- 1Aquaculture Graduate Program, Nilton Lins University, Manaus, Brazil
- 2Laboratory of Ecophysiology and Molecular Evolution, National Institute of Amazonian Research, Manaus, Brazil
Photoperiod is considered an important synchronizer of biological activities and endocrine pathways, including growth. As ectotherms, fish have many physiological functions controlled by the photoperiod. However, the combined effects of photoperiod and temperature should be clarified, particularly for tropical fish living near their upper thermal limit, as is the case of Amazonian fish. The central aim of this study was to evaluate the combined effect of photoperiod and temperature on growth and physiological aspects of tambaqui (Colossoma macropomum). Juveniles of tambaqui were distributed in 70 L tank, following a factorial design that included three photoperiods (light 0 h: darkness 24 h; light 12 h: darkness 12 h; and light 18 h: darkness 6 h) associated with three temperatures (26, 29, and 32°C). They were maintained under these conditions for 2 months. Fish reared in the dark at 29 and 32°C showed better performance compared to fish reared under extended light conditions at all temperatures. Among physiological responses, it has been observed that blood parameters tend to be disturbed with increasing temperature and that extended light conditions at low temperatures caused similar effects on the analyzed fish, suggesting blood hemoconcentration. Fish under extended light conditions showed an increase in glucose, cortisol, cholesterol, and total proteins, indicating additional physiological disturbances. In conclusion, our study shows that, unlike marine and temperate fish, C. macropomum, a fish species endemic to the Amazon, grows better in warmth dark, showing no significant physiological disturbances, similar to observations described for wild animals of this species. Our results support that changes in fish growth occur in response to environmental conditions. Therefore, fish species from freshwater, estuarine, and marine environments, must be specifically analyzed regarding the combination effects of photoperiod and temperature.
Introduction
Climate change affects fish growth and physiology (Cheung et al., 2013; Campos et al., 2017). In the coming years, warming of water bodies will force fish to migrate to areas previously unsuitable for their survival in response to declining food resources (Perry et al., 2005; Pörtner and Farrell, 2008). As a consequence, a decline in fish growth rates of 14–24% is expected, which will affect fisheries and the respective food industry due to changes in the dynamics of marine, estuarine, and freshwater ecosystems (Cheung et al., 2013).
For many decades, environmental manipulation has been used to improve growth of several species in aquaculture (Nerici et al., 2012; Imsland et al., 2013) and light has been shown to be an important synchronizer of biological fish activities, improving breeding conditions, and growth (Boeuf and Le Bail, 1999; Bromage et al., 2001). Photoperiod is an important zeitgeber for fish affecting feeding, behavior, and modulating the responses associated to the stress over seasons (Trippel and Neil, 2003; Imsland et al., 2006). Studies with salmonids and other temperate species have highlighted a positive relationship between prolonged or continuous light and growth, with stimulus of food intake, better feed conversion rate, and nutrient uptake (Taylor et al., 2006). In addition, many studies report an effect of light on the somatotrophic axis, regulating the production of growth hormone, which is responsible for the somatic development in vertebrates (Björnsson, 1997; Handeland et al., 2003; Taylor et al., 2005).
Seasonal changes in photoperiod and temperature are synchronized in natural environments. Fish activity are, in general, highly dependent on temperature (Elliott, 1982). The optimal temperature for growth in freshwater fish can be altered according to changes in the photoperiod (Coutant et al., 1984; Woiwode and Adelman, 1991). However, light also affects marine and temperate species, as showed by Døskeland et al. (2016) for the Atlantic salmon Salmo salar L. Some studies have revealed limiting effects of continuous light at higher temperatures for growth, whereas at lower temperatures these effects appear to increase fish development (Solbakken et al., 1994; Imsland et al., 1995, 2017; Lohne et al., 2012). These studies suggest an interactive effect of photoperiod and temperature on fish growth. Nonetheless, as far as we know, there are no evidences if the impact of the variations of these environmental factors can cause losses on the growth of cultivated animals in the Amazon, althought some studies have indicated effects of light or temperature (not in combination) on metabolic indexes (Biswas and Takii, 2016), and hematological parameters (Biswas et al., 2004; Bani et al., 2009) for others species.
Tambaqui, Colossoma macropomum, belongs to the Serrasalmidae family and is endemic to the Orinoco and Amazon rivers (Goulding and Carvalho, 1982; Mirande, 2010). This species is overexploited in nature, mainly in the Brazilian Amazon basin, and so, aquaculture currently supplies consumer markets in Brazil, Colombia, Peru, and Venezuela (Oliveira et al., 2012). The presence of tambaqui and its hybrids have been reported in several Latin American countries, in the United States of America, and in various countries in Asia, including China, Indonesia, Malaysia, Myanmar, Vietnam, Thailand, and Singapore, either introduced by accident or deliberately in fish farms (FAO, 2017, 2018; Nico and Neilson, 2018).
Studies involving manipulation of environmental factors and their effects on growth are scarce for Amazonian fish. For tambaqui, Oliveira and Val (2017) found a decrease in the feed efficiency of the animals submitted to the drastic scenario A2 (increase of 4.5°C and 850+ ppm of CO2 in relation to the current levels) as predicted for the year 2100 by the IPCC (2007), suggesting increases in the cost of production due to the increase in food consumption and rearing time. Thus, in the coming decades, the great challenge will be to promote the development of techniques that ensure optimal fish growth rates under global warming scenarios (Baudron et al., 2014; Liang et al., 2018).
Therefore, considering that fish may present different growth patterns in the environmental scenarios foreseen for the near future, this study was designed to analyze if the exposure to different combinations between the photoperiod and the temperature can affect the Amazonian tambaqui. We hypothesized that the effect of photoperiod on the growth and physiological aspects of tambaqui is temperature dependent and that environmental manipulation is effective in reducing rearing time, ensuring better growth rates.
Materials and Methods
Fish, Experimental Design and Facilities
All procedures and experimental manipulations used in this study were performed according to the Brazilian Guidelines for Animal Care and were approved by the Ethics Committee for the use of Animals of the Brazilian National Institute for Research of the Amazon (INPA), protocol number 026/2015. Juveniles of tambaqui were purchased from the Center for Technology, Training, and Production in Aquaculture (CTTPA, Manaus, Brazil) (1°55′07.7S/59°27′59.7W) and transported to the Laboratory of Ecophysiology and Molecular Evolution of INPA (LEEM/INPA). In the laboratory, the fish were kept in two 3000 L fiberglass tanks, supplied with flow thru water, under a 12:12 h light:dark (or L:D) photoperiod and temperature varying between 26 and 27°C for 2 weeks for acclimatization to local conditions. During this period, the fish were fed ad libitum with commercial feed of 2–4 mm (Nutripeixe Tr 36–36% protein, Purina Co., São Paulo, SP, Brazil), twice a day (at 9h and 16h) (Table 1). Feeding was suspended 48 h prior resettlement in the experimental tank.
After this period, juveniles weighing 16.22 ± 0.15 g and measuring 10 ± 0.04 cm (N = 270, mean ± standard deviation) were randomly distributed in 70 L tanks (80 × 50 × 40 cm, LxWxD) following a factorial scheme that included three photoperiods and three temperatures to evaluate the effects these variables on growth performance and physiological responses after 60 days. The photoperiods were: dark (D, 24 h dark), regular photoperiod of the Amazon (SNP, 12:12 L:D) and extended Amazonian photoperiod (PNP, 18:6 L:D). The temperatures tested were 26, 29, and 32°C. These temperatures were set in accordance with preferred range of tambaqui in the wild (Araujo-Lima and Goulding, 1998). Thus, nine experimental conditions were established: D-26, D-29, D-32, SNP-26, SNP-29, SNP-32, PNP-26, PNP-29, and PNP-32. Three replicates (n = 3) of each experimental condition were employed.
The tanks were kept in an isolated room and were arranged on benches and covered with black plastic covers to avoid the contact of fish with the external luminosity. All tanks were supplied with 2 L.min−1 of aerated well water from a head tank. A chemical filter was positioned between the head tank and the experimental tank to reduce ammonia levels in the water. Fluorescent lamps (36 watts, AT T10, 5,600 K, ARTEK® Co, São Paulo, Brazil) were placed 50 cm above the surface of the water and controlled by timers (6610, DNI®, Brazil) set to start daily at 6 a.m and shut off according to experimental photoperiods. For the dark treatment (D), the benches received the lamps, but these were off during the experimental period. Heaters (Aquarium, H-606, Hopar®) and coolers coupled to a thermostat (TIC-17RGT, Full Gauge, ± 0.01 accuracy) were placed in the tanks to ensure the maintenance of the experimental temperatures. The food remains were siphoned through a hole in the lid of the tanks opened only for this operation.
Experimental Procedure and Sample Collection
After being transferred to the experimental tanks, the fish remained for 1 week under the same acclimation conditions (12L:12D, 26°C, 5.3 ± 0.10 mg O2.L−1, pH 6.40 ± 0.20, 9 ± 2.40 μS.cm−1; 0.086 ± 0.01 mmol.L−1 Na+; 0.026 ± 0.01 mmol.L−1 K+). The initial health conditions of the animals were verified by sampling one fish per tank for hematological and biochemical analysis (Before the Start of the Experiment—BSE). After this period, temperatures were gradually changed at a rate of 1.0°C per day until reaching 29°C and 32°C, respectively. At the same time, the coolers coupled to the thermostats were activated to reduce the temperature to 26°C at the same rate. The temperature was stabilized within 3 days. Thus, on the fourth day (day 0 of the experiment), the exposure period to the experimental combinations, as indicated above, was started. During the experimental period, fish were fed a commercial feed of 2–4 mm (Nutripeixe Tr 36–36% protein, Purina Co, São Paulo, SP, Brazil), twice a day (9 a.m., 4 p.m., see Table 1). The unconsumed pellets were collected 30 min after feeding in each tank by means of siphoning. The traces were air dried and weighed to calculate feed intake (FI) and feed conversion ratio (FCR). The tank water was renewed daily at a rate of 20% to avoid increasing ammonia levels. The water quality was checked after the last feeding every day throughout the experimental period.
On days 15, 30, and 60, nine fish were collected, three animals per tank, which were anesthetized with buffered anesthetic (1 g.L−1 MS 222 + 2 g.L−1 NaHCO3, Sigma Aldrich®, USA), measured with the aid of an ichthyometer with 0.1 cm of precision and weighed using an analytical balance (Model Marte BL3200H, Minas Gerais, Brazil) with 0.1 g of precision for the analyzes of the growth indices. Immediately after growth measurements, blood samples (1.5 mL) were collected from caudal vein using heparinized syringes (lithium heparin, Sigma Aldrich®, USA) for the determination of hematological and biochemical parameters. After each sampling period, fish marked with fluorescent elastomer (Northwest Marine Technology®, Shaw Island, Washington, USA) on the pectoral fin were transferred to the experimental tank to maintain biomass density.
Growth Parameters
Growth analysis was performed following the parameters described below:
Weight gain (WG, g) = Final Weight (g)—Initial Weight (g)/number of fish per replicate
Specific growth rate (SGR, %) = 100x [ln final weight (g)—ln initial weight (g)]/(days of feeding period)
Feed intake (FI, g) = total feed consumed (g)/number of fish per replicate
Feed conversion ratio (FCR) = feed intake (g)/weight gain (g)
Condition factor (CF) = weight (g)/length (cm)2.84 (2.84 corresponds to the regression coefficient for the growth of tambaqui populations in the wild (Araujo-Lima and Goulding, 1998)
Protein efficiency ratio (PER, %) = [weight gain (g)/feed intake (g) x% protein ration]×100
Survival Rate (S,%) = 100x (final number of fish/initial number of fish)
Blood Analysis
After collection, the blood samples were stored in 2 ml Eppendorf bullets and kept on ice. Total blood aliquots were diluted in a solution of formaldehyde citrate (3.8 g Na3H5O7.2H2O, 2.0 mL 40% H2O formaldehyde and distilled water q.s.p 100 mL) for RBC counts (RBC) using a Neubauer chamber under 40 × magnification on a Motic Professional B5 optical microscope (Motic®, USA). Hematocrit was determined by the microhematocrit method described by Goldenfarb et al. (1971). The hemoglobin concentration was measured using the cyanometahemoglobin method described by van Kampen and Zijlstra (1961). The red cell index MCV (mean corpuscular volume), MCH (mean corpuscular hemoglobin) and MCHC (mean concentration of corpuscular hemoglobin) were calculated according to (Brow, 1976).
Plasma Metabolites
Plasma was obtained by centrifugation of whole blood in an Eppendorf centrifuge (model 5430R, Hamburg, Germany) at 1834 RCF for 7 min and stored at −80°C until analysis. Cortisol concentration was determined by the ELISA method using a commercial kit (Diagnostic Biochem Canada®, Canada) following the manufacturer's recommendations that were adapted for reading on a plate spectrophotometer (Spectra max Plus, model 384, Molecular Devices®, USA) at 450 nm. Cortisol concentrations are expressed as ng.mL−1.
The glucose concentration was quantified by the enzymatic colorimetric method using a commercial kit (In vitro®, Minas Gerais, Brazil), following manufacturer's instructions adapted to a plate spectrophotometer (Spectra max Plus, model 384, Molecular Devices®, USA) at 500 nm. This method measures the oxidation of glucose caused by the enzyme glucose oxidase. Glucose concentrations are expressed in mg.dL−1.
Cholesterol and triglyceride concentrations were also determined using a commercial kit (In vitro®, Minas Gerais, Brazil) following the manufacturer's instructions. Both protocols were adapted to a plate spectrophotometer (Spectra max Plus, model 384, Molecular Devices®;, USA) and determined at 500 nm. The concentrations of both metabolites are expressed in mg.dL−1.
Total protein levels were determined by the biuret method using a commercial kit (In vitro®, Minas Gerais, Brazil) following manufacturer's instructions adapted to a plate spectrophotometer (Spectra max Plus model, 384, Molecular Devices®, USA) at 550 nm. The concentrations of total proteins are expressed in g.dL−1.
Water Quality
Dissolved oxygen (mg.L−1), temperature (°C) and water pH were measured every day using YSI probes (YSI® 60 and 85 models, USA). Ammonia concentrations were determined according to the colorimetric method described by Verdouw et al. (1978) and are expressed in μmol.L−1.
Statistics
All data are presented as mean ± standard error of the mean (SEM; N = 9; n = 3). Prior to the analyzes, normality and homogeneity of the variances were verified using the Sigma Plot 11.0 package (Systat Software Inc., USA). Data transformation was applied when the assumptions for parametric analysis were violated. Two-way ANOVA was used to test the effects of time and experimental combinations (photoperiod and temperature), and the presence or absence of interaction between these two factors on growth parameters and physiological responses. To evaluate the water quality and differences between treatments in relation to initial experimental group (BSE) during the experiment, a one-way ANOVA was performed. The Tukey test was used to determine the significance of the differences among the means. Significant differences were accepted when p < 0.05.
Results
Water Quality
The water quality are presented in Table 2. The levels of ammonia, dissolved oxygen, and pH presented no significant differences over the experimental period, while the temperature varied as we expected, according to the values preset for this study (p < 0.05).
Growth
Tambaqui performance was influenced by combinations of photoperiod and temperature as shown in Figures 1, 2 and Table 3. In our study, the increase in temperature combined with photoperiod had a great effect on growth. Overall results show an increase for WG, SGR, FI, CF, PER, and lower FCR for fish reared under D-32 conditions in contrast to the lower performance observed for fish reared under SNP-26 and SNP-32 conditions. PNP-fish at all temperatures had a similar performance, although the final WG, SGR, FCR, CF, and PER were lower than the D-32 fish, whose performance was also higher when compared to other treatments (p < 0.05). The increase of the photoperiod at lower temperatures, as observed for the treatment with PNP-26, increases FI and SGR, but is not compensated with better FCR, CF, and PER compared to fish at the same temperature in shorter photoperiods (p < 0.05).
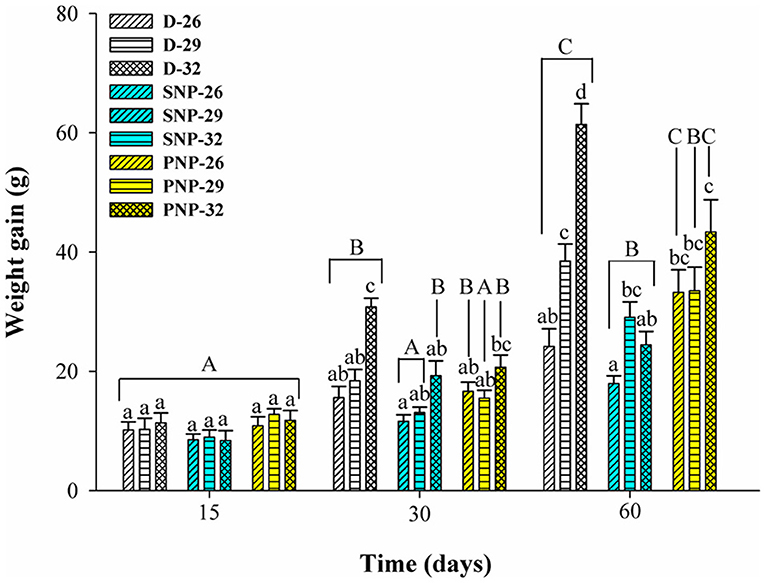
Figure 1. Weight gain (g) of juveniles of Colossoma macropomum under controlled conditions of photoperiod and temperature. Data are mean ± SEM (n = 3). The different capital letters indicate significant differences in the same treatments over the experimental period (p < 0.05), while the lower-case letters indicate significant differences in the different treatments in the same experimental period (p < 0.05).
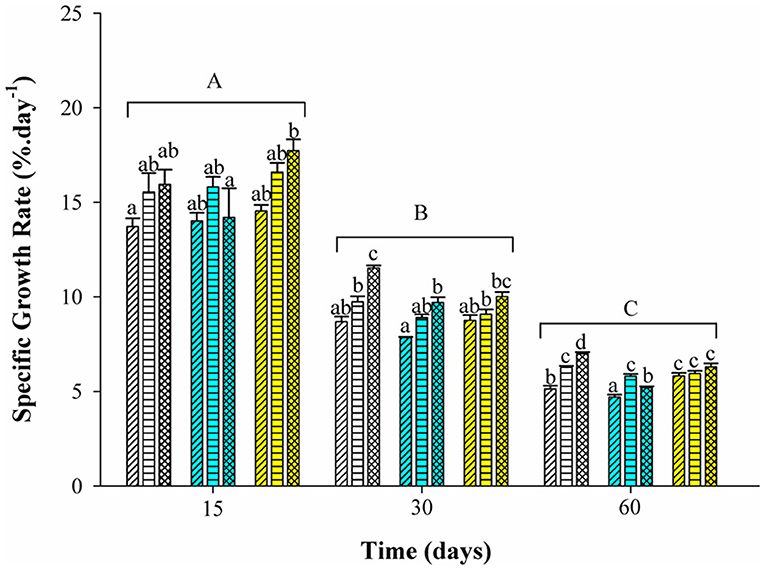
Figure 2. Specific growth rate (%.day−1) of juveniles of Colossoma macropomum under controlled conditions of photoperiod and temperature. Data are expressed as mean ± SEM (n = 3). Different lowercase letters indicate significant differences in the different treatments at the same experimental period (p < 0.05). The horizontal lines on the bars represent differences in experimental periods (p < 0.05).
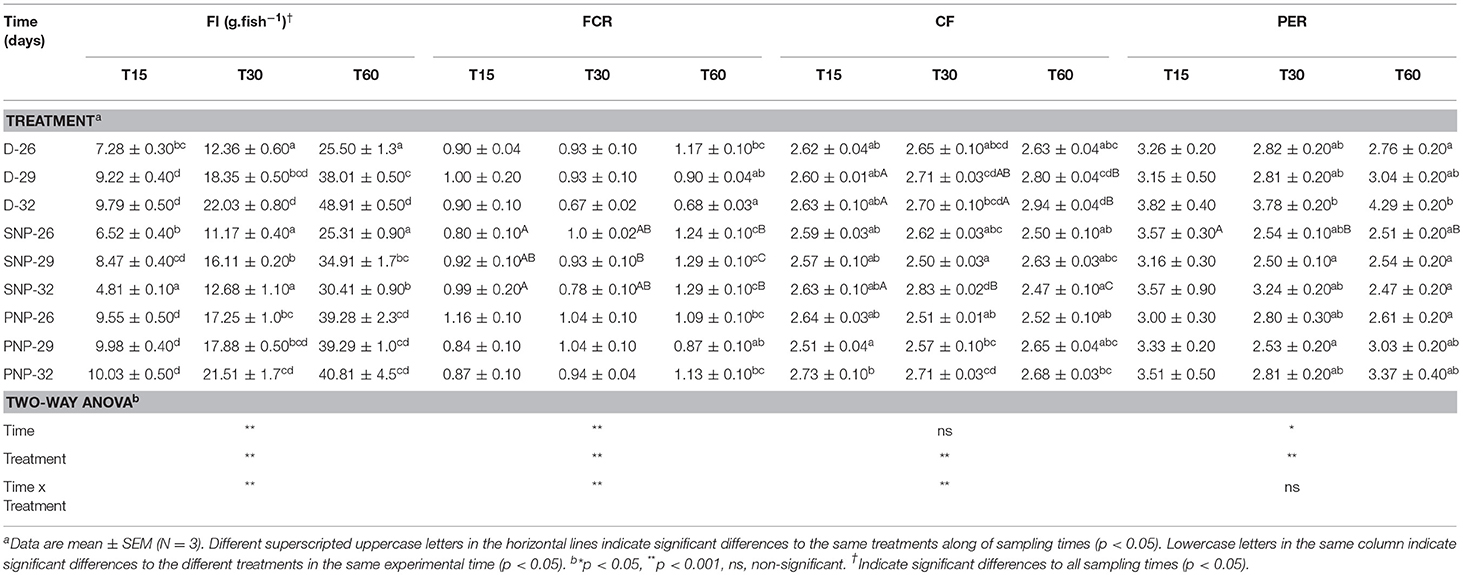
Table 3. Feed intake (FI), feed conversion ratio (FCR), condition factor (CF), and protein efficiency ratio (PER) of juveniles of Colossoma macropomum at different experimental trials (D-26, D-29, D-32, SNP-26, SNP-29, SNP-32, PNP-26, PNP-29, and PNP-32).
Blood Parameters
The blood parameters of tambaqui juveniles under different photoperiod and temperature conditions are shown in Table 4. It was observed an increase in Hb, Ht, and RBC in fish raised at 32°C in D, SNP, and PNP, compared with fish at 26°C (p < 0.05), except for the PNP-26 treatment, where these variables were higher (p > 0.05, Table 4). At the end of the experiment, fish under PNP-29 had Hb, Ht, and RBC similar to fish grown at 26°C, although they remained similar to fish in the same photoperiod, for Ht and RBC, and for all other photoperiods at 32°C (p > 0.05). MCV, MCH, and MCHC were similar among treatments at 29 and 32°C over the experimental period. However, high VCM was observed for SNP-26 and PNP-26 compared to fish groups exposed to 29 and 32°C at 15 days; at 30 days, these differences were also observed between these two groups (SNP-26 and PNP-26). Lower MCH and MCHC were observed for fish under PNP-26 when compared to the SNP-32 group (p < 0.05). In general, the main differences between treatments and the group before the start of the experiment (BSE) for the hematological variables were verified at the highest temperatures and the prolonged photoperiod (p < 0.05).
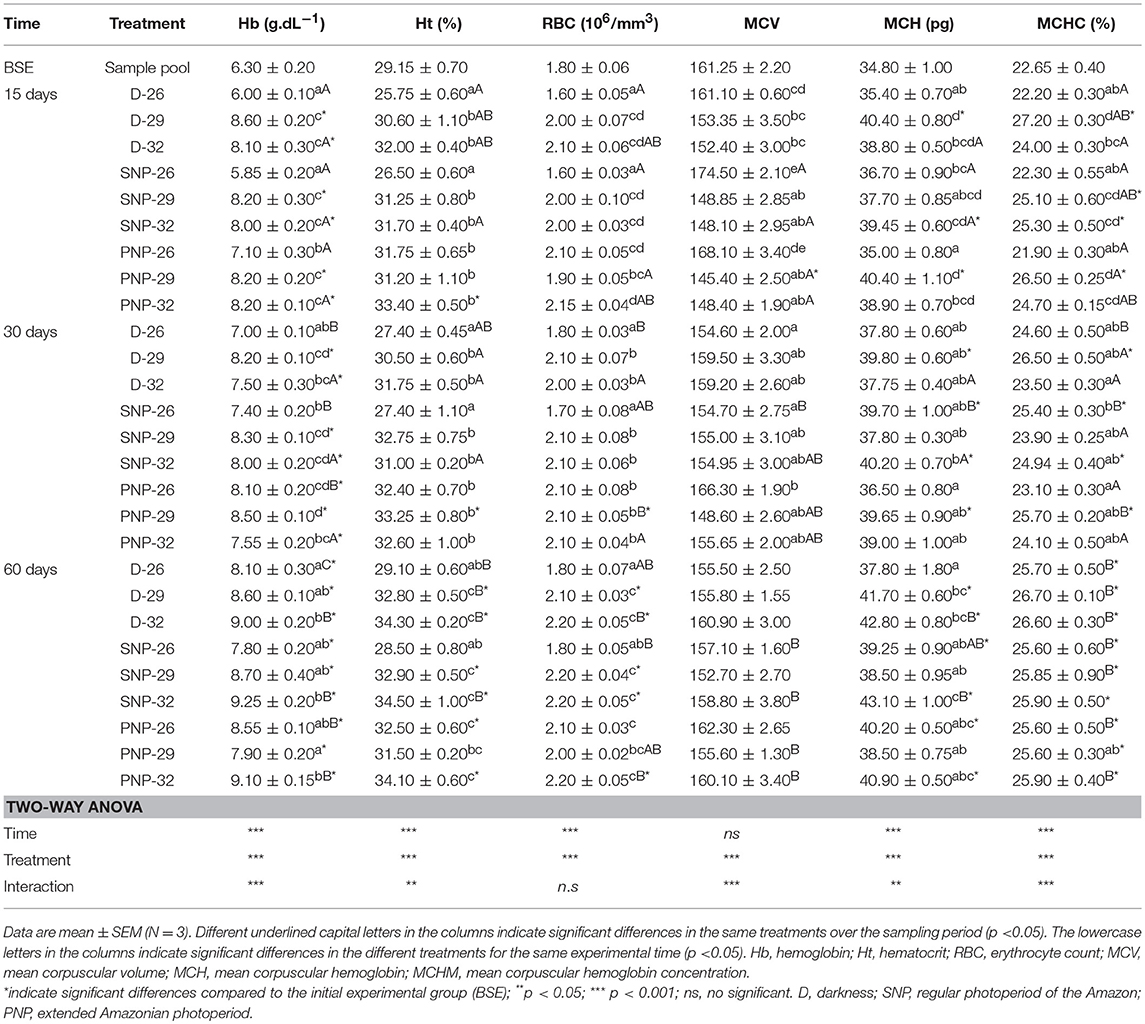
Table 4. Hematological parameters of juveniles of Colossoma macropomum at different experimental trials (D-26, D-29, D-32, SNP-26, SNP-29, SNP-32, PNP-26, PNP-29, and PNP-32).
Plasma Metabolites
Cortisol
An increase in cortisol levels was observed mainly at 30 days in all treatments (Figure 3). A similar pattern was observed among treatments in the same photoperiod, excluding the lowest level observed for the SNP-26 treatment when compared to the SNP-32 fish (p < 0.05). The increase in photoperiod and temperature were the main factors for the elevation of cortisol levels in tambaqui compared to BSE fish at the end of the experimental period (p < 0.05, see Figure 3).
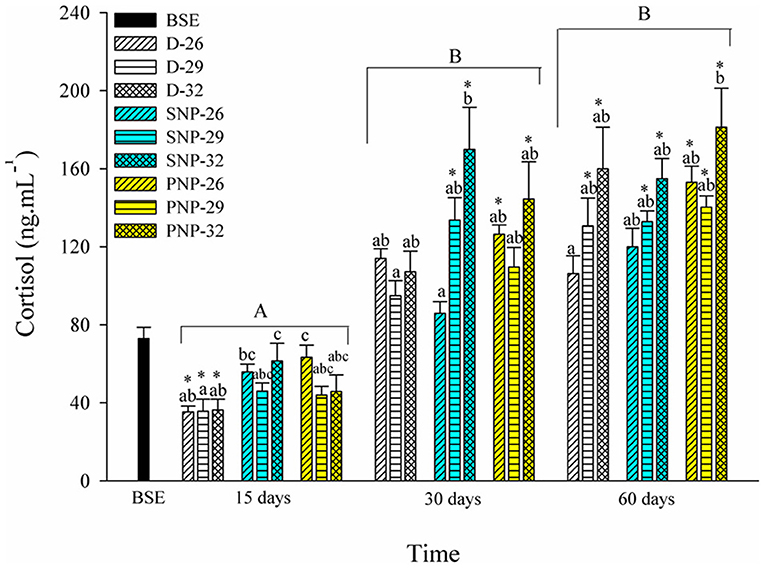
Figure 3. Plasma cortisol of juveniles of Colossoma macropomum under controlled conditions of photoperiod and temperature. Data are expressed as mean ± SEM (N = 3). Different lowercase letters indicate significant differences between treatments at the same experimental period (p < 0.05). The horizontal lines on the bars represent differences in experimental periods (p < 0.05). * Indicate differences of the treatments in relation to BSE group.
Glucose
PNP-26 and SNP-32 treatments showed the most significant effect on plasma glucose levels of tambaqui (Figure 4). Glucose levels were significantly higher at 32°C for all photoperiods (p < 0.05). Compared to BSE, an overall increase of plasma glucose levels were observed.
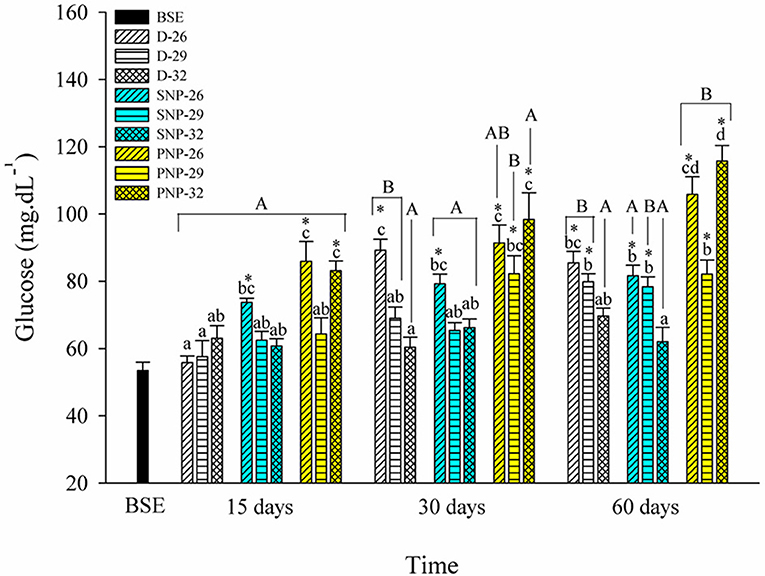
Figure 4. Plasma glucose of juveniles of Colossoma macropomum under controlled conditions of photoperiod and temperature. Data are expressed as mean ± SEM (N = 3). The different capital letters indicate significant differences in the same treatments over the experimental period (p < 0.05), while the lowercase letters indicate significant differences in the different treatments in the same experimental period (p < 0.05). * Indicate differences of the treatments in relation to BSE group.
Cholesterol and Triglycerides
Cholesterol and triglycerides varied between treatments (see Table 5). Elevated cholesterol levels were observed in PNP-32 fish compared to other treatments, except for 15 days for PNP-26 and for SNP-32 at 60 days (p < 0.05). In contrast, triglycerides were higher in the treatment of SNP-26 with remarkable differences in relation to fish of treatments D-32, SNP-32, and PNP-32 at 30 days (p < 0.05).
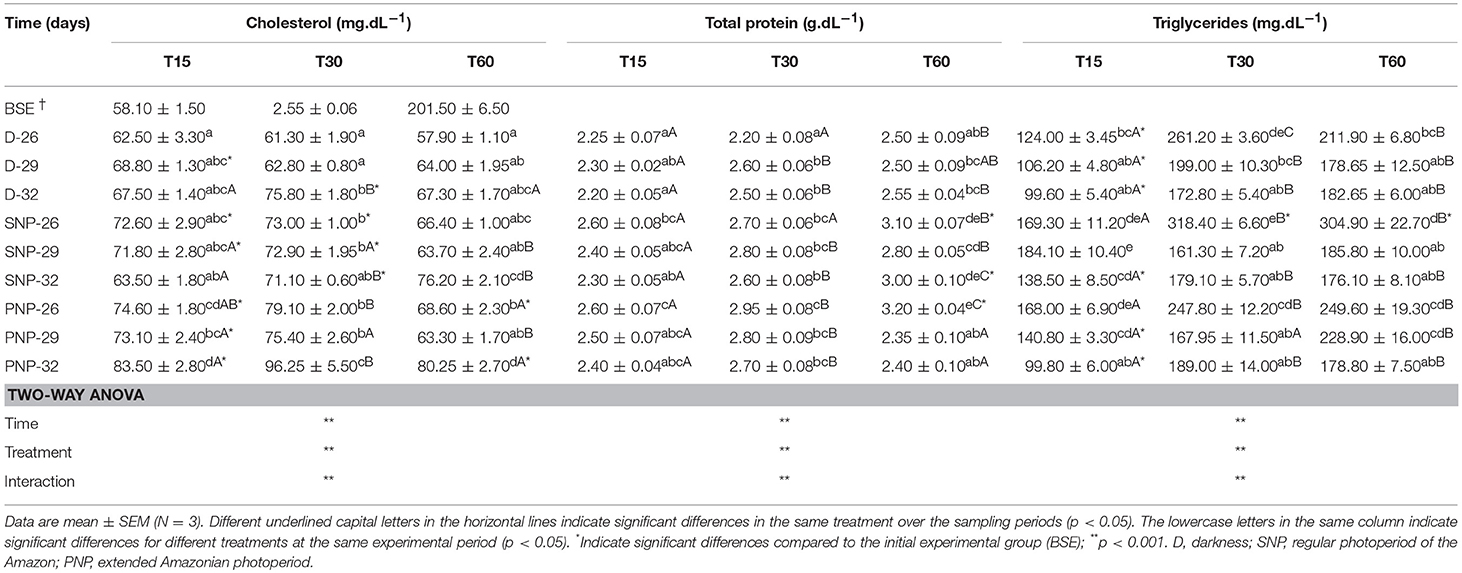
Table 5. Plasma cholesterol, total proteins, and triglycerides of juveniles of Colossoma macropomum at different experimental trials (D-26, D-29, D-32, SNP-26, SNP-29, SNP-32, PNP-26, PNP-29, and PNP-32).
Total Proteins
Total protein levels in tambaqui plasma were similar among treatments (p > 0.05), except for fish under PNP-26 which exhibited high concentrations over the experiment, when compared to fish under the same photoperiod at 60 days and fish under darkness at all temperatures (p < 0.05) (Table 5). On the other hand, high levels of total proteins were observed only for PNP-26 and SNP-32 at the end of the experiment, in contrast to fish of the BSE group (p < 0.05).
Discussion
Water Quality
The water quality showed no significant differences, except for the temperature that varied according to preset levels, as expected (Table 2). The observed variations of water quality remained within the range required for rearing tambaqui, as reviewed by several authors (Almeida-Val and Val, 1995; Araujo-Lima and Goulding, 1998; Souza-Bastos et al., 2017; Wood et al., 2017). For these authors, the rusticity of the species is based on its resistance to hypoxia, water acidity, and total ammonia, which preserves its growth performance in aquaculture.
Growth
Tambaqui growth varied according to the combination of photoperiod and temperature, as previously described in studies for temperate species such as Salmo salar L. (Knutsson and Grav, 1976), Perca flavescens and Stizostedion vitreum cf. Sander vitreus (Huh et al., 1976). These studies showed a positive relationship between the increase in daylight extension and the increase in temperature, and that photoperiod effects on growth were strongly modulated or dependent on the preferred environmental temperature of the species (Clarke et al., 1978; Imsland et al., 2007a). Photoperiod effects on tambaqui growth are modulated by temperature, as we hypothesized. However, different from other studies, darkness, when associated with higher temperatures (29 and 32°C for this study), is more efficient to promote better growth in tambaqui (Figures 1, 2 and Table 3). Thus, the best performance observed for the animals of the D-32 treatment, when compared to the other treatments, is explained by the natural life history of the studied species. In wild areas, tambaqui lives near or under aquatic vegetation and in forest-shaded water bodies where it finds shelter, food, and poor lighting in the muddy waters of Amazonian floodplains (Araujo-Lima and Goulding, 1998). Our data contrast with Mendonça et al. (2012) that recommended 15-h or more of light for a better growth performance of tambaqui. We believe that the darkness associated with temperatures above 30°C offer better conditions for better growth, since they mimic the light and temperature conditions found in nature and in fish farms, especially in the dry season, when Secchi disk reads are 20 cm (Sioli, 1984; Araujo-Lima and Goulding, 1998). Our study demonstrates that such effects appear to be persistent in laboratory and field conditions, as also observed for Gadus morhua (Atlantic cod) by Imsland et al. (2007b).
Similar growth rates were recorded in the same photoperiod of the SNP-26 animals and higher growth rates in relation to D-26 and SNP-26 (Figures 1, 2 and Table 3). A growth-stimulating effect has been reported in continuous light at low temperatures for species such as Hippoglossus hippoglossus L. and S. salar (Jonassen et al., 2000; Lohne et al., 2012; Handeland et al., 2013). According to the findings of Døskeland et al. (2016), the magnitude of these effects is inversely related to the increase in temperature indicating higher efficiency for animals raised at low temperatures when in continuous light, than at temperatures close to the optimum of the species. Even not testing a continuous light condition, the increase in the natural photoperiod found in nature by the species indicated the same effect in our study for tambaqui, although it was not accompanied by good indicators of food efficiency and body condition. Thus, our data contrast with the results of Imsland et al. (2007a) who reported lower growth rates for Gadus morhua raised in continuous light and low temperatures compared to those animals raised at 10 and 13°C in the same photoperiod, as the growth rates for tambaqui were similar over time (Figures 1, 2 and Table 3).
In the treatments where it was applied the natural photoperiod found by tambaqui in nature, the feed efficiency was compromised, as previously described by Aride et al. (2006). Thus, in our study, the best feed conversion for the D-32 group may be associated with the fact that the animal is prepared to process the food near or at night. We believe that exposure to darkness, associated with increased temperatures, promotes an optimization of digestive enzyme activities, and absorption of nutrients from the ingested food. Previous studies with tambaqui and pirapitinga Piaractus brachypomum support our hypothesis (Baras et al., 1996; Van der Meer et al., 1997). Thus, the increase in protein and condition factor efficiency was correlated with the increase in body mass as a consequence of better conversion of the food after 30 days (Table 3). The maintenance of high food intake in fish raised under the Amazon natural photoperiod, which presented a lower performance than the D-32 group, occurred due to the increase in the energetic costs due to the longer time for social contact, swimming, and competition for food. Thus, our data are in agreement with the observations of Adewolu et al. (2008) when they suggested a lower performance for the African catfish Clarias gariepinus subjected to natural photoperiod (12L: 12D) and continuous light. In addition, our personal observations suggest that these behaviors are more influenced by the temperature increase because the smaller fish take more time to obtain the food in relation to the larger ones, pointing out the existence of hierarchy of dominance associated with the size of the animals.
Physiological Indexes
Blood reflects the physiological state of animals in the face of environmental challenges (Zhou et al., 2009). The increase in water temperature tends to cause elevation of blood oxygen transport capacity suggesting a greater need for oxygen transfer. Under hypoxemic conditions or high demand for oxygen, splenic contractions followed by release of young erythrocytes improve oxygen transfer (Houston and Murad, 1995). However, the associated effects between photoperiod and temperature on these responses need to be further clarified.
Our study shows that the increase in temperature associated with all photoperiods caused an increase of RBC, Hb, Ht, and corpuscular constants (Table 4). These data are in agreement with those of Valenzuela et al. (2008) who observed an increase for RBC, Hb, and Ht of rainbow trout Oncorhynchus mykiss under 12L: 12D and under continuous light at warmer temperatures (18°C), suggesting that respiratory capacity is temperature dependent. Surprisingly, our study also showed that prolonged photoperiod at lower temperatures cause hemoconcentration. We believe that changes in blood properties occurred in response to increased respiratory demand due to additional costs with activities such as swimming, digestive needs, and social contact (Chudzik and Houston, 1983).
Fish under stressful conditions exhibit elevated levels of glucose and cortisol, which compromise vital activities (Barton and Iwama, 1991; Barton, 2002). Several studies reported acute stress conditions after handling, short exposure or even absence of photoperiod variation (Biswas et al., 2006, 2008; Bani et al., 2009; Veras et al., 2013). The increase in cortisol levels, for all treatments, is strongly influenced by the increase in temperature after 30 days (Figure 3) and suggests that exposure time is a stressful condition itself. It is noteworthy that in nature, fish can look for places where their vital activities are optimal. In this study, the impossibility of choosing environmental optima and the need to mobilize energy more quickly for the supply of metabolic needs under stressful conditions triggered such responses. These effects are more evident both when the photoperiod is increased as in the present study and when the temperature is increased as observed by Jaxion-Harm and Ladich (2014) for the common carp Cyprinus carpio.
The elevation of glucose levels for the PNP-26 and PNP-32 animals suggests increased energy mobilization (Figure 4). The slight increase for the SNP-26 fish, at 30 and 60 days, and the PNP-26 may be associated with the feeding regime (see Table 1). In addition, fish reared at 29 and 32°C showed lower levels of glucose (Figure 4). The data of Biswas et al. (2004) and Biswas and Takii (2016) did not show differences in glucose levels for Oreochromis niloticus and Oplegnathus fasciatus submitted to intermittent photoperiods for 3 months and also different luminosity. Our study, however, shows that higher temperatures combined with shorter photoperiods promote a better use of glucose and that longer photoperiods increase the energy cost for tambaqui when associated with different temperature levels.
We observed that fish at lower temperatures tended to maintain high triglyceride levels compared to the other treatments, regardless the photoperiod interval (Table 5). So, this elevation may have ensured greater thermal stability and protection to the animals in these temperatures due to the time of exposition and impossibility of the animals to move to confortable temperatures under the experimental conditions, as they would do in nature (see Figure 4). However, our data suggest that the elevation of cholesterol levels in the fish reared under PNP-32 is a result of the impact caused by the combination of the higher temperature and photoperiod. For tambaqui, in contrast to what has been suggested for marine and temperate species, the simultaneous elevation of photoperiod and temperature causes physiological impacts that do not ensure good conditions for fish growth, explaining the lower growth of animals under these conditions compared to the animals in the dark at 32°C.
Plasma total protein levels in this study were relatively stable (Table 5). Fish under PNP-26 maintained the highest levels of plasma protein in contrast to other treatments (Table 5). Contrary to what we have observed, Sala-Rabanal et al. (2003) found lower protein levels for Sparus aurata maintained at 8°C for 20 days. Tambaqui under the present experimental protocol may have increased plasma protein levels due to the reduced use of available proteins in the diet, which did not result in different growth rates as observed for SNP-32 and SNP-26 fish (Table 5).
Seasonal and estuarine species have their growth rates controlled by seasonal changes resulting from increased photoperiod and environmental temperatures in the summer and spring. However, this pattern is not similar among species, since the potentiating effect of growth promoted by periods of prolonged or continuous light may become limited at elevated temperatures (Lohne et al., 2012; Døskeland et al., 2016). In natural environments, the photoperiod affects fish development, in addition to plankton productivity and migration, an important food source, and oxygen producer in these ecosystems. Nowadays, anthropogenic activities, pollution levels and global warming potentiate the harmful effects of temperature on aquatic communities (Harley et al., 2006). The decline in productivity in marine regions, changes in migratory patterns, animal metabolic stress, and increased mortality are expected effects in the coming years, resulting in direct impacts on fisheries and aquaculture industries (Brander, 2007). Our results, in this context, show that fish exposure to temperatures out of the ranges occurring in their pristine habitats, even for a short period of time, reduce growth. From a climate change perspective, protocols to improve performance of captive animals under controlled conditions are needed to ensure the supply of protein in the near future, contributing to food security.
Conclusions
The general data of this work showed that the growth and the physiological responses of the tambaqui are adjusted according to photoperiod and the temperature. For this species the simultaneous increase of photoperiod and temperature causes hemoconcentration, higher levels of stress, and loss of food efficiency in contrast to marine, and estuarine species. Due the great metabolic costs in these conditions growth performance is not compensated for, and is not recommended as an adequate condition for rearing the species. Considering that tambaqui lives near its thermal limits, an association of increased photoperiod with low or high temperatures has a significant impact on the development of this species that grows better in the dark warmth (between 29 and 32°C).
Author Contributions
RA designed the work, performed the experiment, the data collection, analysis, and wrote the manuscript, JS helped to perform the experiment, the collection of samples, and discussed the data, and AV designed and supervised this work, obtained the financial support, discussed the data, and revised the manuscript.
Conflict of Interest Statement
The authors declare that the research was conducted in the absence of any commercial or financial relationships that could be construed as a potential conflict of interest.
Acknowledgments
This work is part of RA Master's thesis and was supported by the National Research Council of Brazil (CNPq)/Amazonas State Foundation Research (FAPEAM) INCT/ADAPTA grant to AV (Process number 573976/2008-2). RA was the recipient of a MSc. fellowship from CAPES (Brazil). AV is a recipient of a research fellowship from CNPq. We would like to thank MSc. Maria de Nazaré Paula Silva, Victor Lira, Dr. Alzira Oliveira, MSc. Rúbia Brandão, Dr. Luciana Souza-Bastos, and MSc. Arlan Paz for all their help and support.
Abbreviations
ADAPTA, Adaptations of Aquatic Biota of the Amazon; AOAC, Association of official analytical chemists; BSE, Before the Start of the Experiment; CAPES, Brazilian Coordination of Improvement of Personnel at Superior Level; CF, Condition Factor; cf., Check out; CNPq, National Research Council of Brazil; CTTPA, Center for Technology, Training and Production in Aquaculture;D,Dark; ELISA, Enzyme Linked ImmunonoSorbent Assay; FAPEAM, Amazonas State Foundation Research; FCR, Feed conversion ratio; FI, Feed intake; INPA, Brazilian National Institute for Research of the Amazon; IPCC, Intergovernmental Panel on Climate Change; L, Light; LEEM, Laboratory of Ecophysiology and Molecular Evolution; MCH, Mean corpuscular hemoglobin; MCHC, Mean concentration of corpuscular hemoglobin; MCV, Mean corpuscular volume; nm, Nanometer; PER, Protein efficiency ratio; PNP, Extended Amazonian photoperiod; RBC, Red Blood Cells; S, Survival Rate; SEM, Standard error of mean; SGR, Specific Growth Rate; SNP, Regular photoperiod of the Amazon;WG,Weight gain.
References
Adewolu, M. A., Adeniji, C. A., and Adejobi, A. B. (2008). Feed utilization, growth and survival of Clarias gariepinus (Burchell 1822) fingerlings cultured under different photoperiods. Aquaculture 283, 64–67. doi: 10.1016/j.aquaculture.2008.07.020
Almeida-Val, V. M. F., and Val, A. L. (1995). “A adaptação de peixes aos ambientes de criação,” in Criando peixes na Amazônia, eds A. L. Val and A. Honczaryk (Manaus: INPA), 45–60.
Araujo-Lima, C. A. R. M., and Goulding, M. (1998). Os Frutos do Tambaqui: Ecologia, Conservação e Cultivo na Amazônia. Brasília: Sociedade Civil Mamirauá, CNPq.
Aride, P. H. R., Roubach, R., Nozawa, S. R., and Val, A. L. (2006). Tambaqui growth and survival when exposed to different photoperiods. Acta Amaz. 36, 381–384. doi: 10.1590/S0044-59672006000300015
Association of official analytical chemists (AOAC) (1995). Official Methods of Analysis of AOAC International, 16th Edn., ed P. Cunniff. Virginia, NV.
Bani, A., Tabarsa, M., Falahatkar, B., and Banan, A. (2009). Effects of different photoperiods on growth, stress and haematological parameters in juvenile great sturgeon Huso huso. Aquac. Res. 40, 1899–1907. doi: 10.1111/j.1365-2109.2009.02321.x
Baras, E., Mélard, C., Grignard, J. C., and Thoreau, X. (1996). Comparison of food conversion by pirapatinga Piaractus brachypomus under different feeding times. Prog. Fish Cult. 58, 59–61. doi: 10.1577/1548-8640(1996)058<0059:COFCBP>2.3.CO;2
Barton, B. A. (2002). Stress in fishes: a diversity of responses with particular reference to changes in circulating corticosteroids. Integr. Comp. Biol. 42, 517–525. doi: 10.1093/icb/42.3.517
Barton, B. A., and Iwama, G. K. (1991). Physiological changes in fish from stress in aquaculture with emphasis on the response and effects of corticosteroids. Annu. Rev. Fish Dis. 1, 3–26. doi: 10.1016/0959-8030(91)90019-G
Baudron, A. R., Needle, C. L., Rijnsdorp, A. D., and Marshall, C. T. (2014). Warming temperatures and smaller body sizes: synchronous changes in growth of North Sea fishes. Glob. Change Biol. 20, 1023–1031. doi: 10.1111/gcb.12514
Biswas, A., and Takii, K. (2016). Effects of light intensity on the growth performance and stress response in striped knifejaw, (Oplegnathus fasciatus). J. World 47, 806–811. doi: 10.1111/jwas.12313
Biswas, A. K., Maita, M., Yoshizaki, G., and Takeuchi, T. (2004). Physiological responses in Nile tilapia exposed to different photoperiod regimes. J. Fish Biol. 65, 811–821. doi: 10.1111/j.1095-8649.2004.00487.x
Biswas, A. K., Seoka, M., Takii, K., Maita, M., and Kumai, H. (2006). Stress response of red sea bream Pagrus major to acute handling and chronic photoperiod manipulation. Aquaculture 252, 566–572. doi: 10.1016/j.aquaculture.2005.06.043
Biswas, A. K., Seoka, M., Ueno, K., Takii, K., and Kumai, H. (2008). Stimulation of growth performance without causing stress response in young red sea bream, Pagrus major (Temminck and Schlegel), by photoperiod manipulation. Aquac. Res. 39, 457–463. doi: 10.1111/j.13652109.2008.01897.x
Björnsson, B. T. (1997). The biology of salmon growth hormone : from daylight to dominance. Fish Physiol. Biochem. 17, 9–24.
Boeuf, G., and Le Bail, P. Y. (1999). Does light have an influence on fish growth? Aquaculture 177, 129–152. doi: 10.1016/S0044-8486(99)00074-5
Brander, K. M. (2007). Global fish production and climate change. Proc. Natl. Acad. Sci. U.S.A. 104, 19709–19714. doi: 10.1073/pnas.0702059104
Bromage, N., Porter, M., and Randall, C. (2001). The environmental regulation of maturation in farmed finfish with special reference to the role of photoperiod and melatonin. Aquaculture 197, 63–98. doi: 10.1016/S0044-8486(01)00583-X
Brow, B. A. (1976). Hematology: Principles and Procedures, 2nd Edn. Philadelphia, PA: Lea and Febiger.
Campos, D. F., Jesus, T. F., Kochhann, D., Heinrichs-Caldas, W., Coelho, M. M., and Almeida-Val, V. M. F. (2017). Metabolic rate and thermal tolerance in two congeneric Amazon fishes: Paracheirodon axelrodi Schultz, 1956 and Paracheirodon simulans Géry, 1963 (Characidae). Hydrobiologia 789, 133–142. doi: 10.1007/s10750-016-2649-2
Cheung, W. W. L., Sarmiento, J. L., Dunne, J., Frölicher, T. L., Lam, V. W. Y., Palomares, M. L. D., et al. (2013). Shrinking of fishes exacerbates impacts of global ocean changes on marine ecosystems. Nat. Clim. Change 3, 254–258. doi: 10.1038/nclimate1691
Chudzik, J., and Houston, A. H. (1983). Temperature and erythropoiesis in goldfish. Can. J. Zool. 61, 1322–1325.
Clarke, W. C., Shelbourn, J. E., and Brett, J. R. (1978). Growth and adaptation to sea water in “underyearling” sockeye (Oncorhynchus nerka) and coho (O. kisutch) salmon subjected to regimes of constant or changing temperature and day length. Can. J. Zoo. 56, 1421–2413.
Coutant, C. C., Zachman, K. L., Cox, D. K., and Pearman, B. L. (1984). Temperature selection by juvenile striped bass in laboratory and field. Trans. Am. Fish. Soc. 113, 666–671.
Døskeland, I., Imsland, A. K. D., Fjelldal, P. G., Stefansson, S. O., Roth, B., Eriksen, K. E., et al. (2016). The effect of low temperatures and photoperiods on growth and vertebra morphometry in Atlantic salmon. Aquac. Int. 24, 1421–1434. doi: 10.1007/s10499-016-9999-7
Elliott, J. M. (1982). The effects of temperature and ration size on the growth and energetics of salmonids in captivity. Comp. Biochem. Physiol. Part B Biochem. 73, 81–91. doi: 10.1016/0305-0491(82)90202-4
FAO (2017). Colossoma macropomum Introduced to Thailand from Hong Kong, Singapore. Available online at: http://www.fao.org/fishery/introsp/3885/en
FAO (2018). FishStat 2018 – Global Aquaculture Production. Available online at: http://www.fao.org/fishery/statistics/ global-aquaculture-production/query/en
Goldenfarb, P. B., Bowyer, F. P., and Hall, E. (1971). Reproducibility in the hematology laboratory: the microhematocrit determination. Am. J. Clin. Pathol. 56, 35–39.
Goulding, M., and Carvalho, M. (1982). Life history and management of the tambaqui (Colossoma macropomum, Characidae): an important Amazonian food fish. Rev. Bras. Zool. 1, 107–133. doi: 10.1590/S0101-81751982000200001
Handeland, S. O., Imsland, A. K., Björnsson, B. T., and Stefansson, S. O. (2013). Long-term effects of photoperiod, temperature and their interaction on growth, gill Na+, K+-ATPase activity, seawater tolerance and plasma growth-hormone levels in Atlantic salmon Salmo salar. J. Fish Biol. 83, 1197–1209. doi: 10.1111/jfb.12215
Handeland, S. O., Porter, M., Björnsson, B. T., and Stefansson, S. O. (2003). Osmoregulation and growth in a wild and a selected strain of Atlantic salmon smolts on two photoperiod regimes. Aquaculture 222, 29–43. doi: 10.1016/S0044-8486(03)00100-5
Harley, C. D., Randall Hughes, A., Hultgren, K. M., Miner, B. G., Sorte, C. J., Thornber, C. S., et al. (2006). The impacts of climate change in coastal marine systems: climate change in coastal marine systems. Ecol. Lett. 9, 228–241. doi: 10.1111/j.1461-0248.2005.00871.x
Houston, A. H., and Murad, A. (1995). Erythrodynamics in fish : recovery of the goldfish Carassius auratus from acute anemia. Can. J. Zool. 73, 411–418.
Huh, H. T., Calbert, H. E., and Stuiber, D. A. (1976). Effects of temperature and light on growth of yellow perch and walleye using formulated feed. Trans. Am. Fish. Soc. 105, 254–258. doi: 10.1577/1548-8659(1976)105<254
Imsland, A. K., Folkvord, A., and Stefansson, S. O. (1995). Growth, oxygen consumption and activity of juvenile turbot (Scophthalmus maximus L.) reared under different temperatures and photoperiods. Netherlands J. Sea Res. 34, 149–159. doi: 10.1016/0077-7579(95)90023-3
Imsland, A. K., Foss, A., Alvseike, T., Folkvord, A., Stefansson, O., and Jonassen, T. M. (2007a). Interaction between temperature and photoperiod on growth and feeding of Atlantic cod (Gadus morhua): possible secondary effects. Can. J. Fish. Aquat. Sci. 64, 239–248. doi: 10.1139/f07-006
Imsland, A. K., Foss, A., Koedijk, R., Folkvord, A., Stefansson, S. O., and Jonassen, T. M. (2007b). Persistent growth effects of temperature and photoperiod in Atlantic cod Gadus morhua. J. Fish Biol. 71, 1371–1382. doi: 10.1111/j.1095-8649.2007.01600.x
Imsland, A. K., Foss, A., Stefansson, S. O., Mayer, I., Norberg, B., Roth, B., et al. (2006). Growth, feed conversion efficiency and growth heterogeneity in Atlantic halibut (Hippoglossus hippoglossus) reared at three different photoperiods. Aquac. Res. 37, 1099–1106. doi: 10.1111/j.1365-2109.2006.01533.x
Imsland, A. K., Gunnarsson, S., Roth, B., Foss, A., Le Deuff, S., Norberg, B., et al. (2013). Long-term effect of photoperiod manipulation on growth, maturation and flesh quality in turbot. Aquaculture 416–417, 152–160. doi: 10.1016/j.aquaculture.2013.09.005
Imsland, A. K. D., Roth, B., Fjelldal, P. G., Stefansson, S. O., Handeland, S., and Mikalsen, B. (2017). The effect of continuous light at low temperatures on growth in Atlantic salmon reared in commercial size sea pens. Aquaculture 479, 645–651. doi: 10.1016/j.aquaculture.2017.07.014
IPCC (2007). “Summary for Policymakers,” in Climate Change 2007: The Physical Science Basis. Contribution of Working Group I to the Fourth Assessment Report of the Intergovernmental Panel on Climate Change, eds S. Solomon, D. Qin, M. Manning, Z. Chen, M. Marquis, K. B. Averyt, et al. (Cambridge; New York, NY: Cambridge University Press), 1–17.
Jaxion-Harm, J., and Ladich, F. (2014). Effects of temperature change on cortisol release by common carp Cyprinus carpio. J. Fish Biol. 84, 1221–1227. doi: 10.1111/jfb.12331
Jonassen, T. M., Imsland, A. T., Kadowaki, S., and Stefansson, S. O. (2000). Interaction of temperature and photoperiod on growth of Atlantic halibut Hippoglossus hippoglossus L. Aquac. Res. 31, 219–227. doi: 10.1046/j.1365-2109.2000.00447.x
Knutsson, S., and Grav, T. (1976). Seawater adaptation in Atlantic salmon (Salmo salar L.) at different experimental temperatures and photoperiods. Aquaculture 8, 169–187.
Liang, C., Xian, W., and Pauly, D. (2018). Impacts of ocean warming on China's fisheries catches: an application of “mean temperature of the catch” concept. Front. Mar. Sci. 5:26. doi: 10.3389/fmars.2018.00026
Lohne, P., Imsland, A. K., Larsen, S., Foss, A., and Pittman, K. (2012). Interactive effect of photoperiod and temperature on the growth rates, muscle growth and feed intake in juvenile Atlantic halibut. Aquac. Res. 43, 187–197. doi: 10.1111/j.1365-2109.2011.02815.x
Mendonça, P. P., Vazquez, M., Junior, V., Polese, M. F., Barbosa, V., Rezende, F. P., et al. (2012). Morphometrical development of tambaqui ( Colossoma macropomum, Cuvier, 1818) under different photoperiods. Rev. Bras. Zool. 41, 1337–1341. doi: 10.1590/S1516-35982012000600003
Mirande, J. M. (2010). Phylogeny of the family Characidae (Teleostei : Characiformes): from characters to taxonomy. Neotrop. Ichthyol. 8, 385–568. doi: 10.1590/S1679-62252010000300001
Nerici, C., Merino, G., and Silva, A. (2012). Effects of two temperatures on the oxygen consumption rates of Seriolella violacea (Palm fish) juveniles under rearing conditions. Aquac. Eng. 48, 40–46. doi: 10.1016/j.aquaeng.2011.12.011
Nico, L., and Neilson, M. (2018). Colossoma macropomum (Cuvier, 1816): U.S. Geological Survey, Nonindigenous Aquatic Species Database, Gainesville, FL. Available online at: https://nas.er.usgs.gov/queries/FactSheet.aspx?speciesID=418
Oliveira, A. M., Paula, M. N., Almeida-Val, V. M. F., and Val, A. L. (2012). Caracterização da atividade de piscicultura nas mesoregiões do estado do Amazonas, Amazônia Brasileira. Rev. Colomb. Ciência Anim. 4, 154–162.
Oliveira, A. M., and Val, A. L. (2017). Effects of climate scenarios on the growth and physiology of the Amazonian fish tambaqui (Colossoma macropomum) (Characiformes: Serrasalmidae). Hydrobiologia 789, 167–178. doi: 10.1007/s10750-016-2926-0
Perry, A. L., Low, P. J., Ellis, J. R., and Reynolds, J. D. (2005). Ecology: climate change and distribution shifts in marine fishes. Science 308, 1912–1915. doi: 10.1126/science.1111322
Pörtner, H. O., and Farrell, A. (2008). Physiology and climate change. Science 322, 690–692. doi: 10.1126/science.1163156
Sala-Rabanal, M., Sanchez, J., Ibarz, A., Fernandez-Borras, J., Blasco, J., and Gallardo, M. A. (2003). Effects of low temperatures and fasting on hematology and plasma composition of gilthead sea bream (Sparus aurata). Fish Physiol. Biochem. 29, 105–115. doi: 10.1023/B:FISH.0000035904.16686.b6
Sioli, H. (1984). “The Amazon and its main afluents: hidrography, morphology of the rivers courses, and river types,” in The Amazon. Limnology and Landscape Ecology of a Mighty Tropical River and its Basin, ed H. Sioli (Dordrecht: Junk), 127–166.
Solbakken, V. A., Hansenb, T., and Stefanssona, S. (1994). Effects of photoperiod and temperature on growth and Parr-smolt transformation in Atlantic salmon (Salmo salar L.) and subsequent performance in seawater. Aquaculture 121, 13–27.
Souza-Bastos, L. R., Val, A. L., and Wood, C. M. (2017). Are Amazonian fish more sensitive to ammonia? Toxicity of ammonia to eleven native species. Hydrobiologia 789, 143–155. doi: 10.1007/s10750-015-2623-4
Taylor, J. F., Migaud, H., Porter, M. J., and Bromage, N. R. (2005). Photoperiod influences growth rate and plasma insulin-like growth factor-I levels in juvenile rainbow trout, Oncorhynchus mykiss. Gen. Comp. Endocrinol. 142, 169–185. doi: 10.1016/j.ygcen.2005.02.006
Taylor, J. F., North, B. P., Porter, M. J. R., Bromage, N. R., and Migaud, H. (2006). Photoperiod can be used to enhance growth and improve feeding efficiency in farmed rainbow trout, Oncorhynchus mykiss. Aquaculture 256, 216–234. doi: 10.1016/j.aquaculture.2006.02.027
Trippel, E. A., and Neil, S. R. (2003). Effects of photoperiod and light intensity on growth and activity of juvenile haddock (Melanogrammus aeglefinus). Aquaculture 217, 633–645. doi: 10.1016/S0044-8486(02)00198-9
Valenzuela, A. E., Silva, V. M., and Klempau, A. E. (2008). Effects of different artificial photoperiods and temperatures on haematological parameters of rainbow trout (Oncorhynchus mykiss). Fish Physiol. Biochem. 34, 159–167. doi: 10.1007/s10695-007-9156-3
Van der Meer, M. B., van Herwaarden, H., and Verdegem, M. C. J. (1997). Effect of number of meals and frequency of feeding on voluntary feed intake of Colossoma macropomum (Cuvier). Aquac. Res. 28, 419–432. doi: 10.1046/j.1365-2109.1997.00874.x
van Kampen, E. J., and Zijlstra, W. G. (1961). Standardization of hemoglobinometry II. The hemiglobincyanide method. Clin. Chim. Acta 6, 538–544. doi: 10.1016/0009-8981(61)90145-0
Veras, G. C., Zangeronimo, M. G., Rosa, P. V., and Salaro, A. L. (2013). Fotoperíodo sobre parâmetrosparâmetros fisiológicos relacionados ao estresse em alevinos de tilápia-do-nilo. Arq. Bras. Med. Vet. e Zootec. 65, 1434–1440. doi: 10.1590/S0102-09352013000500023
Verdouw, H., Van Echteld, C. J. A., and Dekkers, E. M. J. (1978). Ammonia determination based on indophenol formation with sodium salicylate. Water Res. 12, 399–402. doi: 10.1016/0043-1354(78)90107-0
Woiwode, J. G., and Adelman, I. R. (1991). Effects of temperature, photoperiod, and ration size on growth of hybrid striped bass X white bass. Trans. Am. Fish. Soc. 120, 217–229. doi: 10.1577/1548-8659(1991)120
Wood, C. M., Gonzalez, R. J., Ferreira, M. S., Braz-Mota, S., and Val, A. L. (2017). The physiology of the Tambaqui (Colossoma macropomum) at pH 8.0. J. Comp. Physiol. B 188, 393–408. doi: 10.1007/s00360-017-1137-y
Keywords: amazon, aquaculture, climate change, environmental factors, metabolism, neotropical fish
Citation: Amanajás RD, Silva JM and Val AL (2018) Growing in the Dark Warmth: The Case of Amazonian Fish Colossoma macropomum. Front. Mar. Sci. 5:492. doi: 10.3389/fmars.2018.00492
Received: 13 September 2018; Accepted: 04 December 2018;
Published: 18 December 2018.
Edited by:
Mario Barletta, Universidade Federal de Pernambuco (UFPE), BrazilReviewed by:
Rodrigo Roubach, Food and Agriculture Organization delle Nazioni Unite, ItalyJack Falcon, Université Pierre et Marie Curie, France
Copyright © 2018 Amanajás, Silva and Val. This is an open-access article distributed under the terms of the Creative Commons Attribution License (CC BY). The use, distribution or reproduction in other forums is permitted, provided the original author(s) and the copyright owner(s) are credited and that the original publication in this journal is cited, in accordance with accepted academic practice. No use, distribution or reproduction is permitted which does not comply with these terms.
*Correspondence: Renan D. Amanajás, cmVuYW4uYW1hbmFqYXNAZ21haWwuY29t
†Present Address: Adalberto L. Val, Freshwater Biology and Inland Fisheries Graduate Program, Brazilian National Institute Research of the Amazon, Manaus, Brazil