- 1Molecular Invertebrate Systematics and Ecology Laboratory, Graduate School of Engineering and Science, University of the Ryukyus, Nishihara, Japan
- 2Tropical Biosphere Research Center, University of the Ryukyus, Nishihara, Japan
- 3Advanced Science-Technology Research (ASTER) Program, Institute for Extra-cutting-edge Science and Technology Avant-garde Research (X-star), Japan Agency for Marine-Earth Science and Technology (JAMSTEC), Yokosuka, Japan
- 4Laboratory of Aquatic Ecology, School of Marine Bioscience, Kitasato University, Sagamihara, Japan
- 5Bernice P. Bishop Museum, Honolulu, HI, United States
- 6Hawaii Institute of Marine Biology, Kaneohe, HI, United States
- 7Okinawa Churaumi Aquarium, Okinawa Churashima Foundation, Motobu, Japan
- 8Department of Zoology, George S. Wise Faculty of Life Sciences, Tel Aviv University, Tel Aviv, Israel
Research on so-called “minor taxa” at mesophotic depths has lagged behind that of more commonly researched groups such as fish or hard corals. Exemplar taxa include species of the order Zoantharia, benthic colonial anemones that are cosmopolitan in distribution but understudied in many ecoregion and ecosystems. In this study, we examine the results of rare mesophotic to deep sea surveys (one rebreather, one remotely operated submersible (ROV) + net survey, two ROV surveys) from Japan and Israel. We examined the collected images and video data to provide a first estimate of Zoantharia diversity at mesophotic depths. Zoantharians were observed in all surveys, indicating their ubiquitous presence in mesophotic and deeper ecosystems. Additionally, specimens (n = 12) acquired via these surveys were phylogenetically analyzed with three DNA markers. Phylogenetic results showed the presence of undescribed species based on the uniqueness of acquired DNA sequences, including one specimen belonging to the family Abyssoanthidae, previously only reported from depths below 2000 m. Other specimens belong to groups that inhabit shallower and deeper depths (Antipathozoanthus, Epizoanthus, and Parazoanthidae). These results depart from surveys of mesophotic coral ecosystem fishes and crustaceans, which indicate affiliations almost exclusively with shallow genera and families. We hypothesize that mesophotic depths are an ecotone for zoantharian diversity, with links to both deeper and shallower ecosystem diversity. Future surveys in mesophotic depths should incorporate phylogenetic methods to better catalog so-called “minor taxa” (not only including Zoantharia) and enhance the scientific foundation for conserving the biodiversity of these threatened ecosystems.
Introduction
Marine biodiversity has always been less well understood than that of terrestrial ecosystems. The obvious hazards and economic challenges of in situ surveys and sampling underwater have been and will remain barriers to scientific research at depth. While the marine biodiversity surveys in past centuries focused on the infralittoral and shallow depths, by the 1970s submersible technology allowed surveys of the deep-sea (Grassle et al., 1975; Heirtzler and Grassle, 1976; Barnes et al., 1977; Corliss et al., 1979; Tunnicliffe et al., 1986), with surprising discoveries of marine life that have resulted in a dramatic re-thinking of marine biodiversity patterns (e.g., Rex, 1981; Grassle, 1985). However, the marine realms between the deep-sea (>200 m depths) and shallow waters (0–∼30 m), the so-called “twilight” mesophotic zone, were scarcely examined, as the depths were too shallow for safe submersible operation yet too deep for conventional SCUBA diving (Pyle, 1996; Hinderstein et al., 2010; Kahng et al., 2010).
However, technological advances in both smaller, remotely operated submersibles (ROVs) (Fricke and Knauer, 1986; Stewart and Auster, 1989; Love et al., 1994; Lindsay et al., 2012), and in rebreather diving equipment (Stone, 1990; Pyle, 1999, 2000), have allowed exploration of these mesophotic ecosystems to expand greatly in scale and frequency. Research in these ecosystems have yielded important new discoveries about marine biodiversity (Erdmann et al., 1998; Pyle, 2000; Kane et al., 2014; Rowley, 2014; Kosaki et al., 2017). However, as seen from both shallow-water and deep-sea work, studies on understudied, or so-called “minor taxa”, have lagged.
One such understudied taxon is the order Zoantharia (=Zoanthidea, within Cnidaria: Anthozoa: Hexacorallia), commonly known as “colonial anemones.” Zoantharians have been reported from most marine regions of the world, from tidal zones of shallow tropical coral reefs (e.g., Karlson, 1988) to the deep seafloor greater than 5500 m (Reimer and Sinniger, 2010). The most commonly encountered zoantharian are the shallow coral reef zooxanthellate genera Palythoa and Zoanthus. Due to ease of observation, these groups are better documented than other shallow water Zoantharia (e.g., Karlson, 1988; Acosta et al., 2005; Irei et al., 2011). On the other hand, at mesophotic to deeper depths, many zoantharian are epizoic, living on other various organisms including sponges, hydroids, antipatharians, octocorals, eunicid worm tubes, and even echinoderms (e.g., Sinniger et al., 2010a; Swain, 2010; Kise and Reimer, 2016; Kise et al., 2018), utilizing these hosts to help obtain energy from the water column (Swain, 2010). Diversity-focused research in the deep sea has revealed previously unknown taxa that have reshaped our understanding of the order (Reimer et al., 2007; Reimer and Sinniger, 2010; Sinniger et al., 2013; Carreiro-Silva et al., 2017). We now know that the deep-sea harbors high levels of zoantharian diversity, mandating more research to catalog and understand this diversity (Reimer et al., 2007; Swain, 2010; Sinniger et al., 2013; Carreiro-Silva et al., 2017). However, studies on zoantharian at mesophotic and deeper depths are generally lacking, and collection of Zoantharia in mesophotic areas lags behind other benthic organisms such as sponges, stony corals, and octocorals. Thus, it is not known whether such depths harbor species affiliated with shallow (0–30 m) or deep (>200 m) communities, a mixture of both (=ecotone; region of transition between two biological communities), or unique depth-specific biodiversity as observed in brachyuran crabs (Hurley et al., 2016).
Here we report on mesophotic and deeper adjacent ecosystem (30–>200 m depths) surveys from four regions in Japan and Israel based on a variety of technologies including rebreathers, ROVs, traps, and phylogenetic tools. As an example of how our understanding of the biodiversity of understudied taxa can benefit from these platforms, we examine collated data (video images + specimens) from these surveys while focusing on the order Zoantharia. We use these findings to guide future research on neglected minor taxa at mesophotic and deeper depths.
Materials and Methods
Surveys and Specimen Identification
Specimens examined in this study were collected by ROV and mixed gas diving (Table 1), supplemented with in situ video from both ROV and mixed gas dives. Details are provided below.
Specimens were initially identified to family or genus-level (when possible) based on their epizoic associations and external characters, following Low et al. (2016) and relevant literature therein. Final identification of specimens to genus/species-level was based on molecular barcodes and phylogenetic results as detailed below.
Specimens from Japan were deposited in Fujukan University of the Ryukyus Museum (RUMF) under specimen numbers RUMF-ZG-04447 to RUMF-ZG-04455 (Table 1).
PICASSO Surveys (Okinawa, Sagami Bay)
The untethered remotely operated vehicle (uROV) PICASSO-1 (Lindsay et al., 2012, 2013) was controlled in real time from a surface support vessel via a ϕ 0.9 mm fiber optic cable (Figure 1A). The main camera was a SONY compact high-definition camera system (HDC-X300K) and environmental data (temperature, salinity) were also collected concurrently using a CTD-DO (XR-420CTDm, RBR Ltd.). Images from Sagami Bay were taken on 26 April 2007 at 473 m depth on the Sagami Knoll (35°09.7’N 139°23.1’E) during Cruise YK07-06 of the R/V Yokosuka. Images from Okinawa were taken during surveys on 26 September 2010 at 111 m depth off Ishigaki Island, Okinawa, from the diving boat “Sea Gull” (15.23 m, 19 t). No specimens were collected during dives.
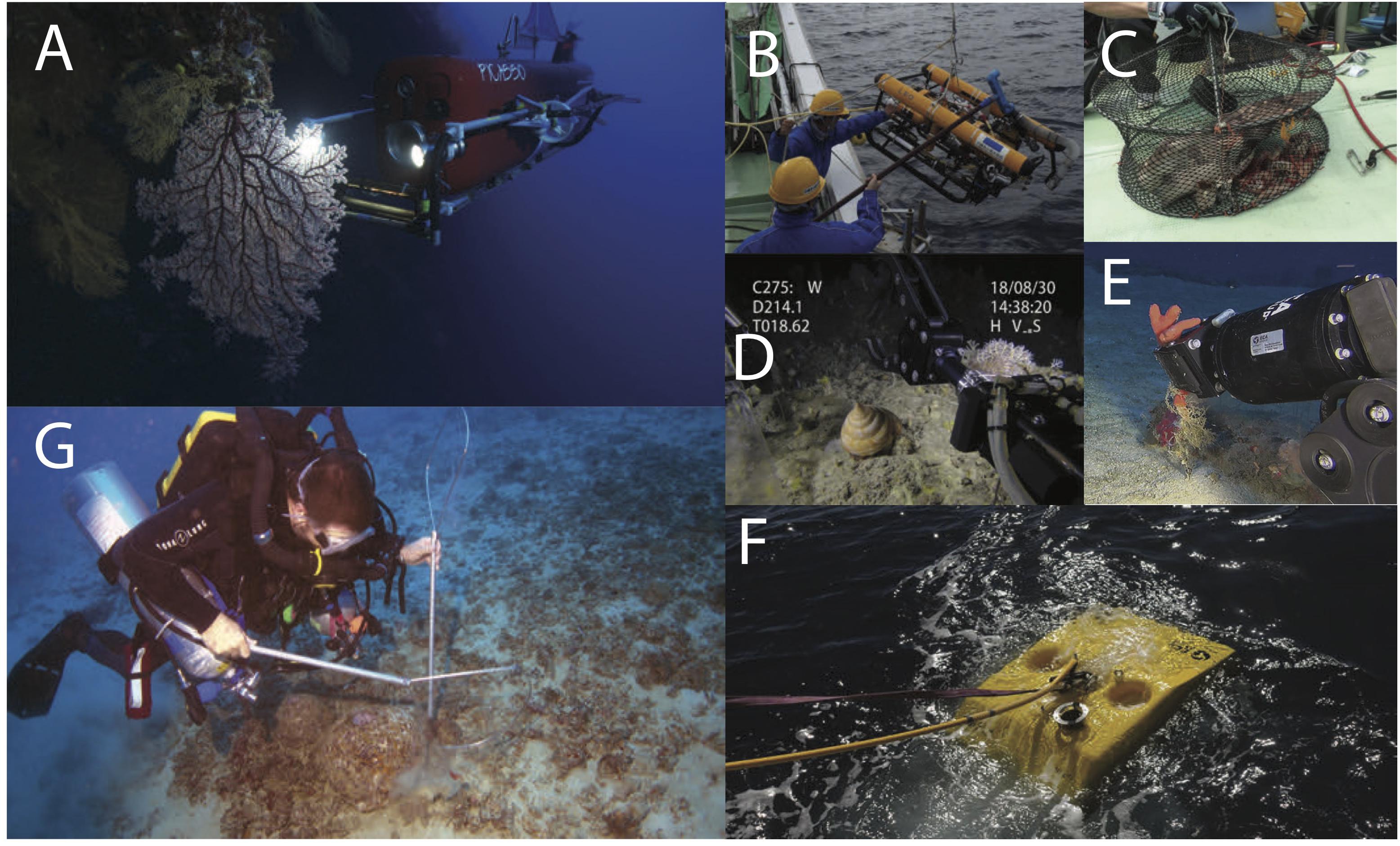
Figure 1. Various methods of sampling employed in this study, including (clockwise) (A) PICASSO ROV (JAMSTEC) in situ acquiring high-resolution video; (B) Okinawa Churaumi Aquarium ROV external view during recovery; (C) An Okinawa Churaumi Aquarium baited trap; (D) Okinawa Churaumi Aquarium ROV frame grab from in situ sampling, (E) ROV (ECA H800) frame grab from in situ sampling in Eilat, Red Sea, Israel; (F) ECA H800 during recovery, and (G) J. Copus during rebreather surveys in Okinawa, Japan at 80 m.
Okinawa Churaumi Aquarium Surveys
The Okinawa Churaumi Aquarium (OCA) regularly (approximately monthly, weather permitting) samples deep-water fauna around Okinawa-jima Main Island, in the Ryukyu Islands, using various methods including ROVs (Figure 1B), gill nets, and traps (Figure 1C). Collected specimens (Figure 1D) are generally brought back alive to OCA and kept in holding tanks in temperature similar to those at the collection depths. Among specimens collected between 2008 and 2018 at depths of 200–650 m were some unidentified colonies of the order Zoantharia (n = 8, Table 1). Specimens were preserved in 99% ethanol for molecular analyses, or 5% to 10% SW formalin for future morphological analyses. Material studied is deposited at RUMF (Table 1).
Rebreather Surveys (Okinawa)
Surveys were conducted using mixed-gas closed-circuit rebreathers from the surface to 100 m depth between November 9 to November 16, 2017 at two locations, near Seragaki Harbor (26.51°N, 127.88°E) and near Cape Hedo, both in Okinawa, Japan (26.85°N, 128.25°E) during daylight hours between 10:30 AM and 5:00 PM (Figure 1G). Benthic video surveys of MCEs at both sites were opportunistically completed using a Sony RX-100IV video camera in a Nauticam NA-RX100IV housing. Video was used to evaluate presence of Zoantharia. In total, 62 min of video were captured during 9.5 h of diving. No specimens were collected during dives.
Red Sea Surveys
Specimens were collected by ROV (ECA H800) operated by the Sam Rothberg R/V of the Interuniversity Institute for Marine Sciences in Eilat, Red Sea, Israel (Figures 1E,F). In situ photography was carried out using a low light black and white camera VS300 (ECA Robotics) and 1CAM Alpha HD camera (SubC imaging). Specimens were obtained using the ROV arm between 153 to 173 m. Colony fragments (subsamples) were preserved in 100% ethanol for genetic analyses. The original samples were preserved in 70% ethanol for morphological identification, and are deposited at the Steinhardt Museum of Natural History, National Center for Biodiversity Studies, Tel Aviv University, Israel (ZMTAU).
Mainland Japan Survey
A single specimen of unidentified zoantharian consisting of three polyps was collected at depths of 300–400 m via net off Sukumo Bay, Kochi, Japan on 11 November 2015 (Table 1). The specimen is deposited at the Molecular Invertebrate Systematics and Ecology (MISE), University of the Ryukyus.
Specimens and Phylogenetic Analyses
DNA Extraction, PCR Amplification and Sequencing
Total DNA was extracted using spin-column DNeasy Blood and Tissue Extraction kit (Qiagen, Hilden, Germany). PCR amplification was performed using HotStarTaq Master Mix Kit (Qiagen) for mitochondrial cytochrome oxidase subunit I (COI), mitochondrial 16S ribosomal DNA (16S-rDNA), and the nuclear internal transcribed spacer region of ribosomal DNA (ITS-rDNA) including 5.8S ribosomal DNA (5.8S-rDNA), and internal transcribed spacer regions 1 and 2. Loci were amplified utilizing the following primer sets; LCO1490 (5′-GGT CAA CAA ATC ATA AAG ATA TTG G-3′) and HCO2198 (5′-TAA ACT TCA GGG TGA CCA AAA AAT CA-3′) (Folmer et al., 1994) for COI, 16SarmL (5′-GGC CTC GAC TGT TTA CCA AA-3′) (Fujii and Reimer, 2011) and 16SbmH (5′-CGA ACA GCC AAC CCT TGG-3′) (Sinniger et al., 2005) for 16S-rDNA, and ITSf (5′-CTA GTA AGC GCG AGT CAT CAG C-3′), and ITSr (5′-GGT AGC CTT GCC TGA TCT GA-3′) (Swain, 2009) for ITS-rDNA. All genetic markers were amplified with the following thermal-cycle condition: 5 min at 95°C followed by 35 cycles of: 30 s at 94°C, 1 min at 40°C, and 1 min 30 s at 72°C, and followed by a 7 min extension at 72°C for COI; 5 min at 95°C and then 35 cycles of: 1 min at 95°C, 1 min at 52°C, and 2 min at 72°C, followed by a 7 min extension at 72°C for 16S-rDNA; and 5 min at 95°C then 35 cycles of: 1 min at 94°C, 1 min at 50°C, and 2 min at 72°C, followed by a 10 min extension at 72°C for ITS-rDNA. Amplified PCR products were checked by 1.5% agarose gel and positive PCR products were sequenced in both directions by Fasmac (Kanagawa, Japan) after clean up using shrimp alkaline phosphatase (SAP) and Exonuclease I (Takara Bio Inc., Shiga, Japan).
Phylogenetic Reconstruction
Sequences of COI, 16S-rDNA and ITS-rDNA were manually inspected and edited using Geneious v10.2.3 (Kearse et al., 2012) and deposited in GenBank (MK377410-MK377426 and MK385646-MK385652). Consensus sequences of all genetic markers were aligned with previous published sequences from various genera within the Parazoanthidae, Abyssoanthus and Epizoanthus (Table 2). All alignments were performed using the Muscle algorithm (Geneious plug-in; Edgar, 2004) with default settings and manual editing. Microzoanthus occultus was selected as the outgroup for all genetic markers in this study. Five alignment datasets were generated; 457 base pairs (bp) of 50 sequences for COI; 900 bp of 51 sequences for 16S-rDNA, 461 bp of 40 sequences for ITS1, 165 bp of 42 sequences for 5.8S-rDNA, and 256 bp of 42 sequences for ITS2. The alignment data are available from the corresponding author.
The generated alignments were used to construct a collated dataset including 2239 bp and 54 sequences. All missing data were replaced with “N” for phylogenetic analyses. Maximum likelihood (ML) phylogenetic analyses were performed using RAxML v8 (Stamatakis, 2014) with the collated dataset with 1000 pseudoreplicates of bootstrapping. Gene partitions set for ML were selected in RAxML.
Results
PICASSO Surveys
Analyses of the PICASSO video surveys showed the presence of zoantharians on the stalks of numerous glass sponges at the summit of the Sagami Knoll in Sagami Bay at 473 m, and these were identified as Epizoanthus cf. stellaris based on their epizoic association on the stalks of these sponges (Beaulieu, 2001). During collection, temperature was 6.0°C and salinity was 33.90.
Additionally, an image of a zoantharian on a eunicid worm from 111 m was acquired from a site off Ishigaki Island, Okinawa (24°15.69’N 124°4.24’E), and was identified as E. cf. illoricatus based on its epizoic association with eunicid worms (Kise and Reimer, 2016). During collection, temperature was 25.4°C and salinity was 34.87.
Okinawa Rebreather Surveys
Video from the Okinawa rebreather surveys revealed the presence of Umimayanthus sp. (video C0058, 0:20–31) based on its association with an encrusting sponge, E. cf. illoricatus (C0048, whole video; C0084, 0:28 and 0:36) based on its epizoic association with eunicid worms, as well as possible Antipathozoanthus (C0102, 0:33) based on the association with an antipatharian.
Okinawa Surveys
During the Churaumi ROV surveys, eight specimens of zoantharians, which were associated with Porifera [glass sponges; MISE-MZ1, MISE-MZ3 (Figure 2A), and Demospongiae; MISE-MZ5 on sponge on dead gorgonian or antipatharian, Figure 2C], antipatharians (MISE-MZ4, Figure 2B), gastropods (MISE-MZ6), empty mollusk shells [MISE-MZ8 (Figure 2D), and with hermit crab MISE-MZ9], and as well as on rope (MISE-MZ7, Figure 2E). Specimens were preliminarily identified to family or generic level based on these associations (Table 1). Further identifications were conducted with DNA sequences.
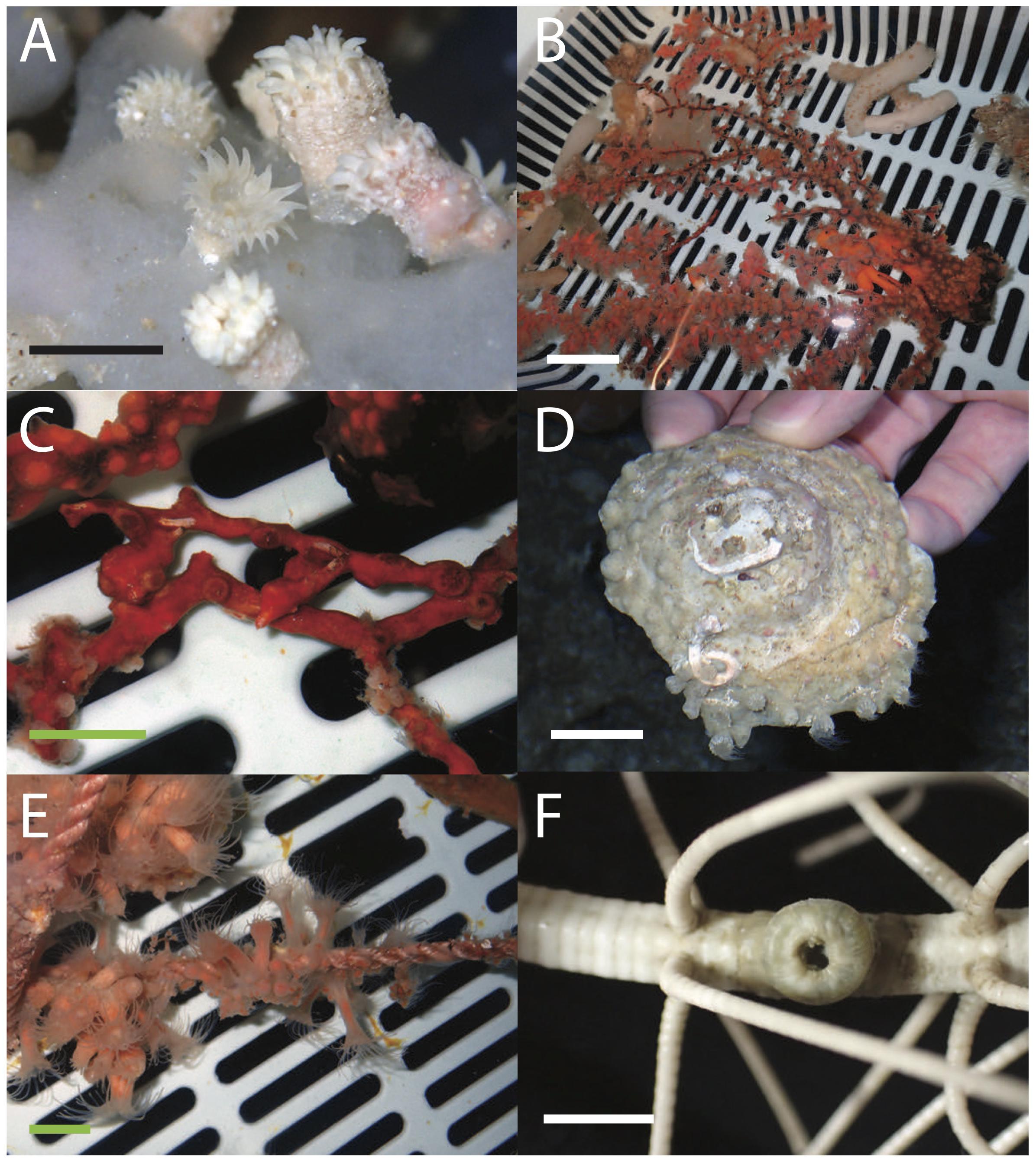
Figure 2. Images of living zoantharian specimens collected from Japan phylogenetically examined in this study. (A) Parazoanthidae sp. 1 MISE-MZ1 on glass sponge, (B) Savalia sp. 1 MISE-MZ4 on a dead gorgonian or antipatharian, (C) Umimayanthus sp. 1 MISE-MZ5 on an encrusting sponge, (D) Epizoanthus sp. 1 MISE-MZ8 on an empty mollusk shell, (E) Epizoanthus sp. 2 MISE-MZ7 growing on rope, and (F) preserved Abyssoanthidae sp. 1 MISE-Kochi1 on the arm of a crinoid. Scale bars: A, F = approximately 1 cm; B = approximately 5 cm; C, D, E = approximately 2 cm.
Mainland Japan Surveys
Surveys from Mainland Japan resulted a single specimen of unidentified zoantharian, which was associated with a crinoid (MISE-Kochi1, Figure 2F). Further identification was conducted via genetic analyses.
Red Sea Surveys
Surveys from Eilat resulted in the collection of four zoantharian specimens (Table 1) along with in situ images (Figure 3). Three of the specimens (ZMTAU Co37438, ZMTAU Co37440, and ZMTAU Co37441) were associated with antipatharians and were preliminarily identified as Antipathozoanthus sp. (Figure 3A), while one specimen (ZMTAU Co37525) was associated with octocorals and identified as Parazoanthidae sp. 2 (Figures 3B,C). Further identifications were conducted with DNA sequences (Table 1).
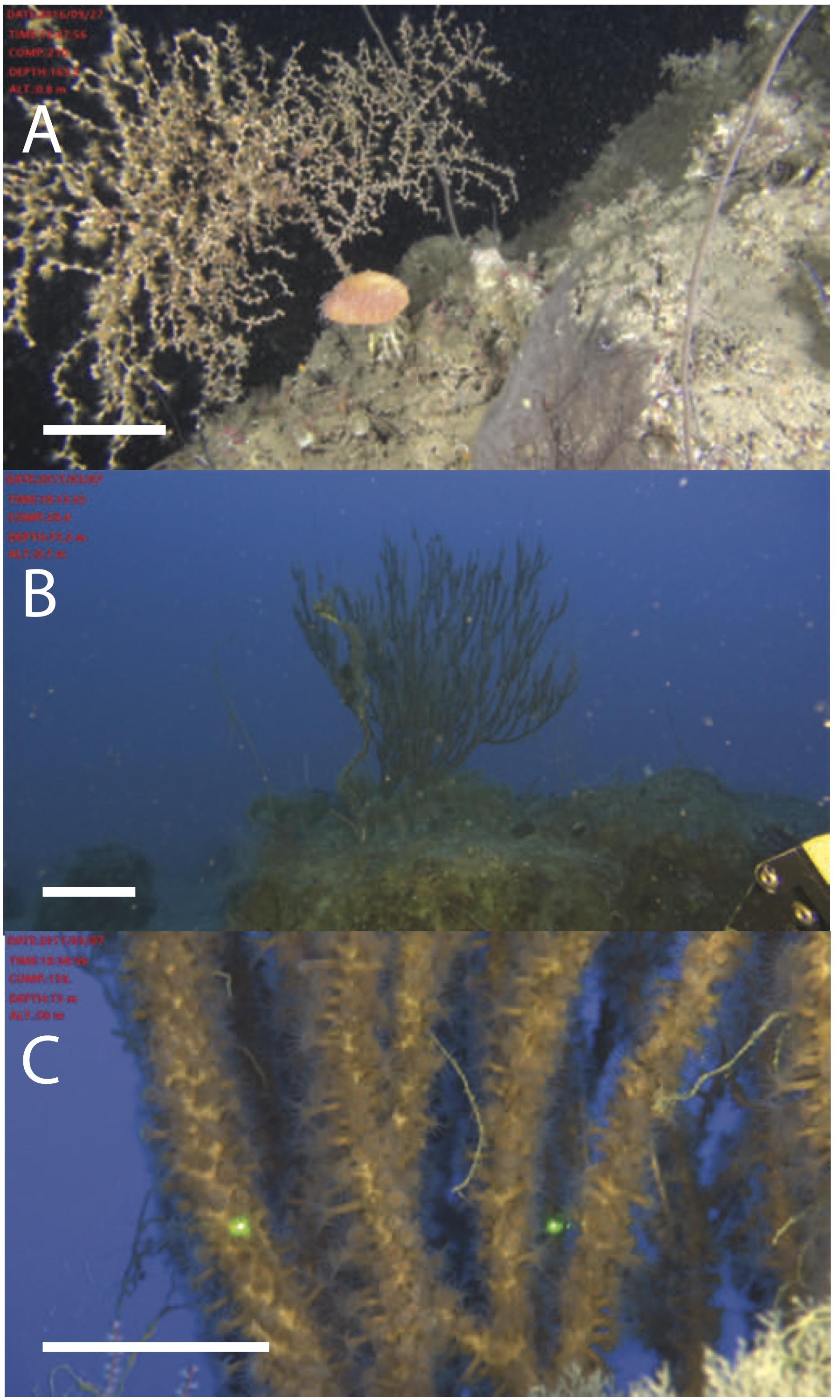
Figure 3. Zoantharian specimens collected from Israel phylogenetically examined in this study. (A) Antipathozoanthus sp. ZMTAU Co 37440 colony in situ at 170 m, (B) Parazoanthidae sp. 2 ZMTAU Co 37525 colony in situ at 173 m, and (C) closeup of the same colony. Scale bars: A, B = approximately 10 cm; C = approximately 5 cm.
Specimens and Phylogenetic Analyses
The phylogenetic tree resulting from the concatenated (COI + 16S + ITS1 + 5.8S + ITS2) analyses showed that the specimens in this study from Okinawa and the Red Sea belonged to three families of zoantharians; Abyssoanthidae, Epizoanthidae, and Parazoanthidae (Figure 4).
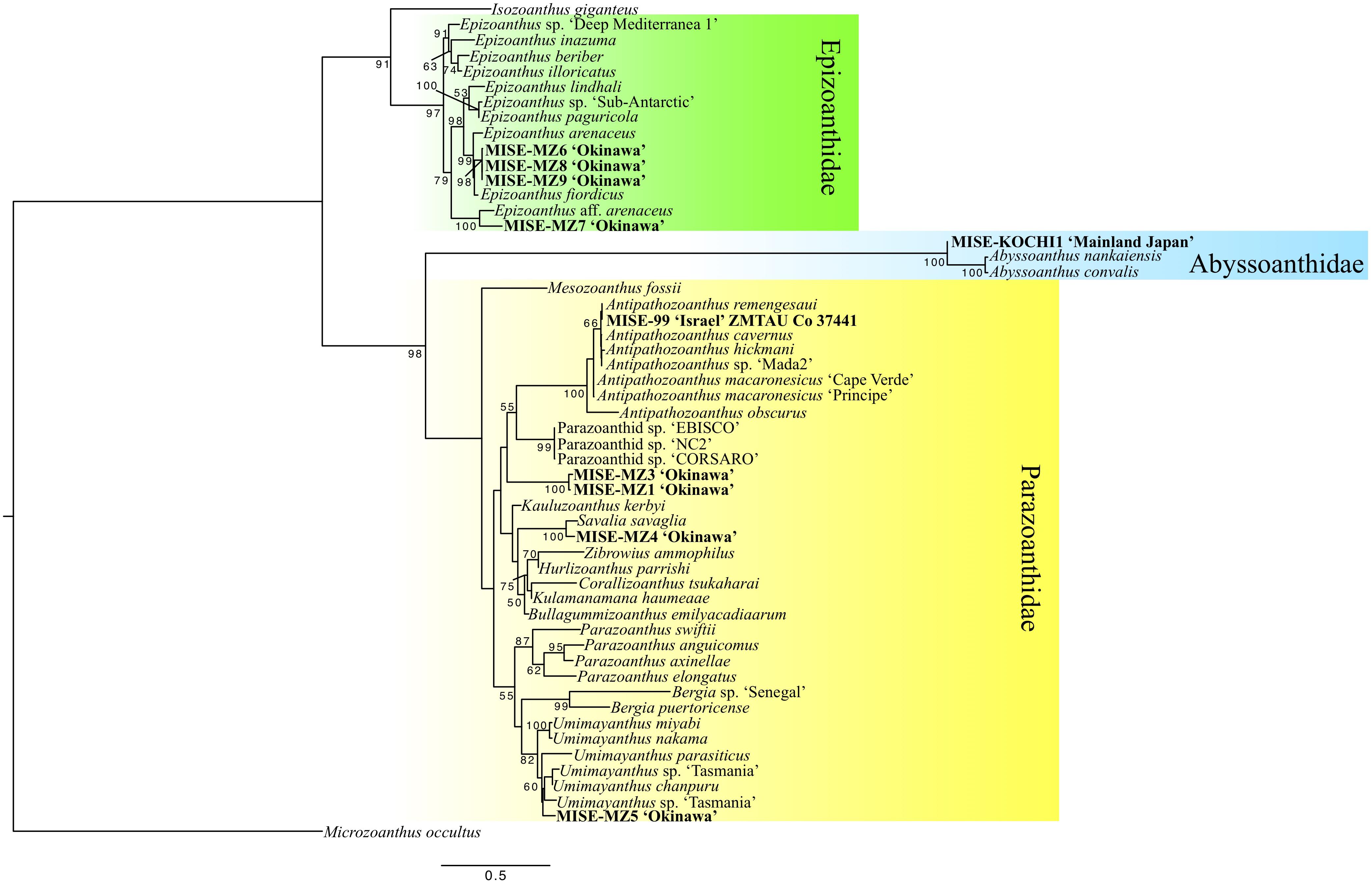
Figure 4. Maximum likelihood (ML) phylogenetic tree of zoantharians including specimens newly collected in this study (in bold). Numbers at nodes represent bootstrap support (ML).
Within the Epizoanthidae clade, sequences for specimens MISE-MZ6, MISE-MZ8, and MISE-MZ9 from Okinawa were observed to be nearly identical, and were included in a subclade with Epizoanthus arenaceus from Marseille, France (Reimer et al., 2007) and E. fiordicus from Chile (Sinniger and Häussermann, 2009) with high support (ML = 98%). Additionally, specimen MISE-MZ7 from Okinawa was phylogenetically affiliated with Epizoanthus, forming a well-supported subclade with E. aff. arenaceus (ML = 100%) (Figure 4).
One specimen, MISE-Kochi1 from 300 to 400 m off Sukumo Bay, Kochi, Japan grouped with very high support in a clade basal to previously reported sequences of two species of Abyssoanthus from the deep-sea around Japan with very high support (ML = 100%) (Figure 4).
Within the large Parazoanthidae clade, specimens MISE-MZ1 and MISE-MZ3 from approximately 600 m near Iejima, Okinawa formed a unique, highly supported subclade different from all previously reported zoantharian sequences (ML = 100%). Sequences from specimen MISE-MZ4 from Okinawa grouped closely with previously reported sequences of Savalia savaglia from Marseille, France (Sinniger et al., 2005) with ML = 100%. Similarly, sequences from specimen ZMTAU Co 37441 from Israel formed a clade (ML = 66%) with Antipathozoanthus remengesaui from Japan, the Maldives, the Red Sea and Palau (Kise et al., 2017). Finally, specimen MISE-MZ5 from Okinawa was placed within the large Umimayanthus subclade with moderate support (ML = 60%) (Figure 4).
Discussion
While Zoantharia are “minor taxa”, they are nearly ubiquitous, reported from almost all marine regions of the world, and are considered important components of the marine fauna (e.g., Karlson, 1983; Irei et al., 2011). In this study, we observed Zoantharia in every survey with each type of technology employed, even though these surveys were not specifically focused on Zoantharia, demonstrating that these anthozoans may be widespread at mesophotic and deeper depths, at least in Okinawa and the Red Sea. These findings support previous research from other locations that has also indicated the potential for high diversity of zoantharians in deeper waters (Reimer et al., 2007; Swain, 2010; Sinniger et al., 2013; Carreiro-Silva et al., 2017).
Of particularly interest from our results are the presence of an Abyssoanthidae specimen at 300 to 400 m from Kochi, Japan. Previously, Abyssoanthidae has only been observed from depths of approximately 2000 to 5600 m in Japan (Reimer et al., 2007; Reimer and Sinniger, 2010) and the Mediterranean (Sinniger et al., 2010b), and the discovery of a specimen at these comparatively shallow depths is very surprising. Previously, E. planus has been the only zoantharian known to associate with Echinodermata, in association with sea urchins (Carlgren, 1923; Kise et al., 2018), and this newly reported Abyssoanthidae specimen represents the first record of a zoantharian in association with the class Crinoidea. This record also represents the first record of the family Abyssoanthidae as epizoic, as previously described specimens were found living directly on hard substrates (Reimer et al., 2007; Reimer and Sinniger, 2010).
Additionally, specimens MISE-MZ1 and MISE-MZ3 represent a potentially undescribed genus associated with glass sponges. Currently, the only formally described glass sponge-associated species belong to family Epizoanthidae (genera Epizoanthus, Thoracactis) or to genus Isozoanthus within Parazoanthidae (Carlgren, 1923; Low et al., 2016). The current specimens clearly belong within Parazoanthidae in the phylogenetic analyses, and thus may be an undescribed species from either Isozoanthus or an undescribed genus-level clade within Parazoanthidae.
Four specimens were nested within the genus Epizoanthus, and constitute two species-groups. Specimens MISE-MZ6, MISE-MZ8, and MISE-MZ9, which were sampled several years ago and have proliferated in Churaumi Aquarium holding tanks, covering various substrates including living slit snails, hermit crab shells, and empty shell rubble, indicating that this species, at least in aquaria, is a facultative symbiont. The other Epizoanthus (MISE-MZ7) was found on nylon rope, and was also apparently sampled some time ago, although no specific date was recorded. Both specimens do not match described species in the region, but without more in situ information such as depth or original substrate, no conclusions on their specific identities can be made.
Our work also discovered Parazoanthidae genera deeper than has been previously reported; Umimayanthus sp. in Okinawa (depth >200 m; MISE-MZ5) and Antipathozoanthus sp. in the northern Red Sea (153–169 m), as well as the first formal records of Savalia (MISE-MZ4) in Okinawa. Such results should be taken with caution. Although they represent range and depth extensions, the overall dearth of Zoantharia data from the deep may hinder our ability to make clear conclusions of depth ranges and distributions of species.
From the current results, as well as findings in previous recent research (Reimer et al., 2007; Swain, 2010; Sinniger et al., 2010b, 2013; Carreiro-Silva et al., 2017), we can make two preliminary conclusions. Firstly, it appears that most mesophotic and deep-sea Zoantharia genera are widely distributed in the world’s oceans, and across a much greater range of depths than is currently understood. Many genera have been reported from both the Atlantic and Indo-Pacific (Sinniger et al., 2010b; Carreiro-Silva et al., 2017), and our current work found genera both deeper (Umimayanthus; Antipathozoanthus) and much shallower (Abyssoanthidae) than has previously been recorded. Secondly, these results highlight the dearth of information and distributional records of Zoantharia, and thus, comparisons on mesophotic and deeper water diversity of zoantharians between different regions, such Japan and Israel in this study, remain premature. Moving forward, basic biological data such as records of occurrences of zoantharians from any mesophotic or deeper depths regardless of location should be a research priority, allowing future biogeographical analyses.
The affiliation of mesophotic Zoantharia with both shallow and deep (abyssal) realms invokes a novel biogeographic enigma. Previous studies of fishes and crabs have revealed that mesophotic biodiversity is affiliated almost exclusively with the genera and families of the shallow shelf fauna (Kahng et al., 2014; Hurley et al., 2016). Either Abyssoanthidae and other deep-sea zoantharians have a greater presence in shallow habitat than previously known, or the mesophotic Zoantharia have a “hybrid” origin that includes some deep-sea fauna. Further surveys will likely resolve this dilemma. Previously, it has been hypothesized that shallow-water zooxanthellate zoantharians evolved from deeper water, epibiotic species (Swain, 2010), and this could partially explain the presence of deep-sea specimens in shallower waters.
Examining the different technologies employed in this study, each has its own strengths and weaknesses. Rebreather surveys allow high resolution in situ images and sampling, while ROV surveys are costlier yet safer, longer, and able to reach greater depths than rebreathers. However, many ROVs such as PICASSO cannot collect specimens, relying instead on high-resolution in situ images. Both ROVs and rebreather divers can still provide initial photographic records of species (e.g., Nishikawa et al., 2011), an enormous step forward given the almost complete lack of data for many marine taxa in many regions of the world, including Zoantharia (e.g., Reimer et al., 2014). The combination of ROVs or submersibles covering wide areas of the benthic environment followed by rebreather divers targeting specific sites of interest may be the most productive use of both technologies (e.g., Pyle et al., 2016). Dredging and nets, employed by Churaumi Aquarium, are effective in recovering many specimens, but even when equipped with cameras do not often result in high-resolution images of small benthos such as zoantharians, and are indiscriminate in their sampling, potentially damaging other, non-target benthos.
The scant state of knowledge about mesophotic invertebrate fauna in general and Zoantharia in particular is underscored by the substantial gains presented here, based on a modest data set. The need for biodiversity data on understudied and unknown MCE taxa is critical to understanding burgeoning anthropogenic threats (Rocha et al., 2018) including ongoing climate change. With the majority of MCE and deep-sea biological research focusing instead on more recognizable taxa such as fishes and scleractinian corals, observations of “minor taxa” should be incorporated into these ongoing sampling and field protocols to maximize efficiency. For example, rebreather divers or ROVs could take a photographic benthic quadrat or high-resolution image every 10 min. Archiving and depositing such information would rapidly amplify the available distribution data. Specimen deposits are also greatly needed for many other “minor taxa”, not only Zoantharia. In addition to specimen vouchers, genetic voucher subsamples are critical to identify novel species and evolutionary processes (Hurley et al., 2016).
Further research at mesophotic and deep-sea depths will undoubtedly enhance our knowledge of zoantharian diversity, which potentially includes species that have a major ecological role in MCEs, such as the zoantharian “gold coral” S. savaglia (e.g., Cerrano et al., 2010). As Zoantharia is a cosmopolitan group, data on species distributions are key to a better understanding of marine biogeography (Briggs and Bowen, 2016). Such information will also help contribute to management decisions in the face of threats to mesophotic habitats (Rocha et al., 2018), such as tailing disposal (Edinger, 2012) and oil exploration (Francini-Filho et al., 2018). These minor taxa are a major component of marine ecosystems, and integral to understanding anthropogenic impacts and consequences.
Ethics Statement
Collection of Red Sea animals complied with a permit issued by the Israel Nature and National Parks Protection Authority. No permits are needed for the collection of Zoantharia in Japan, nor are there any ethical permits required.
Author Contributions
All authors contributed to the conception and design of the research. All authors wrote sections of the manuscript. JR performed field work, collected specimens, conducted analyses, wrote the first draft of the manuscript, and provided funding. HK and MS conducted analyses and helped edit the manuscript. DL, BB, and YB performed field work, collected specimens, helped edit the manuscript, and provided funding. RP, JC, and TH performed field work, collected specimens, and helped edit the manuscript. MN helped edit the manuscript and provided funding. All authors read and approved the submitted version.
Funding
JR was supported by a JSPS KAKENHI grant 16H04834 Kiban B entitled “Global evolution of Brachycnemina and their Symbiodinium.” DL and PICASSO were partially funded by JSPS KAKENHI grants (24248032 and 23405031), MEXT Grant-in-Aid for Scientific Research on Innovative Areas TAIGA (20109003), as well as JST and CREST. HK was supported by a Sasakawa Scientific Research grant (2018-5021) from the Japan Science Society. MEAS was additionally supported by a Japanese Government (MEXT) scholarship. BB, RP, and JC were supported by NSF-OCE-1558852, Poseidon Diving Systems, and Shearwater Research. Red Sea work was supported by the TASCMAR project (Tools and Strategies to access original bioactive compounds from cultivated marine invertebrates and associated symbionts) which received funding from the European Union’s Horizon 2020 Research and Innovation Program under grant agreement No 634674; and by the Israel Cohen Chair in Environmental Zoology to YB.
Conflict of Interest Statement
The authors declare that the research was conducted in the absence of any commercial or financial relationships that could be construed as a potential conflict of interest.
Acknowledgments
The authors thank MISE laboratory members, and C. Lewis and M. Hoban (HIMB) for their logistical help in the Okinawa surveys. YB thanks the Interuniversity Institute for Marine Sciences in Eilat (IUI) for the use of the Sam Rothberg R/V and the professional assistance of its crew members. YB is indebted to EcoOcean staff members for operating the ROV. YB acknowledges M. Weis and R. Liberman for their help in the field, A. Shlagman for curatorial skills. Collection of Red Sea animals complied with a permit issued by the Israel Nature and National Parks Protection Authority. Comments from the editor and two reviewers improved an earlier version of this manuscript.
References
Acosta, A., Sammarco, P. W., and Duarte, L. F. (2005). New fission processes in the zoanthid Palythoa caribaeorum: description and quantitative aspects. Bull. Mar. Sci. 76, 1–26.
Barnes, A. T., Questin, L. B., Childress, J., and Pawson, D. L. (1977). Deep-sea macroplanktonic sea cucumbers: suspended sediment feeders captured from deep submergence vehicle. Science 194, 1083–1085.
Beaulieu, S. E. (2001). Life on glass houses: sponge stalk communities in the deep sea. Mar. Biol. 138, 803–817.
Briggs, J. C., and Bowen, B. W. (2016). Marine shelf habitat: biogeography and evolution. J. Biogeogr. 40, 1023–1035. doi: 10.1111/jbi.12082
Carlgren, O. (1923). Ceriantharia and Zoantharia. Wissensch Ergebn Deutsch Tiefsee-Exp Dampfer ‘Valdivia’ 1898–99, 242–337.
Carreiro-Silva, M., Ocaña, O., Stankoviæ, D., Sampaio,Í, Porteiro, F. M., Fabri, M. C., et al. (2017). Zoantharians (Hexacorallia: Zoantharia) associated with cold-water corals in the Azores region: new species and associations in the deep sea. Front. Mar. Sci. 4:88. doi: 10.3389/fmars.2017.00088
Cerrano, C., Danovaro, R., Gambi, C., Pusceddu, A., Riva, A., and Schiaparelli, S. (2010). Gold coral (Savalia savaglia) and gorgonian forests enhance benthic biodiversity and ecosystem functioning in the mesophotic zone. Biodiver. Conserv. 19, 153–167.
Corliss, J. B., Dymond, J., Gordon, L. I., Edmond, J. M., von Herzen, R. P., Ballard, R. D., et al. (1979). Submarine thermal springs on the Galapagos Rift. Science 203, 1073–1083.
Edgar, R. C. (2004). MUSCLE: multiple sequence alignment with high accuracy and high throughput. Nucleic Acid Res. 32, 1792–1797.
Edinger, E. (2012). Gold mining and submarine tailings disposal: review and case study. Oceanography 25, 184–199.
Erdmann, M. V., Caldwell, R. L., and Moosa, M. K. (1998). Indonesian ‘king of the sea’ discovered. Nature 395:335.
Folmer, O., Black, M., Hoeh, W., Lutz, R., and Vrijenhoek, R. (1994). DNA primers for amplification of mitochondrial cytochrome c oxidase subunit I from diverse metazoan invertebrates. Mol. Mar. Biol. Biotechnol. 3, 294–297.
Francini-Filho, R. B., Asp, N. E., Siegle, E., Hocevar, J., Lowyck, K., D’Avila, N., et al. (2018). Perspectives on the great amazon reef: extension, biodiversity and threats. Front. Mar. Sci. 5:142. doi: 10.3389/fmars.2018.00142
Fricke, H. W., and Knauer, B. (1986). Diversity and spatial pattern of coral communities in the Red Sea upper twilight zone. Oecologia 71, 29–37. doi: 10.1007/BF00377316
Fujii, T., and Reimer, J. D. (2011). Phylogeny of the highly divergent zoanthid family Microzoanthidae (Anthozoa, Hexacorallia) from the Pacific. Zool. Scr. 40, 418–431.
Grassle, J. F., Sanders, H. L., Hessler, R. R., Rowe, G. T., and McLellan, T. (1975). Pattern and zonation: a study of the bathyal megafauna using the research submersible Alvin. Deep-Sea Res. 22, 457–481.
Heirtzler, J. R., and Grassle, J. F. (1976). Deep-sea research by manned submersibles. Science 194, 294–299.
Hinderstein, L. M., Marr, J. C. A., Martinez, F. A., Dowgiallo, M. J., Puglise, K. A., Pyle, R., et al. (2010). Theme section on “mesophotic coral ecosystems: characterization, ecology, and management”. Coral Reefs 29, 247–251.
Hurley, K. K. C., Timmers, M. A., Godwin, L. S., Copus, J. M., Skillings, D. J., and Toonen, R. J. (2016). An assessment of shallow and mesophotic reef brachyuran crab assemblages on the south shore of Oahu, Hawaii. Coral Reefs 35, 103–112.
Irei, Y., Nozawa, Y., and Reimer, J. D. (2011). Distribution patterns of five zoanthid species in Okinawa Island, Japan. Zool. Stud. 50, 426–433.
Kahng, S. E., Copus, J. M., and Wagner, D. (2014). Recent advances in the ecology of mesophotic coral ecosystems. Curr. Opin. Environ. Sustain. 7, 72–81.
Kahng, S. E., Garcia-Sais, J. R., Spalding, H. L., Brokovich, E., Wagner, D., Weil, E., et al. (2010). Community ecology of mesophotic coral reef ecosystems. Coral Reefs 29, 255–275.
Kane, C., Kosaki, R. K., and Wagner, D. (2014). High levels of mesophotic reef fish endemism in the northwestern Hawaiian Islands. Bull. Mar. Sci. 90, 693–703. doi: 10.1371/journal.pone.0157861
Karlson, R. H. (1983). Disturbance and monopolization of a spatial resource by Zoanthus sociatus (Coelenterata, Anthozoa). Bull. Mar. Sci. 33, 118–131.
Karlson, R. H. (1988). Size-dependent growth in two zoanthid species: a constrast in clonal strategies. Ecology 69, 1219–1232.
Kearse, M., Moir, R., Wilson, A., Stones-Havas, S., Cheung, M., Sturrock, S., et al. (2012). Geneious Basic: an integrated and extendable desktop software platform for the organization and analysis of sequence data. Bioinformatics 28, 1647–1649. doi: 10.1093/bioinformatics/bts199
Kise, H., Dewa, N., and Reimer, J. D. (2018). First record of sea urchin-associated Epizoanthus planus from Japanese waters and its morphology and molecular phylogeny. Plankton Benthos Res. 13, 136–141.
Kise, H., Fujii, T., Masucci, G., Biondi, P., and Reimer, J. D. (2017). Three new species and the molecular phylogeny of Antipathozoanthus from the Indo-Pacific Ocean (Anthozoa, Hexacorallia, Zoantharia). ZooKeys 725, 97–122. doi: 10.3897/zookeys.725.21006
Kise, H., and Reimer, J. D. (2016). Unexpected diversity and a new species of Epizoanthus (Anthozoa, Hexacorallia) attached to eunicid worm tubes from the Pacific Ocean. ZooKeys 562, 49–71. doi: 10.3897/zookeys.562.6181
Kosaki, R. K., Pyle, R. L., Leonard, J. C., Hauk, B. B., Whitton, R. K., and Wagner, D. (2017). 100% endemism in mesophotic reef fish assemblages at Kure Atoll Hawaiian Islands. Mar. Biodiv. 47, 783–784.
Lindsay, D. J., Yoshida, H., Ishibashi, S., Umetsu, M., Yamaguchi, A., Yamamoto, H., et al. (2013). “The uROV PICASSO, the Visual plankton recorder, and other attempts to image plankton,” in Proceedings of the 2013 IEEE International Underwater Technology Symposium (UT), (Tokyo: IEEE), doi: 10.1109/UT.2013.6519854
Lindsay, D. J., Yoshida, H., Uemura, K., Yamamoto, H., Ishibashi, S., Nishikawa, J., et al. (2012). The untethered remotely-operated vehicle PICASSO-1 and its deployment from chartered dive vessels for deep sea surveys off Okinawa, Japan, and Osprey Reef, Coral Sea, Australia. Mar. Technol. Soc. J. 46, 20–32.
Love, M., Hyland, J., Ebeling, A., Herrlinger, T., Brooks, A., and Imamura, E. (1994). A pilot study of the distribution and abundances of rockfishes in relation to natural environmental factors and an offshore oil and gas production platform off the coast of southern California. Bull. Mar. Sci. 55, 1062–1085.
Low, M. E., Sinniger, F., and Reimer, J. D. (2016). The order Zoantharia Rafinesque, 1815 (Cnidaria, Anthozoa: Hexacorallia): supraspecific classification and nomenclature. ZooKeys 641, 1–80. doi: 10.3897/zookeys.641.10346
Montenegro, J., Sinniger, F., and Reimer, J. D. (2015). Unexpected diversity and new species in the sponge-Parazoanthidae association in southern Japan. Mol. Phylogenet. Evol. 89, 73–90. doi: 10.1016/j.ympev.2015.04.002
Nishikawa, J., Fitzpatrick, R., Reimer, J. D., Beaman, R. J., Yamamoto, H., and Lindsay, D. J. (2011). In situ observation of the Denise’s pygmy seahorse Hippocampus denise associated with a gorgonian coral Annella reticulata at Osprey Reef, Australia. Galaxea. J. Coral Reef Stud. 13, 25–26.
Pyle, R. L. (1996). Exploring deep coral reefs: how much biodiversity are we missing? Glob. Biodiv. 6, 3–7.
Pyle, R. L. (1999). “Keeping up with the times: application of technical diving practices for in-water recompression,” in Proceedings of the Water Recompression: The Forty Eighth Workshop of the undersea and Hyperbaric Medical Society, eds E. Kay and M. P. Spencer (Durham: Undersea and Hyperbaric Medical Society and Diver’s Alert Network),74–88.
Pyle, R. L. (2000). Assessing undiscovered fish biodiversity on deep coral reefs using advanced self-contained diving technology. Mar. Technol. Soc. J. 34, 82–91.
Pyle, R. L., Boland, R., Bolick, H., Bowen, B. W., Bradley, C. J., Kane, C., et al. (2016). A comprehensive investigation of mesophotic coral ecosystems in the Hawaiian Archipelago. PeerJ. 4:e2475.
Reimer, J. D., Nonaka, M., Sinniger, F., and Iwase, F. (2008). Morphological and molecular characterization of a new genus and new species of parazoanthid (Anthozoa: Hexacorallia: Zoantharia) associated with Japanese Red Coral. Coral Reefs 27, 935–949.
Reimer, J. D., Poliseno, A., and Hoeksema, B. W. (2014). Shallow-water zoantharians (Cnidaria, Hexacorallia) from the Central Indo-Pacific. ZooKeys 444, 1–57. doi: 10.3897/zookeys.444.7537
Reimer, J. D., and Sinniger, F. (2010). Discovery and description of a new species of Abyssoanthus (Zoantharia: Hexacorallia) at the Japan Trench: the world’s deepest known zoanthid. Cah. Biol. Mar. 51, 451–457.
Reimer, J. D., Sinniger, F., Fujiwara, Y., Hirano, S., and Maruyama, T. (2007). Morphological and molecular characterisation of Abyssoanthus nankaiensis, a new family, new genus and new species of deep-sea zoanthid (Anthozoa: Hexacorallia: Zoantharia) from a north-west Pacific methane cold seep. Invert. Syst. 21, 255–262.
Rocha, L. A., Pinheiro, H. T., Shepherd, B., Papastamatiou, Y. P., Luiz, O. J., Pyle, R. L., et al. (2018). Mesophotic coral ecosystems are threatened and ecologically distinct from shallow water reefs. Science 361, 281–284. doi: 10.1126/science.aaq1614
Rowley, S. J. (2014). Refugia in the ‘twilight zone’: discoveries from the Philippines. Mar. Biol. 2, 16–17.
Sinniger, F., and Häussermann, V. (2009). Zoanthids (Cnidaria: Hexacorallia: Zoantharia) from shallow waters of the southern Chilean fjord region, with descriptions of a new genus and two new species. Org. Div. Evol. 9, 23–36.
Sinniger, F., Montoya-Burgos, J. I., Chevaldonné, P., and Pawlowski, J. (2005). Phylogeny of the order Zoantharia (Anthozoa, Hexacorallia) based on the mitochondrial ribosomal genes. Mar. Biol. 147, 1121–1128.
Sinniger, F., Ocana, O. V., and Baco, A. R. (2013). Diversity of zoanthids (Anthozoa: Hexacorallia) on Hawaiian seamounts: description of the Hawaiian gold coral and additional zoanthids. PLoS One 8:e52607. doi: 10.1371/journal.pone.0052607
Sinniger, F., Reimer, J. D., and Pawlowski, J. (2008). Potential of DNA sequences to identify zoanthids (Cnidaria: Zoantharia). Zool. Sci. 25, 1253–1260. doi: 10.2108/zsj.25.1253
Sinniger, F., Reimer, J. D., and Pawlowski, J. (2010a). The Parazoanthidae (Hexacorallia: Zoantharia) DNA taxonomy: description of two new genera. Mar. Biodivers. 40, 57–70.
Sinniger, F., Zelnio, K. A., Taviani, M., and Reimer, J. D. (2010b). Presence of Abyssoanthus sp. (Anthozoa: Zoantharia) in the mediterranean sea: an indication of non-dependence of Abyssoanthus to chemosynthetic-based ecosystems? Cah. Biol. Mar. 51, 475–478.
Stamatakis, A. (2014). RAxML version 8: a tool for phylogenetic analysis and post-analysis of large phylogenies. Bioinformatics 30, 1312–1313. doi: 10.1093/bioinformatics/btu033
Stewart, L. L., and Auster, P. J. (1989). “Low cost ROV’s for science,” in Oceans ’89, Vol. 3, ed. J. L. Scott (Piscataway, NJ: Institute of Electrical and electronics engineers), 816–819.
Stone, W. C. (1990). Exploring underwater with a failsafe diving rebreather. Sea Technol. 1990, 17–23.
Swain, T. D. (2009). Phylogeny-based species delimitations and the evolution of host associations in symbiotic zoanthids (Anthozoa, Zoanthidea) of the wider Caribbean region. Zool. J. Linn. Soc. 156, 223–238.
Swain, T. D. (2010). Evolutionary transitions in symbioses: dramatic reductions in bathymetric and geographic ranges of Zoanthidea coincide with loss of symbioses with invertebrates. Mol. Ecol. 19, 2587–2598. doi: 10.1111/j.1365-294X.2010.04672.x
Keywords: biodiversity, cryptic species, ecotone, rebreather, remotely operated vehicles, Okinawa, Red Sea
Citation: Reimer JD, Kise H, Santos MEA, Lindsay DJ, Pyle RL, Copus JM, Bowen BW, Nonaka M, Higashiji T and Benayahu Y (2019) Exploring the Biodiversity of Understudied Benthic Taxa at Mesophotic and Deeper Depths: Examples From the Order Zoantharia (Anthozoa: Hexacorallia). Front. Mar. Sci. 6:305. doi: 10.3389/fmars.2019.00305
Received: 29 December 2018; Accepted: 23 May 2019;
Published: 18 June 2019.
Edited by:
Johannes F. Imhoff, GEOMAR Helmholtz Centre for Ocean Research Kiel, GermanyReviewed by:
Cristina Dorador, University of Antofagasta, ChileAtoosa Noori Koupaei, Islamic Azad University, Iran
Copyright © 2019 Reimer, Kise, Santos, Lindsay, Pyle, Copus, Bowen, Nonaka, Higashiji and Benayahu. This is an open-access article distributed under the terms of the Creative Commons Attribution License (CC BY). The use, distribution or reproduction in other forums is permitted, provided the original author(s) and the copyright owner(s) are credited and that the original publication in this journal is cited, in accordance with accepted academic practice. No use, distribution or reproduction is permitted which does not comply with these terms.
*Correspondence: James Davis Reimer, anJlaW1lckBzY2kudS1yeXVreXUuYWMuanA=