- 1Department of Biological Sciences, Macquarie University, Sydney, NSW, Australia
- 2Zoophysiology, Department of Bioscience, Aarhus University, Aarhus, Denmark
- 3Anderson Cabot Center for Ocean Life, New England Aquarium, Boston, MA, United States
- 4Sea Mammal Research Unit, Scottish Oceans Institute, University of St Andrews, St Andrews, United Kingdom
- 5School of Biological Sciences, The University of Auckland, Auckland, New Zealand
All three extant right whales [Eubalaena australis (Southern; SRW), glacialis (North Atlantic; NARW), and japonica (North Pacific; NPRW)] were heavily exploited, and the status of the two northern hemisphere species remains precarious. Recently, limited gains made by the NARW have been reversed and urgent changes to management approaches are needed if extinction is to be averted. By contrast, some SRW populations are recovering. Given their close phylogenetic relationship, morphological, demographic, and ecological similarities, the contrasting recovery rates between populations and species provide an opportunity to apply a comparative approach to inform the differences in recovery as follows. (1) Recovery: All right whale species were internationally protected in 1931, but NARW, eastern NPRW and some SRW populations have barely recovered from whaling, while others are doing so at maximal rates. Are these differences a legacy of extreme depletion (e.g., loss of genetic diversity and cultural knowledge) or primarily due to anthropogenic factors (e.g., high mortality from ship strike and fisheries entanglement)? If modern anthropogenic threats are not affecting remote SRW populations, can these serve as baseline populations for comparison with NARW and NPRW? (2) Linking individuals to population-level responses: In wild mammals, strong links exist between reproductive indices and environmental conditions within the context of life-history strategies. Individual identification of whales provides the ability to track survival, reproduction and other demographic parameters, and their population-level consequences, providing the tools with which to uncover these links. Robust life-history analyses are now available for NARW and several SRW populations, linking demography with environmental conditions, providing the potential for teasing out important influencing factors. (3) Adapting to shifting resources: Recent reproductive declines in NARW appear linked to changing food resources. While we know some large-scale movement patterns for NARW and a few SRW populations, we know little of mesoscale movements. For NPRW and some SRW populations, even broad-scale movements are poorly understood. In the face of climate change, can methodological advances help identify Eubalaena distributional and migratory responses? (4) Emergent diseases and the vulnerability of populations under stress: Marine mammals are vulnerable to infectious diseases, particularly when subjected to stressors such as fishing gear entanglements, acoustic disturbance, and prey shortages. New tools to assess large whale health include body condition imaging, viromes, microbiomes, as well as metabolic and stress hormones. Comparative analysis of the three Eubalaena spp. could identify causes of varying recovery. (5) Comparative synthesis and cumulative effects: The lack of a good analytical approach for cumulative effects is an urgent bio-statistical problem in conservation biology. Without such a framework every stressor is managed in isolation, limiting efficacy. We propose a comparative synthesis to inform future cumulative effect analyses and outline future research priorities to achieve these goals.
Recovery
Introduction – Exploitation/Devastation
The Eubalaena genus comprises three species of right whale that have the dubious honor of being named by whalers for their desirability: North Atlantic (Eubalaena glacialis), North Pacific (E. japonica), and southern (E. australis) right whales (Braham and Rice, 1984; Rosenbaum et al., 2000). The ‘right’ whale to kill, these species are large baleen whales that tend to float when dead and yielded long baleen plates (known as whalebone historically), and much valuable oil that was used in industries from cosmetics to commercial lubricant (Allen, 1916; International Whaling Commission [IWC], 2001). The family (Balaenidae) also includes a fourth member, the bowhead whale (Balaena mysticetus), which was also heavily exploited but the Pacific population has had a major recovery and numbers more than the combined populations of the other three species (Givens et al., 2016). Exploitation of right whales began in earnest in the eastern North Atlantic with Basque shore and Basque pelagic whaling as early as 1000 AD (Reeves and Smith, 2006). The Basque shore-whaling techniques were exported to the South Atlantic by 1603. In the North and South Pacific, following the decline of Basque whaling, American shore and pelagic whaling methods predominated, appearing in the southern Indo-Pacific by 1805. Within 20 years the French whaling vessel Gange became the first pelagic whaling vessel to venture north of 50°N where it harpooned the first NPRW in 1835 (Reeves and Smith, 2006).
Shore whaling targeted right whales on their wintering grounds, as these are generally in sheltered coastal waters. Shore stations set up in these regions routinely killed calves as a way to secure the larger, more valuable, female, as this description from 1844 shows; “the object is to harpoon the calf …the maternal affection of the whale causes it to follow the calf till it gets ashore” (New Zealand Blue Book, 1841). Females show fidelity to nursery grounds, frequently returning to the same bay to calve, so shore stations were successful for several years until they had wiped out those whales philopatric to a particular region. Offshore whaling, although termed Basque or American (“Yankee”) whaling due to its origins, was in fact conducted by many nations, including British, French, Norwegian and Dutch, and was indiscriminate in its killing of right whales (Reeves et al., 1999; Richards, 2009; Smith et al., 2012).
The decline of the SRW is the best understood of all the right whales. It is estimated that prior to whaling there were approximately 120,000 SRW found in 12 wintering grounds (Figure 1, International Whaling Commission [IWC], 2001, 2012; Jackson et al., 2008). However, between 1790 and 1971 up to 150,000 SRW were killed, leading to a hemispheric decline to as few as 400 whales around 1920 (International Whaling Commission [IWC], 2001, 2012; Jackson et al., 2008). Full protection from commercial whaling was provided for all right whale species under the Convention for Regulation of Whaling (CRW) in 1931 under the League of Nations. Japan did not accede to the CRW, but the CRW provided the framework for the future regulation of whaling that continues until today as the 1946 International Convention for the Regulation of Whaling (ICRW). Both the Soviet Union and Japan acceded to the ICRW. However, like the CRW, the ICRW was not perfect and even as the various species recovered from historical whaling, they were targeted illegally by the Soviet whaling fleet in the 1960s and 1970s. For the SRW, this killed half the extant population at the time (Tormosov et al., 1998; Jackson et al., 2008). Today, the SRW is estimated to have recovered to 12,000–15,000 individuals across its circumpolar distribution (Figure 1; International Whaling Commission [IWC], 2012).
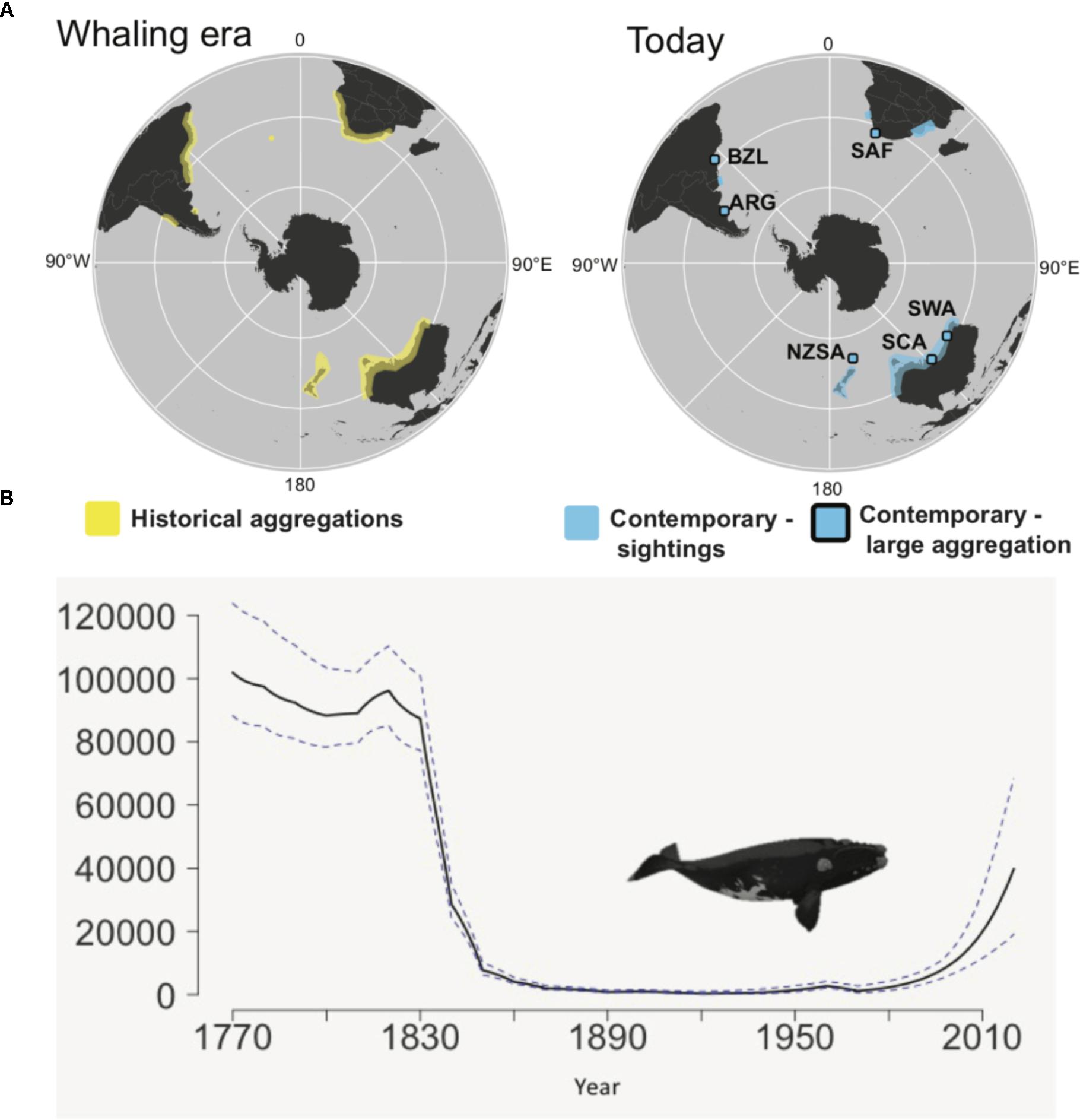
Figure 1. Change in distribution and abundance of southern right whales. (A) Shows historical and contemporary wintering distributions (Figure 1 from Carroll et al., 2018), and (B) shows decline in abundance and subsequent recovery (solid line is the mean, dashed line shows upper and lower 95% CI). Modified Figure 1 from Jackson et al. (2008). Contemporary sightings are divided into regions where large aggregations are seen during winter: Argentina (ARG), Brazil (BZL), South Africa (SAF), southwest Australia (SWA), south central Australia (SCA), and New Zealand sub-Antarctic (NZSA) and regions where sightings are typically of small numbers of individuals per year. The large aggregations are IWC management units and correspond to historical whaling grounds, although another 5 whaling grounds show little sign of recovery. Summer feeding areas are poorly described and so not shown.
NARW have a much longer and poorly documented catch history, and the ability to estimate historical abundance for this species has proved challenging. Reeves et al. (2007) used catch records, whale oil, and baleen import records to estimate at least 5500 (and possibly twice that number) NARW were killed in the western North Atlantic between 1634 and 1950 and suggested a population of at least a few thousand whales in the mid-1600’s. Early studies indicating that Basque whalers took tens of thousands of NARW in the 1500’s from eastern Canada have been corrected by genetic analyses of bones from the Labrador whaling stations. These show that Basque whalers took primarily bowheads, and rarely caught right whales, leading to suggestions that small population size may be a long-term characteristic of this species (Rastogi et al., 2004; McLeod et al., 2008). Nevertheless, Reeves et al. (1992) suggested that fewer than 100 right whales were alive at the end of the 1700’s, and Katona and Kraus (1999) hypothesized that only a few dozen survived by the early 1900’s (Figure 2). As of 2015, there were an estimated 458 individuals in the western NARW population (Pace et al., 2017).
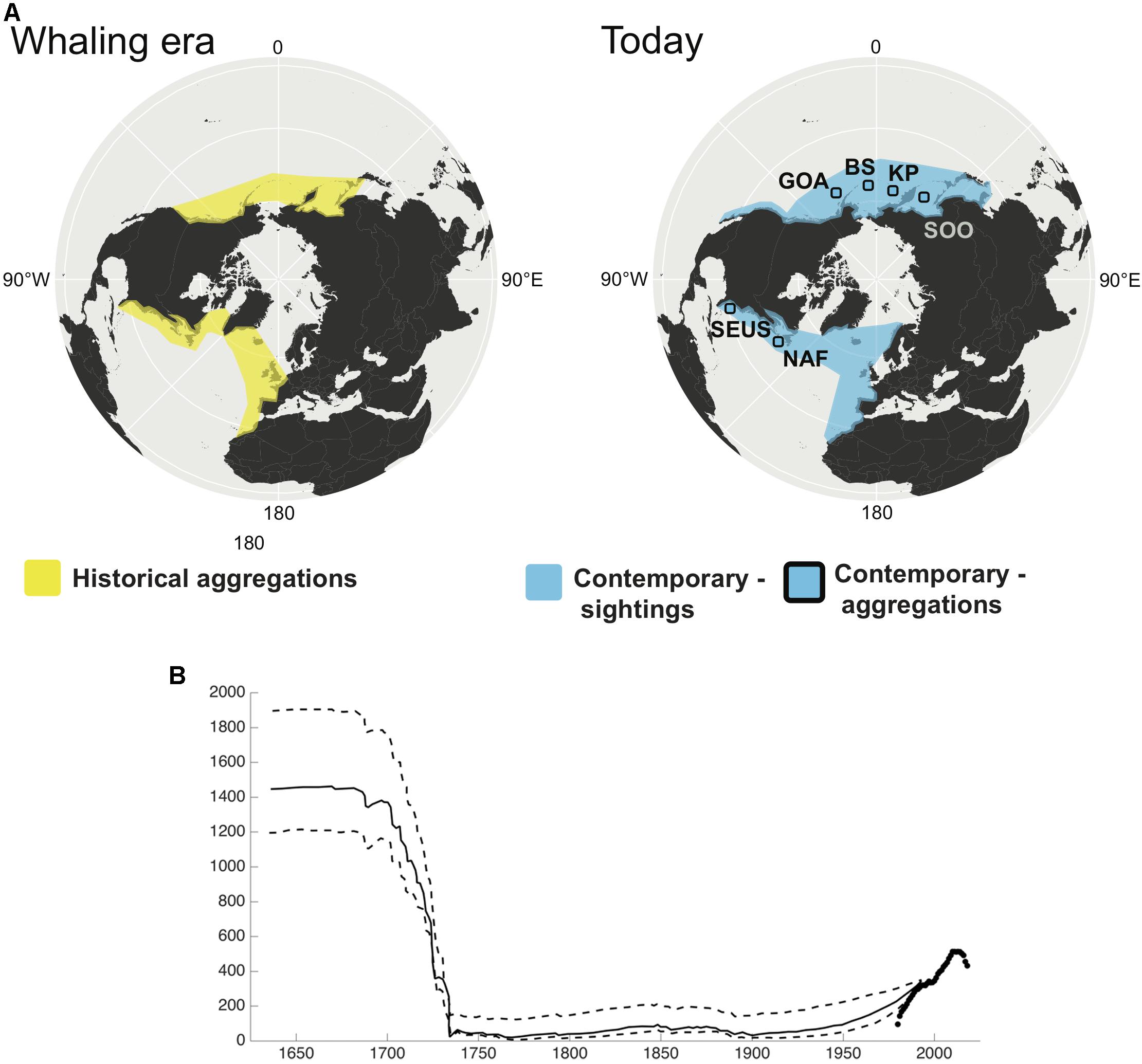
Figure 2. (A) Change in distribution of North Pacific and North Atlantic right whales. The North Pacific right whale was hunted across the North Pacific, although the whaling grounds were primarily summering grounds and no wintering areas were identified. This mystery continues today, as the contemporary distribution of these two stocks are characterized by small aggregations seen in summer feeding grounds in the Gulf of Alaska (GOA) and southeast Bering Sea (BS) in the east, and the Sea of Okhotsk (SOO) and the Kamchatka Peninsula (KP) in the west. The North Atlantic right whale was hunted around the New England coast during spring months in the northwest Atlantic, and around the southeast United States coast during winter. Whales were occasionally seen and killed north of New England up to Greenland. In the northeast Atlantic, the species was found off Greenland, Iceland and the United Kingdom in the summer months, and the Bay of Biscay and Cintra Bay, Western Sahara, during the winter. There are now no regular aggregations in the northeast Atlantic, although there are occasional sightings. In the northwest Atlantic, there is a well-described winter calving ground off the coast of Florida and Georgia, United States (SEUS), and summer feeding grounds around the Bay of Fundy and Nova Scotia, off the coast of North America (NAF). The North Pacific and North Atlantic right whales are, by IWC convention, divided into east and west stocks. Recent genetic data supports differentiation of eastern and western stocks of North Pacific right whales (Pastene et al., 2018). (B) Modeled population trajectory of North Atlantic right whales off the eastern United States, with Maximum Sustainable Yield rates of 0.03 (solid line) and 0.01 and 0.05 (dashed lines; modified from Reeves et al., 1992). Black points are from modern observations of the minimum number alive from the North Atlantic right whale catalog (NARWC, 2018).
Two centuries of aboriginal, commercial, and then illegal Soviet whaling significantly reduced the NPRW (Scarff, 1991, 2001; Ivashchenko and Clapham, 2012; Ivashchenko et al., 2013). There are two distinct populations of NPRW, on the eastern and western sides of the Pacific (Gregr and Coyle, 2009), which are genetically distinct (Pastene et al., 2018). Early estimates based on sightings data suggested the species as a whole totaled 100–200 whales (Wada, 1973; Sekiguchi et al., 2014; Ovsyanikova et al., 2015). For the western stock, surveys by Miyashita and Kato (1998) in summers of 1989, 1990, and 1992 in the Okhotsk Sea estimated abundance at 922 (95% CI 404–2108). More recently, surveys by Hakamada and Matsuoka (2016) suggest an abundance estimate of 1,147 individuals on the feeding ground off the southern end of the Kamchatka Peninsula in 2011 and 2012. In contrast, the eastern stock is on the order of 20–30 times smaller. Mark-recapture estimates for the eastern NPRW stock from photographic (18 identified individuals) and genotype (21 identified individuals) data through 2008 were 31 (95% CI: 23–54) and 28 whales (95% CI: 24–42), respectively (Wade et al., 2011b) and the photo-ID and genetic catalogs have 20 and 23 unique individuals, respectively (1997–2011; LeDuc et al., 2012; Muto et al., 2017). Confirmed sightings since 1996 (Goddard and Rugh, 1998), and a recent increase in effort have led to more frequent detections in the region, including calves (Wade et al., 2006, 2011a; Zerbini et al., 2015; Ford et al., 2016).
All contemporary right whale populations reflect remnants of their pre-whaling populations. As such, they appear to occupy a portion of their historical ranges (Figures 1, 2). Whaling logbooks have been useful in describing the distribution of the species prior to and during their exploitation (Dawbin, 1986; Du Pasquier, 1986; Reeves et al., 1992; Clapham et al., 2004; Reeves et al., 2007; Smith et al., 2012; Carroll et al., 2014; Rocha et al., 2014; Thomas et al., 2016) as well as identifying potential habitats that members of the modern populations may be re-exploiting.
While this short summary demonstrates the dramatic impact of whaling, it does not capture the spatially variable patterns of decline and, in some cases, recovery. There are several aspects of right whale ecology, population dynamics, as well as anthropogenic impacts that need to be considered when contemplating why there has not been uniform recovery across populations or stocks. Each of these are explored with the intent of providing an overarching discussion of the roles of intrinsic and extrinsic drivers of recovery in the different species of right whales.
Migratory Culture, Extirpation and Recovery
SRW have a circumpolar distribution and rates of recovery have varied from near maximum growth rates (rm) in some populations (southwest Australia, South Africa, East South America, and subantarctic New Zealand), to poor or not measurable in others. We now know that SRW show a form of migratory culture, with females transmitting preferences for both winter calving/breeding areas and summer foraging areas to their calves during the 1st year of life (Valenzuela et al., 2009; Carroll et al., 2015, 2016). This has led to genetic structuring across the species’ migratory network (Patenaude et al., 2007; Carroll et al., 2015; Carroll et al., 2018), and has been invoked to explain the species’ patchy recovery (Clapham et al., 2008; Carroll et al., 2011). Essentially, when whales inhabiting a region were extirpated, the memory of that area as a good migratory destination was also lost. This loss of ‘cultural memory’, exacerbated by low density and loss of adjacent populations, mean it is unlikely that once-inhabited areas will be recolonized on a timeframe relevant to management (i.e., decades; Clapham et al., 2008). This may help explain the lack of recovery around mainland New Zealand and east Australia wintering grounds compared with the strongly recovering populations in the New Zealand sub-Antarctic Islands and southwest Australia (Carroll et al., 2011, 2015). Comparable findings from the NARW show female philopatry to summering grounds (Malik et al., 1999), and population structuring that is probably due to migratory patterns of behavior (Schaeff et al., 1993). NPRW also show significant differentiation in maternally inherited DNA markers between Eastern and Western stocks that are likely partially attributable to female philopatry and stock identity (Pastene et al., 2018). However, historical bottlenecks may have influenced population structuring and migratory behavior prior to major whaling impacts (Waldick et al., 2002).
While vertical transmission (cow to calf) of migratory destinations provides valuable information on suitable foraging and nursery areas in a vast ocean (Whitehead, 2007, 2010), decisions conserved across generations can become problematic in the face of environmental change (Keith and Bull, 2017). This is because of the potential for ecological or evolutionary traps; a behavior that originally increased fitness (e.g., fidelity to rich feeding ground) becomes a hindrance in the face of rapid environmental change (e.g., changes in distribution of food resources due to climate variation and/or anthropogenic activities: Schlaepfer et al., 2002; Keith and Bull, 2017).
This information is critical to understanding the variable rates of recovery of all right whales; foraging ground quality seems to be a strong determinant of the reproductive rate of SRW populations, and hence recovery rates (Leaper et al., 2006; Seyboth et al., 2016). The Patagonian Shelf, and South Georgia, south of the Polar Front, are an important feeding ground for the Argentina SRW population based on stable isotope, photo-ID and satellite track data. This high productivity means that some of these foraging grounds are shared with whales that winter in South Africa (Table 1; Best et al., 1993; Moore et al., 1999; Rowntree et al., 2001; Valenzuela et al., 2009; Mate et al., 2011; Zerbini et al., 2016). Both of these populations are thriving; although the Argentinean population is now increasing at a slower rate (Crespo et al., 2018) and it has been subject to a recent die off (Rowntree et al., 2013). In the NARW, there are strong links between prey availability, condition, and fecundity: poor prey years lead to low population health and body condition, and longer inter-birth intervals (Hlista et al., 2009; Miller et al., 2011, 2012; Fortune et al., 2013; Rolland et al., 2016; Meyer-Gutbrod and Greene, 2018). NARW move between habitats, especially foraging grounds, many times within a season (Mate et al., 2011; Baumgartner et al., 2017). Connectivity to alternative feeding grounds could buffer a population against poor conditions in a single feeding ground, ensuring that some of the population had sufficient resources to reproduce.
However, some feeding grounds of the SRW appear to have been completely abandoned, and this may be due to a loss of cultural memory rather than resource quality. For example, New Zealand right whales historically used foraging grounds around the Chatham Rise and Louisville and Kermadec ridges (Smith et al., 2012; Jackson et al., 2016), but there are no contemporary records of the whales from this region (Carroll et al., 2014).
Fortunately, like NARW, there is evidence of mixed foraging strategies within populations of SRW with whales visiting multiple foraging grounds, both within and between years. Satellite tagging in Argentinean wintering grounds showed that some whales will visit both the Patagonian Shelf and South Georgia in a single season (Zerbini et al., 2016). This is consistent with findings reported by Rowntree et al. (2008), who used isotopic signals in baleen plates to suggest a mixture of foraging strategies. Some whales appeared to specialize in either low- or high-latitude foraging grounds but not both, while others exhibited a mixture of latitudes over the 6–8 years represented in the baleen record (Rowntree et al., 2008). It remains to be seen whether right whales are able to learn from each other, and horizontally transmit foraging-ground preferences. Horizontal transmission of behavior or information could facilitate spread of innovation; in this case, potentially new foraging grounds (Dautenhahn and Nehaniv, 2002; Keith and Bull, 2017); and could buffer right whales from changes in prey distribution and abundance driven by climate.
Inverse Density Dependence: Genetic Diversity and Stochastic Demographic Influences on Recovery
Standard density-dependent population theory, exemplified by logistic growth models (Tsoularis and Wallace, 2002), holds that populations reduced to low densities should in the absence of migration increase at close to the maximum biological rate. There is some evidence from the whaling era to support density-dependent changes in growth curves and fecundity in sperm whales (Kasuya, 1991) and other cetacean species (Fowler, 1984), but these data may be erroneous (NOAA, 2017) and cannot be relied upon. However, the lack and/or slow recovery of many populations of SRW, has led to speculation about the role of inverse density dependence at low densities or the Allee effect (Baker and Clapham, 2004). There are generally three primary categories of Allee effects; inbreeding, or a loss of heterozygosity; demographic stochasticity; and the reduction in cooperative interactions when there are fewer individuals (Allee, 1931; Courchamp et al., 1999).
Loss of genetic diversity due to demographic bottlenecks depends on the severity of the event, in terms of effective population size, and its duration, in terms of generations (Nei, 1975; Allendorf, 1986). Genetic erosion can lead to inbreeding, maladaptation, and loss of adaptive potential, increasing the risk of population extinction (Leroy et al., 2017). Simulation studies suggest that the centuries-long demographic bottleneck due to whaling did reduce mitochondrial genetic diversity in SRW (Jackson et al., 2008), with Indo-Pacific populations showing significantly lower mtDNA diversity than their South Atlantic counterparts (Carroll et al., 2018). However, it is not yet resolved whether this has impacted nuclear DNA diversity, as microsatellite studies show high levels of heterozygosity in the extant SRW wintering grounds (Carroll et al., 2018). In the North Pacific, LeDuc et al. (2012) analyzed 49 biopsy samples from 24 right whales, of which all but one were from the eastern North Pacific. The analysis revealed a male-biased sex ratio and a loss of genetic diversity that appeared to be midway between that observed for right whales in the North Atlantic and the Southern Hemisphere. The analysis also suggested a degree of separation between eastern and western populations, a male:female ratio of 2:1, and a low effective population size for the Eastern North Pacific stock, which LeDuc et al. (2012) considered to be at “extreme risk” of extirpation. In contrast, the NARW has long been recognized as having low levels of genetic diversity, although genetic erosion does not seem to have coincided with 19th century whaling (Waldick et al., 2002; McLeod et al., 2010). It is possible that the NARW historically had a lower effective population size and hence harbored lower genetic diversity than its more numerous southern counterpart. Today, the high variability in male reproductive success seen in NARW, less prominent in SRW, could contribute to further lowering effective population size by limiting genetic input from all apart from those few successful males (Frasier et al., 2007; Carroll et al., 2012).
Demographic stochasticity in inverse density dependent effects are most likely to enact through sex ratio fluctuations in very small populations and this may be impeding the recovery of the eastern NPRW. Wade et al. (2011b) estimated the eastern NPRW population contained only eight females (95% CI: 7–18) and 20 males (95% CI: 17–37). Fujiwara and Caswell (2001) suggested that demographic stochasticity was less likely to impact the NARW population, but Pace et al. (2017) have shown a strong trend to a male biased sex ratio that is worrisome. The current estimate of breeding females is 105, in a population of 458. Equivalent data for SRW is lacking for the smallest sub-populations where demographic stochasticity might be enacted.
Anthropogenic Impacts on Recovery
The role of anthropogenic impacts on right whale recovery is best understood in the NARW, due to comprehensive population monitoring over the last four decades (Pettis, 2009; Rolland et al., 2016) and consistent necropsy effort (Moore et al., 2004, 2013; van der Hoop et al., 2013a). The toll of human-induced mortality is, and continues to be, high; from 1970 to 2009, 44% (38/87) of detected and diagnosed NARW mortalities were due to ship strikes and 35% (31/87) were due to entanglements (van der Hoop et al., 2013a), despite widespread regulations attempting to reduce these threats. Entanglement has now surpassed vessel strike as the leading cause of death to the population (van der Hoop et al., 2014): from 2010 to 2015, 15% of diagnosed mortalities were due to ship strikes and 85% were due to entanglements (Pettis and Hamilton, 2015; Hayes et al., 2017). Demographic models emphasize the importance of individual reproductive females in the population (e.g., Fujiwara and Caswell, 2001) and suggest that a loss of 4–6 females per year will lead the population to extinction (Meyer-Gutbrod and Greene, 2018). In 2017, at least 17 NARW mortalities were detected, including five diagnosed vessel-strike mortalities, and four entanglement mortalities, leading to the declaration of an Unusual Mortality Event (NOAA, 2017). As of 2009, 83% of NARW showed evidence of entanglement; 26% showed new entanglement scars every year, and 59% had been entangled more than once (Knowlton et al., 2012a). Rates of serious injury from entanglement (i.e., those that will likely lead to death, NOAA, 2008) have increased from on average 0.7/year (2000-2009) to >3/year (2010–2017) (van der Hoop et al., 2014; Hayes et al., 2017). The sublethal effects of entanglements have been quantified with respect to individual energetic cost (van der Hoop et al., 2016a; van der Hoop et al., 2017a), stress (Hunt et al., 2016; Pettis et al., 2017; Rolland et al., 2017; Lysiak et al., 2018) and reproductive output (Knowlton et al., 2012b), as is their role in combination with fluctuations in prey availability on population level health (Rolland et al., 2016).
The situation is comparatively positive for SRW populations. At its best, <1% of SRW in New Zealand show anthropogenic scarring (W. Rayment, pers. comm.) compared with the 83% for the NARW. Nevertheless, particularly for the smaller, more vulnerable populations there may still be critical impacts. While there have been ten ship-strikes with at least four mortalities and 28 entanglements with two deaths in Australian waters, the fatalities include mother and calf pairs from the small, remnant southeast population (Kemper et al., 2008; Carroll et al., 2015; Lanyon and Janetzki, 2016; Peel et al., 2018; Tulloch et al., unpublished). Vessel strikes to the Brazilian population still occur at low levels (<0.5 per year) but between 1999 and 2014, 38 entanglement cases were reported (Figueiredo et al., 2017; Groch, 2018). In Argentina, 6% of whales identified in 2007, 2008, 2009 seasons in Golfo Nuevo showed evidence of vessel interactions via scarring (International Whaling Commission [IWC], 2011). The potential for increased negative interactions between recovering right whale populations and increased human development has been identified as an issue of concern in Brazil (Figueiredo et al., 2017), New Zealand (Suisted and Neale, 2004), and eastern Australia (Carroll et al., 2015).
The level of human-induced mortality to NPRW is currently unknown, due to their rare occurrence and poorly known distribution (Muto et al., 2017); however, threats are assumed to be similar to other right whale populations and bowhead whales (Reeves et al., 2012) and their observed distribution does overlap heavy vessel traffic and fishing areas. The threats from vessels will only increase as Arctic shipping increase in the near future and the shipping lanes will be through the summer feeding habitat in the southeastern Bering Sea, as it will for their cousin the bowhead whale (Reeves et al., 2012; Pirotta V. et al., 2018). Despite the low likelihood of observing anthropogenic mortality or serious injury to NPRW in the eastern population, some live individuals show scars as evidence of fisheries interactions (Ford et al., 2016). For the much larger western NPRW population, 25 whales have been reported entrapped in fishing gear or stranded from Japan, South Korea, and Russia since 1996 and at least 10 of these are confirmed fishing mortalities (Brownell et al., 2001; Burdin et al., 2004: Brownell and Mallette, 2018).
Synthesis
Whaling nearly extirpated all right whale species worldwide. Numerous factors have influenced the recolonization and recovery of species and populations. The lack of obvious differences in anthropogenic impacts and to date, climate change, between SRW wintering grounds has led to intrinsic factors, such as migratory culture, extirpation and Allee effects, being inferred as strong drivers and/or inhibitors of recovery. In what is potentially the world’s smallest whale population, the eastern NPRW, demographic stochasticity could play a deciding role in population persistence if the proportion of females in the population does not increase. Finally, the NARW has been reduced to a single, small population that is highly susceptible to extrinsic factors influencing recovery. The combination of anthropogenic impacts, both lethal and sublethal, and environmental fluctuations, appear to shape health and reproductive success in the NARW (Corkeron et al., 2018). This has only been elucidated by long-term, scientific monitoring projects using a range of approaches combined with intensive necropsy efforts; next, we discuss how this has worked and how it may be applied elsewhere.
Linking Individual to Population-Level Responses
Introduction
The predictable aggregations of right whales that once attracted whalers are now the foundation of some of the longest-running, continuous whale studies in the world. These long-term datasets began with pioneering work that used natural markings to identify individuals and follow them through time (Kraus et al., 1986; Payne, 1986), and have been extended into multi-disciplinary studies (e.g., genetics, health) to gain a comprehensive understanding of population and individual status.
Catalogs
The foundation of long-term monitoring projects is the identification of individuals, typically using natural markings, but also DNA profiles. In right whales, individuals are identified using callosity patterns, lip ridges, unusual color or patches, or scarring (Kraus et al., 1986), from both aerial and boat-based photographs (Patenaude et al., 1998). Photo-identification studies started on NARW in the United States in the 1960s (Kraus et al., 1986), on SRW in South Africa, southwest Australia, and Argentina in the 1970s (Payne et al., 1981; Best, 1990), in Brazil in the 1980s (Groch et al., 2005), in New Zealand in the 1990s (Patenaude et al., 1998), and in Chile in the 2000s (Vernazzani et al., 2014). NOAA/NMFS began compiling the NPRW catalog in 2008, and this now includes photos from 1979 to present (Kennedy et al., 2012). DNA profiles, constructed using a combination of hyper-variable microsatellite markers and sex-specific loci, have also been used to identify individuals primarily in NARW, NPRW and SRW in the Indo-Pacific region (Frasier et al., 2007; Carroll et al., 2011; Wade et al., 2011b; Carroll et al., 2012, 2015).
The strength of these catalogs is derived from the ability to use individual recognition to record all of the significant events that occur in an individual’s life. This is best exemplified in the NARW where sightings locations, entanglement history, body condition, calving, tagging history and many other factors such as health and paternity can and are recorded. Having this detailed information provides us with immensely greater confidence in estimating demographic parameters and linking factors such as entanglement, environmental parameters, foraging success with reproductive output, health status and likelihood of survivorship.
Estimating Demographic Parameters
The ability to identify and track individuals across time is fundamental to assessments of population abundance and growth using open capture-recapture models for eastern NPRW and New Zealand SRW (Carroll et al., 2011, 2013; Wade et al., 2011b). These models are appropriate when heterogeneity in capture probability can be modeled by sex (i.e., genotype mark-recapture) or where there is no segregation by demographic class (e.g., feeding ground studies). Heterogeneity in female capture probability on wintering grounds is associated with reproductive cycles, i.e., females are more likely to be sighted in calving years, means that standard capture-recapture models cannot be used for estimating abundance or demographic trends in long-term photo-identification studies. Instead, stage-structured demographic models that estimate population parameters based on sightings of females in calving years are used to estimate abundance and trend in Argentina and South Africa (Cooke et al., 2015; Brandão et al., 2018). In southwest Australia, the total number of calving females seen in 3 years of aerial surveys has been assumed to represent the total number of reproductive females (see section “Linking Reproductive Success and Health to Environmental and Anthropogenic Factors”), which is then subject to a correction factor to estimate the total population size (Bannister, 2017). Population trends, estimated from the mark-recapture or stage-structured models, or from the linear regression analyses of aerial count data, show that most of the recovering SRW populations have been growing at ∼7% per annum (Table 1).
The NARW is one of the best-studied whale populations in the world, with concentrated survey efforts in the species’ known habitats since 1979 producing a near-complete census of the entire population (Hamilton et al., 2007). Abundance estimates have typically been produced by calculating the total cataloged population minus the cumulative number of individuals detected or presumed dead (Kraus et al., 2001). However, habitat use patterns have changed in recent years, meaning that capture probabilities have declined. Therefore, Pace et al. (2017) conducted a mark-recapture estimate of abundance of NARW, and showed the population increased at ∼2.8% from 270 whales in 1990 to 482 whales in 2010. Since then, however, there are strong indications that the population is declining, with abundance estimated to be 458 whales in 2015 (95% credible interval: 444-471, Pace et al., 2017).
Linking Reproductive Success and Health to Environmental and Anthropogenic Factors
Cataloging females through multiple calving events in SRW, and the ability to monitor almost all individual NARW, have provided powerful datasets from which to assess environmental and anthropogenic drivers of reproductive success and health. This, in turn, provides the ability to determine drivers of population growth and persistence. In particular, the low or negative growth rate of NARW stands in contrast to the higher growth rates of SRW (Table 1), and has been explicitly linked to changes in calving intervals between the two species (Corkeron et al., 2018).
Right whales typically have a 3-year reproductive cycle; 1 year gestation, 1 year calving and 1 year resting (Knowlton et al., 1994). Right whale calves grow extremely fast compared with other cetacean species, with SRW calves estimated to grow between 2.2 and 3.5 cm per day (Whitehead and Payne, 1978; Best and Rüther, 1992; Christiansen et al., 2018). During their typically fasting wintering period, lactating females are estimated to lose up to 3.4 cm of blubber thickness (Miller et al., 2011) or 25% of their body volume (Christiansen et al., 2018). NARW have significantly thinner blubber than SRW (Miller et al., 2011). Unsurprisingly these factors all demonstrate that female health and body condition are strong determinants of calving rates.
Since 1984, nearly all of the new calves in the NARW population have been observed due to aerial and ship surveys on calving grounds (Browning et al., 2010). Using this comprehensive dataset for years 1988–2008, Rolland et al. (2016) showed that females that transition from resting to pregnant had a significantly higher annual mean health score compared with those that did not calve (Rolland et al., 2016). This and other work shows that the greatest fluctuations in NARW body condition and health scores are linked to the cost of lactation in the calving cycle, with the lowest estimated health scores in resting and lactating females, and blubber thicknesses increasing with the years since the last calving event (Pettis et al., 2004; Miller et al., 2011, 2012; Rolland et al., 2016). Recovery of condition post calving may, however, be impeded by external factors and chronic entanglement in fishing gear can result in the consumption of endogenous lipid energy reserves on the same order of magnitude as is consumed during lactation (van der Hoop et al., 2016a).
As foraging success helps determine body condition, work in SRW has investigated the link between foraging ground conditions and reproductive success. Leaper et al. (2006) showed a relationship between breeding success at the Argentinean wintering ground and sea surface temperature (SST) anomalies at their feeding ground in South Georgia. The SST anomalies in late summer to autumn prior to winter conception seemed to have the largest impact, and the authors hypothesized it was due to relationship between SST and krill biomass (Trathan et al., 2003). Variation in krill abundance and density, linked with the phase of the Antarctic Oscillation and El Nino events, were also found to be significantly correlated with the number of calves observed on the Brazilian wintering ground (Seyboth et al., 2016).
A similar relationship has been found in the NARW, where calving success is strongly correlated with the abundance of its primary prey Calanus finmarchicus, which in turn is linked to the North Atlantic Oscillation (Greene and Pershing, 2004; Hlista et al., 2009; Meyer-Gutbrod and Greene, 2018). Recent analyses have sought to understand the relative contribution of anthropogenic impacts and food availability on NARW reproduction and population growth (e.g., Meyer-Gutbrod and Greene, 2018). This work offers an important example to understanding right whale population persistence and recovery more generally. It has become particularly relevant on a global scale as the strong population growth in some SRW’s appears to have slowed in recent years, with lower counts since 2015 (Charlton, 2017; Brandão et al., 2018). In Australia this appears to be due to a redistribution of calving females to historical calving areas such as Fowlers Bay (Charlton, 2017). But in South Africa this has been attributed to an increase in the calving interval, with models suggesting females are resting longer than in the past (Brandão et al., 2018). Furthermore, major die offs of calves at the Argentinean wintering ground may reduce population growth in the future, and the drivers of these events remain as yet unknown (Rowntree et al., 2013; Marón et al., 2015).
Synthesis
Long-term monitoring studies have linked environmental factors such as prey availability and its proxies, as well as anthropogenic impacts, with right whale health, body condition and reproductive success. The analyses conducted in NARW using over three decades of detailed sightings histories of individually identified whales showcases the way forward for explaining the impact of environmental variation and human impacts on reproduction and population persistence. As some SRW populations begin to experience a decline in reproductive success with population-level implications, we suggest adopting a similar strategy and in particular targeting efforts toward monitoring those that appear most vulnerable. Given that SRW travel between feeding grounds shared by more than one population, we also recommend developing a unified catalog strategy between regions and research groups.
Adapting to Shifting Resources
The distribution of right whale species reflects evolutionary, demographic, ecological, habitat and anthropogenic processes that come together over various scales of space and time (e.g., Forcada et al., 2012). Within each species, individuals move through different habitats that provide appropriate conditions for specific ecological and physiological needs, often governed by life-history stages or characteristics (e.g., Corkeron and Connor, 1999). All three right whale species show some migratory tendency, though the identification of calving, breeding and foraging grounds varies for each species. Moving between these grounds can require multi-month migrations over thousands of kilometers (Figure 1). For the most part these migratory pathways between grounds remain only partially defined and may indeed be very diffuse. Within grounds, individuals will move to fulfill their needs of foraging, socializing, courtship (Best et al., 1993; Kraus and Hatch, 2001) and calving (Patenaude and Baker, 2001; Elwen and Best, 2004; Torres et al., 2013; Rayment et al., 2015). As reported above, within a season, some (though emphatically not all) individuals may move between different habitats that support the same needs (e.g., movement between different foraging grounds), depending on proximity and suitability (Vanderlaan, 2010; Schick et al., 2013), and perhaps, experience or cultural knowledge.
Factors Driving Distribution
The single most important habitat feature for the NARW is the occurrence of concentrated patches of copepod prey (Murison and Gaskin, 1989; Wishner et al., 1995; Mayo et al., 2001; Baumgartner and Mate, 2003). Similarly, the probability of detecting NPRW in the Southeastern Bering Sea is strongly associated with the abundance of their primary prey, Calanus marshallae, with patch densities around right whales as high as 105 copepods m-3 (Baumgartner et al., 2013b). Copepod patches result from a combination of physical (supply, removal, and resuspension) and biological (diapause and density) mechanisms occurring across scales. Together, these factors can lead to predictable and persistent, high-energy food sources, or can cause high inter-annual variability in the abundance, concentration, and energy density in the same foraging habitat (Zakardjian, 2003; Jiang et al., 2007; Davies et al., 2013; Davies et al., 2014). The same features that tend to concentrate NARW prey (Calanus spp.) have not necessarily been shown to concentrate NPRW or SRW prey, nor have NPRW or SRW been associated with similar foraging-type environments (Baumgartner et al., 2013b). The latter do not appear to be obligative frontal foragers (Reid et al., 2000), although Mate et al. (2011) reported that some SRW tagged in South Africa were associated with the Subtropical Convergence. There is limited information on foraging behavior or prey in NPRW and SRW (Hamner et al., 1988), though current efforts are underway. For example, D’Agostino et al. (2018) describe seasonal phytoplankton and mesozooplankton dynamics along with environmental factors in the northern Patagonian gulfs in addition to mesozooplankton composition and abundance measured close to foraging SRW.
Calving areas share many features between SRW and NARW. Mother-calf pairs select shallow areas closer to shore (Winn et al., 1986; Elwen and Best, 2004; Keller et al., 2012; Rayment et al., 2012; Rayment et al., 2015), with calm waters (Winn et al., 1986; Rowntree et al., 2001), protection from ocean swell and seasonal winds (Elwen and Best, 2004), and soft substrates, when compared to unaccompanied or non-calving individuals. Historical and contemporary calving grounds for either NPRW stock remain a mystery.
Changes in distribution of different demographic classes have been linked with changes in density in multiple SRW populations. Increases in the number of cow-calf pairs displaces social groups and solitary individuals to other habitat in Argentina (Rowntree, 2001; Arias et al., 2018) New Zealand (Carroll et al., 2014; Torres et al., 2017), and Australia (Charlton, 2017).
Studies of social and cultural drivers remain in their infancy, currently restricted to SRW (Valenzuela et al., 2009; Carroll et al., 2015). Application to NARW may help characterize site fidelity and transmission of migratory patterns, e.g., Fundy vs. non-Fundy females in NARW (Schaeff et al., 1993; Malik et al., 1999), and the segregation of mature males to, e.g., Browns Bank and Roseway Basin prior to 1993 (Clapham et al., 1999).
Contemporary Distribution
SRW
The SRW are circumpolar in the Southern Hemisphere between about 12°S and 65°S, albeit with a large historical and contemporary discontinuation between New Zealand and Chile. The austral winter distribution of SRW (Figure 1) includes the New Zealand sub-Antarctic Auckland and Campbell Islands (Carroll et al., 2013; Torres et al., 2017), with far fewer whales reported around mainland New Zealand (Carroll et al., 2014). In Australian coastal waters, SRW occur along the southern coastline including Tasmania, generally as far north as Sydney on the east coast and Perth on the west coast (Carroll et al., 2011). Similarly, SRW migrate up the east coast of South America to Brazil to breed in coastal shallows (De Oliveira et al., 2009), and along the west coast through Chile into Peru as far as 12°S (and possibly 4°S) (Aguayo-Lobo et al., 2008; Van Waerebeek et al., 2009), and on both the east and west coasts of southern Africa (Best et al., 1993; Mate et al., 2011, Figure 1). When in coastal habitat, SRW are usually within 2 km of shore and distinctly clumped in aggregation areas (Figure 1). SRWs tend to group into different demographic class along the coast, with cow-calf pairs and single adults or socializing groups using different sections of the coast or region, e.g., cow-calf pairs concentrate in Port Ross, Auckland Islands, while Campbell Island seems to contain more single whales (Torres et al., 2017).
In summer SRW probably forage between about 40°S and 65°S, including Magellan Strait and along the Antarctic Peninsula (Aguayo-Lobo et al., 2008; Table 1). Foraging and summer distribution have been determined from multiple sightings (Table 1); stable isotope studies (e.g., Rowntree et al., 2001; Valenzuela et al., 2009) and a small number of satellite tracking studies (Mate et al., 2011, see section “Migratory Culture, Extirpation and Recovery”). Direct movement between wintering and feeding grounds is known from photo-identification with movement from southwest Australia to south of 60° (Bannister et al., 1999), and between Península Valdés and South Georgia (Best et al., 1993). Illegal Soviet whaling in the 1960s and 1970s supports these inferences, as SRWs were killed during the austral summer around South Georgia and other high latitude areas (Tormosov et al., 1998). Stomach content data from these whales showed that SRW were feeding on copepods north of 40°S and euphausiids south of 50°S and a mixture in between (Tormosov et al., 1998). The summer distribution of contemporary SRW is one of the least well understood aspects of the species’ biology.
NARW
In the western North Atlantic, individual right whales routinely range from Florida to the Gulf of St. Lawrence (Kraus and Rolland, 2007); however, they can be considered “condition-dependent partial migrators” (Krzystan et al., 2018). Recent sightings of “western” NARW have been reported from Iceland, Norway, Portugal and the Azores. The calving ground is in the coastal waters of the southeastern United States during the winter months, although there are at least two records of calves being born around Cape Cod in the spring. Non-calving females, and adult and juvenile males are also observed on the calving grounds (Schick et al., 2013). Winter aggregations also have been observed in the middle of the Gulf of Maine (Cole et al., 2013) and south of Cape Cod (Leiter et al., 2017), but the location of most of the non-calving whales is unknown. Historical whaling records suggest a winter-spring presence along the United States Mid-Atlantic coast (NY – DE; Reeves et al., 1999). In the spring, aggregations of right whales occur in Massachusetts Bay, and sometimes in the great South Channel east of Cape Cod. In the summer and fall, right whales are observed in the Bay of Fundy (between Maine and Nova Scotia), in Roseway Basin (50 km south of Nova Scotia), and recently in the southern Gulf of St. Lawrence. Photo-identification and some limited satellite tagging data show that seasonal movements into, out of, between, and around the so-called critical habitats are frequent and extensive (Baumgartner and Mate, 2005; Brillant et al., 2015). There is significant substructuring within the NARW population. Schaeff et al. (1993) inferred from genetic and photo-identification data that about one-third of the cows in the population do not bring their calves to the Bay of Fundy nursery area. This suggests that another summer and fall nursery area may exist, although its location is unknown.
NPRW
The seasonal distribution of NPRW is largely understood through analysis of historical catch records and sightings data (Miyashita and Kato, 1998; Clapham et al., 2004), along with more recent visual and acoustic survey effort since the early 2000s. Critical Habitat for the eastern NPRW has been established in the southeast Bering Sea and in a small portion of the Gulf of Alaska based on consistent sightings in these areas (NOAA, 2008). Aerial and shipboard surveys, satellite tracking and acoustic monitoring efforts are largely focused in the southeast Bering Sea and confirm it is an important summer foraging area (Wade et al., 2006, 2011a; Zerbini et al., 2015). There is considerably less survey effort in the northern Gulf of Alaska compared to the southeast Bering Sea, but individual sightings and acoustic detections (Waite et al., 2003; Mellinger et al., 2004; Allen and Angliss, 2012; Širović et al., 2015) including further south (Ford et al., 2016) suggest that alternative foraging grounds may exist. Eastern NPRW arrive in the southeast Bering Sea as early as May, move into mid-shelf waters throughout the summer, and are detected through December (Munger et al., 2008). Visual sightings and year-round acoustic detections of NPRW in Unimak Pass suggest this region may be used as a corridor between summer foraging and unknown winter grounds (Wright, 2015, 2016; Wright et al., 2017).
In fall and spring, NPRW sightings and detections are much more diffuse across the species’ range from South Korea, Japan, and the Okhotsk Sea through British Columbia and California (Brownell et al., 2001; Clapham et al., 2004; Ford et al., 2016), and acoustic detections are less common (Munger et al., 2008; Wright, 2015, 2016).
Few historical sightings suggest that in winter, eastern NPRW ranged from between the Hawaiian Islands to the west coast of North America (Klumov, 1962) and for western NPRW, from offshore Kamchatka south to the Yellow Sea (Omura et al., 1969; Scarff, 1986; Brownell et al., 2001). Contemporary sightings remain within these ranges, and acoustic detections indicate winter residence in Alaskan waters (Wright, 2015, 2016). Mitochondrial DNA comparisons suggest that the populations in the eastern and western North Pacific are genetically distinct (Pastene et al., 2018). Calving areas remain unknown, and have never been reported by whalers; modeling studies have identified coastal areas in California, Hawaii, southern China and northern Vietnam as potential calving regions of based on habitat features (Good and Johnston, 2010). There is a single photo-identification match between Hawaii and the Bering Sea (Kennedy et al., 2012).
Investigating the Distribution of Species and Movement of Individuals Within and Between Populations
Many methods have been implemented to study the distribution of species and movement of individuals within and between populations (Table 1). These methods provide insight at different spatial and temporal scales and rely on different types of observations or samples. For example, biopsy, necropsy or sloughed skin can provide material for genetic analysis to determine identity, sex, parentage and population membership and stable isotope analysis to ascertain foraging location and trophic level. Baleen can also provide material for stable isotope analysis, providing more detailed histories of individual foraging locations (Best and Schell, 1996; Rowntree, 2001; Valenzuela et al., 2009).
Acoustic detections provide the approximate location of a whale, but only when it is calling and within the detection range of the hydrophone, but then will provide evidence of presence of one or more whales (Mellinger et al., 2004, 2007, 2011; Wade et al., 2006; Van Parijs et al., 2009; Širović et al., 2015) and seasonal distribution in space and in time (Morano et al., 2012; Davis et al., 2017). Behavior is the primary determinant of right whale calling rates, and sound production varies between habitats (Matthews et al., 2001; Parks et al., 2011).
Photo identification of individuals has been used to demonstrate longer-range movements of all three right whale species (e.g., Knowlton et al., 1992; Best et al., 1993; Pirzl et al., 2009; Kennedy et al., 2012), as have genetic data in SRW (Carroll et al., 2014), but these paired observations essentially only provide information on the presence of the individual at both places at both times, usually lacking information on residence time, and how the animal moved from A to B (e.g., Whitehead, 2001). The different right whale catalogs contain individual sightings at scales from <1 day to 17 years (Hamilton et al., 2007). Eighty percent of sightings in the NARW catalog occur in consecutive years (Hamilton et al., 2007), compared to 3 years in SRW (Bannister, 2017; Charlton, 2017).
Various satellite telemetry systems have been developed for large whales and have been deployed on right whales since the mid 1980s (Mate et al., 1992, 1997; Goodyear, 1996; Andrews et al., 2008). The technology provides information at a higher temporal resolution compared to visual sightings and has been useful in confirming that right whales can travel long distances over short periods of time between subsequent sightings in the same habitat (e.g., Mate et al., 1992), as well as tracking movements between known summer and winter habitat areas (Mate et al., 1997, 2011; Childerhouse et al., 2010; Zerbini et al., 2016). All three right whale species have been satellite tagged (Table 1). Tag systems have evolved over decades (Mate et al., 2007; Andrews et al., 2008) improving attachment mechanisms and increasing deployment duration, which can now last >200 days (Zerbini et al., 2016). However, tag impacts must be evaluated in the context of the value of the information, and continued improvement of attachment mechanisms is necessary to address health and welfare concerns (Moore et al., 2012; Best et al., 2015; Moore and Zerbini, 2017; Norman et al., 2018).
Going From Sightings and Distributions to Individual Movements
The ability to identify individuals (see section “Catalogs” above) and follow the change in location of subsequent sightings allows for the transition from describing general population movement, habitat use, and arrival times down to the resolution of specific individuals. Tracking individual movements provides a greater understanding of behaviors (Mayo and Marx, 1990), decisions, and corridors (Schick et al., 2009; Zerbini et al., 2016), and allows for movements to be predicted across a range of scales (Pendleton et al., 2012; Brillant et al., 2015, 2017). For example, Schick et al. (2013) characterized transition probabilities between broad geographic regions (i.e., feeding and breeding habitats) that differed between male and female NARW. Do similar differences in movement exist based on health or entanglement status (van der Hoop et al., 2016a)? Predicting how and when individuals, especially of different age, sex or life stage, distribute throughout the species’ range has implications for marine spatial planning (Vanderlaan, 2010; van der Hoop et al., 2014). How individual movements scale to reflect the overall distribution of populations and species are open questions in movement ecology. For example, our understanding of SRW connectivity, and transition probabilities, would be highly informed by cross-catalog matching. Measuring large-scale and mesoscale movements with the approaches described above will help reveal individual residency and movement patterns and clarify how these scale to observed population distributions (Table 2).
Fine-Scale Movement and Orientation
Detailed measurements of how right whales orient and behave, especially in relation to prey patches and anthropogenic threats, e.g., fishing gear or near the surface, can be difficult to obtain. Detailed field observations (Mayo and Marx, 1990) have provided careful measurements of swimming paths and turning angles in traveling vs. foraging modes, and at a finer scale than offered by satellite telemetry. Similar measurements in other environments, populations or species are rare, yet these values are critical in informing modeling approaches for, e.g., spatio-temporal risk assessment (van der Hoop et al., 2012). We know that obstacle avoidance (Kraus et al., 2014), prey patch exploitation and decision making occur at these fine scales.
Bio-logging and bio-telemetry tags have been used to assess NARW diving behavior, specifically comparing different diving modes that may be associated with foraging, as well as the tracking of diel vertically migrating prey layers (Winn et al., 1995; Goodyear, 1996, Table 1; see section “Biologgers”). Simultaneous use of echosounders and tags can record the depth to which animals dive with respect to the vertical distribution of their prey (Baumgartner and Mate, 2003). Depth-related time budgets have been effective in assessing the risk of entanglement in fishing gear or vessel strike (Parks et al., 2012; Baumgartner et al., 2017) as well as assessing potential avenues to reduce those risks (Nowacek et al., 2004). Diving behavior and fine-scale body orientation have been linked to differences in total buoyancy and changes in body condition (Nowacek et al., 2001; Nousek-McGregor et al., 2013), while tags have also been used to resolve changes in diving behavior, biomechanics and thrust production with entanglement in fishing gear (van der Hoop et al., 2013b, 2017b; Table 1). The fine-scale 3D movement, orientation, and behavior of right whales in the context of variable prey densities, conspecifics, or anthropogenic disturbances or threats is poorly known, especially for SRW and NPRW. For SRW, this is linked to poorly described and typically offshore and somewhat inaccessible location of foraging grounds.
Detecting Changes: What Do We Need to Know?
“Any effective study of the ecology, behavior, or patterns of distribution of western North Atlantic right whales must include studies of C. finmarchicus.” – Winn et al. (1995).
Predicting how movements at any scale will change with environmental change requires a mechanistic understanding of the processes that drive movement and distribution. It also requires sufficient sightings or detections to quantify change; shifts in distribution are difficult to detect when annual sightings are incredibly low. For example, Tynan et al. (2001) reported changes in eastern NPRW distribution and prey selection with climate forcing; however, the prey species were no different than those observed in the historical record of NPRW (Shelden et al., 2005), and the observed distribution of whales was likely the result of inadequate survey coverage (Wade et al., 2006, 2011b; Zerbini et al., 2015). Sightings presence is a poor indicator when annual sightings are fewer than ten individuals.
Only recently have we begun to understand and quantify the plasticity of migration in NARW. In recent years, fewer individuals have been sighted on the calving grounds (Krzystan et al., 2018), whereas more individuals are overwintering in northern habitats, e.g., Cape Cod Bay and the Gulf of Maine (Bort et al., 2015; Davis et al., 2017; Mayo et al., 2018). Other causal factors have been identified and described across a range of scales. For example, NARW were almost completely absent from their Roseway Basin feeding habitat in the mid-to-late 1990s (Brownell et al., 2001; Kenney et al., 2001; Figure 3 in van der Hoop et al., 2012), whereas over 100 individuals have been sighted in that habitat in other years. This multi-year abandonment has been linked to interannual variation in copepod abundance (Patrician and Kenney, 2010), attributed to the volume, strength, and density of water masses transported to the basin (Davies et al., 2013, 2014). Studies emphasize the importance of prey mapping, modeling, and in situ and satellite-derived oceanographic conditions (Baumgartner et al., 2003; Jiang et al., 2007; Hlista et al., 2009; Krumhansl et al., 2018) at resolutions that match effort-corrected whale detections, as well as coordination between researchers in these disciplines.
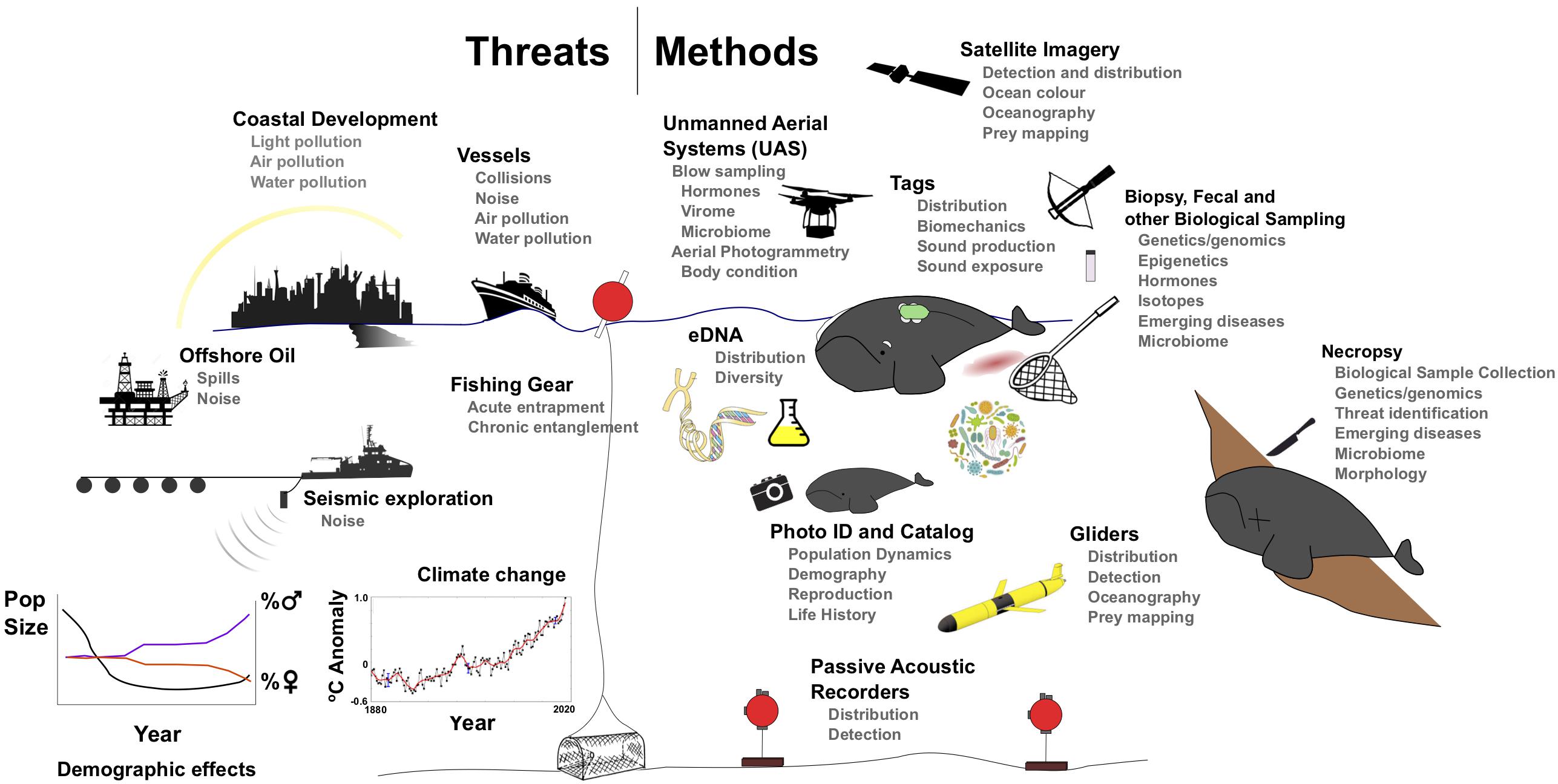
Figure 3. Current and future threats to (left) and methods to study (right) global right whale populations.
Large scale changes in the overall distribution of NARW from 2004 to 2014 have also been resolved via acoustic monitoring. Davis et al. (2017) compiled acoustic records from Florida, United States to Greenland, and documented an increased presence of NARW in the mid-Atlantic and simultaneous decrease in the Gulf of Maine; these changes coincided with seasonal sightings data for the regions. From acoustic data, it is difficult to assess how movements of individuals within the population have led to these measured changes, or the strength of various drivers; however, such studies emphasize how the combination of many smaller-scale acoustic projects or programs can provide a larger-scale assessment of seasonality and habitat use, and changes therein.
Small-scale vertical and horizontal distributions of diving right whales correspond to the vertical and horizontal distributions of their prey. Collocated measurements of diving behavior (see sections “Automated Image Analysis” and “Biologgers”) and oceanographic and prey-field measurements have been useful in characterizing inter- and intra-annual changes in foraging activity in NARW (Winn et al., 1995; Baumgartner et al., 2017), but little similar work has been carried out in SRW or NPRW.
Technological Advances
Application of new and developing methodologies will help answer questions regarding how right whales adapt to their changing environment. Here we discuss several of these.
Passive Acoustic Monitoring
The development and improvement of tools to collect, store, and analyze underwater acoustic recordings have expanded their scope and application. Archival and real-time systems can be low-cost solutions to monitor cetacean presence at the mesoscale (Van Parijs et al., 2009, Figure 3). Fixed, autonomous acoustic recorders can operate continuously for months to years, through poor weather and darkness, and have proven useful in detecting eastern NPRW in known and new habitats (Mellinger et al., 2004; Širović et al., 2015; Wright et al., 2018). This greatly enhances aerial and vessel surveys (Rone et al., 2012; Baumgartner, 2014), helps identify changes in overall distribution, and arrival to and departure from specific habitats (Van Parijs et al., 2009; Davis et al., 2017). The coordination of many smaller-scale acoustic programs into larger-scale networks (Van Parijs et al., 2015) and post hoc analyses can describe changes at large scales, despite technical challenges in unifying datasets with varying duty cycles or deployment durations (Davis et al., 2017). However, it remains a challenge to estimate the number of individuals (and which individuals) or animal density from acoustic detections that reflect only pseudo-presence at variable detection ranges (but see Marques et al., 2011).
The development and implementation of detection and classification algorithms has helped automate species identification and ease data burden (Gillespie and Caillat, 2008; Baumgartner and Mussoline, 2011) and can be used for real-time monitoring for risk management and mitigation. For example, the Right Whale Listening Network created following the proposed building of deep-water ports for liquefied natural gas offloading near right whale critical habitat is comprised of 10 right whale automatic detection buoys, provided near-real time notifications to LNG vessels (Wiley et al., 2013). Other similar real- or near-real-time transmission systems have been deployed (e.g., in the New York Bight), and communicate by, e.g., twitter (@robots4whales) and the Whale Alert App. Real-time moored systems are being considered for other high-density areas in the NARW range with high vessel density (e.g., the Gulf of St. Lawrence, Canada) and could be implemented in similar habitat for SRW such as west of Melbourne, Victoria, Australia near the only known SRW calving habitat for the at-risk remnant south eastern population.
Autonomous Platforms
Incorporation of these types of acoustic monitoring devices and detection and classification algorithms into autonomous ocean platforms (i.e., gliders Figure 3) has extended the duration, season, and track-line distance of sampling and surveying ability through poor weather (vs. shipboard surveys), while sampling simultaneous relevant in situ oceanographic information. Gliders have successfully detected fin, sei, humpback, and NARW in habitats ranging from the Gulf of Maine to the Gulf of St. Lawrence1 (Baumgartner et al., 2013a; Davis et al., 2016). Gliders have also been deployed in the Chukchi sea area, primarily to detect bowhead, beluga, and fin whales (Baumgartner et al., 2014b); however, this region is further north than the most frequented eastern NPRW habitats. Autonomous gliders could prove useful in surveying contemporary and historical right whale habitats around the world, especially in the context of oceanographic features.
Satellite Imagery
It is possible to detect and count individual animals in Very High Resolution (VHR) satellite imagery available either from open source platforms (e.g., Google Earth Imagery) or private companies (e.g., Digital globe; Cubaynes et al., 2018) (Figure 3). Fretwell et al. (2014) identified at least 55 SRW in a single image of the calving grounds of Golfo Nuevo, Península Valdéz, Argentina. This method can detect right whales where real-time sightings are available to validate detections, where weather conditions are favorable for cloud-free imagery, and where whales are frequently observed at or just below the surface. Satellite imagery suffers from availability biases in deeper waters, as dive times and surface detectability may differ across locations, seasons, activity patterns and demographic groups. This method might also prove useful for detecting beach-cast mortalities in remote environments.
Environmental DNA (eDNA)
Ecological questions are now being addressed using environmental sampling of water in combination with high through-put sequencing. Work in marine mammals shows that the method can be used to identify species (Foote et al., 2012) and even genetically distinct killer whale ecotypes up to 2 h after the species was present in an area (Baker et al., 2018). Population-level data are also able to be obtained from aggregations of marine animals, as shown by work in whale sharks (Sigsgaard et al., 2016), which could be useful in the context of right whale wintering ground aggregations. In the future, eDNA could be used to detect the presence of right whales, in combination with other systematic survey methods, or in regions where other survey types (e.g., aerial) may be difficult due to logistical considerations (Figure 3).
Unmanned Aerial Systems
Aerial photographs from manned aircraft have long been used to identify and measure NARW, SRW, and eastern NPRW (Best and Rüther, 1992; Miller et al., 2011; Clapham et al., 2012). The recent development of small unmanned aerial systems (UAS) with lightweight, high-resolution cameras, provides improved image resolution at lower cost and risk compared to manned flight (Figure 3; Durban et al., 2015; Koski et al., 2015). UAS photogrammetry and sampling has recently been applied to SRW (Apprill et al., 2017; Christiansen et al., 2018) and NARW (Moore et al., 2017) to address a variety of research questions. One direct benefit of UAS photography is obtaining high-resolution aerial images that can be used to identify individuals. UAS imagery represents a small but growing proportion of the NARW and SRW catalogs. This could be expanded in future if the technology is adapted to more challenging weather conditions seen in high latitudes.
Automated Image Analysis
Automated and semi-automated computer image analysis methods have been increasingly used to detect whales in images, and match whales to a catalog of known individuals (Hillman et al., 2008; Kniest et al., 2010) including SRW (Hiby and Lovell, 2001) and NARW (Bogucki et al., 2018). Bogucki et al. (2018) identified individual NARW with 87% accuracy using a series of convolutional neural networks in a fully automated pipeline with no need for user input. These computer vision identification methods may increase the efficiency of matching, and enable the processing of larger volumes of data, especially from different sources (still images and video taken from aircraft, UAS, and land and shipboard surveys). These methods could be especially helpful in coordinating catalogs from different populations and right whale species to assess connectivity, ranging and movement.
Biologgers
Instrumenting animals with high-resolution inertial sensors (e.g., accelerometers, magnetometers, and gyroscopes; Figure 3) has provided detailed information on their fine-scale movements and biomechanics in foraging patches. Time-depth recorders have been deployed on NARW (Winn et al., 1995; Baumgartner and Mate, 2003; Baumgartner et al., 2017) and SRW (Argüelles et al., 2016), while tags with additional sensors (acoustic and/or inertial, e.g., DTAGs; Johnson and Tyack, 2003) have been deployed on NARW (Nowacek et al., 2001, 2004; Parks et al., 2011; Nousek-McGregor et al., 2013; van der Hoop et al., 2017b, Table 1). Efforts to bridge the gap toward high-resolution, medium-duration tags with minimally invasive attachment are underway (Baumgartner et al., 2014a; Johnson et al., 2014; Mate et al., 2017).
Synthesis
Despite our best efforts to sight, photograph, record, sample, and track individual right whales, and to synthesize these observations into general consensus on the species’ ecology and distributions, individuals will continue to surprise us. NARW have given birth in Cape Cod, a generalized springtime foraging ground (Patrician et al., 2009), and NARW and SRW show out-of-season foraging (Mayo and Marx, 1990; Best and Schell, 1996; Kenney et al., 2001; Hoffmeyer et al., 2010). All species and populations show rare long-distance movements, and acoustic detections indicate that our understanding of all species’ seasonal distributions and contemporary ranges is always changing. This highlights the continued need to survey, identify, and acoustically monitor right whale habitats year-round, and in all weather conditions, expanding survey efforts beyond current methods (Table 2).
Quantifying changes in movement patterns at any temporal or spatial scale requires data at sufficient resolution, a mechanistic understanding, and long-term measures of potential drivers. Whaling records and sightings provide a reasonable foundation for many basic questions on the populations and their movements. Combing these sightings with individual identification (by genetics or visible marking), isotopes, acoustics, and simultaneous oceanographic measurements or models will increase our understanding of where animals are, why they are there, and where they might go next. Synthesizing data from across different populations is particularly important in this context. There may be local (wintering or feeding ground) or global (climate change) drivers that can be inferred from such a comparative approach, particularly in SRW where there are multiple wintering grounds with long-term monitoring projects (Table 2).
Methods for Assessing Factors Affecting Right Whale Health
Marine mammals are vulnerable to a variety of intrinsic stressors, including infectious diseases, harmful algal blooms, prey shortages, and climate change, particularly when subjected to extrinsic stressors such as fishing gear entanglements, pollution, and acoustic disturbance (Figure 3). Both independently and in synergy these stressors may have an effect on infectious disease transmission dynamics (Gulland and Hall, 2007), and they are also likely to affect reproduction and the potential for mortality. Although stress is beneficial in the short-term to respond to and recover from a stimulus or perceived threat, remaining in a stressed state in the long-term is maladaptive. Maintaining this unbalanced ‘allostatic state’ can impair future stress response and fecundity and result in immune and other physiological dysfunction (McEwen and Wingfield, 2003; McEwen and Wingfield, 2010; Houser et al., 2016).
In aquatic mammals, contemporary assessments of marine mammal health are almost invariably biased toward animals whose health is already compromised, such as stranded animals (Gulland and Hall, 2007; Hunt et al., 2015), which in part reflects the difficulties in sampling aquatic environments. Yet identification of chronic changes requires monitoring of free-living animals. There are several tools available and more are emerging (Figure 3).
Dolphins and seals are vulnerable to viral infection, with serious pandemics of influenza virus (Cox and Subbarao, 2000; Groth et al., 2014), poxvirus (Fiorito et al., 2015) and morbillivirus (Sierra et al., 2014, 2016). Although no pandemics have been reported for large whales, as right whales undertake large scale migrations they could be the source of, and vectors for, potentially serious zoonoses (Bogomolni et al., 2008). Their inshore movements into urbanized coastal waters further increase their vulnerability. As Gulland and Hall (2007) point out, morbidity and mortality in marine mammals have resulted from terrestrial pathogens spreading to the ocean, from harmful algal blooms to epidemics of virulent viruses and bacteria (Geraci et al., 1999; Miller et al., 2002). Brucellosis, a pathogen that affects reproduction in mammals, has been reported in whales (Van Bressem et al., 2009), with potential for widespread population consequences. SRW adults and calves in Argentinian waters that suffer injuries from kelp gull attacks (Rowntree et al., 1998; Sironi et al., 2009), are exposed to pathogens such as Klebsiella, Salmonella, and Shigella confirmed in kelp gull feces (Fazio et al., 2012). Necropsies with pathology studies and metagenomic surveys of viral and bacterial diversity are therefore important for developing a greater understanding of the impact of oceanic pathogens (Geoghegan et al., 2018) on right whales’ population biology.
In NARW, there has been a long-standing visual health assessment program that uses individual identification photographs to monitor individual whale health (Pettis et al., 2004). This tool has been applied to predictions of outcomes for animals that are entangled (Pettis et al., 2017), and has been used to model the health of the population and individual demographic groups (Rolland et al., 2016). These methods could easily be applied to other right whale populations where photo-identification catalogs are maintained (Figure 3).
Another approach is the use of various matrices to assess reproductive and stress hormones in feces of individual right whales (Hunt et al., 2015). Hormonal profiles can reveal reproductive status (Rolland et al., 2005; Corkeron et al., 2017), increases in stress due to chronic entanglement (Rolland et al., 2017) and evidence of chronic stress due to shipping noise (NRC, 2005; Hunt et al., 2006; Rolland et al., 2012; Hunt et al., 2015). The findings of this program highlight the need to monitor free-ranging animals. Fecal collection is largely opportunistic and not viable for animals that are only available during fasting periods (e.g., most SRW). A potentially more accessible and repeatable method is to use whale respiratory vapor to assess hormone levels, disease organisms, and microbiome information. Burgess et al. (2018) demonstrated the feasibility of using pole-mounted collection of whale blow for hormone analyses with the caveat that sample concentrations must be normalized as an unknown amount of salt water is mixed with every exhalation (Burgess et al., 2018). UAS collection provides the opportunity to sample while keeping the boat further away from individual whales, Pirotta et al. (2017) used a drone to collect an extraordinary diversity and abundance of microbiota from the blow in migrating populations of humpback whales, clearly and unambiguously distinguishing microbial communities from surrounding control seawater and air samples (Pirotta et al., 2017). Drones such as these can sample whale blow and provide an assessment of respiratory bacteria, lipids, proteins, and even viruses (Apprill et al., 2017; Pirotta et al., 2017; Geoghegan et al., 2018). As with all new techniques, further refinement is needed to determine how consistently representative bacterial and viral samples may be for the animal, and therefore its health. Nevertheless, this area shows great promise and is evolving rapidly.
During whaling, body condition measurements included measures of girth and blubber thickness, with fine-scale measurements of percent lipid, protein, and ash contents in different parts of the body (Lockyer et al., 1985). More recently approximations to body condition have used indirect estimates of buoyancy (e.g., with bio-logging tags; Nowacek et al., 2001; Nousek-McGregor et al., 2013), direct measurements of blubber thickness with pole-mounted ultrasound (Miller et al., 2011) or body shape changes from photogrammetry, from aerial photographs (Best and Rüther, 1992; Miller et al., 2012) including from drones (Moore et al., 2017; Christiansen et al., 2018).
Harmful algal blooms and marine pollutants remain a concern. In NARW whales feeding close inshore are repeatedly exposed to multiple environmental neurotoxins produced by marine algae (Durbin et al., 2002; Doucette et al., 2012). Less well understood across the three species is the impact of marine pollutants: NARW are exposed to low but chronic levels. In this context, understanding the level of genomic diversity in populations and susceptibility of individuals to pathogens, harmful algal blooms and toxins will also help understand individual and population level impacts (Leroy et al., 2017).
Finally, in the case of animals that have been entangled, the increased drag, altered behavior, and additional energy demand, leads to decreases in body condition (Cassoff et al., 2011; Moore et al., 2013; van der Hoop et al., 2013b; Barratclough et al., 2014; van der Hoop et al., 2016b), elevated stress hormones (Rolland et al., 2017), poor health condition (Rolland et al., 2016; Pettis et al., 2017) and reduced reproductive success (Knowlton et al., 2012b). Future research along this cascade includes clarifying the interactions between stress, energetic demand, nutrition, and metabolism, and ultimately the population consequences of those stressors (Figure 3).
Comparative Synthesis and Cumulative Effects
The lack of a cumulative effects analytical approach impedes any understanding of multi-stressor conditions affecting marine mammals, especially those in more industrialized sections of ocean (Figure 3). It also hampers effective management, as it is impossible to know that managing stressor A is a better approach than managing stressor B, if stressor B is exacerbated by interactions with stressors C, D, and E. “If cumulative effects cannot be accounted for, then unexpected adverse impacts from interactions between stressors pose a risk to marine mammal populations and the marine ecosystems on which people and marine mammals depend” (National Academies of Sciences, and Engineering and Medicine, 2017). Without such a framework every stressor is managed in isolation, wasting resources, and reducing efficacy. There are many marine mammal examples. In harbor porpoise, Hall et al. (2006) showed that previous exposure to PCBs increased the risk of death from infectious diseases. In killer whales, Ford et al. (2010) suggest high POP concentrations may have acted synergistically with limited food resources, increasing mortality during times of low prey abundance. In baleen whales, the poster child for the cumulative effect problem is the NARW. As discussed earlier, the NARW is subject to multiple anthropogenic stressors that are well studied, and multiple environmental stressors that are known, although the effects are not fully understood (Figure 3).
Early work in this area was the development of a conceptual model of the Population Consequences of Acoustic Disturbance (PCAD), with subsequent models discussed by New et al. (2014); Fleishman et al. (2016), and Pirotta E. et al. (2018). Further developments in the field resulted in the National Academies of Sciences, and Engineering and Medicine (2017) report, and another conceptual model called Population Consequences of Multiple Stressors (PcoMS: National Academies of Sciences, and Engineering and Medicine, 2017). Both of these models are dependent upon a series of transfer functions that describe how exposure to stressors affects individual behavior or health, which is defined as all factors that comprise “fitness” or homeostasis. Variations in health can affect individual vital rates, and the variation in severity or level of exposure to a given stressor can be used to scale up to predictions of population level effects. National Academies of Sciences, and Engineering and Medicine (2017) provided a decision tree for thinking about cumulative effects analyses, and also recommended stepwise, hypothesis driven, adaptive management strategies to deal with the uncertainties inherent in most marine mammal/stressor interactions. The NAS Committee concluded that current scientific knowledge is not up to the task of predicting cumulative effects of different combinations of stressors on marine mammal populations.
Nevertheless, there are many things that we can do to enhance future PcoMS or other cumulative effects models. A comparative synthesis of biological parameters, known ecological parameters, and know anthropogenic threats, using data from many well-studied right whale populations, can inform ongoing and future cumulative effect analyses on this taxon and we outline research priorities to move toward this goal as we see them (Table 2).
The findings of such cumulative impact models, and its components, could be integrated into international legislation to ensure the continued survival of the right whales. Today, the hunting of whales is controlled by the International Whaling Commission (IWC), and the trade in whale products is governed by the Convention on the International Trade of Endangered Species of wild fauna and flora (CITES). In addition, the Convention on the Conservation of Migratory Species of Wild Animals (CMS), which operates under the auspices of the United Nations Environmental Programme, provides a cross-border mechanism for protecting right whales throughout their migratory networks. All three species are listed under Appendix I of the CMS convention, and could be subject to a special agreement under the treaty. Such agreements should promote co-ordinated conservation and management plans across the range of species, in addition to promoting conservation and restoration plans for important habitat areas2. This would link together national level legislation to provide a web of protection for the species around the world.
Conclusion
The name right whale was not a misnomer. The three right whale species were easy to exploit and all driven very close to extinction. Only a few populations of the SRW can be considered to have recovered well and the status of the eastern NPRW and NARW along with some populations of the SRW remain precarious. In this review we have outlined the manner in which the right whales were exploited and how we have monitored their recovery. The fragile status and in particular the recent reversal in recovery trajectory by NARW call for urgent action. Yet the close phylogenetic relationship and ecological equivalence between the three species may provide fruitful avenues for avoiding catastrophe. Insights from careful examination of the populations that have recovered, combined with approaches that draw on novel methods developed separately for different populations may provide guiding principles for future conservation research, to hopefully make these the right whales to survive.
Author Contributions
All authors listed have made a substantial, direct and intellectual contribution to the work, and approved it for publication.
Conflict of Interest Statement
The authors declare that the research was conducted in the absence of any commercial or financial relationships that could be construed as a potential conflict of interest.
Footnotes
References
Aguayo-Lobo, A., Acevedo, J., Brito, J. L., Olavarría, C., Moraga, R., and Olave, C. (2008). La ballena franca del sur, Eubalaena australis (Desmoulins, 1822) en aguas chilenas: análisis de sus registros desde 1976 a 2008. Rev. Biol. Mar. Oceanogr. 43, 653–668. doi: 10.4067/S0718-19572008000300024
Allee, W. C. (1931). Animal Aggregations A Study in General Sociology. Chicago, IL: University of Chicago press. doi: 10.5962/bhl.title.7313
Allen, B. M., and Angliss, R. P. (2012). Alaska Marine Mammal Stock Assessments NPRW NOAA-TM-AFSC-245. Washington, DC: NOAA.
Allendorf, F. W. (1986). Genetic drift and the loss of alleles versus heterozygosity. Zoo Biol. 5, 181–190. doi: 10.1002/zoo.1430050212
Andrews, R., Pitman, R., and Ballance, L. (2008). Satellite tracking reveals distinct movement patterns for type b and type c killer whales in the southern Ross Sea, Antarctica. Polar Biol. 31, 1461–1468. doi: 10.1007/s00300-008-0487-z
Apprill, A., Miller, C. A., Moore, M. J., Durban, J. W., Fearnbach, H., and Barrett-Lennard, L. G. (2017). Extensive core microbiome in drone-captured whale blow supports a framework for health monitoring. mSystems 2:00119-17. doi: 10.1128/mSystems.00119-17
Argüelles, M. B., Fazio, A., Fiorito, C., Pérez-Martínez, D., Coscarella, M., and Bertellotti, M. (2016). Diving behavior of Southern right whales (Eubalaena australis) in a maritime traffic area in Patagonia, Argentina. Aquat. Mamm. 42, 104–108. doi: 10.1578/AM.42.1.2016.104
Arias, M., Coscarella, M., Romero, M. A., Sueyro, N., Svendsen, G. M., Crespo, E. A., et al. (2018). “Southern right whale Eubalaena australis recolonizes golfo san Matías (Patagonia, Argentina),” in Proceedings of Annual Meeting of the International Whaling Commission SC, Patagonia, 24.
Baker, C. S., and Clapham, P. J. (2004). Modelling the past and future of whales and whaling. Trends Ecol. Evol. 19, 365–371. doi: 10.1016/j.tree.2004.05.005
Baker, C. S., Patenaude, N. J., Bannister, J. L., Robins, J., and Kato, H. (1999). Distribution and diversity of mtDNA lineages among southern right whales (Eubalaena australis) from Australia and New Zealand. Mar. Biol. 134, 1–7. doi: 10.1007/s002270050519
Baker, C. S., Steel, D., Nieukirk, S., and Klinck, H. (2018). Environmental DNA (eDNA) From the wake of the whales: droplet digital PCR for detection and species identification. Front. Mar. Sci. 5:133. doi: 10.3389/fmars.2018.00133
Bannister, J. (2017). Project A7 - Monitoring Population Dynamics of ‘Western’ Right Whales off Southern Australia 2015–2018 Progress Report on activities for 2017. Muscatine, IA: Hon Associate.
Bannister, J. L., Pastene, A., and Burnell, S. R. (1999). First record of movement of a southern right whale (Eubalaena australis) between warm water breeding grounds and the Antarctic Ocean, south of 60° S. Mar. Mamm. Sci. 15, 1337–1342. doi: 10.1111/j.1748-7692.1999.tb00895.x
Barendse, J., and Best, P. B. (2014). Shore-based observations of seasonality, movements, and group behavior of southern right whales in a nonnursery area on the South African west coast. Mar. Mamm. Sci. 30, 1358–1382. doi: 10.1111/mms.12116
Barratclough, A., Jepson, P. D., Hamilton, P. K., Miller, C. A., Wilson, K., and Moore, M. J. (2014). How much does a swimming, underweight, entangled right whale (Eubalaena glacialis) weigh? Calculating the weight at sea, to facilitate accurate dosing of sedatives to enable disentanglement. Mar. Mamm. Sci. 30, 1589–1599. doi: 10.1111/mms.12132
Baumgartner, M., and Mussoline, S. E. (2011). A generalized baleen whale call detection and classification system. J. Acoust. Soc. Am. 129, 2889–2902. doi: 10.1121/1.3562166
Baumgartner, M. F. (2014). Real-time passive acoustic monitoring of baleen whales from autonomous platforms. J. Acoust. Soc. Am. 136, 2116–2117. doi: 10.1121/1.4816406
Baumgartner, M. F., Cole, T. V. N., Clapham, P. J., and Mate, B. R. (2003). North Atlantic right whale habitat in the lower Bay of Fundy and on the SW Scotian Shelf during 1999-2001. Mar. Ecol. Prog. Ser. 264, 137–154. doi: 10.3354/meps264137
Baumgartner, M. F., Fratantoni, D. M., Hurst, T. P., Brown, M. W., Cole, T. V., Van Parijs, S. M., et al. (2013a). Real-time reporting of baleen whale passive acoustic detections from ocean gliders. J. Acoust. Soc. Am. 134, 1814–1823. doi: 10.1121/1.4816406
Baumgartner, M. F., Lysiak, N. S. J., Carter Esch, H., Zerbini, A. N., Berchok, C. L., and Clapham, P. J. (2013b). Associations between North Pacific right whales and their zooplanktonic prey in the southeastern Bering Sea. Mar. Ecol. Prog. Ser. 490, 267–284. doi: 10.3354/meps10457
Baumgartner, M. F., Hammar, T., Robbins, J., and Kurle, C. (2014a). Development and assessment of a new dermal attachment for short-term tagging studies of baleen whales. Methods Ecol. Evol. 6, 289–297. doi: 10.1111/2041-210X.12325
Baumgartner, M. F., Stafford, K. M., Winsor, P., Statscewich, H., and Fratantoni, D. M. (2014b). Glider-based passive acoustic monitoring in the Arctic. Mar. Technol. Soc. J. 48, 40–51. doi: 10.4031/MTSJ.48.5.2
Baumgartner, M. F., and Mate, B. R. (2003). Summertime foraging ecology of North Atlantic right whales. Mar. Ecol. Progress Ser. 264, 123–135. doi: 10.3354/meps264123
Baumgartner, M. F., and Mate, B. R. (2005). Summer and fall habitat of North Atlantic right whales (Eubalaena glacialis) inferred from satellite telemetry. Can. J. Fish. Aquat. Sci. 62, 527–543. doi: 10.1139/f04-238
Baumgartner, M. F., Wenzel, F. W., Lysiak, N. S. J., and Patrician, M. R. (2017). North Atlantic right whale foraging ecology and its role in human-caused mortality. Mar. Ecol. Prog. Ser. 581, 165–181. doi: 10.3354/meps12315
Best, P., and Rüther, H. (1992). Aerial photogrammetry of southern right whales Eubalaena australis. J. Zool. 228, 595–614. doi: 10.1111/j.1469-7998.1992.tb04458.x
Best, P., and Schell, D. M. (1996). Stable isotopes in southern right whale (Eubalaena australis) baleen as indicators of seasonal movements, feeding and growth. Mar. Biol. 124, 483–494. doi: 10.1007/BF00351030
Best, P. B. (1990). Natural markings and their use in determining calving intervals in right whales off South Africa. S. Afr. J. Zool. 25, 114–123. doi: 10.1080/02541858.1990.11448199
Best, P. B., Mate, B., and Lagerquist, B. (2015). Tag retention, wound healing, and subsequent reproductive history of southern right whales following satellite-tagging. Mar. Mamm. Sci. 31, 520–539. doi: 10.1111/mms.12168
Best, P. B., Payne, R., Rowntree, V., Palazzo, J. T., and Both, M. D. C. (1993). Long-range movements of South Atlantic right whales Eubalaena australis. Mar. Mamm. Sci. 9, 227–234. doi: 10.1111/j.1748-7692.1993.tb00451.x
Bogomolni, A. L., Gast, R. J., Ellis, J. C., Dennett, M., Pugliares, K. R., Lentell, B. J., et al. (2008). Victims or vectors: a survey of marine vertebrate zoonoses from coastal waters of the Northwest Atlantic. Dis. Aquat. Org. 81:13. doi: 10.3354/dao01936
Bogucki, R., Cygan, M., Khan, C. B., Klimek, M., Milczek, J. K., and Mucha, M. (2018). Applying deep learning to right whale photo identification. Conserv. Biol. doi: 10.1111/cobi.13226 [Epub ahead of print].
Bort, J., Van Parijs, S. M., Stevick, P. T., Summers, E., and Todd, S. (2015). North Atlantic right whale Eubalaena glacialis vocalization patterns in the central Gulf of Maine from October 2009 through October 2010. Endanger. Species Res. 26, 271–280. doi: 10.3354/esr00650
Braham, H. W., and Rice, D. W. (1984). The right whale, Balaena glacialis. Mar. Fish. Rev. 46, 38–47.
Brandão, A., Vermeulen, E., Ross-Gillespie, A., Findlay, K., and Butterworth, D. S. (2018). Updated application of a Photo-Identification Based Assessment Model to Southern Right Whales in South African Waters, Focussing on Inferences to be Drawn from a Series of Appreciably Lower Counts of Calving Females Over 2015 to 2017. Ulsan: International Whaling Commission.
Brillant, S. W., Vanderlaan, A. S. M., Rangeley, R. W., and Taggart, C. T. (2015). Quantitative estimates of the movement and distribution of North Atlantic right whales along the northeast coast of North America. Endanger. Species Res. 27, 141–154. doi: 10.3354/esr00651
Brillant, S. W., Wimmer, T., Rangeley, R. W., and Taggart, C. T. (2017). A timely opportunity to protect North Atlantic right whales in Canada. Mar. Policy 81, 160–166. doi: 10.1016/j.marpol.2017.03.030
Brownell, R. J., Clapham, P. J., Miyashita, T., and Kasuya, T. (2001). Conservation status of North Pacific right whales. J. Cetacean Res. Manag. 2, 269–286.
Brownell, R. J., and Mallette, S. D. (2018). Global Baleen Whale Bycatch: The Most Threatened Population. Ulsan: International Whaling Commission, 12.
Browning, C. L., Rolland, R. M., and Kraus, S. D. (2010). Estimated calf and perinatal mortality in western North Atlantic right whales (Eubalaena glacialis). Mar. Mamm. Sci. 26, 648–662.
Burdin, A., Nikulin, V., and Brownell, R. Jr. (2004). Cases of Entanglement of Western North-Pacific Right Whales (Eubalaena japonica) in fishing gear: serious threat for species survival Marine mammals of the Holarctic. Moscow: KMK Scientific Press, 95–97.
Burgess, E. A., Hunt, K. E., Kraus, S. D., and Rolland, R. M. (2018). Quantifying hormones in exhaled breath for physiological assessment of large whales at sea. Sci. Rep. 8:10031. doi: 10.1038/s41598-018-28200-8
Carroll, E. L., Alderman, R., Bannister, J., Bérubé, M., Best, P., Boren, L., et al. (2018). Incorporating non-equilibrium dynamics into demographic history inferences of a migratory marine species. Heredity 122, 53–68. doi: 10.1038/s41437-018-0077-y
Carroll, E. L., Baker, C. S., Watson, M., Alderman, R., Bannister, J., Gaggiotti, O. E., et al. (2015). Cultural traditions across a migratory network shape the genetic structure of southern right whales around Australia and New Zealand. Sci. Rep. 5:16182. doi: 10.1038/srep16182
Carroll, E. L., Childerhouse, S., Fewster, R., Patenaude, N., Steel, D., Dunshea, G., et al. (2013). Accounting for female reproductive cycles in a superpopulation capture–recapture framework. Ecol. Appl. 23, 1677–1690. doi: 10.1890/12-1657.1
Carroll, E. L., Childerhouse, S. J., Christie, M., Lavery, S., Patenaude, N., Alexander, A., et al. (2012). Paternity assignment and demographic closure in the New Zealand southern right whale. Mol. Ecol. 21, 3960–3973. doi: 10.1111/j.1365-294X.2012.05676.x
Carroll, E. L., Fewster, R., Childerhouse, S., Patenaude, N., Boren, L., and Baker, C. S. (2016). First direct evidence for natal wintering ground philopatry and estimate of juvenile survival in the New Zealand Southern right whale Eubalaena australis. PLoS One 11:e0146590. doi: 10.1371/journal.pone.0146590
Carroll, E. L., Patenaude, N., Alexander, A., Steel, D., Harcourt, R., Childerhouse, S., et al. (2011). Population structure and individual movement of southern right whales around New Zealand and Australia. Mar. Ecol. Prog. Ser. 432, 257–268. doi: 10.3354/meps09145
Carroll, E. L., Rayment, W. J., Alexander, A. M., Baker, C. S., Patenaude, N. J., Steel, D., et al. (2014). Reestablishment of former wintering grounds by New Zealand southern right whales. Mar. Mamm. Sci. 30, 206–220. doi: 10.1111/mms.12031
Cassoff, R. M., Moore, K. M., McLellan, W. A., Barco, S. G., Rotstein, D. S., and Moore, M. J. (2011). Lethal entanglement in baleen whales. Dis. Aquat. Organ. 96, 175–185. doi: 10.3354/dao02385
Charlton, C. M. (2017). Southern Right Whale (Eubalaena australis) Population Demographics in Southern Australia. Ph.D. thesis, Curtin University, Bentley.
Childerhouse, S., Double, M. C., and Gales, N. J. (2010). Satellite Tracking of Southern Right Whales (Eubalaena australis) at the Auckland Islands, New Zealand. Washington, DC: International Whaling Commission, 5.
Christiansen, F., Vivier, F., Charlton, C., Ward, R., Amerson, A., Burnell, S., et al. (2018). Maternal body size and condition determine calf growth rates in southern right whales. Mar. Ecol. Prog. Ser. 592, 267–281. doi: 10.3354/meps12522
Clapham, P. J., Aguilar, A., and Hatch, L. T. (2008). Determining spatial and temporal scales for management: lessons from whaling. Mar. Mamm. Sci. 24, 183–201. doi: 10.1111/j.1748-7692.2007.00175.x
Clapham, P. J., Good, C., Quinn, S. E., Reeves, R. R., Scarff, J. E., and Brownell, R. L. Jr. (2004). Distribution of North Pacific right whales (Eubalaena japonica) as shown by 19th and 20th century whaling catch and sighting records. J. Cetacean Res. Manag. 6, 1–6.
Clapham, P. J., Kennedy, A. S., Rone, B. K., Zerbini, A. N., Crance, J. L., and Berchok, C. L. (2012). North Pacific right whales in the southeastern Bering Sea. Final Rep. Bureau Ocean Energy Manag. 144.
Clapham, P. J., Young, S., and Brownell, R. J. (1999). Baleen whales: conservation issues and the status of the most endangered populations. Mamm. Rev. 29, 35–60. doi: 10.1046/j.1365-2907.1999.00035.x
Cole, T. V. N., Hamilton, P., Henry, A. G., Duley, P., Pace, R. M., White, B. N., et al. (2013). Evidence of a North Atlantic right whale Eubalaena glacialis mating ground. Endanger. Species Res. 21, 55–64. doi: 10.3354/esr00507
Cooke, J., Rowntree, V., and Sironi, M. (2015). Southwest Atlantic Right Whales: Interim Updated Population Assessment from Photo-Id Collected at Península Valdéz, Argentina, Washington, DC: International Whaling Commission.
Corkeron, P., Hamilton, P., Bannister, J., Best, P., Charlton, C., Groch, K. R., et al. (2018). The recovery of North Atlantic right whales, Eubalaena glacialis, has been constrained by human-caused mortality. R. Soc. Open Sci. 5:180892. doi: 10.1098/rsos.180892
Corkeron, P., Rolland, R. M., Hunt, K. E., and Kraus, S. D. (2017). A right whale pootree: classification trees of faecal hormones identify reproductive states in North Atlantic right whales (Eubalaena glacialis). Conserv. Physiol. 5:cox006. doi: 10.1093/conphys/cox006
Corkeron, P. J., and Connor, R. C. (1999). Why do baleen whales migrate? Mar. Mamm. Sci. 15, 1228–1245. doi: 10.1111/j.1748-7692.1999.tb00887.x
Courchamp, F., Clutton-Brock, T., and Grenfell, B. (1999). Inverse density dependence and the Allee effect. Trends Ecol. Evol. 14, 405–410. doi: 10.1016/S0169-5347(99)01683-3
Cox, N., and Subbarao, K. (2000). Global epidemiology of influenza: past and present. Annu. Rev. Med. 51, 407–421. doi: 10.1146/annurev.med.51.1.407
Crespo, E. A., Pedraza, S. N., Dans, S. L., Svendsen, G. M., Degrati, M., and Coscarella, M. A. (2018). The southwestern Atlantic southern right whale, Eubalaena australis, population is growing but at a decelerated rate. Mar. Mamm. Sci. 35, 93–107. doi: 10.1111/mms.12526
Cubaynes, H. C., Fretwell, P. T., Bamford, C., Gerrish, L., and Jackson, J. A. (2018). Whales from space: four mysticete species described using new VHR satellite imagery. Mar. Mamm. Sci. (in press). doi: 10.1111/mms.12544
D’Agostino, V. C., Degrati, M., Santinelli, N., Sastre, V., Dans, S. L., and Hoffmeyer, M. S. (2018). The seasonal dynamics of plankton communities relative to the foraging of the southern right whale (Eubalaena australis) in northern Patagonian gulfs, Península Valdés, Argentina. Cont. Shelf Res. 164, 45–57. doi: 10.1016/j.csr.2018.06.003
Dautenhahn, K., and Nehaniv, C. L. (2002). Imitation in Animals and Artifacts. Cambridge, MA: MIT Press. doi: 10.7551/mitpress/3676.001.0001
Davies, K. T. A., Ross, T., and Taggart, C. T. (2013). Tidal and subtidal currents affect deep aggregations of right whale prey, Calanus spp., along a shelf-basin margin. Mar. Ecol. Prog. Ser. 479, 263–282. doi: 10.3354/meps10189
Davies, K. T. A., Taggart, C. T., and Smedbol, R. K. (2014). Water mass structure defines the diapausing copepod distribution in a right whale habitat on the Scotian Shelf. Mar. Ecol. Prog. Ser. 497, 69–85. doi: 10.3354/meps10584
Davis, G. E., Baumgartner, M. F., Bonnell, J. M., Bell, J., Berchok, C., Bort Thornton, J., et al. (2017). Long-term passive acoustic recordings track the changing distribution of North Atlantic right whales (Eubalaena glacialis) from 2004 to 2014. Sci. Rep. 7:13460. doi: 10.1038/s41598-017-13359-3
Davis, R., Baumgartner, M., Comeau, A., Cunningham, D., Davies, K., Furlong, A., et al. (2016). “September tracking whales on the scotian shelf using passive acoustic monitoring on ocean gliders,” in Oceans 2016 MTS/IEEE Monterey, (Piscataway, NJ: IEEE), 1–4.
Dawbin, W. H. (1986). Right whales caught in waters around south eastern Australia and New Zealand during the nineteenth and early twentieth centuries. Rep. Int. Whaling Comm. 10, 261–267.
De Oliveira, L. R., Ott, P. H., Flores, P. A., Siciliano, S., De Almeida, R. S., and Bonatto, S. L. (2009). First molecular estimate of sex-ratio of southern right whale calves, Eubalaena australis, for Brazilian waters. J. Mar. Biol. Assoc. U. K. 89, 1003–1007. doi: 10.1017/S0025315409003178
Doucette, G. J., Mikulski, C. M., King, K. L., Roth, P. B., Wang, Z., Leandro, L. F., et al. (2012). Endangered North Atlantic right whales (Eubalaena glacialis) experience repeated, concurrent exposure to multiple environmental neurotoxins produced by marine algae. Environ. Res. 112, 67–76. doi: 10.1016/j.envres.2011.09.010
Du Pasquier, T. (1986). Catch history of French right whaling mainly in the South Atlantic. Rep. Int. Whaling Comm. 10, 268–274.
Durban, J. W., Fearnbach, H., Barrett-Lennard, L. G., Perryman, W. L., and Leroi, D. J. (2015). Photogrammetry of killer whales using a small hexacopter launched at sea. J. Unman. Veh. Syst. 3, 131–135. doi: 10.1139/juvs-2015-0020
Durbin, E., Teegarden, G., Campbell, R., Cembella, A., Baumgartner, M. F., and Mate, B. R. (2002). North Atlantic right whales, Eubalaena glacialis, exposed to paralytic shellfish poisoning (PSP) toxins via a zooplankton vector, Calanus finmarchicus. Harmful Algae 1, 243–251. doi: 10.1016/S1568-9883(02)00046-X
Elwen, S. H., and Best, P. (2004). Environmental factors influencing the distribution of southern right whales (Eubalaena australis) on the south coast of South Africa I: broad scale patterns. Mar. Mamm. Sci. 20, 567–582. doi: 10.1111/j.1748-7692.2004.tb01180.x
Fazio, A., Bertellotti, M., and Villanueva, C. (2012). Kelp gulls attack Southern right whales: a conservation concern? Mar. Biol. 159, 1981–1990. doi: 10.1371/journal.pone.0139291
Figueiredo, G. C., Marcos, C. D. O., Siciliano, S., and Moura, J. F. (2017). Southern right whales (Eubalaena australis) in an urbanized area off the Southwestern Atlantic Ocean: Updated records and conservation issues. Aquat. Mamm. 43:52. doi: 10.1578/AM.43.1.2017.52
Fiorito, C., Palacios, C., Golemba, M., Bratanich, A., Argüelles, M. B., Fazio, A., et al. (2015). Identification, molecular and phylogenetic analysis of poxvirus in skin lesions of southern right whale. Dis. Aquat. Organ. 116, 157–163. doi: 10.3354/dao02918
Fleishman, E., Costa, D. P., Harwood, J., Kraus, S., Moretti, D., New, L. F., et al. (2016). Monitoring population-level responses of marine mammals to human activities. Mar. Mamm. Sci. 32, 1004–1021. doi: 10.1111/mms.12310
Foote, A. D., Thomsen, P. F., Sveegaard, S., Wahlberg, M., Kielgast, J., Kyhn, L. A., et al. (2012). Investigating the potential use of environmental DNA (eDNA) for genetic monitoring of marine mammals. PLoS One 7:e41781. doi: 10.1371/journal.pone.0041781
Forcada, J., Trathan, P. N., Boveng, P. L., Boyd, I. L., Burns, J. M., Costa, D. P., et al. (2012). Responses of Antarctic pack-ice seals to environmental change and increasing krill fishing. Biol. Conserv. 149, 40–50. doi: 10.1016/j.biocon.2012.02.002
Ford, J. K. B., Ellis, G. M., Olesiuk, P. F., and Balcomb, K. C. (2010). Linking killer whale survival and prey abundance: food limitation in the oceans’ apex predator? Biol. Lett. 6, 139–142. doi: 10.1098/rsbl.2009.0468
Ford, J. K. B., Pilkington, J. F., Gisborne, B., Frasier, T. R., Abernethy, R. M., and Ellis, G. M. (2016). Recent observations of critically endangered North Pacific right whales (Eubalaena japonica) off the west coast of Canada. Mar. Biodivers. Rec. 9:50. doi: 10.1186/s41200-016-0036-3
Fortune, S. M. E., Trites, A. W., Mayo, C. A., Rosen, D. A. S., and Hamilton, P. K. (2013). Energetic requirements of North Atlantic right whales and the implications for species recovery. Mar. Ecol. Prog. Ser. 478, 253–272. doi: 10.3354/meps10000
Fowler, C. W. (1984). Density Dependence in Cetacean Populations. Report of the International Whaling Commission. Silver Spring, MD: NOAA, 373–379.
Frasier, T. R., Hamilton, P. K., Brown, M. W., Conger, L. A., Knowlton, A. R., Marx, M. K., et al. (2007). Patterns of male reproductive success in a highly promiscuous whale species: the endangered North Atlantic right whale. Mol. Ecol. 16, 5277–5293. doi: 10.1111/j.1365-294X.2007.03570.x
Fretwell, P. T., Staniland, I. J., and Forcada, J. (2014). Whales from space: counting southern right whales by satellite. PLoS One 9:e88655. doi: 10.1371/journal.pone.0088655
Fujiwara, M., and Caswell, H. (2001). Demography of the endangered North Atlantic right whale. Nature 414, 537–541. doi: 10.1038/35107054
Geoghegan, J. L., Pirotta, V., Harvey, E., Smith, A., Buchmann, J. P., Ostrowski, M., et al. (2018). Virological sampling of inaccessible wildlife with drones. Viruses 10:E300. doi: 10.3390/v10060300
Geraci, J. R., Harwood, J., and Lounsbury, V. J. (1999). Conservation and Management of Marine Mammals, eds R. John, Jr. Twiss, and R. Randall Reeves (Washington, DC: Smithsonian Institution Press), 471.
Gillespie, D., and Caillat, M. (2008). Statistical classification of odontocete clicks. Can. Acoust. 36, 20–26. doi: 10.1121/1.4927695
Givens, G. H., Edmondson, S. L., George, J. C., Suydam, R., Charif, R. A., Rahaman, A., et al. (2016). Hawthorne-Thompson whale abundance estimation adjusting for uncertain recapture, temporal availability variation and intermittent effort. Environmetrics 27, 134–146. doi: 10.1002/env.2379
Goddard, P. D., and Rugh, D. J. (1998). A group of right whales seen in the Bering Sea in July 1996. Mar. Mamm. Sci. 14, 344–349. doi: 10.1111/j.1748-7692.1998.tb00725.x
Good, C., and Johnston, D. (2010). “Spatial modeling of optimal North Pacific right whale (Eubalaena japonica) calving habitats,” in Proceedings of the Alaska Marine Science Symposium, Hotel Captain Cook, Anchorage, 71.
Goodyear, J. D. (1996). Significance of Feeding Habitats of North Atlantic Right Whales Based on Studies of Diel Behaviour, Diving, Food Ingestion Rates, and Prey. Ph.D. thesis. University of Guelph, Guelph, ON.
Greene, C. H., and Pershing, A. J. (2004). Climate and the conservation biology of North Atlantic right whales: the right whale at the wrong time? Front. Ecol. Environ. 2, 29–34. doi: 10.2307/3868292
Gregr, E. J., and Coyle, K. O. (2009). The biogeography of the North Pacific right whale (Eubalaena japonica). Prog. Oceanogr. 80, 188–198. doi: 10.1016/j.pocean.2008.12.004
Groch, K. (2018). “Conservation advances for the southern right whales in brazil,” in Advances in Marine Vertebrate Research in Latin America: Technological Innovation and Conservation, eds M. R. Rossi-Santos and C. W. Finkl (Cham: Springer International Publishing), 441–475.
Groch, K. R., Palazzo, J. T. Jr., Flores, P. A. C., Adler, F. R., and Fabian, M. E. (2005). Recent rapid increases in the right whale (Eubalaena australis) population off southern Brazil. Lat. Am. J. Aquat. Mamm. 4, 41–47. doi: 10.5597/lajam00068
Groth, M., Lange, J., Kanrai, P., Pleschka, S., Scholtissek, C., Krumbholz, A., et al. (2014). The genome of an influenza virus from a pilot whale: Relation to influenza viruses of gulls and marine mammals. Infect. Genet. Evol. 24, 183–186. doi: 10.1016/j.meegid.2014.03.026
Gulland, F. M. D., and Hall, A. J. (2007). Is marine mammal health deteriorating? trends in the global reporting of marine mammal disease. Ecohealth 4, 135–150. doi: 10.1007/s10393-007-0097-1
Hain, J. H. W., Hampp, J. D., McKenney, S. A., Albert, J. A., and Kenney, R. D. (2013). Swim speed, behavior, and movement of North Atlantic right whales (Eubalaena glacialis) in coastal waters of Northeastern Florida, USA. PLoS One 8:e54340. doi: 10.1371/journal.pone.0054340
Hakamada, T., and Matsuoka, K. (2016). The number of blue, fin, humpback, North Pacific right whales in the western North Pacific in the JARPN II offshore survey area. Paper Presented at the SC/F16/JR13 Presented to the IWC SC JARPNII Review Workshop, Tokyo.
Hall, A. J., Hugunin, K., Deaville, R., Law, R. J., Allchin, C. R., and Jepson, P. D. (2006). The risk of infection from polychlorinated biphenyl exposure in the harbor porpoise (Phocoena phocoena): a case–control approach. Environ. Health Perspect. 114:704. doi: 10.1289/ehp.8222
Hamilton, P. K., Knowlton, A. R., and Marx, M. K. (2007). “Right whales tell their own stories: the photo-identification catalog,” in The Urban Whale: North Atlantic right whales at the crossroads, eds S. D. Kraus and R. M. Rolland (Cambridge, MA: Harvard University Press), 75–104.
Hamner, W. A., Stone, G. S., and Obst, B. S. (1988). Behavior of southern right whales, Eubalaena australis, feeding on the Antarctic krill, Euphausia superba. Fish. Bull. 86, 143–150.
Hayes, S. A., Josephson, E., Maze-Foley, K., and Rosel, P. E. (2017). US Atlantic and Gulf of Mexico Marine Mammal Stock Assessments- 2016 NOAA Tech Memo NMFS-NE 241. Silver Spring, MD: NOAA.
Hiby, L., and Lovell, P. (2001). A note on an automated system for matching the callosity patterns on aerial photographs of southern right whales. J. Cetacean Res. Manag. 2, 291–295.
Hillman, G. R., Trask, K. D., Sweeney, K. L., Davis, A. R., Koski, W. R., Mocklin, J., et al. (2008). Photo-Identification Software for Bowhead Whale Images SC/60/BRG24. Cambridge: International Whaling Commission Scientific Committee.
Hlista, B. L., Sosik, H. M., Martin Traykovski, L. V., Kenney, R. D., and Moore, M. J. (2009). Seasonal and interannual correlations between right-whale distribution and calving success and chlorophyll concentrations in the Gulf of Maine, USA. Mar. Ecol. Prog. Ser. 394, 289–302. doi: 10.3354/meps08267
Hoffmeyer, M. S., Lindner, M. S., Carribero, A., Fulco, V. K., Meneìndez, M. C., Fernaìndez Severini, M. D., et al. (2010). Planktonic food and foraging of Eubalaena australis, on Peniìnsula Valdeìs (Argentina) nursery ground. Rev. Biol. Mar. Oceanogr. 45, 131–139. doi: 10.4067/S0718-19572010000100013
Houser, D. S., Champagne, C. D., Crocker, D. E., Kellar, N. M., Cockrem, J., Romano, T., et al. (2016). Natural Variation in Stress Hormones, Comparisons Across Matrices, and Impacts Resulting From Induced Stress in the Bottlenose Dolphin The Effects of Noise on Aquatic Life II. New York, NY: Springer, 467–471.
Hunt, K. E., Lysiak, N. S., Moore, M. J., and Rolland, R. M. (2016). Longitudinal progesterone profiles in baleen from female North Atlantic right whales (Eubalaena glacialis) match known calving history. Conserv. Physiol. 4:cow014. doi: 10.1093/conphys/cow014
Hunt, K. E., Rolland, R. M., and Kraus, S. D. (2015). Conservation Physiology of an uncatchable animal: The North Atlantic Right Whale (Eubalaena glacialis). Integr. Comp. Biol. 55, 577–586. doi: 10.1093/icb/icv071
Hunt, K. E., Rolland, R. M., Kraus, S. D., and Wasser, S. K. (2006). Analysis of fecal glucocorticoids in the North Atlantic right whale (Eubalaena glacialis). Gen. Comp. Endocrinol. 148, 260–272. doi: 10.1016/j.ygcen.2006.03.012
International Whaling Commission [IWC] (2001). Report of the workshop on comprehensive assessment of right whales: a worldwide comparison. J. Cetacean Res. Manag. 2, 1–60.
Ivashchenko, Y. V., and Clapham, P. J. (2012). Soviet catches of right whales Eubalaena japonica and bowhead whales Balaena mysticetus in the North Pacific Ocean and the Okhotsk Sea. Endanger. Species Res. 18, 201–217. doi: 10.3354/esr00443
Ivashchenko, Y. V., Clapham, P. J., and Brownell, R. Jr. (2013). Soviet catches of whales in the North Pacific: revised totals. J. Cetacean Res. Manag. 13, 59–71.
International Whaling Commission [IWC] (2011). Report of the southern right whale die-off workshop. J. Cetacean Res. Manag.t 12, 367–398.
International Whaling Commission [IWC] (2012). Report of the workshop on the assessment of southern right whales, Buenos Aires, Argentina 13-16 September 2011. Cambridge: International Whaling Commission Scientific Committee.
Jackson, J., Patenaude, N., Carroll, E., and Baker, C. S. (2008). How few whales were there after whaling? Inference from contemporary mtDNA diversity. Mol. Ecol. 17, 236–251. doi: 10.1111/j.1365-294X.2007.03497.x
Jackson, J. A., Carroll, E. L., and Smith, T. D. (2016). An integrated approach to historical population assessment of the great whales. R. Soc. Open Sci. 3:150669. doi: 10.1098/rsos.150669
Jacobs, E., Duffy, M., Magolan, J., Galletti Vernazzani, B., Cabrera, E., Landea, R., et al. (2018). First acoustic recordings of critically endangered eastern South Pacific southern right whales (Eubalaena australis). Mar. Mamm. Sci. 35, 284–289. doi: 10.1111/mms.12519
Jiang, M., Brown, M. W., Turner, J. T., Kenney, R. D., Mayo, C. A., Zhang, Z., et al. (2007). Springtime transport and retention of Calanus finmarchicus in Massachusetts and Cape Cod Bays, USA, and implications for right whale foraging. Mar. Ecol. Prog. Ser. 349, 183–197. doi: 10.3354/meps07088
Johnson, M., Tyack, P., Gillespie, D., and McConnell, B. (2014). A Multi-Week Behavioral Sampling Tag for Sound Effects Studies: Design Trade-Offs and Prototype Evaluation. St Andrews, KY: St Andrews University.
Johnson, M. P., and Tyack, P. L. (2003). A digital acoustic recording tag for measuring the response of wild marine mammals to sound. IEEE J. Ocean. Eng. 28, 3–12. doi: 10.1109/JOE.2002.808212
Kasuya, T. (1991). Density dependent growth in North Pacific sperm whales. Mar. Mamm. Sci. 7, 230–257. doi: 10.1111/j.1748-7692.1991.tb00100.x
Katona, S. K., and Kraus, S. D. (1999). “Efforts to conserve the North Atlantic right whale,” in Conservation and Management of Marine Mammals, eds J. R. Twiss and R. R. Reeves (Washington, DC: Smithsonian Institution Press),311–331.
Keith, S. A., and Bull, J. W. (2017). Animal culture impacts species’ capacity to realise climate-driven range shifts. Ecography 40, 296–304. doi: 10.1111/ecog.02481
Keller, C. A., Garrison, L., Baumstark, R., Ward-Geiger, L. I., and Hines, E. (2012). Application of a habitat model to define calving habitat of the North Atlantic right whale in the southeastern United States. Endanger. Species Res. 18, 73–87. doi: 10.3354/esr00413
Kemper, C., Coughran, D., Warneke, R., Pirzl, R., Watson, M., Gales, R., et al. (2008). Southern right whale (Eubalaena australis) mortalities and human interactions in Australia, 1950-2006. J. Cetacean Res. Manag. 10, 1–8.
Kennedy, A. S., Salden, D. R., and Clapham, P. J. (2012). First high- to low-latitude match of an eastern North Pacific right whale (Eubalaena japonica). Mar. Mamm. Sci. 28, E539–E544. doi: 10.1111/j.1748-7692.2011.00539.x
Kenney, R. D., Mayo, C. A., and Winn, H. E. (2001). Migration and foraging strategies at varying spatial scales in western North Atlantic right whales: a review of hypotheses. J. Cetacean Res. Manag. 2, 251–260.
Klumov, S. K. (1962). The right whales in the Pacific Ocean. Biol. Mar. Stud. Trud. Institute Oceanogr. 58, 202–297.
Kniest, E., Burns, D., and Harrison, P. (2010). Fluke matcher: a computer-aided matching system for humpback whale (Megaptera novaeangliae) flukes. Mar. Mamm. Sci. 26, 744–756.
Knowlton, A. R., Hamilton, P. K., Marx, M. K., Pettis, H. M., and Kraus, S. D. (2012a). Monitoring North Atlantic right whale Eubalaena glacialis entanglement rates: a 30 yr retrospective. Mar. Ecol. Prog. Ser. 466, 293–302. doi: 10.3354/meps09923
Knowlton, A. R., Hamilton, P. K., and Pettis, H. M. (2012b). Status of Reproductive Females in the North Atlantic Right Whale Population and Impacts of Human Activities on Their Reproductive Success. Silver Spring, MD: NOAA, 41.
Knowlton, A. R., Kraus, S. D., and Kenney, R. D. (1994). Reproduction in North Atlantic right whales (Eubalaena glacialis). Can. J. Zool. 72, 1297–1305. doi: 10.1139/z94-173
Knowlton, A. R., Sigurjosson, J., Ciano, J. N., and Kraus, S. D. (1992). Long-distance movements of North Atlantic right whales (Eubalaena glacialis). Mar. Mamm. Sci. 8, 397–405. doi: 10.1111/j.1748-7692.1992.tb00054.x
Koski, W. R., Gamage, G., Davis, A. R., Mathews, T., LeBlanc, B., and Ferguson, S. H. (2015). Evaluation of UAS for photographic re-identification of bowhead whales, Balaena mysticetus. J. Unmanned Veh. Syst. 3, 22–29. doi: 10.1139/juvs-2014-0014
Kraus, S., Fasick, J., Werner, T., and McFarron, P. (2014). Enhancing the Visibility of Fishing Ropes to Reduce Right Whale Entanglements Report to the Bycatch Reduction Engineering Program (BREP). Silver Spring, MD: Office of Sustainable Fisheries, 67–75.
Kraus, S. D., Hamilton, P. K., Kenney, R. D., Knowlton, A. R., and Slay, C. K. (2001). Reproductive parameters of the North Atlantic right whale. J. Cetacean Res. Manag. 2, 231–236.
Kraus, S. D., and Hatch, J. J. (2001). Mating strategies in the North Atlantic right whale (Eubalaena glacialis). J. Cetacean Res. Manag. 2, 237–244.
Kraus, S. D., Moore, K. E., Price, C. A., Crone, M. J., Watkins, W. A., Winn, H. E., et al. (1986). The Use of Photographs to Identify Individual North Atlantic Right Whales (Eubalaena glacialis). Cambridge: International Whaing Commnission, 145–151.
Kraus, S. D., and Rolland, R. (2007). The Urban Whale: North Atlantic Right Whales at the Crossroads. Cambridge: Harvard University Press.
Krumhansl, K. A., Head, E. J., Pepin, P., Plourde, S., Record, N. R., Runge, J. A., et al. (2018). Environmental drivers of vertical distribution in diapausing Calanus copepods in the Northwest Atlantic. Prog. Oceanogr. 162, 202–222. doi: 10.1016/j.pocean.2018.02.018
Krzystan, A. M., Gowan, T. A., Kendall, W. L., Martin, J., Ortega-Ortiz, J. G., Jackson, K., et al. (2018). Characterizing residence patterns of North Atlantic right whales in the southeastern USA with a multistate open robust design model. Endanger. Species Res. 36, 279–295. doi: 10.3354/esr00902
Lanyon, J. M., and Janetzki, H. (2016). Mortalities of Southern right whales (Eubalaena australis) in a subtropical wintering ground, Southeast Queensland. Aquat. Mamm. 42:470. doi: 10.1578/AM.42.4.2016.470
Leaper, R., Cooke, J., Trathan, P., Reid, K., Rowntree, V., and Payne, R. (2006). Global climate drives southern right whale (Eubalaena australis) population dynamics. Biol. Lett. 2, 289–292. doi: 10.1098/rsbl.2005.0431
LeDuc, R. G., Taylor, B. L., Martien, K. K., Robertson, K. M., Pitman, R. L., Salinas, J. C., et al. (2012). Genetic analysis of right whales in the eastern North Pacific confirms severe extirpation risk. Endangered Species Research 18, 163–167. doi: 10.3354/esr00440
Leiter, S., Stone, K., Thompson, J., Accardo, C., Wikgren, B., Zani, M., et al. (2017). North Atlantic right whale Eubalaena glacialis occurrence in offshore wind energy areas near Massachusetts and Rhode Island, USA. Endanger. Species Res. 34, 45–59. doi: 10.3354/esr00827
Leroy, G., Carroll, E. L., Bruford, M. W., DeWoody, J. A., Strand, A., Waits, L., et al. (2017). Next-generation metrics for monitoring genetic erosion within populations of conservation concern. Evol. Appl. 11, 1066–1083. doi: 10.1111/eva.12564
Lockyer, C. H., McConnell, L. C., and Waters, T. D. (1985). Body condition in terms of anatomical and biochemical assessment of body fat in North Atlantic fin and sei whales. Can. J. Zool. 63, 2328–2338. doi: 10.1139/z85-345
Lysiak, N. S. J. (2009). Investigating the Migration and Foraging Ecology of North Atlantic Right Whales with Stable Isotope Geochemistry of Baleen and Zooplankton. Ph.D. thesis, Boston University, Boston, MA.
Lysiak, N. S. J., Trumble, S. J., Knowlton, A. R., and Moore, M. J. (2018). Characterizing the duration and severity of fishing gear entanglement on a North Atlantic right whale (Eubalaena glacialis) using stable isotopes, steroid and thyroid hormones in baleen. Front. Mar. Sci. 5:168. doi: 10.3389/fmars.2018.00168
Malik, S., Brown, M. W., Kraus, S. D., Knowlton, A. R., Hamilton, P. K., and White, B. N. (1999). Assessment of mitochondrial DNA structuring and nursery use in the North Atlantic right whale (Eubalaena glacialis). Can. J. Zool. 77, 1217–1222. doi: 10.1139/z99-073
Marón, C. F., Beltramino, L., Di Martino, M., Chirife, A., Seger, J., Uhart, M., et al. (2015). Increased wounding of Southern right whale (Eubalaena australis) calves by kelp gulls (Larus dominicanus) at Península Valdés, Argentina. PLoS One 10:e0139291. doi: 10.1371/journal.pone.0139291
Marques, T. A., Munger, L., Thomas, L., Wiggins, S., and Hildebrand, J. A. (2011). Estimating North Pacific right whale Eubalaena japonica density using passive acoustic cue counting. Endanger. Species Res. 13, 163–172. doi: 10.3354/esr00325
Mate, B., Mesecar, R., and Lagerquist, B. (2007). The evolution of satellite-monitored radio tags for large whales: one laboratory’s experience. Deep Sea Res. Part II Top. Stud. Oceanogr. 54, 224–247. doi: 10.1016/j.dsr2.2006.11.021
Mate, B., Neukirk, S. L., Mesecar, R., and Martin, T. (1992). Application of Remote Sensing Methods for Tracking Large Cetaceans: North Atlantic Right Whales (Eubalaena glacialis). Washington, DC: United States Department of the Interior, 183.
Mate, B., Nieukirk, S. L., and Kraus, S. (1997). Satellite-monitored movements of the Northern right whale. J. Wildl. Manag. 61, 1393–1405. doi: 10.2307/3802143
Mate, B. R., Best, P. B., Lagerquist, B. A., and Winsor, M. H. (2011). Coastal, offshore, and migratory movements of South African right whales revealed by satellite telemetry. Mar. Mamm. Sci. 27, 455–476. doi: 10.1111/j.1748-7692.2010.00412.x
Mate, B. R., Irvine, L. M., and Palacios, D. M. (2017). The development of an intermediate-duration tag to characterize the diving behavior of large whales. Ecol. Evol. 7, 585–595. doi: 10.1002/ece3.2649
Matthews, J., Brown, S., Gillespie, D., Johnson, M., McLanaghan, R., Moscrop, A., et al. (2001). Vocalisation rates of the North Atlantic right whale (Eubalaena glacialis). J. Cetacean Res. Manag. 3, 271–282. doi: 10.1121/1.3562166
Mayo, C. A., Ganley, L., Hudak, C. A., Brault, S., Marx, M. K., Burke, E., et al. (2018). Distribution, demography, and behavior of North Atlantic right whales (Eubalaena glacialis) in Cape Cod Bay, Massachusetts, 1998-2013. Mar. Mamm. Sci. 34, 979–996. doi: 10.1111/mms.12511
Mayo, C. A., Letcher, B. H., and Scott, S. (2001). Zooplankton filtering efficiency of the baleen of a North Atlantic right whale, Eubalaena glacialis. J. Cetacean Res. Manag. 2, 225–229.
Mayo, C. A., and Marx, M. K. (1990). Surface foraging behaviour of the North Atlantic right whale, Eubalaena glacialis, and associated zooplankton characteristics. Can. J. Zool. 68, 2214–2220. doi: 10.1139/z90-308
McEwen, B. S., and Wingfield, J. C. (2003). The concept of allostasis in biology and biomedicine. Horm. Behav. 43, 2–15. doi: 10.1016/S0018-506X(02)00024-7
McEwen, B. S., and Wingfield, J. C. (2010). What’s in a name? Integrating homeostasis, allostasis and stress. Horm. Behav. 57:105. doi: 10.1016/j.yhbeh.2009.09.011
McLeod, B. A., Brown, M. W., Frasier, T. R., and White, B. N. (2010). DNA profile of a sixteenth century western North Atlantic right whale (Eubalaena glacialis). Conserv. Genet. 11, 339–345. doi: 10.1007/s10592-009-9811-6
McLeod, B. A., Brown, M. W., Moore, M. J., Stevens, W., Barkham, S. H., Barkham, M., et al. (2008). Bowhead whales, and not right whales, were the primary target of 16th-to 17th-century Basque whalers in the western North Atlantic. Arctic 1, 61–75.
Mellinger, D. K., Nieukirk, S. L., Klinck, K., Klinck, H., Dziak, R. P., Clapham, P. J., et al. (2011). Confirmation of right whales near a nineteenth-century whaling ground east of southern Greenland. Biol. Lett. 7, 411–413. doi: 10.1098/rsbl.2010.1191
Mellinger, D. K., Nieukirk, S. L., Matsumoto, H., Heimlich, S. L., Dziak, R. P., Haxel, J., et al. (2007). Seasonal occurrence of North Atlantic right whale (Eubalaena glacialis) vocalizations at two sites on the Scotian Shelf. Mar. Mamm. Sci. 23, 856–867. doi: 10.1111/j.1748-7692.2007.00144.x
Mellinger, D. K., Stafford, K. M., Moore, S. E., Munger, L., and Fox, C. G. (2004). Detection of North Pacific right whale (Eubalaena japonica) calls in the Gulf of Alaska. Mar. Mamm. Sci. 20, 872–879. doi: 10.1121/1.2799905
Meyer-Gutbrod, E. L., and Greene, C. H. (2018). Uncertain recovery of the North Atlantic right whale in a changing ocean. Glob. Change Biol. 24, 455–464. doi: 10.1111/gcb.13929
Miller, C. A., Best, P. B., Perryman, W. L., Baumgartner, M. F., and Moore, M. J. (2012). Body shape changes associated with reproductive status, nutritive condition and growth in right whales Eubalaena glacialis and E. australis. Mar. Ecol. Prog. Ser. 459, 135–156. doi: 10.3354/meps09675
Miller, C. A., Reeb, D., Best, P. B., Knowlton, A. R., Brown, M. W., and Moore, M. J. (2011). Blubber thickness in right whales Eubalaena glacialis and Eubalaena australis related with reproduction, life history status and prey abundance. Mar. Ecol. Prog. Ser. 438, 267–283. doi: 10.3354/meps09174
Miller, M., Gardner, I., Kreuder, C., Paradies, D., Worcester, K., Jessup, D., et al. (2002). Coastal freshwater runoff is a risk factor for Toxoplasma gondii infection of southern sea otters (Enhydra lutris nereis). Int. J. Parasitol. 32, 997–1006. doi: 10.1016/S0020-7519(02)00069-3
Miyashita, T., and Kato, H. (1998). Recent Data on the Status of Right Whales in the New Pacific Ocean SC/M98/AWL. Washington, DC: International Whaling Commission.
Moore, M., Andrews, R., Austin, T., Bailey, J., Costidis, A., George, C., et al. (2012). Rope trauma, sedation, disentanglement, and monitoring-tag associated lesions in a terminally entangled North Atlantic right whale (Eubalaena glacialis). Mar. Mamm. Sci. 29, E98–E113. doi: 10.1111/j.1748-7692.2012.00591.x
Moore, M., Berrow, S., Jensen, B., Carr, P., Sears, R., Rowntree, V., et al. (1999). Relative abundance of large whales around South Georgia (1979–1998). Mar. Mamm. Sci. 15, 1287–1302. doi: 10.1111/j.1748-7692.1999.tb00891.x
Moore, M. J., Durban, J., Fearnbach, H., Lynn, M., Pettis, H. M., and Perryman, W. L. (2017). “Photogrammetry from manned and unmanned aircraft reveals constrained growth of North Atlantic right whales,” in Proceedings of the 22nd Biennial Conference on the Biology of Marine Mammals, Halifax.
Moore, M. J., Knowlton, A. R., Kraus, S. D., McLellan, W. A., and Bonde, R. K. (2004). Morphometry, gross morphology and available histopathology in North Atlantic right whale (Eubalaena glacialis) mortalities (1970–2002). J. Cetacean Res. Manag. 6, 199–214.
Moore, M. J., van der Hoop, J. M., Barco, S. G., Costidis, A. M., Gulland, F. M., Jepson, P. D., et al. (2013). Criteria and case definitions for serious injury and death of pinnipeds and cetaceans caused by anthropogenic trauma. Dis. Aquat. Organ. 103, 229–264. doi: 10.3354/dao02566
Moore, M. J., and Zerbini, A. N. (2017). Dolphin blubber/axial muscle shear: implications for rigid transdermal intramuscular tracking tag trauma in whales. J. Exp. Biol. 220, 3717–3723. doi: 10.1242/jeb.165282
Morano, J. L., Rice, A. N., Tielens, J. T., Estabrook, B. J., Murray, A., Roberts, B. L., et al. (2012). Acoustically detected year-round presence of right whales in an urbanized migration corridor. Conserv. Biol. 26, 698–707. doi: 10.1111/j.1523-1739.2012.01866.x
Munger, L. M., Wiggins, S. M., Moore, S. E., and Hildebrand, J. A. (2008). North Pacific right whale (Eubalaena japonica) seasonal and diel calling patterns from long-term acoustic recordings in the southeastern Bering Sea, 2000-2006. Mar. Mamm. Sci. 24, 795–814. doi: 10.1111/j.1748-7692.2008.00219.x
Murison, L., and Gaskin, D. E. (1989). The distribution of right whales and zooplankton in the Bay of Fundy, Canada. Can. J. Zool. 67, 1411–1420. doi: 10.1139/z89-200
Muto, M. M., Helker, V. T., Angliss, R. P., Allen, B. A., Boveng, P. L., Breiwick, J. M., et al. (2017). Alaska Marine Mammal Stock Assessments, 2017. Washington, D.C: U.S. Dep. Commer.
NARWC (2018). North Atlantic Right Whale Consortium Database (Anderson Cabot Center for Ocean Life at the New England Aquarium. Boston, MA: NARWC.
National Academies of Sciences, and Engineering and Medicine (2017). Approaches to Understanding the Cumulative Effects of Stressors on Marine Mammals. Washington, DC: The National Academies Press.
Nei, M. (1975). Molecular Population Genetics and Evolution. Amsterdam: North-Holland Publishing Company. doi: 10.3354/meps10547
New, L. F., Clark, J. S., Costa, D. P., Fleishman, E., Hindell, M. A., Klanjšcek, T., et al. (2014). Using short-term measures of behaviour to estimate long-term fitness of southern elephant seals. Mar. Ecol. Prog. Ser. 496, 99–108.
New Zealand Blue Book (1841). Blue Book of Statistics. 1841–1853 Australian Joint Copying Project Colonial Office (CO213) Pro reel. Canberra: National Library of Australia, 1502–1504.
NOAA (2008). Differentiating Serious and Non-Serious Injury of Marine Mammals: Technical Memorandum NMFS-OPR-39. Silver Spring, MD: NOAA.
NOAA (2017). 2017-2018 North Atlantic Right Whale Unusual Mortality Event. 2018: NOAA Fisheries. Silver Spring, MD: NOAA. doi: 10.1111/mms.12443
Norman, S. A., Flynn, K. R., Zerbini, A. N., Gulland, F., Moore, M. J., Raverty, S., et al. (2018). Assessment of wound healing of tagged gray (Eschrichtius robustus) and blue (Balaenoptera musculus) whales in the eastern North Pacific using long-term series of photographs. Mar. Mamm. Sci. 34, 27–53. doi: 10.1086/671811
Nousek-McGregor, A. E., Miller, C. A., Moore, M. J., and Nowacek, D. P. (2013). Effects of body condition on buoyancy in endangered North Atlantic right whales. Physiol. Biochem. Zool. 87, 160–171. doi: 10.1086/671811
Nowacek, D. P., Johnson, M. P., and Tyack, P. L. (2004). North Atlantic right whales (Eubalaena glacialis) ignore ships but respond to alerting stimuli. Proc. R. Soc. Lond. Series B Biol. Sci. 271, 227–231. doi: 10.1098/rspb.2001.1730
Nowacek, D. P., Johnson, M. P., Tyack, P. L., Shorter, K. A., McLellan, W. A., and Pabst, D. A. (2001). Buoyant balaenids: the ups and downs of buoyancy in right whales. Proc. R. Soc. B Biol. Sci. 268, 1811–1816.
NRC (2005). Marine Mammal Populations and Ocean Noise: Determining When Noise Causes Biologically Significant Effects. Washington, DC: National Academies Press.
Omura, H., Ohsumi, S., Nemoto, T., Nasu, K., and Kasuya, T. (1969). Black right whales in the North Pacific. Sci. Rep. Whales Res. Institute 21, 1–66. doi: 10.1111/mms.12243
Ovsyanikova, E., Fedutin, I., Belonovich, O., Burdin, A., Burkanov, V., Dolgova, E., et al. (2015). Opportunistic sightings of the endangered North Pacific right whales (Eubalaena japonica) in Russian waters in 2003-2014. Mar. Mamm. Sci. 31, 1559–1567. doi: 10.1002/ece3.3406
Pace, R. M. III, Corkeron, P. J., and Kraus, S. D. (2017). State-space mark-recapture estimates reveal a recent decline in abundance of North Atlantic right whales. Ecol. Evol. 7, 8730–8741. doi: 10.1002/ece3.3406
Parks, S. E., Searby, A., Célérier, A., Johnson, M. P., Nowacek, D. P., and Tyack, P. L. (2011). Sound production behavior of individual North Atlantic right whales: implications for passive acoustic monitoring. Endanger. Species Res. 15, 63–76. doi: 10.1098/rsbl.2011.0578
Parks, S. E., Warren, J. D., Stamieszkin, K., Mayo, C. A., and Wiley, D. (2012). Dangerous dining: surface foraging of North Atlantic right whales increases risk of vessel collisions. Biol. Lett. 8, 57–60. doi: 10.1098/rsbl.2011.0578
Pastene, L., Taguchi, M., Lang, A., Goto, M., and Matsuoka, K. (2018). Population Genetic Structure and Historical Demography of North Pacific Right Whales. Washington, D.C: International Whaling Commission, 18.
Patenaude, N. J., and Baker, C. S. (2001). Population status and habitat use of southern right whales in the sub-Antarctic Auckland Islands of New Zealand. J. Cetacean Res. Manag. 2, 111–116.
Patenaude, N. J., Baker, C. S., and Gales, N. J. (1998). Observations of southern right whales on New Zealand’s subantarctic wintering grounds. Mar. Mamm. Sci. 14, 350–355. doi: 10.1111/j.1748-7692.1998.tb00726.x
Patenaude, N. J., Portway, V. A., Schaeff, C. M., Bannister, J. L., Best, P. B., Payne, R. S., et al. (2007). Mitochondrial DNA diversity and population structure among southern right whales (Eubalaena australis). J. Heredity 98, 147–157. doi: 10.1093/jhered/esm005
Patrician, M. R., Biedron, I. S., Esch, H. C., Wenzel, F. W., Cooper, L. A., Hamilton, P. K., et al. (2009). Evidence of a North Atlantic right whale calf (Eubalaena glacialis) born in northeastern U.S. waters. Mar. Mamm. Sci. 25, 462–477. doi: 10.1111/j.1748-7692.2008.00261.x
Patrician, M. R., and Kenney, R. D. (2010). Using the continuous plankton recorder to investigate the absence of North Atlantic right whales (Eubalaena glacialis) from the roseway basin foraging ground. J. Plankton Res. 32, 1685–1695. doi: 10.1093/plankt/fbq073
Payne, R. (1986). Long Term Behavioral Studies of the Southern Right Whale, Eubalaena australis. Washington, DC: International Whaling Commission 10, 161–167.
Payne, R., Brazier, O., Dorsey, E. M., Perkins, J. S., Rowntree, V. J., and Titus, A. (1981). External Features in Southern Right Whales (Eubalaena australis) and their Use in Identifying individuals. Bethesda, MD: US Marine Mammal Commission.
Peel, D., Smith, J. N., and Childerhouse, S. (2018). Vessel strike of whales in Australia: the challenges of analysis of historical incident data. Front. Mar. Sci. 5:69. doi: 10.3389/fmars.2018.00069
Pendleton, D. E., Sullivan, P. J., Brown, M. W., Cole, T. V. N., Good, C. P., Mayo, C. A., et al. (2012). Weekly predictions of North Atlantic right whale Eubalaena glacialis habitat reveal influence of prey abundance and seasonality of habitat preferences. Endanger. Species Res. 18, 147–161. doi: 10.3354/esr00433
Pettis, H., and Hamilton, P. (2015). North Atlantic Right Whale Consortium 2015 Annual Report card Report to the North Atlantic Right Whale Consortium. Available at: https://www.narwc.org/uploads/1/1/6/6/116623219/2015_report_card.pdf
Pettis, H. M. (2009). North Atlantic Right Whale Consortium Annual Report Card International Whaling Commission SC/61/BRG11. Cambridge: IWC.
Pettis, H. M., Rolland, R. M., Hamilton, P. K., Brault, S., Knowlton, A. R., and Kraus, S. D. (2004). Visual health assessment of North Atlantic right whales (Eubalaena glacialis) using photographs. Canadian Journal of Zoology 82, 8–19. doi: 10.1139/z03-207
Pettis, H. M., Rolland, R. M., Hamilton, P. K., Knowlton, A. R., Burgess, E. A., and Kraus, S. D. (2017). Body condition changes arising from natural factors and fishing gear entanglements in North Atlantic right whales Eubalaena glacialis. Endange. Species Res. 32, 237–249. doi: 10.3354/esr00800
Pirotta, E., Booth, C. G., Costa, D. P., Fleishman, E., Kraus, S. D., Lusseau, D., et al. (2018). Understanding the population consequences of disturbance. Ecol. Evol. 8, 9934–9946. doi: 10.1002/ece3.4458
Pirotta, V., Grech, A., Jonsen, I. D., Laurence, W., and Harcourt, R. (2018). Consequences of global shipping traffic for marine giants. Front. Ecol. Environ. doi: 10.1002/fee.1987
Pirotta, V., Smith, A., Ostrowski, M., Russell, D., Jonsen, I. D., Grech, A., et al. (2017). An economical custom-built drone for assessing whale health. Front. Mar. Sci. 4:425. doi: 10.3389/fmars.2017.00425
Pirzl, R., Patenaude, N. J., Burnell, S., and Bannister, J. (2009). Movements of southern right whales (Eubalaena australis) between Australian and subantarctic New Zealand populations. Mar. Mamm. Sci. 25, 455–461. doi: 10.1111/j.1748-7692.2008.00276.x
Rastogi, T., Brown, M. W., McLeod, B. A., Frasier, T. R., Grenier, R., Cumbaa, S. L., et al. (2004). Genetic analysis of 16th-century whale bones prompts a revision of the impact of Basque whaling on right and bowhead whales in the western North Atlantic. Can. J. Zool. 82, 1647–1654. doi: 10.1139/z04-146
Rayment, W., Davidson, A., Dawson, S., Slooten, E., and Webster, T. (2012). Distribution of southern right whales on the Auckland Islands calving grounds. N. Z. J. Mar. Freshwater Res. 46, 431–436. doi: 10.1080/00288330.2012.697072
Rayment, W., Dawson, S., Webster, T., and Crame, A. (2015). Breeding status affects fine-scale habitat selection of southern right whales on their wintering grounds. J. Biogeogr. 42, 463–474. doi: 10.1111/jbi.12443
Rayment, W., Webster, T., Brough, T., Jowett, T., and Dawson, S. (2018). Seen or heard? A comparison of visual and acoustic autonomous monitoring methods for investigating temporal variation in occurrence of southern right whales. Mar. Biol. 165:12. doi: 10.1007/s00227-017-3264-0
Reeves, R., Rosa, C., George, J. C., Sheffield, G., and Moore, M. (2012). Implications of Arctic industrial growth and strategies to mitigate future vessel and fishing gear impacts on bowhead whales. Mar. Policy 36, 454–462. doi: 10.1016/j.marpol.2011.08.005
Reeves, R. R., Breiwick, J. M., and Mitchell, E. D. (1992). “Pre-exploitation abundance of right whales off the eastern United States,” in The Right Whale in the Western North Atlantic: A Science and Management Workshop, ed. J. Hain (Silver Spring, MD: Northeast Fisheries Science Center), 5–7.
Reeves, R. R., Breiwick, J. M., and Mitchell, E. D. (1999). History of whaling and estimated kill of right whales, Balaena glacialis, in the Northeastern United States, 1620–1924. Mar. Fish. Rev. 61, 1–36.
Reeves, R. R., Smith, T. D., and Josephson, E. A. (2007). Near-Annihilation of a Species: Right Whaling in the North Atlantic. The Urban Whale: North Atlantic Right Whales at the Crossroads. Cambridge, MA: Harvard University Press, 39–74.
Reeves, R. R. and Smith, T. (2006). A Taxonomy of World Whaling. Whales, Whaling, and Ocean Ecosystems eds J. A. Estes, D. P. DeMaster, D. F. Doak, T. M. Williams, and R. L. Brownell (Berkeley: University of California Press), 82–101.
Reid, K., Brierley, A. S., and Nevitt, G. A. (2000). An initial examination of relationships between the distribution of whales and Antarctic krill Euphausia superba at South Georgia. J. Cetacean Res. Manag. 2, 143–149.
Richards, R. (2009). Past and present distributions of southern right whales (Eubalaena australis). N. Z. J. Zool. 36, 447–459. doi: 10.1080/03014223.2009.9651477
Rocha, R. C., Clapham, P. J., and Ivashchenko, Y. V. (2014). Emptying the oceans: a summary of industrial whaling catches in the 20th century. Mar. Fish. Rev. 76, 37–48. doi: 10.7755/MFR.76.4.3
Rolland, R. M., Hunt, K. E., Kraus, S. D., and Wasser, S. K. (2005). Assessing reproductive status of right whales (Eubalaena glacialis) using fecal hormone metabolites. Gen. Comp. Endocrinol. 142, 308–317. doi: 10.1016/j.ygcen.2005.02.002
Rolland, R. M., McLellan, W. A., Moore, M. J., Harms, C. A., Burgess, E. A., and Hunt, K. E. (2017). Fecal glucocorticoids and anthropogenic injury and mortality in North Atlantic right whales Eubalaena glacialis. Endange. Species Res. 34, 417–429. doi: 10.3354/esr00866
Rolland, R. M., Parks, S. E., Hunt, K. E., Castellote, M., Corkeron, P. J., Nowacek, D. P., et al. (2012). Evidence that ship noise increases stress in right whales. Proc. R. Soc. Biol. Sci. B 279, 2363–2368. doi: 10.1098/rspb.2011.2429
Rolland, R. M., Schick, R. S., Pettis, H. M., Knowlton, A. R., Hamilton, P. K., Clark, J. S., et al. (2016). Health of North Atlantic right whales Eubalaena glacialis over three decades: from individual health to demographic and population health trends. Mar. Ecol. Prog. Ser. 542, 265–282. doi: 10.3354/meps11547
Rone, B. K., Berchok, C. L., Crance, J. L., and Clapham, P. J. (2012). Using air-deployed passive sonobuoys to detect and locate critically endangered North Pacific right whales. Mar. Mamm. Sci. 28, E528–E538. doi: 10.1111/j.1748-7692.2012.00573.x
Rosenbaum, H. C., Brownell, R. L. Jr., Brown, M. W., Schaeff, C., Portway, V., White, B. N., et al. (2000). World-wide genetic differentiation of Eubalaena: questioning the number of right whale species. Mol. Ecol. 9, 1793–1802. doi: 10.1046/j.1365-294x.2000.01066.x
Rowntree, V., McGuinness, P., Marshall, K., Payne, R., Sironi, M., and Seger, J. (1998). Increased harassment of right whales (Eubalaena australis) by kelp gulls (Larus dominicanus) at Península Valdés, Argentina. Mar. Mamm. Sci. 14, 99–115. doi: 10.1111/j.1748-7692.1998.tb00693.x
Rowntree, V. J. (2001). Foraging behaviour of southern right whales (Eubalaena australis) inferred from variation of carbon stable isotope ratios in their baleen. J. Cetacean Res. Manag..
Rowntree, V. J., Payne, R. S., and Schell, D. (2001). Changing patterns of habitat use by southern right whales (Eubalaena australis) on their nursery ground at Península Valdés, Argentina, and in their long-range movements. J. Cetacean Res. Manag. 2, 133–143.
Rowntree, V. J., Uhart, M. M., Sironi, M., Chirife, A., Di Martino, M., La Sala, L., et al. (2013). Unexplained recurring high mortality of southern right whale Eubalaena australis calves at Penïnsula Valdïs, Argentina. Mar. Ecol. Progr. Ser. 493, 275–289. doi: 10.3354/meps10506
Rowntree, V. J., Valenzuela, L. O., Fraguas, P. F., and Seger, J. (2008). Foraging Behaviour of Southern Right Whales (Eubalaena australis) Inferred from Variation of Carbon Stable Isotope Ratios in their Baleen. Cambridge: International Whaling Commission.
Scarff, J. E. (1986). Historic and present distribution of the right whale (Eubalaena glacialis) in the Eastern North Pacific, South of 50 Nn and East Of 180 W. Int. Whaling Comm. 10, 43–63.
Scarff, J. E. (1991). Historic distribution and abundance of the right whale (Eubalaena glacialis) in the North Pacific, Bering Sea, Sea of Okhotsk and Sea of Japan from the Maury whale charts. Int. Whaling Comm. 41, 467–489.
Scarff, J. E. (2001). Preliminary estimates of whaling-induced mortality in the 19th century North Pacific right whale (Eubalaena japonicus) fishery, adjusting for struck-but-lost whales and non-American whaling. J. Cetacean Res. Manag. 2, 261–268.
Schaeff, C. M., Kraus, S. D., Brown, M. W., and White, B. N. (1993). Assessment of the population structure of western North Atlantic right whales (Eubalaena glacialis) based on sighting and mtDNA data. Can. J. Zool. 71, 339–345. doi: 10.1139/z93-047
Schick, R. S., Halpin, P. N., Read, A. J., Slay, C. K., Kraus, S. D., Mate, B. R., et al. (2009). Striking the right balance in right whale conservation. Can. J. Fish. Aquat. Sci. 66, 1399–1403. doi: 10.1139/F09-115
Schick, R. S., Kraus, S. D., Rolland, R. M., Knowlton, A. R., Hamilton, P. K., Pettis, H. M., et al. (2013). Using hierarchical Bayes to understand movement, health, and survival in the endangered North Atlantic right whale. PLoS One 8:e64166. doi: 10.1371/journal.pone.0064166
Schlaepfer, M. A., Runge, M. C., and Sherman, P. W. (2002). Ecological and evolutionary traps. Trends Ecol. Evol. 17, 474–480. doi: 10.1016/j.tree.2013.04.004
Sekiguchi, K., Onishi, H., Sasaki, H., Haba, S., Iwahara, Y., Mizuguchi, D., et al. (2014). Sightings of the western stock of North Pacific right whales (Eubalaena japonica) in the far southeast of the Kamchatka Peninsula. Mar. Mamm. Sci. 30, 1199–1209. doi: 10.1111/mms.12105
Seyboth, E., Groch, K. R., Dalla Rosa, L., Reid, K., Flores, P. A., and Secchi, E. R. (2016). Southern right whale (Eubalaena australis) reproductive success is influenced by krill (Euphausia superba) density and climate. Sci. Rep. 6:28205. doi: 10.1038/srep28205
Shelden, K. E., Moore, S. E., Waite, J. M., Wade, P. R., and Rugh, D. J. (2005). Historic and current habitat use by North Pacific right whales Eubalaena japonica in the Bering Sea and Gulf of Alaska. Mamm. Rev. 35, 129–155. doi: 10.1111/j.1365-2907.2005.00065.x
Sierra, E., Fernández, A., Suárez-Santana, C., Xuriach, A., Zucca, D., de Quirós, Y. B., et al. (2016). Morbillivirus and pilot whale deaths, Canary Islands, Spain, 2015. Emerg. Infect. Dis. 22, 740–742. doi: 10.3201/eid2204.150954
Sierra, E., Zucca, D., Arbelo, M., García-Álvarez, N., Andrada, M., Déniz, S., et al. (2014). Fatal systemic morbillivirus infection in bottlenose dolphin, Canary Islands, Spain. Emerg. Infect. Dis. 20:269–271. doi: 10.3201/eid2002.131463
Sigsgaard, E. E., Nielsen, I. B., Bach, S. S., Lorenzen, E. D., Robinson, D. P., Knudsen, S. W., et al. (2016). Population characteristics of a large whale shark aggregation inferred from seawater environmental DNA. Nat. Ecol. Evol. 1:0004. doi: 10.1038/s41559-016-0004
Sironi, M., Rowntree, V. J., Snowdon, C. T., Valenzuela, L., and Marón, C. (2009). Kelp Gulls (Larus dominicanus) Feeding on Southern Right Whales (Eubalaena australis) at Península Valdés, Argentina: Updated Estimates and Conservation Implications. Washington, DC: International Whaling Commission.
Širović, A., Johnson, S. C., Roche, L. K., Varga, L. M., Wiggins, S. M., and Hildebrand, J. A. (2015). North Pacific right whales (Eubalaena japonica) recorded in the northeastern Pacific Ocean in 2013. Mar. Mamm. Sci. 31, 800–807. doi: 10.1111/mms.12189
Smith, T. D., Reeves, R. R., Josephson, E. A., and Lund, J. N. (2012). Spatial and seasonal distribution of American whaling and whales in the age of sail. PLoS One 7:e34905. doi: 10.1371/journal.pone.0034905
Suisted, R., and Neale, D. M. (2004). Department of Conservation Marine Mammal Action Plan For 2005-2010. Te Aro: Department of Conservation Wellington.
Thomas, P. O., Reeves, R. R., and Brownell, R. Jr. (2016). Status of the world’s baleen whales. Mar. Mamm. Sci. 32, 682–734. doi: 10.1111/mms.12281
Tormosov, D., Mikhaliev, Y., Best, P., Zemsky, V., Sekiguchi, K., and Brownell, R. Jr. (1998). Soviet catches of southern right whales Eubalaena australis, 1951–1971. Biol. Data Conserv. Implic. 86, 185–197. doi: 10.1016/S0006-3207(98)00008-1
Torres, L. G., Rayment, W., Olavarría, C., Thompson, D. R., Graham, B., Baker, C. S., et al. (2017). Demography and ecology of southern right whales Eubalaena australis wintering at sub-Antarctic Campbell Island, New Zealand. Polar Biol. 40, 95–106. doi: 10.1007/s00300-016-1926-x
Torres, L. G., Smith, T. D., Sutton, P., MacDiarmid, A., Bannister, J., and Miyashita, T. (2013). From exploitation to conservation: habitat models using whaling data predict distribution patterns and threat exposure of an endangered whale. Divers. Distrib. 19, 1138–1152. doi: 10.1111/ddi.12069
Trathan, P., Brierley, A., Brandon, M., Bone, D., Goss, C., Grant, S., et al. (2003). Oceanographic variability and changes in Antarctic krill (Euphausia superba) abundance at South Georgia. Fish. Oceanogr. 12, 569–583. doi: 10.1046/j.1365-2419.2003.00268.x
Tsoularis, A., and Wallace, J. (2002). Analysis of logistic growth models. Math. Biosci. 179, 21–55. doi: 10.1016/S0025-5564(02)00096-2
Tynan, C. T., DeMaster, D. P., and Peterson, W. T. (2001). Endangered right whales on the southeastern Bering Sea shelf. Science 294, 1894–1894. doi: 10.1126/science.1065682
Valenzuela, L. O., Rowntree, V. J., Sironi, M., and Seger, J. (2018). Stable isotopes (δ15N, δ13C, δ34S) in skin reveal diverse food sources used by southern right whales Eubalaena australis. Mar. Ecol. Prog. Ser. 603, 243–255. doi: 10.3354/meps12722
Valenzuela, L. O., Sironi, M., and Rowntree, V. J. (2010). Interannual variation in the stable isotope differences between mothers and their calves in Southern Right Whales (Eubalaena australis). Aquat. Mamm. 36, 138–147. doi: 10.1578/AM.36.2.2010.138
Valenzuela, L. O., Sironi, M., Rowntree, V. J., and Seger, J. (2009). Isotopic and genetic evidence for culturally inherited site fidelity to feeding grounds in southern right whales (Eubalaena australis). Mol. Ecol. 18, 782–791. doi: 10.1111/j.1365-294X.2008.04069.x
Van Bressem, M.-F., Raga, J. A., Di Guardo, G., Jepson, P. D., Duignan, P. J., Siebert, U., et al. (2009). Emerging infectious diseases in cetaceans worldwide and the possible role of environmental stressors. Dis. Aquat. Organ. 86, 143–157. doi: 10.3354/dao02101
van der Hoop, J. M., Corkeron, P., and Moore, M. (2016a). Entanglement is a costly life-history stage in large whales. Ecol. Evol. 7, 92–106. doi: 10.1002/ece3.2615
van der Hoop, J. M., Corkeron, P., Kenney, J., Landry, S., Morin, D., Smith, J., et al. (2016b). Drag from fishing gear entangling North Atlantic right whales. Mar. Mamm. Sci. 32, 619–642. doi: 10.1111/mms.12292
van der Hoop, J. M., Corkeron, P., Henry, A. G., Knowlton, A. R., and Moore, M. J. (2017a). Predicting lethal entanglements as a consequence of drag from fishing gear. Mar. Pollut. Bull. 115, 91–104. doi: 10.1016/j.marpolbul.2016.11.060
van der Hoop, J. M., Nowacek, D. P., Moore, M. J., and Triantafyllou, M. S. (2017b). Swimming kinematics and efficiency of entangled North Atlantic right whales. Endanger. Species Res. 32, 1–17. doi: 10.3354/esr00781
van der Hoop, J. M., Moore, M. J., Barco, S. G., Cole, T. V., Daoust, P. Y., Henry, A. G., et al. (2013a). Assessment of management to mitigate anthropogenic effects on large whales. Conserv. Biol. 27, 121–133. doi: 10.1111/j.1523-1739.2012.01934.x
van der Hoop, J. M., Moore, M. J., Fahlman, A., Bocconcelli, A., George, C., Jackson, K., et al. (2013b). Behavioral impacts of disentanglement of a right whale under sedation and the energetic cost of entanglement. Mar. Mamm. Sci. 30, 282–307. doi: 10.1111/mms.12042
van der Hoop, J. M., Vanderlaan, A. S. M., Cole, T. V. N., Henry, A. G., Hall, L., Mase-Guthrie, B., et al. (2014). Vessel strikes to large whales before and after the 2008 Ship Strike Rule. Conserv. Lett. 8, 24–32. doi: 10.1111/conl.12105
van der Hoop, J. M., Vanderlaan, A. S. M., and Taggart, C. T. (2012). Absolute probability estimates of lethal vessel strikes to North Atlantic right whales in Roseway Basin, Scotian Shelf. Ecol. Appl. 22, 2021–2033. doi: 10.1890/11-1841.1
Van Parijs, S. M., Baumgartner, M., Cholewiak, D., Davis, G., Gedamke, J., Gerlach, D., et al. (2015). NEPAN: A U.S. Northeast passive acoustic sensing network for monitoring, reducing threats and the conservation of marine animals. Mar. Technol. Soc. J. 49, 70–86. doi: 10.4031/MTSJ.49.2.16
Van Parijs, S. M., Clark, C. W., Sousa-Lima, R. S., Parks, S. E., Rankin, S., Risch, D., et al. (2009). Management and research applications of real-time and archival passive acoustic sensors over varying temporal and spatial scales. Mar. Ecol. Progr. Ser. 395, 21–36. doi: 10.3354/meps08123
Van Waerebeek, K., Santillán, L., and Suazo, E. (2009). On the native status of the southern right whale Eubalaena australis in Peru. Bol. Museo Nacional Hist. Nat. 58, 75–82.
Vanderlaan, A. S. M. (2010). Estimating Risk to the North Atlantic Right Whale (Eubalaena glacialis) from Ocean-going Vessels and Fishing Gear. Ph.D. thesis, Dalhousie University, Halifax.
Vernazzani, B. G., Cabrera, E., and Brownell, R. Jr. (2014). Eastern South Pacific southern right whale photo-identification catalog reveals behavior and habitat use patterns. Mar. Mamm. Sci. 30, 389–398. doi: 10.1111/mms.12030
Wada, S. (1973). The ninth memorandum on the stock assessment of whales in the North Pacific. Int. Whaing Comm. 23, 164–169.
Wade, P., Heide-Jorgensen, M. P., Shelden, K., Barlow, J., Carretta, J., Durban, J., et al. (2006). Acoustic detection and satellite-tracking leads to discovery of rare concentration of endangered North Pacific right whales. Biol. Lett. 2, 417–419. doi: 10.1098/rsbl.2006.0460
Wade, P. R., De Robertis, A., Hough, K. R., Booth, R., Kennedy, A., LeDuc, R. G., et al. (2011a). Rare detections of North Pacific right whales in the Gulf of Alaska, with observations of their potential prey. Endanger. Species Res. 13, 99–109. doi: 10.3354/esr00324
Wade, P. R., Kennedy, A., LeDuc, R., Barlow, J., Carretta, J., Shelden, K., et al. (2011b). The world’s smallest whale population? Biol. Lett. 7, 83–85. doi: 10.1098/rsbl.2010.0477
Waite, J. M., Wynne, K., and Mellinger, D. K. (2003). Documented sighting of a North Pacific right whale in the Gulf of Alaska and post-sighting acoustic monitoring. Northwestern Nat. 84, 38–43. doi: 10.2307/3536721
Waldick, R., Kraus, S., Brown, M., and White, B. (2002). Evaluating the effects of historic bottleneck events: an assessment of microsatellite variability in the endangered, North Atlantic right whale. Mol. Ecol. 11, 2241–2249. doi: 10.1046/j.1365-294X.2002.01605.x
Webster, T. A., Dawson, S. M., Rayment, W. J., Parks, S. E., and Van Parijs, S. M. (2016). Quantitative analysis of the acoustic repertoire of southern right whales in New Zealand. J. Acoust. Soc. Am. 140, 322–333. doi: 10.1121/1.4955066
Whitehead, H. (2001). Analysis of animal movement using opportunistic individual identifications: application to sperm whales. Ecology 82, 1417–1432. doi: 10.1890/0012-9658(2001)082[1417:AOAMUO]2.0.CO;2
Whitehead, H. (2007). Learning, climate and the evolution of cultural capacity. J. Theor. Biol. 245, 341–350. doi: 10.1016/j.jtbi.2006.10.001
Whitehead, H. (2010). Conserving and managing animals that learn socially and share cultures. Learn. Behav. 38, 329–336. doi: 10.3758/LB.38.3.329
Whitehead, H., and Payne, R. (1978). New techniques for assessing populations of right whales without killing them. Mamm. Seas Rep. 3:189.
Wiley, D., Hatch, L., Thompson, M., Schwehr, K., and MacDonald, C. (2013). Marine sanctuaries and marine planning: protecting endangered marine life. Proc. Mar. Safety Secur. Council 70, 10–15.
Winn, H. E., Goodyear, J. D., Kenney, R. D., and Petricig, R. O. (1995). Dive patterns of tagged right whales in the Great South Channel. Cont. Shelf Res. 15, 593–611. doi: 10.1016/0278-4343(94)00061-Q
Winn, H. E., Price, C. A., and Sorensen, P. W. (1986). The distributional biology of the right whale (Eubalaena glacialis) in the Western North Atlantic. Rep. Int. Whaling Comm. 10, 129–138.
Wishner, K. F., Schoenherr, J. R., Beardsley, R., and Chen, C. (1995). Abundance, distribution and population structure of the copepod Calanus finmarchicus in a springtime right whale feeding area in the southwestern Gulf of Maine. Cont. Shelf Res. 15, 475–507. doi: 10.1016/0278-4343(94)00057-T
Wright, D., Crance, J., Woodrich, D., Brewer, A., and Berchok, C. L. (2017). Contemporary distribution of the eastern North Pacific right whale in the Bering Sea. J. Acoust. Soc. Am. 142, 2503–2503. doi: 10.1121/1.5014139
Wright, D. L. (2015). Simultaneous Identification of Four Mysticete Species in the Bering Sea Using Passive Acoustic Monitoring Increases Confidence in Acoustic Identification of the Critically Endangered North Pacific Right Whale (Eubalaena japonica) Final Report to the International Fund for Animal Welfare. Silver Spring, MD: NOAA, 63.
Wright, D. L. (2016). Passive Acoustic Monitoring of the Critically Endangered Eastern North Pacific Right Whale (Eubalaena japonica). Bethesda, MD: Marine Mammal Commission. 56.
Wright, D. L., Berchok, C. L., Crance, J. L., and Clapham, P. J. (2018). Acoustic detection of the critically endangered North Pacific right whale in the northern Bering Sea. Mar. Mamm. Sci. 35, 311–326. doi: 10.1111/mms.12521
Zakardjian, B. A. (2003). Effects of temperature and circulation on the population dynamics of Calanus finmarchicus in the Gulf of St. Lawrence and Scotian Shelf: study with a coupled, three-dimensional hydrodynamic, stage-based life history model. J. Geophys. Res. 108, 8016–8016. doi: 10.1029/2002JC001410
Zerbini, A. N., Baumgartner, M. F., Kennedy, A. S., Rone, B. K., Wade, P. R., and Clapham, P. J. (2015). Space use patterns of the endangered North Pacific right whale Eubalaena japonica in the Bering Sea. Mar. Ecol. Prog. Ser. 532, 269–281. doi: 10.3354/meps11366
Keywords: right whale, conservation, cumulative effects analysis, conservation technology, threats, recovery
Citation: Harcourt R, van der Hoop J, Kraus S and Carroll EL (2019) Future Directions in Eubalaena spp.: Comparative Research to Inform Conservation. Front. Mar. Sci. 5:530. doi: 10.3389/fmars.2018.00530
Received: 04 August 2018; Accepted: 31 December 2018;
Published: 30 January 2019.
Edited by:
Jeremy Kiszka, Florida International University, United StatesReviewed by:
Robert L. Brownell, Southwest Fisheries Science Center (NOAA), United StatesDaniel M. Palacios, Oregon State University, United States
Copyright © 2019 Harcourt, van der Hoop, Kraus and Carroll. This is an open-access article distributed under the terms of the Creative Commons Attribution License (CC BY). The use, distribution or reproduction in other forums is permitted, provided the original author(s) and the copyright owner(s) are credited and that the original publication in this journal is cited, in accordance with accepted academic practice. No use, distribution or reproduction is permitted which does not comply with these terms.
*Correspondence: Rob Harcourt, cm9iZXJ0LmhhcmNvdXJ0QG1xLmVkdS5hdQ==