- 1ECIMAT, Universidade de Vigo, Vigo, Spain
- 2Departamento de Ecoloxía e Bioloxía Animal, Universidade de Vigo, Vigo, Spain
The marine flagellate Tisochrysis lutea (T-iso), with a global distribution, is one of the most common microalgae used as natural food in aquaculture. In recent years T-iso has also been increasingly used in toxicity testing, although it is not included in current international protocols such as ISO that relies on Phaeodactylum tricornutum and Skeletonema costatum as marine species, and Raphidocelis subcapitata as freshwater species. Sensitivity of Isochrysis galbana to selected model toxicants was compared with that of those recommended species using the 72 h growth-rate inhibition response. Internationally accepted standard methods with fixed test conditions (light intensity, photoperiod, temperature, nutrients concentrations, initial cell density, time of exposure and endpoint) were followed to allow this comparison. Toxicity of model chemicals representative of the main environmental toxicants—a trace metal (zinc), a polyaromatic hydrocarbon (fluoranthene), an herbicide (benzalkonium chloride), an insecticide (chlorpyrifos), a surfactant (4-nonylphenol), and a microbiocide (triclosan)—were evaluated to determine EC50 and EC10 values. In general, T-iso showed higher sensitivity for most groups of toxicants, meeting the acceptability criteria in terms of control growth set in standard protocols. For example, EC50 and EC10 of T-iso for chlorpyrifos were 246 μg L–1 and 132 μg L–1, whereas for P. tricornutum these values were ca. fivefold higher: 1117 and 746 μg L–1 respectively. Therefore, the use of T-iso in marine toxicity testing as a standard model representative of photosynthetic organisms is recommended.
Introduction
Unicellular planktonic algae are primary producers that support oceanic food webs. Microalgae are well suited for toxicity bioassays because they are easily cultured and sensitive to organic and inorganic pollutants (Klaine and Lewis, 1995). Algal tests are chronic and sublethal tests conducted with several generations of organisms and have the advantage of a short duration (48–96 h). The significance of the algal toxicity test has been recognized internationally and numerous test guidelines have been published (ASTM, 2004; ISO, 2006a; OECD, 2011) and incorporated in various regulatory procedures. The Organization for Economic Cooperation and Development (OECD) recommended a growth-inhibition test with microalgae in the base set of ecotoxicological tests, and it is legally demanded for all the substances produced or imported in Europe exceeding 10 t/year (REACH annexes VII-X). Green algae (Division Chlorophyta) and diatoms (Division Chromophyta, Class Bacillariophyceae) are the most commonly used microalgae in toxicity tests (Walsh, 1993). However standard methods did not take into account the sensitivity differences between algae species. In the marine environment, the International Standardization Organization (ISO) propose Phaeodactylum tricornutum and Skeletonema costatum, both worldwide spread marine diatoms. In recent years, Isochrysis galbana, culture strain isolated from Tahiti (hereafter T-iso), recently renamed Tisochrysis lutea (Bendif et al., 2013), has been one of the most used marine microalgae in toxicity tests (Moreno-Garrido et al., 2000; Hampel et al., 2001; Yap et al., 2004; Satoh et al., 2005; Campa-Córdova et al., 2006; Correa-Reyes et al., 2007; Garrido-Perez et al., 2008; Debelius et al., 2009; Pérez et al., 2010a; Liu et al., 2011; Fisher et al., 2014; Suratno et al., 2015; Trenfield et al., 2015). This species was selected on the basis of its sensitivity and the ease to culture (Shaw and Chadwick, 1998; Pérez et al., 2009) as well as its economic importance in aquaculture (Renaud et al., 1991). Due to its nutritive value, it is used in marine aquaculture to feed early life stages of mollusks and crustaceans, and in fish larvae culture (Sukenik and Wahnon, 1991). T-iso cells are round shaped, 3–7.5 μm in diameter, typically covered with several layers of organic scales, and have two apical flagella (Heimann and Huerlimann, 2015). They have a fast growth rate and wide physicochemical tolerance ranges (O’Shea et al., 2008).
Marine organisms are increasingly exposed to a suite of natural and synthetic anthropogenic pollutants, including trace metals, polycyclic aromatic hydrocarbons, pesticides, antifouling biocides, and other emerging pollutants like components of plastic and cosmetic products.
Metals, mostly accumulated in bottom sediment and later released into water bodies, have long been recognized as major marine pollutants (Ansari et al., 2004). Although zinc is not regarded as being especially toxic, it is sometimes present in seawater and sediment interstitial water in estuaries at quantities exceeding the toxicity thresholds to some species (Stauber and Florence, 1990).
Fluoranthene (FLU) is a low molecular weight polycyclic aromatic hydrocarbons (PAH). According to Neff and Stubblefield (1995), FLU, naphthalene, phenanthrene and pyrene are the most toxic components of oil for marine biota in the short term. It has also been reported to be toxic to photosynthetic aquatic organisms (Spehar et al., 1999; Pérez et al., 2010b).
The organophosphate (OP) broad spectrum insecticide chlorpyrifos (CPF) is extensively used in agriculture and residential pest control (Saulsbury et al., 2009). OPs are considered hazardous environmental pollutants since they are persistent, non-biodegradable and bioaccumulative (Readman et al., 1992; Bondarenko et al., 2009). Schimmel et al. (1983) found CPF to be the most hydrophobic pesticide of six pesticides studied in his work, reporting a Log Kow = 5.2, a solubility in seawater of 73 μg L–1 and a half-life of 24 days in seawater. Bioconcentration factor (BCF) in Mytilus galloprovincialis was 400 ± 119 L kg–1 (Serrano et al., 1997). Thus, contamination by pesticides is a serious water pollution issue, and CPF is listed as a priority substance according to Annex I of the Directive 2013/39/EU.
Benzalkonium chloride (BAC) is a quaternary ammonium compound broadly used in disinfection treatments in aquaculture species (Hoskins and Dalziel, 1984; Lio-Po and Sanvictores, 1986), as an algaecide (Lee et al., 1994) and as an antifouling component (His et al., 1996; Parr et al., 1998; Smith et al., 2002). Effects on phytoplankton species were studied in Pérez et al. (2009).
Nonylphenols (NP) are degradation products of detergents (NP ethoxylates), cleaning products and are also directly used as pesticides and as plasticizers for high-density polyethylene (HDPE), polyethylene terephthalate (PET) and polyvinyl chloride (PVC) (Loyo-Rosales et al., 2004). It poses remarkable environmental risk in marine environments (Tato et al., 2018), and the EU has included 4-NP on the list of priority hazardous substances for surface waters, with chronic and acute environmental quality standards of 0.3 and 2 mg L–1, respectively (Directive 2013/39/EU).
Triclosan (TCS) is a broad spectrum antimicrobial used in pharmaceutical and personal-care products (Singer et al., 2002) and as a preservative in textile fibers and plastics (Dann and Hontela, 2011). TCS has been detected in rivers, lakes and coastal marine waters of several European countries, United States, Canada, Australia, Japan and Hong Kong (EC, 2010; Dann and Hontela, 2011; Pintado-Herrera et al., 2014a,b). Previous work identified TCS as highly toxic for marine microalgae (Tato et al., 2018).
The aim of this study was to assess the sensitivity of T-iso in comparison with other microalgal species recommended in standard methods: P. tricornutum and R. subcapitata, using selected model toxicants representative of the main groups of aquatic pollutants. With that aim, the standard 72 h growth inhibition test was used, and effective concentrations that cause a decrease of 10 and 50% on the biological variable (EC10 and EC50) were estimated for each chemical for T-iso and P. tricornutum and for zinc, 4-n-nonylphenol and triclosan for R. subcapitata.
Materials and Methods
Test Chemicals
All standards were of the highest commercially available purity (>98%): ZnCl2 (CAS 7646-85-7) obtained from Merck (Germany), FLU (CAS 206-44-0), CPF (CAS 2921-88-2), BAC (CAS 63449-41-2), 4-NP (CAS 104-40-5) and TCS (CAS number 3380-34-5) obtained from Sigma-Aldrich (Madrid, Spain). FLU, CPF, 4-NP and TCS stock solutions were prepared in dimethyl sulfoxide (DMSO, CAS 67-68-5). ZnCl2 and BAC stocks were made in water of ultrapure quality, obtained by means of Milli-Q apparatus (Millipore, Bedford, MA, United States), ultrapure water hereinafter. Exposure solutions were made up by diluting the stock solutions in 0.22 μm-filtered sea water (FSW) of oceanic characteristics (34 ± 2 psu salinity, 8.2 ± 0.1 pH, 8.0 ± 0.1 mg L–1 dissolved oxygen) from an uncontaminated area in the outer part of Ría de Vigo (Galicia, NW Iberian Peninsula) for the marine species, or ultrapure water for R. subcapitata.
Test Organisms
Isochrysis affinis galbana recently renamed Tisochrysis lutea clone Tahiti (T-iso) (strain ECC038) and Phaeodactylum tricornutum Bohlin (strain ECC028), and the freshwater microalga Raphidocelis subcapitata (Korshikov) Nygaard, Komárek, Kristiansen and Skulbertg (formerly known as Selenastrum capricornutum or Pseudokirchneriella subcapitata) strain ECC029 were obtained from the ECIMAT (University of Vigo) microalgae collection.
Algal Growth Inhibition Test
Toxicity tests were performed under strict quality assurance/quality control tools following internationally standard methods. Growth-rate inhibition tests followed ISO (2006a) for T-iso and P. tricornutum and ISO (2012) for R. subcapitata. Experimental solutions and the inoculum for the algal culture (10,000 ± 2,000 cells mL–1) were added to 250 mL borosilicate conical flasks in triplicate and six additional flasks as control cultures. For tests with FLU, CPF, 4-NP and TCS controls were DMSO solutions in FSW (0.1% v/v), below NOEC for T-iso (0.4%) and R. subcapitata 1% (El Jay, 1996). Flasks were kept in an isothermal room at 20°C with a 24 h light period (cool daylight lamps Osram L36W/865, emission spectrum range 380–780 nm, and light intensity 80–100 μE m–2 s–1; Biospherical Instruments Inc., QSL2101). No agitation was provided during incubation and pH was recorded initially and after 72 ± 2 h. Cells were counted at the beginning and after the 72 ± 2 h of exposure with a Z2 Coulter Counter particle size analyzer (Beckman-Coulter, United States). Growth rate (GR) was calculated as:
The response (GR) was expressed as growth inhibition (I) using the following equation:
Where GRc is the average growth rate in control cultures and GRi is the growth rate in each flask.
Validity criteria described in ISO (2006a) and ISO (2012) were adopted (see Table 1). The zinc assay required a modification of the protocol (ISO, 2006b) that implies the use of growth medium lacking EDTA, and reducing incubation time to 48 ± 2 h. In addition, as an internal quality control, the marine algae bioassays were also performed on the reference chemical 3,5-dichlorophenol (ISO, 2006a).
Statistical Analyses
The EC50 and EC10 values and their 95% confidence intervals were calculated by fitting data to a Probit dose-response model using IBM SPSS statistics version 22.0.
Results
Dose-response curves and the effective concentrations derived for the different chemicals tested are shown in Figure 1 and Table 2. For all the chemicals evaluated, T-iso was more sensitive than P. tricornutum and also than R. subcapitata for 4-n-NP and TCS. For the three chemicals tested with the three algal species, the ranking of toxicity according to the EC50 values was the same: 4-n-NP > TCS > Zn. For the extra three chemicals tested with the marine species only, the ranking of toxicity was also the same: BAC > CPF > FLU. Therefore the choice of test species will not affect toxicity rankings provided if a single species is used. In contrast, sensitivity was consistently higher for T-iso compared to P. tricornutum, and the EC50 values for the former were on average fivefold lower than for the latter.
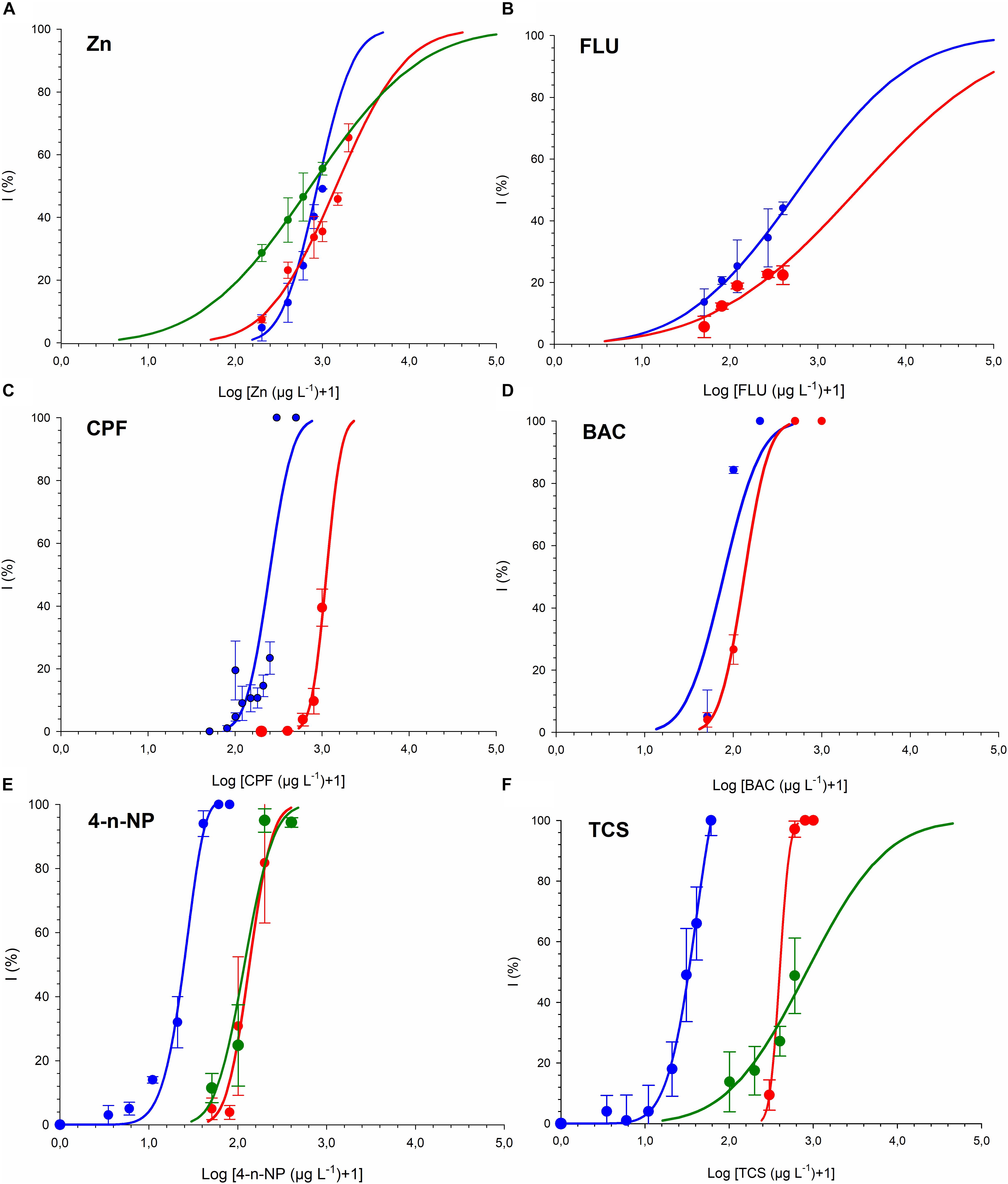
Figure 1. Growth inhibition of T-iso (blue), P. tricornutum (red) and R. subcapitata (green) exposed to (A) Zinc (B) Fluoranthene (C) Chlorpyrifos (D) Benzalkonium chloride (E) 4-n-Nonylphenol. (F) Triclosan. Blue curve from panel (E,F) published in Tato et al. (2018).
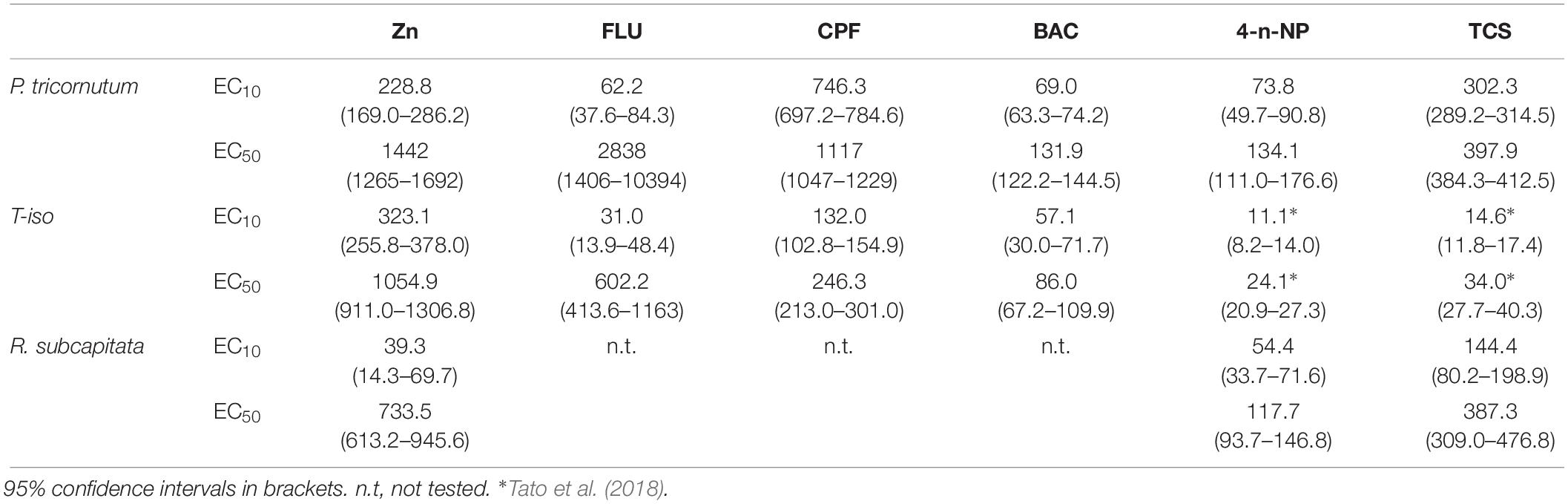
Table 2. Effective concentrations (μg L–1) obtained in the growth inhibition test for zinc (Zn), fluoranthene (FLU), chlorpyrifos (CPF), Benzalkonium chloride (BAC), 4-n-nonylphenol (4-n-NP) and triclosan (TCS).
Validity criteria were met in all the assays and values of reference chemical 3,5-dichlorophenol were EC50 = 2.31 (2.26–2.36) mg L–1 and EC50 = 1.63 (1.53–1.74) mg L–1 for P. tricornutum and T-iso, respectively.
Discussion
Methods standardization is crucial to ensure comparability of results, and in the case of microalgae tests, numerous recent publications use different methodologies (see Table 3). Incubation temperatures ranging from 24 to 30°C (Trenfield et al., 2015), culture media and cellular density changes to improve sensitivity (Moreno-Garrido et al., 2000), in vivo fluorescence endpoint (Pérez et al., 2010a) and microplate test (Satoh et al., 2005) are some examples of sources of interassay and interlaboratory variability. Interlaboratory calibrations and definition of strict acceptability criteria are needed in order to increase robustness of results and improve the reliability of the toxicity thresholds obtained, used in risk assessment and for derivation of environmental quality regulations.
Standard Test Conditions
Test guidelines incorporated in regulatory procedures (ASTM, 2004; ISO, 2006a, 2012; OECD, 2011) are strict protocols that seek robust and reproducible results. The recommended test format is glass Erlenmeyer flasks and polycarbonate for tests with metals. The comparability of toxicity responses between tests in microplates and standard flask has been the subject of discussion (Ismail et al., 2002; Eisentraeger et al., 2003; Blaise and Vasseur, 2005; Pavlic et al., 2006). Microplate tests have advantages such as reduction of the volume of sample needed and the possibility to perform high throughput analyses, reducing also costs in glassware and reagents. However, problems of adsorption of substances in microplate walls (Araújo et al., 2010), evaporation of volatile chemicals that can interfere with neighbor wells, and cell density increase due to evaporation are some of the significant interferences that can occur (Pavlic et al., 2006).
Microalgae require constant light conditions which supports exponential growth. This can be accomplished providing light above saturation intensity, applying mechanical mixing and lowering the culture volume and density. Continuous light is proposed in these standard methods with the advantages to allow constant growth conditions, the obtaining of desynchronized algal populations and the practice in routine testing (Nyholm and Källqvist, 1989). The algal growth rate exponentially increases with temperature until an optimum temperature is reached and rapidly declines thereafter. Nyholm and Källqvist (1989) highlighted that the main cause of temperature differences is created by the heat evolved from light tubes. Again, standard methods recommend using cool white fluorescent lighting that avoids this heating effect and has the main peak in the wavelengths corresponding to blue and green (20.8 and 24.7%), decreasing progressively to 8.5% for the red waveband (Sánchez-Saavedra and Voltolina, 2006), and the temperature of 20 ± 2°C is proposed. Algae growth limitation should be avoided to obtain comparable data (Klaine and Ward, 1983). Growth rates are related to intracellular nutrient concentrations and not to extracellular concentrations, and uptake of nutrients is not coupled with growth under nutrient-deficient conditions (Nyholm and Källqvist, 1989). Results obtained by Garrido-Perez et al. (2008) show that test medium composition is a determining factor in the EC50 value of alkylbenzene sulfonate (LAS) in I. galbana. Therefore a strict nutrient composition in the incubation medium is recommended. In seawater tests, the medium is usually synthetic or natural filtered seawater enriched with salts, nutrients (nitrates and phosphates, trace metals) and vitamins. ISO gives a formulation for P. tricornutum and S. costatum. The present study demonstrates that in the case of T-iso, f/2 nutritive medium (Guillard and Ryther, 1962) allows obtaining reproducible growth rate results. CO2 is the microalgae carbon source for photosynthesis and airtight vessels should be avoided in order to allow flow of CO2. ISO recommends to continuously shake, stir and aerate the culture flasks to maintain the cells in suspension and ease CO2 mass transfer form air to water, and reduce pH drift. However, test solutions in our case were not shaken, stirred or aerated and ISO validity criteria for marine species were met for both T-iso and P. tricornutum. In the case of the freshwater species R. subcapitata, in contrast, pH occasionally increased more than the prescribed 1.5 units in control flasks for the 72 h test. Unlike freshwater, the carbonate content of seawater provides an efficient pH buffer. Therefore, we recommend for the marine test static conditions in order to reduce the laboratory equipment required.
Algal toxicity tests are more sensitive when initial cell density is low (Moreno-Garrido et al., 2000; Trenfield et al., 2015). Standard protocols recommend a maximum of 10,000 cells mL–1, the lowest cell density suitable to be counted with the Neubauer chamber, but electronic particle counters, such as the Coulter counter, enable counting lower cell concentrations. Again, in order to establish a strict protocol and control sensitivity of the test due to cell density, initial cell density should be fixed, and we propose 10,000 ± 2,000 cells mL–1 as acceptable values.
Algae tests are restricted to the initial period of exponential growth, between 2 and 4 days of duration. This short duration avoids the nutrient limitation effect in control flasks, loss of toxicity by adsorption of chemicals on algal cells and detoxification caused by excretion of organics and volatilization (Nyholm and Källqvist, 1989). ISO and OECD established 72 ± 2 h for all algae tests with the exception of metal testing, where 48 ± 2 h is recommended in order to restrict the final algal biomass density.
A common endpoint in microalgae bioassays is growth rate, assessed in terms of cell density, recommended in several protocols and guidelines dealing with the standardization of these assays (ASTM, 2004; ISO, 2006a, 2012; OECD, 2011). Many authors discuss this issue and several other endpoints are suggested (see Table 3). Growth rate may also be assessed through chlorophyll content, and has also been applied to microscale assay using microplates (Ismail et al., 2002; Satoh et al., 2005). Pérez et al. (2010a) found fluorescence more suitable for assays with fuel but Othman et al. (2012) found greater sensibility in the population density endpoint at 72 h (absorbance at 650 nm) than the photosynthetic endpoint at 24 h (fluorescence of chlorophyll at 450 and 680 nm) for benz(a)anthracene and fluoranthene. Furthermore, fluorescence endpoint also requires previous measurement of chlorophyll a (Chl a) concentration and establishment of a relation between Chl a and fluorescence intensity. Hampel et al. (2001) compared esterase activity endpoint with growth inhibition resulting in 2 and 5 times less sensitivity than growth inhibition rate. The need for a standardization of the toxicological endpoints was evident by the large disparity between the EC values found in literature for the same chemicals. Algal biomass growth rate, recorded through counts of cell densities, is the toxicity test endpoint proposed in ISO and OECD, and we recommend using an electronic particle counter for rapid and objective measurements of this endpoint.
Test Organism
The excessive variety in results from algal toxicity tests can be decreased by suitable standardization/harmonization of test methods, and this includes the selection of standard test species. Several test species and strains were studied in the past (Walsh et al., 1988), and published guidelines (ASTM, 2004; ISO, 2006a, 2012; OECD, 2011) recommend the diatoms P. tricornutum and S. costatum for marine environment and the green algae R. subcapitata (formerly S. capricornutum or P. subcapitata) for freshwater ecosystems. OECD also recommends for freshwater Desmodesmus subspicatus (green algae), Navicula pelliculosa (diatoms), Anabaena flos-aquae and Synechococcus leopoliensis (cyanobacteria).
There are diverse factors that influence algae species’ susceptibility to pollutants, including cell size, cell wall type and thickness and taxonomic group characteristics. Small cells have large surface area to volume ratios which increase their potential uptake rates for solutes and in turn increase their sensitivity to pollutants, reported for copper (Quigg et al., 2006) and PAHs (Echeveste et al., 2010; Othman et al., 2012). In the case of metals, there is not a clear tendency. Levy et al. (2007) found the wall-less D. tertiolecta more tolerant to copper than M. pusilla and Tetraselmis sp., both with cell wall. On the other hand, Macfie et al. (1994) compared a naked clone and clone with cell wall of the freshwater green alga C. reinhardtii and reported more sensitivity to cadmium, copper and nickel in the naked strain. Regarding detergents, Corre et al. (1996) found higher sensitivity in Chlorella species with different outer layer composition and Lewis (1990) contemplated the thickness of the cell wall as a key factor to explain the damage caused by a surfactant to microalgae. Later Pérez et al. (2009) reported differences in toxicity response to BAC regarding the penetration of the surfactant into the cell in two species with different outer layer characteristics, T-iso and C. gracilis. According to the EC50 values, T-iso shows more sensibility to all tested chemicals than P. tricornutum (with outer frustule). The absence of a cell wall in T-iso (membrane covered with body scale), compared to the outer frustule of the diatom, may account for these differences. In fact, T-iso was the most sensitive species for all tested chemicals except for the Zn where, as expected, the freshwater species was the most sensitive. In seawater, toxicity of divalent metal ions is reduced because these ions compete for cell binding sites with the non-toxic Ca2+ and Mg2+ (Penttinen et al., 1998; Heijerick et al., 2002, 2009).
Marine Species
Several studies compare the sensitivity of algae related to the taxonomic group. Hampel et al. (2001) evaluated the toxicity of alkylbenzene sulfonate (LAS) on N. gaditana, T. suecica, R. salina, and I. galbana, reporting the highest sensibility for R. salina. Satoh et al. (2005) studied the toxicity of five heavy metals (Cu, As, Sb, Pb, and Cd) using nine marine microalgal species of five divisions and seven genera) and a fluorometric growth-inhibition assay with microplates resulting in haptophyta I. galbana, the most sensitive species for all metals except for Sb. Using Cu as a reference toxicant and 11 algal species, Levy et al. (2007) did not find any large taxonomic class of algae was more sensitive than another. Values of 72 h EC50 < 5 μg L–1 were reported in five different classes of algae (a diatom, a prasinophytes, a cryptomonad, a prymnesiophyte and a dinoflagellate). Again using Cu and 16 marine diatoms, Stauber and Florence (1990) only highlighted the high tolerance of D. tertiolecta. Algae from the genera Dunaliella and Tetraselmis have been classified as very tolerant species. For example the euryhaline D. salina and T. chuii are also tolerant to metals and have a competitive advantage against other algal species in metal-stressed conditions (Moreno-Garrido et al., 2005; Millán de Kuhn et al., 2006).
Freshwater Species
Blanck et al. (1984) showed with 13 freshwater species and 19 compounds that a universally more sensitive species of alga could not be identified, but suggested that some species are more regular and others more selective in their response to pollutants, recommending Scenedesmus obtusiusculus, Chlorella emersonii and Raphidocelis subcapitata. Later, Larsen et al. (1986) studied the toxicity of Atrazine to eight different algal species and identified R. subcapitata, Scenedesmus obliquus and Chlamydomonas reinhardtii as the most sensitive species. This agrees with Fairchild et al. (1998) who studied the toxicity of four pesticides to six species of algae and found that green algae (R. subcapitata, Chlorella vulgaris, Chlamydomonas reinhardtii and Scenedesmus quadricauda) were more sensitive than the blue-green algae (Microcystis sp. and Anabaena flosque).
Conclusion
The marine microalgae Tisochrysis lutea (T-iso), formerly Isochrysis galbana, has the ideal characteristics to be used as a model in toxicity testing. It is easily available because of its worldwide use in aquaculture, is suitable for culture in low volumes displaying high growth rates in standard culture media, and shows equal or higher sensitivity to a broad range of toxic chemicals compared to alternative marine species.
Author Contributions
RB designed the experiment. TT conducted the toxicity tests and data analysis. Both authors wrote the manuscript.
Funding
This work was supported by the Spanish Government through the ARPA-ACUA (CTM2016-77945-C3) and EPHEMARE (PCIN-2015-187-C03-03) projects, the latter under the framework of JPI Oceans.
Conflict of Interest Statement
The authors declare that the research was conducted in the absence of any commercial or financial relationships that could be construed as a potential conflict of interest.
Acknowledgments
The authors acknowledge Damián Costas and all the staff of ECIMAT for their helpful technical support.
References
Ansari, T. M., Marr, I. L., and Tariq, N. (2004). Heavy metals in marine pollution perspective–a mini review. J. Appl. Sci. 4, 1–20. doi: 10.3923/jas.2004.1.20
Araújo, C. V. M., Diz, F. R., Lubián, L. M., Blasco, J., and Moreno-Garrido, I. (2010). Sensitivity of Cylindrotheca closterium to copper: influence of three test endpoints and two test methods. Sci. Total Environ. 408, 3696–3703. doi: 10.1016/j.scitotenv.2010.05.012
Arensberg, P., Hemmingsen, V. H., and Nyholm, N. (1995). A miniscale algal toxicity test. Chemosphere 30, 2103–2115. doi: 10.1016/0045-6535(95)00090-U
ASTM (2004). E1218-04e1 Standard Guide for Conducting Static Toxicity Tests with Microalgae. West Conshohocken, PA: American Society for Testing and Materials.
Bendif, E. M., Probert, I., Schroeder, D. C., and de Vargas, C. (2013). On the description of Tisochrysis lutea gen. nov. sp. nov. and Isochrysis nuda sp. nov. in the Isochrysidales, and the transfer of Dicrateria to the Prymnesiales (Haptophyta). J. Appl. Phycol. 25, 1763–1776.
Blaise, C., and Vasseur, P. (2005). “Algal Microplate Toxicity Test,” in Small-Scale Freshwater Toxicity Investigations, eds C. Blaise and J. Férard (Dordrecht: Springer).
Blanck, H., Wallin, G., and Wängberg, S. -Å. (1984). Species-dependent variation in algal sensitivity to chemical compounds. Ecotoxicol. Environ. Saf. 8, 339–351. doi: 10.1016/0147-6513(84)90003-90004
Bondarenko, S., Gan, J., Haver, D. L., and Kabashima, J. N. (2009). Persistence of selected organophosphate and carbamate insecticides in waters from a coastal watershed. Environ. Toxicol. Chem. 23, 2649–2654. doi: 10.1897/03-436
Campa-Córdova, A. I., Luna-González, A., Ascencio, F., Cortés-Jacinto, E., and Cáceres-Martínez, C. J. (2006). Effects of chloramphenicol, erythromycin, and furazolidone on growth of Isochrysis galbana and Chaetoceros gracilis. Aquaculture 260, 145–150. doi: 10.1016/j.aquaculture.2006.06.014
Corre, G., Templier, J., Largeau, C., Rousseau, B., and Berkaloff, C. (1996). Incluence of cell wall composition on the resistance of two Chlorella species (Chlorophyta) to detergents. J. Phycol. 32, 584–590. doi: 10.1111/j.0022-3646.1996.00584.x
Correa-Reyes, G., Viana, M. T., Marquez-Rocha, F. J., Licea, A. F., Ponce, E., and Vazquez-Duhalt, R. (2007). Nonylphenol algal bioaccumulation and its effect through the trophic chain. Chemosphere 68, 662–670. doi: 10.1016/j.chemosphere.2007.02.030
Dann, A. B., and Hontela, A. (2011). Triclosan: environmental exposure, toxicity and mechanisms of action. J. Appl. Toxicol. 31, 285–311. doi: 10.1002/jat.1660
Debelius, B., Forja, J. M., DelValls, Á., and Lubián, L. M. (2009). Toxicity and bioaccumulation of copper and lead in five marine microalgae. Ecotoxicol. Environ. Saf. 72, 1503–1513. doi: 10.1016/j.ecoenv.2009.04.006
EC (2010). Preliminary Opinion on Triclosan-Antimicrobial Resistance. Brussels: Scientific Committee on Consumer Safety, European Commission.
Echeveste, P., Agustí, S., and Dachs, J. (2010). Cell size dependent toxicity thresholds of polycyclic aromatic hydrocarbons to natural and cultured phytoplankton populations. Environ. Pollut. 158, 299–307. doi: 10.1016/j.envpol.2009.07.006
Eisentraeger, A., Dott, W., Klein, J., and Hahn, S. (2003). Comparative studies on algal toxicity testing using fluorometric microplate and Erlenmeyer flask growth-inhibition assays. Ecotoxicol. Environ. Saf. 54, 346–354. doi: 10.1016/S0147-6513(02)00099-94
El Jay, A. (1996). Toxic effects of organic solvents on the growth of Chlorella vulgaris and Selenastrum capricornutum. Bull. Environ. Contam. Toxicol. 57, 191–198. doi: 10.1007/s001289900174
Fairchild, J. F., Ruessler, D. S., and Carlson, A. R. (1998). Comparative sensitivity of five species of macrophytes and six species of algae to atrazine, metribuzin, alachlor, and metolachlor. Environ. Toxicol. Chem. 17, 1830–1834. doi: 10.1002/etc.5620170924
Fisher, D., Yonkos, L., Ziegler, G., Friedel, E., and Burton, D. (2014). Acute and chronic toxicity of selected disinfection byproducts to Daphnia magna, Cyprinodon variegatus, and Isochrysis galbana. Water Res. 55, 233–244. doi: 10.1016/j.watres.2014.01.056
Galgani, F., Cadiou, Y., and Gilbert, F. (1992). Simultaneous and iterative weighted regression analysis of toxicity tests using a microplate reader. Ecotoxicol. Environ. Saf. 23, 237–243. doi: 10.1016/0147-6513(92)90061-90067
Garrido-Perez, M. C., Perales-VargasMachuca, J. A., Nebot-Sanz, E., and Sales-Márquez, D. (2008). Effect of the test media and toxicity of LAS on the growth of Isochrysis galbana. Ecotoxicology 17, 738–746. doi: 10.1007/s10646-008-0223-z
Gilbert, F., Galgani, F., and Cadiou, Y. (1992). Rapid assessment of metabolic activity in marine microalgae: application in ecotoxicological tests and evaluation of water quality. Mar. Biol. 112, 199–205. doi: 10.1007/BF00702462
Guillard, R. R., and Ryther, J. H. (1962). Studies of marine planktonic diatoms. I. Cyclotella nana Hustedt, and Detonula confervacea (cleve) Gran. Can. J. Microbiol. 8, 229–239. doi: 10.1139/m62-029
Hampel, M., Moreno-Garrido, I., Sobrino, C., Lubián, L. M., and Blasco, J. (2001). Acute toxicity of LAS homologues in marine microalgae: esterase activity and inhibition growth as endpoints of toxicity. Ecotoxicol. Environ. Saf. 48, 287–292. doi: 10.1006/eesa.2000.2028
Heijerick, D. G., De Schamphelaere, K. A. C., and Janssen, C. R. (2002). Biotic ligand model development predicting Zn toxicity to the alga Pseudokirchneriella subcapitata: possibilities and limitations. Comp. Biochem. Physiol. C Toxicol. Pharmacol. 133, 207–218. doi: 10.1016/S1532-0456(02)00077-77
Heijerick, D. G., De Schamphelaere, K. A. C., and Janssen, C. R. (2009). Predicting acute zinc toxicity for Daphnia magna as a function of key water chemistry characteristics: development and validation of a biotic ligand model. Environ. Toxicol. Chem. 21, 1309–1315. doi: 10.1002/etc.5620210628
Heimann, K., and Huerlimann, R. (2015). “Chapter 3 - microalgal classification: major classes and genera of commercial microalgal species,” in Handbook of Marine Microalgae, ed. S.-K. Kim (Boston, MA: Academic Press), 25–41. doi: 10.1016/b978-0-12-800776-1.00003-0
His, E., Beiras, R., Quiniou, F., Parr, A. C. S., Smith, M. J., Cowling, M. J., et al. (1996). The non-toxic effects of a novel antifouling material on oyster culture. Water Res. 30, 2822–2825. doi: 10.1016/S0043-1354(96)00167-164
Hoskins, G. E., and Dalziel, F. C. (1984). Survival of chinook fry (Oncorhynchus tschawytscha) following exposure to benzalkonium chloride in soft water. Progr. Fish. Cult. 46, 98–101. doi: 10.1577/1548-8640(1984)46<98:socfot>2.0.co;2
Ismail, M., Phang, S.-M., Tong, S.-L., and Brown, M. T. (2002). A modified toxicity testing method using tropical marine microalgae. Environ. Monit. Assess. 75, 145–154. doi: 10.1023/A:1014483713719
ISO (2006a). ISO 10253:2006 Water quality - Marine Algal Growth Inhibition Test with Skeletonema costatum and Phaeodactylum tricornutum. Geneva: ISO.
ISO (2006b). ISO 14442:2006 Water Quality - Guidelines for Algal Growth Inhibition Tests with Poorly Soluble Materials, Volatile Compounds, Metals and Waste Water. Geneva: ISO.
ISO (2012). ISO 8692:2012 Water quality - Fresh Water Algal Growth Inhibition Test with Unicellular Green Algae. Geneve: ISO.
Klaine, S. J., and Lewis, M. A. (1995). “Algal and plant toxicity testing,” in Handbook of Ecotoxicology, eds D. J. Hoffman and B. A. Rattner (Boca Raton, FL: CRC Press), 163–184.
Klaine, S. J., and Ward, C. H. (1983). Growth optimized algal bioassays for toxicity evaluation. Environ. Toxicol. Chem. 2, 245–249. doi: 10.1002/etc.5620020212
Kusui, T., and Blaise, C. (1995). “Acute exposure phytotoxicity assay based on motility inhibition of Chlamydomonas variabilis,” in Environmental Toxicology Assessment, ed. M. Richardson (London: Taylor & Francis), 125–135.
Larsen, D. P., Stay, F., Shiroyama, T., and Frank, D. (1986). Comparisons of single-species, microcosm and experimental pond responses to atrazine exposure. Environ. Toxicol. Chem. 5, 179–190. doi: 10.1002/etc.5620050209
Lee, C.-L., Chen, I. M., and Fong, S.-C. (1994). Effects of benzalkonium chloride on growth of phytoplankton. J. Fish. Soc. Taiwan 21, 69–77. doi: 10.29822/JFST
Levy, J. L., Stauber, J. L., and Jolley, D. F. (2007). Sensitivity of marine microalgae to copper: the effect of biotic factors on copper adsorption and toxicity. Sci. Total Environ. 387, 141–154. doi: 10.1016/j.scitotenv.2007.07.016
Lewis, M. A. (1990). Chronic toxicities of surfactants and detergent builders to algae: a review and risk assessment. Ecotoxicol. Environ. Saf. 20, 123–140. doi: 10.1016/0147-6513(90)90052-90057
Lio-Po, G. D., and Sanvictores, E. G. (1986). Tolerance of Penaeus monodon eggs and larvae to fungicides against Lagenidium sp. and Haliphthoros philippinensis. Aquaculture 51, 161–168. doi: 10.1016/0044-8486(86)90305-90304
Liu, G., Chai, X., Shao, Y., Hu, L., Xie, Q., and Wu, H. (2011). Toxicity of copper, lead, and cadmium on the motility of two marine microalgae Isochrysis galbana and Tetraselmis chui. J. Environ. Sci. 23, 330–335. doi: 10.1016/S1001-0742(10)60410-X
Loyo-Rosales, J. E., Rosales-Rivera, G. C., Lynch, A. M., Rice, C. P., and Torrents, A. (2004). Migration of nonylphenol from plastic containers to water and a milk surrogate. J. Agric. Food Chem. 52, 2016–2020. doi: 10.1021/jf0345696
Macfie, S. M., Tarmohamed, Y., and Welbourn, P. M. (1994). Effects of cadmium, cobalt, copper, and nickel on growth of the green alga Chlamydomonas reinhardtii: the influences of the cell wall and pH. Arch. Environ. Con. Tox. 27, 454–458. doi: 10.1007/BF00214835
Mhadhbi, L., Fumega, J., and Beiras, R. (2012a). Toxicological effects of three polybromodiphenyl ethers (BDE-47, BDE-99 and BDE-154) on growth of marine algae Isochrysis galbana. Water Air Soil Pollut. 223, 4007–4016. doi: 10.1007/s11270-012-1167-1168
Mhadhbi, L., Rial, D., Pérez, S., and Beiras, R. (2012b). Ecological risk assessment of perfluorooctanoic acid (PFOA) and perfluorooctanesulfonic acid (PFOS) in marine environment using Isochrysis galbana, Paracentrotus lividus, Siriella armata and Psetta maxima. J. Environ. Monit. 14, 1375–1382. doi: 10.1039/C2EM30037K
Millán de Kuhn, R., Streb, C., Breiter, R., Richter, P., Neeße, T., and Häder, D.-P. (2006). Screening for unicellular algae as possible bioassay organisms for monitoring marine water samples. Water Res. 40, 2695–2703. doi: 10.1016/j.watres.2006.04.045
Moreno Garrido, I., Lubián, L. M., and Soares, A. M. V. M. (1999). Oxygen production rate as a test for determining toxicity of copper to Rhodomonas salina hill and wehterbee (Cryptophyceae). Bull. Environ. Contam. Toxicol. 62, 776–782. doi: 10.1007/s001289900939
Moreno-Garrido, I., Campana, O., Lubián, L. M., and Blasco, J. (2005). Calcium alginate immobilized marine microalgae: experiments on growth and short-term heavy metal accumulation. Mar. Pollut. Bull. 51, 823–829. doi: 10.1016/j.marpolbul.2005.06.008
Moreno-Garrido, I., Lubián, L. M., and Soares, A. M. V. M. (2000). Influence of cellular density on determination of EC50 in microalgal growth inhibition tests. Ecotoxicol. Environ. Saf. 47, 112–116. doi: 10.1006/eesa.2000.1953
Neff, J. M., and Stubblefield, W. A. (1995). “Chemical and toxicological evaluation of water quality following the Exxon Valdez oil spill,” in Exxon Valdez Oil Spill: Fate and Effects in Alaskan Waters, eds P. G. Wells, J. N. Butler, and J. S. Hughes (Philadelphia, PA: ASTM), 141–177.
Nyholm, N., and Källqvist, T. (1989). Methods for growth inhibition toxicity tests with freshwater algae. Environ. Toxicol. Chem. 8, 689–703. doi: 10.1002/etc.5620080807
OECD (2011). Test No. 201: Freshwater Alga and Cyanobacteria, Growth Inhibition Test, OECD Guidelines for the Testing of Chemicals, Section 2. París: OECD Publishing.
O’Shea, S. K., Holland, F., and Bilodeau, A. (2008). Modeling the effects of salinity and pH on the cadmium bioabsorptive properties of the microalgae Isochrysis galbana (T-Iso) in coastal waters. J. Coast. Res. 26, 59–66. doi: 10.2112/08-1073.1
Othman, H. B., Leboulanger, C., Le Floc’h, E., Hadj Mabrouk, H., and Sakka Hlaili, A. (2012). Toxicity of benz(a)anthracene and fluoranthene to marine phytoplankton in culture: does cell size really matter? J. Hazardous Mater. 243, 204–211. doi: 10.1016/j.jhazmat.2012.10.020
Parr, A. C. S., Smith, M. J., Beveridge, C. M., Kerr, A., Cowling, M. J., and Hodgkiess, T. (1998). Optical assessment of a fouling-resistant surface (PHEMA/benzalkonium chloride) after exposure to a marine environment. Adv. Mater. Opt. Electr. 8, 187–193. doi: 10.1002/(sici)1099-0712(1998070)8:4<187::aid-amo353>3.0.co;2-7
Pavlic, Z., Stjepanovic, B., Horvatic, J., Persic, V., Puntaric, D., and Culig, J. (2006). Comparative sensitivity of green algae to herbicides using erlenmeyer flask and microplate growth-inhibition assays. Bull. Environ. Contam. Toxicol. 76, 883–890. doi: 10.1007/s00128-006-1001-1003
Penttinen, S., Kostamo, A., and Kukkonen, J. V. K. (1998). Combined effects of dissolved organic material and water hardness on toxicity of cadmium to Daphnia magna. Environ. Toxicol. Chem. 17, 2498–2503. doi: 10.1002/etc.5620171217
Pérez, P., Estévez-Blanco, P., Beiras, R., and Fernández, E. (2006). Effect of copper on the photochemical efficiency, growth, and chlorophyll-a biomass of natural phytoplankton assemblages. Environ. Toxicol. Chem. 25, 137–143. doi: 10.1897/04-392R1.1
Pérez, P., Fernández, E., and Beiras, R. (2009). Toxicity of benzalkonium chloride on monoalgal cultures and natural assemblages of marine phytoplankton. Water Air Soil Pollut. 201, 319–330. doi: 10.1007/s11270-008-9947-x
Pérez, P., Fernández, E., and Beiras, R. (2010a). Fuel toxicity on Isochrysis galbana and a coastal phytoplankton assemblage: growth rate vs. variable fluorescence. Ecotoxicol. Environ. Saf. 73, 254–261. doi: 10.1016/j.ecoenv.2009.11.010
Pérez, P., Fernández, E., and Beiras, R. (2010b). Use of fast repetition rate fluorometry on detection and assessment of PAH toxicity on microalgae. Water Air Soil Pollut. 209, 345–356. doi: 10.1007/s11270-009-0203-209
Pintado-Herrera, M. G., González-Mazo, E., and Lara-Martín, P. A. (2014a). Atmospheric pressure gas chromatography–time-of-flight-mass spectrometry (APGC–ToF-MS) for the determination of regulated and emerging contaminants in aqueous samples after stir bar sorptive extraction (SBSE). Anal. Chim. Acta 851, 1–13. doi: 10.1016/j.aca.2014.05.030
Pintado-Herrera, M. G., González-Mazo, E., and Lara-Martín, P. A. (2014b). Determining the distribution of triclosan and methyl triclosan in estuarine settings. Chemosphere 95, 478–485. doi: 10.1016/j.chemosphere.2013.09.101
Quigg, A., Reinfelder, J. R., and Fisher, N. S. (2006). Copper uptake kinetics in diverse marine phytoplankton. Limnol. Oceanogr. 51, 893–899. doi: 10.4319/lo.2006.51.2.0893
Readman, J. W., Liong Wee Kwong, L., Mee, L. D., Bartocci, J., Nilve, G., Rodriguez-Solano, J. A., et al. (1992). Persistent organophosphorus pesticides in tropical marine environments. Mar. Pollut. Bull. 24, 398–402. doi: 10.1016/0025-326X(92)90500-90506
Renaud, S. M., Parry, D. L., Thinh, L.-V., Kuo, C., Padovan, A., and Sammy, N. (1991). Effect of light intensity on the proximate biochemical and fatty acid composition of Isochrysis sp. and Nannochloropsis oculata for use in tropical aquaculture. J. Appl. Phycol. 3, 43–53. doi: 10.1007/BF00003918
Sánchez-Saavedra, M. D. P., and Voltolina, D. (2006). The growth rate, biomass production and composition of Chaetoceros sp. Grown with different light sources. Aquacult. Eng. 35, 161–165. doi: 10.1016/j.aquaeng.2005.12.001
Satoh, A., Vudikaria, L. Q., Kurano, N., and Miyachi, S. (2005). Evaluation of the sensitivity of marine microalgal strains to the heavy metals, Cu, As, Sb, Pb and Cd. Environ. Int. 31, 713–722. doi: 10.1016/j.envint.2005.01.001
Saulsbury, M. D., Heyliger, S. O., Wang, K., and Johnson, D. J. (2009). Chlorpyrifos induces oxidative stress in oligodendrocyte progenitor cells. Toxicology 259, 1–9. doi: 10.1016/j.tox.2008.12.026
Schimmel, S. C., Garnas, R. L., Patrick, J. M., and Moore, J. C. (1983). Acute toxicity, bioconcentration, and persistence of AC 222,705, benthiocarb, chlorpyrifos, fenvalerate, methyl parathion, and permethrin in the estuarine environment. J. Agric. Food Chem. 31, 104–113. doi: 10.1021/jf00115a027
Schreiber, U., Quayle, P., Schmidt, S., Escher, B. I., and Mueller, J. F. (2007). Methodology and evaluation of a highly sensitive algae toxicity test based on multiwell chlorophyll fluorescence imaging. Biosens. Bioelectron. 22, 2554–2563. doi: 10.1016/j.bios.2006.10.018
Serrano, R., López, F. J., Hernández, F., and Peña, J. B. (1997). Bioconcentration of chlorpyrifos, chlorfenvinphos, and methidathion in Mytilus galloprovincialis. Bull. Environ. Contam. Toxicol. 59, 968–975. doi: 10.1007/s001289900577
Singer, H., Müller, S., Tixier, C., and Pillonel, L. (2002). Triclosan: occurrence and fate of a widely used biocide in the aquatic environment: field measurements in wastewater treatment plants, surface waters, and lake sediments. Environ. Sci. Technol. 36, 4998–5004. doi: 10.1021/es025750i
Smith, M. J., Adam, G., Duncan, H. J., and Cowling, M. J. (2002). The effects of cationic surfactants on marine biofilm growth on hydrogels. Estuarine Coast. Shelf Sci. 55, 361–367. doi: 10.1006/ecss.2001.0910
Spehar, R. L., Poucher, S., Brooke, L. T., Hansen, D. J., Champlin, D., and Cox, D. A. (1999). Comparative toxicity of fluoranthene to freshwater and saltwater species under fluorescent and ultraviolet light. Arch. Environ. Contam. Toxicol. 37, 496–502. doi: 10.1007/s002449900544
Stauber, J. L., and Florence, T. M. (1990). Mechanism of toxicity of zinc to the marine diatom Nitzschia closterium. Mar. Biol. 105, 519–524. doi: 10.1007/BF01316323
Sukenik, A., and Wahnon, R. (1991). Biochemical quality of marine unicellular algae with special emphasis on lipid composition. I. Isochrysis galbana. Aquaculture 97, 61–72. doi: 10.1016/0044-8486(91)90279-G
Suratno, S., Puspitasari, R., Purbonegoro, T., and Mansur, D. (2015). Copper and cadmium toxicity to marine phytoplankton, Chaetoceros gracilis and Isochrysis sp. Indones. J. Chem. 15, 172–178. doi: 10.22146/ijc.21211
Tato, T., Salgueiro-González, N., León, V. M., González, S., and Beiras, R. (2018). Ecotoxicological evaluation of the risk posed by bisphenol A, triclosan, and 4-nonylphenol in coastal waters using early life stages of marine organisms (Isochrysis galbana, Mytilus galloprovincialis, Paracentrotus lividus, and Acartia clausi). Environ. Pollut. 232, (Suppl. C), 173–182. doi: 10.1016/j.envpol.2017.09.031
Trenfield, M. A., van Dam, J. W., Harford, A. J., Parry, D., Streten, C., Gibb, K., et al. (2015). Aluminium, gallium, and molybdenum toxicity to the tropical marine microalga Isochrysis galbana. Environ. Toxicol. Chem. 34, 1833–1840. doi: 10.1002/etc.2996
Walsh, G. E. (1993). “Marine producers,” in Handbook of Ecotoxicology, ed. Calow (Oxford: Blackwell Scientic).
Walsh, G. E., McLaughlin, L. L., Yoder, M. J., Moody, P. H., Lores, E. M., Forester, J., et al. (1988). Minutocellus polymorphus: a new marine diatom for use in algal toxicity tests. Environ. Toxicol. Chem. 7, 925–929. doi: 10.1002/etc.5620071109
Keywords: growth rate, zinc, benzalkonium chloride, fluoranthene, chlorpyrifos, nonylphenol, triclosan, toxicity testing
Citation: Tato T and Beiras R (2019) The Use of the Marine Microalga Tisochrysis lutea (T-iso) in Standard Toxicity Tests; Comparative Sensitivity With Other Test Species. Front. Mar. Sci. 6:488. doi: 10.3389/fmars.2019.00488
Received: 31 October 2018; Accepted: 18 July 2019;
Published: 08 August 2019.
Edited by:
Julian Blasco, Spanish National Research Council (CSIC), SpainReviewed by:
Adam Lillicrap, Norwegian Institute for Water Research (NIVA), NorwayIgnacio Moreno-Garrido, Institute of Marine Sciences of Andalusia (ICMAN), Spain
Copyright © 2019 Tato and Beiras. This is an open-access article distributed under the terms of the Creative Commons Attribution License (CC BY). The use, distribution or reproduction in other forums is permitted, provided the original author(s) and the copyright owner(s) are credited and that the original publication in this journal is cited, in accordance with accepted academic practice. No use, distribution or reproduction is permitted which does not comply with these terms.
*Correspondence: Tania Tato, dGFuaWF0YXRvQHV2aWdvLmVz