- 1Centre for Tropical Water and Aquatic Ecosystem Research, College of Science and Engineering, James Cook University, Townsville, QLD, Australia
- 2Science for Integrated Coastal Ecosystem Management, School of Marine Biology and Aquaculture, College of Science and Engineering, James Cook University, Townsville, QLD, Australia
- 3Rural Economic Development, Department of Agriculture and Fisheries, Queensland Government, Townsville, QLD, Australia
- 4Department of Environment and Science, Queensland Government, Brisbane, QLD, Australia
- 5Great Barrier Reef Marine Park Authority, Australian Government, Townsville, QLD, Australia
Managers are moving toward implementing large-scale coastal ecosystem restoration projects, however, many fail to achieve desired outcomes. Among the key reasons for this is the lack of integration with a whole-of-catchment approach, the scale of the project (temporal, spatial), the requirement for on-going costs for maintenance, the lack of clear objectives, a focus on threats rather than services/values, funding cycles, engagement or change in stakeholders, and prioritization of project sites. Here we critically assess the outcomes of activities in three coastal wetland complexes positioned along the catchments of the Great Barrier Reef (GBR) lagoon, Australia, that have been subjected to restoration investment over a number of decades. Each floodplain has been modified by intensive agricultural production, heavy industry and mining infrastructure, urban/peri urban expansion, aquaculture development and infrastructure expansion. Most development has occurred in low-lying coastal floodplains, resulting in major hydrological modifications to the landscape. This has left the floodplain wetlands in a degraded and hydrologically modified state, with poor water quality (hypoxic, eutrophication, sedimentation, and persistent turbidity), loss of habitat, and disconnected because of flow hydraulic barriers, excessive aquatic plant growth, or establishment of invasive species. Successful GBR wetland ecosystem restoration and management first requires an understanding of what constitutes “success” and must be underpinned by an understanding of complex cause and effect pathways, with a focus on management of services and values. This approach should recognize that these wetlands are still assets in a modified landscape. Suitable, long term, scientific knowledge is necessary to provide government and private companies with the confidence and comfort that their investment delivers dividend (environmental) returns.
Introduction
Connectivity of wetlands and drainage channels crossing coastal floodplains provides an essential habitat for a range of flora and fauna that hold cultural, social and economic values that deliver diverse amenity and ecosystem services (Baran et al., 2001; Amoros and Bornette, 2002; Buijse et al., 2002; King et al., 2003; Elliott and Whitfield, 2011; Elliott et al., 2016; Waltham and Fixler, 2017). Because of their low-lying positions, these ecosystems are the receiving environment for runoff from urban, agricultural and industrial sites (Bulleri and Chapman, 2010; Sheaves et al., 2010; Barbier, 2013), as well as being the first line of exposure to climate change and sea level rise (Kelleway et al., 2017; Pettit et al., 2018). They have also been extensively impacted by drainage and reclamation, with global floodplain wetland loss estimated at 95 km2/years (Coleman et al., 2008), that is not likely to slow (Davidson, 2014). There is an increasing global movement by managers and community to halt this loss and degradation, and to commence large-scale programs to repair and restore coastal wetland ecosystem habitat and connectivity (Barbier, 2013).
Large-scale restoration has a long history in north America (e.g., Repair America’s Estuaries1), and plans for extensive restoration efforts are advanced in many other parts of the world. For instance, China plans to invest $1 billion on more than 50 large programs by 2030 – with the aim of restoring and recreating wetland connectivity to mitigate poor water quality and lost habitat for local wildlife species (Dobson et al., 2007). While restoration efforts are vital (Creighton et al., 2016), access to relevant and appropriate scientific data demonstrating biodiversity and ecosystem service return for the investment are lacking (Zedler, 2016; Waltham et al., 2017).
In planning restoration projects, it is important to recognize that stakeholders (beneficiaries) have different and sometimes conflicting views or priorities when determining coastal wetland ecosystem services (Harris and Heathwaite, 2012). For example, placing high value on services such as the freshwater extraction for agriculture from floodplains can directly undermine cultural ecosystem service values related to aquatic biodiversity (Boulton et al., 2016), not to mention reduce duration and frequency of water connection across floodplains, which has biological consequences (Baran et al., 2001; Rayner et al., 2009). Ecosystem repair strategies seem to be most effective when values of all stakeholders are incorporated, a process best facilitated through discussions to set objectives early in the project lifecycle (Sheaves et al., 2014; Zedler, 2016; Guerrero et al., 2017). Scale is another important aspect, e.g., local-scale improvement of fish habitat vs. catchment-scale amelioration of agricultural fertilizer loads exported to coastal waters. Focusing at an appropriate scale is important not only for informing technical aspects of the restoration management activities, but also ensures appropriate management bodies are involved (Butler et al., 2013).
Great Barrier Reef World Heritage Area – Lost Coastal Floodplain Wetlands
Australia faces a legacy of degraded coastal wetland habitats despite a small population and a relatively short 200 years of urban/industrial development and agricultural intensification (Creighton et al., 2016). The Great Barrier Reef (GBR) lagoon (Figure 1), a World Heritage Area and National Marine Park, protected under an assortment of international agreements, and national, and state legislation/policies is suffering on-going poor water quality from catchment agricultural runoff and intensification (Bainbridge et al., 2009; Brodie and Waterhouse, 2012; Waterhouse et al., 2016; Dubuc et al., 2017). A causative factor is loss of coastal wetland habitats associated with agricultural and urban development expansion (Sheaves and Johnston, 2010; Sheaves et al., 2014; Waltham and Sheaves, 2015), which is reducing the GBR’s resilience to future development and climate change pressures (Department of Environment Heritage and Protection [DEHP], 2016). Conservation and repair of the GBR coastal wetland ecosystems’ and connectivity has only recently come into focus due to the threat of ongoing decline of the GBR, particularly around major agricultural regions (Department of Environment Heritage and Protection [DEHP], 2016; Waterhouse et al., 2016). In response, ecosystem protection and restoration has been recognized as key to reef resilience, and is now reflected in long-term strategic planning policies (e.g., Reef 2050 Plan). Reef 2050 Plan recognizes that freshwater floodplain wetlands form an important biological component of the GBR seascape and are part of the broader coral reef system that it is most famous for (Figure 2). However, there is still a lack of data to quantify the change that has occurred from “natural” floodplain wetland areas to the current state (Sheaves, 2016). Here the objectives are to review and assess the learnings from floodplain connectivity repair projects from the GBR catchments. In doing so, we evaluate current and developing institutional settings, in addition to funding opportunities that enable effective coastal wetland connectivity repair.
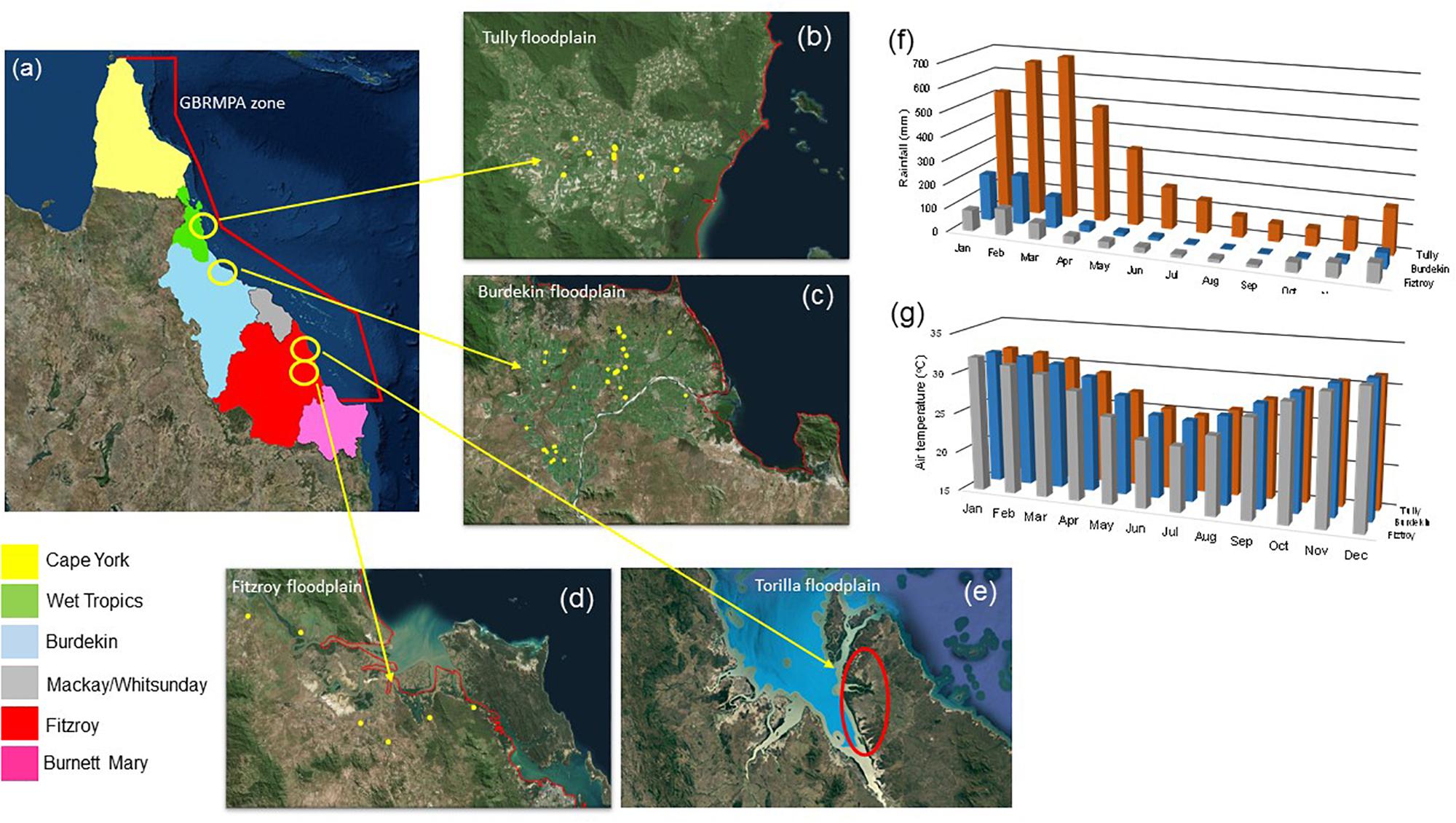
Figure 1. (a) Location map of the Great Barrier Reef World Heritage Area (GBRMPA) along the Queensland coastline, including NRM regional bodies (see Figure 3). (b) Tully floodplain; (c) Burdekin floodplain; (d) Fitzroy floodplain along with field fish sampling completed in previous surveys; (e) Torilla floodplain in Broad Sound catchment. (f) Monthly average rainfall totals at gauging stations in each floodplain area [Tully gauging station 32042 (1925–2018); Burdekin station 33001 (1887–2018); Fitzroy station 39083 (1939–2018)]; and (g) monthly ambient air temperature records at BOM recording stations [Tully station 032004 (1871–2018); Burdekin station 33002 (1951–2018); and Fitzroy station 39083 (1939–2018)].
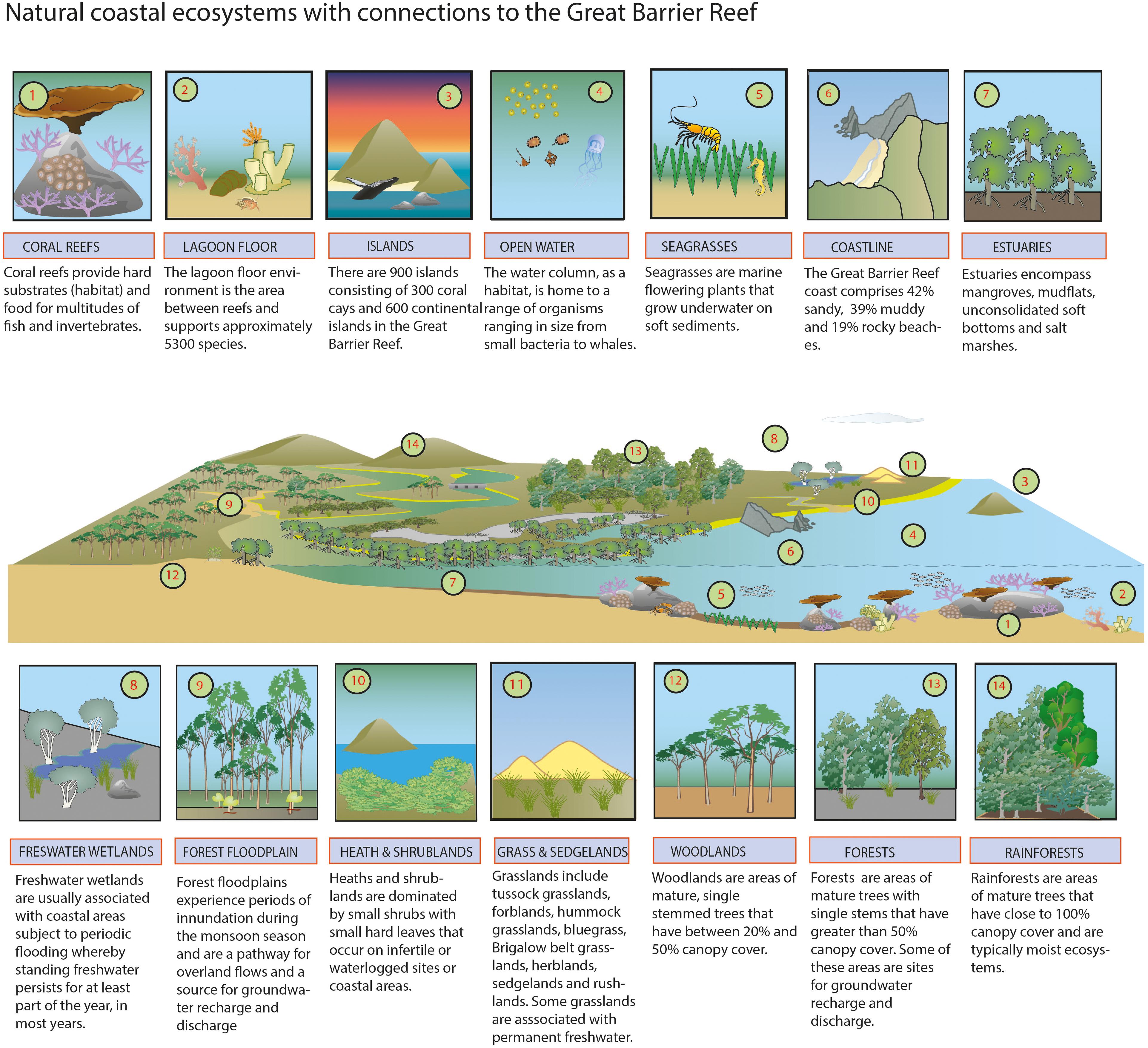
Figure 2. The range of different coastal floodplain wetland habitats within the GBR lagoon, and a description for each habitat.
Reef 2050 Plan and Seeking the Outcomes
Numerous Australian and Queensland Government laws and policies contain provisions related to coastal habitat management in the GBR, notably the Australian Government’s Environment Protection and Biodiversity Conservation Act 1999 and the Queensland Government’s Environmental Protection Act 1994, Fisheries Act 1994, Marine Parks Act 2004, Planning Act 2016, Vegetation Management Act 1999, and Water Act 2000. These generally regulate development or disturbance of coastal habitats. Offset laws and associated policies provide a mechanism to support restoration of coastal habitats. The Queensland Environmental Offsets Act 2014 provides a framework to facilitate environmental offsets that may lead to restoration of degraded habitats, as well as point-source water quality offsets policies that may also support investment into constructing wetlands or other systems with demonstrable water quality benefits. Proposed changes to reef protection regulations would include a water quality offset framework, which may trigger coastal habitat restoration for water quality improvement purposes (State of Queensland, 2017). In addition, landholders and government will also need to take care to fully consider tidal boundary laws and amendments when considering ponded pasture reconversion projects (Bell-James and Lovelock, in press).
The overarching framework for managing the GBR is the Reef 2050 Long-term Sustainability Plan (Reef 2050 Plan hereafter) (Great Barrier Reef Marine Park Authority [GBRMPA], 2015). In the Reef 2050 Plan, “coastal habitat” is recognized as supporting the ecological and biological processes of the Reef, providing habitat for biodiversity, community and economic benefits and increasing resilience to climate change. Reef 2050 Plan has a target for 2020 that “There is no net loss of the extent, and a net improvement in the condition, of natural wetlands and riparian vegetation that contribute to Reef resilience and ecosystem health.” An important and necessary action has been to implement the Wetlands in the Great Barrier Reef Catchments Management Strategy 2016–2021 that outlines on-ground actions, education, and scientific research necessary to improve management and repair of wetlands in the GBR catchment. The Reef 2050 Water Quality Improvement Plan 2017–2022 (Reef WQ Plan) is nested under the water quality theme in the Reef 2050 Plan and is charged with the role to address all land-based sources of water pollution. The Reef WQ Plan identifies the need for targeted catchment repair projects, which is best achieved via a whole-of-system catchment management approach (State of Queensland, 2018b).
A key challenge to achieving these outcomes is that, since European settlement, the GBR catchment has continued to be modified (Figure 3), with estimates around 64% of the catchment area cleared of the predominant native vegetation, most occurring south of Cooktown (QLUMP data 2009, Queensland Government), though the rate of clearing has slowed and in some places has changed very little in the past few decades. The remaining undeveloped areas are predominately used for grazing, with around 10% of the catchment area within Protected Areas. The impacts of grazing on remnant natural areas include increased erosion, weed transfer, nutrient enrichment and loss of riparian understory (Brodie and Waterhouse, 2012). Feral pigs and cattle are also impacting Protected Areas (Waltham and Schaffer, 2018). Noticeably a major limitation in the success of repair efforts is the ongoing competing land uses (e.g., sugar cane production), and that so much of the GBR floodplain wetland and connectivity loss is not readily reversable. Investment is needed in order to understand these complex landscapes, aquatic floodplain connectivity, and how to restore function and achieve solutions that balance the environment with the desires of the community. With further investment for implementation, we could then be in a position to scale up efforts in order to work toward achieving the objectives set in the Reef 2050 Plan.
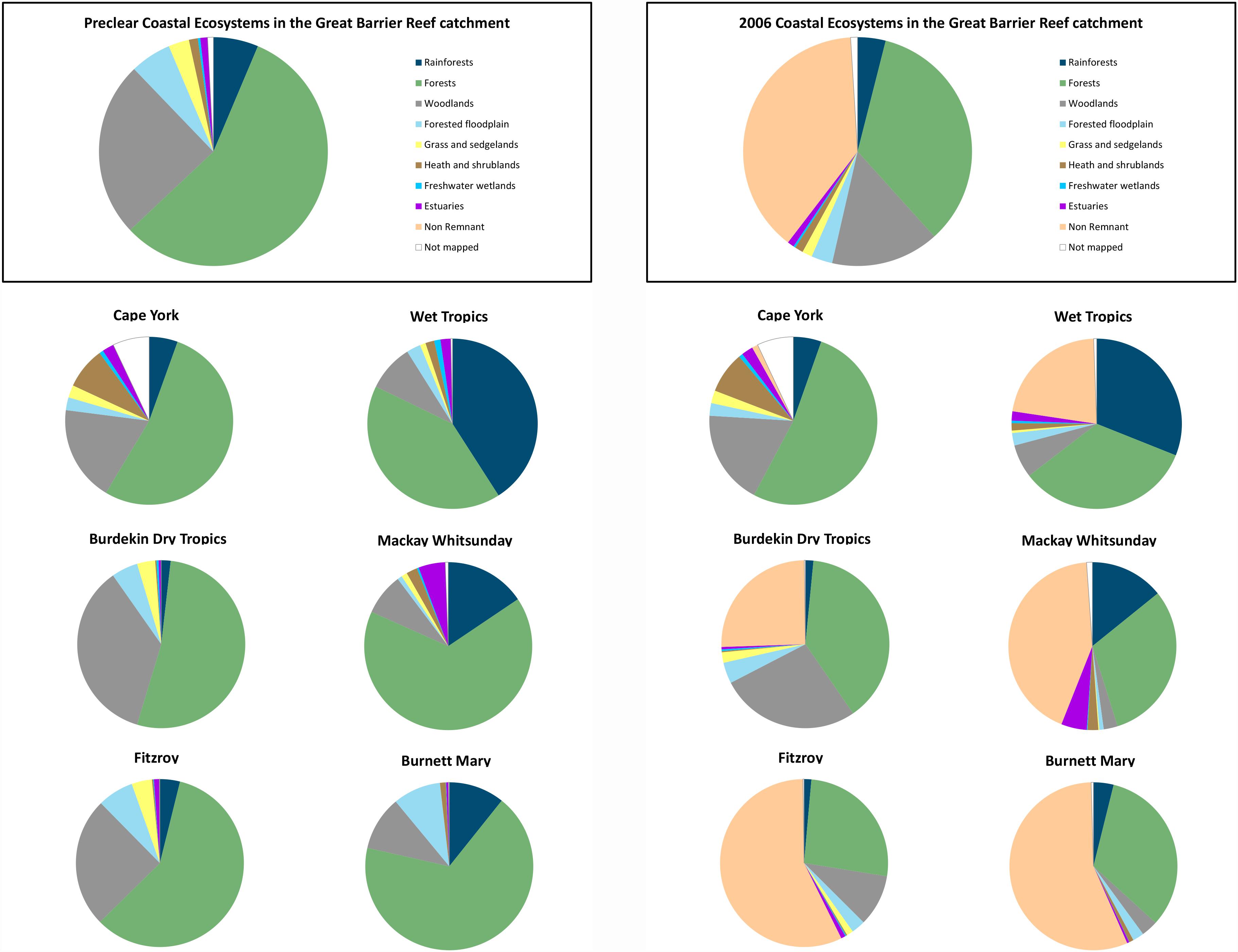
Figure 3. Mapped land use change between preclear and 2009 coastal ecosystems in the GBR. Natural Resource Management areas mapped in Figure 1.
Critical Review and System Repair Attempts
In this perspective, we draw attention to three major wetland complexes subjected to years of intensive land use change in the GBR catchment: (1) Tully/Murray floodplain; (2) Burdekin floodplain, and (3) Broad Sound floodplain (Figure 1). These regions represent different climate conditions, land uses and different pressures on wetland systems, and restoration approaches. A review of available published scientific reports and government reports reveal that GBR floodplain wetlands (note that there are no fish data for the Torillia floodplain, which is evaluated below) provide habitat for nearly 80 fish species, across the types of wetland areas in this review (Table 1). Many species have a diadromous life history, requiring migration to freshwater or estuarine habitat areas at critical life stages, and as such a migration barrier or delay could avert future reproduction success (Sheaves, 2009). An example is the mangrove jack (Lutjanus argentimaculatus), which spawns on offshore coral reef areas (Russell and McDougall, 2005), with larvae drifting into nearshore waters and estuaries where new recruits then use estuaries for feeding and shelter, while continuing further upstream to floodplain wetlands (Figure 4). Mature fish eventually migrate to sea again to complete their lifecycle. This complex pattern of life-history connectivity between coral reefs and low-land freshwater wetlands (Sheaves, 2009) emphasizes the obligate requirement for a connected seascape in GBR catchments (Russell and McDougall, 2005).
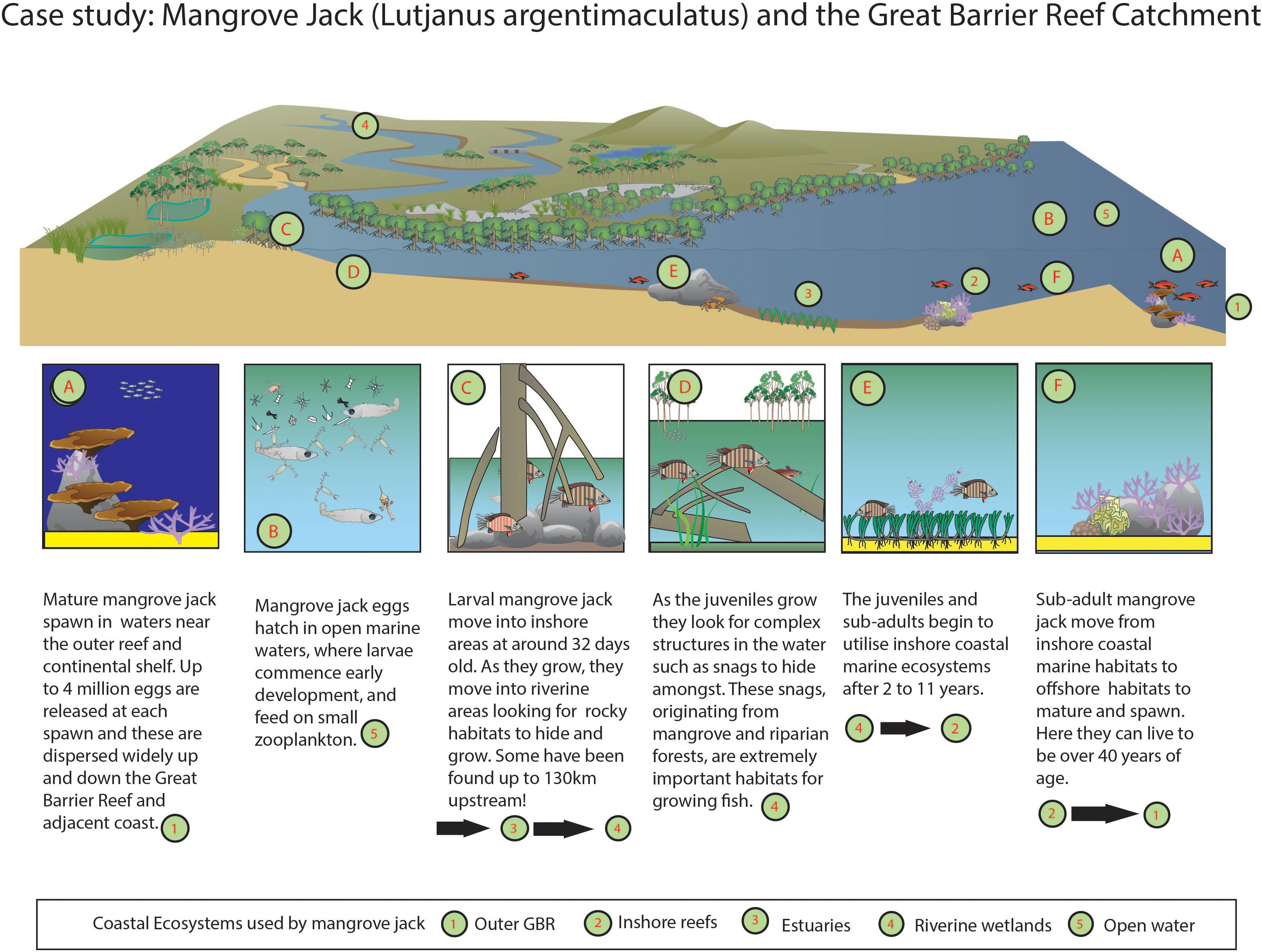
Figure 4. Lifecycle of the mangrove jack (Lutjanus argentimaculatus) outlining critical need for access to freshwater and marine coastal wetland areas to complete lifecycle stages. This lifecycle outlines that any disturbance in the seascape, that prevents access to habitats may implicate successful reproduction success for this diadromous species.
Restoration Case Study 1: Ponded Pastures in the Torilla Plain, Broad Sound Catchment
The Torilla Plain (Figure 1d), which is listed under the Directory of Important Wetlands in Australia (DIWA), has been identified as high value wetland through state-wide Aquatic Conservation Assessment (Inglis and Howell, 2009), and provides multiple ecosystem services including: cattle grazing, fisheries nursery habitat, high bird biodiversity, and threatened-species habitat (Table 2 and Figure 5A). The coastal wetland complex is an intricate and dense network of small ponds and channels, and small earth-walls (∼20 m wide) which were constructed by graziers to preclude tidal flow, as well as improve and extend ponded pasture opportunities for cattle during the late dry season (WetlandInfo, 2016).
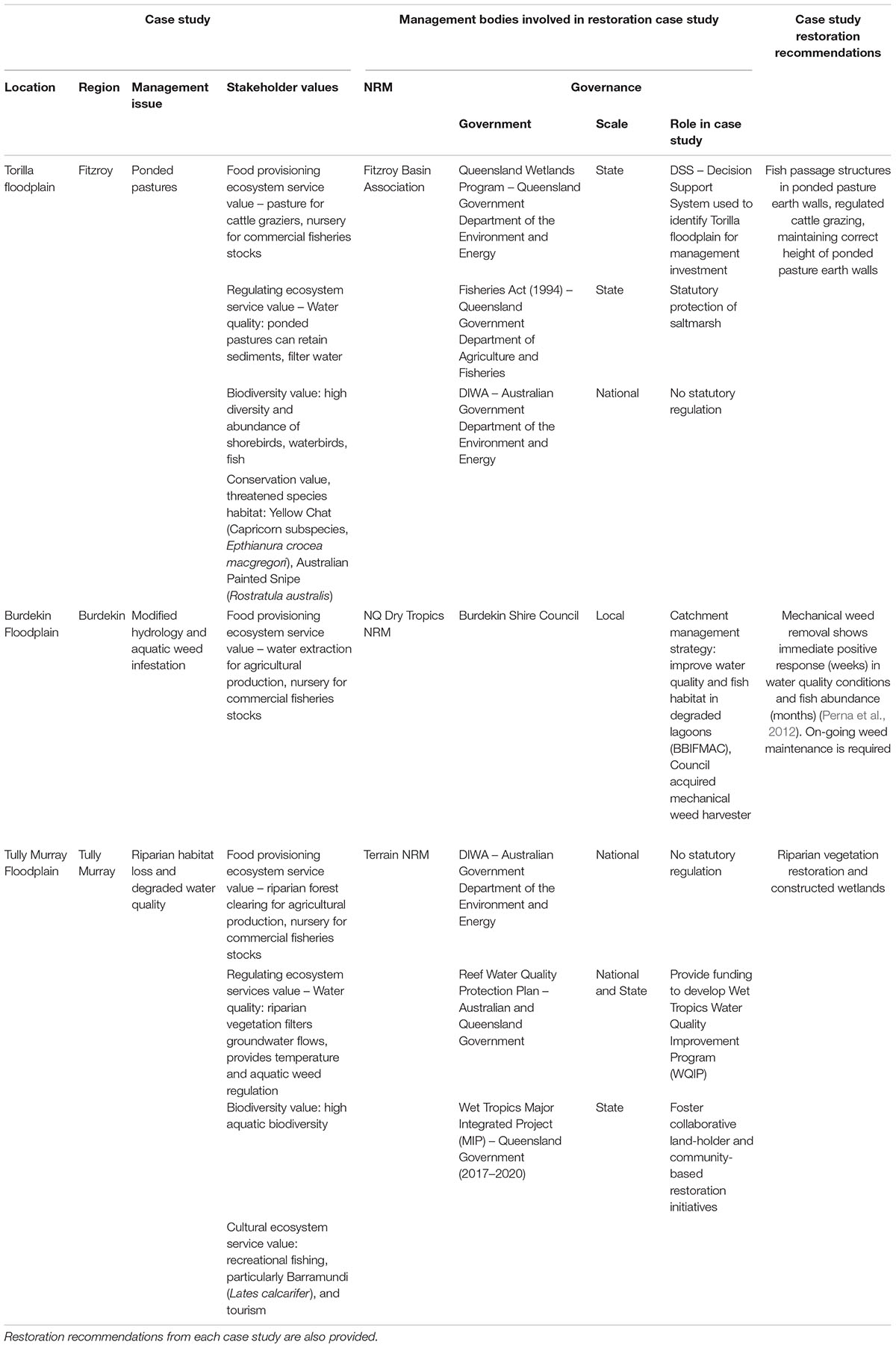
Table 2. Overview of stakeholder values and management bodies involved in three coastal wetland restoration case studies across catchments of the Great Barrier Reef.
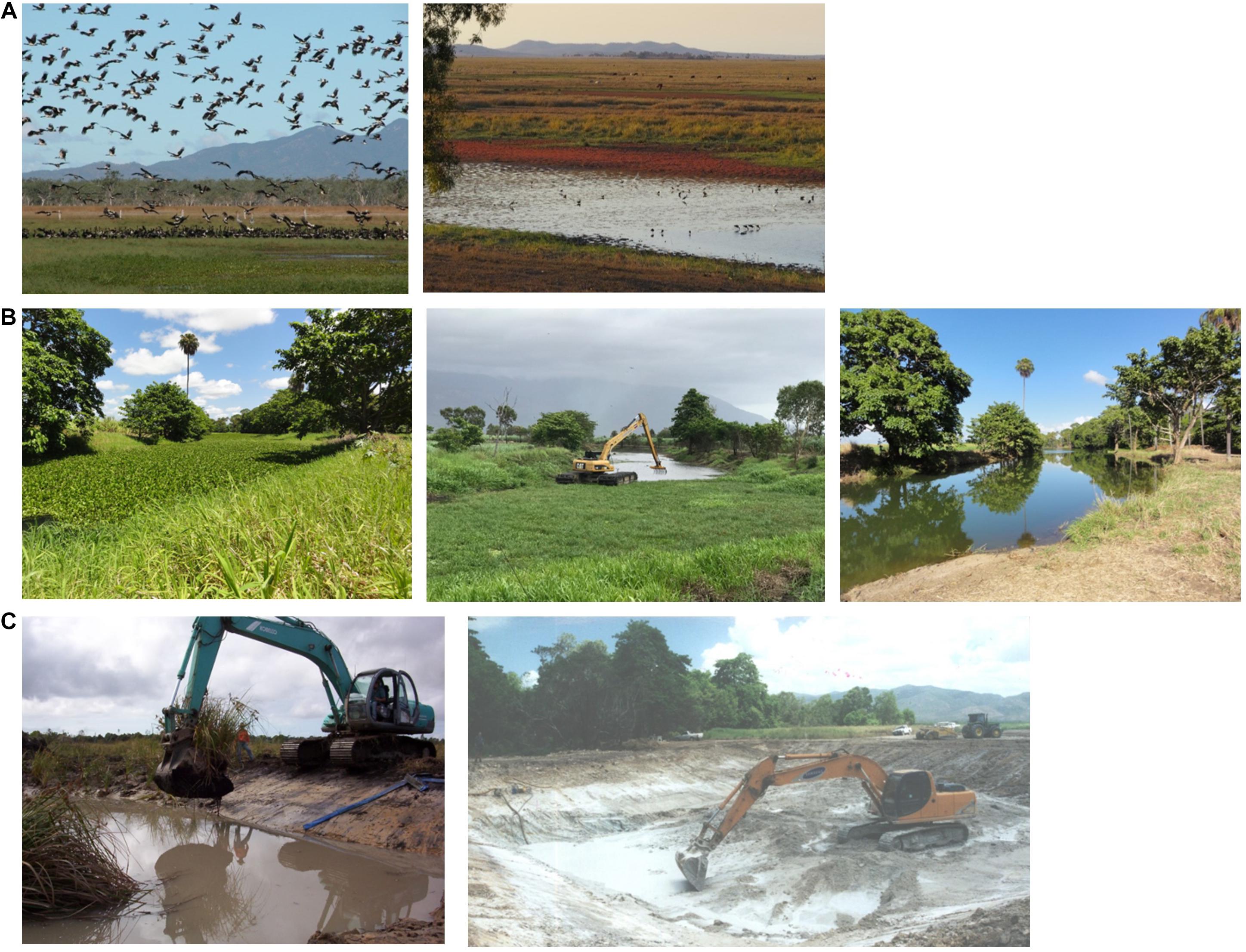
Figure 5. Photographs illustrating restoration case studies: (A) bird habitat and cattle grazing in the Torilla floodplain (Photo credits: R. Jaensch); (B) aquatic weed removal in the Burdekin floodplain (Photo credits: P. Groves); and (C) Tully Murray floodplain constructing wetlands (Photo credits: R. Digman).
Although saltmarsh is a protected habitat in Queensland (Fisheries Act 1994) and establishment of new ponded pastures is subsequently precluded, landholders are allowed to maintain existing earth walls. This infrastructure benefits Yellow Chats (Epthianura crocea macgregori) – a threatened species that require freshwater wetland vegetation for breeding and foraging purposes (Houston et al., 2004). However, ponded pastures may reduce overall productivity of the wetlands by restricting saltwater flow. This can negatively impact fish by impairing connectivity to nursery areas, particularly for diadromous species [such as Barramundi (Lates calcarifer which are known in the region more broadly, Table 1)], and by diminishing water quality (in particular high temperatures and pH, and low dissolved oxygen which occur in pond pasture areas elsewhere; Waltham and Fixler, 2017).
In 2015, the Torilla floodplain was identified as a priority wetland for local National Resource Management (NRM) investment, due in part due to its diverse stakeholder values (i.e., cattle grazing, threatened species habitat, biodiversity, and fisheries stocks), and the wetland’s national importance (DIWA listed) (Jaensch et al., 2015). To restore and maintain ecological function of the Torilla wetland plain complex, while also sustaining cattle production services, landholders and local NRM groups are working to deliver a coordinated management strategy (WetlandInfo, 2016). Sustainable grazing practices (i.e., correct rotation between upland forest and floodplain, and low to moderate stocking densities; Houston et al., 2006, 2013), as well as fish passage infrastructure in earth walls are actions to improve cattle production, threatened-species habitat and connectivity for diadromous fish species. Earth walls installed at the correct height could simultaneously promote the extension of the area of freshwater vegetation, while also allowing tidal connection aims to maintain high productivity in the Torilla floodplain. Creation of deep-water refuges in ponded pastures to ensure water quality (particularly water temperature) is suitable for fish (for example, avoiding acute thermal risks; Wallace et al., 2015) could also be considered as an additional engineering solution for maintaining high quality habitat (Hyland, 2002).
Whether this restoration case study constitutes success is dependent on which of the many ecosystem functions and services provided are most highly valued by the community (e.g., bird habitat, water quality, or fish habitat). Furthermore, whether this and similar local-scale management efforts contribute in any real way to broader wetland restoration priorities of the Reef 2050 Plan has not been evaluated. A more pressing question is: Which stakeholder values should take priority? The Wetlands in the Great Barrier Reef Catchments Management Strategy 2016–2021 recognizes this issue and suggests that a balanced approach is needed to effectively manage for multiple and conflicting values (Department of Environment Heritage and Protection [DEHP], 2016). However, as is the case in the Torilla Plains, management effort and outcomes will be determined by which stakeholders are engaged in the restoration area/activity, and the scale at which they have capacity or will to manage. Management of these ponded pastures here, and indeed elsewhere in the GBR catchments will also require careful legislative consideration around the tidal boundary laws (Bell-James and Lovelock, in press).
Restoration Case Study 2: Modified Hydrology and Aquatic Weed Infestation, Burdekin Floodplain
The Burdekin floodplain (Figure 1c) is one of the largest sugar cane production regions in northern Australia (Davis et al., 2014), placing high value on agricultural ecosystem service provisioning for the nation’s economy. The Bowling Green Bay Ramsar site is within the northern part of the Burdekin floodplain and supports rich and abundant birdlife, with more than 20,000 waterbirds occurring seasonally, including some of the largest colonies of fish-feeding birds in eastern Queensland (Queensland Wetlands Program, 2013). The area also supports commercial, recreational, and Indigenous fisheries (Davis et al., 2014).
Since the 1800’s extensive hydrological changes have occurred to the Burdekin floodplain due to infrastructure development for the purpose of irrigating agricultural land (Davis et al., 2014). Extreme hydrological changes have resulted in the loss of wetland ephemerality (wet and dry seasonality), and in doing so, have increased aquatic weed infestation (Perna and Burrows, 2005; Burrows and Butler, 2007; Connolly et al., 2012; Waltham and Fixler, 2017). Changes in hydrology, increased prevalence of natural weed barriers to fish movement, and high levels of nutrient pollution from agriculture have had negative consequences for water quality (increased turbidity and decreased dissolved oxygen) and fish accessing the floodplain (Perna and Burrows, 2005; Waltham and Fixler, 2017). Management intervention has been necessary to restore ecological function, and connectivity, in degraded areas of the Burdekin floodplain, and continues to be a priority (Great Barrier Reef Marine Park Authority [GBRMPA], 2015).
Restoring natural hydrological processes of the Burdekin floodplain requires significant investment and may not, indeed, be a feasible course of action because of the scale and severity of floodplain modification (Davis et al., 2014). Approximately a decade ago, the Burdekin Shire Council and the Burdekin Bowen Integrated Floodplain Management Advisory Committee prioritized local management actions to repair degraded water quality and to improve hydrologic connectivity associated with natural weed barriers (Table 2). Mechanical weed removal was identified as the best strategy (Figure 5B), and via a community-based initiative/partnership (i.e., local council, industry, and landholders) aquatic weeds were excavated, water quality improved, native vegetation returned, and fish diversity and abundance was restored (Perna and Burrows, 2005; Butler et al., 2007; Perna et al., 2012). Increases in the abundance and diversity of freshwater fish species is partly attributed to the return of native freshwater plants, which provide a suitable micro-habitat for dependent freshwater fish species (e.g., Melanotaenia splendida, Glossamia aprion, Ambassis agrammus; Table 1; Perna et al., 2012). However, it is important to note that for diadromous fish (in particular Lates calcarifer, Chanos chanos, Scatophagus argus; Table 1) to successfully recolonize upstream areas following weed removal, it would require a flood event to permit connection to a downstream recruitment source, or major investment to construct fish ladders over barriers across the floodplain (Perna et al., 2012).
Although restoration efforts are localized here, broader investment and restoration action is necessary across the floodplain. An important lesson is that even local-scale restoration efforts require on-going maintenance. To begin upscaling restoration, this would require a coordinated effort including funding and resources for long-term maintenance (Waltham and Fixler, 2017). Further research is necessary to examine the sustainability of restoration by active, expensive, mechanical aquatic weed removal against alternative passive restoration that requires minimal on-going maintenance, such as the reinstatement of natural tidal flows in the floodplain – using saltwater ingress as a means of controlling invasive freshwater aquatic plants (Reid et al., 2018).
Restoration Case Study 3: Riparian Habitat Loss and Reduced Water Quality, Tully Murray Floodplain
The Tully Murray floodplain (Figure 1a),located in the Wet Tropics bioregion, hosts a broader diversity of agricultural land-uses than the preceding case studies, primarily sugar cane, bananas, and grazing. Intense agricultural activity has resulted in the classification of the Tully Murray floodplain as a “pollutant hotspot,” and degraded water quality, in particular low dissolved oxygen, has reduced aquatic biodiversity in the region (Figure 5C) (Pert et al., 2010; Pearson et al., 2013). Additionally, most native riparian vegetation has been cleared and fragmented, contributing to degradation of the coastal wetland complex as a whole (Pert et al., 2010). Riparian vegetation is tightly linked to coastal wetland ecosystem function, particularly through regulation of sunlight, thereby limiting aquatic weed growth and stabilizing temperature and dissolved oxygen levels (Bunn et al., 1998; Pert et al., 2010). Available data outlines that restoration of riparian vegetation provides additional benefits for aquatic macrophyte, macroinvertebrate, and fish assemblages on this floodplain (Arthington et al., 2007; Pearson et al., 2013; Arthington et al., 2015).
Given its status as a “pollutant hotspot” and high biodiversity and cultural ecosystem service values (Table 2), the Tully Murray floodplain has received management attention through the Tully Water Quality Improvement Program (Tully WQIP), with objectives to restore water quality and aquatic biodiversity (Kroon et al., 2009). Restoration of riparian vegetation was a key directive of the Tully WQIP for its potential to deliver supporting and regulating water quality ecosystem services (Kroon et al., 2009). In addition to shade for temperature and aquatic weed regulation, riparian vegetation may also filter groundwater nitrate, trap sediments, and reduce erosion (Rassam and Pagendam, 2009; Pert et al., 2010). To locate target areas for restoration that would provide the best return on investment, riparian ecosystem services in the Tully Murray floodplain have been mapped (Pert et al., 2010), including riparian areas with high denitrification ability near agricultural land with elevated nutrient loads (Rassam and Pagendam, 2009).
An evaluation of riparian restoration across catchments of the GBR shows that overall water quality improvements are dependent upon: the length of time since restoration initiation and the width and connectivity of riparian re-vegetation sites (Paul et al., 2018). In addition to water quality improvements, riparian re-vegetation projects also provide biodiversity and bio sequestration co-benefits (Paul et al., 2018). However, to achieve meaningful outcomes for the Reef 2050 Plan, a whole-of-ecosystem approach to coastal wetland restoration is required to effectively “scale-up” improvements in water quality. Riparian re-vegetation projects could be coordinated by means of other restoration activities such as: weed or pollutant removal; reinstatement of tidal connectivity, and the construction of wetlands in marginal cane land (Waltham et al., 2017). Constructed wetlands can improve water quality by reducing nutrient and suspended sediment levels (Jia et al., 2016). Additionally, transitioning low-lying, marginal cane land to constructed wetlands has received recent interest as a cost-effective water quality improvement solution in the Tully Murray floodplain (Waltham et al., 2017). This interest extends beyond water quality benefits, with potential to provide an economic benefit to landholders through public/private market schemes investing in restoration “green” projects (Waltham et al., 2017). Additional water quality co-benefits could be gained by planting riparian buffers around constructed wetlands, enhancing filtration capacity of the low-lying land through denitrification by riparian vegetation (Rassam and Pagendam, 2009).
Plan of Action
Framework for Assessing Rehabilitation Activities
The three case studies highlight that coastal floodplain restoration projects are underway, however, there is a major risk if they continue to occur in a “stakeholder silo.” A coordinated restoration strategy matching local capacity, land holder willingness, with regional incentives is needed. When assigning or making management decisions about a wetland, it is important that these consider the full range of floodplain ecological, economic, and social values (Maes et al., 2012). Wetland management decisions can favor either conversion or management for a single ecosystem service, such as water supply or food production (Villamagna et al., 2013; Broszeit et al., 2017). As wetlands become scarce and under more pressure (Finlayson, 2013; Mitsch et al., 2013), and as we develop a better understanding of the full range of values provided by them, the best options will increasingly involve managing wetlands for a broader array of services (stacked services; Waltham et al., 2017), in addition to aligning with the wise use principles of the Ramsar Convention.
Numerous prioritization or decision support tools are available2. A wetland prioritization decision support system (WDSS) was developed specifically for the GBR catchment to strategically prioritize wetlands for rehabilitation. It uses biophysical, socio-economic, community capacity, and threat data, as well as expert input to identify high priority wetlands for strategic investment (Francis and Tait, 2006). Although the WDSS is an effective tool for prioritizing project sites, it does not adequately represent the interplay between the terrestrial and aquatic environment, and the influence of processes happening in the broader catchment – the WDSS wasn’t available or used as part of the three case studies examined here. There is a need to take a whole-of-catchment approach to planning activities, consistent with the Queensland Government’s Wetlands in the Great Barrier Reef Catchment Management Strategy 2016–2021. A decision support framework is needed that firstly establishes what stakeholders value and want (e.g., through a structured decision making process; Kozak and Piazza, 2015; Guerrero et al., 2017), the biophysical components, processes and drivers at the site and broader catchment, and relevant policy drivers or constraints that might pose a barrier to success. The framework then would need to consider a range of possible management interventions (e.g., on-ground restoration, education, research) at different scales (site, catchment, or regional), the spatial thresholds of interventions to achieve the desired objective, the synergistic or aggregative effects (i.e., the cumulative benefit of several interventions), and the time frame in which the objectives will be met (Guerrero et al., 2017). We need to revisit objectives and ensure that the selected intervention/s achieve the objectives, ensure probity of the knowledge underpinning the restoration decision, clearly define what constitutes success and the limits of predictability of outcomes in uncertain floodplain systems (this is also the case for the three case studies examined here, to determine whether further improvements and restoration outcomes are possible or necessary). We also need to ensure restoration efforts conform to budgetary constraints (Creighton et al., 2015), and what, if any, trade-offs there may be to other services, such as biodiversity, water quality or carbon (Waltham et al., 2017). A recent framework developed for climate change adaption in the coastal zone (Sheaves et al., 2016) could be adopted here for the floodplain wetland restoration situation in the GBR.
Government and Market-Based Investment Mechanisms to Facilitate Restoration
The Reef 2050 Plan and Reef WQ Plan guides Australian and Queensland Government investment into initiatives to manage and protect the values of the Reef, including investment into coastal habitat restoration. To value-add to government investment, the Australian and Queensland Governments’ are seeking private industry and philanthropic co-investment (Commonwealth of Australia, 2016). The Reef Trust Partnership outlines opportunities for co-investment, including riparian rehabilitation, fish habitat repair, connectivity, and improving coastal ecosystem functioning through weed and pest control (examples of which are provided in the case studies). The first project involving matched co-investment through the Reef Trust commenced in 2016 with Greening Australia, in partnership with Birdlife Australia, Conservation Volunteers Australia, and Wetland Care Australia. This arrangement includes a $4 million project to restore 200 hectares of wetlands in priority areas along the GBR coast (Commonwealth of Australia, 2015). Based on the success of that partnership model, the Australian Government’s Reef Trust committed another $5 million to Greening Australia, who will match this investment dollar-for-dollar through its Reef Aid program for a total investment of $10 million to restore an additional 500 hectares of priority coastal wetland habitat (State of Queensland, 2018b). A Reef Credits Initiative will also be rolled out to provide a market based incentive approach to achieve water quality improvement through agronomic practice change or habitat restoration, including constructing wetlands (State of Queensland, 2018b). The Australian and Queensland Governments also invest in habitat “system repair” through the Australian Government’s Reef Trust and Queensland Government’s natural resource management program and Reef Water Quality Program (Commonwealth of Australia, 2016).
Long-Term Investment and Maintenance
Government funding is usually project based, it is short-term (1–5 years) and generally does not invest public funds for necessary long-term maintenance. Restoration projects usually require maintenance beyond the life of the initial funding (Moore and Rutherfurd, 2017). Those projects involving revegetation or weed control can (and mostly do) rapidly revert to a degraded state without planned and funded ongoing maintenance support. Therefore, there is a need for careful consideration of the value, and particularly so when considering longer-term funding for maintenance and new mechanisms to ensure project sites are maintained. One option could be funding through local government environment levees, and maintenance by landholders or Landcare groups (as outlined in Restoration Case Study 2). Payments for ecosystem services, to value the fisheries and other services provided by coastal habitats, will require better quantification of fisheries values of coastal habitats (Wegscheidl et al., 2017), and support tools such as benefit calculators and capacity building (Waltham et al., 2017). Recognition of the multiple benefits that may be accrued, resulting in new and emerging investment opportunities are emerging. These include carbon additionally programs, such as the Australian Government’s Clean Energy Finance Corporations Reef Funding Program and the Queensland Government’s $500 million Land Restoration Fund, that support carbon projects that can also leverage co-benefits such as enhancing wetlands for fisheries and restoring ecosystems and degraded land (State of Queensland, 2018a).
Conclusion
There has been considerable investment in coastal wetlands and floodplains along the GBR coastline, tackling catchment water quality runoff, floodplain aquatic habitat connectivity, and land use practice changes to improve water quality – more projects are on the horizon as interest from private investor groups grows. All these wetlands are valued for their role in ameliorating pollution entering the GBR lagoon. However, they form part of the broader GBR ecosystem, and indeed, require a similar level of ecosystem protection and restoration necessary under the objective of GBR ecosystem protection. Some data are emerging around the ecosystem value of GBR coastal wetlands, but more is needed particularly when considering the connected seascape (reef to freshwater wetlands). Exciting opportunities to access philanthropic and corporate investment that supports social responsibility toward environmental management and protection are on the horizon, which require careful planning and assessment. Repairing and protecting the GBR coastal wetland ecosystems and connection with offshore coral reef ecosystems is challenging, but the social, environmental, and economic returns for this investment outweighs not doing anything.
Author Contributions
All authors contributed to the discussion. NW, CW, and CB wrote the manuscript. DB, CC, MR, DM-A, PG, and MS edited the manuscript. DA and PG prepared the figures.
Funding
This project was supported by funding provided from the Australian Government’s National Environmental Science Program Tropical Water Quality Research Hub – Project 3.3.2 (https://nesptropical.edu.au/index.php/project-3-3-2/).
Conflict of Interest Statement
The authors declare that the research was conducted in the absence of any commercial or financial relationships that could be construed as a potential conflict of interest.
Acknowledgments
This paper is the collimation of years of research and knowledge developed by the authors, some published, or contained in technical reports and databases. The authors thank the farmers, government, and industry colleagues for sharing the input and knowledge over the years. The authors also thank the reviewers for providing valuable comments, which improved this manuscript.
Footnotes
- ^www.estuaries.org
- ^https://wetlandinfo.ehp.qld.gov.au/wetlands/resources/tools/assessment-search-tool/
References
Amoros, C., and Bornette, G. (2002). Connectivity and biocomplexity in waterbodies of riverine floodplains. Freshw. Biol. 47, 761–776. doi: 10.1046/j.1365-2427.2002.00905.x
Arthington, A. H., Godfrey, P. C., Pearson, R. G., Karim, F., and Wallace, J. (2015). Biodiversity values of remnant freshwater floodplain lagoons in agricultural catchments: evidence for fish of the Wet Tropics bioregion, northern Australia. Aquat. Conserv. Mar. Freshw. Ecosyst. 25, 336–352. doi: 10.1002/aqc.2489
Arthington, A. H., Pearson, R. G., Connolly, N. M., James, C. S., Kennard, M. J., Loong, D., et al. (2007). Biological Indicators of Ecosystem Health in Wet Tropics Streams. Final Report to the Catchment to Reef Research Program, CRC for Rainforest Ecology and Management and CRC for the Great Barrier Reef. Townsville: James Cook University.
Bainbridge, Z. T., Brodie, J., Faithful, J., and Sydes, D. A. (2009). Identifying the land-based sources of suspended sediments, nutrients and pesticides discharged to the Great Barrier Reef from the Tully–Murray Basin, Queensland, Australia. Mar. Freshw. Res. 60, 1081–1090. doi: 10.1071/MF08333
Baran, E., Van Zalinge, N., and Bun, N. P. (2001). “Floods, floodplains and fish production in the Mekong basin: present and past trends,” in Proceedings of the Second Asian Wetlands Symposium, Penang, 27–30.
Barbier, E. B. (2013). Valuing ecosystem services for coastal wetland protection and restoration: progress and challenges. Resources 2, 213–230. doi: 10.3390/resources2030213
Bell-James, J., and Lovelock, C. (in press). “Tidal boundaries and climate change mitigation – the curious case of ponded pastures,” in Australian Property Law Journal.
Boulton, A. J., Ekebom, J., and Gislason, G. M. (2016). Integrating ecosystem services into conservation strategies for freshwater and marine habitats: a review. Aquat. Conserv. Mar. Freshw. Ecosyst. 26, 963–985. doi: 10.1002/aqc.2703
Brodie, J., and Waterhouse, J. (2012). A critical review of environmental management of the ‘not so Great’ Barrier Reef. Estuar. Coast. Shelf Sci. 104–105, 1–22. doi: 10.1016/j.ecss.2012.03.012
Broszeit, S., Beaumont, N. J., Uyarra, M. C., Heiskanen, A.-S., Frost, M., Somerfield, P. J., et al. (2017). What can indicators of good environmental status tell us about ecosystem services: Reducing efforts and increasing cost-effectiveness by reapplying biodiversity indicator data. Ecol. Indic. 81, 409–442. doi: 10.1016/j.ecolind.2017.05.057
Buijse, A. D., Coops, H., Staras, M., Jans, L., Van Geest, G., Grift, R., et al. (2002). Restoration strategies for river floodplains along large lowland rivers in Europe. Freshw. Biol. 47, 889–907. doi: 10.1046/j.1365-2427.2002.00915.x
Bulleri, F., and Chapman, M. G. (2010). The introduction of coastal infrastructure as a driver of change in marine environments. J. Appl. Ecol. 47, 26–35. doi: 10.1111/j.1365-2664.2009.01751.x
Bunn, S., Davies, P., Kellaway, D., and Prosser, I. (1998). Influence of invasive macrophytes on channel morphology and hydrology in an open tropical lowland stream, and potential control by riparian shading. Freshw. Biol. 39, 171–178. doi: 10.1046/j.1365-2427.1998.00264.x
Burrows, D. W., and Butler, B. (2007). “Determining end-point goals and effective strategies for rehabilitation of coastal wetlands: examples from the Burdekin River, North Queensland,” in Proceedings of the 5th Australian Stream Management Conference: Australian Rivers: Making a Difference, eds A. L. Wilson, R. L. Deehan, R. J. Watts, K. J. Page, K. H. Bower, and A. Curtis (Albury, NSW: Institute for Land, Water and Society, Charles Sturt University), 49–54.
Butler, B., Burrows, D. W., and Pearson, R. G. (2007). Providing Regional NRM with Improved Aquatic Health Risk Assessment and Monitoring Tools: The Nationally Significant Indicator – Dissolved Oxygen. ACTFR Report No. 07/31. Australian Centre for Tropical Freshwater Research (ACTFR). Townsville: James Cook University.
Butler, J. R., Wong, G. Y., Metcalfe, D. J., Honzák, M., Pert, P. L., Rao, N., et al. (2013). An analysis of trade-offs between multiple ecosystem services and stakeholders linked to land use and water quality management in the Great Barrier Reef, Australia. Agric. Ecosyst. Environ. 180, 176–191. doi: 10.1016/j.agee.2011.08.017
Coleman, J. M., Huh, O. K., and Braud, D. Jr. (2008). Wetland loss in world deltas. J. Coast. Res. 24, 1–14. doi: 10.2112/05-0607.1
Commonwealth of Australia (2015). Reef Trust Partnerships for the Reef. Canberra: Commonwealth of Australia.
Commonwealth of Australia (2016). Reef 2050 Plan - Investment Framework. Canberra: Commonwealth of Australia.
Connolly, N., Kahler, C., Mackay, S., Fry, S., and Cameron, R. (2012). Variations in Wetland Condition Across Land Zones in the Lower Burdekin. Aquatic Weed Distributions Determined by Underlying Differences in Water and Salinity Regimes. A report prepared for the NQ Dry Tropics NRM. Brisbane: Department of Environment and Heritage Protection, 50.
Creighton, C., Boon, P. I., Brookes, J. D., and Sheaves, M. (2015). Repairing Australia’s estuaries for improved fisheries production–what benefits, at what cost? Mar. Freshw. Res. 66, 493–507. doi: 10.1071/MF14041
Creighton, C., Hobday, A. J., Lockwood, M., and Pecl, G. T. (2016). Adapting management of marine environments to a changing climate: a checklist to guide reform and assess progress. Ecosystems 19, 187–219. doi: 10.1007/s10021-015-9925-2
Davidson, N. C. (2014). How much wetland has the world lost? Long-term and recent trends in global wetland area. Mar. Freshw. Res. 65, 934–941. doi: 10.1071/MF14173
Davis, A. M., Lewis, S. E., O’Brien, D. S., Bainbridge, Z. T., Bentley, C., Mueller, J. F., et al. (2014). “Water resource development and high value coastal wetlands on the lower Burdekin floodplain, Australia,” in Estuaries of Australia in 2050 and Beyond, ed. E. Wolanski (Dordrecht: Springer), 223–245.
Department of Environment Heritage and Protection [DEHP] (2016). Wetlands in the Great Barrier Reef Catchment Management Strategy 2016-2021. Brisbane: Department of Environment Heritage and Protection.
Dobson, M., Magana, A. M., Lancaster, J., and Mathooko, J. M. (2007). Aseasonality in the abundance and life history of an ecologically dominant freshwater crab in the Rift Valley, Kenya. Freshw. Biol. 52, 215–225. doi: 10.1111/j.1365-2427.2006.01648.x
Dubuc, A., Waltham, N., Malerba, M., and Sheaves, M. (2017). Extreme dissolved oxygen variability in urbanised tropical wetlands: the need for detailed monitoring to protect nursery ground values. Estuar. Coast. Shelf Sci. 198, 163–171. doi: 10.1016/j.ecss.2017.09.014
Elliott, M., Mander, L., Mazik, K., Simenstad, C., Valesini, F., Whitfield, A., et al. (2016). Ecoengineering with ecohydrology: successes and failures in estuarine restoration. Estuar. Coast. Shelf Sci. 176, 12–35. doi: 10.1016/j.ecss.2016.04.003
Elliott, M., and Whitfield, A. K. (2011). Challenging paradigms in estuarine ecology and management. Estuar. Coast. Shelf Sci. 94, 306–314. doi: 10.1016/j.ecss.2011.06.016
Finlayson, C. M. (2013). Climate change and the wise use of wetlands: information from Australian wetlands. Hydrobiologia 708, 145–152. doi: 10.1007/s10750-013-1474-0
Francis, A., and Tait, J. (2006). Wetland Prioritisation Decision Support System Great Barrier Reef Catchment. Melbourne: Envirosciences.
Great Barrier Reef Marine Park Authority [GBRMPA] (2015). Reef 2050 Long Term Sustainability Plan. Great Barrier Reef Marine Park Authority. Canberra: Commonwealth of Australia.
Guerrero, A. M., Shoo, L., Iacona, G., Standish, R. J., Catterall, C. P., Rumpff, L., et al. (2017). Using structured decision-making to set restoration objectives when multiple values and preferences exist. Restor. Ecol. 25, 858–865. doi: 10.1111/rec.12591
Harris, G. P., and Heathwaite, A. L. (2012). Why is achieving good ecological outcomes in rivers so difficult? Freshw. Biol. 57, 91–107. doi: 10.1111/j.1365-2427.2011.02640.x
Houston, W., Elder, R., Black, R., and McCabe, J. (2006). Conservation significance of coastal wetland habitats for birds at Twelve Mile Creek, Fitzroy River, central Queensland. Sunbird 36, 20–36.
Houston, W., Porter, G., Elder, R., Black, R., and Sheaves, M. (2004). Rediscovery of yellow chats (Capricorn subspecies) on the Fitzroy River delta central Queensland. Sunbird 34, 36–42.
Houston, W. A., Black, R. L., and Elder, R. J. (2013). Distribution and habitat of the critically endangered Capricorn yellow chat Epthianura crocea macgregori. Pac. Conserv. Biol. 19, 39–54. doi: 10.1071/PC130039
Hyland, S. L. (2002). An Investigation of the Impacts of Ponded Pastures on Barramundi and Other Finfish Populations in Tropical Coastal Wetlands. Brisbane: Queensland Government.
Inglis, S. N., and Howell, S. (2009). Aquatic Conservation Assessments (ACA) Using AquaBAMM for the Riverine Wetlands of the Great Barrier Reef Catchment. Brisbane: Department of Environment and Resource Management.
Jaensch, R., Westley, S., and Peter, S. (2015). A Priortisation of Fitzroy Basin Wetlands for NRM Investment. Report for Fitzroy Basin Association. Rockhampton: Fitzroy Basin Association.
Jia, Z., Tang, S., Luo, W., and Hai, Y. (2016). Water quality improvement through five constructed serial wetland cells and its implications on nonpoint-source pollution control. Hydrol. Sci. J. 61, 2946–2956. doi: 10.1080/02626667.2016.1171323
Kelleway, J. J., Cavanaugh, K., Rogers, K., Feller, I. C., Ens, E., Doughty, C., et al. (2017). Review of the ecosystem service implications of mangrove encroachment into salt marshes. Glob. Chang. Biol. 23, 3967–3983. doi: 10.1111/gcb.13727
King, A., Humphries, P., and Lake, P. (2003). Fish recruitment on floodplains: the roles of patterns of flooding and life history characteristics. Can. J. Fish. Aquat. Sci. 60, 773–786. doi: 10.1139/f03-057
Kozak, J. P., and Piazza, B. P. (2015). A proposed process for applying a structured decision-making framework to restoration planning in the Atchafalaya River Basin, Louisiana, USA. Restor. Ecol. 23, 46–52. doi: 10.1111/rec.12125
Kroon, F., Robinson, C., and Dale, A. (2009). Integrating knowledge to inform water quality planning in the Tully–Murray basin, Australia. Mar. Freshw. Res. 60, 1183–1188. doi: 10.1071/MF08349
Maes, J., Paracchini, M., Zulian, G., Dunbar, M., and Alkemade, R. (2012). Synergies and trade-offs between ecosystem service supply, biodiversity, and habitat conservation status in Europe. Biol. Conserv. 155, 1–12. doi: 10.1016/j.biocon.2012.06.016
Mitsch, W. J., Bernal, B., Nahlik, A. M., Mander,Ü, Zhang, L., Anderson, C. J., et al. (2013). Wetlands, carbon, and climate change. Landsc. Ecol. 28, 583–597. doi: 10.1007/s10980-012-9758-8
Moore, H. E., and Rutherfurd, I. D. (2017). Lack of maintenance is a major challenge for stream restoration projects. River Res. Appl. 33, 1387–1399. doi: 10.1002/rra.3188
Paul, K. I., Bartley, R., Larmour, J. S., Micah, J., Davis, J., Crawford, D., et al. (2018). Optimising the Management of Riparian Zones to Improve the Health of the Great Barrier Reef. Report to the National Environmental Science Program. Cairns: Reef and Rainforest Research Centre Limited.
Pearson, R., Godfrey, P., Arthington, A., Wallace, J., Karim, F., and Ellison, M. (2013). Biophysical status of remnant freshwater floodplain lagoons in the Great Barrier Reef catchment: a challenge for assessment and monitoring. Mar. Freshw. Res. 64, 208–222. doi: 10.1071/MF12251
Perna, C., and Burrows, D. W. (2005). Improved dissolved oxygen status following removal of exotic weed mats in important fish habitat lagoons of the tropical Burdekin River floodplain, Australia. Mar. Pollut. Bull. 51, 138–148. doi: 10.1016/j.marpolbul.2004.10.050
Perna, C. N., Cappo, M., Pusey, B. J., Burrows, D. W., and Pearson, R. G. (2012). Removal of aquatic weeds greatly enhances fish community richness and diversity: an example from the Burdekin River floodplain, tropical Australia. River Res. Appl. 28, 1093–1104. doi: 10.1002/rra.1505
Pert, P., Butler, J., Brodie, J., Bruce, C., Honzak, M., Kroon, F., et al. (2010). A catchment-based approach to mapping hydrological ecosystem services using riparian habitat: a case study from the Wet Tropics, Australia. Ecol. Complex. 7, 378–388. doi: 10.1016/j.ecocom.2010.05.002
Pettit, N. E., Bayliss, P., and Bartolo, R. (2018). Dynamics of plant communities and the impact of saltwater intrusion on the floodplains of Kakadu National Park. Mar. Freshw. Res. 69, 1124–1133. doi: 10.1071/MF16148
Queensland Wetlands Program (2013). Bowling Green Bay - a Wetland of International Importance. Brisbane: Queensland Government.
Rassam, D., and Pagendam, D. (2009). Development and application of the Riparian Mapping Tool to identify priority rehabilitation areas for nitrogen removal in the Tully–Murray basin, Queensland, Australia. Mar. Freshw. Res. 60, 1165–1175. doi: 10.1071/MF08358
Rayner, T. S., Pusey, B. J., and Pearson, R. G. (2009). Seasonal flooding, instream habitat structure and fish assemblages in the Mulgrave River, north-east Queensland: towards a new conceptual framework for understanding fish-habitat dynamics in small tropical rivers. Mar. Freshw. Res. 59, 97–116. doi: 10.1071/MF07129
Reid, B., Grice, T., Holtum, J., Nicholas, M., Wallace, J., and Waltham, N. (2018). Assessing the Potential for Controlling Four Invasive Species (Aleman Grass, Water Hyacinth, Olive Hymenachne and Para Grass) Using Seawater in Northern Australian Coastal Wetlands. Centre for Tropical Water & Aquatic Ecosystem Research (TropWATER) Report No. 18/31. Townsville: James Cook University.
Russell, D., and McDougall, A. (2005). Movement and juvenile recruitment of mangrove jack, Lutjanus argentimaculatus (Forsskål), in northern Australia. Mar. Freshw. Res. 56, 465–475. doi: 10.1071/MF04222
Sheaves, M. (2009). Consequences of ecological connectivity: the coastal ecosystem mosaic. Mar. Ecol. Prog. Ser. 391, 107–115. doi: 10.3354/meps08121
Sheaves, M. (2016). Simple processes drive unpredictable differences in estuarine fish assemblages: baselines for understanding site-specific ecological and anthropogenic impacts. Estuar. Coast. Shelf Sci. 170, 61–69. doi: 10.1016/j.ecss.2015.12.025
Sheaves, M., Brookes, J., Coles, R., Freckelton, M., Groves, P., Johnston, R., et al. (2014). Repair and revitalisation of Australia× s tropical estuaries and coastal wetlands: opportunities and constraints for the reinstatement of lost function and productivity. Mar. Policy 47, 23–38. doi: 10.1016/j.marpol.2014.01.024
Sheaves, M., and Johnston, R. (2010). Implications of spatial variability of fish assemblages for monitoring of Australia’s tropical estuaries. Aquat. Conserv. Mar. Freshw. Ecosyst. 20, 348–356. doi: 10.1002/aqc.1093
Sheaves, M., Johnston, R., and Connolly, R. M. (2010). Temporal dynamics of fish assemblages of natural and artificial tropical estuaries. Mar. Ecol. Prog. Ser. 410, 143–157. doi: 10.3354/meps08655
Sheaves, M., Sporne, I., Dichmont, C. M., Bustamante, R., Dale, P., Deng, R., et al. (2016). Principles for operationalizing climate change adaptation strategies to support the resilience of estuarine and coastal ecosystems: an Australian perspective. Mar. Policy 68, 229–240. doi: 10.1016/j.marpol.2016.03.014
State of Queensland (2017). Broadening and Enhancing Reef Protection Regulations. Consultation Regulartory Impact Statement. Brisbane: State of Queensland.
State of Queensland (2018b). Reef 2050 Water Quality Improvement Plan. Brisbane: Queensland Government.
Villamagna, A. M., Angermeier, P. L., and Bennett, E. M. (2013). Capacity, pressure, demand, and flow: a conceptual framework for analyzing ecosystem service provision and delivery. Ecol. Complex. 15, 114–121. doi: 10.1016/j.ecocom.2013.07.004
Wallace, J., Waltham, N., Burrows, D., and McJannet, D. (2015). The temperature regimes of dry-season waterholes in tropical northern Australia: potential effects on fish refugia. Freshw. Sci. 34, 663–678. doi: 10.1086/681278
Waltham, N., and Fixler, S. (2017). Aerial herbicide spray to control invasive water hyacinth (Eichhornia crassipes): water quality concerns fronting fish occupying a tropical floodplain wetland. Trop. Conserv. Sci. 10:1940082917741592. doi: 10.1177/1940082917741592
Waltham, N. J., and Schaffer, J. R. (2018). Thermal and asphyxia exposure risk to freshwater fish in feral-pig-damaged tropical wetlands. J. Fish Biol. 93, 723–728. doi: 10.1111/jfb.13742
Waltham, N. J., and Sheaves, M. (2015). Expanding coastal urban and industrial seascape in the great barrier reef world heritage area: critical need for coordinated planning and policy. Mar. Policy 57, 78–84. doi: 10.1016/j.marpol.2015.03.030
Waltham, N. J., Wegscheidl, C. J., Smart, J. C. R., Volders, A., Hasan, S., and Waterhouse, J. (2017). Scoping Land Conversion Options for High DIN Risk, Low-Lying Sugarcane, to Alternative Use for Water Quality Improvement in Wet Tropics Catchments. Report to the National Environmental Science Programme. Cairns: Reef and Rainforest Research Centre Limited, 142.
Waterhouse, J., Brodie, J., Lewis, S., and Audas, D. M. (2016). Land-sea connectivity, ecohydrology and holistic management of the Great Barrier Reef and its catchments: time for a change. Ecohydrol. Hydrobiol. 16, 45–57. doi: 10.1016/j.ecohyd.2015.08.005
Wegscheidl, C. J., Sheaves, M., McLeod, I. M., Hedge, P. T., Gillies, C. L., and Creighton, C. (2017). Sustainable management of Australia’s coastal seascapes: a case for collecting and communicating quantitative evidence to inform decision-making. Wetl. Ecol. Manag. 25, 3–22. doi: 10.1007/s11273-016-9515-x
WetlandInfo (2016). Case Study: Integrating High Value Grazing and Wetland Management on the Torilla Plain. Brisbane: Queensland Government.
Keywords: estuaries, floodplains, restoration, fisheries, water quality, connectivity
Citation: Waltham NJ, Burrows D, Wegscheidl C, Buelow C, Ronan M, Connolly N, Groves P, Marie-Audas D, Creighton C and Sheaves M (2019) Lost Floodplain Wetland Environments and Efforts to Restore Connectivity, Habitat, and Water Quality Settings on the Great Barrier Reef. Front. Mar. Sci. 6:71. doi: 10.3389/fmars.2019.00071
Received: 30 November 2018; Accepted: 07 February 2019;
Published: 26 February 2019.
Edited by:
Mario Barletta, Departamento de Oceanografia da Universidade Federal de Pernambuco (UFPE), BrazilReviewed by:
A. Rita Carrasco, Universidade do Algarve, PortugalJacob L. Johansen, New York University Abu Dhabi, United Arab Emirates
Copyright © 2019 Waltham, Burrows, Wegscheidl, Buelow, Ronan, Connolly, Groves, Marie-Audas, Creighton and Sheaves. This is an open-access article distributed under the terms of the Creative Commons Attribution License (CC BY). The use, distribution or reproduction in other forums is permitted, provided the original author(s) and the copyright owner(s) are credited and that the original publication in this journal is cited, in accordance with accepted academic practice. No use, distribution or reproduction is permitted which does not comply with these terms.
*Correspondence: Nathan J. Waltham, TmF0aGFuLndhbHRoYW1AamN1LmVkdS5hdQ==