The Effect of Hydrological Connectivity on Fish Assemblages in a Floodplain System From the South-East Gulf of California, Mexico
- 1Instituto de Ciencias del Mar y Limnología, Universidad Nacional Autónoma de México, Unidad Académica Mazatlan, Mazatlan, Mexico
- 2Marine Biology Research Group, Ghent University, Ghent, Belgium
- 3Instituto de Ciencias del Mar y Limnología, Universidad Nacional Autónoma de México, Mexico City, Mexico
The effect of hydrological connectivity of the fish assemblages was assessed on a floodplain in the SE Gulf of California, Mexico using a before–after control-impact (BACI) design. Community structure attributes of species abundance, biomass, richness, diversity, and differences in the structure of fish assemblages were compared between two periods (January to June in 2011 and 2015) and two flood plains, one designated as the control zone which was divided by a road, and another one designated as the treatment zone which from January to June 2011 was divided by a road, and then sampled from January to June 2015, as the site was rehabilitated by removing the road in the year 2012. Fish were sampled at monthly intervals using a seine net at different stations in both floodplains. A total of 7024 organisms, comprising of 14 species belonging to 11 families, were analyzed. In the control zone during both periods and in the treatment zone before removal of the road Poecilia butleri was the most abundant species and two exotic species (Tilapia) accounted for more than 95% of the total biomass. After the removal of the road in the treatment zone Poeciliopsis latidens was the most abundant species and the relative biomass of both species of tilapia decreased to 85%, but was still the most important in terms of biomass. However, richness and diversity were higher and the structure of the fish assemblages was different in the treatment zone after the road was removed, due to the presence of species with estuarine and marine affinity in this zone and period. Our results indicate that hydrological connectivity proved to be a key factor influencing the fish composition and abundance in the floodplain system in the region. Hence, a better hydrological connectivity implied higher abundance and diversity, likely related to an increase in the habitat complexity. The priority in the management of the ecological functioning of the floodplain system should be set on minimizing the modifications of the natural flow and thus avoiding the changes on the fish biota.
Introduction
Seasonal coastal floodplains along tropical latitudes are amongst the most biologically productive and diverse ecosystems on earth (Tockner and Stanford, 2002; Junk et al., 2006) and are an important component of rivers and wetlands, acting as a nursery habitat and refuge for fish (Junk and Wantzen, 2004). These dynamic systems are seasonally flooded during flow and ebb currents resulting on water level variations. Particularly, wetlands on estuary floodplains are dynamic entities, driven by connections of tidal habitats and freshwater flooding which can have positive consequences for the fish inhabiting these systems, and from the ecological point of view, these ecosystems play an important role in improving the water quality by filtering and recycling nutrients, storing sediments, and supporting high biodiversity (Junk et al., 1989; Bayley, 1995). Also, considering that tropical floodplain systems are home to diverse fish species representing an important source of protein for human populations (Welcomme, 2001), these sites also possess an economic importance, which is altered seasonally depending on the wet and dry seasons. The response of the fish to these variations is expressed in particular adaptations across the seasonal floodplain (Bayley, 1995). During the wet season the inundation covers large areas and thereby increases the availability of food and shelter for fishes and other organisms (Welcomme, 2001). Contrary, during the dry season the floodplains are prone to an alteration of the hydrological connectivity (Jardine et al., 2012), which limits the fish movement and certainly provokes the mortality of many organisms trapped in dried habitats.
The estuarine complex of Marismas Nacionales is located in the alluvial plain of the States of Nayarit and southern Sinaloa on the North-Central Pacific coast of Mexico. This large complex comprises approximately 175,300 ha of mangrove wetlands, saltwort (Salicomia spp. and Balis maritima), vegetated and un-vegetated extensive seasonal flood plains, including some saltpans, coastal lagoons, and tidal channels (Ramírez Zavala et al., 2012). It is estimated that this region alone accounts for 50–70% of Mexico’s annual small-scale fisheries production (Spalding et al., 2007). However a high percentage of its ecosystems are under threat (Tockner et al., 2008) and despite its low population density there is still increasing pressure on the floodplains and wetlands in this region due to human activities. The continued decline of floodplain and wetland ecosystems is mainly caused by habitat alteration (Taylor et al., 2007), transforming these areas into agriculture or aquaculture fields, and tourism developments (Páez-Osuna et al., 1999; Glenn et al., 2006). Additionally, hydrological modifications such as construction of marinas, channels, coastal erosion, dam infrastructure, and urban development are also an important threat for these coastal ecosystems (Giri et al., 2011). As a consequence habitat diversity patterns are strongly affected (Bunn and Arthington, 2002) because the construction of these structures often changes the rate of the flow (Sparks et al., 1998). At present different floodplains in this area have their flooding seasonality altered and get less water than in the past (Kingsford, 2000). This anthropogenic activity may lead to the ecosystem fragmentation, which could potentially cause the isolation of some populations by limiting the migrations and/or species movements, but on the other hand exotic species can benefit from the stabilization of the habitat and invade the system (Bunn and Arthington, 2002). As a result of these modifications many ecological benefits of the floodplains may be restricted.
The awareness of the value of seasonal floodplains has gradually increased (Ratti et al., 2001) and there is a need to integrate the sustainable management of seasonal floodplains and their associated fishes. Firstly, it is important to understand the relationship between the habitats and fishes, not only for sustainable fisheries but for the overall management of these ecosystems. One of the strategies of how to conserve and preserve these systems is through habitat restoration (Ormerod, 2003). Restoration has been suggested as a mechanism for enhancing the fisheries/higher biodiversity in degraded areas (Levings, 1991). In order to implement successful restoration strategies it has been agreed that hydrology is key (Wolanski et al., 1992). The enhancement of the hydrological connectivity is one of the techniques for the ecosystem rehabilitation which aims to restore the natural component of the flow (Arthington et al., 2010). Most of these enhancement projects are focused on wildlife and are rarely designed to benefit fish communities, therefore there are gaps in the understanding of the use of seasonal floodplains by fishes. However, in general, higher spatial connectivity contributes to the creation of better conditions for fish, shrimps, and other fishing resources (Rozas et al., 2013). Several studies have investigated the relation between hydrological connectivity, wetland characteristics, and the fish community with the conclusion that connectivity is a key factor determining the diversity and structure of fish assemblages (e.g., Lasne et al., 2007; Pearson et al., 2011). Likewise, it has been proven that a better hydrologic connectivity increases the diversity of native fish species, whilst the number of exotic species increases with isolation (Lasne et al., 2007). Isolation could alter the aquatic environment, thereby increasing hypoxia and indirectly affecting the structure of fish assemblages (Rozas et al., 2013). However, even though the rehabilitation process has been widely used over the broad range of riverine and wetland ecosystems resulting in a positive outcome, within the field of conservation biology hydrologic connectivity remains a largely neglected dimension.
The present study assessed the influence of attributes of hydrological connectivity based on a road removal performed in October 2012 on the fish assemblages in a subtropical seasonal floodplain. Rural communities in the studied region, such as fishing villages, constructed many roads through the floodplains during the dry season in order to access suitable locations for their small fishing boats. These dirt roads were usually built at 50 to 80 cm above the maximum local tidal amplitude in order to avoid flooding during the spring tides. The specific dirt road in this study consisted of a pathway for off-road vehicles that was abandoned for unknown reasons. The hypothesis is that the lack of hydrological communication created a less suitable environment for the fish community, and thus local fishermen abandoned this seasonal floodplain in order to find a more suitable location. Consequently, it was decided to remove this abandoned dirt road in order to assess the diversity of fish species by enhancing tidal hydrological connectivity. The fish response was measured as the abundance, diversity, and structure of the fish assemblages before and after the road was removed. The working hypothesis was that opening of the channel through the removal of the road would increase the hydrologic connectivity in the system, therefore increasing the fish abundance and diversity, and altering the structure of the fish assemblage. In order to do this a before–after control-impact (BACI) design was employed.
Materials and Methods
Study Area
The study was conducted in the salt marsh of Las Cabras (Figure 1), located on an alluvial plain in the State of Sinaloa, within the Marismas Nacionales complex. The study area presents a hot semiarid-climate (BSh) (García, 1998). Mean annual air temperature ranges from 24 to 28°C and the annual total precipitation, which occurs between the months of July and September, ranges between 900 and 1,300 mm (INEGI and Gobierno del Estado de Sinaloa, 1999). The salt marsh Las Cabras is a seasonal floodplain of approximately 60 km2, with no direct connection to the adjacent Pacific Ocean. The underground salt wedge that passes through the coastal sand barrier depends on the local tide, which presents a semi-diurnal pattern with maximum amplitude of 1.8 m in spring tides during the summer months of June to September. This subtropical semiarid saltmarsh includes multiple tidal creeks, seasonal flood plains, and an extensive saltpan area with hypersaline conditions (Flores-Verdugo et al., 1993).
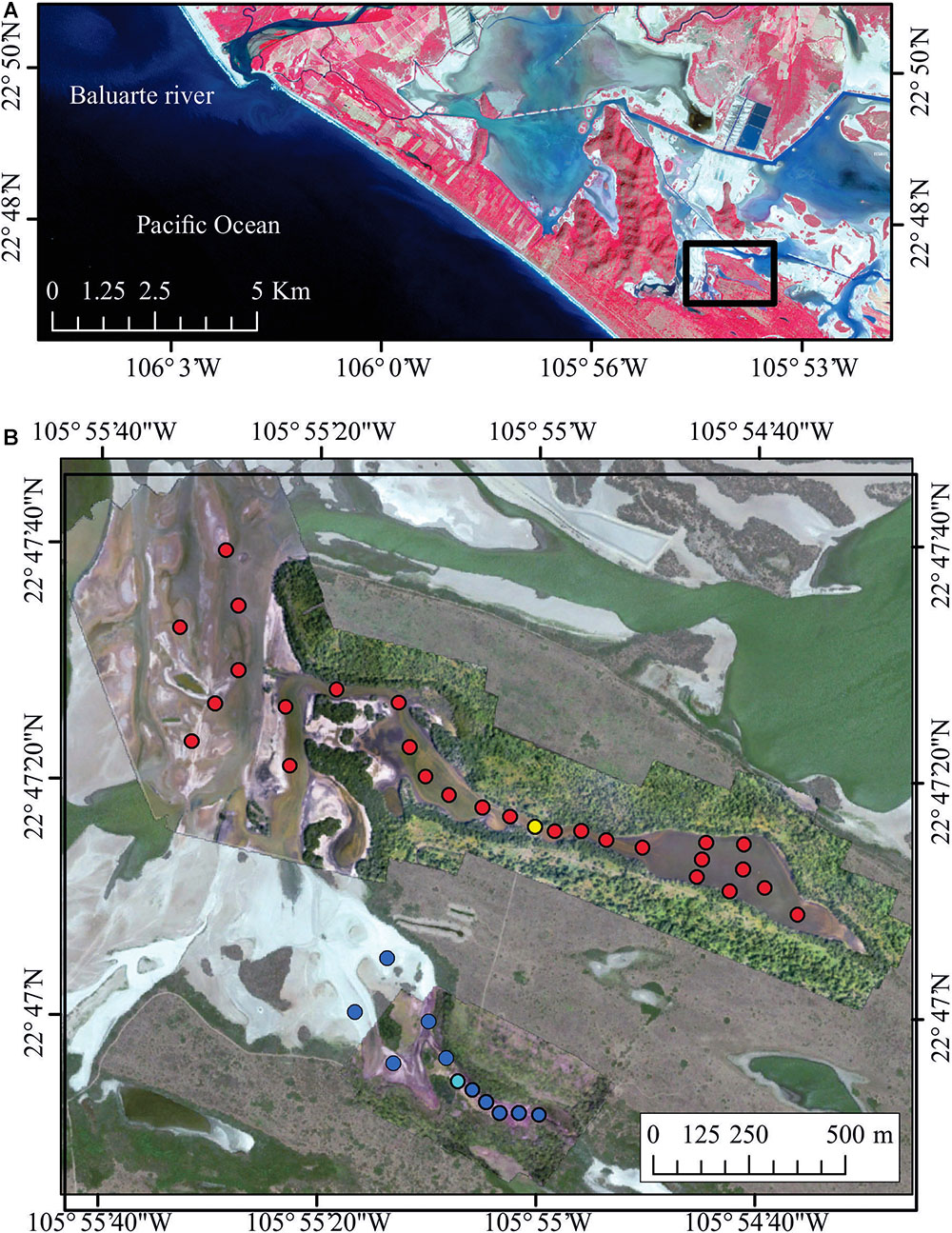
Figure 1. (A) Study site (Las Cabras floodplain), in the southeast Gulf of California (enhanced near infrared, red, and green composition from a Sentinel-2 image). (B) Detailed digital image of the sampling sites. Red dots indicate the zones where the fish were collected, and yellow point indicates the place where the road was placed in the treatment area. Dark blue dots indicate the zones where the fish were collected, and light blue dot point indicates the place where the road is still placed in the control area.
Black mangrove (Avicennia germinans), white mangrove (Laguncularia racemosa), and buttonwood (Conocarpus erectus) are commonly found where topographic profile is regular, and flood and ebb currents occur regularly without retaining standing water. Conversely, a depression is commonly found where there is a more complex geomorphology, such as the Easter section of this area, where tidal influence is minimal, creating a less frequent inundation zone with an extensive community of Batis sp. and Salicornia sp. Consequently, these saltmarshes have hypersaline pore-water conditions for much of the year (Flores Verdugo et al., 2007). The remaining terrestrial vegetation consists of dry deciduous forest and palm trees at relatively higher elevations.
Sampling Design
In order to perform an assessment of the effect of the road removal on the fish assemblage’s diversity and structure a BACI design was employed. This design consists of measurements taken at the treatment (impacted) site and at a control site both before and after the impact occurs (Smokorowski and Randall, 2017). Thus, there are a total of N observations with multiple observations over time or space, and the resulting data can be analyzed with a factorial ANOVA (Green and Green, 1979). Usually this design is used to evaluate environmental impacts due to anthropogenic induced changes. However, in this case, it was used to evaluate a restoration effort.
The region of our study went through degradation and now it is in the process of restoration through hydrological rehabilitation. In the analyzed flood plain (i.e., the impacted area) rehabilitation was initiated in 2012 when a road was removed, connecting habitats and enhancing the water circulation in this ecosystem. Further rehabilitation includes planting of mangrove seedlings and construction of new channels at other sites nearby. However, due to its recent progress and locations these factors were not considered in the present study.
In the same region there is a flood plain which is divided by a dirt road which has remained there since it was constructed during the 1990s (i.e., control area).
As the area where this flood plain is located consists of private lands, with very limited access, it was only possible to conduct the study at two different time intervals; monthly from January to June 2011, before the road was removed, and monthly from January to June 2015, after the road was removed. A total of 27 stations were sampled each month along the impacted/restored seasonal floodplain, and 10 stations were sampled each month in the control area (Figure 1). In the impacted area there was limited water circulation before the road was removed, and the only connection was through a passage with a diameter of approximately 1 m. In the control area the connection to the main body of the floodplain was also through a channel located under the road with a diameter of about 1 m.
Environmental Measurements
The environmental factors of dissolved oxygen (mg/l), salinity (ppm), depth (m), and temperature (°C) were recorded at each station in each sampling month using a YSI multiparameter.
Fish Sampling and Laboratory Processing
The fish were sampled with seine nets (70 m in length, 3.4 m in height, and 1 cm mesh size) hauled by four people at each station in each sampling month. After each fishing operation fish were kept on ice and transported to the laboratory. In the laboratory, fish were taxonomically identified to species, counted, weighted to the nearest 0.01 g, and measured (total length in cm).
Data Analysis
The initial and end positions were recorded with a GPS in order to estimate the mean catch per unit effort (CPUE). This was computed for every station and was used for all analyses. This index was obtained according to the method proposed by Viana et al. (2010) by estimating the number of individuals (n) according to the equation: y = 100 n(A)-1, where A is area swept.
A randomized cumulative species curve was constructed for every year and every location sampled to determine if sample sizes were sufficient to describe the total number of species from our modeled samples (Flather, 1996). The order in which samples were analyzed was randomized 1000 times, for each new cumulative species sample using Chao’s estimator of the absolute number of species in an assemblage. It is based upon the number of rare classes found in a sample (Chao, 1984), and the notation is:
Where Sest is the estimated number of species, Sobs is the observed number of species in the sample, f1 is the number of singleton taxa (taxa represented by a single occurrence in the assemblage), and f2 is the number of doubleton taxa (two or more occurrences in the assemblage). Further details of this method can be found in Magurran (2004).
Each station was treated as a replica; therefore, fish species diversity was estimated for every sampling station at every month, year, and location using the Shannon index of diversity (H′). The form of the index is:
Where pi is the proportion of individuals found in the ith species (Magurran, 2004).
The diversity values were used to perform a simple BACI – 1 year before/after; one site impact; one site control, design, although in this case the time after the event was more than 2 years long. The analysis was performed with a three-factor completely randomized design (three-way ANOVA). According to Magurran (2004), this diversity index follows a normal distribution; therefore, a parametric test can be performed. The factors were location (control and impact floodplain), year (before: 2011, after: 2015), and month (January to June). Homoscedasticity of variances was tested with Cochran’s C test, and a Tukey test was performed in case statistical differences were found to do pairwise comparisons.
Multivariate analyses were also used to test the same BACI design, comparing the fish assemblages between the different locations, months, and years. A matrix containing the sampling month and locality per year as columns, and fish species as rows was created and from this a Bray–Curtis similarity matrix was generated. The factors assigned to this matrix were month, year, and location. To test the H0 that the fish assemblages did not differ according to these factors, a PERMANOVA was employed using the same three-way design as it was used with the ANOVA. If significant results were found the data were graphically represented using a distance based redundancy analysis (dbRDA) (McArdle and Anderson, 2001). This analysis predicts the multivariate variation of the fish assemblages in the months and years in multivariate space. Multivariate analyses and the estimation of the diversity index were completed using the PRIMER 6 statistical package with the PERMANOVA+ add-on (PRIMER-E, Plymouth Marine Laboratory, United Kingdom). All parametric statistical analyses were performed on STATISTICA 13 (TIBCO Software, Inc.).
Results
Environmental Patterns
All the sampling stations were shallow, with a highest water level of 75.2 cm during January 2015, and lowest of 18.5 cm in the restored area during May 2011, and 15.0 cm in the control site during May 2015. During June 2015 at the restored area the depth was of 26.0 cm. During June 2011 in the control and restored area, as well as June 2015 in the control site, the systems were completely dry. In both periods and sites, the temperature behaved similarly with a variation through the sampling months (Figure 2), ranging between 27.7 and 36.9°C during 2011, and lower during 2015, with a range of 24.2–36.2°C. Salinity increased gradually as months passed. Before the opening of the road, and in both periods at the control site the salinity increased from 11.85 ppt in January to 79.3 ppt at the end of June. After the road was removed in the rehabilitation site, salinity ranged from 4 ppt in January to 66.2 ppt in May. Dissolved oxygen (mg/l) decreased as months passed in all sites, from 10.8 in January, to 0.1 mg/1 in June 2011, and from 9.7 in January 2015 to 0.1 mg/1 in June 2015.
Fish Assemblages
A total of 7024 individuals were captured over the course of this study, from 11 families, representing 12 native and 2 exotic species (Tilapia, Cichlidae). The sampled fish collected on the floodplain were represented by both small and large bodied species; however, small-bodied species were dominant. The size range of all fish species was 1.2–33.5 cm (mean = 3.75 cm) before the road was removed, and 1.2 to 43 cm (mean = 4.5 cm) after the road was removed. In both periods and locations, the majority of species in the system were found according to Chao’s model when fitting the species accumulation curve; an asymptote was reached in both periods and both locations (Figure 3).
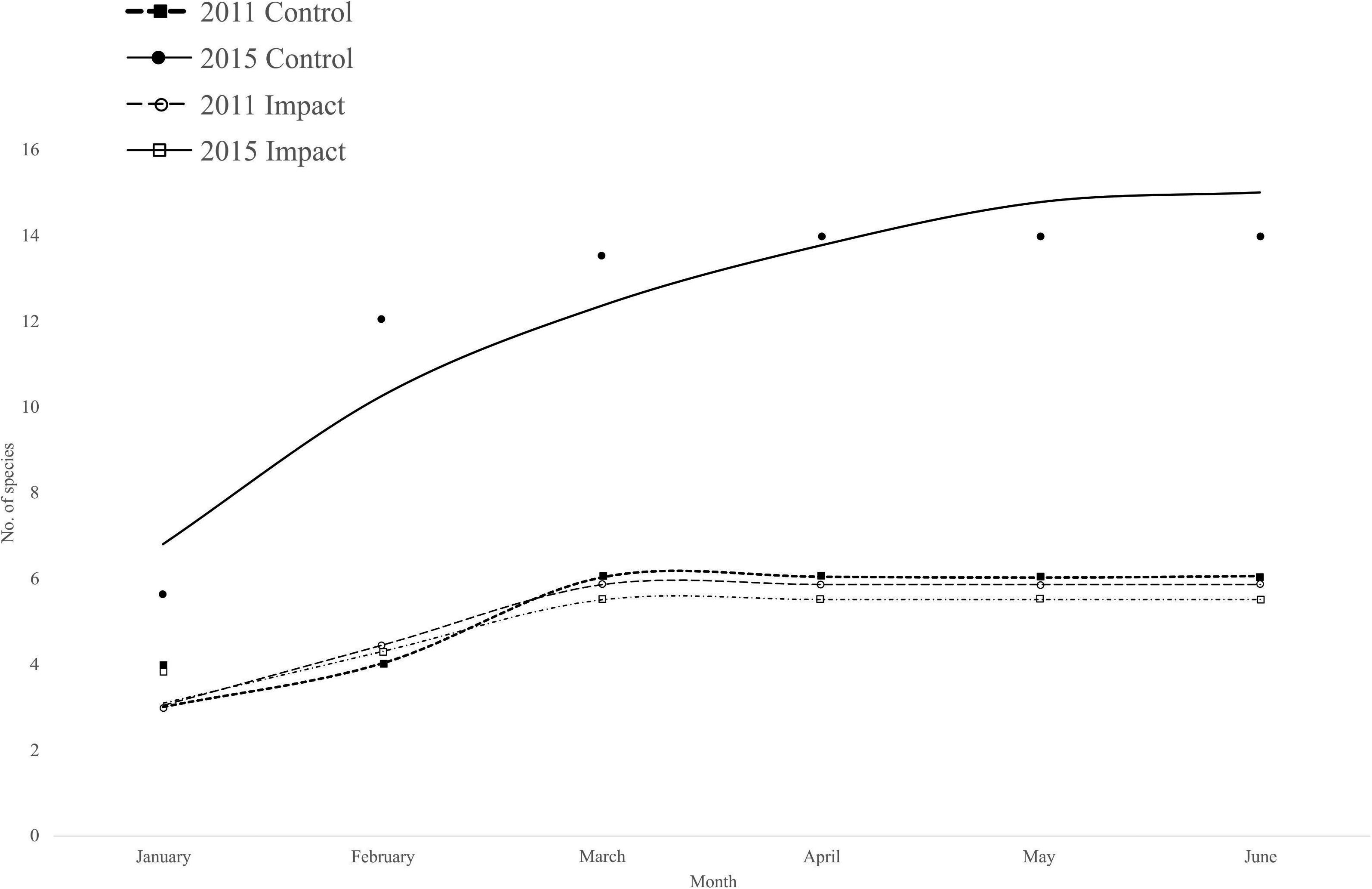
Figure 3. Fish species accumulation model for the two different sampling periods. The model used was Chao.
The number and composition of species in the restored system varied before and after the road was removed. Before the removal of the road six species were captured from four families. Poecilia butleri (48.2%) was the dominating species in terms of numeric abundance, followed by Poeciliopsis latidens (23.9%), Orechromis aureus (22.9%), Orechromis sp. (2.8%), Lile stolifera (0.8%), and Atherinella crystallina (0.03%). However, in terms of total biomass, both species of tilapia were the most important species and accounted for more than 95% of the total biomass (Orechromis aureus: 54.9%, Orechromis sp.: 40.4%).
After the road was removed 14 species from 11 families were recorded. These included the six species found during the previous sampling period plus another eight. The top five in terms of numeric abundance were Poeciliopsis latidens (42.4%), Oreochromis aureus (30.0%), Poecilia butleri (22.1%), Atherinella crystalina (1.5%), and Lile stolifera (1.1%), which accounted for more than 97% of the total abundance. In terms of biomass, both tilapia species remained dominant, but in this period their importance decreased from more than 95% before the road was removed, to approximately 80% after the removal of the road (Orechromis aureus: 44.8%, Orechromis sp.: 35.6%). In 2015 4 other species accounted for 95% of the biomass; these were Poecilia butleri (5.3%), Dormitator latifrons (3.6%), Chanos chanos 3.2%, and Poeciliopsis latidens (2.6%).
In the control site the number of species found during 2011 and 2015 were the same (i.e., 5), and these included the identical ones as found in the restored site during 2011, with the exception of Atherinella crystalina, which was not found in the control site. During 2011, Poecilia butleri was the most numerically abundant (61.4%), followed by Poeciliopsis latidens (22.7%), and Orechromis aureus (14.9%). These three species accounted for 99% of the total relative numeric abundance. In terms of relative weight, both Tilapia species accounted for more than 97% of biomass (Orechromis aureus 51.1%, O. sp. 46.3%), followed by Poeciliopsis latidens (1.4%). Between these three they accounted for almost 99% of the relative biomass during that period of time.
During 2015 at the control site Poecilia butleri was also the most numeric abundant species, but the relative numeric abundance was smaller (45.7%), followed also by Poeciliopsis latidens although during this period, the relative numeric abundance of this species increased to 28.7%. Orechromis aureus showed a relative numeric abundance similar to the previous period (13.8%), but in this year, O. sp. showed a much higher numeric abundance that during 2011 (10.3%). These four species accounted for 98.5% in terms of biomass, both species of Tilapia were also the ones accounting for the higher relative biomass with 98.5% (Oreochromis aureus 61.4%, O. sp. 37.1%). The total number of species found together with their relative numeric abundances and biomasses can be observed in Table 1.
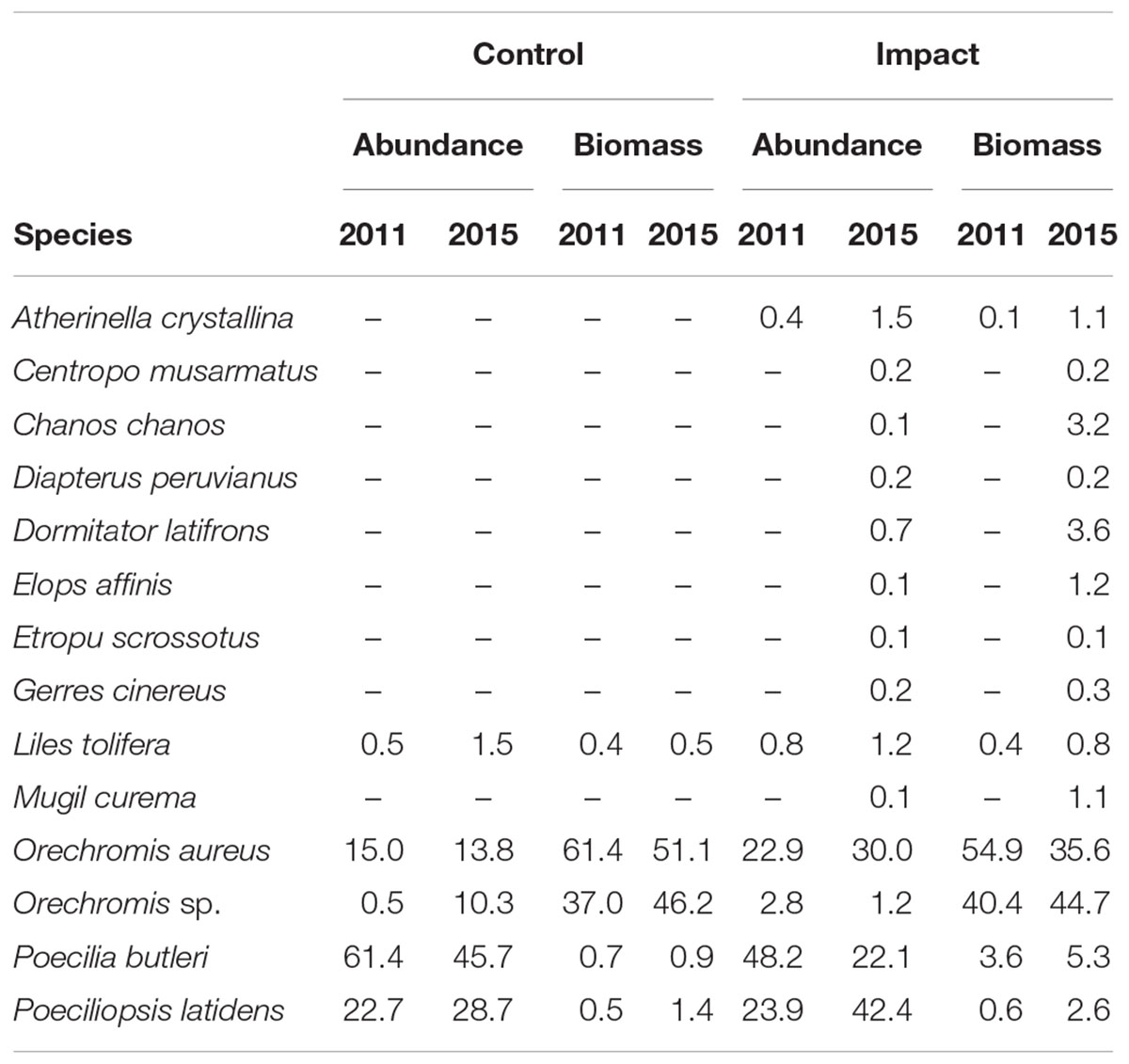
Table 1. Relative numeric abundance and biomass of the fish species found in the system at each period.
Upon examination of fish diversity among both sites, periods, and sampled months, statistical differences were found according to year (F(1,46) = 131.5, p < 0.05), month (F(5,46) = 19.64, p < 0.05), site (F(1,46) = 136.4, p < 0.05), interaction year/month (F(5,46) = 2.5, p < 0.05), interaction year/site (F(1,46) = 18.6, p < 0.05), interaction month/site (F(5,46) = 13.0, p < 0.05), and interaction year/month/site (F(5,46) = 3.2, p < 0.05).
The mean diversity was higher in the restored site in both years; however, it showed a statistically significant increment after the road was removed. In the control site, although the mean diversity was higher for all months during 2015, these differences were not statistically significant (Figure 4, Tukey HSD test p > 0.05). In both sites and years diversity decreased as time passed, always being higher in January, and decreasing toward May and June. The control site was completely dried up in June in both years; therefore, the fish diversity was 0 at that time. The restored site was also dried up in June 2011, and during June 2015 it was very shallow, but there were a few individuals of both Tilapia species inhabiting the system, therefore the diversity index was low, but not 0 (Figure 4).
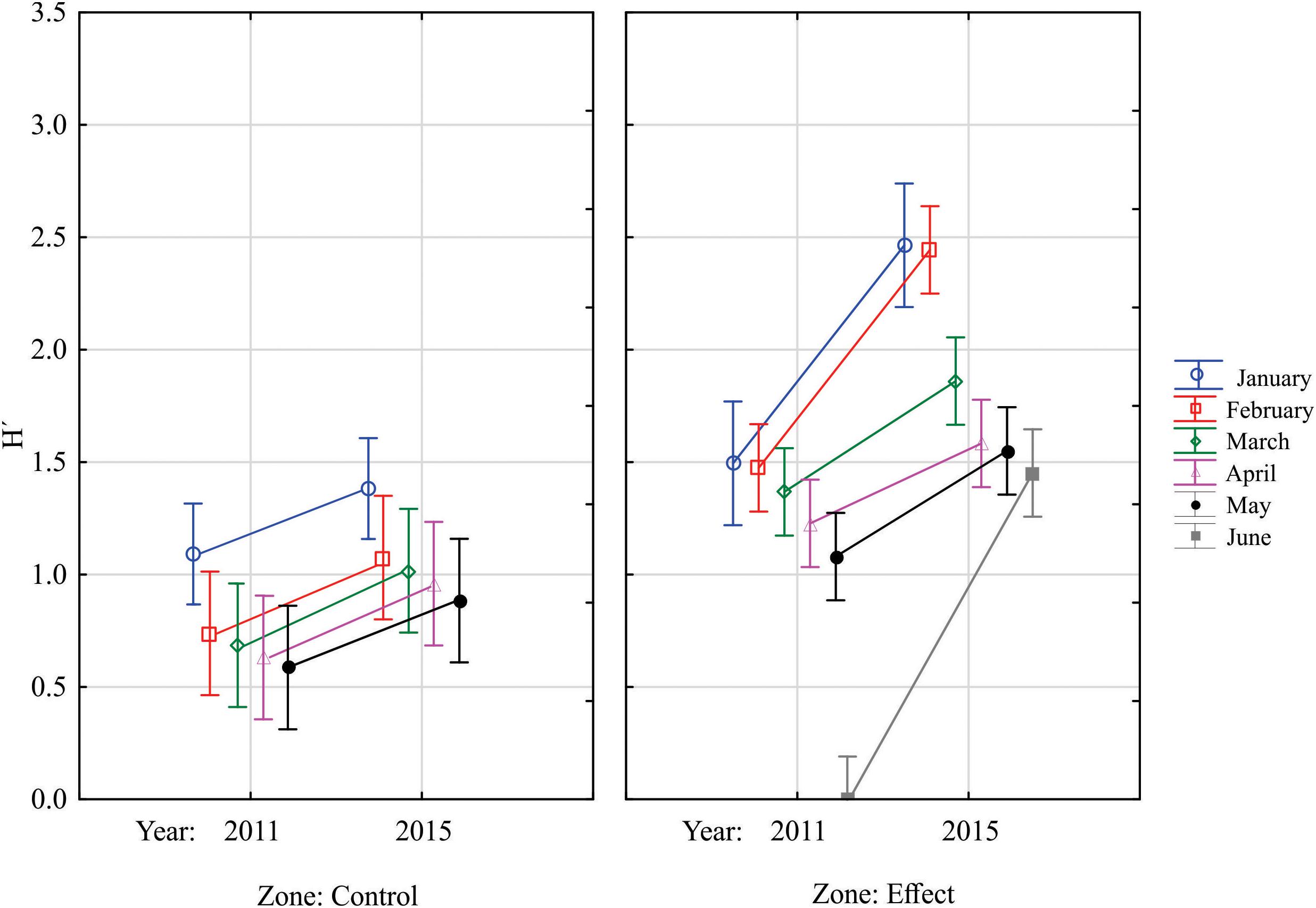
Figure 4. Mean values of the Shannon diversity index in both analyzed sites during the sampled years and months. Vertical bars denote 95% confidence intervals.
General PERMANOVA results were similar to those obtained with the three-way ANOVA, as significant results were also found in the fish assemblages according to year (pseudo-F(1,46) = 16.8, p < 0.05), month (pseudo-F(5,46) = 13.7, p < 0.05), site (pseudo-F(1,46) = 226.3, p < 0.05), interaction year/month (pseudo-F(5,46) = 5.7, p < 0.05), interaction year/site (pseudo-F(1,46) = 21.8, p < 0.05), interaction month/site (pseudo-F(5,46) = 11.2, p < 0.05), and interaction year/month/site (pseudo-F(5,46) = 5.5, p < 0.05).
The dbRDA plot (Figure 5) shows that the fish assemblages were very similar in the control site in both analyzed years, as no clear separation was distinguished from 1 year to another, and no statistical differences were found according to the PERMANOVA test, when testing for differences between years in the control site (Year pseudo-F(1,13) = 2.84, p > 0.05; Month pseudo-F(5,13) = 2.64, p < 0.05; interaction Year-Month pseudo-F(5,13) = 0.65, p > 0.05). These results indicate monthly differences, as previously indicated, but no differences between the analyzed years.
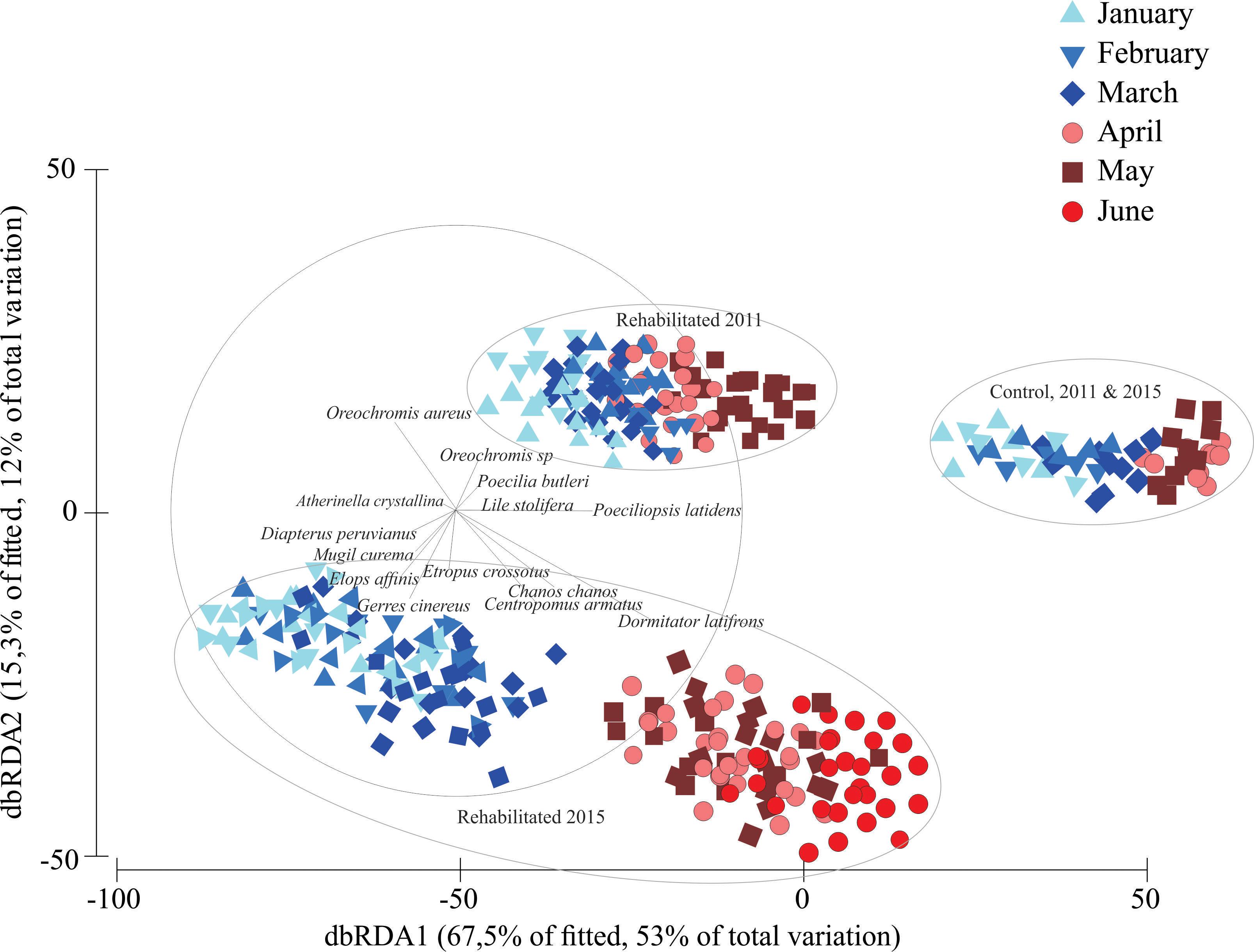
Figure 5. Distance based redundancy analysis (dbRDA) describing the patterns in the fish assemblages in both sampling periods.
The assemblages found in both years in the control site, were also similar to the fish assemblage found in the restored area prior to the road being removed. However, the fish assemblage in the area to be rehabilitated was different to the fish assemblages found in the control site in both years (Site pseudo-F(1,46) = 4.21, p < 0.05).
A clear-cut group containing the fish assemblages in the rehabilitation site after the removal of the road is observed in the lower part of the graph. The fish assemblages were changing chronologically through the sampling months, as a pattern can be seen from January to May or June during 2015 in the rehabilitated zone.
The dbRDA vectors indicate that Poecilia butleri, Poeciliopsis latidens, Lile stolifera, and both Tilapia species were the characteristic species in the control site in both years and during 2011 in the rehabilitated site, prior to the removal of the road. After the road was removed more species appeared in the rehabilitated zone only, and their importance seems to relate to seasonal changes. For example, the species Dormitator latifrons, Chanos chanos, and C. armatus seemed to be characteristic during the warmest months of 2015. These species are known for their tolerance to high salinities and high-water temperatures.
Discussion
Environmental Patterns and Their Effect on Fish Assemblages
Shallow aquatic environments, such as floodplains, are strongly influenced by local driving factors (Thomaz et al., 2007). Therefore, the adaptations and life history of the biota might reflect the temporal environmental changes determined by the seasonal flooding (Cucherousset et al., 2007). In spatially heterogeneous environments such as floodplain mangrove systems, the variability of the accessibility and environmental conditions influence the fish assemblages. The importance of the environmental variables in fish assemblages has been recognized by Jackson et al. (2001). This same pattern was observed in the present study, as abiotic factors seem to meaningfully define changes in fish composition through time. Regardless of road removal, the water temperature and salinity increased gradually from January to June, and the depth and dissolved oxygen decreased, and at some points, the system became completely dry. The floodplains analyzed were brackish at the beginning of the year, and in general had good water quality, but as time passed they became hypersaline and hypoxic, until the control zone in both studied periods, and the rehabilitated zone during 2011, dried up by June. In this area the dry season runs from mid-November to mid-June (Amezcua et al., 2019), so all the sampled months occurred during the dry season, and as time passed, the temperature increased, causing evaporation, and therefore a reduction in the depth of the system, an increase in the salinity and temperature of the water, and also a decrease in the dissolved oxygen. These results are likely influencing the changes in the fish assemblages observed throughout the course of this study; regardless of the rehabilitation. In both periods and in both systems, the fish assemblages changed according to a clear monthly pattern that is likely associated with the changes in these abiotic factors. However, different fish species were found in the sites even in very harsh conditions. Inland aquatic environments are subject to drying periods with the highest variations in concentrations of dissolved oxygen (Okada et al., 2003). Most of the fish species found exhibited tolerance to shifts in the salinity and dissolved oxygen which demonstrates their morphological and physiological abilities to maximize survival and adapt to changing conditions in the environment such as variations in hydrological regime. Most of the sampled fish were freshwater species with higher environmental tolerances, inhabiting shallow, warm, brackish waters.
Fish Assemblages Before and After the Rehabilitation
Considering that we were able to obtain samples in the rehabilitated and control floodplains before and after the road was removed, the use of a BACI designed seemed to be optimal, as this design is one of the best models for environmental effects monitoring programs (Smokorowski and Randall, 2017). However it has been discussed that a proper BACI design would require about 3 to 5 years of sampling prior to and after the impact, as this is the period of time in which most of the fish species would reach their maximum age, and also when most species would complete one generation. It is necessary to consider that those studies were undertaken in temperate and cold zones where the water bodies are permanent and the fish species have longer fish spans (Smokorowski and Randall, 2017). This is opposite to what we have in our study: ephemeral water bodies that last less than a year, because at some point the system dries up, or the conditions become too harsh to support any living organisms, and also the life spans of some of the analyzed fish species are very short, as is the case of the guppies that have a life span of less than 2 years (Reznick et al., 2005), or the fringed flounder, whose life span is 1 year (Reichert, 1998), and although the biology of most of the fish species found in the present study is not known, it is very likely that some of the other fish species have similar short life spans.
Also, as previously stated, access to the study site was very limited, and only during the months when the samplings took place, therefore the pre and post -treatment periods were limited to only the months analyzed. However, we were able to use the months as replicas in the study, and considering that the studied sites are ephemeral, as previously stated, we consider that the results of the present work can be considered as valid.
Results indicate significant changes in richness, diversity, and structure of the fish assemblages in the treatment zone before and after the road was removed, and with the control zone. Prior to the removal of the road, the number of fish species and the structure of fish assemblages was very similar between the control and the treatment zones. After the road was removed, the diversity, richness, and the structure of the fish assemblage changed in the treatment zone.
During 2011 both zones were dominated by three species in terms of abundance, and two in terms of biomass. Two poeciliids Poecilia butleri and Poeciliopsis latidens, and the exotic species of tilapia (Orechromis aureus), were the most abundant, and in terms of biomass, both tilapia species found accounted for more than 95% of the biomass. In the control site during 2015, the tilapia (O. sp.) increased its abundance, and was also dominant in similar numbers to Oreochromis aureus, but in terms of biomass, both species of tilapia accounted for more than 97%. It is necessary to consider that the conditions in these sites were harsh. Finding these species is likely related to the adaptations that these present to tolerate such conditions. It is known that the members of the family Poecilidae have a tolerance for such environmental conditions, especially thermal and wide range of salinity (0–135 ppt), which explains its high abundances and presence at all sampling sites (Meffe and Sheldon, 1988). These shallow, sheltered environments with soft sediment bottoms in the floodplain wetland appear to provide an ideal habitat for the formation of a breeding area for poecilids. Their diet mostly consists of detritus, zooplankton, and insects. For the case of the tilapia species, severe conditions, such as the ones found in this area, permit the high presence of exotic species. They have become the dominant species in many of their introduced ranges. It is known that Oreochromis aureus is a freshwater fish with a high tolerance for brackish water and to a wide range of the water quality and habitat conditions (McKaye et al., 1995). This ability explains its presence during all the sampled months, as this species is capable of withstanding severe conditions and poor water quality. This species is considered a competitor with the native species for food and space (Buntz and ManoochIII, 1969). Atherinella crystalina and Lile stolifera were not very abundant species during 2011 in the treatment area, and in the control area Atherinella crystallina was never collected. However, these species were found in some stations, from January to March, when the water quality was acceptable. Atherinella crystallina is restricted to freshwater areas of Sinaloa state and northern Nayarit, and is prone to declines due to habitat degradation, especially coastal development and pollution, and very little is known about its biology and habits (González-Díaz et al., 2015). Lile stolifera is known to enter and be very common in estuarine systems in the region, and is also an abundant species (Amezcua et al., 2006).
In the treatment zone after the road was removed the system was still dominated by the same three species in terms of abundance, and the two species of tilapia were still the dominant species in terms of biomass, although during this time its biomass accounted for 80%, 15% less than prior to the removal of the road, and other species appeared with some importance, and the diversity and richness of species increased in a significant manner. The species found during 2011 were also present in 2015, but additional species that are typical inhabitants of estuarine systems were also found, such as Dormitator latifrons, mojarras (Gerreidae), milk fish (Chanos chanos), snooks (Centropomidae), and the machete (Elops affinis). The studied area is close to the sea (approximately 3 km), and two estuarine systems (Huizache-Caimanero and Teacapan). Although there is no direct connection to these during the flooded period there are indirect connections. The closest is approximately 13 km to the north (Huizache-Caimanero), and the other is 20 km to the south (Teacapan), so there is the potential of estuarine species to find its way to the studied system, which occurred after the road was removed, as the number of species as well as the diversity increased, and the relative presence of both tilapias species decreased.
Nevertheless, the richness and diversity were low in both zones and both periods when compared with values observed in nearby systems [Huizache-Caimanero: species richness 61, Shannon diversity 4.0; Teacapan: species richness 51, Shannon diversity 3.8; (Amezcua et al., 2019)]. Previous studies indicate that a lower richness and diversity in similar sites is associated with the physiological limits of the species by salinity and dissolved oxygen (Okada et al., 2003). Although the conditions of the water improved with the removal of the road the water quality was still not optimal.
After the removal of the road however, there was an increment in diversity and richness. This increase might be related to rise in the volume of the water due to new hydrological connectivity, which is also related to the diversity and richness (Amezcua et al., 2019). Bayley (1995) pointed out the importance of the influence of the hydrological connectivity in the floodplain for the maintenance of biological and physical diversity. Furthermore, with the floods, there is a higher inundation which brings a greater amount of food and shelter for the fish (Welcomme, 2001).
Biodiversity is often used for assessing the success of the hydrological connectivity. However a single metric for quantifying the connectivity might be not enough, as there are more complex interactions occurring in the floodplains (Amoros and Bornette, 2002). Other factors such as connection frequency or the water body permanency, the intensity, degree, and duration of hydrological connectivity, etc., can strongly affect the biological characteristics of the aquatic environment (de Macedo-Soares et al., 2010), therefore analyzing the structure of the fish assemblage and its spatiotemporal changes might better reflect the success of an increase in hydrological connectivity. To do this assessment, a multivariate analysis was performed, and the results clearly reflected significant differences between sites, years, and months. The distance-based redundancy analysis clearly shows these changes, as clear-cut groups were formed depending on the site and year, and a change is observed regarding the sampling month. Before and after the road was removed seasonal changes occurred in both sites. This is likely related to the decrease in water quality as the time passed, because the temperature and salinity increased, whilst the depth and dissolved oxygen decreased, therefore, at the end of the sampling regime, only species that were able to withstand these harsh conditions were able to remain there. However, these changes might also be related to the specific biological rhythm, foraging ecology, and behavior of each species, besides the variability in hydrological terms (Dantas et al., 2012). The removal of the road had a significant influence in the fish assemblages in the treatment area, because, as pointed out before, species able to withstand these harsh conditions, such as Dormitator latifrons, were not recorded before the road was removed. Before the road was opened the low degree of hydrological connection in the environment probably promoted spatial heterogeneity and environmental gradients that shaped the structure of the fish assemblages according to tolerances of individual species (de Macedo-Soares et al., 2010). This would explain why during 2011, and in both periods in the control zone, there were fewer species, and the fish assemblage was dominated by poecilids and cichlids (tilapias), with an important presence of exotic species. On the other hand, enhanced hydrological connectivity which was based on the road removal implied a higher number of species, because the opportunity of movements within the system was enhanced, and they could easily move through the system. A larger area of the wetlands flooded implies more time that the fish can spend in the system and benefit from the wetland’s services. Previous studies in similar environments have also documented that an increase in the connectivity also increases the number of fish species (Petry et al., 2003; Lasne et al., 2007; Sheaves et al., 2007).
Hydrological connectivity can also influence the trophic structure. Based on the food web theory, larger ecosystems support longer food chains because they have a higher species diversity and habitat availability (Post et al., 2007). Hydrological connectivity after the road was removed made the patches in the aquatic habitat more frequently connected, which may contribute to longer food chains, as opposed to habitats which are infrequently connected. This enhanced the higher species richness and diversity, which might explain the absence of species such as Dormitator latifrons prior to the road being removed; being able to withstand harsh conditions might not be the only factor that determines the presence of certain species, but aspects such as the availability of food are also important. This also might be the reason why different fish species appeared after the road was removed, as the connection and the general habitat complexity might have increased as a consequence of the removal of the road.
Floodplains are today amongst the most threatened ecosystems, and for the preservation and the enhancement of native fishes it is essential to recognize the factors that influence the ecological functions of this ecosystem. The key for the conservation of the floodplain system and its biota is the understanding and maintenance of connectivity pathways. Our results show that limited hydrological connectivity and flow is a factor which relates to a diminishing of the abundance and diversity of the aquatic biota, the distribution of native fish, and favors the presence of exotic species such as tilapia, as previous studies have highlighted (Lasne et al., 2007). The fish assemblages have seasonal variations, and it is likely that in similar conditions the fish species composition is prone to variation among years; therefore there is a need to focus on the drivers of diversity of assemblages to understand the habitat function and improve the conservation and the management. It has been suggested that the alteration of the flow in the system could lead to the loss of the floodplain habitat and a decrease of the taxonomic and functional diversity (White et al., 2012). Our results precisely indicate that poor connectivity is associated with lower diversity, which also implies a more reduced and simple trophic structure, while an increase in habitat connectivity incrementally increases the abundance of a diversity of fish, and also seems an important factor to help the presence of native species.
Author Contributions
FA, leading author, responsible for the whole project, organized the contributions of all the authors, and put everything together, in charge of the final edition of the manuscript, the collection and analysis of fish in 2015, all statistical analysis, and the conception and design of the final objective of the work on its present form. JR, M.Sc. student, elaborated her M.Sc. thesis with the results of this project, in charge of all sampling during 2015, and contributed to the design of the work. FF-d-S contributed to the conception of this work by linking the connectivity with the increase in diversity, in charge of all the abiotic factors, the description of the studied area, and the elaboration of the maps, and helped with the BACI design. FF-V contributed to the idea, in charge of the restoration trough the road removal, established a control and a treatment area, and helped to link the connectivity with the fish assemblages. FA-L in charge of the design, sampling and analysis of all the fish samples collected during 2011, and helped with the final redaction of the manuscript.
Funding
Support for this research was provided by the Project PAPIIT-UNAM IA100218, and Instituto de Ciencias del Mar y Limnología from the Universidad Nacional Autonoma de Mexico.
Conflict of Interest Statement
The authors declare that the research was conducted in the absence of any commercial or financial relationships that could be construed as a potential conflict of interest.
Acknowledgments
We thank V. Muro, O. Nateras, D. Partida, and J. Yacomelo for their help during the sampling program. Lucinda Green edited the English text.
References
Amezcua, F., Madrid-Vera, J., and Aguirre-Villaseñor, H. (2006). Effect of the artisanal shrimp fishery on the ichthyofauna in the coastal lagoon of Santa María la Reforma, southeastern Gulf of California. Cienc. Mar. 32, 97–109. doi: 10.7773/cm.v32i12.1025
Amezcua, F., Ramirez, M., and Flores-Verdugo, F. (2019). Classification and comparison of five estuaries in the southeast Gulf of California based on environmental variables and fish assemblages. Bull. Mar. Sci. 95, 139–159. doi: 10.5343/bms.2018.0018
Amoros, C., and Bornette, G. (2002). Connectivity and biocomplexity in waterbodies of riverine floodplains. Freshw. Biol. 47, 761–776. doi: 10.1046/j.1365-2427.2002.00905.x
Arthington,ÁH., Naiman, R. J., Mcclain, M. E., and Nilsson, C. (2010). Preserving the biodiversity and ecological services of rivers: new challenges and research opportunities. Freshw. Biol. 55, 1–16. doi: 10.1111/j.1365-2427.2009.02340.x
Bayley, P. B. (1995). Understanding large river: floodplain ecosystems. Bioscience 45, 153–158. doi: 10.2307/1312554
Bunn, S. E., and Arthington, A. H. (2002). Basic principles and ecological consequences of altered flow regimes for aquatic biodiversity. Environ. Manage. 30, 492–507. doi: 10.1007/s00267-002-2737-0
Buntz, J., and Manooch, C. S. III (1969). Tilapia aurea (Steindachner), a rapidly spreading exotic in south central Florida. Proc. Ann. Conf. SE. Assoc. Game Fish Comm. 22, 495–501.
Chao, A. (1984). Nonparametric estimation of the number of classes in a population. Scand. J. Stat. 11, 265–270.
Cucherousset, J., Carpentier, A., and Paillisson, J. (2007). How do fish exploit temporary waters throughout a flooding episode? Fish. Manag. Ecol. 14, 269–276. doi: 10.1111/j.1365-2400.2007.00555.x
Dantas, D. V., Barletta, M., Lima, A. R. A., Ramos, J., de, A. A., Da Costa, M. F., et al. (2012). Nursery habitat shifts in an estuarine ecosystem: patterns of use by sympatric catfish species. Estuar. Coasts 35, 587–602. doi: 10.1007/s12237-011-9452-0
de Macedo-Soares, P. H. M., Petry, A. C., Farjalla, V. F., and Caramaschi, E. P. (2010). Hydrological connectivity in coastal inland systems: lessons from a neotropical fish metacommunity. Ecol. Freshw. Fish 19, 7–18. doi: 10.1111/j.1600-0633.2009.00384.x
Flather, C. (1996). Fitting species–accumulation functions and assessing regional land use impacts on avian diversity. J. Biogeogr. 23, 155–168. doi: 10.1046/j.1365-2699.1996.00980.x
Flores Verdugo, F., Moreno Casasola, P., Agraz Hernàndez, C. M., López Rosas, H., Benítez Pardo, D., and Travieso Bello, A. C. (2007). La topografía y el hidroperiodo: dos factores que condicionan la restauración de los humedales costeros. Boletín la Soc. Botánica México 80S, S33–S47. doi: 10.1186/1742-4755-12-11
Flores-Verdugo, F., González-Farias, F., and Zaragoza-Araujo, U. (1993). “Ecological parameters of the mangroves of semi-arid regions of Mexico: important for ecosystem management,” in Towards the Rational Use of High Salinity Tolerant Plants, Vol. 1, eds H. Lieth and A. Al Masoon (Berlin: Springer), 123–132. doi: 10.1007/978-94-011-1858-3_12
García, E. (1998). Climas (Clasificación de Köppen, Modificada por García) Escala 1: 1 000 000. México, DF: Comisión Nacional para el Conocimiento y Uso de la Biodiversidad.
Giri, C., Ochieng, E., Tieszen, L. L., Zhu, Z., Singh, A., Loveland, T., et al. (2011). Status and distribution of mangrove forests of the world using earth observation satellite data. Glob. Ecol. Biogeogr. 20, 154–159. doi: 10.1016/j.jenvman.2014.01.020
Glenn, E. P., Nagler, P. L., Brusca, R. C., and Hinojosa-Huerta, O. (2006). Coastal wetlands of the northern Gulf of California: inventory and conservation status. Aquat. Conserv. Mar. Freshw. Ecosyst. 16, 5–28. doi: 10.1002/aqc.681
González-Díaz, A. Á, Soria-Barreto, M., Martínez-Cardenas, L., and Blanco, M. (2015). Fishes in the lower san pedro mezquital River, Nayarit, Mexico. Check List 11:7.
Green, R. H., and Green, R. M. (1979). Sampling Design and Statistical Methods for Environmental Biologists. Hoboken, NJ: John Wiley & Sons.
INEGI and Gobierno del Estado de Sinaloa (1999). Anuario Estadístico del Estado de Sinaloa. Aguascalientes: INEGI.
Jackson, J. B. C., Kirby, M. X., Berger, W. H., Bjorndal, K. A., Botsford, L. W., Bourque, B. J., et al. (2001). Historical overfishing and the recent collapse of coastal ecosystems. Science 293, 629–637. doi: 10.1126/science.1059199
Jardine, T. D., Pusey, B. J., Hamilton, S. K., Pettit, N. E., Davies, P. M., Douglas, M. M., et al. (2012). Fish mediate high food web connectivity in the lower reaches of a tropical floodplain river. Oecologia 168, 829–838. doi: 10.1007/s00442-011-2148-0
Junk, W. J., Bayley, P. B., and Sparks, R. E. (1989). The flood pulse concept in river-floodplain systems. Can. Spec. Publ. Fish. Aquat. Sci. 106, 110–127.
Junk, W. J., Brown, M., Campbell, I. C., Finlayson, M., Gopal, B., Ramberg, L., et al. (2006). The comparative biodiversity of seven globally important wetlands: a synthesis. Aquat. Sci. 68, 400–414. doi: 10.1007/s00027-006-0856-z
Junk, W. J., and Wantzen, K. M. (2004). “The flood pulse concept: new aspects, approaches and applications-an update,” in Proceedings of the Second International Symposium on the Management of Large Rivers for Fisheries, (Rome: FAO), 117–149.
Kingsford, R. T. (2000). Ecological impacts of dams, water diversions and river management on floodplain wetlands in Australia. Aust. Ecol. 25, 109–127. doi: 10.1046/j.1442-9993.2000.01036.x
Lasne, E., Lek, S., and Laffaille, P. (2007). Patterns in fish assemblages in the Loire floodplain: the role of hydrological connectivity and implications for conservation. Biol. Conserv. 139, 258–268. doi: 10.1016/j.biocon.2007.07.002
Levings, C. D. (1991). Strategies for restoring and developing fish habitats in the Strait of Georgia—Puget Sound Inland Sea, northeast Pacific Ocean. Mar. Pollut. Bull. 23, 417–422. doi: 10.1016/0025-326x(91)90710-a
McArdle, B. H., and Anderson, M. J. (2001). Fitting multivariate models to community data: a comment on distance-based redundancy analysis. Ecology 82, 290–297. doi: 10.1890/0012-9658(2001)082%5B0290:fmmtcd%5D2.0.co;2
McKaye, K. R., Ryan, J. D., Stauffer, J. R. Jr., Perez, L. J. L., Vega, G. I., and van den Berghe, E. P. (1995). African tilapia in Lake Nicaragua. Bioscience 45, 406–411. doi: 10.2307/1312721
Meffe, G. K., and Sheldon, A. L. (1988). The influence of habitat structure on fish assemblage composition in southeastern blackwater streams. Am. Midl. Nat. 120, 225–240.
Okada, E. K., Agostinho,ÂA., Petrere, M. Jr., and Penczak, T. (2003). Factors affecting fish diversity and abundance in drying ponds and lagoons in the upper Paraná River basin, Brazil. Ecohydrol. Hydrobiol. 3, 97–110.
Ormerod, S. J. (2003). Restoration in applied ecology: editor’s introduction. J. Appl. Ecol. 40, 44–50. doi: 10.1046/j.1365-2664.2003.00799.x
Páez-Osuna, F., Guerrero-Galván, S. R., and Ruiz-Fernández, A. C. (1999). Discharge of nutrients from shrimp farming to coastal waters of the Gulf of California. Mar. Pollut. Bull. 38, 585–592. doi: 10.1016/s0025-326x(98)00116-7
Pearson, R. G., Arthington, A. H., and Godfrey, P. C. (2011). Ecosystem Health of Wetlands of the Great Barrier Reef Catchment: Tully-Murray Floodplain Case Study. Cairns: Reef and Rainforest Research Centre.
Petry, A. C., Agostinho, A. A., and Gomes, L. C. (2003). Fish assemblages of tropical floodplain lagoons: exploring the role of connectivity in a dry year. Neotrop. Ichthyol. 1, 111–119. doi: 10.1590/s1679-62252003000200005
Post, D. M., Doyle, M. W., Sabo, J. L., and Finlay, J. C. (2007). The problem of boundaries in defining ecosystems: a potential landmine for uniting geomorphology and ecology. Geomorphology 89, 111–126. doi: 10.1016/j.geomorph.2006.07.014
Ramírez Zavala, J. R., Cervantes Escobar, A., and Hernández Tapia, F. J. (2012). Marismas Nacionales Sinaloa : futuro y conservacián. Culiacán: Universidad Autonoma de Sinaloa/Pronatura Noroeste AC.
Ratti, J. T., Rocklage, A. M., Giudice, J. H., Garton, E. O., and Golner, D. P. (2001). Comparison of avian communities on restored and natural wetlands in North and South Dakota. J. Wildl. Manage. 65, 676–684.
Reichert, M. J. M. (1998). Etropus crossotus, an annual flatfish species; age and growth of the fringed flounder in South Carolina. J. Sea Res. 40, 323–332. doi: 10.1016/s1385-1101(98)00022-7
Reznick, D., Bryant, M., and Holmes, D. (2005). The evolution of senescence and post-reproductive lifespan in guppies (Poecilia reticulata). PLoS Biol. 4:e7. doi: 10.1371/journal.pbio.0040007
Rozas, L. P., Martin, C. W., and Valentine, J. F. (2013). Effects of reduced hydrological connectivity on the nursery use of shallow estuarine habitats within a river delta. Mar. Ecol. Prog. Ser. 492, 9–20. doi: 10.3354/meps10486
Sheaves, M., Johnston, R., and Abrantes, K. (2007). Fish fauna of dry tropical and subtropical estuarine floodplain wetlands. Mar. Freshw. Res. 58, 931–943.
Smokorowski, K. E., and Randall, R. G. (2017). Cautions on using the before-after-control-impact design in environmental effects monitoring programs. Facets 2, 212–232. doi: 10.1139/facets-2016-0058
Spalding, M. D., Fox, H. E., Allen, G. R., Davidson, N., Ferdana, Z. A., Finlayson, M. A. X., et al. (2007). Marine ecoregions of the world: a bioregionalization of coastal and shelf areas. Bioscience 57, 573–583. doi: 10.1641/b570707
Sparks, R. E., Nelson, J. C., and Yin, Y. (1998). Naturalization of the flood regime in regulated rivers: the case of the upper Mississippi River. Bioscience 48, 706–720. doi: 10.2307/1313334
Taylor, D. S., Reyier, E. A., Davis, W. P., and McIvor, C. C. (2007). Mangrove removal in the Belize cays: effects on mangrove-associated fish assemblages in the intertidal and subtidal. Bull. Mar. Sci. 80, 879–890.
Thomaz, S. M., Bini, L. M., and Bozelli, R. L. (2007). Floods increase similarity among aquatic habitats in river-floodplain systems. Hydrobiologia 579, 1–13. doi: 10.1007/s10750-006-0285-y
Tockner, K., Bunn, S. E., Gordon, C., Naiman, R. J., Quinn, G. P., and Stanford, J. A. (2008). “Flood plains: critically threatened ecosystems,” in Aquatic Ecosystems. Trends and Global Prospects, eds N. V. C. Polunin (Cambridge: Cambridge University Press), 45–61.
Tockner, K., and Stanford, J. A. (2002). Riverine flood plains: present state and future trends. Environ. Conserv. 29, 308–330. doi: 10.1017/s037689290200022x
Viana, A. P., Lucena Frédou, F., Frédou, T., Torres, M. F., and Bordalo, A. O. (2010). Fish fauna as an indicator of environmental quality in an urbanised region of the Amazon estuary. J. Fish Biol. 76, 467–486. doi: 10.1111/j.1095-8649.2009.02487.x
White, S. M., Ondraèková, M., and Reichard, M. (2012). Hydrologic connectivity affects fish assemblage structure, diversity, and ecological traits in the unregulated Gambia River, West Africa. Biotropica 44, 521–530. doi: 10.1111/j.1744-7429.2011.00840.x
Keywords: floodplain restoration, fish assemblages, estuarine fish, freshwater fish, multivariate analyses, BACI design
Citation: Amezcua F, Rajnohova J, Flores-de-Santiago F, Flores-Verdugo F and Amezcua-Linares F (2019) The Effect of Hydrological Connectivity on Fish Assemblages in a Floodplain System From the South-East Gulf of California, Mexico. Front. Mar. Sci. 6:240. doi: 10.3389/fmars.2019.00240
Received: 17 December 2018; Accepted: 18 April 2019;
Published: 10 May 2019.
Edited by:
Mario Barletta, Universidade Federal de Pernambuco (UFPE), BrazilReviewed by:
Joao Vieira, Fundação Universidade Federal do Rio Grande, BrazilJosé Lino Vieira De Oliveira Costa, Universidade de Lisboa, Portugal
Copyright © 2019 Amezcua, Rajnohova, Flores-de-Santiago, Flores-Verdugo and Amezcua-Linares. This is an open-access article distributed under the terms of the Creative Commons Attribution License (CC BY). The use, distribution or reproduction in other forums is permitted, provided the original author(s) and the copyright owner(s) are credited and that the original publication in this journal is cited, in accordance with accepted academic practice. No use, distribution or reproduction is permitted which does not comply with these terms.
*Correspondence: Felipe Amezcua, famezcua@ola.icmyl.unam.mx