- 1Consortium for Ocean Leadership, Washington, DC, United States
- 2Earth, Ocean, and Atmospheric Sciences, Oregon State University, Corvallis, OR, United States
- 3Applied Physics Laboratory, University of Washington, Seattle, WA, United States
- 4School of Oceanography, University of Washington, Seattle, WA, United States
- 5Woods Hole Oceanographic Institution, Woods Hole, MA, United States
The Ocean Observatories Initiative (OOI) is a United States National Science Foundation-funded major research facility that provides continuous observations of the ocean and seafloor from coastal and open ocean locations in the Atlantic and Pacific. Multiple cycles of OOI infrastructure deployment, recovery, and refurbishment have occurred since operations began in 2014. This heterogeneous ocean observing infrastructure with multidisciplinary sampling in important but challenging locations has provided new scientific and engineering insights into the operation of a sustained ocean observing system. This paper summarizes the challenges, successes, and failures experienced to date and shares recommendations on best practices that will be of benefit to the global ocean observing community.
Introduction
The Ocean Observatories Initiative (OOI)1 is a United States (US) National Science Foundation (NSF) major research facility and is the NSF’s contribution to the Global Ocean Observing System. It provides continuous observations spanning the seafloor, overlying ocean, and atmosphere of coastal and open ocean locations in the Atlantic and Pacific (Smith et al., 2018). Data collected, maintained, and disseminated by the OOI address significant scientific challenges such as coupling between the atmosphere and ocean (Chen et al., 2018; Ogle et al., 2018), coastal ocean dynamics (Zhang and Gawarkiewicz, 2015; Henderikx Freitas et al., 2018), climate and ecosystem health (Barth et al., 2018; Femke et al., 2018), the global carbon cycle (Palevsky and Nicholson, 2018), plate-scale seismicity (Nooner and Chadwick, 2016; Wilcock et al., 2016), and linkages between seafloor volcanism and life (Philip et al., 2016; Spietz et al., 2018). More information about the background and science objectives of the OOI can be found in Trowbridge et al. (this issue).
The OOI comprises a network of technologically advanced cabled and uncabled platforms that includes surface and subsurface moorings, moored profilers, seafloor nodes, and autonomous vehicles (Figure 1) with sensors that measure physical, chemical, geological, and biological properties at scales of centimeters to kilometers and microseconds to decades. The OOI was designed to evolve through incorporation of new technologies as well as novel research and educational proposals from the global community.
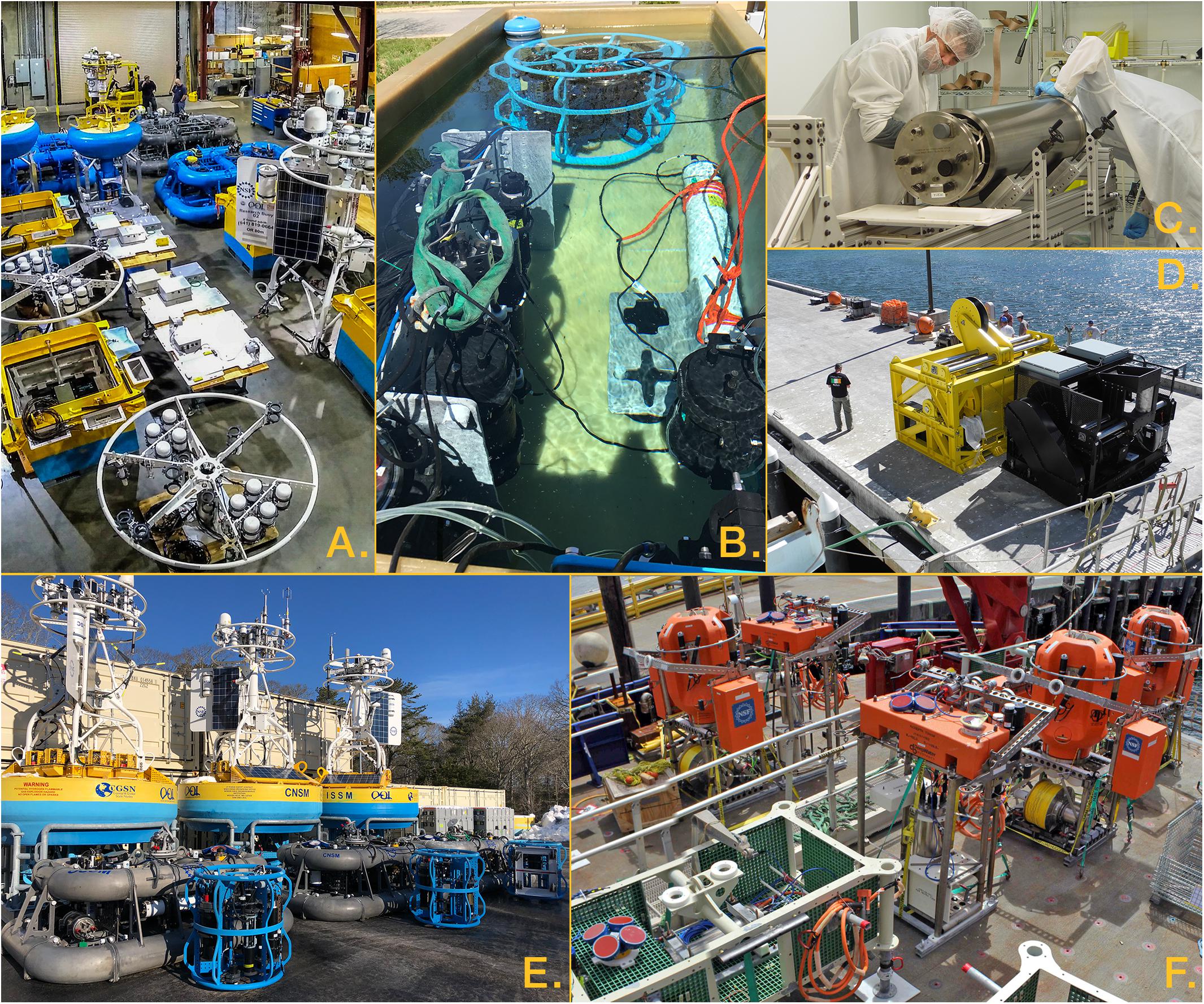
Figure 1. Ocean Observatories Initiative (OOI) Equipment and Testing centers. (A) OSU Ocean Observing Center high bay; (B) Coastal mooring instruments being tested for operation in saltwater at WHOI; (C) Cabled Array Platform Interface Controller being assembled in clean room; (D) OOI Medium and Heavy Lift Winches staged for installation on R/V Thomas G. Thompson; (E) Coastal Moorings await deployment; (F) Cabled Array Secondary Nodes, Shallow Profilers, and Platform Interface Assemblies mobilized on aft deck of R/V Sikuliaq. Credits: Craig Risien, OSU (A); Sheri White, WHOI (B); Eric McRae, UW (C); Geoffrey Cram, UW (D); Kristopher Newhall, WHOI (E); Deborah Kelley, UW (F).
The OOI Cyberinfrastructure makes these data freely available online to a global audience, providing as much data as possible in real- and near-real-time. Data are available in multiple forms, including raw data received from sensors prior to quality control through processed data products. During the third quarter of 2018, the OOI data portal2 received 6,100 visits from 56 countries with a total data download of 45.64 GB. The machine-to-machine interface and raw data archive were also heavily utilized and allow for the transfer of larger files, such as HD video files and hydrophone audio files. Over 31 million successful requests were made to the machine-to-machine interface totaling 573 GB of data transferred. The raw data archive received 5,000 visits from 29 countries for a total of 14.56 TB of data transferred.
Multiple cycles of OOI infrastructure deployment, recovery, and refurbishment have now occurred. The OOI facility, which includes over 800 instruments and almost 3,000 data streams, provides multidisciplinary sampling in important but challenging environments and can provide new scientific and engineering insights into the operation of sustained ocean observing systems. As noted in Lindstrom (2018), the OOI, through construction and transition to operations, has provided a pathway for the development of observatory infrastructure and testing of technology readiness that can be utilized by observatories across the globe.
This paper summarizes some key challenges, successes, and lessons learned to date by the OOI and shares recommendations on best practices and considerations that may benefit the global ocean observing community.
Lessons Learned Workshop
Representatives from organizations involved in OOI implementation, operation, and program management met 2–4 May 2018 to discuss important lessons learned since operations began in 2014. Topics discussed included field verification sampling and data quality control, platform communication and tracking, technology refresh priorities, cables and connectors, design issues and ease of use, profilers, and cruise operations. Lessons and details discussed during the workshop provide the basis for this paper focused on observing technology development, testing, and operation.
Pre-Deployment Testing
Prior to deployment, instruments and platforms undergo rigorous testing, separately and as integrated units, to ensure successful operation (Figure 1). Pre-deployment instrument testing occurs after manufacturer calibration and includes physical inspection, power-on test, operation and burn-in in saltwater, and integration with the platform for all OOI systems. Data transmissions and communications are tested during the burn-in phase. Cabled Array components are additionally subjected to in-house pressure testing, electrical characterization, electrical isolation testing, and corrosion mitigation. Disassembly after testing is minimized prior to deployment. Whenever possible, similar instrument models are tested together, and consistent testing procedures are conducted for the same instruments across program facilities.
Improvements have been made to enhance documentation and consistency in testing procedures and checklists. These documents are available through the OOI website3. Additionally, improvements such as testing instrumentation and electronics housings in saltwater tanks to find potential ground faults in sub-assemblies (Figure 1B) have enhanced testing capability and improved reliability.
The addition of non-core or Principal Investigator (PI)-based instruments – instruments that were not part of the OOI Final Network Design4 – brings new challenges. Researchers interested in adding instrumentation must work with OOI operators during the proposal process to conduct a technical feasibility assessment. This is necessary to ensure new platforms and instruments operate correctly when interfaced with OOI infrastructure and do not cause adverse effects on existing infrastructure. PI-supplied platforms and instruments must be delivered months prior to deployment to ensure sufficient time for integration and testing. Examples of PI-added instruments and platforms are in Section “Community Engagement.”
Deployments
Several lessons have been learned and improvements made in the deployment of specific infrastructure elements. Given the large size and quantity of equipment to be deployed on a given maintenance cruise (e.g., buoys, seafloor nodes, anchors, cables, profilers, gliders, instruments), deck space is a precious commodity; not all vessels can accommodate the OOI’s cruise requirements.
Though deck space is limited, commonly there are available berths on OOI cruises that provide opportunities for students and other researchers to participate and conduct ancillary scientific activities. A clear process and guidelines are being developed to maximize such opportunities with broad user community engagement5.
Improved coordination between the OOI, ship operators, and schedulers is needed to ensure that proper equipment can be installed if necessary to meet the operational requirements of the OOI. For example, the large moorings deployed on the Cabled Array required construction of two different, purpose-built, portable deck winches (8,000 and 25,000 lb full-drum pull) to safely handle the various mooring needs (Figure 1D). Ships must be able to accommodate the installation and operation of both winches for deployment and recovery of the Shallow Profiler Moorings.
Improving ease of use and transport of equipment is a priority and should be considered during technology refresh and refurbishment. Minor design improvements have been made to infrastructure to ensure safer deployment and recovery without altering the overall function. For example, the Coastal Profiler Mooring anchor design was modified to include a top plate with multiple bales for recovery by ROV and safer handling on deck (Figures 2C,D).
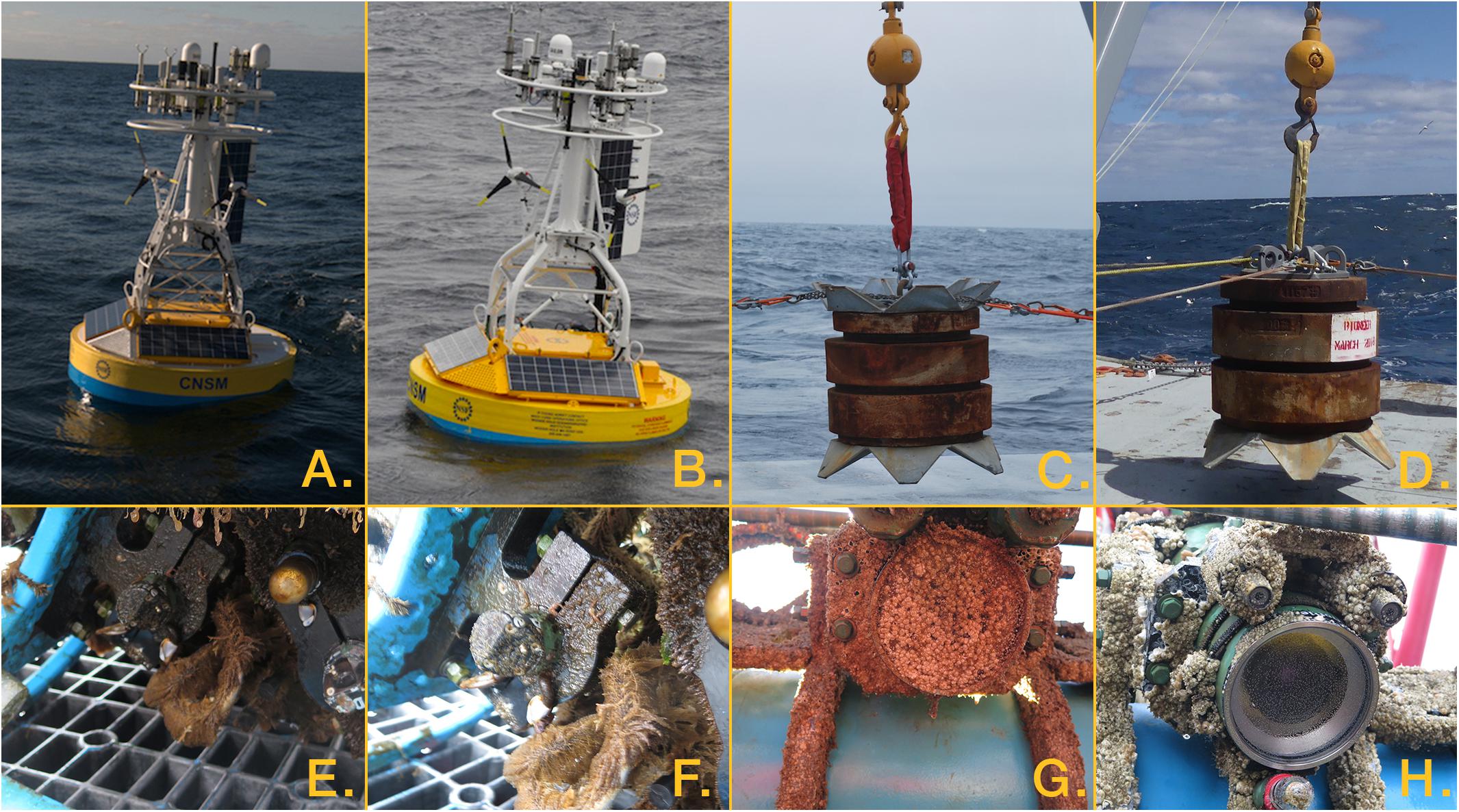
Figure 2. Examples of equipment modifications. Enhancements in Surface Buoy tower modifications from (A,B) include, rearranging halo components to move telemetry sensors down and wind sensors up; replacing flat tower legs with tubular legs to prevent torsion; widening of vane to improve ability of buoy to point toward the wind. Updates to the Coastal Profiler Mooring Anchor design (C,D) to include top plate with multiple bales for recovery by ROV and safer handling on deck. Pre- and Post-biofouling mitigation photos on oxygen optodes (E,F) and cameras (G,H) on the Endurance Array. Credits: (A–D) WHOI; (E,H) OSU.
Ocean Observatories Initiative Coastal Arrays (uncabled Endurance and Pioneer) are serviced biannually, while the Global Arrays (Irminger Sea, Station Papa and, previously, Southern Ocean and Argentine Basin) and the Cabled Array (including cabled Endurance Array) are serviced annually. In addition to the biannual maintenance schedule, some coastal assets require quarterly servicing. These include Coastal Surface Piercing Profilers (CSPP) due to battery limitations and Coastal Gliders. As these are both relatively small pieces of infrastructure, cruises are conducted via a small ship with an A-frame.
Cruises must be timed to optimal weather conditions and to ensure sufficient intervals between cruises for refurbishment and recalibration of equipment. For example, northern hemisphere Global cruises (Irminger Sea and Station Papa) must occur between June-August for optimal wind and wave conditions; southern hemisphere (Argentine Basin and Southern Ocean) conditions are best in December-February. Equipment must be shipped 2 months before cruises in the southern hemisphere to arrive in time. Coastal cruises must be spaced as close to 6 months apart as possible (Spring and Fall time periods) to allow sufficient time for refurbishment and recalibration of equipment before redeployment.
During cruises, flexibility is critical because of the weather-dependence of many activities. For example, each type of mooring has a certain maximum sea state it can be deployed or recovered in, such as 3-m significant wave height and 10 m/s winds from typical Global Class ships. Looking at four cruises to the Southern Ocean Array between February 2015–December 2017, even though these cruises were scheduled during the ideal climatological window, at least a third of the days during each cruise had weather sufficiently bad that moorings were not able to be deployed. During the 2017 cruise, two thirds of the days had sufficiently bad weather to prevent operations.
Optimal service frequencies of components based on historical experience with similar technologies are being reviewed and updated continually after each turn cruise. For uncabled moorings, the degradation of mooring components, biofouling of instruments, and depletion of batteries are the main drivers of the maintenance schedule. Annual inspections of deployed junction boxes on the Cabled Array confirmed they may only require refurbishment on a 5-year timeframe, assuming no refresh is needed to accommodate new PI-supplied instruments. In some cases, plans for repeated use of specific infrastructure components were found untenable based on inspection of recovered gear, e.g., cuts/breaks in wire rope jacketing and failure of stretch hoses.
Field Verification
Field verification sampling is an important component of all OOI cruises to determine whether data coming from sensors are reliable when first deployed. This is done using a variety of sensors and sampling, including shipboard meteorological, upper ocean and flow-through systems, CTD casts, bottle samples, and deployment of co-located sensors. Field verification data have been used to confirm the correct assignment of configuration metadata (calibration coefficients) and update software algorithms used to process raw data into derived data products.
Recommendations for further enhancement of field verification include, (1) increasing use of OOI sensors in place of ship sensors; (2) increasing verification of buoy meteorological sensors either by remaining onsite longer and/or developing mechanisms to compare ship and mooring data in real time; (3) adopting a common format for CTD sampling logs; (4) modeling platform-specific flow distortion of OOI buoys; (5) evaluating advantages of having additional data processing skills onboard; and (6) increasing ease of access for users to verification data.
Platform and Sensor Performance Assessment
Quantitative performance assessment of infrastructure while deployed and upon retrieval is critical to improving operational performance. A collective, comprehensive performance assessment mechanism is a future goal of the OOI. These metrics will help to optimize the OOI and inform the broader observing community of performance issues and solutions. Though a comprehensive system has yet to be developed, we include metrics where possible in examples in the following sections.
Cables and Connectors
All OOI cables are serialized and tested allowing for results to be tracked to identify trends and determine appropriate replacement cycles (e.g., neoprene cables do not appear to hold up as well as polyurethane). The OOI has collaborated with vendors to improve quality control and capture data to calculate component life cycle, predict failure, improve platform reliability, and reduce refurbishment costs. For example, between 2015 and 2017 the percentage of cables that failed the manual 50 V insulation test dropped from 30 to <5%. After the initial failure, it was discovered that the majority of the failed cables used one specific connector. Working closely with the vendor, a leak path was discovered, generated by cathodic delamination between the metal connector shell and the polyurethane material that molds it to the polyurethane cable. The vendor modified the connector design and molding process and the following year all cables passed the post-recovery test. By engaging with vendors, these technological enhancements and best practices are made more broadly available to the global observing community.
In 2016 the program shifted from manual testing to an automated cable test system for all copper wire cables. Consistent procedures have resulted in improvements in testing speed, accuracy, and data storage. Whereas manual testing for 2,500 cables in 2015 required the work of 1.1 full time employees, the automated testing of 2,000 cables in 2017 only required 0.24 full time employees. Additional adjustments include the implementation of a visual inspection regimen and cable protection and handling best practices (e.g., cages, shipping containers, strain relief, service loops, and lubrication). Lastly, instrument cables are now tested as soon as possible after recovery, since faults can disappear when cables are tested dry.
Profilers
The OOI incorporates multiple types of profilers: Coastal and Global Wire-Following Profilers (WFPs), Coastal Surface-Piercing Profilers, Cabled Deep Profilers, and Cabled Shallow Profilers. Two additional profilers (Global Array Surface-Piercing Profilers, and Endurance Array Cabled Surface-Piercing Profilers) were descoped due to poor reliability. The OOI has made significant design improvements to the reliability of the remaining profilers.
Profilers intended for long, unattended deployments on the OOI have substantial design challenges compared to fixed instrument moorings, yet offer unique advantages including collection of continuous vertical measurements. In the following subsections, we describe some challenges and successes.
Wire-Following Profilers
The Coastal and Global WFPs and Cabled Deep Profilers are based on the McLane Moored Profiler, from McLane Research Labs, Inc. The cabled profilers are additionally modified to add Wi-Fi and battery recharge capabilities. In general, OOI WFPs are robust and have been successfully deployed and maintained across the program. However, some notable operational issues remain, such as slippage, fouling, docking challenges, and power limitations.
Slippage describes the situation when the drive wheel of a WFP fails to maintain traction with the wire. Slippage occurs at either end of the profiler path, but usually at the top. This is likely associated with mooring wire motion from waves and currents, which tends to be greater near the sea surface. Parking at the top of the profile can lead to additional issues for the WFPs. Extended time in the euphotic zone can exacerbate biofouling of the onboard instruments (e.g., fluorometers and PAR sensors lack biofouling mitigation strategies). To reduce the threat of biofouling and slippage, round-trip profiling allowing parking at the bottom is used when possible.
Improvements were made to the Cabled Deep Profiler Moorings to mitigate docking challenges. The Wi-Fi antenna in the dock was redesigned allowing a good wireless connection when the profiler is parked in any orientation in the dock.
Cabled Shallow Profilers
Winched Science Pods on the Cabled Shallow Profiler Moorings have been highly reliable, as have the instruments on the accompanying 200-m stationary platforms. Some profiler issues have been noted, including failure of a dynamic seal, cable wear, and an oil leak; however, since 2015 they have successfully completed more than 27,000 profiles.
Coastal Surface-Piercing Profilers
Coastal Surface-Piercing Profilers (CSPPs) provide unique data as they are the only OOI profilers to sample across the air-sea interface. However, they also have had performance challenges. The cabled Endurance CSPP was replaced with an uncabled version due to tether-handling issues with the winch when the profiler was near the air-sea interface and insufficient power was available from the seafloor cable due to the needed cable length. The uncabled CSPP can adaptively respond to wave motions to prevent overwrapping from snap loads, using minimal power. CSPP deployments have also been suspended at the Pioneer Array due to issues such as battery problems and breakaways. Breakaways are more problematic at Pioneer as the moorings are further offshore than at Endurance where successful uncabled CSPP deployments have taken place.
Across Endurance Array deployments, uncabled CSPPs have made round-trip profiles (upcast and downcast) 70% of planned days. The main cause of profiler stoppages is power system control problems. While improvements have been made (redesigning the battery interface, switching units from Iridium to cellular when close to shore, lengthening the antenna, etc.), some electrical, firmware, and communications issues remain.
Coastal Surface-Piercing Profilers are relatively new to ocean observing and their technology is constantly evolving as more is learned about these platforms during deployment. Work will continue to refine these profilers since they are of considerable scientific value and are the OOI’s least expensive platform to buy or service.
Surface Moorings
After initial test deployments, the original design for the Coastal and Global surface buoys was improved by inclusion of hydrogen gas detection (to monitor and ensure safe hydrogen levels in the buoy well), as well as enhancements to charging circuitry, and tower design (Figures 2A,B). Additionally, these moorings were modified to better adapt to harsh conditions, including winds, waves, and icing. For example, during winter at the Irminger Sea Array, sub-zero air combined with waves and wind-driven spray have led to icing of the surface meteorology and air-sea flux sensors, interrupting their data collection. Technical upgrades, including heating of the buoy superstructure to prevent ice build-up, are being investigated. Work to upgrade the meteorological sensors to better cope with icing conditions and improve meteorological sensor placement to avoid flow distortion is being continually evaluated. Additional challenges include generating sufficient power during times of little wind combined with extended darkness.
Biofouling Mitigation
Biofouling is an issue for a number of instruments, particularly those making optical measurements. Wipers and shutters have proven effective in keeping optical surfaces clean. The OOI implements additional biofouling mitigation measures to those provided by instrument vendors, including copper tape and silicon bronze hardware at the shallow Endurance Array sites. The use of UV-light has also been tested and is being implemented on Endurance Array cameras and Coastal and Global dissolved oxygen sensors (Figures 2E–H).
Technology Refresh: Process and Priorities
Technology refresh refers to all deployed infrastructure (e.g., sensors, platform elements, cables, and connectors). The need for a refresh process is motivated by the need for improved instrument and platform reliability and data quality, deprecation of an instrument by its vendor, and/or the existence of new instruments.
The OOI is creating a long-term programmatic strategy for technology updates. To guide this process, prioritization matrices are being developed based on lessons learned during operations for sensors, platforms, and software tools. Input from within the program, its advisory structure, and subject matter experts (SMEs) are being solicited for matrix development. These matrices will serve as programmatic best practices documentation and guidance for program planning.
Suspension of Southern Hemisphere Arrays
Budget pressures on the OOI are reflected in the decision by the NSF to scale back the Global Arrays as suggested in the “Sea Change” Decadal Survey Summary Report (National Research Council, 2015). The Argentine Basin Array has been fully terminated. Infrastructure at the Southern Ocean Array has been reduced to only the surface mooring, which is being continued with collaboration from the Natural Environment Research Council in the United Kingdom for an additional year.
Community Engagement
The OOI is operated as a community facility with open access to scientists, educators, and the general public. Scientists and educators can submit proposals to utilize and modify the system (adding sensors, adjusting sampling rates) and anyone can download the data from the website for free.
Since the NSF announced in 2016 it was accepting proposals to add onto OOI infrastructure, there have been 8 awards (∼20 instruments) made from NSF, United State National Aeronautics and Space Administration, United States Office of Navy Research, and the German Federal Ministry of Education and Research to add infrastructure onto the Cabled Array. The awards span two to 5 years and extend from Axial Seamount to Southern Hydrate Ridge. The instruments span geophysical – geodetic-focused sensors to examine deformation at Axial Seamount with follow-on applications at the Cascadia subduction zone, multibeam sonars to quantify the spatial and temporal evolution of methane plumes at Southern Hydrate Ridge, a multibeam sonar to image diffuse, high temperature venting at Axial Seamount, and a suite of laser raman spectrometers for an exobiology study at the ASHES vent field. A Natural Environment Research Council-funded proposal has added nutrient sensors to the Southern Ocean Surface Mooring, and an NSF-funded proposal has adapted oxygen sampling in the Irminger Sea Array.
Not only is the OOI a resource for use by the oceanographic community, it relies on input from the broad knowledge base of the community. Community engagement allows the OOI to maximize its scientific and educational value through ancillary activities on OOI maintenance cruises (e.g., Bigham, 2018; Reimers and Wolf, 2018) and engagement with students6 and SMEs7 to assist with quality control efforts. The wide diversity of data types and specialized sensors requires additional effort, including SME validation of data and consistent treatment of metadata.
Discussion
As described in Lindstrom (2018), the OOI helps fill a niche within the global community in the development, testing, and operation of sensors and platforms for using within an observing system. Given the diversity and volume of instruments and cables deployed long-term, the OOI has acted in many ways as a de facto laboratory and field-testing group for vendors and the oceanographic community. The development of OOI internal instrument testing pipelines and best practices as discussed in this paper can serve as a model for observatories across the globe.
Lessons have been learned in many areas since construction of the OOI began in 2009 and particularly since operations began in 2014, this paper focuses on lessons in technological development and quality control. These and future lessons in instrument and platform development, testing, deployment, and maintenance can provide a wealth of information to the global observing community as they seek to develop sustained observation systems at a variety of scales.
Author Contributions
KY, MK, JB, JF, MH, OK, DK, MP, AP, MV, and SW attended the OOI Lessons Learned workshop in May 2018 and contributed to the creation of an internal lessons learned report. MK led the writing of the internal report. LS wrote this paper based on that report with substantial contributions from KY and LV. AP, JF, RW, SW, JB, GC, MH, OK, MV, PM, and KN edited the manuscript, providing corrections, clarifications, and additions to the text, and images.
Funding
Preparation of this manuscript was funded by the United States National Science Foundation through a Cooperative Support Agreement with the Consortium for Ocean Leadership (OCE-1026342).
Conflict of Interest Statement
The authors declare that the research was conducted in the absence of any commercial or financial relationships that could be construed as a potential conflict of interest.
Acknowledgments
We thank the many scientists, engineers, technicians, students, captain and crews, field teams, and support staff who have worked on the OOI program. We thank the vendors and manufacturers who have worked with the OOI through many of the challenges and successes in this paper. Lastly, we also thank the OOI user community.
Footnotes
- ^ https://oceanobservatories.org/
- ^ https://ooinet.oceanobservatories.org
- ^ https://alfresco.oceanobservatories.org
- ^ https://oceanobservatories.org/planning-history/final-network-design/
- ^ https://oceanobservatories.org/information-for-researchers/
- ^ https://oceanobservatories.org/data-workshops/
- ^ https://oceanobservatories.org/researchers/subject-matter-experts/
References
Barth, J. A., Fram, J. P., Dever, E. P., Risien, C. M., Wingard, C. E., Collier, R. W., et al. (2018). Warm blobs, low-oxygen events, and an eclipse: the ocean observatories initiative endurance array captures them all, oceanography. Oceanogr. Soc. 31, 90–97. doi: 10.2307/26307792
Bigham, K. (2018). Axial seamount biology catalog. Oceanography 31:127. doi: 10.5670/oceanog.2018.119
Chen, K., Gawarkiewicz, G., and Plueddemann, A. (2018). Atmospheric and offshore forcing of temperature variability at the shelf break: observations from the OOI pioneer array. Oceanography 31, 72–79. doi: 10.5670/oceanog.2018.112
Femke, D. J. M., Oltmanns, M., Karstensen, J., and de Steur, L. (2018). Deep convection in the irminger sea observed with a dense mooring array. Oceanography 31, 50–59. doi: 10.5670/oceanog.2018.109
Henderikx Freitas, F., Saldías, G. S., Goñi, M., Shearman, R. K., and White, A. E. (2018). Temporal and spatial dynamics of physical and biological properties along the endurance array of the California current ecosystem. Oceanography 31, 80–89. doi: 10.5670/oceanog.2018.113
Lindstrom, E. (2018). On the relationship between the global ocean observing system and the ocean observatories initiative. Oceanography 31, 38–41. doi: 10.5670/oceanog.2018.107
National Research Council. (2015). Sea Change: 2015-2025 Decadal Survey of Ocean Sciences. Washington, DC: The National Academies Press, doi: 10.17226/21655
Nooner, S. L., and Chadwick, W. W. (2016). Inflation-predictable behavior and co-eruption deformation at axial seamount. Science 354, 1399–1403. doi: 10.1126/science.aah4666
Ogle, S. E., Tamsitt, V., Josey, S. A., Gille, S. T., Ceroveèki, I., Talley, L. D., et al. (2018). episodic southern ocean heat loss and its mixed layer impacts revealed by the farthest south multiyear surface flux mooring. Geophys. Res. Lett. 45, 5002–5010. doi: 10.1029/2017GL076909
Palevsky, H. I., and Nicholson, D. P. (2018). The North Atlantic biological pump: insights from the ocean observatories initiative irminger sea array. Oceanography 31, 42–49. doi: 10.2307/26307786
Philip, B. T., Denny, A. R., Solomon, E. A., and Kelley, D. S. (2016). Time-series measurements of bubble plume variability and water column methane distribution above southern hydrate ridge, Oregon. Geochem. Geophys. Geosyst. 17, 1182–1196. doi: 10.1002/2016GC006250
Reimers, C. E., and Wolf, M. (2018). Power from benthic microbial fuel cells drives autonomous sensors and acoustic modems. Oceanography 31, 98–103. doi: 10.5670/oceanog.2018.115
Smith, L. M., Barth, J. A., Kelley, D. S., Plueddemann, A., Rodero, I., Ulses, G. A., et al. (2018). The ocean observatories initiative. Oceanography 31, 16–35. doi: 10.5670/oceanog.2018.105
Spietz, R., Butterfield, D., Buck, N., Larson, B., Chadwick, W., Walker, S., et al. (2018). Deep-sea volcanic eruptions create unique chemical and biological linkages between the subsurface lithosphere and the oceanic hydrosphere. Oceanography 31, 128–135. doi: 10.5670/oceanog.2018.120
Wilcock, W. S., Tolstoy, M., Waldhauser, F., Garcia, C., Tan, Y. J., Bohnenstiehl, D. R., et al. (2016). Seismic constraints on caldera dynamics from the 2015 Axial Seamount eruption. Science 354, 1395–1399. doi: 10.1126/science.aah5563
Keywords: ocean observing, lessons learned, technology development, best practices, equipment testing
Citation: Smith LM, Yarincik K, Vaccari L, Kaplan MB, Barth JA, Cram GS, Fram JP, Harrington M, Kawka OE, Kelley DS, Matthias P, Newhall K, Palanza M, Plueddemann AJ, Vardaro MF, White SN and Weller RA (2019) Lessons Learned From the United States Ocean Observatories Initiative. Front. Mar. Sci. 5:494. doi: 10.3389/fmars.2018.00494
Received: 28 September 2018; Accepted: 06 December 2018;
Published: 04 January 2019.
Edited by:
Laura Lorenzoni, University of South Florida, United StatesReviewed by:
George Petihakis, Hellenic Centre for Marine Research (HCMR), GreeceAndrea J. Fassbender, Monterey Bay Aquarium Research Institute (MBARI), United States
Copyright © 2019 Smith, Yarincik, Vaccari, Kaplan, Barth, Cram, Fram, Harrington, Kawka, Kelley, Matthias, Newhall, Palanza, Plueddemann, Vardaro, White and Weller. This is an open-access article distributed under the terms of the Creative Commons Attribution License (CC BY). The use, distribution or reproduction in other forums is permitted, provided the original author(s) and the copyright owner(s) are credited and that the original publication in this journal is cited, in accordance with accepted academic practice. No use, distribution or reproduction is permitted which does not comply with these terms.
*Correspondence: Kristen Yarincik, kyarincik@oceanleadership.org