis of nutrient loads and stoichiometry from the principal tributary (Nemunas River) revealed large interannual differences in the delivery of key elements, but summer months were always characterized by a strong dissolved inorganic N (and Si) limitation, that depresses diatoms and favors the dominance of cyanobacteria. Cyanobacteria blooms occurred during high water temperatures, long water residence time and low-wind conditions. The blooms induce transient (night-time) hypoxia, which stimulates the release of iron-bound P, producing a positive feedback for blooms of N-fixing cyanobacteria. Consumer-mediated nutrient recycling by dreissenid mussels, chironomid larvae, cyprinids and large bird colonies, may also affect P availability, but their role as drivers of cyanobacteria blooms is understudied.
Introduction
Human activities impact biogeochemical cycles, biological communities and ecosystem functioning of inland and coastal waters on a global scale (Bernot and Dodds, 2005; Muhlolland et al., 2008; Paerl, 2009; Han and Allan, 2012). Estuaries and lagoons have become enriched with nutrients due to wastewater discharge, aquaculture, and agriculture (Galloway et al., 2008; Paerl, 2009). Excess nutrients result in blooms, where algal biomass accumulates and exceeds the mineralization capacity of the heterotrophic community (Valiela et al., 1997). In fresh-brackish waters, algal blooms may include one or more types of harmful cyanobacteria, resulting in the presence of cyanotoxins. The development of hypoxic or anoxic conditions can lead to die-offs of fish and benthic organisms (Norkko and Bonsdorff, 1996; Ye et al., 2011). In addition, changes in food web structure brought about by invasive species may accelerate eutrophication by reducing grazing pressure and allowing the proliferation of algae, including toxic forms (Carpenter et al., 1998; Rabalais et al., 2002). The alteration of nutrient stoichiometry (sensu Redfield) and changes in climate (e.g., warmer temperatures, precipitation timing and intensity) have also received attention as potential drivers of harmful algal blooms (Cloern, 2001; Yunev et al., 2007; Moore et al., 2008; Howarth et al., 2011). In this review, we analyze the drivers of algal blooms in the Curonian Lagoon, a hypereutrophic freshwater estuary. We discuss the relevance of nutrient loads and their stoichiometry on algal blooms, we analyze how algal blooms affect the ecosystem functioning (e.g., nutrient mass balances) and provide a mechanistic interpretation for positive feedbacks promoting the dominance of cyanobacteria.
The Curonian Lagoon: General Features of a Hypertrophic Freshwater Estuary
The Curonian Lagoon is a large (surface area = 1500 km2), shallow (mean depth = 3.5 m) waterbody located along the south-eastern portion of the Baltic Sea (Figure 1). The Curonian Spit (a UNESCO heritage site) divides the lagoon from the Baltic Sea. The main source of water and nutrients is the Nemunas River, although the lagoon also exchanges water with the Baltic Sea via the narrow Klaipeda Strait (Vybernaite-Lubiene et al., 2017). Exchange of water through the strait is episodic; during wind-driven forcing events, the salinity of the lagoon rises to ∼7. The principal tributary (Nemunas River) bisects the lagoon such that the northern lagoon is subject to greater fluvial (and marine) influence, whereas the southern portion of the lagoon is more lentic, and has a longer water residence time (Umgiesser et al., 2016). The lagoon has a relatively small hydrologic loading factor (ratio of watershed area to surface area), which makes this system similar to a flow-through reactor, and provides an opportunity for mass balance studies (Bresciani et al., 2012; Zilius et al., 2014; Vybernaite-Lubiene et al., 2017). Prior work by our multidisciplinary and multinational team has included assessment of nutrient loads from the Nemunas watershed, application of hydraulic models to simulate water circulation in response to changing discharge and wind conditions, investigation of seasonal dynamics of biogeochemical cycles, and use of satellite remote sensing to monitor phytoplankton blooms (Vaičiutė et al., 2015; Petkuviene et al., 2016; Umgiesser et al., 2016; Vybernaite-Lubiene et al., 2017, 2018). Our work at this site has also benefitted from long-term monitoring carried out by the Marine Research Department of the Lithuanian Ministry of Environment.
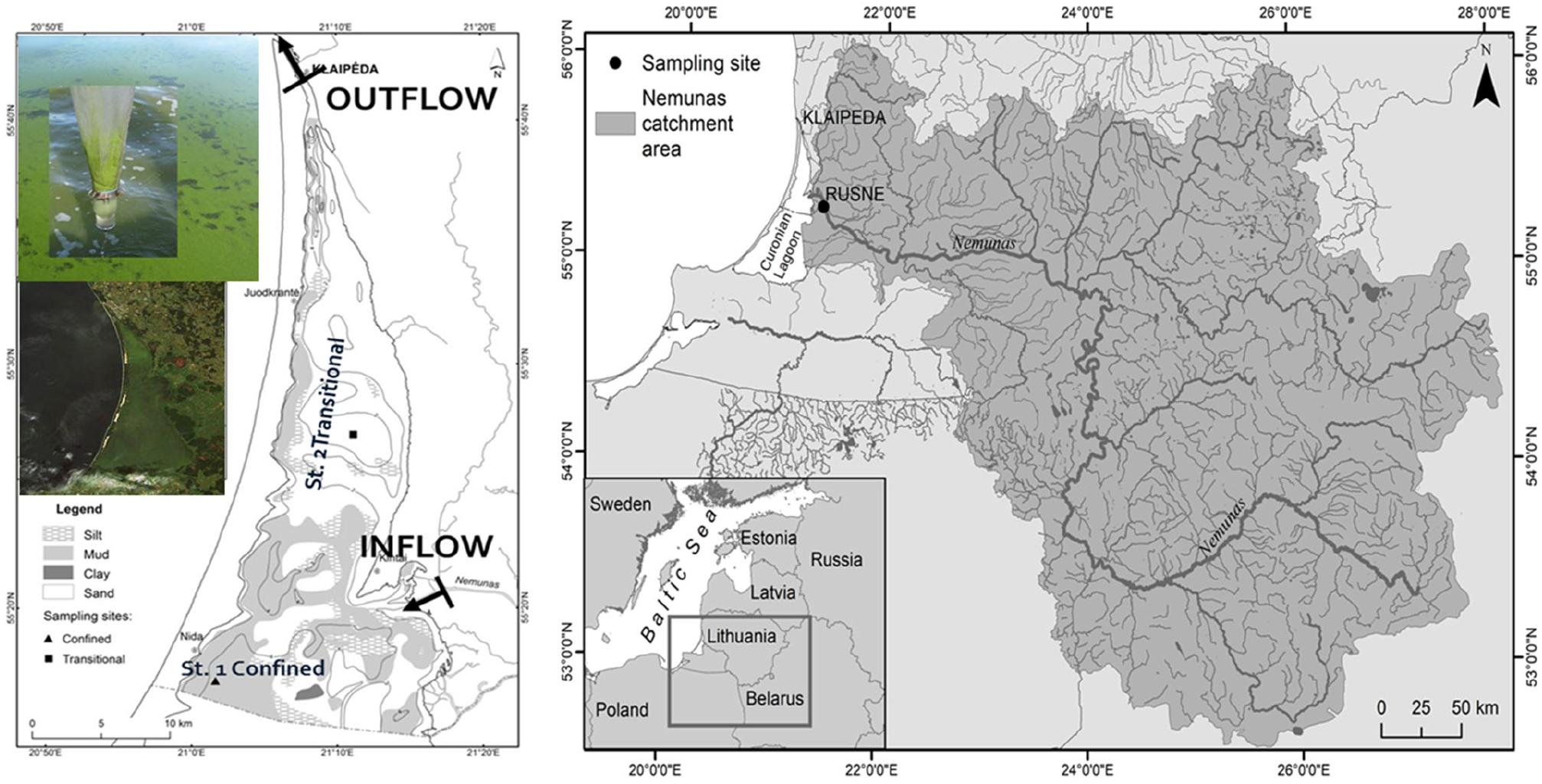
FIGURE 1. Maps of the Nemunas River watershed (right) and the Lithuanian part of the Curonian Lagoon (left). The lagoon map includes water sampling stations at the lagoon inlet and outlet, and the main sediment sampling sites located at the confined muddy and transitional sandy areas. Color photos show the Curonian Lagoon during a summer cyanobacteria bloom.
Data arising from these efforts have helped to guide management of the Curonian Lagoon via a number of national and international programs (HELCOM Baltic Sea Action Plan, European Water Framework Directive, various habitat and bird conservation initiatives). Despite the intensive studies carried out in the lagoon, there remains the question whether and how cyanobacteria blooms can be mitigated. These blooms extend over large areas of the lagoon and negatively impact ecosystem functions, including tourism and recreational activities, as well as local fisheries (Giardino et al., 2010; Belykh et al., 2013; Šulčius et al., 2015). The use of science for informing management decisions is dependent upon the interpretation and integration of available data, which is the focus of this paper.
Seasonal Succession of Phytoplankton
Detailed studies of plankton communities in the Curonian Lagoon have examined seasonal patterns, species interactions, production of cyanotoxins and the role of phytoplankton in food web energetics (e.g., Pilkaitytė and Razinkovas, 2006, Razinkovas, 2007; Lesutienė et al., 2014; Bukaveckas et al., 2017). Diatoms dominate the spring phytoplankton community, after which, following a short clear-water phase, cyanobacteria biomass increases (Gasiunaitė et al., 2005; Pilkaitytė and Razinkovas, 2007). Fresh-brackish species dominate the phytoplankton community of the Curonian Lagoon. Stephanodiscus hantzschii, Diatoma tenuis, Aulacoseira islandica, Asterionella formosa are the dominant diatom species during spring while the N-fixing cyanobacteria Aphanizomenon flosaquae, Dolichospermum affine, D. flosaquae, as well as other cyanobacteria such as Microcystis aeruginosa, M. wesenbergii, M. viridis, and Planktothrix agardhii contribute to the summer biomass peak (Pilkaitytė and Razinkovas, 2007; Gasiunaitė et al., 2008). According to long-term monitoring data (2001–2012), monthly average chlorophyll a (chl-a) concentrations reach 47 ± 14 mg m-3 during the spring diatom bloom and 96 ± 56 mg m-3 during the summer bloom (Marine Research Department of the Lithuanian Ministry of Environment).
Wind Effects on Algal Bloom Development
Algal blooms in the Curonian Lagoon have been tracked since the 1930’s via synoptic sampling (Schmidt-Ries, 1940). More recently, satellite remote sensing has substantially improved our ability to track the spatial and temporal dynamics of bloom events and draw links to local weather conditions. The first attempt to map algal blooms in the Curonian Lagoon utilized the MEdium Resolution Imaging Spectrometer (MERIS) on board the Envisat-1 satellite (Giardino et al., 2010; Bresciani et al., 2012; Vaičiutė et al., 2015). The combination of high spatial resolution (300 m) and short revisit time (2–3 days) greatly enhanced our ability to map chl-a. More recently, the Operational Land Imager (OLI, on board Landsat-8) and Multispectral Instrument (MSI, on board Sentinel-2A/B) have further enhanced spatial resolution (10–30 m) and allowed us to investigate the patchy distribution of cyanobacteria blooms (INFORM, 2017).
Results based on a large number of images from 2004 to 2016 revealed temporal variability and small-scale spatial patchiness of chl-a (Bresciani et al., 2012, 2014; Vaičiutė et al., 2015). The southern part of the lagoon exhibited high chl-a (up to 500 mg m-3) while the northern areas were characterized by lower values (∼50 mg m-3) (Bresciani et al., 2012; Figure 2). Differences between the northern and southern portions of the lagoon were documented by earlier studies (Olenina, 1998; Krevs et al., 2007). However, the use of satellite images allowed us to identify hot spots of chl-a (up to 400 mg m-3) and the presence of surface scums (e.g., Bresciani et al., 2014; Figure 2). Highest concentrations were coincident with prevailing wind conditions, suggesting that wind speed and direction was a significant driver for spatial distribution of positively buoyant cyanobacteria (Bresciani et al., 2014).
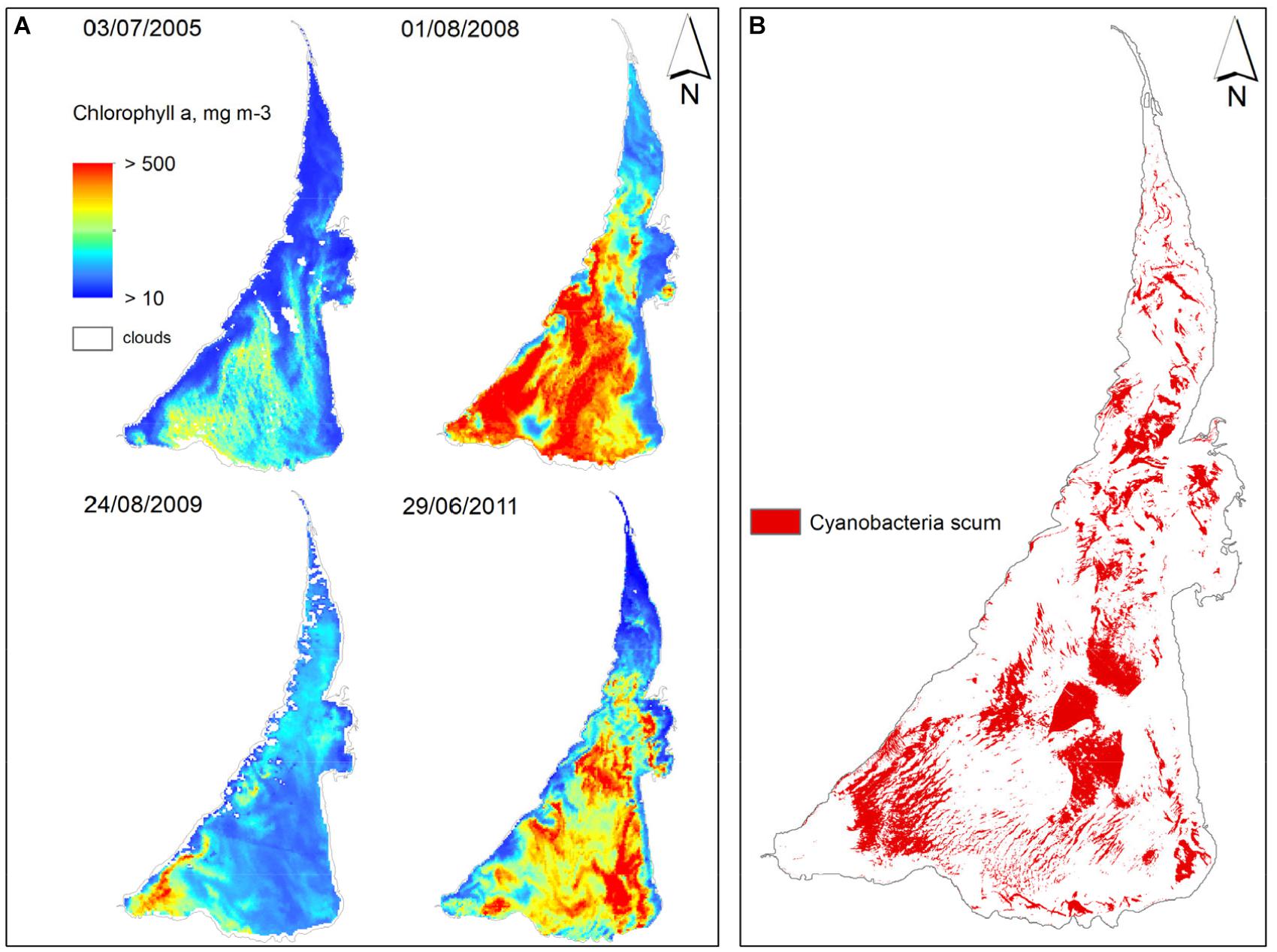
FIGURE 2. Maps of Chl-a concentrations in the Curonian Lagoon from MERIS images (A) and cyanobacteria surface accumulations as retrieved by Landsat-8 OLI and Sentinel-2 MSI during June-September 2013-2016 (B).
Wind speed affects not only the spatial distribution of cyanobacteria, but also influences water column mixing. Wind speeds less than 2 m s-1 are common and allow for the development of transient (daytime) thermal gradients within the water column (Zilius et al., 2014). The lagoon, though shallow, is relatively turbid and it is thought that stagnant conditions associated with low wind allow positively buoyant cyanobacteria to obtain favorable, near-surface light conditions. The combination of remote sensing, in situ biogeochemical studies, and local meteorological data allowed us to investigate these linkages over large spatial scales. Measurements of benthic and pelagic oxygen metabolism along with spatial patterns of MERIS-derived chl-a showed that 60–95% of the area of the lagoon was vulnerable to transient hypoxia when blooms coincide with calm conditions (Zilius et al., 2014).
Hydrodynamic Factors as Drivers of Blooms
Freshwater inputs to the lagoon are dominated by the Nemunas River, which has an annual average discharge of 21.8 km3, and accounts for 96% of total inputs (Jakimavičius and Kriaučiunienė, 2013). The Nemunas River discharges into the central part of lagoon, dividing the system in a northern and a southern region that differ in water renewal time (Umgiesser et al., 2016). The northern part of the lagoon is characterized by strong riverine influence and short renewal time ( < 80 days), which result in limited accumulation of suspended matter (Ferrarin et al., 2008; Remeikaitė-Nikienė et al., 2016). The southern part of the lagoon has a longer water residence time ( > 190 days) with minimal fluvial influence. The latest efforts to analyze the water exchange within the Curonian Lagoon (Umgiesser et al., 2016) revealed different seasonal patterns of residence time, primarily driven by changes in hydrographic forcing by the Nemunas River. During elevated spring discharge, the entire lagoon is strongly flushed by Nemunas River. During summer, river discharge decreases, resulting in increased water residence time, particularly in the southern lagoon. Summer stagnation has implications for water temperature, stratification, nutrient availability and stoichiometry, and phytoplankton abundance and composition. In summer, wind forcing appears to be the most important factor influencing water column mixing and exchange between the southern and the northern part of the lagoon (Umgiesser et al., 2016).
Nutrient Loads and Their Ecological Stoichiometry
A study coupling the Curonian Lagoon with its watershed was started in 2012 to better characterize the timing of nutrient inputs and their stoichiometry. From 2012, on at least a monthly basis and more frequent (weekly) during high discharge periods, discharge and water chemistry (including all dissolved and particulate forms of N, Si, and P ) were monitored near the inflow of the Nemunas River to the lagoon (Vybernaite-Lubiene et al., 2017; Figure 1). Discharge and nutrient concentrations displayed strong seasonality. Nitrate and reactive Si concentrations decreased by two orders of magnitude from spring to summer (e.g., NO3- from > 300 μM to < 1, SiO2 from > 200 to < 1 μM) while reactive P concentrations showed comparatively smaller changes (from 0.2 to 4 μM). These seasonal patterns resulted in reduced DIN:DSi and DIN:DIP ratios, which shift the lagoon from an excess of N and Si in colder months, to P excess (DIN:DIP < 16) in warmer months (Figure 3). These findings support the hypothesis that cyanobacterial blooms are favored during summer by the limited N and Si supply via riverine inputs (Pilkaitytė and Razinkovas, 2007).
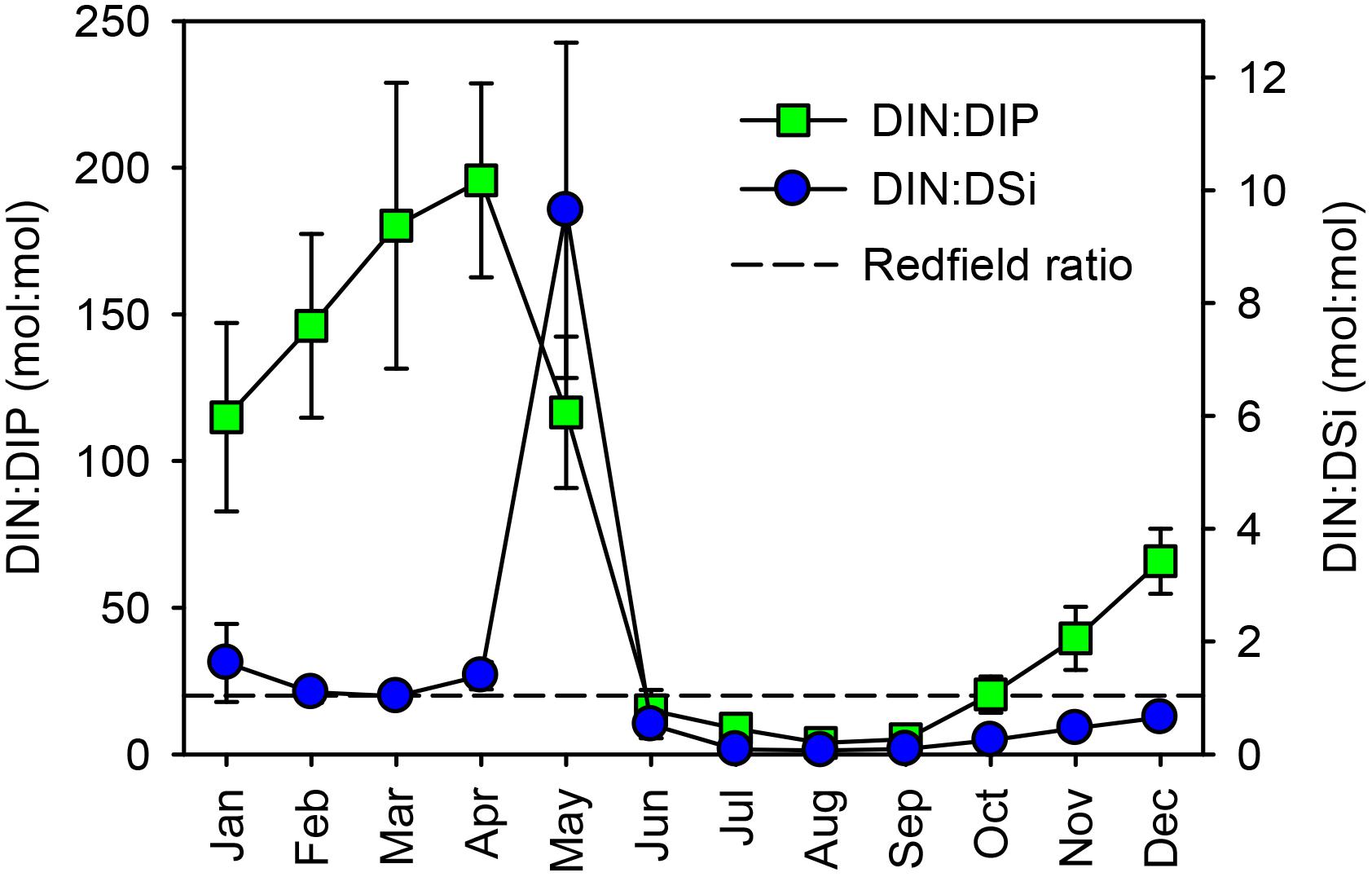
FIGURE 3. The stoichiometric DIN:DIP and DIN:DSi ratios during 2012–2016 at the Nemunas River gaging station. Dashed lines indicate the theoretical Redfield ratio (DIN:DSi = 16:15 and DIN:DIP = 16:1). Averages ± standard deviations (n = 6) are reported.
Our analyses of nutrient loads showed that recent (2012–2016) N export from the Nemunas River basin is similar to historical data (1986–2002), whereas P loads have declined by nearly 60 % as a result of sewage treatment plant improvements (Vybernaite-Lubiene et al., 2018). Despite reductions in P loads, the lagoon remains imbalanced with an excess of P relative to N, thereby favoring the growth of N-fixing cyanobacteria. Further P reductions are needed to promote- limitation or co- limitation and thereby diminish the dominance of cyanobacteria. It is also important to stress that despite strong N limitation in the lagoon, hyperblooms of cyanobacteria do not occur every summer due to the influence of other factors that regulate blooms.
Sediments and Benthic Processes as Drivers of Blooms
The distribution of sediment types in the Curonian Lagoon is determined by hydrodynamic factors and by contributions from autochthonous and allochthonous materials (Pustelnikovas, 1994; Ferrarin et al., 2008). Curonian Lagoon sediments include a broad spectrum of deposits, from sand-dominated in the northern (riverine-influenced) sector to silt-dominated in the southern (more lentic) area (Trimonis et al., 2003). Declines in external (riverine) loads during the transition from spring to summer enhances the importance of internal recycling from the benthic compartment as a nutrient source for pelagic primary production. Studies of sedimentary processes revealed a shift in dominant microbial processes and benthic fluxes from spring to summer (Zilius et al., 2012, 2014; Petkuviene et al., 2016). For example, net N2 production suggests the dominance of denitrification over N-fixation during spring; however N2 fluxes are reversed during summer, suggesting net N import to the benthic compartment (Zilius et al., 2018).
Since 2009, oxygen penetration depth, total and diffusive sedimentary oxygen demand, pore water chemical environments, sedimentary pools and benthic fluxes were measured or calculated at sites representative of dominant sedimentary environments including littoral, pelagic transitional and confined zones (Zilius et al., 2012; Figure 1). In this turbid system, benthic photosynthesis was measurable only in shallow littoral illuminated sediments (∼1 m depth) representing a minor fraction (5%) of the total lagoon surface (Benelli et al., 2018). Here, benthic algae oxidize the upper sediment layer and efficiently retain nutrients, thereby impeding regeneration to the water column (Zilius et al., 2012; Benelli et al., 2018). Deeper sites were always heterotrophic and their seasonal oxygen metabolism and nutrient regeneration was driven by water temperature and phytoplankton blooms; recently settled fresh phytoplankton resulted in significantly higher oxygen uptake, limited oxygen penetration in sediments ( < 1 mm), and high rates of anaerobic to aerobic metabolism (Zilius et al., 2012, 2016).
The mechanisms underlying P release from sediments were analyzed in detail, as they contribute to lower inorganic DIN:DIP ratio in the water column and favor cyanobacteria (Zilius et al., 2014, 2015, 2016; Petkuviene et al., 2016). The distribution of sedimentary pools of P, Fe, Mn and S in the Curonian Lagoon was related to riverine influence; sandy sediments adjacent the Nemunas delta were oxidized and have a large geochemical buffer capacity against the effects of anoxia, with limited accumulation of free sulfide (Petkuviene et al., 2016). Muddy areas along the western and southern portion of the lagoon had chemically reduced sediments where the reduction of iron may result in large P release (Petkuviene et al., 2016). In manipulative experimental studies, simulated deposition of phytoplankton material, primarily composed of cyanobacteria, resulted in an increase of dissimilative nitrate reduction over denitrification and large methane production, but with limited reactive P release (Zilius et al., 2016). Experimental manipulations of intact cores, targeting short-term effects of anoxia, revealed that pools of detritial Ca bound P ( > 70 % of inorganic P) and oxidized Fe and Mn, prevent or buffer redox-dependent reactive P release from sediments (Zilius et al., 2015). However, seasonal measurements of reactive P fluxes at sandy and muddy areas revealed large summer P release at muddy sites coinciding with the occurrence of cyanobacterial blooms, and the onset of hypoxia and anoxia in the water column (Zilius et al., 2014; Petkuviene et al., 2016). These events occurred under specific conditions during prolonged stable weather, with no wind and high water temperature. Benthic P release occurred when the oxidized pools of metals within sediments were exhausted and contributed to the imbalanced stoichiometry by further lowering the DIN:DIP ratio. P regeneration from sediment, despite occurring over short period, had a significant effect on the lagoon P budget, resulting in a large export of P (Petkuviene et al., 2016).
Top-Down Drivers of Blooms
Zooplankton
The shift from diatom- to cyanobacteria- dominated phytoplankton communities was accompanied by a decline in relative zooplankton grazing. During the spring diatom bloom, maximum consumption by zooplankton corresponded to 34% of NPP (324 μgCL-1d-1), whereas during the summer cyanobacteria bloom grazing decreased to 8 % of NNP (470 μgCL-1d-1) (Figure 4). A similar pattern was observed in the southern part of the lagoon where zooplankton grazing declined from 60% of phytoplankton production during spring to 4 % in summer (Semenova and Aleksandrov, 2009). Despite reduced grazing rates, stable isotope studies show that cyanobacteria blooms support secondary production in a diverse group of benthic and pelagic consumers within the lagoon (Lesutienė et al., 2014). Our studies also show that cyanotoxins (microcystin) are found in tissues of fish and shellfish, indicating that cyanobacteria production supports higher trophic levels in this system (Bukaveckas et al., 2017).
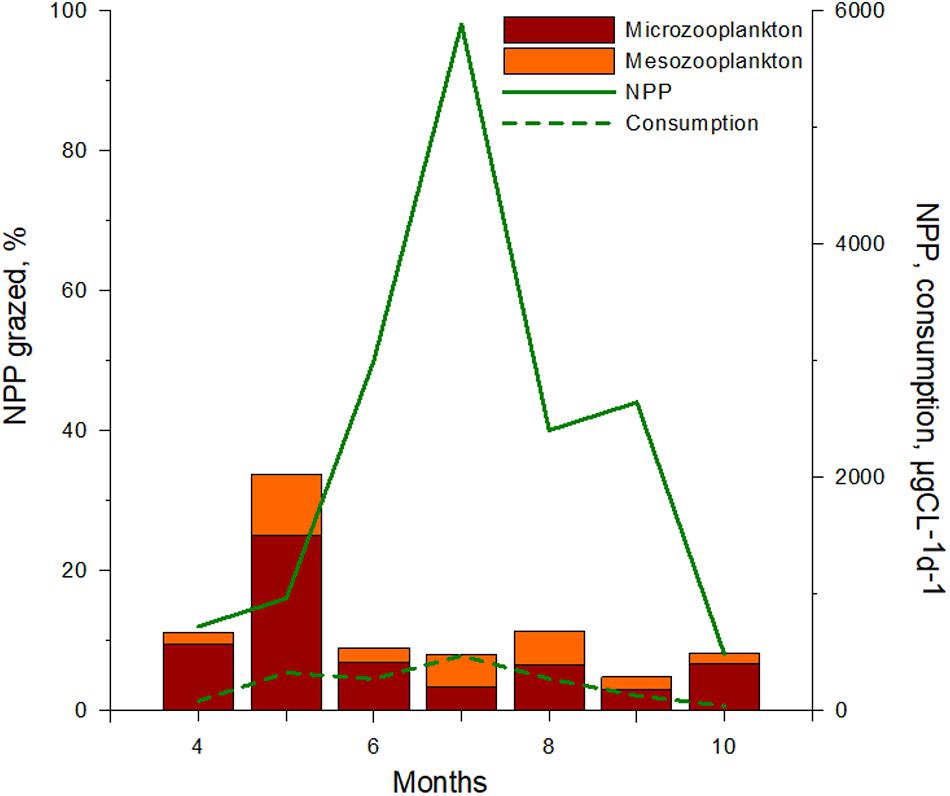
FIGURE 4. Dynamics of net primary production and consumption by zooplankton (NPP, consumption, μgCL-1d-1), and estimated grazed NPP (%) by micro- and mesozooplankton during vegetative season in the Curonian Lagoon.
Grazing by zooplankton may be an important driver of cyanobacteria bloom development. During winter, ciliate growth is limited by low biomass of phytoplankton. In the early spring, when small-sized phytoplankton are dominant, the ciliate assemblage was dominated by small naked oligotrichs and prostomatids. After the late spring diatom bloom, the ciliate assemblage shifted to medium sized tintinnids, which feed on the same nano-fraction of phytoplankton or/and heterotrophic flagellates as ciliates. The summer/autumn phase was characterized by increased taxonomical and functional diversity of ciliates indicating exploitation of a wide size range of food. Small sized naked oligotrichs (Strobilidium spp.) and peritrichs (Vorticella spp.) (mainly bacterivorous ciliates) dominated in summer, indicating a shift from algal food to bacteria (Grinienė, 2013; Grinienė et al., 2016). The shift from large Daphnia to small-bodied Chydorus sphaericus coincides with the dominance of cyanobacteria (Gasiunaitė and Razinkovas, 2004; Figure 5). Chydorus graze on smaller algae and therefore give an advantage to large cyanobacteria (Gasiunaitė and Olenina, 1997). In addition, the presence of large filamentous colonies and toxic strains may foster the dominance of bloom forming cyanobacteria (Pilkaitytė and Razinkovas, 2007).
Macrofauna
Excluding the littoral zone, sediments of the Curonian Lagoon host few macrofauna species due to high organic content and poor oxygen conditions (Zettler and Daunys, 2007). Among them, oligochaetes, chironomid larvae and freshwater mussels, including native unionids and invasive dreissenids, are dominant groups (Daunys, 2001). Chironomid larvae and mussel aggregations may, due to their high densities, influence phytoplankton composition and abundance (Dame et al., 1980; Officer et al., 1982; Gili and Coma, 1998). We discuss here if and under which circumstances macrofauna may favor the onset of cyanobacterial blooms in the Curonian Lagoon.
In the lagoon, periods of short water residence time may impede efficient removal of particulate matter by suspension feeding. In spring (average residence time 7 days) only 10 % of particulate matter was removed by zebra mussels, while in summer (average residence time up to 15 days), the proportion of particulate matter removed increased to 30% (Daunys et al., 2006). Chironomid larvae and mussels may exert a top-down control of pelagic primary production but they may simultaneously excrete large amount of nutrients. Their activities also enhance the organic matter content of sediment via biodeposition, stimulating microbial activity and re-mineralization (Caraco et al., 1997; Stief, 2013; Ruginis et al., 2014; Benelli et al., 2017). It is unclear whether the net effect of phytoplankton removal via grazing is offset by nutrient regeneration via excretion and whether these processes have a specific benefit to cyanobacteria. Dreissena polymorpha was intensively studied due to its top-down control on phytoplankton and the possible management of its biomass to reverse eutrophication. However, such top-down control on pelagic primary production resulted to be site-specific and context-dependent (e.g., in shallow, well-mixed environments with low nutrient background more than in deep, stratified ecosystems with high nutrient inputs) (Conroy et al., 2005; Caraco et al., 2006). Furthermore, dreissenids excrete large amounts of reactive P and different authors have suggested that these mussels may change nutrient stoichiometry, via P mobilization and by enhancing N removal via denitrification (Zhang et al., 2008; Ruginis et al., 2014). The inability of zebra mussels to graze on larger forms of cyanobacteria may provide a competitive advantage over other algae, which, in combination with increased rates of reactive P re-cycling, enhances the potential for cyanobacteria blooms. These aspects need further study, but suggest that the presence of dreissenids mussels on the Curonian Lagoon may exacerbate the effects of nutrient loading, and favor increased dominance by cyanobacteria.
Birds
The Curonian Lagoon hosts a large bird community, including tufted ducks and common pochards with 24,500–54,700 and 1,800–41,000 individuals, respectively (Stanevičius et al., 2009), goosanders (Žydelis, 2001), cormorants, with more than 10,000 breading birds (Švažas et al., 2011; Dagys and Zarankaitë, 2013), mallards, geese (3,000–6,500 ind/day) and little and black-headed gulls (1,000–1,500 ind./day). High densities of water birds are vectors of seeds, invertebrates, bacteria and phytoplankton (Tobiessen and Wheat, 2000), and also contribute to nutrient loads (Manny et al., 1994; Hahn et al., 2007; Green and Elmberg, 2014; Han et al., 2017).
During nesting, breeding and roosting periods, water birds enrich the water with guano (Klimaszyk et al., 2015). In enclosed aquatic ecosystems bird feces may contribute 50–69%, 27–40%, and 70–75% of total C, N, and P loads, respectively (Manny et al., 1994; Post et al., 1998; Boros et al., 2008; Gwiazda et al., 2014). Bird feces have low N:P, implying that water bird excretions may strengthen N limitation and promote cyanobacteria blooms (Rönicke et al., 2008; Han et al., 2017). Birds also have indirect effects on nutrient cycling by removing macrophytes, invertebrates and fish. Herbivorous birds, by removing plants, remove those elements that trap nutrients in the benthic compartment, provide shelter for zooplankton and allow sediment oxidation via radial oxygen loss. While grazing on macrophytes, birds resuspend sediments and mobilize pore water nutrients (Klimaszyk et al., 2015; Klimaszyk and Rzymski, 2016). Furthermore, a large fraction of macrophyte-associated P is released to the water column in the reactive form and is readily available to phytoplankton. The mechanisms that regulate P mobility in sediments are redox-dependent such that the removal of roots and macrofauna, together with particle resuspension, has the potential to mobilize sediment sources of P. In the Curonian Lagoon herbivorous birds represent the second largest water bird group, peaking in spring and distributed throughout the Nemunas River deltaic area and the littoral zone.
Benthivorous birds, feeding on macrofauna, produce an effect on the benthic system similar to that of fishes, removing animals that may keep the sediment oxidized and resuspending sediments and nutrients (Werner et al., 2005; Sánchez et al., 2006; Rodríguez-Pérez et al., 2007; Matuszak et al., 2014). Piscivorous birds convert fish-associated P into reactive P (Putys and Zarankaitė, 2010). Large colonies of cormorants have their peak activity during summer. The large bird community in the Curonian Lagoon may therefore affect by various direct and indirect mechanisms the cycling of nutrients and that of P in particular.
Fish
Benthivorous fish, including carp, roach, bream and perch, represent the dominant fish component in the Curonian Lagoon (Cline et al., 1994; Persson and Svensson, 2006; Lithuanian Environmental Protection Agency (EPA), 2008; Adámek and Maršálek, 2013). Fish may produce both top-down (e.g., removal of grazers and competitors) and bottom-up effects (nutrient mobilization) that favor eutrophic conditions and cyanobacterial blooms (Shormann and Cotner, 1997; Roozen et al., 2007). The diet of benthivorous fish in the Curonian Lagoon includes mussels, chironomidae larvae, detritus, zooplankton and plants (Bubinas and Ložys, 2000). Benthivorous fish may impact the water quality, leading to nutrient accumulation and algal growth, by suspending the sediments and by feeding on filter-feeding zooplankton, burrowing macrofauna and macrophytes (Zambrano and Hinojosa, 1999; Williams et al., 2002; Parkos et al., 2003). Sediment resuspension by the benthic fish community increases water turbidity, limits light penetration and rooted macrophytes and favors P release and cyanobacteria growth (Hellström, 1991; Breukelaar et al., 1994). By removing invertebrates from the sediments, benthivorous fishes mobilize nutrients from the pore water (Tarvainen et al., 2002; Phan-Van et al., 2008). Resuspension itself may oxidize sediments, but this is a short-term and local effect, while reductions in invertebrate abundance impacts N removal via denitrification and P sequestration. Moreover, fish predation reduces zooplankton populations, resulting in low grazing on phytoplankton (Jeppesen et al., 1999). Fish excretions are very soluble and rich in N and P which stimulate periphyton growth and negatively affect macrophytes (Tarvainen et al., 2002; Williams et al., 2002). Excreted nutrients are dispersed horizontally and vertically and from littoral to pelagic areas (Schindler et al., 1996; Persson and Svensson, 2006).
Synthesis
Cyanobacterial blooms in the Curonian Lagoon arise from multiple interacting factors, which include external forcing (riverine discharge and wind conditions) and internal processes (consumer-mediated nutrient cycling and sediment-water nutrient exchange). We summarize the information discussed in this review through a graphical representation of the multiple mechanisms that drive cyanobacterial blooms in the Curonian Lagoon (Figure 6).
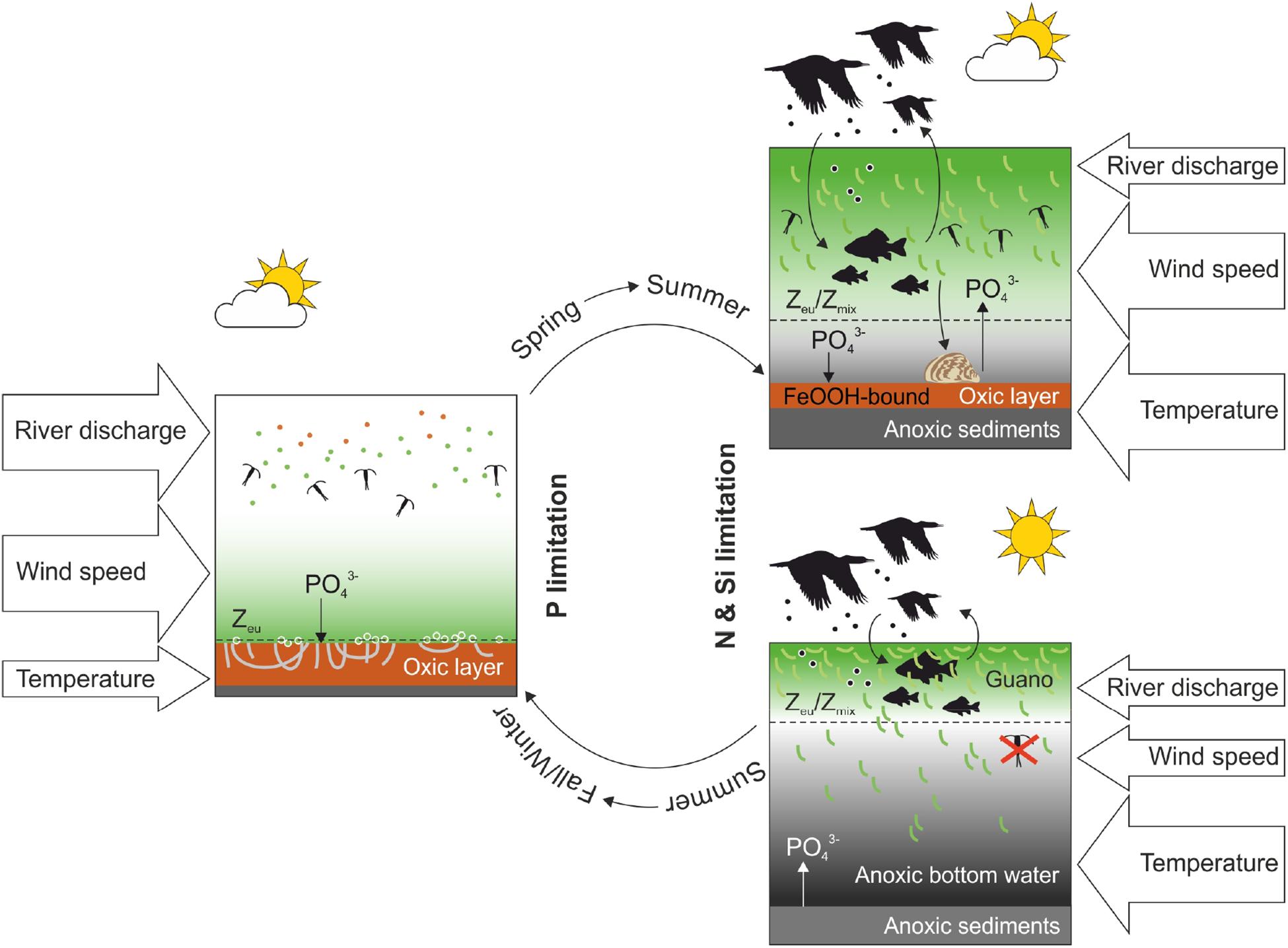
FIGURE 6. Drivers of cyanobacterial blooms in the Curonian Lagoon during the transition from spring to summer. The lower panel on the right represents a hyperbloom event, leading to water anoxia.
During spring, the lagoon is diatom-dominated due to a combination of low water temperatures, high river discharge and availability of inorganic N and Si, in excess to P. The system alternates phases with clear and turbid water depending on the intensity of the spring diatom bloom and the occurrence of wind-associated sediment resuspension events. During spring, light penetration may attain 1–2 m and grazers exert appreciable control of algal biomass accrual. The water column is generally well mixed and normoxic; under these circumstances the upper sediment layer is oxidized and acts as a nutrient sink.
The spring-summer transition is marked by a decline in discharge of the Nemunas River, which is accompanied by the depletion of N and Si within the lagoon. Reductions in external loadings, together with processes occurring within the lagoon (high spring denitrification rates and Si sequestration via uptake and accumulation in sediment), result in the onset of N and Si limitation. Cyanobacteria become dominant, resulting in a series of cascade effects that include increased algal-associated turbidity and water stratification. Positive feedbacks arise as large colonies of cyanobacteria limit the capacity of grazers to control biomass accrual and high respiration rates promote oxygen under-saturation. Climatic conditions, which are highly variable, play an important role, as low wind conditions may further push the system toward hyperbloom events, with extensive surface scums (Figure 7). Water column respiration, not sediment oxygen demand, promote oxygen depletion in the system, due to the large availability of labile organic matter from decaying algal cells. By this mechanism, hyperblooms promote their persistence as hypoxia results in sediment P release. Other factors that may contribute to these large periodic outbreaks include the presence of waterbirds. Large colonies of cormorants settle in spring along the Curonian Spit and have their most intense period of activity during summer. Cormorants, through the production of guano, make large amounts of P available in surface water. Besides cormorants, colonies of seagulls, swans and duck have large numbers and may contribute to make fish or macrophyte P pools readily available to cyanobacteria. Other biological agents supporting algal blooms include the invasive freshwater mussel (D. polymorpha), which excretes large amounts of reactive P relative to the native unionid mussels and may therefore contribute to the low DIN:DIP ratio in the lagoon.
Management Policies and Perspectives
The Curonian Lagoon ecosystem provides a number of provisioning and cultural ecosystem services, most directly linked to the main economic activities in the lagoon area – recreation and fishery (Rashleigh et al., 2012; Razinkovas-Baziukas et al., 2012). The lagoon also provides ecosystem services of relevance to the Baltic Sea region such as denitrification and phosphorus burial. Management efforts to improve water quality in the Curonian Lagoon have targeted reductions of nitrogen loads by 15 % and phosphorus by 8%. Model simulations (Ertürk et al., 2016) revealed that reductions of nitrogen loads by 14 % and phosphorus loads by 6%, will bring about a 10% reduction in the abundance of cyanobacteria (Razinkovas et al., 2008). Further reductions in riverine nutrient loads (40% decrease in both N and P) produced only a 10% decrease in peak chl-a concentrations (Razinkovas et al., 2008). Further efforts to improve water quality may require within-system bioengineering solutions (biomanipulations, mussels, reed harvesting).
Climate change projections for the Curonian Lagoon (Jakimavičius et al., 2018) indicate an increase of average water temperature up to 1.7–2°C by the middle of this century, consistent with trends observed during the last three decades (Dailidienë et al., 2011). According to this modeling study, the increase in water temperature was mostly confined to the summer-early autumn period, which may therefore favor the development of cyanobacteria blooms. Biogeochemical cycles of the Curonian Lagoon will be affected by changes in the water balance of the lagoon. A decline in contributions from Nemunas River coupled with an increase in Baltic water intrusions (due to sea level rise) will alter the water balance during the winter–spring period. A shift in the timing of peak discharge from spring to winter, as observed in recent decades (Dailidienë et al., 2011), may diminish algal blooms if a larger proportion of the nitrogen load from the Nemunas River passes through the Curonian Lagoon during the period of low phytoplankton productivity. However, the predicted decrease of ice cover is expected to reduce winter hypoxia, which would result in reductions in denitrification. Despite the intensive studies of this system, we are not able to predict whether climate change will exacerbate or mitigate cyanobacteria blooms. However, it is apparent that further management actions are needed to reduce nutrient loads and restore ecosystem services. There is a need for additional studies, both at the watershed-scale and the lagoon scale, to facilitate science-based management decision. At the watershed scale, long-term monitoring is needed to better understand the effectiveness of improved agricultural practices and water treatment on N, Si, and P export from the Nemunas basin to the Curonian Lagoon. Watershed practices may differentially affect the three elements further modifying their ecological stoichiometry, with implication for algal blooms (Yunev et al., 2007; Bresciani et al., 2014; Vybernaite-Lubiene et al., 2017).
Conclusion
This review analyzes the available information on the mechanisms driving cyanobacterial blooms in the Curonian Lagoon. Results from our analysis suggest that blooms are a consequence of multiple, interplaying factors, producing a cascade of processes and positive feedbacks. The hot moment for cyanobacteria blooms is the summer, due to combination of favorable nutrient stoichiometry (N and Si limitation), elevated water temperature, low wind speed, unbalanced internal recycling (P > N) and low grazing pressure. The hot spots of cyanobacteria are stagnant areas where limited water circulation and stratification provide these organisms a competitive advantage. These hot spots may serve as bloom initiation areas from which cyanobacteria are dispersed by prevailing winds. Ecological interactions among aquatic organisms, and how these respond to changes in climate and to species invasions remain understudied. The combination of satellite remote sensing, traditional monitoring of environmental parameters, detailed analysis of processes at the macro and microscale and the application of ecological network models, have proved to be useful tools for understanding the mechanisms underlying the development of cyanobacteria blooms. Our further efforts seek to improve our capacity to predict the occurrence and severity of algal blooms and guide prevention measures.
Author Contributions
MBa collected and homogenized the material with the help of MZ, who realized the conceptual scheme of Figure 6 and PB, who organized the different sections and improved the clarity of the paper. All authors contributed to the writing.
Funding
MBr, JP, and DV were supported by Research Council of Lithuania (LMT) grant “Phosphorus as driver of cyanobacterial hyperblooms in the Curonian Lagoon (Patchy)” (Agreement No. S-MIP-17-11), MZ was supported by LMT grant “The role of atmospheric nitrogen fixation in the largest eutroficated European lagoon (NitFix)” (Agreement No. P-MIP-17-126), IV-L and AR-B were supported by the BONUS project “Nutrient Cocktails in Coastal zones of the Baltic Sea (COCOA)” (No. BONUS-2/2014). This work was partly funded by the European Community’s Seventh Framework Program (FP7/2007-2013) under Grant 606865 (INFORM) and by the EOMORES project belonging to the European Union’s Horizon 2020 research and innovation Program (grant agreement n° 730066). Participation in this research effort by PB was aided by a Fulbright award.
Conflict of Interest Statement
The authors declare that the research was conducted in the absence of any commercial or financial relationships that could be construed as a potential conflict of interest.
References
Adámek, Z., and Maršálek, B. (2013). Bioturbation of sediments by benthic macroinvertebrates and fish and its implication for pond ecosystems: a review. Aquac. Int. 21, 1–17. doi: 10.1007/s10499-012-9527-3
Belykh, O. I., Dmitrieva, O. A., Gladkikh, A. S., and Sorokovikova, E. G. (2013). Identification of toxigenic Cyanobacteria of the genus Microcystis in the Curonian Lagoon (Baltic Sea). Oceanology 53, 71–79. doi: 10.1134/S0001437013010025
Benelli, S., Bartoli, M., Racchetti, E., Moraes, P. C., Zilius, M., Lubiene, I., et al. (2017). Rare but large bivalves alter benthic respiration and nutrient recycling in riverine sediments. Aquat. Ecol. 51, 1–16. doi: 10.1007/s10452-016-9590-3
Benelli, S., Bartoli, M., Zilius, M., Vybernaite-Lubiene, I., Ruginis, T., Petkuviene, J., et al. (2018). Microphytobenthos and chironomid larvae attenuate nutrient recycling in shallow-water sediments. Freshw. Biol. 63, 187–201. doi: 10.1111/fwb.13052
Bernot, M. J., and Dodds, W. K. (2005). Nitrogen retention, removal, and saturation in lotic ecosystems. Ecosystems 8, 442–453. doi: 10.1007/s10021-003-0143-y
Boros, E., Nagy, T., Pigniczki, C., Kotymán, L., Balogh, K. V., and Vörös, L. (2008). The effect of aquatic birds on the nutrient load and water quality of soda pans in Hungary. Acta Zool. Acad. Sci. Hung. 54(Suppl. 1), 207–224.
Bresciani, M., Adamo, M., De Carolis, G., Matta, E., Pasquariello, G., Vaièiûtë, D., et al. (2014). Monitoring blooms and surface accumulation of Cyanobacteria in the Curonian Lagoon by combining MERIS and ASAR data. Remote Sens. Environ. 146, 124–135. doi: 10.1016/j.rse.2013.07.040
Bresciani, M., Giardino, C., Stroppiana, D., Pilkaitytë, R., Bartoli, M., and Razinkovas, A. (2012). Retrospective analysis of spatial and temporal variability of chlorophyll-a in the Curonian Lagoon. J. Coast. Conserv. 16, 511–519. doi: 10.1007/s11852-012-0192-5
Breukelaar, A. W., Lammens, E. H. R. R., Klein Breteler, J. G. P., and Ta’trai, I. (1994). Effects of benthivorous bream (Abramis brama) and carp (Cyprinus carpio) on sediment resuspension and concentrations of nutrients and chlorophyll a. Freshw. Biol. 32, 113–121. doi: 10.1111/j.1365-2427.1994.tb00871.x
Bubinas, A., and Ložys, L. (2000). The nutrition of fish in the Curonian lagoon and the coastal zone of the Baltic sea. Acta Zool. Litu. 10, 56–67. doi: 10.1080/13921657.2000.10512346
Bukaveckas, P. A., Lesutienë, J., Gasiûnaitë, Z. R., Ložys, L., Olenina, I., Pilkaitytë, R., et al. (2017). Microcystin in aquatic food webs of the Baltic and Chesapeake Bay regions. Estuar. Coast. Shelf Sci. 191, 50–59. doi: 10.1016/j.ecss.2017.04.016
Caraco, N. F., Cole, J. J., Raymond, P. A., Strayer, D. L., Pace, M. L., Findlay, S. E., et al. (1997). Zebra mussel invasion in a large, turbid river: phytoplankton response to increased grazing. Ecology 78, 588–602. doi: 10.1890/0012-9658 (1997)078[0588:ZMIIAL]2.0.CO;2
Caraco, N. F., Cole, J. J., and Strayer, D. L. (2006). Top-down control from the bottom: regulation of eutrophication in a large river by benthic grazing. Limnol. Oceanogr. 51, 664–670. doi: 10.4319/lo.2006.51.1_part_2.0664
Carpenter, S. R., Caraco, N. F., Correll, D. L., Howarth, R. W., Sharpley, A. N., and Smith, V. H. (1998). Nonpoint pollution of surface waters with phosphorus and nitrogen. Ecol. Appl. 8, 559–568. doi: 10.1890/1051-0761 (1998)008[0559:NPOSWW]2.0.CO;2
Cline, J. M., East, T. L., and Threlkeld, S. T. (1994). Fish interactions with the sediment–water interface. Hydrobiologia 275, 301–311. doi: 10.1007/BF00026721
Cloern, J. E. (2001). Our evolving conceptual model of the coastal eutrophication problem. Mar. Ecol. Prog. Ser. 210, 223–253. doi: 10.3354/meps210223
Conroy, J. D., Edwards, W. J., Pontius, R. A., Kane, D. D., Zhang, H., Shea, J. F., et al. (2005). Soluble nitrogen and phosphorus excretion of exotic freshwater mussels (Dreissena spp.): potential impacts for nutrient remineralisation in western Lake Erie. Freshw. Biol. 50, 1146–1162. doi: 10.1111/j.1365-2427.2005.01392.x
Dagys, M., and Zarankaitë, J. (2013). “Status of the breeding population of Great Cormorants in Lithuania in 2012,” in National Reports from the 2012 Breeding Census of Great Cormorants Phalacrocorax carbo in Parts of the Western Palearctic. IUCN-Wetlands International Cormorant Research Group Report. Technical Report from DCE –Danish Centre for Environment and Energy, eds T. Bregnballe, J. Lynch, R. Parz-Gollner, L. Marion, S. Volponi, J.-Y. Paquet, et al. (Aarhus: Aarhus University), 69–71.
Dailidienë, I., Baudler, H., Chubarenko, B., and Navrotskaya, S. (2011). Long term water level and surface temperature changes in the lagoons of the southern and eastern Baltic. Oceanologia 53, 293–308. doi: 10.5697/oc.53-1-TI.293
Dame, R., Zingmark, R., Stevenson, R., and Nelson, D. (1980). “Filter feeder coupling between the estuarine water column and benthic subsystems,” in Estuarine Perspectives, ed. V. S. Kennedy (San Diego, CA: Academic Press), 521–526. doi: 10.1016/B978-0-12-404060-1.50048-4
Daunys, D. (2001). Patterns of Bottom Macrofauna Variability and its Role in the Shallow Coastal Lagoon. Doctoral dissertation, Klaipeda, Klaipeda University.
Daunys, D., Zemlys, P., Olenin, S., Zaiko, A., and Ferrarin, C. (2006). Impact of the zebra mussel Dreissena polymorpha invasion on the budget of suspended material in a shallow lagoon ecosystem. Helgol. Mar. Res. 60, 113–120. doi: 10.1007/s10152-006-0028-5
Ertürk, A., Razinkovas-Baziukas, A., Zemlys, P., and Umgiesser, G. (2016). Linking carbon-nitrogen-phosphorus cycle and foodweb models of an estuarine lagoon ecosystem. Comput. Sci. Tech. 3, 350–412. doi: 10.15181/csat.v3i1.1093
Ferrarin, C., Razinkovas, A., Gulbinskas, S., Umgiesser, G., and Bliûdžiutë, L. (2008). Hydraulic regime-based zonation scheme of the Curonian Lagoon. Hydrobiologia 611, 133–146. doi: 10.1007/s10750-008-9454-5
Galloway, J. N., Townsend, A. R., Erisman, J. W., Bekunda, M., Cai, Z., Freney, J. R., et al. (2008). Transformation of the nitrogen cycle: recent trends, questions, and potential solutions. Science 320, 889–892. doi: 10.1126/science.1136674
Gasiunaitė, Z. R., Cardoso, A. C., Heiskanen, A. S., Henriksen, P., Kauppila, P., Olenina, I., et al. (2005). Seasonality of coastal phytoplankton in the Baltic Sea: influence of salinity and eutrophication. Estuar. Coast. Shelf Sci. 65, 239–252. doi: 10.1016/j.ecss.2005.05.018
Gasiunaitė, Z. R., Daunys, D., Olenin, S., and Razinkovas, A. (2008). “The Curonian lagoon,” in Ecology of Baltic Coastal Waters, ed. U. Schiewer (Berlin: Springer).
Gasiunaitė, Z. R., and Olenina, I. (1997). Zooplankton-phytoplankton interactions: a possible explanation of the seasonal succession in the Kursiu Marios lagoon. Hydrobiologia 363, 333–339. doi: 10.1023/A:1003161319026
Gasiunaitė, Z. R., and Razinkovas, A. (2004). Temporal and spatial patterns of crustacean zooplankton dynamics in a transitional lagoon ecosystem. Hydrobiologia 514, 139–149. doi: 10.1023/B:hydr.0000018214.93205.32
Giardino, C., Bresciani, M., Pilkaitytė, R., Bartoli, M., and Razinkovas, A. (2010). In situ measurements and satellite remote sensing of case 2 waters: preliminary results from the Curonian Lagoon. Oceanologia 52, 197–210. doi: 10.5697/oc.52-2.197
Gili, J. M., and Coma, R. (1998). Benthic suspension feeders: their paramount role in littoral marine food webs. Trends Ecol. Evol. 13, 316–321. doi: 10.1016/S0169-5347(98)01365-2
Green, A. J., and Elmberg, J. (2014). Ecosystem services provided by waterbirds. Biol. Rev. 89, 105–122. doi: 10.1111/brv.12045
Grinienė, E. (2013). Functional Role of Plankton Ciliates in a Eutrophic Coastal Lagoon. Ph.D. thesis, Klaipëda, Klaipëda University: 123.
Grinienė, E., Šulčius, S., and Kuosa, H. (2016). Size-selective microzooplankton grazing on the phytoplankton in the Curonian Lagoon (SE Baltic Sea). Oceanologia 58, 292–301. doi: 10.1016/j.oceano.2016.05.002
Gwiazda, R., Woźnica, A., Łozowski, B., Kostecki, M., and Flis, A. (2014). Impact of waterbirds on chemical and biological features of water and sediments of a large, shallow dam reservoir. Oceanol. Hydrobiol. Stud. 43, 418–426. doi: 10.2478/s13545-014-0160-9
Hahn, S., Bauer, S., and Klaassen, M. (2007). Estimating the contribution of carnivorous waterbirds to nutrient loading in freshwater habitats. Freshw. Biol. 52, 2421–2433. doi: 10.1111/j.1365-2427.2007.01838.x
Han, H., and Allan, J. D. (2012). Uneven rise in N inputs to the Lake Michigan Basin over the 20th century corresponds to agricultural and societal transitions. Biogeochemistry 109, 175–187. doi: 10.1007/s10533-011-9618-7
Han, I., Yoo, K., Wee, G. N., No, J. H., Park, J., Min, S. J., et al. (2017). Short-term effects of great cormorant droppings on water quality and microbial community of an artificial agricultural reservoir. J. Environ. Qual. 46, 470–476. doi: 10.2134/jeq2016.11.0459
Hellström, T. (1991). The effect of resuspension on algal production in a shallow lake. Hydrobiologia 213, 183–190. doi: 10.1007/BF00016421
Howarth, R., Chan, F., Conley, D. J., Garnier, J., Doney, S., Marino, R., et al. (2011). Coupled biogeochemical cycles: eutrophication and hypoxia in temperate estuaries and coastal marine ecosystems. Front. Ecol. Environ. 9, 18–26. doi: 10.1890/100008
Jakimavičius, D., and Kriaučiunienė, J. (2013). The climate change impact on the water balance of the Curonian Lagoon. Water Resour. 40, 120–132. doi: 10.1134/S0097807813020097
Jakimavičius, D., Kriaučiunienė, J., and Šarauskienė, D. (2018). Impact of climate change on the Curonian Lagoon water balance components, salinity and water temperature in the 21st century. Oceanologia 60, 378–389. doi: 10.1016/j.oceano.2018.02.003
Jeppesen, E., Jensen, J. P., Søndergaard, M., and Lauridsen, T. (1999). Trophic dynamics in turbid and clearwater lakes with special emphasis on the role of zooplankton for water clarity. Hydrobiologia 408–409, 217–231. doi: 10.1007/978-94-017-2986-4_24
Klimaszyk, P., Brzeg, A., Rzymski, P., and Piotrowicz, R. (2015). Black spots for aquatic and terrestrial ecosystems: impact of a perennial cormorant colony on the environment. Sci. Total Environ. 517, 222–231. doi: 10.1016/j.scitotenv.2015.02.067
Klimaszyk, P., and Rzymski, P. (2016). The complexity of ecological impacts induced by great cormorants. Hydrobiologia 771, 13–30. doi: 10.1007/s10750-015-2618-1
Krevs, A., Koreiviene, J., Paskauskas, R., and Sulijiene, R. (2007). Phytoplankton production and community respiration in different zones of the Curonian lagoon during the midsummer vegetation period. Transl. Waters Bull. 1, 17–26. doi: 10.1285/i1825229Xv1n1p17
Lesutienė, J., Bukaveckas, P. A., Gasiunaitė, Z. R., Pilkaitytė, R., and Razinkovas-Baziukas, A. (2014). Tracing the isotopic signal of a Cyanobacteria bloom through the food web of a Baltic Sea coastal lagoon. Estuar. Coast. Shelf Sci. 138, 47–56. doi: 10.1016/j.ecss.2013.12.017
Lithuanian Environmental Protection Agency (EPA) (2008). The Assessment of Ichtiofauna Research and Ecological State According to Fish Indicators in the Curonian Lagoon and Baltic Sea. Available at: http://vanduo.gamta.lt/files/2008m_tyrimu_rezultatu_analizes_ataskaita_Kursiu_mariose_ir_Baltijos_juroje.pdf
Manny, B. A., Johnson, W. C., and Wetzel, R. G. (1994). Nutrient additions by waterfowl to lakes and reservoirs: predicting their effects on productivity and water quality. Hydrobiologia 279–280, 121–132. doi: 10.1007/BF00027847
Matuszak, A., Mörtl, M., Quillfeldt, P., and Bauer, H. G. (2014). Macrophyte-associated macroinvertebrates as an important food source for wintering waterbirds at Lake Constance. Limnology 15, 69–76. doi: 10.1007/s10201-013-0414-z
Moore, S. K., Trainer, V. L., Mantua, N. J., Parker, M. S., Laws, E. A., Backer, L. C., et al. (2008). Impacts of climate variability and future climate change on harmful algal blooms and human health. Environ. Health 7(Suppl. 2):S4. doi: 10.1186/1476-069X-7-S2-S4
Muhlolland, P. J., Helton, A. M., Poole, G. C., Hall, R. O., Hamilton, S. K., Peterson, B. J., et al. (2008). Stream denitrification across biomes and its response to anthropogenic nitrate loading. Nature 452, 203–205. doi: 10.1038/nature06686
Norkko, A., and Bonsdorff, E. (1996). Population responses of coastal zoobenthos to stress induced by drifting algal mats. Mar. Ecol. Prog. Ser. 140, 141–151. doi: 10.3354/meps140141
Officer, C. B., Smayda, T. J., and Mann, R. (1982). Benthic filter feeding: a natural eutrophication control. Mar. Ecol. Prog. Ser. 9, 203–210. doi: 10.3354/meps009203
Olenina, I. (1998). Long-term changes in the Kuršiø Marios Lagoon: eutrophication and phytoplankton response. Ekologija 1, 56–65.
Paerl, H. W. (2009). Controlling eutrophication along the freshwater-marine continuum: dual nutrient (N and P) reductions are essential. Estuaries Coast. 32, 593–601. doi: 10.1007/s12237-009-9158-8
Parkos, J. J. III, Santucci, V. J. Jr., and Wahl, D. H. (2003). Effects of adult common carp (Cyprinus carpio) on multiple trophic levels in shallow mesocosms. Can. J. Fish. Aquat. Sci. 60, 182–192. doi: 10.1139/f03-011
Persson, A., and Svensson, J. M. (2006). Effects of benthivorous fish on biogeochemical processes in lake sediments. Freshw. Biol. 51, 1298–1309. doi: 10.1111/j.1365-2427.2006.01569.x
Petkuviene, J., Žilius, M., Lubiene, I., Ruginis, T., Giordani, G., Razinkovas-Baziukas, A., et al. (2016). Phosphorus cycling in a freshwater estuary impacted by cyanobacterial blooms. Estuaries Coast. 39, 1386–1402. doi: 10.1007/s12237-016-0078-0
Phan-Van, M., Rousseau, D., and De Pauw, N. (2008). Effects of fish bioturbation on the vertical distribution of water temperature and dissolved oxygen in a fish culture-integrated waste stabilization pond system in Vietnam. Aquaculture 281, 28–33. doi: 10.1016/j.aquaculture.2008.04.033
Pilkaitytė, R., and Razinkovas, A. (2006). Factors controlling phytoplankton blooms in a temperate estuary: nutrient limitation and physical forcing. Hydrobiologia 555, 41–48. doi: 10.1007/s10750-005-1104-6
Pilkaitytė, R., and Razinkovas, A. (2007). Seasonal changes in phytoplankton composition and nutrient limitation in a shallow Baltic lagoon. Boreal Environ. Res. 12, 551–559. doi: 10.1007/s11274-015-1903-5
Post, D. M., Taylor, J. P., Kitchell, J. F., Olson, M. H., Schindler, D. E., and Herwig, B. R. (1998). The role of migratory waterfowl as nutrient vectors in a managed wetland. Conserv. Biol. 12, 910–920. doi: 10.1046/j.1523-1739.1998.97112.x
Pustelnikovas, O. (1994). Transport and accumulation of sediment and contaminants in the Lagoon of Kuršiu marios (Lithuania) and Baltic Sea. Neth. J. Aquat. Ecol. 28, 405–411. doi: 10.1007/BF02334210
Putys,Ž., and Zarankaitė, J. (2010). Diet of the great cormorant (Phalacrocorax carbo sinensis) at the Juodkrantė colony, Lithuania. Acta Zool. Litu. 20, 179–189. doi: 10.2478/v10043-010-0031-6
Rabalais, N. N., Turner, R. E., and Wiseman, W. J. (2002). Gulf of Mexico hypoxia, AKA “The dead zone”. Annu. Rev. Ecol. Syst. 33, 235–263. doi: 10.1146/annurev.ecolsys.33.010802.150513
Rashleigh, B., Razinkovas, A., and Pilkaitytė, R. (2012). Ecosystem services assessment of the Nemunas River delta. Transl. Waters Bull. 5, 75–84. doi: 10.1285/i1825229Xv5n2p75
Razinkovas, A., Dailidienė, I., and Pilkaitytė, R. (2008). “Reduction of the land-based discharges to the Curonian Lagoon in a view of a climate change perspective,” in Sustainable Use and Development of Watersheds, NATO Science for Peace and Security Series (Series C: Environmental Security), eds Ý. E. Gönenç, A. Vadineanu, J. P. Wolflin, and R. C. Russo (Dordrecht: Springer), 403–413.
Razinkovas-Baziukas, A., Margoǹski, P., and Povilanskas, R. (2012). “Management challenges for the South Baltic transboundary transitional waters,” in Transboundary Management of Transitional Waters – Code of Conduct and Good Practice Examples, eds H. Nilsson, R. Povilanskas, and N. Stybel (Warnemünde: EUCC), 25–37.
Remeikaitė-Nikienė, N., Lujanienė, G., Malejevas, V., Barisevičiutė, R., Žilius, M., Garnaga-Budrė, G., et al. (2016). Distribution and sources of organic matter in sediments of the south-eastern Baltic Sea. J. Mar. Syst. 157, 75–81. doi: 10.1016/j.jmarsys.2015.12.011
Rodríguez-Pérez, H., Green, A. J., and Figuerola, J. (2007). Effects of Greater flamingo Phoenicopterus ruber on macrophytes, chironomids and turbidity in natural marshes in Doñana, SW Spain. Fundam. Appl. Limnol. 170, 167–175. doi: 10.1127/1863-9135/2007/0170-0167
Rönicke, H., Doerffer, R., Siewers, H., Büttner, O., Lindenschmidt, K. E., Herzsprung, P., et al. (2008). Phosphorus input by nordic geese to the eutrophic Lake Arendsee, Germany. Fundam. Appl. Limnol. 172, 111–119. doi: 10.1127/1863-9135/2008/0172-0111
Roozen, F. C., Lürling, M., Vlek, H., Van Der Pouw Kraan, E. A., Ibelings, B. W., and Scheffer, M. (2007). Resuspension of algal cells by benthivorous fish boosts phytoplankton biomass and alters community structure in shallow lakes. Freshw. Biol. 52, 977–987. doi: 10.1111/j.1365-2427.2007.01729.x
Ruginis, T., Bartoli, M., Petkuviene, J., Zilius, M., Lubiene, I., Laini, A., et al. (2014). Benthic respiration and stoichiometry of regenerated nutrients in lake sediments with Dreissena polymorpha. Aquat. Sci. 76, 405–417. doi: 10.1007/s00027-014-0343-x
Sánchez, M. I., Green, A. J., and Alejandre, R. (2006). Shorebird predation affects density, biomass, and size distribution of benthic chironomids in salt pans: an exclosure experiment. J. North Am. Benthol. Soc. 25, 9–18. doi: 10.1899/0887-3593 (2006)25[9:SPADBA]2.0.CO;2
Schindler, D. E., Carpenter, S. R., Cottingham, K. L., He, X., Hodgson, J. R., Kitchell, J. F., et al. (1996). “Food web structure and littoral zone coupling to pelagic trophic cascades,” in Food Webs: Integration of Pattern and Dynamics, eds G. A. Polis and K. O. Winemiller (Boston, MA: Springer), 96–105. doi: 10.1007/978-1-4615-7007-3_9
Schmidt-Ries, H. (1940). Untersuchung zur kenntnis des pelagials eines strand gewassers (Kurisches Haff). Z. Fischerei Deren Hilfswissenschaften B 37:330.
Semenova, A. S., and Aleksandrov, S. V. (2009). The zooplankton consumption of primary production and an assessment of the waterbody trophic state on the basis of its structural and functional characteristics. Inland Water Biol. 2, 57–63. doi: 10.1134/S1995082909040099
Shormann, D. E., and Cotner, J. B. (1997). The effects of benthivorous smallmouth buffalo (Ictiobus bubalus) on water quality and nutrient cycling in a shallow floodplain lake. Lake Reserv. Manage. 13, 270–278. doi: 10.1080/07438149709354318
Stanevičius, V., Švažas, S., Raudonikis, L., and Gražulevičius, G. (2009). Non-breeding concentrations of the Tufted Duck Aythya fuligula in Lithuania. Ekologija 55, 29–39. doi: 10.2478/v10055-009-0004-x
Stief, P. (2013). Stimulation of microbial nitrogen cycling in aquatic ecosystems by benthic macrofauna: mechanisms and environmental implications. Biogeosciences 10, 7829–7846. doi: 10.5194/bg-10-7829-2013
Šulčius, S., Pilkaitytė, R., Mazur-Marzec, H., Kasperovièienë, J., Ezhova, E., Błaszczyk, A., et al. (2015). Increased risk of exposure to microcystins in the scum of the filamentous cyanobacterium Aphanizomenon flos-aquae accumulated on the western shoreline of the Curonian Lagoon. Mar. Pollut. Bull. 99, 264–270. doi: 10.1016/j.marpolbul.2015.07.057
Švažas, S., Chukalova, N., Grishanov, G., Putys,Ž., Sruoga, A., Butkauskas, D., et al. (2011). The role of Great Cormorant (Phalacrocorax carbo sinensis) for fish stock and dispersal of helminthes parasites in the Curonian Lagoon area. Vet. Med. Zoot. 55, 79–85.
Tarvainen, M., Sarvala, J., and Helminen, H. (2002). The role of phosphorus release by roach [Rutilus rutilus (L.)] in the water quality changes of a biomanipulated lake. Freshw. Biol. 47, 2325–2336. doi: 10.1046/j.1365-2427.2002.00992.x
Tobiessen, P., and Wheat, E. (2000). Long and short term effects of waterfowl on Collins Lake, an urban lake in upstate New York. Lake Reserv. Manage. 16, 340–344. doi: 10.1080/07438140009354241
Trimonis, E., Gulbinskas, S., and Kuzavinis, M. (2003). The Curonian Lagoon bottom sediments in the Lithuanian water area. Baltica 16, 13–20.
Umgiesser, G., Zemlys, P., Erturk, A., Razinkova-Baziukas, A., Mezine, J., and Ferrarin, C. (2016). Seasonal renewal time variability in the Curonian Lagoon caused by atmospheric and hydrographical forcing. Ocean Sci. 12, 391–402. doi: 10.5194/os-12-391-2016
Vaičiutė, D., Bresciani, M., Bartoli, M., Giardino, C., and Bučas, M. (2015). Spatial and temporal distribution of coloured dissolved organic matter in a hypertrophic freshwater lagoon. J. Limnol. 74, 572–583. doi: 10.4081/jlimnol.2015.1176
Valiela, I., McClelland, J., Hauxwell, J., Behr, P. J., Hersh, D., and Foreman, K. (1997). Macroalgal blooms in shallow estuaries: controls and ecophysiological and ecosystem consequences. Limnol. Oceanogr. 42, 1105–1118. doi: 10.4319/lo.1997.42.5_part_2.1105
Vybernaite-Lubiene, I., Žilius, M., Giordani, G., Petkuviene, J., Vaičiutė, D., Bukaveckas, P. A., et al. (2017). Effect of algal blooms on retention of N, Si and P in Europe’s largest coastal lagoon. Estuar. Coast. Shelf Sci. 194, 217–228. doi: 10.1016/j.ecss.2017.06.020
Vybernaite-Lubiene, I., Zilius, M., Saltyte-Vaisiauske, L., and Bartoli, M. (2018). Recent Trends (2012-2016) of N, Si, and P export from the Nemunas River Watershed: loads, unbalanced stoichiometry, and threats for downstream aquatic ecosystems. Water 10:1178. doi: 10.3390/w10091178
Werner, S., Moertl, M., Bauer, H. G., and Rothhaupt, K. O. (2005). Strong impact of wintering waterbirds on zebra mussel (Dreissena polymorpha) populations at Lake Constance, Germany. Freshw. Biol. 50, 1412–1426. doi: 10.1111/j.1365-2427.2005.01411.x
Williams, A. E., Moss, B., and Eaton, J. (2002). Fish induced macrophyte loss in shallow lakes: top–down and bottom–up processes in mesocosm experiments. Freshw. Biol. 47, 2216–2232. doi: 10.1046/j.1365-2427.2002.00963.x
Ye, N., Zhang, X., Mao, Y., Liang, C., Xu, D., Zou, J., et al. (2011). ’Green tides’ are overwhelming the coastline of our blue planet: taking the world’s largest example. Ecol. Res. 26, 477–485. doi: 10.1007/s11284-011-0821-8
Yunev, O. A., Carstensen, J., Moncheva, S., Khaliulin, A., Ærtebjerg, G., and Nixon, S. (2007). Nutrient and phytoplankton trends on the western Black Sea shelf in response to cultural eutrophication and climate changes. Estuar. Coast. Shelf Sci. 74, 63–76. doi: 10.1016/j.ecss.2007.03.030
Zambrano, L., and Hinojosa, D. (1999). Direct and indirect effects of carp (Cyprinus carpio L.) on macrophyte and benthic communities in experimental shallow ponds in central Mexico. Hydrobiologia 408, 131–138. doi: 10.1023/A:1017085129620
Zettler, M. L., and Daunys, D. (2007). Long-term macrozoobenthos changes in a shallow boreal lagoon: comparison of a recent biodiversity inventory with historical data. Limnologica 37, 170–185. doi: 10.1016/j.limno.2006.12.004
Zhang, H., Culver, D. A., and Boegman, L. (2008). A two-dimensional ecological model of Lake Erie: application to estimate dreissenid impacts on large lake plankton populations. Ecol. Model. 214, 219–241. doi: 10.1016/j.ecolmodel.2008.02.005
Zilius, M., Bartoli, M., Bresciani, M., Kataržytė, M., Ruginis, T., Petkuvienė, J., et al. (2014). Feedback mechanisms between cyanobacterial blooms, transient hypoxia, and benthic phosphorus regeneration in shallow coastal environments. Estuar. Coast. 37, 680–694. doi: 10.1007/s12237-013-9717-x
Zilius, M., Bartoli, M., Daunys, D., Pilkaityte, R., and Razinkovas, A. (2012). Patterns of benthic oxygen uptake in a hypertrophic lagoon: spatial variability and controlling factors. Hydrobiologia 699, 85–98. doi: 10.1007/s10750-012-1155-4
Zilius, M., De Wit, R., and Bartoli, M. (2016). Response of sedimentary processes to cyanobacteria loading. J. Limnol. 75, 236–247. doi: 10.4081/jlimnol.2015.1296
Zilius, M., Giordani, G., Petkuviene, J., Lubiene, I., Ruginis, T., and Bartoli, M. (2015). Phosphorus mobility under short-term anoxic conditions in two shallow eutrophic coastal systems (Curonian and Sacca di Goro lagoons). Estuar. Coast. Shelf Sci. 164, 134–146. doi: 10.1016/j.ecss.2015.07.004
Zilius, M., Vybernaite-Lubiene, I., Vaiciute, D., Petkuviene, J., Zemlys, P., Liskow, I., et al. (2018). The influence of cyanobacteria blooms on the attenuation of nitrogen throughputs in a Baltic coastal lagoon. Biogeochemistry 141, 143–165. doi: 10.1007/s10533-018-0508-0
Keywords: Curonian Lagoon, nitrogen, phosphorus, silica, fluxes, stoichiometry, remote sensing, cyanobacteria
Citation: Bartoli M, Zilius M, Bresciani M, Vaiciute D, Vybernaite-Lubiene I, Petkuviene J, Giordani G, Daunys D, Ruginis T, Benelli S, Giardino C, Bukaveckas PA, Zemlys P, Griniene E, Gasiunaite ZR, Lesutiene J, Pilkaitytė R and Baziukas-Razinkovas A (2018) Drivers of Cyanobacterial Blooms in a Hypertrophic Lagoon. Front. Mar. Sci. 5:434. doi: 10.3389/fmars.2018.00434
Received: 13 May 2018; Accepted: 30 October 2018;
Published: 15 November 2018.
Edited by:
Riina Klais, University of Tartu, EstoniaReviewed by:
Sai Elangovan S, National Institute of Oceanography (CSIR), IndiaFranziska Wemheuer, University of New South Wales, Australia
Copyright © 2018 Bartoli, Zilius, Bresciani, Vaiciute, Vybernaite-Lubiene, Petkuviene, Giordani, Daunys, Ruginis, Benelli, Giardino, Bukaveckas, Zemlys, Griniene, Gasiunaite, Lesutiene, Pilkaitytė and Baziukas-Razinkovas. This is an open-access article distributed under the terms of the Creative Commons Attribution License (CC BY). The use, distribution or reproduction in other forums is permitted, provided the original author(s) and the copyright owner(s) are credited and that the original publication in this journal is cited, in accordance with accepted academic practice. No use, distribution or reproduction is permitted which does not comply with these terms.
*Correspondence: Marco Bartoli, bWFyY28uYmFydG9saUB1bmlwci5pdA==