- 1Department of Ocean Sciences, Rosenstiel School of Marine and Atmospheric Sciences, University of Miami, Miami, FL, United States
- 2Instituto de Oceanografia, Universidade Federal do Rio Grande (FURG), Rio Grande, Brazil
The spatial distribution and species identification of Trichodesmium was assessed during two fall cruises along the Southwest Atlantic Ocean shelf break. Organisms from the microplankton >50 μm were collected using a vertical plankton net for quantification and identification of the microplanktonic community associated with the genus. Additional sub-samples were filtered and prepared for quantification and discrimination of phycotoxins from the particulate matter using High Performance Liquid Chromatography. Physical parameters such as temperature, salinity, wind speed, and mixed layer depth were used in order to evaluate the environmental conditions at the time of sampling and correlate with Trichodesmium occurrence. Overall, Trichodesmium abundances were higher in the northernmost stations under wind speeds of <8 knots and shallow mixed layer depths <40 m. Besides frequent reports on the occurrence of T. erythraeum and T. thiebautii for this region, we identified three species as T. clevei, T. hildebrandtii, and T. radians. In the majority of stations where Trichodesmium was not the dominant organism, other microplanktonic groups were present such as centric diatoms and dinoflagellates. The toxin analysis was positive for saxitoxins predominantly when Trichodesmium was at high numbers of trichomes per liter in the stations; however, there was an inverse relationship between abundance of trichomes and toxin concentrations. Using information from the environmental variables and Trichodesmium abundance, we suggest that the toxin production might take place during the aggregation phase of trichomes at surface, and that saxitoxins could be inhibiting the growth of other microplanktonic organisms.
Introduction
The marine cyanobacteria Trichodesmium (Ehrenberg, 1830) form colonies that aggregate in the water surface as visible patches in tropical and subtropical oceans. The high abundance of trichomes in the upper water layer is often associated with environmental factors such as hot and calm weather and the presence of a shallow mixed layer (Karl et al., 2002; Agawin et al., 2013). The global occurrence of Trichodesmium is of undoubted importance to biogeochemical cycles, especially nitrogen and carbon (Capone et al., 1997; Westberry and Siegel, 2006); however, the majority of studies involving Trichodesmium are restricted to the North Atlantic and North Pacific Subtropical Gyres (Villareal and Carpenter, 2003; LaRoche and Breithbarth, 2005), and the genus is often under-studied in other oceans. More recent reports of Trichodesmium in the Andaman Sea (Arun Kumar et al., 2012), coast of India (Srinivas and Sarin, 2013) and Southwest Atlantic Ocean (Silva, 2005; Silva et al., 2008; Detoni et al., 2016a,b; Bif and Yunes, 2017) suggest they are important members of the microbial community composition in those regions.
In terms of ecological role, Trichodesmium is often reported in co-occurrence with other members of phytoplankton and zooplankton. The symbionts Rhizosolenia hebetata and Richelia intracellularis, for example, were present with dense aggregations of Trichodesmium (Madhu et al., 2013). The association between the harpacticoid Macrosetella gracilis and Trichodesmium colonies are thought to benefit the zooplankton, since trichomes were reported containing eggs and larvae attached, and served as food and substrate (Björnberg, 1965; O'Neil et al., 1996). Understanding the microbial community structure surrounding Trichodesmium aggregations is therefore an important key to understand its ecology and species interactions, especially in understudied regions such as the South Atlantic Ocean where the genus is often reported (Gianesella-Galvão, 1995; Rörig et al., 1998; Carvalho et al., 2008; Monteiro et al., 2010, 2012). Recent works from our group have identified colonies in the Southwest Atlantic Ocean associated with iron-rich dust particles (Bif and Yunes, 2017) and producing toxins (Detoni et al., 2016a); however, the association of these potentially toxic aggregations with other microbial communities was never assessed. Previous works associated the occurrence of large aggregations of Trichodesmium (T. erythraeum and T. thiebautii) with anoxia-related mortality of fish and crabs in India (D'Silva et al., 2012 and references therein) and bryozoans in the South Brazilian coast (Silva, 2005); however, no toxin analysis was performed at that time (Silva et al., 2008). Given the reports of Trichodesmium aggregations along the Brazilian coast (Silva et al., 2008 and references therein), our work aims to investigate their distribution, morphological taxonomy, association with microplanktonic groups and the potential toxicity to these organisms.
Materials and Methods
Study Area and Analysis of Physical Parameters
This study was carried out in the area encompassing the Brazilian shelf slope on board the R/V Atlântico Sul with TALUDE project (Table 1). Samples were collected between 24° and 35°S (Figure 1) during two consecutive fall cruises, the first between June 4th and 11th of 2013 and the second between May 10th and 20th of 2014. Vertical profiles of temperature and salinity were obtained using a CTD sensor (911 SeaBird®). The surface water masses were classified based on the thermohaline intervals described by Möller et al. (2008). The mixed layer depth (MLD) was determined from vertical density profiles (∂ρ/∂z), excluding the first 5 meters of depth due to noise data. Wind speed and direction were obtained from an anemometer mounted on the ship's foremast ~18 m above the sea surface. Both sea surface temperature (SST) and salinity (SSS) were then averaged for the upper 30 m of water depth in order to correlate with other environmental parameters, especially community composition.
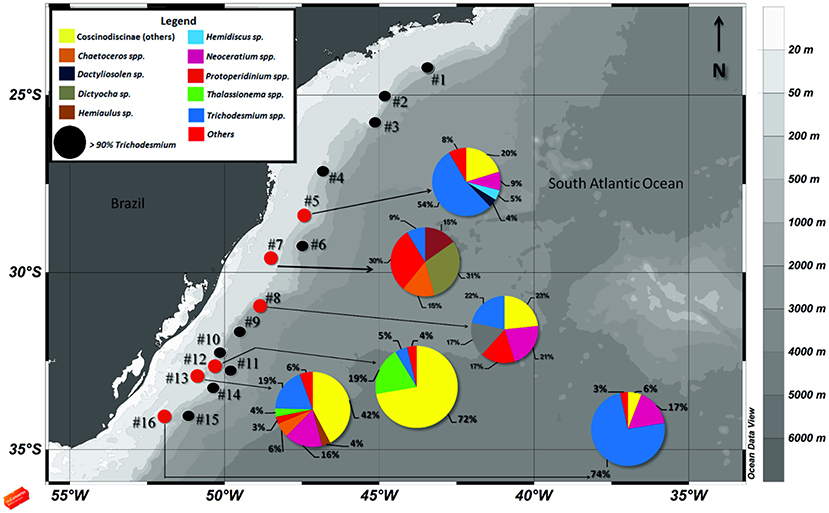
Figure 1. Map of the study area showing the sampling stations along the South Atlantic shelf slope. Colored legend shows the main microplankton species matching with pie charts sections. Stations shown as black circles denote sites where Trichodesmium spp. contributed >90% to the total microplankton cell densities, and small red circles represent stations where Trichodesmium spp. contributed <90% to the total density.
Analysis of Biological Samples
Samples were collected during two different conditions: areas of high density aggregations of Trichodesmium; and in the absence of visible surface aggregations. The sampling efforts were carried out during calm seas and clear skies. During the 2013 cruise we sampled the northernmost section (stations 1 to 4; Figure 1) and focused solely in high density of trichomes. The 2014 cruise was carried out in the southernmost stations and sampling was performed during both presence and absence of trichomes.
The organisms were collected by vertical hauls using conical plankton net with 30 cm mouth diameter and 50 μm mesh size, down to 30 m depth of the water column. These samples were preserved in 4% buffered formalin. Trichodesmium species were identified following Komárek and Anagnostidis (2005) and quantified as number of trichomes per liter. For the description of each species of Trichodesmium, it was necessary to distinguish among cell, trichome (cells filaments) and colony (clusters of trichomes organized as puffs or tufts) (Anagnostidis and Komárek, 1988). As far as possible, co-occurring microplankton (>50 μm) were also identified and counted (minimum 100 cells per group) using an inverted microscope (ZeissAxiovert A1) coupled with a camera (Axio Cam MRc) using the sedimentation chamber technique (Utermöhl, 1958). Samples were counted at ×200, ×400, and ×1000 of magnification and the species were identified according to specific literature for each group i.e., diatoms (Hasle and Syvertsen, 1996) and dinoflagellates (Steindinger, 1996).
Since Trichodesmium spp. trichomes were very concentrated in many samples, it was not possible to estimate their abundance per species; instead, the total abundance was estimated by the sum of free trichomes plus number of colonies ×200, as the average of trichomes on each colony (Carpenter, 1983). Total abundance of Trichodesmium and other taxa were, then, divided by the 30-m of water column × 0.03-m of mouth diameter of the plankton net, so the final abundance is shown as trichomes (Trichodesmium spp.) or cells (other microplankton, excluding Trichodesmium spp.) per liter.
Analysis of Toxins
Samples from 6 different stations were collected from visible aggregations of Trichodesmium during December-2014, at the same region where biological samples took place. Using a GF/F glass fiber filter of 0.7 μm pore size, the water was filtered until the filter was clogged (60–470 mL of sample), washed with distilled water and maintained at −80°C until chemical analysis. We analyzed two kinds of toxins using High Performance Liquid Chromatography (HPLC UV-DAD): Domoic Acid (DA) (Miguez et al., 1996; Costa et al., 2003) and saxitoxins (STX), (Rourke et al., 2008). The collection of samples and analysis of STX were reported in detail (Detoni et al., 2016b), and the same samples were used for the analysis of domoic acid. The abundance of Trichodesmium was correlated with STX concentrations at the stations. We then calculated the STX:trichome ratio, and reported STX as equivalent saxitoxin concentrations (STX-eq) μg L−1 that is, the sum of all the variants present in the analyzed sample.
Statistics
A series of multivariate analyses were conducted in order to verify any spatial pattern in the species distribution, and to verify the relationship between environmental factors (i.e., temperature, water masses, MLD) and the presence of the different taxonomic groups of microphytoplankton.
A non-metric multidimensional scaling (nMDS) was applied together with a dissimilarity matrix based on the non-metric Bray-Curtis index (Bray and Curtis, 1957) in order to group the taxa using an ordination diagram (Wickelmaier, 2003). An analysis of similarities (ANOSIM) was then applied in order to verify possible differences among the stations (Clarke, 1993). The analyses were carried out using the free software Past (v.1.81) (Hammer et al., 2008).
The Canonical Correspondence Analysis (CCA) was performed in order to identify patterns and variabilities of microplankton species with respect to environmental variables (TerBraak and Prentice, 1988). Biotic variables were represented by total abundances of Trichodesmium and other microplankton taxa. Environmental variables included: sea surface temperature; salinity; MLD; wind speed; latitude; and water mass. That latter variable was ascribed as follows: Tropical Water (TW) = 1, Subtropical Shelf Water (STSW) = 2, and Plata Plume Water (PPW) = 3, using latitude as a covariate. All variables were log-transformed before analysis in order to normalize the sets of variables. To test for the CCA significance, we run Monte-Carlo tests based on 499 permutations under a reduced model (p < 0.05). Three stations (#1, #15, and #16) were excluded from the final CCA analysis because they represented very extreme conditions in comparison to others.
Results
Physical Conditions
Considering the physical conditions during the two fall cruises (Table 1), the Tropical Water (TW, Sal. ≥ 36 and Temp. >22.3°C) mainly influenced the region. The Sub-Tropical Shelf Water (STSW, 33.5 < Sal. < 35.5 and Temp. ~20.7°C) was the dominant surface water mass at stations #8 and #10, while the Plata Plume Water (PPW, Sal < 33.5 and Temp ≤ 20.9°C) was noticeable at stations #13 and #16 (Möller et al., 2008). The wind direction varied among stations, but was dominated by Southerly or Westerly directions. Stations under the influence of relatively high wind speed >10 knots were always related to a non-dominant Trichodesmium spp. in the total abundance of trichomes (Figure 1; Table 1). MLD was also variable among stations, from 14 m down to 80 m of depth, and was not correlated to the local wind speed (Table 2).
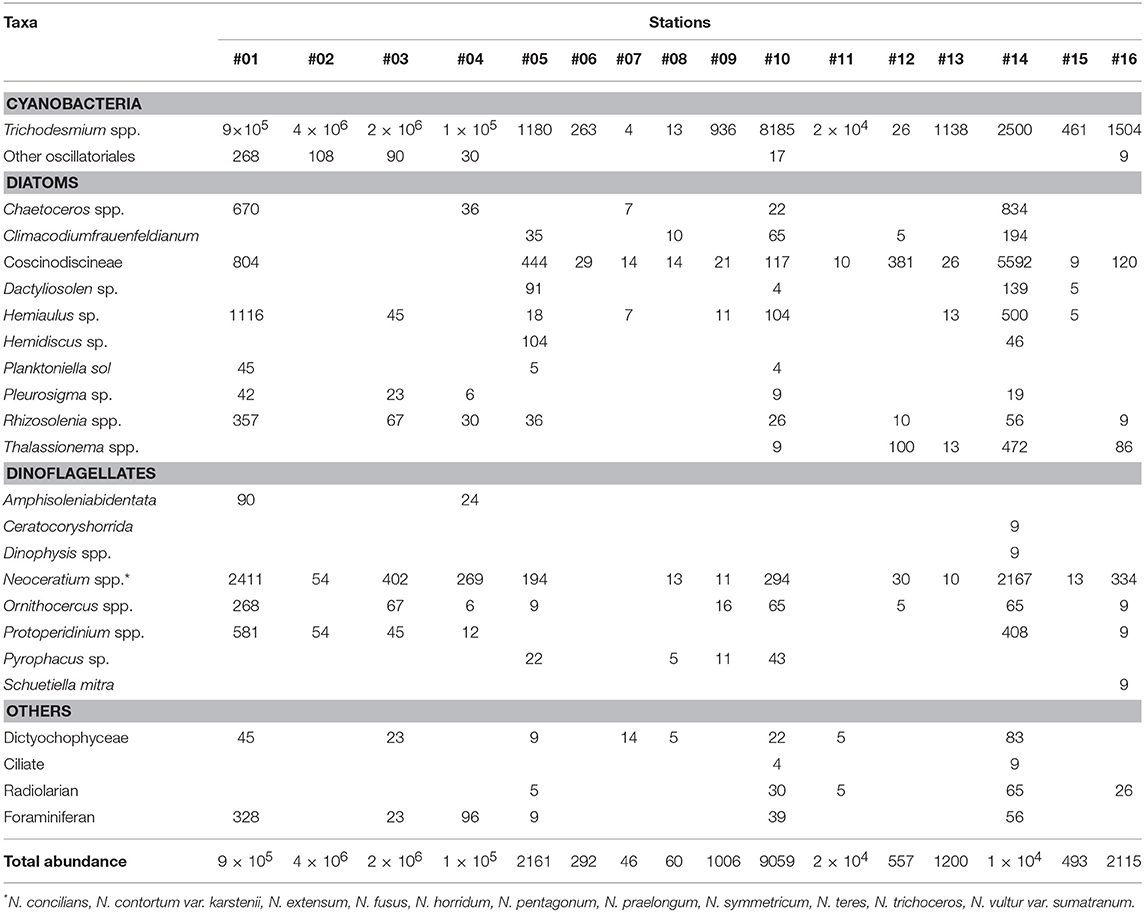
Table 2. Abundance of microplankton groups (trichomes L−1 for Trichodesmium spp. and cells L−1 for others).
Microplankton Identification and Spatial Distribution
Figure 1 compiles the map of the study area with the relative contribution of specific microplanktonic groups to the total abundance of organisms at each station. The results show that Trichodesmium spp. were the greatest contributors for both fall cruises, comprising more than >90% of total abundance in many stations. In a few southernmost stations, the diatoms from Coscinodiscineae group and Thalassionema spp., as well as the dinoflagellates Neoceratium spp. and Protoperidinium spp. co-occurred with the cyanobacterial trichomes at elevated cell numbers (Figure 1). Stations #2 and #3 had the most elevated concentrations of trichomes at surface: 2.1 × 106 and 4.4 × 106 trichomes L−1, respectively. These two stations and other four (#1, #4, #10, and #11) had trichome abundances >8 × 103 trichomes L−1. At these stations, the accumulation of Trichodesmium at surface was visible to the naked eye. In contrast, aggregations were not visible in the remaining stations, where abundances ranged from only ~4 trichomes L−1 to 2.5 × 103 trichomes L−1 (Table 2).
Our statistical analysis separated the stations where Trichodesmium was more abundant from those where their abundance was fairly comparable to the abundance of other organisms. The ordination diagram derived from the nMDS analysis defined the group A as composed by stations with > 8,000 trichomes L−1 and a group B with those with densities <2,500 trichomes L−1 (Figure 2). These two groups were significantly different (ANOSIM, r2 = 0.89, p < 0.0001) and, interestingly, separate visible aggregations from those undetected to the naked eye.
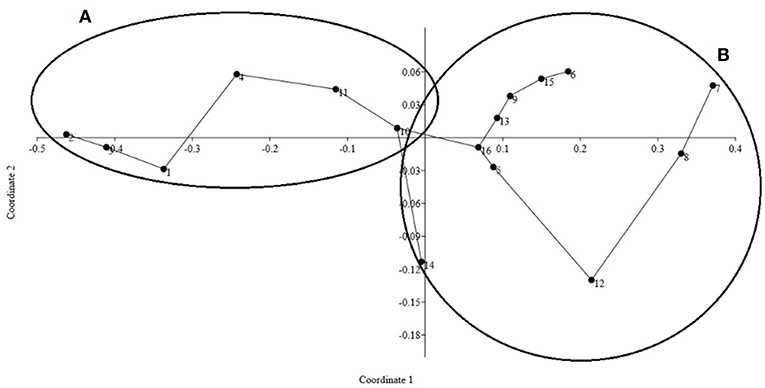
Figure 2. nMDS ordination diagram dividing the stations into two distinctive groups according to Trichodesmium abundance of trichomes per liter. Group (A) where the stations presented > 8,000 trichomes L−1 and Group (B) contains stations with <2,500 trichomes L−1. The ANOSIM test revealed statistical differences between the two groups: p < 0.01, R2 = 0.58 and Stress = 0.04 (<0.5).
In the stations where Trichodesmium spp. contributed <90% for the total abundance of organisms, the species composition varied between different groups of microplankton (Figure 1; Table 2). Stations #14 (18 taxa), #10 (17 taxa) #1, and #5 (13 taxa each) had a relatively high diversity and densities, while #6, #8, #12, and #15 had concentrations of <500 cells L−1 (Table 2). In considering the main microplanktonic organisms present in the area, the centric diatoms from the sub-order Coscinodiscineae, dinoflagellates Neoceratium spp. and silicoflagellates (Class Dictyochophyceae) were fairly common across the region and in relatively high abundance (Table 2). Interestingly, the harpacticoid copepod Macrosetella gracilis was present along most of the stations with eggs and larvae attached to the Trichodesmium trichomes (Figure 3a). In the Southernmost stations, an unidentified pteropod mollusk appeared in high abundances (data not shown). Symbioses between microplanktonic organisms and N2- fixers cyanobacteria were pretty common along the stations such as with Foraminifera members and the diatoms Rhizosolenia sp. and Chaetoceros sp. (Figures 3b–d).
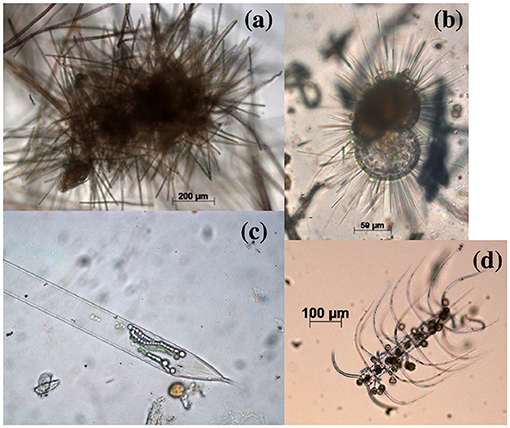
Figure 3. Members of microplankton in association with other organisms found in the stations. Colonies of Trichodesmium with juveniles of Macrosetella gracilis (a); foraminifera in association with picocyanobacteria (b); symbiosis between Rhyzosolenia sp. and Richelia sp. (c); and associations between a chain of Chaetoceros sp. and picocyanobacteria (d).
The CCA analysis coupled with a Monte-Carlo test of the F-ratio associated the presence of microplankton groups correlated with environmental variables (Figure 4). Results indicated that five environmental variables (temperature, salinity, MLD, wind speed and water mass types) and the covariate latitude influenced significantly the spatial distribution of the different groups of microplankton (p < 0.01). In fact, environmental variability explained 44% of the community composition, and the first two significant canonical roots cumulatively explained 71.5% of the observed variance in taxa.
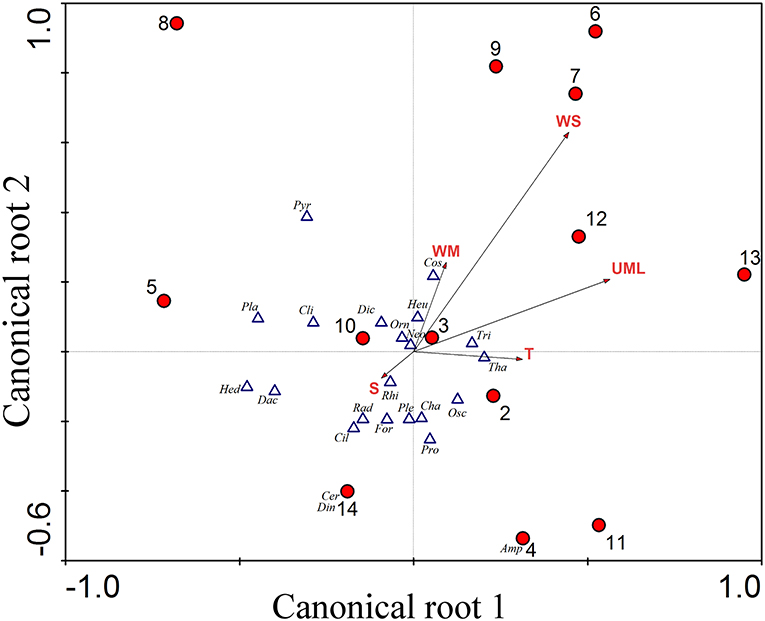
Figure 4. Canonical Correspondence Analysis showing the relationship between the abiotic variables and density of organisms. The first two canonical roots represent 71.5% of microplankton groups vs. environment relationships (1st root explains 40.3% and 2nd root explains 31.2%). The red circles represent the stations, numbered from 1 to 16. For the abiotic variables, represented by arrows: MLD, Upper Mixed Layer; WS, Wind Speed; WM, Water Mass; S, Salinity; T, Temperature. For the taxa, represented by triangles: Tri, Trichodesmium spp, Osc, Other Oscillatoriales; Dia, Diatoms; Cha, Chaetocerosspp; Cli, Climacodiumfrauenfeldianum; Cos, Coscinodiscineae; Dac, Dactyliosolen sp; Heu, Hemiaulus sp; Hed, Hemidiscus sp; Pla, Planktoniella sol; Ple, Pleurosigma sp; Rhi, Rhizosolenia spp; Tha, Thalassionema spp; Amp, Amphisoleniabidentata; Cer, Ceratocoryshorrida; Din, Dinophysis spp; Neo, Neoceratium spp; Orn, Ornithocercus spp; Pro, Protoperidinium spp; Pyr, Pyrophacus sp; Sch, Schuetiellamitra; Dic, Dictyochophyceae; Cil, Ciliate; Rad, Radiolarian; For, Foraminiferan.
Taxonomy of Trichodesmium Based on Morphological Features
We identified 5 different species of Trichodesmium (Anagnostidis and Komárek, 1988; Komárek and Anagnostidis, 2005), which were mainly based on biometric parameters (length and width of cells within trichomes), as well as phenotypic features (presence/absence of calyptra and shape of apical cell). The morphologically different forms generally co-occurred at the different stations; however, we did not differentiate the species composition for each station (Figures 5, 6).
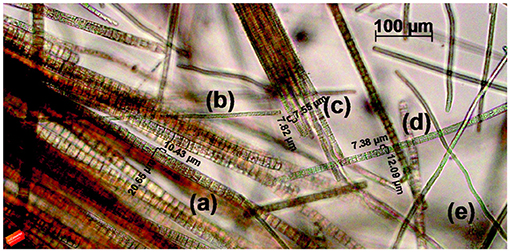
Figure 5. Five species of Trichodesmium co-occurring in a sample (×200 of magnification): T. hildebrandtii (a), T. clevei (b), T. thiebautii (c), T. erythraeum (d), and T. radians (e).
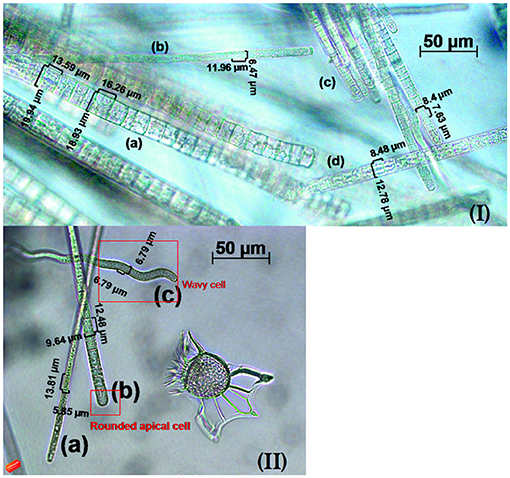
Figure 6. Details of Trichodesmium trichomes co-occuring in the samples (×400 of magnification). In the first image (I) T. hildebrandtii (a), T. clevei (b), T. thiebautii (c), and T. erythraeum (d); in the second image (II) T. clevei (a), a rounded apical cell in T. thiebautii (b) and the wavy trichome of T. radians (c).
T. clevei (J. Schmidt) (Anagnostidis and Komárek, 1988) was found as single trichomes or in colonies containing 20–30 trichomes, with cylindrical and slightly coiled cells. Most of the trichomes were blue-green, but were also found as dark reddish. The cell diameter ranged from 5.9 to 7 μm and were longer than wide, with apical cell rounded and without calyptra. Their occurrence was already registered in Gulf of Siam and Australia, but this is the first time this species is described in South Atlantic waters. T. erythraeum (Ehrenberg ex Gomont) was found as both free-floating or in colonies as purple-red trichomes, constricted at the cross-walls that were gradually attenuated at the ends. Cells were 6–11 μm long, with the apical cell containing a distinctive calyptra. The species is documented as forming dense aggregations in tropical and subtropical oceans, including the Atlantic, and was already described in the present region (Rörig et al., 1998; Siqueira et al., 2006; Carvalho et al., 2008). T. hildebrandtii (Gomont) was easily distinguished among the other species because of the diameter ranging from 13 to 22 μm with significantly shorter cells. It was found as single trichomes or in colonies with a dark-reddish color, had attenuated cells at the ends and apical cells containing a calyptra. The species can be found in warm seas all over the world, but has not been previously described in South Atlantic Ocean. T. radians (Wille) (Golubié) had the most distinctive morphology, characterized by wavy trichomes that were either free or aggregated as star-shaped colonies (Figure 6). The cells were always blue-green with large gas vesicles and a cell diameter range of 6–9 μm, longer than wide. Apical cells were rounded and without calyptra. This specie is largely distributed among the oceans, and this is the first report for the South Atlantic waters. T. thiebautii (Gomont ex Gomont) was found as single trichomes or colonies that were partly rope-like contorted or radially-arranged. Cells were nearly isodiametric, not constricted at the cell walls, with rounded apical cells. This species has a wide distribution range and was previously found offshore the Brazilian coast (Björnberg, 1965).
Toxicity of Trichodesmium
Our toxin analysis were negative for domoic acid and positive for STX concentrations in all samples analyzed (Figure 7). There was an inverse association between density of trichomes and STX concentrations. At the same time, Trichodesmium was dominant in terms of abundance for these stations (Figure 1). In relation to STX concentration, concentrations ranged from 0.45 to ~4 (STX-eq) μg L−1, with the qualified and quantified variants as gonyautoxins (GTX), specifically GTX-4 and GTX-2. The GTX-4 was the main variant contributing for total STX (STX-eq) toxicity.
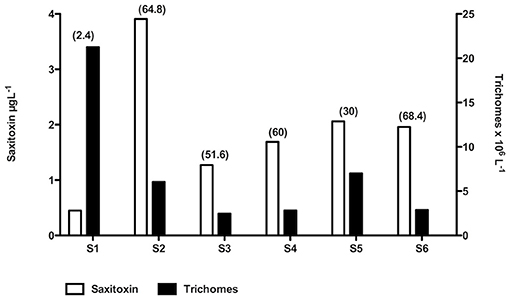
Figure 7. Relationship between aggregations shown as density of trichomes per liter, and Saxitoxin concentrations in μg L−1. The concentration of STX per trichome is shown in parenthesis as μg SXT per trichome−1 × 10 −8. Aggregations are in order of stations; to a better comparison per density of trichomes: S1 > S5 > S2 > S4 > S6 > S3.
Discussion
The aggregations of Trichodesmium were observed during clear skies, calm seas and when wind speed was relatively low <10 knots; such conditions were reported elsewhere as suitable for trichome aggregations (Villareal and Carpenter, 2003; LaRoche and Breithbarth, 2005; Agawin et al., 2013), and this relationship is confirmed by statistical analysis (Figure 4). A noticeable feature observed at station 2 is that the aggregation was accompanied by floating objects such as feathers, wood and plastic. This suggests the objects and organisms were concentrating at the convergence region of a Langmuir cells, in agreement with previous observations elsewhere (Evans and Taylor, 1980). A shallow MLD of 17 m depth kept the trichome aggregations at surface, facilitated by gas vesicles inside the cells that give a positive buoyance to the trichomes (Capone et al., 1997; Villareal and Carpenter, 2003). Moreover, Since the genus is well-known to have very low growth rates ~0.14 d−1 (LaRoche and Breithbarth, 2005), it was unlikely that trichomes formed blooms, such as harmful algal blooms (HABs).
Intermediate densities of Trichodesmium ~8 × 103 trichomes L−1 (some stations from group B, Figure 2) could represent a transient condition before the mixed layer depth (MLD) stabilization and associated with wind speeds <8 knots, suitable for Trichodesmium aggregation and colony formation. On the other hand, the deepening of MLD might dilute Trichodesmium aggregations; in this case, our vertical net hauls of 30 m deep would not account for these organisms. The importance of MLD on the community structure was confirmed in the CCA's ordination diagram (Figure 4).
In stations where Trichodesmium was present in high densities, we found low densities of microphytoplankton co-occurring (Table 2; Figure 1). Inorganic nutrient concentrations through our study area, however, were present at high concentrations (Bif and Yunes, 2017) and do not support such low densities of microphytoplankton. From these organisms, typical representatives of open oceans were found at the stations, such as the dinoflagellates Neoceratium spp., Dinophysis spp. and Ornithocercus spp., and the heterotroph Protoperidinium spp. These genera have been classified as the major consumers of phytoplankton biomass, where Protoperidinium spp. is especially important in open oceans (Sherr and Sherr, 2007). Two genera were found in symbiosis with picocyanobacteria, as the case of Ornithocercus spp. and Rhizosolenia spp.; such associations were previously seen in other oceans (Madhu et al., 2013). The co-occurrence of T. hildebrandtii with other microplankton organisms was already registered in the southern Brazilian coast (Guimarães and Rörig, 1997), but were never detailed.
The presence of STX, thus, is another relevant factor that should possibly control the distribution of microplankton groups across stations. Based on the relationship between trichome densities and toxin production, we found STX (STX-eq) associated with the presence of Trichodesmium (Figure 7). Higher STX (STX-eq) concentrations were correlated with lower trichomes per liter; thus, we suggest that Trichodesmium produces the toxin during the early stage of trichome aggregation. STX are allelochemical compounds produced as secondary metabolites which are not required for metabolism (i.e., growth, development and reproduction), but are considered an important defense against herbivory (Fraenkel, 1959; Stamp, 2003). Our work suggests that STX could be play a central role during initial aggregations of Trichodesmium in order to minimize bottom-up (i.e., competition for nutrients) and top-down (i.e., herbivory) controls (Smayda, 1997). This is corroborated by the fact that other organisms were less abundant in the presence of Trichodesmium, but were dominant in regions where trichomes were not accumulating or forming colonies (Figure 1). Although we identified other potential toxin producers (i.e., STX and domoic acid) in the stations such as Gonyaulax spinifera, Protoceratium reticulatum, Chaetoceros spp., and Pseudo-nitzschia seriata (Table 3), they were not abundant enough to be responsible for the high toxin concentrations.
Despite the solely morphological/traditional identification of Trichodesmium performed in our work, previous studies already linked the traditional taxonomy with molecular approaches and found high aggreement between techniques. Some approaches include 16S rRNA gene sequencing (Komárek, 2010), and genetic and biochemical informations that add to the development of a functional classification scheme (Hynes et al., 2012). A detailed description of Trichodesmium spp. species was never assessed for the Southwest Atlantic Ocean (Silva et al., 2008 and references therein), so the identification of three new species for the region (T. clevei, T. hildebrandtii, and T. radians) adds to the previous reports of T. erythraeum (e.g., Barbosa, 1944; Gianesella-Galvão, 1995; Rörig et al., 1998) and T. thiebautii (Björnberg, 1965). Nevertheless, we suggest that further local studies based on the polyphasic approach (i.e., using morphological, ultra-structural, biochemical and genetic features) are still necessary (Komárek et al., 2014).
From the five species of Trichodesmium found in the region, T. erythraeum and T. thiebautii are already known to be toxic to the environment. In ecological tests performed with both species collected and isolated from Caribbean waters, T. thiebautii was highly toxic for Artemia salina and copepods, and was neurotoxic to mice and some non-grazing copepods (Hawser and Codd, 1992; Hawser et al., 1992). T. erythraeum toxicity was assessed in populations from the Northeast of Brazil through HPLC, Elisa and ecotoxicological tests, and was positive for STX and microcystins (Proença et al., 2009). In this same region, the specie was found in co-occurrence with toxic dinoflagellates; but analagous to our study, the organisms were present in small abundances. The toxigenic potential of T. erythraeum was recently validated through brine shrimp toxicity assay, showing 100% of mortality after 48 h of incubations using aggregations from the Gulf of Mannar (Shunmugam et al., 2017). The study also demonstrated that T. erythraeum is able to synthesize other potent multi-class neurotoxins such as anatoxin-a and gonyautoxin, and microcystins. The toxicity of other Trichodesmium species, however, requires further investigation, and the Southwest Atlantic seems to be a good candidate to perform similar studies, given the diversity of Trichodesmium species and high abundance along the shelf break.
Summary
The Southwest Atlantic Ocean shows suitable conditions for growth and colony formation of Trichodesmium spp. Five species of Trichodesmium (T. clevei, T. erythraeum, T. hildebrandtii, T. radians, and T. thiebautii) were identified, and three out five reported for the first time at the region. During non-Trichodesmium-dominated assemblages in the water surface, dinoflagellates and relatively large diatoms were the dominant groups. Our results on microplankton densities in the stations combined with toxin analysis revealed that STX was likely produced by Trichodesmium aggregations. Higher concentrations of toxins correlated with moderate numbers of trichomes per liter; lower concentrations were present during high densities of the genus. The lack of microplankton diversity during Trichodesmium dominance might be associated with the toxin production, which seems to be especially important during the early aggregation phase at the surface.
Author Contributions
MB developed the research, collected, and analyzed the biological and wrote the article as a result of her Master's Thesis. MdS helped with plankton identification and calculations. LC analyzed the toxins. JY helped with data interpretation. All authors revised and made contributions to methodology, results and discussion in this manuscript.
Funding
MB was funded by a Masters scholarship granted from CAPES. MdS and LC were funded by Post-Doc fellowship grants from CAPES. JY was funded by a productivity grant from CNPq. This study was partially funded by CNPq (Grant # 610012/2011-8) through the Brazilian National Institute of Science and Technology (INCT-Mar-COI).
Conflict of Interest Statement
The authors declare that the research was conducted in the absence of any commercial or financial relationships that could be construed as a potential conflict of interest.
Acknowledgments
The authors want to thank Talude project, granted by Chevron®, for the opportunity to collect samples during their cruise.
References
Agawin, N. S., Tovar-Sánchez, A., de Zarruk, K. K., Duarte, C. M., and Agustí, S. (2013). Variability in the abundance of Trichodesmium and nitrogen fixation activities in the subtropical NE Atlantic. J. Plankton Res. 35, 1126–1140. doi: 10.1093/plankt/fbt059
Anagnostidis, K., and Komárek, J. (1988). Modern approach to the classification system of cyanophytes. 3. Oscillatoriales. Arch. Hydrobiol. Suppl. 80, 327–472.
Arun Kumar, M., Karthik, R., Sai Elangovan, S., and Padmavati, G. (2012). Occurrence of Trichodesmium erythraeum bloom in the coastal waters of South Andaman. Int. J. Curr. Res. 4, 281–284.
Barbosa, F. A. S. (1944). O “Tingui—febre de Tamandaré—uma doença desconhecida. Rev. Bras. Med. 1, 663–665.
Bif, M. B., and Yunes, J. S. (2017). Distribution of the marine cyanobacteria Trichodesmium and association with iron-rich particles in the Southwest Atlantic Ocean. Aqua Microbial Ecol. 78, 107–119. doi: 10.3354/ame01810
Björnberg, T. K. S. (1965). Observations on the development and the biology of the Miraciidae Dana (Copepoda: Crustacea). Bull. Mar. Sci. 15, 512–520.
Bray, J. R., and Curtis, J. T. (1957). An ordination of the upland forest communities of Southern Wisconsin. Ecol. Monograph. 27, 325–349. doi: 10.2307/1942268
Capone, D. G., Zehr, J. P., Paerl, H. W., Bergman, B., and Carpenter, E. J. (1997). Trichodesmium, a globally significant marine cyanobacterium. Science 276, 1221–1229. doi: 10.1126/science.276.5316.1221
Carpenter, E. J. (1983). “Nitrogen fixation by marine oscillatoria (Trichodesmium) in the world's oceans,” in Nitrogen in the Marine Environment, eds E. J. Carpenter and D. G. Capone (New York, NY: Elsevier), 65–103.
Carvalho, M., Gianesella, S. M., and Saldanha-Corrêa, F. M. (2008). Trichodesmium erythraeum bloom on the continental shelf off Santos, Southeast Brazil. Braz. J. Oceanogr. 56, 307–311. doi: 10.1590/S1679-87592008000400006
Clarke, K. R. (1993). Non-parametric multivariate analyses of changes in community structure. Austr. J. Ecol. 18, 117–143. doi: 10.1111/j.1442-9993.1993.tb00438.x
Costa, P. R., Rodrigues, S. M., Botelho, M. J., and de M Sampayo, M. A. (2003). A potential vector of domoic acid: the swimming crab Polybius henslowii Leach (Decapoda brachyura). Toxicon 42, 135–141. doi: 10.1016/S0041-0101(03)00107-7
Detoni, A. M. S., Ciotti, A. M., Calil, P. H. R., Tavano, V. M., and Yunes, J. S. (2016a). Trichodesmium latitudinal distribution on the shelf break in the southwestern Atlantic Ocean during spring and autumn. Global Biogeochem. Cycl. 30, 1738–1753. doi: 10.1002/2016GB005431
Detoni, A. S., Pacheco, L. A., Costa, L. D. F., and Yunes, J. S. (2016b). Toxic Trichodesmium bloom occurrence in the southwestern South Atlantic Ocean. Toxicon 110, 51–55. doi: 10.1016/j.toxicon.2015.12.003
D'Silva, M. S., Anil, A. C., Naik, R. K., and D'Costa, P. M. (2012). Algal blooms: a perspective from the coasts of India. Nat. Hazard 63, 1225–1253. doi: 10.1007/s11069-012-0190-9
Ehrenberg, C. G. (1830). “Organisation, systematik, und geographisches verhältnis der infusionstierchen,” in Druckerei dei Koningliche Akademie der Wissenschaften, ed F. Dümmler (Berlin) 108.
Evans, G. T., and Taylor, F. J. R. (1980). Phytoplankton accumulation in Langmuir cells. Limnol. Oceanogr. 25, 840–845. doi: 10.4319/lo.1980.25.5.0840
Fraenkel, G. S. (1959). The raison d'Etre of secondary plant substances. Science 129, 1466–1470. doi: 10.1126/science.129.3361.1466
Gianesella-Galvão, S. M. F Costa, M. P. F, and Kutner, M. B. B. (1995). “Bloom of Oscillatoria (Trichodesmium) erythreae (Ehr.) Kutz,” in coastal waters of the Southwest Atlantic. (São Paulo: Publicações Especiais do Instituto Oceanográfico, USP-SP) Vol. 11, 133–140.
Guimarães, S. C. P., and Rörig, L. R. (1997). Microrganismos associados a florações da cianobactéria Trichodesmium hildebrandtii na costa de Santa Catarina. Notas Técnicas FACIMAR 1, 43–47.
Hammer, O., Harper, D. A. T., and Ryan, P. D. (2008). PAST—Palaeontological STatistics, ver. 1.81. Brief Notes. Available online at: http://folk.uio.no/ohammer/past
Hasle, G., and Syvertsen, E. (1996). “Marine diatoms,” in Identifying Marine Diatoms and Dinoflagellates, ed C. Tomas (San Diego: Academic Press), 5–385.
Hawser, S. P., and Codd, G. A. (1992). “The toxicity of Trichodesmium blooms from caribbean waters,” in Marine Pelagic Cyanobacteria: Trichodesmium and other Diazotrophs. NATO ASI Series (Series C: Mathematical and Physical Sciences), Vol. 362, 319–329 eds E. J. Carpenter, D. G. Capone, and J. G. Rueter (Dordrecht: Springer).
Hawser, S. P., O'Neil, J. M., Roman, M. R., and Codd, G. A. (1992). Toxicity of blooms of the cyanobacterium Trichodesmium to zooplankton. J. Appl. Phycol. 4, 79–86. doi: 10.1007/BF00003963
Hynes, A. M., Webb, E. A., Doney, S. C., and Waterbury, J. C. (2012). Comparison of cultured Trichodesmium (Cyanophyceae) with species characterized from the field. J. Phycol. 48, 196–210. doi: 10.1111/j.1529-8817.2011.01096.x
Karl, D., Michaels, A., Bergman, B., and Capone, D., et al. (2002). Dinitrogen fixation in the world's oceans. Biogeochemistry 57, 47–98. doi: 10.1023/A:1015798105851
Komárek, J. (2010). Modern taxonomic revision of planktic nostocacean cyanobacteria: a short review of genera. Hydrobiologia 639, 231–243. doi: 10.1007/s10750-009-0030-4
Komárek, J., and Anagnostidis, K. (2005). “Cyanoprokaryota II. Teil Oscillatoriales,”in SüBwasserflora von Mitteleuropa, eds B. Büdel, L. Krienitz, G. Gärtner, and M. Schagerl (Munchen: Elsevier Gmbh) 19:750.
Komárek, J., Kaštovský, J., Mareš, J., and Johansen, J. R. (2014). Taxonomic classification of cyanoprokaryotes (Cyanobacterial genera), using a polyphasic approach. Preslia 86, 295–335.
LaRoche, J., and Breithbarth, E. (2005). Importance of the diazotrophs as a source of new nitrogen in the ocean. J Sea Res. 53, 67–91. doi: 10.1016/j.seares.2004.05.005
Madhu, N. V., Meenu, P., Ullas, N., Ashwini, R., and Rehitha, T. V. (2013). Occurrence of cyanobacteria (Richelia intracellularis)—diatom (Rhizosolenia hebetata) consortium in the Palk Bay, southeast coast of India. Indian J. Mar. Sci. 42, 453–457.
Miguez, A., Fernandez, M. L., and Fraga, S. (1996). “First detection of domoic acid in Galicia (NW of Spain),” in Harmful and Toxic Agal Blooms, eds T. Yasumoto, Y. Oshima, and Y. Fukuyo (Paris: IOC-UNESCO), 143–145.
Möller, O. O. Jr., Piola, A. R., Freitas, A. C., and Campos, E. J. D. (2008). The effects of river discharge and seasonal winds on the shelf off southeastern South America. Continent. Shelf Res. 28, 1607–1624. doi: 10.1016/j.csr.2008.03.012
Monteiro, J. J. F., Leça, E. E., Koening, M. L., and Macedo, S. J. (2010). New record of Trichodesmium thiebautii Gomont ex Gomont (Oscillatoriales-Cyanophyta) for the continental shelf of northeast Brazil. Acta Bot. Bras. 24, 1104–1106. doi: 10.1590/S0102-33062010000400027
Monteiro, J. J. F., Leça, E. E., Koening, M. L., and Macedo, S. J. (2012). Distribution and annual variation of Trichodesmium thiebautii Gomont Ex Gomont (Oscillatoriales - cyanophyta) in tropical waters of northeastern Brazil (Western Atlantic). Trop. Oceanogr. 40, 319–326. doi: 10.5914/tropocean.v40i2.5409
O'Neil, J. M., Metzler, P. M., and Glibert, P. M. (1996). Ingestion of 15N2-1abelled Trichodesmium spp. and ammonium regeneration by the harpacticoid copepod Macrosetella gracilis. Mar. Biol. 125, 89–96.
Proença, L. A. O., Tamanaha, M. S., and Fonseca, R. S. (2009). Screening the toxicity and toxin content of blooms of the cyanobacterium Trichodesmium erythraeum (Ehrenberg) in northeast Brasil. J. Venomous Anim. Toxins Trop. Dis. 15, 204–215. doi: 10.1590/S1678-91992009000200004
Rörig, L. R., Yunes, J. S., Kurohsima, K. N., Schetinni, C. A. F., Pezzuto, P. R., and Proença, L. A. O. (1998). “Studies on the ecology and toxicity of Trichodesmium spp. blooms in southern Brazilian coastal waters,” in Harmful Algae. Proceedings of the VII International Conference on Harmful Alga, eds B. Reguera, J. Blanco, M. L. Fernández, and T. Wyatt (Vigo).
Rourke, W. A., Murphy, C. J., and Pitcher, G., van de Riet, et al. (2008). Rapid postcolumn methodology for determination of paralytic shellfish toxins in shellfish tissue. J. AOAC Int. 91, 589–597.
Sherr, E. B., and Sherr, B. F. (2007). Heterotrophic dinoflagellates: a significant component of microzooplankton biomass and major grazers of diatoms in the sea. Mar. Ecol. Prog. Ser. 352, 187–197. doi: 10.3354/meps07161
Shunmugam, S., Gayathri, M., Prasannabalaji, N., Thajuddin, N., and Muralitharan, G. (2017). Unraveling the presence of multi-class toxins from Trichodesmium bloom in the Gulf of Mannar region of the Bay of Bengal. Toxicon 135, 43–50. doi: 10.1016/j.toxicon.2017.06.003
Silva, L. M. (2005). Ocorrência de Cianobactérias No Estuário e Costa Adjacente à Lagoa dos Patos, Rio Grande, RS: Avaliação Preliminar dos Riscos a Balneabilidade. Master Dissertation. Programa de Pós-Graduação em Oceanografia Biológica (Rio Grande, TX: FURG), 144.
Silva, L. M., Matthiensen, A., and Yunes, J. S. (2008). “Florações de Trichodesmium na costa brasileira,” in Poluição Marinha, eds J. A. Baptista Neto, M. Wallner-Kersanach, S. M. Patchineelam (Rio Grande, TX: Editora Interciência), 3–19.
Siqueira, A., Kolm, H., and E; Brandini, F. P. (2006). Offshore distribution patterns of the cyanobacterium Trichodesmium erythraeum ehrenberg and associated phyto and bacterioplankton in the southern atlantic coast (Paraná-Brazil). Braz. Arch. Biol. Technol. 49, 323–337. doi: 10.1590/S1516-89132006000300018
Smayda, T. J. (1997). Harmful algal blooms: their ecophysiology and general relevance to phytoplankton blooms in the sea. Limnol. Oceanogr. 42, 157–1153. doi: 10.4319/lo.1997.42.5_part_2.1137
Srinivas, B., and Sarin, M. M. (2013). Atmospheric deposition of N, P and Fe to the Northern indian ocean: implications to C-and N-fixation. Sci. Total Environ. 456, 104–114. doi: 10.1016/j.scitotenv.2013.03.068
Stamp, N. (2003). Out of the quagmire of plant defense hypotheses. Q. Rev. Biol. 78, 23–55. doi: 10.1086/367580
Steindinger, T. (1996). “Dinoflagellates,” in Identifying Marine Phytoplankton, ed C. R. Tomas (San Diego, CA: Academic Press), 387–584.
TerBraak, C. J. F., and Prentice, I. C. (1988). A theory of gradient analysis. Adv. Ecol. Res. 18, 271–317. doi: 10.1016/S0065-2504(08)60183-X
Utermöhl, H. (1958). Zurvervollkommnung der quantitativen phytoplankton-methodik. Mitt Int. Vertheorangew. Limnol. 9, 1–38.
Villareal, T. A., and Carpenter, E. J. (2003). Buoyancy regulation and the potential for vertical migration in the oceanic cyanobacterium Trichodesmium. Microbial Ecol. 45, 1–10. doi: 10.1007/s00248-002-1012-5
Westberry, T. K., and Siegel, D. A. (2006). Spatial and temporal distribution of Trichodesmium blooms in the world's oceans. Global Biogeochem. Cycl. 20. doi: 10.1029/2005GB002673
Keywords: marine diazotroph, microbial community composition, phycotoxin, saxitoxins, STX
Citation: Bif MB, de Souza MS, Costa LDF and Yunes JS (2019) Microplankton Community Composition Associated With Toxic Trichodesmium Aggregations in the Southwest Atlantic Ocean. Front. Mar. Sci. 6:23. doi: 10.3389/fmars.2019.00023
Received: 28 May 2018; Accepted: 18 January 2019;
Published: 05 February 2019.
Edited by:
Angel Borja, Centro tecnológico experto en innovación marina y alimentaria (AZTI), SpainReviewed by:
Judith Marie O'Neil, University of Maryland Center for Environmental Science (UMCES), United StatesReut Sorek-abramovich, Dead Sea and Arava Science Center, Israel
Copyright © 2019 Bif, de Souza, Costa and Yunes. This is an open-access article distributed under the terms of the Creative Commons Attribution License (CC BY). The use, distribution or reproduction in other forums is permitted, provided the original author(s) and the copyright owner(s) are credited and that the original publication in this journal is cited, in accordance with accepted academic practice. No use, distribution or reproduction is permitted which does not comply with these terms.
*Correspondence: Mariana Bernardi Bif, bWFyaWFuYWJpZkBnbWFpbC5jb20=