- 1Red Sea Research Center, King Abdullah University of Science and Technology, Thuwal, Saudi Arabia
- 2Red Sea Research Center and Computational Bioscience Research Center, King Abdullah University of Science and Technology, Thuwal, Saudi Arabia
We investigated the occurrence of the sea skater Halobates spp. from samples collected at a fixed time series station and from six cruises along the north-south extend of the Red Sea during 2016–2018. Halobates germanus White, 1883 was the only species found during our study, its distribution ranging between 17° 9′N and 27° 27′N and between 35° 67′E and 41° 49′E. Total H. germanus abundance ranged between zero to 158,610 individuals km-2 with a considerable temporal (at the fixed station) and spatial (cruises) variation. In general, nymphs of different instar stages dominated the population, followed by females and males. The sex ratio was female-biased, with a higher number of females than males. Presence and prevalence of eggs, exuviae (molts), and early nymphal stages indicate a year round breeding cycle by H. germanus. The apparent optimum temperature for H. germanus occurrence in the central Red Sea was at 28.2°C, corresponding to peak abundances in spring and fall. Absence of any significant correlation of the abundances with environmental parameters indicate relative resilience of H. germanus to changes in environmental conditions.
Introduction
The surface microlayer of the sea is a highly dynamic and productive area inhabited by highly adapted organisms of the neuston and pleuston communities. The marine water strider or sea skater Halobates spp. (Hemiptera, Gerridae) is the only truly oceanic group of insects (i.e., completing their entire life-cycle in the open ocean), with a specialized epineustonic habit (Cheng, 1973, 1985; Andersen, 1991; Andersen and Cheng, 2004). Oceanic Halobates species were first discovered during a circumnavigation expedition on board a Russian vessel Rurik between 1815 and 1818, and further studied in the Challenger Expedition, half a century later (Andersen and Cheng, 2004).
Five species of sea skaters Halobates namely H. germanus White 1883, H. micans Eschscholtz, 1822, H. sericeus Eschscholtz, 1822, H. sobrinus White, 1883, and H. speldens Witlaczil, 1886, live permanently in the open ocean, at the air-sea interface (Herring, 1961; Cheng, 1985; Andersen, 1991). Both adults and juveniles spend their entire life on the sea surface, always at some distance from land (Andersen and Cheng, 2004). They prey on other animals of the pleustonic community and are themselves preyed upon by seabirds and pelagic fish (Cheng and Harrison, 1983; Cheng, 1985; Senta et al., 1993; Cheng et al., 2010). Most of the 47 species of Halobates that have been described so far are often associated with mangrove ecosystem and many are endemic to specific islands or island groups (Andersen and Cheng, 2004). The different oceanic species seldom co-occur, and areas of high density rarely overlap (Cheng and Shulenberger, 1976; Ikawa et al., 2004). Typically, only one Halobates species is dominant in any particular area and boundaries between ranges are often sharply delineated (Ikawa et al., 2012). Their occurrence is restricted mainly to the warm tropical and subtropical waters, roughly between latitudes 40°N and 40°S, where the winter temperature does not fall much below 20°C (Cheng, 1989; Andersen, 1991; Andersen and Cheng, 2004; Ikawa et al., 2007).
The Red Sea is an unique tropical oligotrophic embayment harboring the most diverse and endemic biota in the world (Dibattista et al., 2016). It is largely a coastal ocean, with a deep (up to 2,800 m) central zone and extensive shallow areas of varied habitats including mangroves, seagrass beds and coral reefs (Bruckner et al., 2011). This narrow desert-enclosed water body is characterized by distinctly high temperatures, high salinity levels of up to 41‰ (Douabul and Haddad, 1970; Âbdallah, 1985) and receives high surface irradiance (Winters and Geesthacht, 2003) year around. A strong latitudinal gradient of temperature extending from the far north (with an annual mean sea surface temperature of 26°C ± 1°C) to the south (with an annual mean sea surface temperature of 31.3°C ± 1.1°C), and a remarkable differences in salinity and primary productivity along this gradient supports a highly diverse and productive ecosystems along the entire coast (Raitsos et al., 2013; Sawall et al., 2015; Chaidez et al., 2017).
Several studies have explored the planktonic communities of the Red Sea (e.g., Holdway and Maddock, 1983; Bottger Schnack, 1995; Pearman and Irigoien, 2015), however, the knowledge of distribution and occurrence of Halobates in the region is sparse. Presence of three species of the genus Halobates namely H. hayanus, H. melleus (synonym: H. mangrovensis), and H. germanus have been previously reported in the Red Sea and its adjoining waters (Schmidt and Muller, 1973; Cheng, 1985). H. hayanus was found near Mersa Halaib (southern Egypt), in the Gulf of Zula and off Aden. H. germanus has been recorded frequently from the Gulf of Aden and in the Red Sea. H. melleus which lives very close to the shore was found under the mangroves of Nabq (Gulf of Aqaba) and in the Gulf of Zula. Here, we present the distribution, abundance and population structure of Halobates, along an extensive area of the Saudi Arabian coast of the Red Sea extending from the mouth of Gulf of Aqaba in the north (28°.03′N) to Jizan in the south (17°.32′N), based on samples collected from a fixed time series and cruise surveys over a period of 2 years.
Materials and Methods
Study Sites
Samples were collected from a fixed pelagic time series station (22.3093°N, 38.9612°E) between September 2016 and May 2018 and from several locations along the north-south extent of the Saudi Arabian coast of the Red Sea during six cruises during 2016–2017 (Figure 1). The sampling areas were identified along the latitudinal extend of the Red Sea as: the southern Red Sea (SRS) (between 15°and 20°N), the central Red Sea (CRS) (between 20°and 23.3°N) and the northern Red Sea (NRS) (beyond 23.3°N). The pelagic time series station was located in the off-shore waters of the CRS, nearly 13 km from the coast. The stations surveyed during the cruises extended between 2.5 and 151.4 km away from the land, covering coastal habitats of mangrove, saltmarsh, seagrass meadows, coral reefs, and pelagic open waters. Sea surface temperatures ranged between 24.6 and 32.1°C (28.7 ± 2.41) at the fixed station. At the fixed station, fortnightly sampling frequency yielded 38 samples, while a total of 81 samples were collected during the cruises (Figure 1).
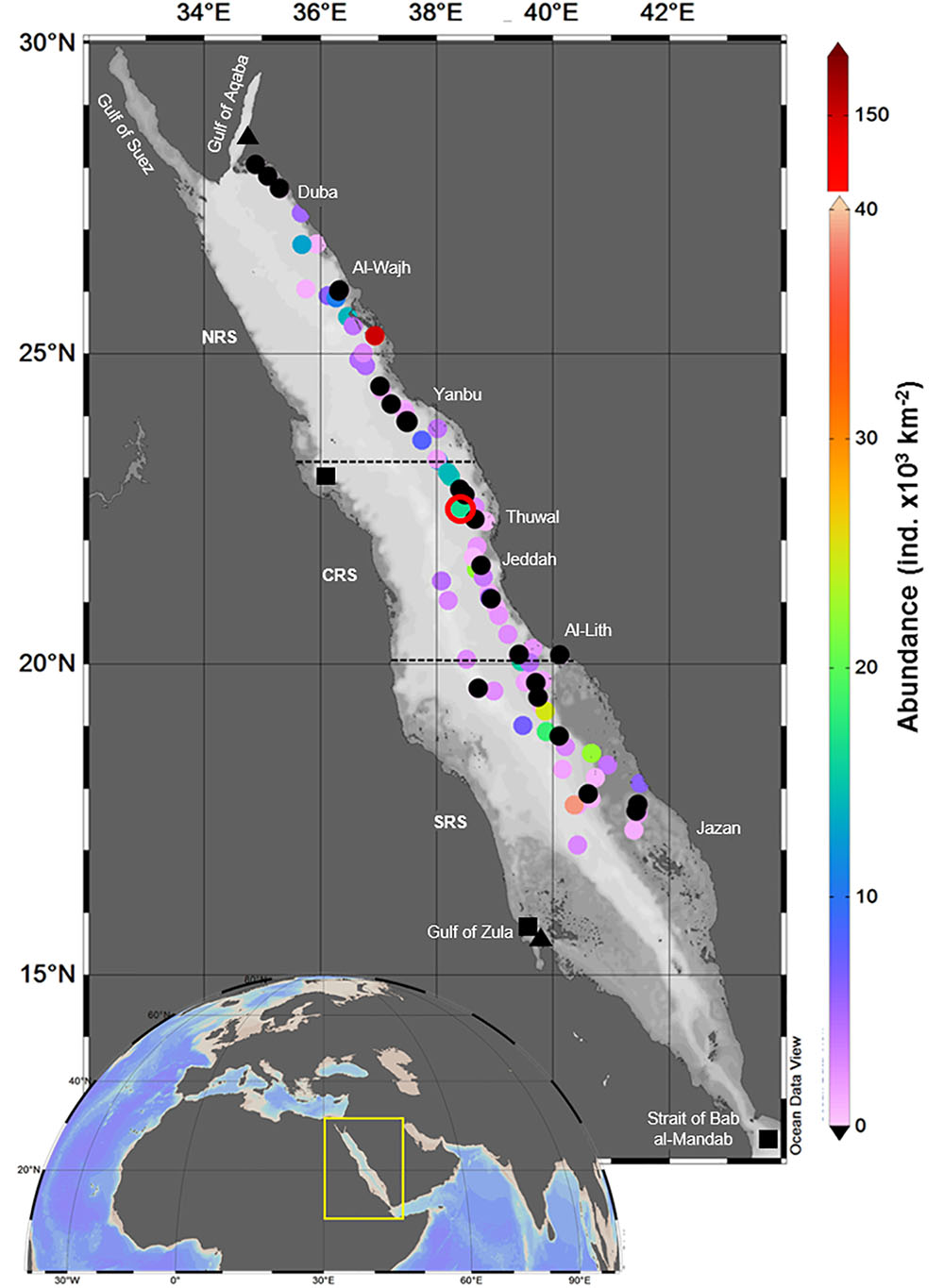
Figure 1. Sampling locations (colored dots, time series station outlined in red) and corresponding abundance of Halobates germanus (individuals × 103 km-2) in the Red Sea during 2016–2018. The regions of the northern Red Sea (NRS), the central Red Sea (CRS), and the Southern Red Sea (SRS) are delineated with black dash lines. Abundance values are represented according to the color palette on the right. Note the difference in the scale bar. Black dots correspond to locations with negative tows (absence of H. germanus in the samples). Abundance at the time series station is the average of 38 samples. Previously reported occurrence of other two species, H. melleus (black triangle) and H. hayanus (black square) is also shown.
Sampling
Sampling was carried out using a 200 μm rectangular neuston net with a 60 cm × 20 cm mouth opening at the fixed station, and a 150 μm net with 50 cm × 15 cm mouth opening for all the cruises. The net was deployed from the side of the vessel to avoid the propellers and was typically towed at the sea surface for 30 min at 2 knots. During the entire towing operation, the net was regularly monitored to ensure that the mouth opening remained 2/3 rd submerged under water. The effective surface area of water sampled was estimated by multiplying the width of the nets and the distance covered during the tows. All samples at the fixed station were collected during day time (mid-day), while sampling during the various cruises was carried out at different times of the day. At the fixed station, temperature and salinity of the sea surface was recorded using an Idronaut Ocean seven 316 Plus profiler. Once aboard, the net was rinsed with seawater and the contents were concentrated into the cod-end jar. The sample was then transferred carefully in labeled storage bottles with the help of a funnel and stored in 96% ethanol.
Identification and Determination of Abundance
The insects were sorted from the rest of the neuston and preserved in 96% alcohol. They were all identified as far as possible to species, sex and developmental stage. The life cycle of Halobates includes egg, nymph (N) instars at different stages of development, and the adult stage of female (F) and male (M) (Cheng, 1981). Identification was carried out using the Key for the Identification of Halobates Eschscholtz and Related Genera (Hemiptera-Heteroptera: Gerridae) (Andersen and Cheng, 2004). Male specimens were mainly used for species level identification and females were used in absence of males in the samples. All the stages of nymphs were assumed to be of the same species as the adults. Nymphs were further separated into four group as nymph I (1st and 2nd instar stages), nymph II (3nd instar stage), nymph III (4th instar stage) and nymph IV (5th instar stage) according to their body size. Presence of eggs and exuviae (molts) of Halobates and occurrence of other insects in the samples was also reported. Identification and counts were performed using a Leica IC80 HD stereoscope microscope.
Data and Statistics Analysis
We have included the data from both positive and negative tows for the estimation of total abundances (F + M + N). Abundance values are individuals caught per standard tow reported as number of individuals km-2 (ind. km-2). Abundance estimates are conservative given the net avoidance behavior exhibited by Halobates. For reporting the percentages of each developmental stage in the entire population, we have used the values only from the positive tows with nymphs (26 and 44 positive tows, at the fixed station and the cruise survey, respectively). Values are presented as mean ± standard error. Each data point represents at least one net tow or the mean of several net tows of comparable sampling efficiency. Seasons were categorized as spring (March–May), summer (June–August), fall (September–November), and winter (December–February).
Sex ratio was estimated as the number of males per 100 females (M:F) and a chi-square test was used to verify departures from a 1:1 sex ratio. Abiotic parameters such as temperature, salinity, chlorophyll a and oxygen were tested by linear correlation to analyze if any of them could explain the variability of Halobates distribution. Also, Pearson’s correlation was used to assess whether there is a correlation between the abundance and the distance from the coast. One-way analysis of variance (ANOVA) was used to test for differences in the abundance and percentage values. Significant differences were recorded at p < 0.05. Data and statistical analyses were carried out using Microsoft® Excel 2013. Multivariate analysis was performed using R.
Results
Distribution and Abundance
During this study, a total of 119 samples were collected across the different locations along the Saudi Arabian coast of the Red Sea. Individuals were recorded up to 17°.09′N in the SRS, however, no individuals were recorded north of 27° 27′N in the NRS, the longitudinal range of their occurrence was between 35° 67′ and 41° 49′E (Figure 1). Although there was no correlation between the abundance and the distance of sampling locations from the coast (Pearson’s correlation coefficient, r = -0.04, p > 0.05), no individuals were found in samples collected at stations within 6.0 km from the coast, irrespective of the habitats.
A total of 464 individuals of Halobates were caught from the 91 positive tows. In general, during the survey, 23.5% of the tows did not yield any insects. Also, for the positive tow, the number of insects caught per tow varied greatly with catches ranging from 1 to 220 individuals (Table 1). The population of Halobates was exclusively of H. germanus White, 1883, individuals (Figure 2). At the fixed station, the abundance ranged between 2,030 and 86,200 ind. km-2, with a mean value of 16,250 ± 2,640 ind. km-2 (Figure 3). Out of the 38 samples, 18% of the samples did not have any sea skaters and 7.89% of samples had only exuviae but no sea skaters. For the cruise samples, the total abundance ranged broadly between 630 and 158,610 ind. km-2, with a mean of 6,180 ± 2,050 ind. km-2, with 25.92% of the 81 samples had no individuals or exuviae present (Table 1). There was no significant difference in the abundance with latitude, the mean abundance values being similar in the NRS, CRS and SRS (ANOVA, p = 0.43).
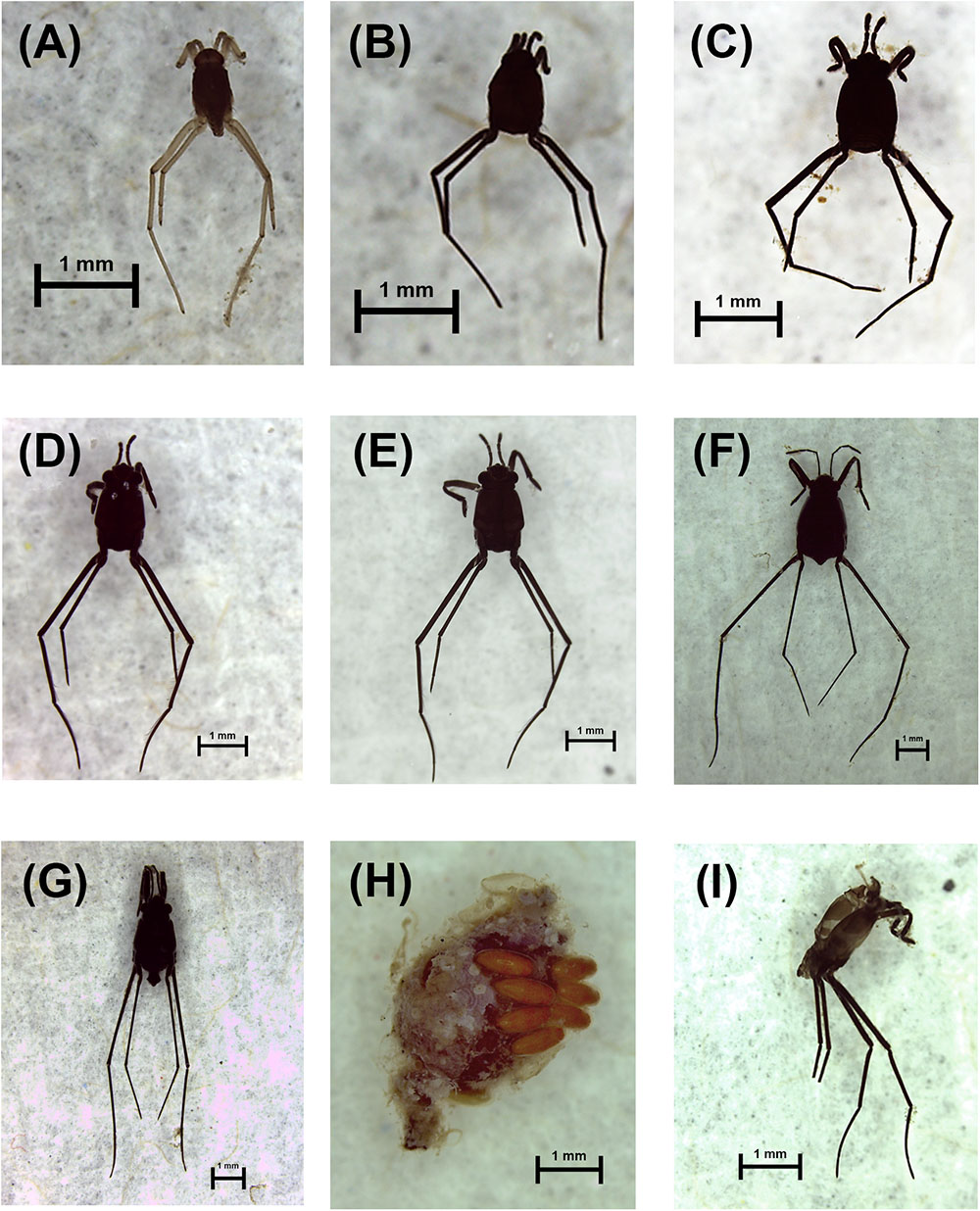
Figure 2. Different stages of life cycle of Halobates germanus. (A–E) 1st to 5th instar stages, (F) adult female, (G) adult male, (H) eggs on Sargassum spp. pneumatocyst, and (I) exuviae. All scale bars = 1 mm.
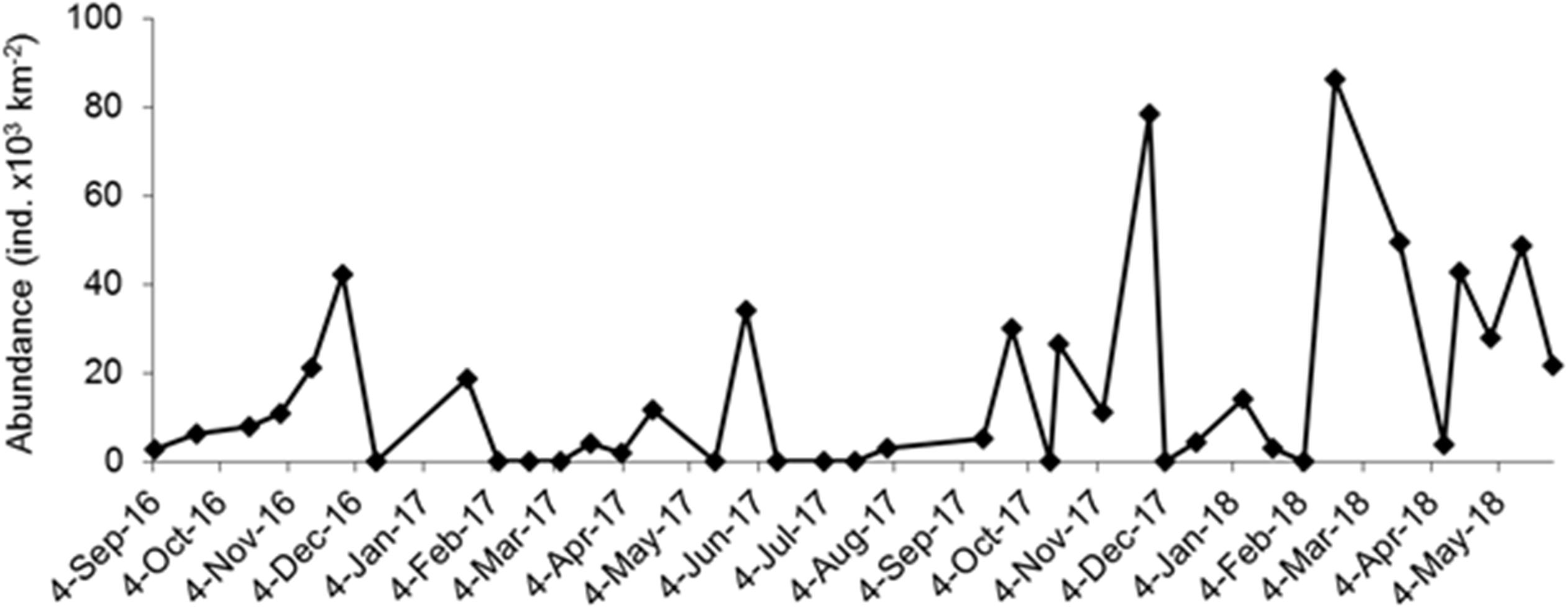
Figure 3. Variation in the abundance (individuals × 103 km-2) of Halobates germanus population (females, males, and nymphs) at the fixed station in central Red Sea during 2016–2018.
Life Cycle Components and Population Structure of H. germanus
The populations of H. germanus at the fixed station and the cruise survey were composed of nymphs at different stages of development (Figures 2A–E), adults (Figures 2F,G), eggs (Figure 2H), and exuviae (Figure 2I). Both at the fixed station and during the cruises surveys, nymphs were dominant and represented 87.2 ± 16.8 and 57.6 ± 4.5% of the total population, respectively, followed by females and males (Figures 4A, 5A). Exuviae were found in 55.2% of the fixed station samples and 28.4% of the cruise samples (Figures 4A, 5A). Eggs, mostly attached to floating Sargassum pneumatocyst (Figure 2H), were found in all seasons; in 7 tows at the fixed station (February, March, September, and November) and in 10 cruise samples, collected in the months of March, April, July, and November. Nymphs including instar 1st, 2nd, 3rd, 4th, and 5th were identified across different samples. In general, the 1st and 2nd instar were more abundant and together comprised 49.9 ± 10.0 and 48. 7 ± 4.7% of the total nymph population at the fixed station and for the cruises, respectively. The abundance of nymphs declined with increasing instars, percentage contribution of the 5th instar being the least at the fixed station and for the cruise stations (Figures 4B, 5B).
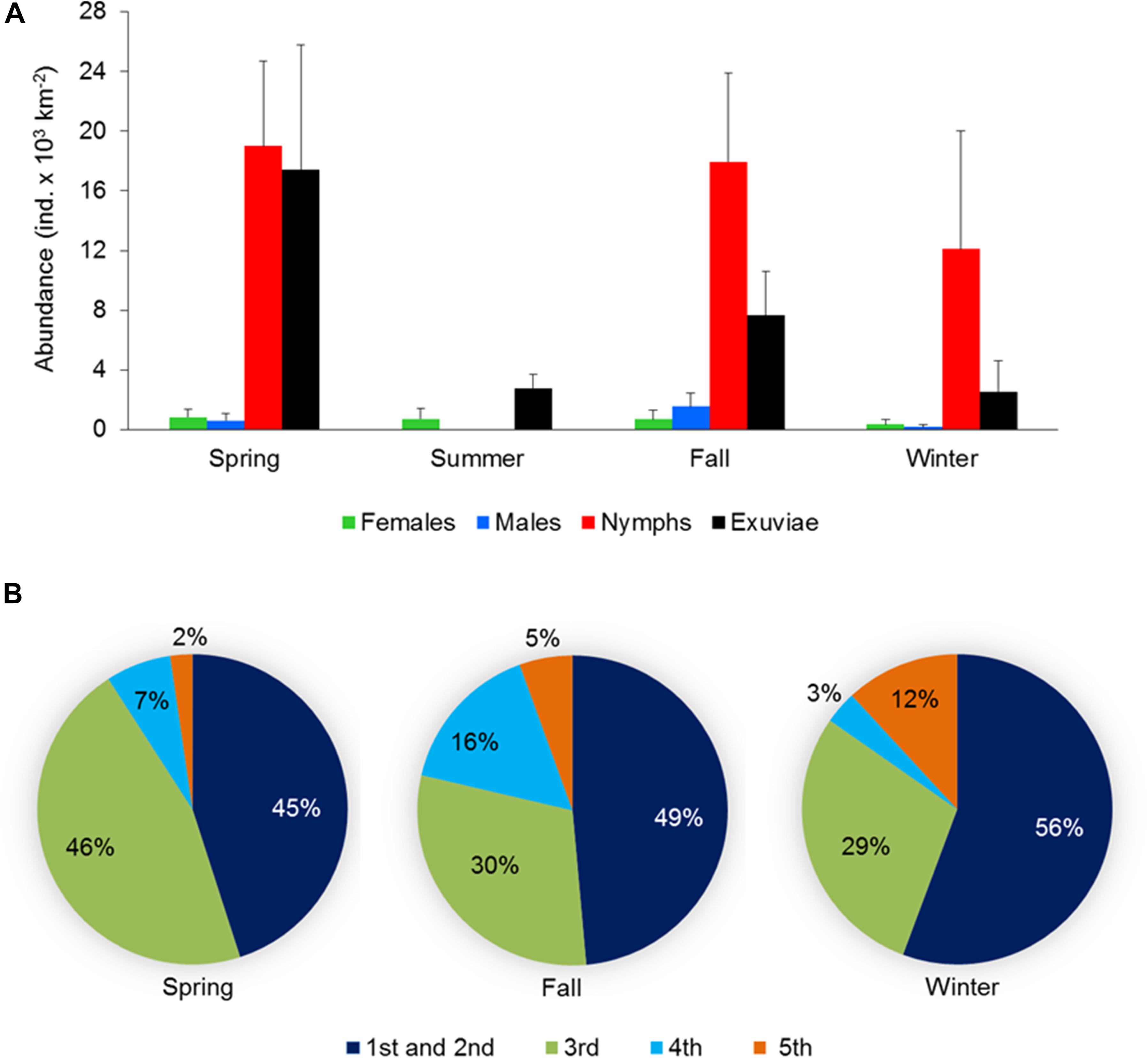
Figure 4. (A) Abundance and population structure (females, males, and nymphs) and exuviae of Halobates germanus during different seasons and (B) relative contribution of different instar stages to the total nymph population observed at the fixed station in central Red Sea during 2016–2018. Error bars indicate standard error of the mean.
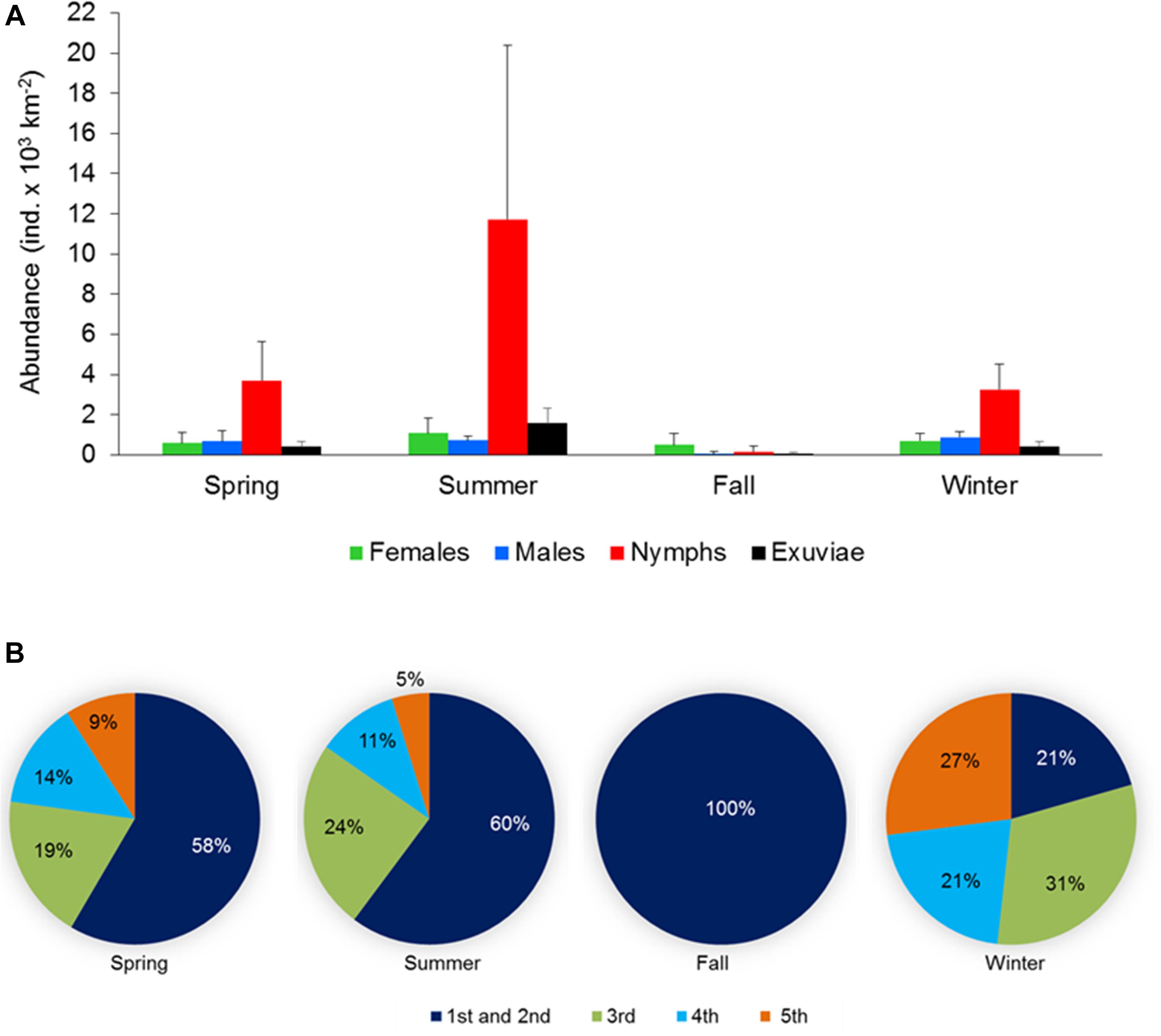
Figure 5. (A) Abundance and population structure (females, males, and nymphs) and exuviae of Halobates germanus and (B) relative contribution of different instar stages to the total nymph population during different season observed at different locations surveyed during the cruises along the north-south extend of the Red Sea during 2016–2018. Error bars indicate standard error of the mean.
Seasonal variations were observed in the population structure at the fixed station as well as in the samples collected during the cruises, although the difference were not significant. At the fixed station, nymphs dominated the population in all seasons except during summer when the population was exclusively of females (Figure 4A). During the remaining seasons, the sex ratio was also female-biased, with highest value of 0.33 during winter. Abundance of exuviae was highest during spring, followed by fall and showed a sharp decline during summer and winter (Figure 4A).
For the cruise samples, adults and nymphs were present in the population during all the seasons despite the seasonal changes in their composition (Figure 5A). The population structure was similar during spring and winter, with the highest percentage of nymphs followed by males and females and a sex ratio of 0.53 and 0.55, respectively. During summer, nymphs still dominated the population, but the percentage of females was higher than that of the males, resulting in a female-biased sex ratio of 0.40. The fall population was dominated by females (67.5%), followed by the nymphs and males. The sex ratio during fall was therefore highly female biased (0.11). Occurrence of exuviae co-occurred with the nymph population, with the highest and lowest number of exuviae found during summer and fall, respectively. During spring and winter, similar to the population structure, the number of exuviae was also similar (Figure 5A).
Environmental Parameters at the Fixed Station
At the fixed station, the temperature recorded ranged between 24.4 and 32.1°C (mean ± SE: 28.7° ± 0.4°C). Salinity ranged from 37.9 to 40.9 PSU (mean ± SE: 39.3 ± 0.1). Oxygen at the surface ranged between 4.2 and 6.4 ppm (mean ± SE: 5.4 ± 0.1 ppm). Chlorophyll a values varied significantly ranging between 0.1 and 0.8 μg L-1 (mean ± SE: 0.4 ± 0.0). Temperature and salinity showed a similar trend with higher values during summer and fall, and relatively lower values during spring and winter. Oxygen was inversely related to the temperature. Chlorophyll a was higher during winter and lowest during summer.
There was no significant correlation of the abundance of H. germanus (total, adults and nymphs) with temperature (r2 = 0.01, p = 0.57), salinity (r2 = 0.00, p = 0.77), or chlorophyll a (r2 = 0.11, p = 0.09) (Figure 6). However, there was a parabolic relationship between the abundance of H. germanus and sea surface temperature, suggesting a narrow temperature range for H. germanus, with an apparent optimum temperature estimated as 28.2° C (Figure 7).
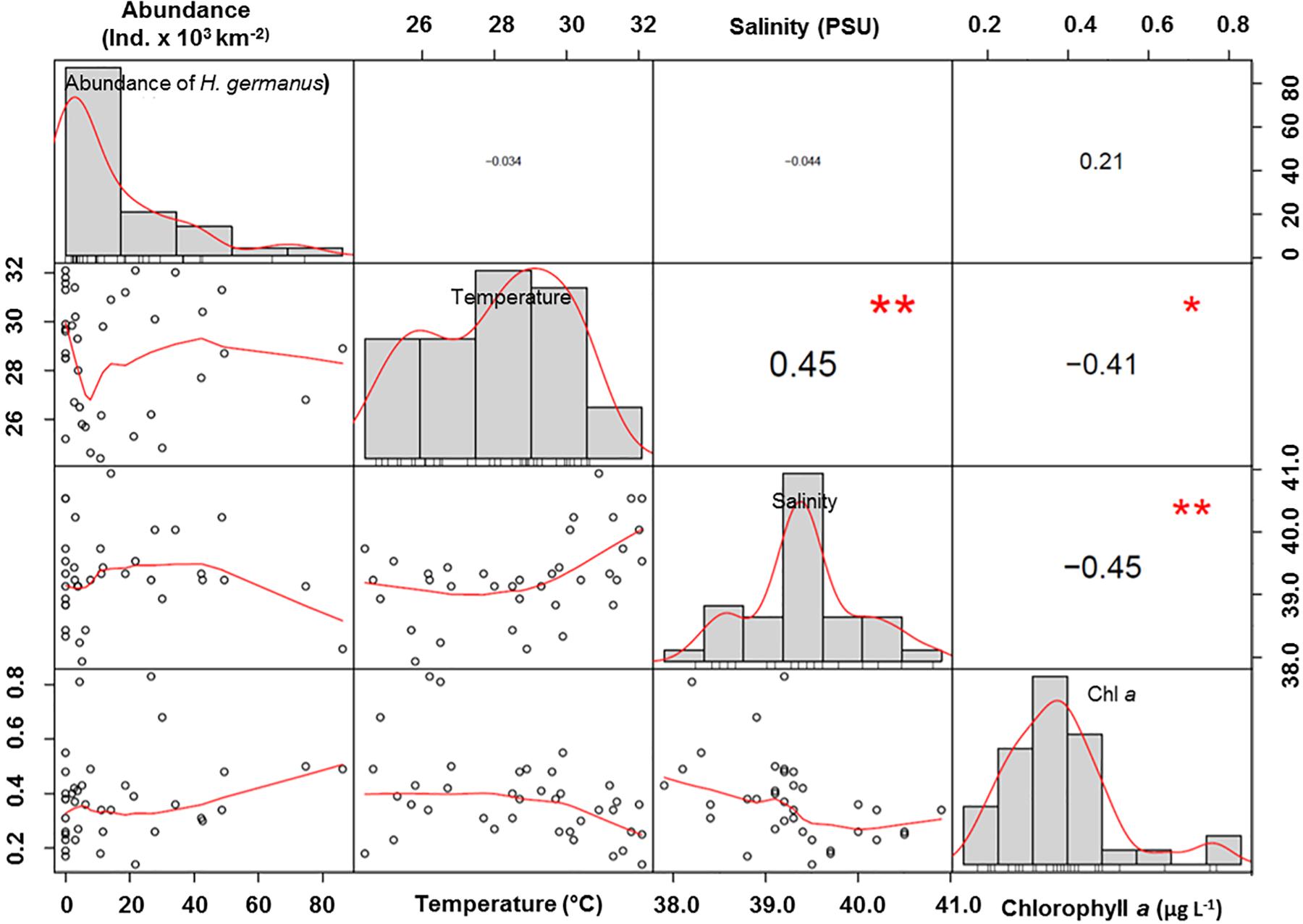
Figure 6. Correlations of Halobates germanus abundance with environmental variables of temperature, salinity and chlorophyll a. The distribution of each variable is shown on the diagonal. Bivariate scatter plots with a fitted line show the relationship between the two variables. Values are Pearson’s correlation co-efficient. Bold values show maximal correlation. ∗p < 0.05; ∗∗p < 0.01; ∗∗∗p < 0.001.
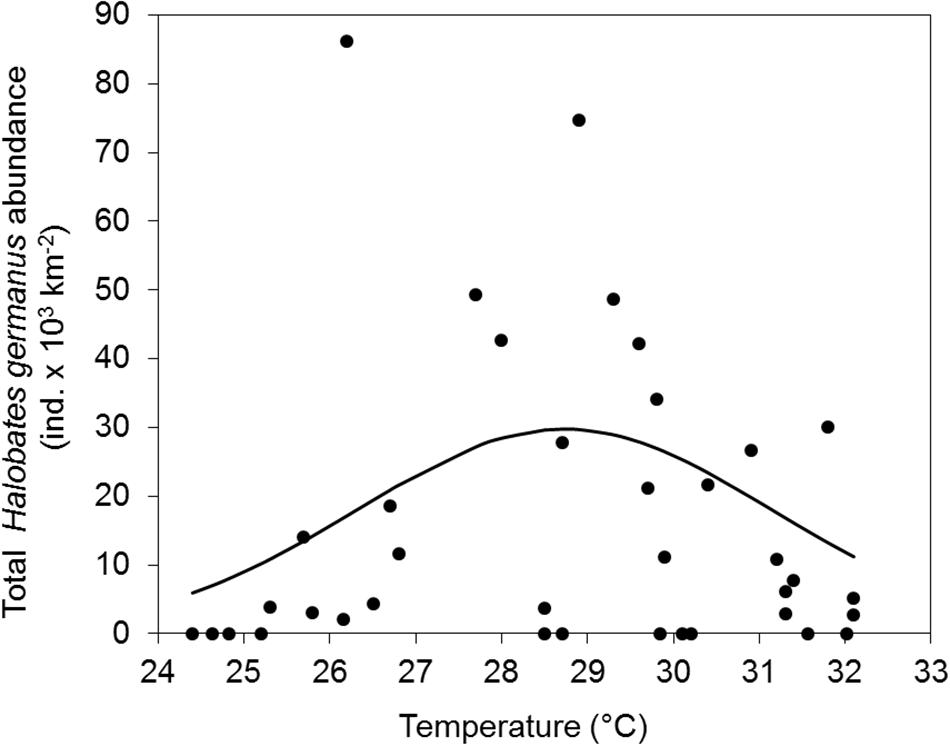
Figure 7. Temperature preference of Halobates germanus at the fixed station in the central Red Sea during 2016–2018. Gaussian curve is adjusted to total H. germanus abundances versus temperature (°C). The optimum temperature estimated is as 28.2°C.
Discussion
Distribution and Abundance
Halobates germanus was the only species found in the samples collected from several locations during this study, extending from the northern tip of the Red Sea to the south. On a global scale, the presence of H. germanus is reported in a latitude band extending from the Equator to 40°N in the northern hemisphere (Andersen and Cheng, 2004), except for the Mediterranean Sea (Cheng et al., 2012). Its distribution is restricted to the western and central half of the Pacific Ocean and the Indian Ocean. In the Indian Ocean, its range skirts the land masses and it is commonly found around the islands and island groups of the Indo-Malaysian region (Herring, 1961). H. germanus is the only pelagic species found in the Red Sea and the Gulf of Aqaba (Schmidt and Muller, 1973; Cheng and Holdway, 1983; Cheng, 1989). Previously, the presence of H. germanus has been documented in the Red Sea and in the adjoining Gulf of Aden (Sagaydachnyy, 1975; Cheng and Holdway, 1983), its distribution extending from near the northern tip of the Red Sea (Schmidt and Muller, 1973) to the southern two-thirds of the sea (Cheng and Holdway, 1983). In addition to H. germanus, two other species of Halobates associated with mangrove habitats, namely, H. hayanus and H. melleus, Linnavuori, 1971 (H. mangrovensis) have also been reported from the Red Sea (Schmidt and Muller, 1973; Por et al., 1977; Andersen and Cheng, 2004), but we did not find any of these species in our study. While we sampled different ecological habitats like mangroves, seagrass meadows and coral reefs during our survey, the sampling stations did not cover areas very close to the land, the closest sampling point being 2.45 km away from the land. Given the distribution of these two species being restricted to the shallower, nearshore areas, very close to the land, it is possible that these two species were not caught during our survey. In fact, Halobates individuals were caught only in locations beyond 6.07 km away from the land during our survey.
Halobates germanus is characterized among the five oceanic Halobates species for a tendency to stay closer to the coasts than other oceanic species (Cheng, 1989). Preference for areas close to islands or margin of continents suggests a stronger affinity of H. germanus for coastal environments (Ikawa et al., 2008, 2012). Polhemus (1990) reported the occurrence of this species nearly one kilometer or more offshore, either over the fore reef or on the deeper offshore waters of the Aldabra and Cosmoledo atolls in the western Indian Ocean. During the 2 year circumnavigational cruise of “Operation Drake,” individuals of H. germanus were caught at several stations in the Gulf of Aden and the Red Sea with population densities up to 24 individuals per standard 30-min tow. No specimen was however, caught in the open stretch of the Indian Ocean (Cheng and Holdway, 1983). Difference in food preferences has been suggested to be a possible explanation for the prevalence of H. germanus in coastal water unlike the other oceanic Halobates species (Sagaydachnyy, 1975). However, based on our samples, we document the presence of H. germanus in the open waters of the CRS (extending 151.3 km) from the coast, the further distance from the shore possible in this narrow sea.
In terms of density (abundance of individuals per square kilometer), the values in this study are comparable to abundances of H. germanus reported in the Pacific Ocean (11,688) (Furuki et al., 2016), Western Pacific Ocean (4,100), East China Sea (12,000) (Ikawa et al., 2004), Banda Sea (15,000) (Cheng et al., 1990), and in the Red Sea (22,500) (Cheng and Holdway, 1983). The average number of individuals caught per tow during this study was 4.3 ± 1.6 (5.1 ± 1.7 considering only the positive tows). These values are much lower than previously reported a mean of 27.3 individuals per tow in the Red Sea (Cheng and Holdway, 1983). Also in the Indian Ocean and Java Sea, similarly higher values with catches of 25.6 and 46.2 individuals of H. germanus have been reported. The highest number of individuals per tow (220) during this study was higher than previously reported catch of 78 and 141 individuals per tow in the Red Sea and the Java sea, respectively (Cheng and Holdway, 1983), but lower than the catch of 584 individuals caught in a single tow in the Banda sea (Cheng et al., 1990).
In addition to the wide range of the number of individuals caught per tow (0–220), a significant difference was observed in abundance values both at the fixed station and the cruise stations. While these variations could be primarily due to geographical and environmental factors, they could also be due to the occasional patchy occurrence of Halobates in the sea. Individuals of Halobates are known to form aggregations called flotillas, where they gather in high densities of 500 m-2 to avoid predation (Treherne and Foster, 1980, 1982). Also, Halobates can sense the approaching nets and are able to escape the nets by skating away (Cheng and Enright, 1973). We, therefore, consider our estimates of abundance, as well as other estimates reported, to be conservative due to possible net avoidance behavior by Halobates.
Life Cycle and Population Structure
During this study, we report the occurrence of adults, nymphs of all five instar stages of H. germanus, eggs as well as exuviae across the extensively surveyed area of the Red Sea. Oceanic Halobates species are independent of land throughout their entire life-cycle, and display specialized reproductive strategies like mating and egg-laying at the sea surface. They seem to reproduce all year around (Spence and Andersen, 1994; Andersen and Cheng, 2004) and the entire life-cycle from egg to adult may take 2–3 months (Herring, 1961; Cheng, 1985). The long oviposition period, rather low growth rates and long life spans could have been selected due to their extremely harsh and unpredictable environment of the ocean surface, where strong winds and storms occur too frequently to allow them to form a stable localized aggregation and to complete even one generation. This adaptive life cycle strategies would give them better chances to find conspecifics, food and suitable habitats to regroup and reproduce even after they are dispersed over long distances (Ikawa et al., 2012).
The nymphs resemble adults in general external morphology but are smaller. The first-instar nymphs measure only about 1 mm in body length, and go through five molts before reaching the adult stage. While there is no information available on the diet of the early nymphal stages, adults and older nymphs are predators, preying upon small zooplankton trapped on the sea surface (Cheng, 1985). Their mouthparts are of piercing and sucking type, an adaptation for preying by penetrating and then sucking the fluids out of the prey. Cannibalism is also not uncommon and usually involves adults feeding on small sized nymphs (Spence and Andersen, 1994). The occurrence of cannibalism seems to be dependent on the availability of alternate food source (Andersen and Cheng, 2004). Moreover, the oceanic species also have a food reserve in the form of neutral triglyceride lipids (Lee and Cheng, 1974), which may allow it to withstand much longer periods of starvation. Higher abundance and dominance of nymphs, especially the early 1st and 2nd instars during this study, indicates their prevalence throughout the year across the surveyed area. Moreover, the presence of eggs and exuviae in the samples during all the seasons also suggests a year round reproductive and growth of this species.
In general, the sex ratio was highly female-biased during this study. Relatively higher proportion of females than males, resulting in female-biased sex ratios, have also been reported for H. micans (Miyamoto and Senta, 1960; Cheng, 1971; Dias and Lopes, 2009). Cheng (1971) suggests that such predominance of females in the population may be due to their prolonged longevity, permitting them to lay their full complement of eggs. An extended lifespan would also increase the chance of finding a suitable flotsam, which is generally scarce in open ocean, for oviposition until some days after egg maturation and mating, a life-cycle strategy important for the reproductive success of the species. Conversely, the predominance of females can also be beneficial for proliferation of the species as each male can mate with several females (Dias and Lopes, 2009).
Relationship of H. germanus Occurrence With Environmental Parameters
The distribution and abundance of Halobates across the ocean surface are known to be influenced by several environmental factors like temperature (e.g., Ikawa et al., 2004; Harada et al., 2018), salinity, wind (Ikawa et al., 2007), precipitation (Harada et al., 2016), oxygen and chlorophyll (Harada et al., 2014).
Higher abundances of the oceanic species H. micans in Indian Ocean were found to be associated with high chlorophyll and low oxygen concentrations, suggesting that the fundamental phytoplankton biomass and the resulting higher zooplankton food availability can affects the population density of Halobates (Harada et al., 2014). On the contrary, abundance of H. germanus were found to be higher under oligotrophic conditions, associated with the lowest chlorophyll (Cheng et al., 1990). The population of H. germanus in this study also did not show any correlation with chlorophyll or oxygen, thereby suggesting its dependence on alternative food source and its carnivorous feeding habit (Cheng, 1985). The Red Sea, despite its prevailing oligotrophic conditions, supports a diverse and abundant zooplankton and neuston community (Echelman and Fishelson, 1990; Pearman and Irigoien, 2015; Al-Aidaroos et al., 2016), which is ample prey for Halobates (Cheng, 1974, 1985). We, therefore, speculate that H. germanus, as a specialized predator (Cheng, 1974), is well adapted to the food conditions of the Red Sea.
Surface temperature has been reported as the most prevailing factor controlling the distribution and abundance of Halobates species (Cheng and Shulenberger, 1976; Cheng, 1985; Nakajo et al., 2013). Experimental work also validates the narrow temperature tolerance that varies between different oceanic species and the critical effect of small variations in the environmental temperature on the physiology of Halobates (Harada et al., 2011, 2013). In general, a temperature range of 24–30°C had been estimated as optimum for oceanic sea skaters (Cheng and Shulenberger, 1976). Harada et al. (2018) reported the distribution of H. sericeus across a wide temperature range of 22–30°C and a critical lower temperature for inhabitation at 21°C, which reflect in its distribution over a wider range of latitudes compared to other oceanic species (Andersen and Cheng, 2004). On the contrary, H. germanus is found across a narrow temperature range of around 27–30°C, and a lower temperature limit of temperature for inhabitation around 28°C (Harada et al., 2014). In our study, however, H. germanus was found within a much wider temperature of 24.8–32.1°C, and an optimum temperature of 28.2°C. The presence of H. germanus over a wide thermal range (7°C) suggests an adaptation of this species to severe seasonal temperature fluctuation as well as its capability to adapt to higher ambient temperatures, a feature common to organisms inhabiting warmer temperature regimes (Schoepf et al., 2015) and, particularly, the Red Sea (Krueger et al., 2017; Osman et al., 2018). The occurrence of H. germanus at temperatures of 32.1°C in the present study is more than the “moderate high” temperature of 31°C which is “maximum” high ambient temperature for tropical oceanic sea skaters, and, very close to 32.5°C, a temperature previously associated with lower survival and lower tolerance for this species in the Pacific Ocean (Furuki et al., 2018). The local population therefore seems to demonstrate a much efficient temperature adaptation system compared to the population of H. germanus in the Pacific Ocean.
Conclusion
This study is the first comprehensive account of occurrence and distribution of Halobates in the Saudi Arabian coast of the Red Sea, conducted over a large spatiotemporal scale. The Red Sea features a gradients of temperature, salinity and nutrients (Edwards, 1987), which reflects in the gradual increase in primary production and nutrient concentrations from the north to south and toward the coasts (Raitsos et al., 2013), which would result in a highly dynamic changes both temporally and spatially on the distribution of Halobates. However, we found a prevalence of H. germanus in all its life stages across the latitudinal extend of the Saudi Arabian Red Sea in diverse ecological habitats. The distribution although variable and patchy, was not controlled by a single environmental factor, although an apparent optimal temperature of 28.2°C was observed. H. germanus in the Red Sea appears to be adapted to resist higher temperatures than populations elsewhere, which needs be confirmed experimentally. This is particularly interesting in the ongoing (Chaidez et al., 2017) and predicted warming of the Red Sea, and the subtropical and tropical ocean.
Data Availability
The datasets generated for this study are available on request to the corresponding author.
Author Contributions
SA and CD conceptualized and designed the study. GM processed the samples, analyzed the data, and led the writing of the manuscript. All authors reviewed and contributed to the writing of the final version of the manuscript.
Funding
This work was supported by the King Abdullah University of Science and Technology (KAUST) through baseline funding to SA and CD.
Conflict of Interest Statement
The authors declare that the research was conducted in the absence of any commercial or financial relationships that could be construed as a potential conflict of interest.
Acknowledgments
We thank Juan de la Cruz Martinez Ayala and Cecilia Martin for collecting the samples during the regular time series sampling and cruises, Juan de la Cruz Martinez Ayala for chlorophyll analyses and Alexandra Coello-Camba for help with data analyses. We also thank the KAUST R/V Thuwal crew for support.
References
Âbdallah, A. M. (1985). The Hydrological Structure and Circulation of the Red Sea. Ph.D. thesis, Oceanography Department, Faculty of Geography, University of Moscow, Russian.
Al-Aidaroos, A. M., El-Sherbiny, M. M., and Mantha, G. (2016). Spatial heterogeneity of zooplankton abundance and diversity in the Saudi coastal waters of the Southern Red Sea. Indian J. Geo Mar. Sci. 45, 70–85.
Andersen, N. M. (1991). Marine insects: genital morphology, phylogeny and evolution of sea skaters, genus Halobates (Hemiptera: Gerridae). Zool. J. Linn. Soc. 103, 21–60. doi: 10.1111/j.1096-3642.1991.tb00896.x
Andersen, N. M., and Cheng, L. (2004). The marie insect Halobates (Heteroptera: Gerridae): biology, adaptations, distribution, and phylogeny. Oceanogr. Mar. Biol. Annu. Rev. 42, 119–179.
Bottger Schnack, R. (1995). Summer distribution of micro- and small mesozooplankton in the Red Sea and Gulf of Aden, with special reference to non-calanoid copepods. Mar. Ecol. Prog. Ser. 118, 81–102. doi: 10.3354/meps118081
Bruckner, A., Rowlands, G., Riegl, B., Purkis, S., Williams, A., and Renaud, P. (2011). Khaled bin Sultan Living Oceans Foundation - Atlas of Saudi Arabian Red Sea Marine Habitats. Phoenix, AZ: Panoramic Press.
Chaidez, V., Dreano, D., Agusti, S., Duarte, C. M., and Hoteit, I. (2017). Decadal trends in Red Sea maximum surface temperature. Sci. Rep. 7, 1–8. doi: 10.1038/s41598-017-08146-z
Cheng, L. (1971). Ocean-striders from Melanesia (Hempitera: Gerridae, Halobates spp. Cah. ORSTOM Série Océanographie 9, 513–518.
Cheng, L. (1973). Marine and freshwater skaters: differences in surface fine structure. Nature 242, 132–133. doi: 10.1038/242132a0
Cheng, L. (1981). Halobates (Heteroptera: Gerridae) from micronesia with notes on a laboratory population of H. mariannarum. Micronesica 17, 97–106.
Cheng, L. (1985). Biology of Halobates (Heteroptera:Gerridae). Annu. Rev. Entomol. 30, 111–135. doi: 10.1146/annurev.ento.30.1.111
Cheng, L. (1989). Biogeography and phylogeny of the sea-skater Halobates. Chin. J. Oceanol. Limnol. 7, 233–239. doi: 10.1007/BF02842613
Cheng, L., Baars, M. A., and Oosterhuis, S. S. (1990). Halobates in the Banda sea (Indonesia): monsoonal differences in abundance and species composition. Bull. Mar. Sci. 47, 421–430.
Cheng, L., Damgaard, J., and Garrouste, R. (2012). The sea-skater Halobates (Heteroptera: Gerridae) - probable cause for extinction in the Mediterranean and potential for re-colonisation following climate change. Aquat. Insects 34, 45–55. doi: 10.1080/01650424.2012.643024
Cheng, L., and Enright, J. T. (1973). Can Halobates dodge nets? II: by moonlight? Limnol. Oceanogr. 18, 666–669. doi: 10.4319/lo.1973.18.4.0666
Cheng, L., and Harrison, C. S. (1983). Seabird predation on the sea-skater Halobates sericeus (Heteroptera: Gerridae). Mar. Biol. 72, 303–309. doi: 10.1007/BF00396836
Cheng, L., and Holdway, P. (1983). Halobates collected during the circumnavigational expedition ‘Operation Drake.’. Zool. J. Linn. Soc. 78, 187–198. doi: 10.1111/j.1096-3642.1983.tb00866.x
Cheng, L., and Shulenberger, E. (1976). Distribution and abundance of Halobates species (Insecta: Heteroptera) in the eastern tropical Pacific. Fish. Bull. Natl. Oceanic Atmos. Admin. 78, 579–591.
Cheng, L., Spear, L., and Ainley, D. G. (2010). Importance of marine insects (Heteroptera: Gerridae, Halobates spp.) as prey of eastern tropical Pacific seabirds. Mar. Ornithol. 38, 91–95.
Dias, J., and Lopes, C. (2009). Occurrence, distribution and abundance of Halobates micans Eschscholtz, 1822 (Heteroptera, Gerridae) along the southeastern Brazilian coast. Braz. J. Biol. 69, 67–73. doi: 10.1590/S1519-69842009000100008
Dibattista, J. D., Choat, J. H., Gaither, M. R., Hobbs, J. A., Lozano-cort, D. F., Myers, R. F., et al. (2016). On the origin of endemic species in the Red Sea. J. Biogeogr. 43, 13–30. doi: 10.1111/jbi.12631
Douabul, A., and Haddad, A. M. (1970). The Red Sea and Yemen’s Red Sea Environments. Yemen: Hassell and Assoc., AMSAT and UNOPS’, 1–16.
Echelman, T., and Fishelson, L. (1990). Surface zooplankton dynamics and community structure in the Gulf of Aqaba (Eilat), Red Sea. Mar. Biol. 107, 179–190. doi: 10.1007/bf01313255
Edwards, F. J. (1987). Climate and Oceanography. Oxford: Pergamon Books Ltd, doi: 10.1016/B978-0-08-028873-4.50008-6
Furuki, T., Fujita, H., Nakajo, M., and Harada, T. (2018). Temperature acclimation ability by an oceanic sea skater, Halobates germanus, inhabiting the tropical Pacific Ocean. Insects 9:90. doi: 10.3390/insects9030090
Furuki, T., Sekimoto, T., Umamoto, N., and Nakajo, M. (2016). Relationship of abundance of oceanic sea skaters, Halobates in the tropical Pacific Ocean to surface biomass and chlorophyll / oxygen concentrations. Nat. Sci. 8, 264–270. doi: 10.4236/ns.2016.86031
Harada, T., Furuki, T., Ohoka, W., Umamoto, N., Nakajo, M., and Katagiri, C. (2016). The first finding of six instars of larvae in Heteroptera and the negative correlation between precipitation and number of individuals collected in sea skaters of Halobates (Heteroptera: Gerridae). Insect 7:73. doi: 10.3390/insects7040073
Harada, T., Nakajo, M., Furuki, T., Umamoto, N., Moku, M., Sekimoto, T., et al. (2018). Seasonal change in distribution and heat coma temperature of oceanic skaters, Halobates (Insecta, heteroptera: Gerridae). Insects 9:133. doi: 10.3390/insects9040133
Harada, T., Osumi, Y., Shiraki, T., Kobayashi, A., Sekimoto, T., Nakajo, M., et al. (2014). Abundance of oceanic sea skaters, Halobates in the tropical Indian Ocean with respect to surface chlorophyll and oxygen concentrations. J. Exp. Mar. Biol. Ecol. 460, 32–36. doi: 10.1016/j.jembe.2014.05.025
Harada, T., Takenaka, S., Iyota, K., Shiraki, T., and Moku, M. (2013). Supercooling points and heat coma temperatures in four species of oceanic sea skaters of the genus Halobates (Heteroptera: Gerridae: Halobatinae). J. Asian Pac. Entomol. 16, 219–222. doi: 10.1016/j.aspen.2013.01.005
Harada, T., Takenaka, S., Sekimoto, T., Ohsumi, Y., and Nakajyo, M. (2011). Heat coma and its relationship to ocean dynamics in the oceanic sea skaters of Halobates (Heteroptera: Gerridae) inhabiting Indian and Pacific Oceans. J. Ther. Biol. 36, 299–305. doi: 10.1016/j.jtherbio.2011.05.001
Holdway, P., and Maddock, L. (1983). A comparative survey of neuston: geographical and temporal distribution patterns. Mar. Biol. 76, 263–270. doi: 10.1007/BF00393027
Ikawa, T., Okabe, H., and Cheng, L. (2012). Skaters of the seas-comparative ecology of nearshore and pelagic Halobates (Hemiptera: Gerridae), with special reference to Japanese species. Mar. Biol. Res. 8, 915–936. doi: 10.1080/17451000.2012.705848
Ikawa, T., Okabe, H., Hoshizaki, S., Kamikado, T., and Cheng, L. (2004). Distribution of the oceanic insects Halobates (Hemiptera: Gerridae) off the south coast of Japan. Entomol. Sci. 7, 351–357. doi: 10.1111/j.1479-8298.2004.00083.x
Ikawa, T., Onodera, S., Okabe, H., Hoshizaki, S., and Cheng, L. (2007). Occurrence and density of Halobates micans (Hemiptera: Gerridae) in the eastern South Indian Ocean. Entomol. Sci. 10, 213–215. doi: 10.1111/j.1479-8298.2007.00215.x
Ikawa, T., Watanabe, M., Aoki, M., and Okabe, H. (2008). Consecutive daily sampling of aggregations of the sea skater Halobates japonicus Esaki (Hemiptera: Gerridae). J. Morioka Univ. 25, 31–37.
Krueger, T., Horwitz, N., Bodin, J., Giovani, M.-E., Escrig, S., Meibom, A., et al. (2017). Common reef-building coral in the Northern Red Sea resistant to elevated temperature and acidification. R. Soc. Open Sci. 4:170038. doi: 10.1098/rsos.170038
Lee, R. F., and Cheng, L. (1974). A comparative study of the lipids of water striders from marine, estuarine, and freshwater environment: Halobates, Rheumatobates, Gerris (Heteroptera: Gerridae). Limnol. Oceanogr. 19, 958–965. doi: 10.4319/lo.1974.19.6.0958
Miyamoto, S., and Senta, T. (1960). Distribution, marine condition and other biological notes of marine waterstriders, Halobates spp., in the south-western sea area of Kyushu and western area of Japan Sea. Sieboldia 2, 171–186.
Nakajo, M., Sekimoto, T., Emi, K., Ide, R., Wada, K., Inoue, T., et al. (2013). Comparison of temperature preference for habitat among three species of oceanic sea skaters. Nat. Sci. 5, 9–15. doi: 10.4236/ns.2013.512a002
Osman, E. O., Smith, D. J., Ziegler, M., Kürten, B., Conrad, C., El-Haddad, K. M., et al. (2018). Thermal refugia against coral bleaching throughout the northern Red Sea. Glob. Chang. Biol. 24, e474–e484. doi: 10.1111/gcb.13895
Pearman, J. K., and Irigoien, X. (2015). Assessment of zooplankton community composition along a depth profile in the central red sea. PLoS One 10:e0133487. doi: 10.1371/journal.pone.0133487
Polhemus, D. A. (1990). Heteroptera of Aldabra atoll and nearby islands, Western Indian ocean, Part1. Marine heteroptera (insecta): gerridae, veliidae, hermatobatidae, saldidae and omaniidae, with notes on ecology and insular zoogeography. Atoll. Res. Bull. 345, 1–16. doi: 10.5479/si.00775630.345.1
Por, F. D., Dor, I., and Amir, A. (1977). The mangal of sinai: limits of an ecosystem. Helgoländer Wissenschaftliche Meeresuntersuchungen 30, 295–314. doi: 10.1007/BF02207843
Raitsos, D. E., Pradhan, Y., Brewin, R. J. W., Stenchikov, G., and Hoteit, I. (2013). Remote sensing the phytoplankton seasonal succession of the Red Sea. PLoS One 8:e64909. doi: 10.1371/journal.pone.0064909
Sagaydachnyy, A. Y. (1975). Distribution and some biological characteristics of Halobates (Hemiptera. Gerridae) in the Indian Ocean. Oceanolgy 15, 211–214.
Sawall, Y., Al-Sofyani, A., Hohn, S., Banguera-Hinestroza, E., Voolstra, C. R., and Wahl, M. (2015). Extensive phenotypic plasticity of a Red Sea coral over a strong latitudinal temperature gradient suggests limited acclimatization potential to warming. Sci. Rep. 5:8940. doi: 10.1038/srep08940
Schmidt, H.-E., and Muller, R. (1973). Marine water strider of the genus Halobates (Hemiptera: Gerridae) from the Red sea and the Gulf of Aden. Isr. J. Zool. 22, 1–12.
Schoepf, V., Stat, M., Falter, J. L., and McCulloch, M. T. (2015). Limits to the thermal tolerance of corals adapted to a highly fluctuating, naturally extreme temperature environment. Sci. Rep. 5, 1–14. doi: 10.1038/srep17639
Senta, T., Kimura, M., and Kanbara, T. (1993). Predation of fishes on open-ocean species of sea-skaters (Halobates spp.). Jpn. J. Ichthyol. 40, 193–198.
Spence, J. R., and Andersen, N. M. (1994). Biology of waterstriders: interactions between systematics and ecology. Annu. Rev. Entomol. 39, 101–128. doi: 10.1146/annurev.ento.39.1.101
Treherne, J. E., and Foster, W. A. (1980). The effect of group size on predator avoidance in a marine insect. Anim. Behav. 28, 1119–1122. doi: 10.1016/s0003-3472(80)80100-x
Treherne, J. E., and Foster, W. A. (1982). Group size and anti-predator strategies in a marine insect. Anim. Behav. 32, 536–542. doi: 10.1016/s0003-3472(82)80066-3
Keywords: Halobates, sea skaters, neuston, abundance, population structure
Citation: Mahadik GA, Agusti S and Duarte CM (2019) Distribution and Characteristics of Halobates germanus Population in the Red Sea. Front. Mar. Sci. 6:408. doi: 10.3389/fmars.2019.00408
Received: 04 March 2019; Accepted: 02 July 2019;
Published: 17 July 2019.
Edited by:
Angel Borja, Centro Tecnológico Experto en Innovación Marina y Alimentaria (AZTI), SpainReviewed by:
Romain Garrouste, Muséum National d’Histoire Naturelle, FranceErika M. D. Porporato, Università Ca’ Foscari Venezia, Italy
Copyright © 2019 Mahadik, Agusti and Duarte. This is an open-access article distributed under the terms of the Creative Commons Attribution License (CC BY). The use, distribution or reproduction in other forums is permitted, provided the original author(s) and the copyright owner(s) are credited and that the original publication in this journal is cited, in accordance with accepted academic practice. No use, distribution or reproduction is permitted which does not comply with these terms.
*Correspondence: Gauri A. Mahadik, Z2F1cmkubWFoYWRpa0BrYXVzdC5lZHUuc2E=