- 1Animal Sciences Unit, Flanders Research Institute for Agriculture, Fisheries and Food, Ostend, Belgium
- 2Operational Directorate Natural Environment, Royal Belgian Institute of Natural Sciences, Brussels, Belgium
- 3Laboratory of Agrozoology, Faculty of Bioscience Engineering, Ghent University, Ghent, Belgium
- 4Department of Biosciences, University of Oslo, Oslo, Norway
- 5Unit of Biogeochemistry and Ecotoxicology, Institut Français de Recherche pour l’Exploitation de la Mer, Nantes, France
The restrictions and the concerted action of the global ban on the use and presence of tributyltin (TBT) in marine applications to protect ecosystems in the marine environment in 2008 was mainly based on the economic impact on shellfish industries and the dramatic extinction of local mollusk populations in the past. In contrast to the vast datasets on effects on mollusks, the knowledge on impacts on species from other taxa remained in the uncertain until almost two decades ago. The assumption on a long-term TBT-mediated pernicious metabolic bottom–up regulation of the crustacean Crangon crangon population was provoked by the outcome of an EU-project ‘Sources, Consumer Exposure and Risks of Organotin Contamination in Seafood.’ This study reported high TBT body burdens in C. crangon in 2003, at the start of the transition period to the global ban. Experimental research on the TBT impact in C. crangon focused on agonistic interference with natural ecdysteroid hormones at the metabolic pathways regulating growth and reproduction and the biogeochemical distribution of the chemical. In this paper, metabolic, topical and population-relevant biological endpoints in C. crangon and other crustaceans are evaluated in relation to the temporal and spatial trends on TBT’s occurrence and distribution in the field during and after the introduction of the tributyltin restrictions and endocrine-related incidents. Arguments are forwarded to relate the German Bight incident on growth and reproduction failure in the C. crangon population, despite the lack of direct evidence, to the pernicious impact of tributyltin in 1990/91 and previous years. The extreme occurrence of TBT in C. crangon from other parts of the southern North Sea and evidence on the high body burdens as dose metrics of exposure also feeds the suspicion on detrimental impacts in those areas. This paper further demonstrates the complexity of distinguishing and assessing the individual roles of unrelated stressors on a population in an integrated evaluation at the ecosystem level.
Introduction
The application of the broad-spectrum organotin biocide tributyltin as antifouling agent (Figure 1) since the mid-1900s was economically a success until, at the end of the 1970s, fertility and calcification impairments on oysters and induction of imposex and intersex in female snails linked TBT to the decline and local extinction of many mollusk species (Smith, 1981; Alzieu et al., 1982; Bryan et al., 1986, 1989; Gibbs et al., 1987; Waldock et al., 1988; Mensink et al., 1996a; Ide et al., 1997; Oehlmann et al., 1998, 2007). TBT-induced imposex and intersex in gastropod mollusks provided some of the strongest evidence on the occurrence of endocrine disruption in the field (Gibbs et al., 1988).
The economic damage on shellfish industries compelled countries to implement measures to assure the sustainable management of these resources. France was the first responding country by issuing a ban in 1982 on the application of organotin-based paints on ships < 25 m waterline and fish farms. Similar bans followed suit between 1987 and 1991 throughout the North Sea countries. In 1989, the EU imposed such measures to all Member States (EU Council Directive 89/677/EEC, European Commission, 1989) and the IMO acted accordingly on a global scale in 1990 (IMO resolution Mepc.29(25), 1990).
In 2001, the IMO adopted the ‘International Convention on the Control of Harmful Antifouling Systems, 2001,’ formalizing a global ban on the application of organotin antifouling agents on marine vessels after the deadline of 17 September, 2008 [AFS Convention, modified in 2010 (IMO resolution Mepc.195(61), 2010)] to include guidelines on survey and certification of antifouling systems on ships). The EU transposed the 2001 AFS Convention into Regulation (EC) 782/2003 (EU Regulation No. 782/2003, European Union, 2003) which banned the application of TBT on EU-flagged vessels starting on 1 January, 2003. That regulation further obliged all ships visiting EU ports from 1 January, 2008 onwards to be free of TBT or at least to bear a barrier coating.
In contrast to the vast datasets and assessments on endocrine TBT disruption in mollusks at population-relevant endpoints up to extinction, metabolic to apical effects on other taxa were seldom identified until during the last two decades. Research on effects in other taxa was initially based on lethal and sublethal endpoints. In crustaceans, acute 96 h-LC50 values of 28.5–41 μg TBT oxide (TBTO)/l did not draw up a particular sensitivity of adult brown shrimp (Crangon crangon; Figure 2; Champ, 1986; Verhaegen, 2012; Verhaegen et al., 2012). The larval stages of C. crangon showed more sensitive acute mortality at 1.5 μg TBTO/l and larval growth of the American lobster (Homarus americanus) nearly stopped at 1 μg TBTO/l (Laughlin and French, 1980; United States Environmental Protection Agency [EPA. US], 1985).
The outcome of the EU-project on Sources, consumer exposure and risks to organotins (OTs) accumulated in seafood (OT-SAFE; Willemsen et al., 2004) alerted serious concerns on the environmental risks related to the transfer of accumulated TBT in C. crangon to the human food chain as well as on the health of the population in its major habitat, the southern North Sea (Verhaegen et al., 2011, 2012; Verhaegen, 2012). The southern North Sea is the main fisheries area on C. crangon. This species was a target organism in the OT-SAFE study for its high economic value. The economic importance in Europe peaked since 2006 till 2014 at average annual catches of 30,000 to 38,000 tons and a third-place ranking in landing value of European fisheries products, exceeding 100 Million Euro in some years (ICES, 2011, 2017). Ecologically, its population can locally comprise up to 80% of the total epibenthic biomass in the southern North Sea (Bamber and Henderson, 1994; Cattrijsse et al., 1997).
This paper assesses different aspects of TBT pollution in C. crangon in its main distribution range, the southern North Sea and does not focus on an overall review on TBT data and effects in the marine environment, as the TBT levels vary by a high factor in different locations. For comprehensive reviews, we refer to, among other (Evans et al., 1996; Fent, 1996; Champ, 2000; Birchenough et al., 2002; Stronckhorst and van Hattum, 2003; Antizar-Ladislao, 2008; Morton, 2009; Oliveira et al., 2009; Rodríguez et al., 2009; Sousa et al., 2009; Bengtsson and Wernersson, 2012; Sunday et al., 2012; Matthiessen, 2013; Wetzel et al., 2013; OSPAR, 2014; Langston et al., 2015; Nicolaus and Barry, 2015; Wilson et al., 2015; Schøyen et al., 2018).
TBT Levels in the Southern North Sea
It is undeniable that the several routes of bioaccumulation generated high TBT body burdens in many species from different taxa in charged areas. Thorough research on the impact of TBT in C. crangon followed on the OT-SAFE project (Willemsen et al., 2004). In 2003, at the start of the global TBT ban transition period, samples from the coastal sampling site BCS3 in Belgium in the southern North Sea contained high TBT levels in the edible tail tissue of the organism (average 326 μg TBT cation/kg tail dw) indicating a high accumulation potential, in comparison to, e.g., plaice (Pleuronectes platessa) muscle tissue, sampled at the same place and date at BCS3, contained TBT levels below the limit of detection (<4 μg TBT cation/kg ww; Willemsen et al., 2004). The levels of the other OTs monobutyltin, dibutyltin, monophenyltin, and diphenyltin were all not quantifiable (Verhaegen et al., 2012). The highest levels of TBT in C. crangon in 2003–2005, measured in the nearby Western Schelde estuary, ranged from 350 to 1700 μg TBT cation/kg dw upstream (Janssen et al., 2007). In 2009, these extreme TBT levels rapidly had decreased as a result of the 2003–2008 global ban. A large-scale geographical one-off survey along the southern North Sea coast in 2009 (Figure 3) revealed a rapid TBT elimination in C. crangon from open sea and the estuaries (Verhaegen, 2012; Verhaegen et al., 2012), compared to the data in Willemsen et al. (2004) and Janssen et al. (2007). In terms of consumer risks of TBT contamination in seafood, there is growing certainty that the current levels in C. crangon from open sea no longer present an intolerable human health risk (Verhaegen, 2012; Verhaegen et al., 2012). The authors calculated an allowed daily consumption of 5.22 ± 2.86 kg peeled C. crangon by a 60 kg weighing person in accordance with the maximum Tolerable Daily Intake (TDI) for TBT set by the EFSA. This renders brown shrimp again a healthy product, in comparison to its status a decade ago. In 2003, a daily consumption of 169 g peeled C. crangon would be sufficient to exceed the TDI (Willemsen et al., 2004). There is to our knowledge no information on risk to consumers available prior to 2001.
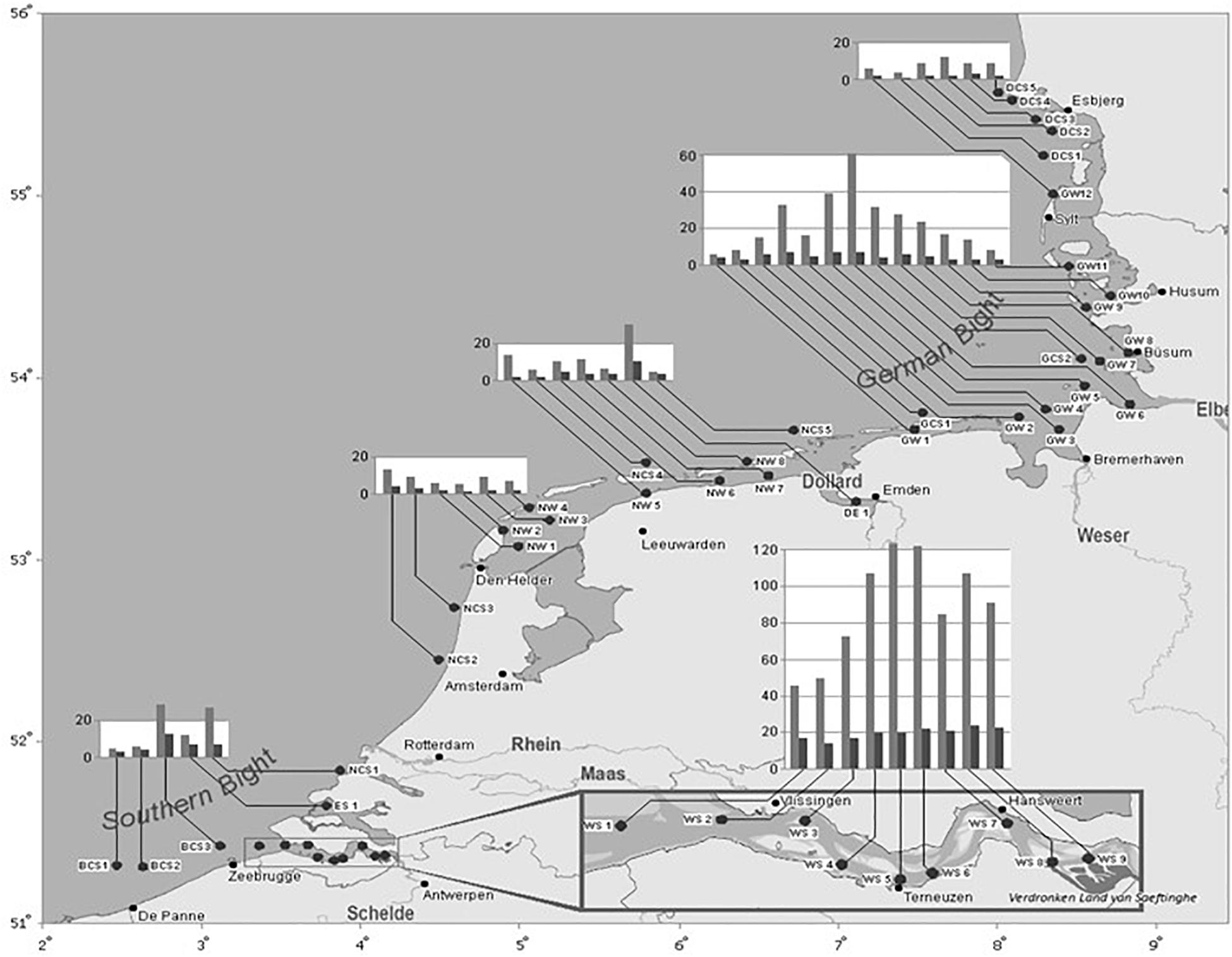
Figure 3. Spatial distribution of TBT (light gray bars) and triphenyltin (dark gray bars) concentrations in C. crangon muscle (Y-axis, in μg/kg dw) along the south coast of the North Sea in 2009 (Verhaegen, 2012; Verhaegen et al., 2012). The X and Y axes represent the longitude Northern length (NL) and the latitude Eastern length (EL), respectively.
A baseline study of the TBT distribution in surface sediment of the Elbe estuary in 2011 ranged from ‘undetectable’ at the mouth to 100 μg TBT cation/kg sediment dw near the port of Hamburg (Wetzel et al., 2013; Sn to TBT cation conversion rate 2.44). At the 2003 and 2009 common sampling site BSC3, a 10-fold decrease in TBT levels was measured in the organism as well as in its habitat sediment.
Based on these TBT data, an average individual heavy metal-type biota to sediment accumulation factor 10 (BSAF ∼ 10) was derived from the sediment < 63 μm fraction data (Verhaegen, 2012; Verhaegen et al., 2012) using the method of Ankley et al. (1994) instead of using suspended solids data, as applied by Veltman et al. (2006). For an earlier year (1999), TBT contamination data in C. crangon were calculated from the 1999 Western Schelde sediment data and extrapolated 2003 BCS3 open sea sediment data using the BSAF: 900 and 650 μg TBT cation/kg dw C. crangon tail, respectively. These 1999 data suggest extreme contamination in the southern North Sea in the previous century prior and after the introduction of the first major TBT ban in 1989 on small ships.
In 1987, a high degree of imposex was observed in the vicinity of pleasure craft activity, fishing harbors and boat yards, reflecting localized inputs from these sources (Bailey and Davies, 1988). Another indication of the widespread TBT contamination in the southern North Sea are the data obtained from the mollusk Buccinum undatum. Data from 1995 on TBT, DBT, and MBT in samples from an offshore sampling station of Helgoland (German Bight, southern North Sea) ranged, respectively, from 2.73 to 38.2, 11.2 to 76.4, and 18.0 to 81.9 μg/kg dw (recalculated from the data of Ide et al., 1997 at a dw/ww conversion factor of 2.73). Similar TBT levels were found in this species from other areas in the southern North Sea: 2.5 to 28.8 μg TBT/kg dw along the coast of the Netherlands (ten Hallers-Tjabbes et al., 1996) and 11.5 to 11.8 μg TBT/kg dw in the Eastern Schelde (Mensink et al., 1996b).
The high distribution ratios of DBT/TBT and MBT/TBT in Buccinum undatum point to enhanced metabolic degradation of TBT which complicates the interpretation of body burden data in those metabolizing species. In snails, there is evidence that incorporated TBT undergoes biotransformation to DBT and MBT in the digestive gland (Bryan et al., 1993). Accumulation and catabolism of TBT is very species specific. Blue crab (Callinectes sapidus), fed contaminated prey grass shrimp (Palaemonetes pugio), significantly debutylated the accumulated TBT while this was not the case in the prey (Rice et al., 1989). In C. crangon, the unquantifiable DBT and MBT levels are a sign of reduced or no catabolism making the measured high TBT levels the actual body residues. Limited metabolic degradation was also observed in Caprellidae (Takahashi et al., 1999).
Tributyltin time trends following on the local and global TBT restrictions indicate sufficiently high elimination rates to drastically reduce the levels in a time span of a few years in recovering areas and have, unequally in time, led to a large-scale progressive recovery of the marine ecosystem (Evans et al., 1996; Birchenough et al., 2002; Morton, 2009; Oliveira et al., 2009; Rodríguez et al., 2009; Sousa et al., 2009; Verhaegen, 2012; Verhaegen et al., 2012; Matthiessen, 2013; OSPAR, 2014, 2017a,b; Langston et al., 2015; Nicolaus and Barry, 2015; Wilson et al., 2015; HELCOM, 2018; Schøyen et al., 2018). The unequal recovery in time is not surprising since the 1989 TBT restrictions on small ships had mainly a local impact in predominantly recreational and small ships areas. There is evidence from sites where commercial vessels were the only source of TBT (Birchenough et al., 2002). The TBT use in antifouling even peaked in 1996, despite the 1989 TBT-ban, when 85% of the larger ships were equipped with a TBT-based coating (OSPAR Commission, 2011). The rapid recovery of C. crangon between 2003/2005 and 2009 was, e.g., also seen in an earlier period in the Baltic Sea between 1998 and 2005 where the levels in mussel (Mytilus edulis) decreased from 250 to 300 μg TBT/kg dw in 1998 to a threshold value of 12 μg TBT cation/kg dw since 2005 (HELCOM, 2018). These threshold values seem long-lasting due to the negative influences of historical contamination in hot spots, repair docks, and harbor areas (Birchenough et al., 2002; Stronckhorst and van Hattum, 2003).
TBT: Distribution, Bioavailability, and Uptake
The scientific debate on the biogeochemical behavior of TBT in the marine environment did not reach a commonly accepted consensus on its occurrence and distribution. Even though neutral TBT derivatives are known to partition to lipid and organic carbon (Meador, 2000; Brändli et al., 2009), the compound is readily ionizable depending on surrounding parameters, firstly pH and related confounding parameters electrochemical ligand binding sites, redox conditions, and salinity.
Partitioning equilibrium values such as the octanol-water partitioning coefficient Kow are not very suitable to predict bioaccumulation of TBT since its derivatives are strongly pH-regulated. Many of the Kow values were calculated or determined at unspecified pH values (Meador, 2011). The Kow of TBT increased from 1600 to 12,000 in a pH range of 5.8 to 8.0 (Tsuda et al., 1990; Arnold et al., 1997). The usefulness of Kow values on TBT in partitioning and bioaccumulation scenarios is therefore strictly conditional.
In aqueous solutions, paint released TBTO and TBT hydride hydrolyze with water forming cations (Eng et al., 1986; Arnold et al., 1997). At the acid-dissociation constant pKa 6.25, half of the TBT is cationic and half is neutralized by anions (Arnold et al., 1997). In seawater at average pH 8 and ionic strength 0.5M 93% of the TBT in solution occurs as the hydroxide complex (Arnold et al., 1997), likely favored by the hydrolyzable nature of TBTO, TBT halides and TBT acetate (Eng et al., 1986). The halides were estimated to have a half-life of 60 days in aqueous media (Sunday et al., 2012) while biodegradation half-lives as low as 6 days have been reported in clean harbor water (Cooney, 1988; Adelman et al., 1990). The predominant occurrence of TBT hydroxide in marine systems was an important indication to link the partitioning behavior of TBT to that observed for neutral hydrophobic substances and predictions based on the Kow equilibrium partitioning (Meador, 2000).
Equilibrium sorption experiments of TBT in estuarine sediments showed similarity of sorption and desorption coefficients indicating that TBT sorption is reversible (Unger et al., 1988). Later research revealed that the TBT sorption in sediment is characterized by a rapid reversible adsorption stage, where 80% of the final sediment concentration is adsorbed within 10 min (Langston and Pope, 1995; Champ and Seligman, 1996), and a slow, non-reversible stage of TBT diffusion into the porous microstructure of the organic material (Pignatello and Xing, 1996; Ma et al., 2000). A similar instant adsorption process was observed in soil while TBT was not easily leached off (Sunday et al., 2012). The sorption of the majority of the neutral TBT hydroxide form, in sediments from coastal waters at average pH 8 to the organic carbon in the sediment might be the principal process. However, a second seemingly less significant underestimated process may be the moored stable metal-type fixation of the remaining approximately 3% TBT cations to electronegative ligands in the sediment, thereby creating a quasi-continuous disequilibrium in the lipophilic partitioning of the neutral OH-form forcing into the direction of fixation on available ligands and steady state conditions. OT compounds are known to interact in the sediment by forming coordination complexes with electronegative oxygen and nitrogen, comparable with the metal-type accumulation in biota (Omae, 1989; Tanabe, 1999).
In biota, the metal-type fixation, accumulation, distribution and bioavailability to cellular processes of bioaccumulating TBT may substantially be activated and regulated by the acidic intracellular pH condition (pHi) which is in electrochemical equilibrium with the pH of the extracellular fluid. A study of Carter (1972) suggests four hypothetical intracellular compartments of different pH within large muscle cells of the crustacean giant barnacle (Balanus nubilus). The pHi of the largest compartment was 6.76 in a relative volume of 20 on 22 and a lowest mean pHi value of 6.12 ± 0.03 was measured in a relative volume of 0.5 on 22, closely in compliance with the mean calculated equilibrium pHi of 6.09 ± 0.03. According to Carter (1972) it is highly likely that the acidic compartment represents the aqueous phase of the cell cytoplasm. The two remaining compartments had a pHi of 7.0 and 7.5 in, respectively, relative volumes of 1.0 and 0.5 on 22. The pHi in all compartments and especially the acidic predominant part of the cells is thus much lower than the pH 8 in the surroundings in coastal waters. Carter (1972) supports the view that hydrogen protons are not distributed homogenously throughout the cytoplasm and that there exist intracellular compartments which have markedly different pHi, in contrast to the mean pHi measurements following, e.g., the homogenate technique of Pörtner et al. (1990) yielding higher integrated pH values. Integrated pHi values of 7.32 ± 0.04 in homogenized tail muscle of C. crangon, as reported by Abele-Oeschger et al. (1997) seem therefore not accurate enough to elucidate the actual TBT distribution and aggressive behavior in cells and tissues. The compartmentalized nature of the pHi and the predominant acidic conditions in the cells may provide new insights in the process of distribution and behavior. Acidic pHi values close to the TBT pKa will increase the ratio of the cation, compared to the ‘neutral’ form (hydroxo-complex?) and increase the proportional interactions of the metal-type behavior of TBT by complex formation with ligands in phospholipids and proteins, as postulated by Arena et al. (1995), Tanabe (1999), Hunziker et al. (2001, 2002), and Strand and Jacobsen (2005). The neutral form appears to partition only to the lipid fraction of the biomembrane (Hunziker et al., 2001) while Doop et al. (2007) suggested that the toxicological potential depends on membrane permeability. In experimental conditions, the biomembrane-water distribution ratio of TBT exceeded the liposome-water distribution ratio at acidic pH 3, which is attributed to complex formation of the cationic species with ligands of the protein fraction. The distribution ratio of the TBT cation exceeded that of the neutral hydroxo-complex by a factor of 2. At higher pH 8.0 the biomembrane-water distribution ratio was found lower than the liposome-water distribution ratio (Hunziker et al., 2001).
The passive migration or electrochemical carrier-translocation of TBT cations or neutral forms through the plasma membrane into the cytoplasm is unclear and both pathways may operate simultaneously at different affinities in the prevailing pHi environment. Passive migration related to compound lipophilicity was demonstrated by Zucker et al. (1989) who measured decreased biomembrane potentials in murine erythroleukemic cells in the presence of TBT. On the other hand, in crustacean copepods, the TBT tissue residue as the dose metric associated with 50% mortality showed no correlation with the lipid content (Meador, 1997).
Conclusively, it is argued that the metal-type fixation in the acidic conditions in biota occurs at a higher ionic activity compared to the metal-type process in sediments at higher pH.
In experimental conditions, the TBT-exposed water pathway became standard in uptake and exposure studies since the toxic responses in mollusks, held in laboratories, showed good correlations with the direct TBT toxicity from bioconcentration. Some mollusks, e.g., Mytilus edulis, and especially predatory gastropods possess high TBT BCFs up to 105 compared to the moderate 103 in crustaceans (ECHA, 2008). A strong correlation between the LC50 and the BCF was also found in amphipod species (Meador, 1997). An individual BCF for C. crangon is not known, instead, the TBT body residues are clearly related to the total TBT content of its habitat sediment (Verhaegen, 2012; Verhaegen et al., 2012).
A higher uptake in mollusks from water may be obvious due to the high BCF. Given the moderate BCF in crustaceans, the extremely high body burdens in C. crangon should be linked to biomagnification and the high average BSAF from contaminated sediment, rather than bioconcentration, confirming the findings of Veltman et al. (2006) on high accumulation rates in C. crangon. More studies describe both accumulation routes from diet and direct uptake from water (Strand and Jacobsen, 2005; Lee et al., 2006). Bryan et al. (1989) described a 50/50 accumulation ratio from diet and surroundings in the carnivorous gastropod Nucella lapillus.
Mechanism of Action of TBT in Crustaceans
Several authors postulated in initial research that the site of the basic mechanism of action of TBT toxicity is the biomembrane (Massaro et al., 1989; Zucker et al., 1989; Gadd, 2000; Hunziker et al., 2001). It is in the meantime known that TBT-mediated endocrine disruption and imposex/intersex developments in the sensitive gastropods Nucella lapillus, Nassarius reticulatus (= Hinia reticulata) and other mollusks are related to TBT’s agonistic interference with the growth- and reproduction NR retinoid-X and its ecdysteroid hormone triggers (Nishikawa et al., 2004; Sousa et al., 2010; Sternberg et al., 2010). It is this ligand-dependent nature that makes many NRs susceptible for exogenous chemicals at extremely low exposure doses. These adverse metabolic alterations at a range of cellular pathways in the mollusks rapidly translated into severe population-level changes in the past.
Similarly, ecdysteroid hormones trigger the specific regulation of several genes in different tissues and developmental stages of crustaceans, through interactions with the NRs EcR and/or RXR (Hopkins, 2009). In contrast to vertebrate RXR, invertebrate RXRs do not form active homodimers but serve as a heterodimerization partner for many other NRs, and as such are involved in the control of multiple endocrine pathways. EcR is the best known invertebrate partner protein for RXR. The name RXR refers to the natural 9-cis-RA, the putative ligand of vertebrate RXRs (Wolf, 2006), whereas the natural agonist(s) for invertebrate RXRs is still under debate. The putative endogenous ligand for crustacean RXR is the terpenoid methyl farnesoate, which has been confirmed in several crustacean species (Reddy et al., 2004; Wu et al., 2004; Laufer et al., 2005) but, e.g., not in the water flea Daphnia magna (Wang and LeBlanc, 2009).
Our current understanding of the principal endocrine mechanism of action of TBT in crustaceans is very similar to that in mollusks and refers to the interactions at the physical, functional and gene-expression levels of the ecdysteroid receptor – retinoid-X receptor dimer (CrcEcR-CrcRXR) complex (Figure 4; Verhaegen et al., 2010, 2011, 2012; Verhaegen, 2012). TBT caused a strong down-regulation of Ponasterone A-induced transactivation in vitro and strong down-regulated expression of CrcEcR/CrcRXR transcripts in vivo in the ovaries (Figure 5). The ovary-specific inhibition (Figure 6) is an initial indication of evolving reproductive impairment, similar to the impact on mollusks. In silico 3D-modeling confirmed a very good docking and blocking accommodation of TBT in the electrostatic and neutral areas of the full-length modeled CrcRXR–Ligand Binding Pocket (Verhaegen, 2012; Verhaegen et al., 2012). cDNA microarray analysis of 604 gender-related cDNA fragments, appended to CrcEcR and CrcRXR isoforms, revealed affected expression of 43 gene fragments and disruption of calcium homeostasis. Upregulation of vitellogenin and up- as well as downregulation of several cuticular proteins may cause a second endocrine disruption on the Y-organ – ecdysteroidal endocrine axis and evolving growth and reproduction impairment (Verhaegen, 2012). The crustacean endocrine axis comprises two major neuroendocrine glands situated in the eyestalks: the X-organ-sinus gland complex, and the Y-organ (Chang and Mykles, 2011).
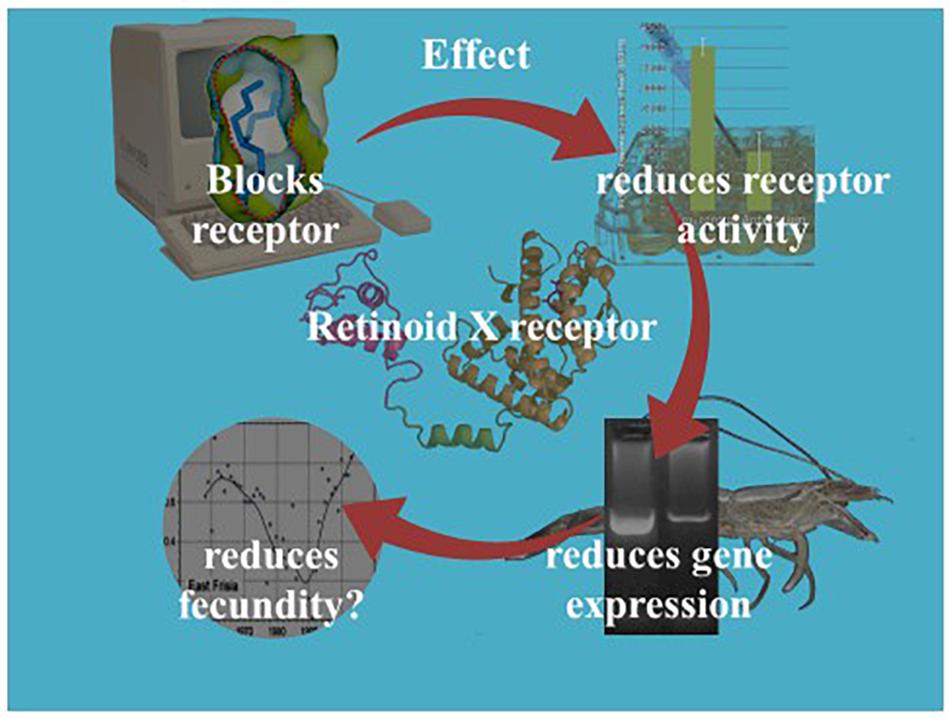
Figure 4. Illustration of the retrospective/diagnostic ecotoxicological research on TBT’s endocrine disrupting role in C. crangon, incorporating in silico, in vitro, and in vivo technologies.
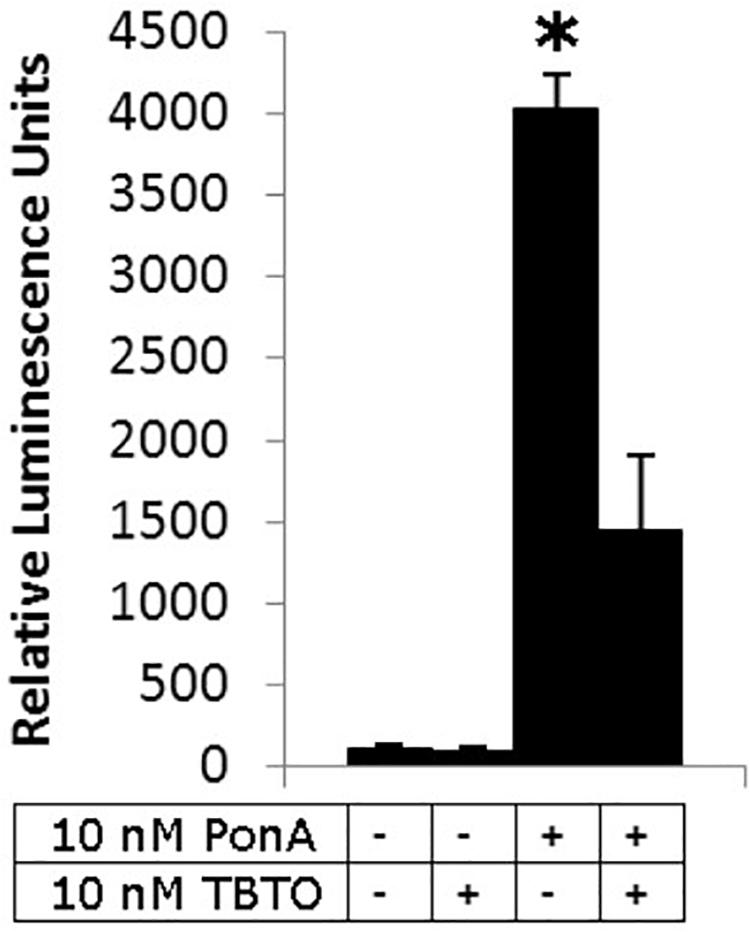
Figure 5. Influence of 10 nM TBTO on reporter gene trans-activation by 10 nM PonA through CrcEcR–CrcRXR. Four different treatments were performed in quadruplicate (from left to right): a negative control, exposure to 10 nM TBTO, a positive control (10 nM PonA) and exposure to 10 nM TBTO in the presence of 10 nM PonA. Data are presented as the mean ± SEM (n = 4). An asterisk denotes a significant difference with the respective control (Student’s t-test, two tailed, p < 0.05; Verhaegen et al., 2011; Verhaegen, 2012).
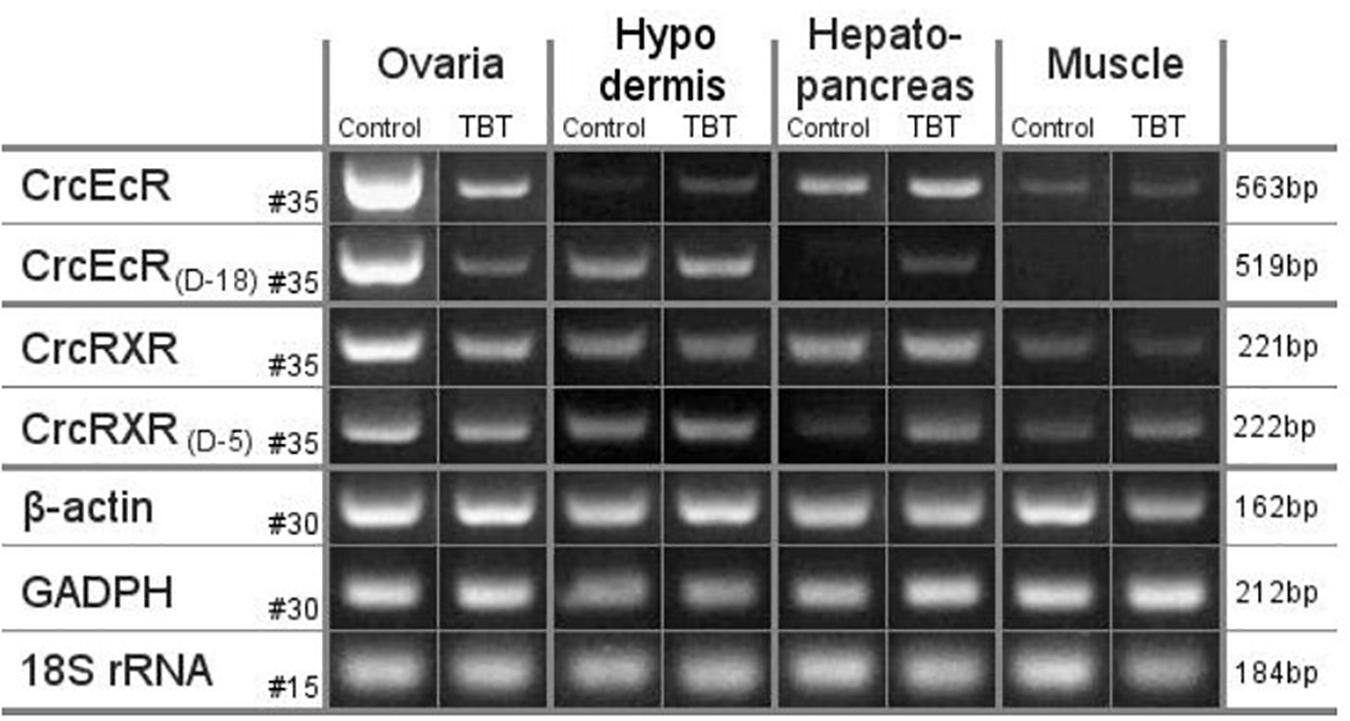
Figure 6. mRNA levels in ovaries, hypodermis, hepatopancreas and muscle of C. crangon after exposure to TBT. Semiquantitative RT-PCR results of C. crangon exposed to 134 nM TBT for 96 h compared with mRNA expression in the control group for the non-truncated CrcEcR and CrcRXR isoforms. mRNA levels of the control genes b-actin, glyceraldehyde-3-phosphate (GADPH) and 18S rRNA are also represented. Amplified gene names and cycle number are given on the left, template tissue and experimental group are given at the top, amplicon size is given at the right. Truncated CrcEcR and CrcRXR isoforms are not shown as they were only detected in the ovaries at higher PCR cycle numbers. CrcEcRD-18 bands were clearly visible for hepatopancreas tissue of the control group and muscle tissue at 40 PCR cycles but not at 35 cycles (Verhaegen et al., 2011; Verhaegen, 2012).
In contrast to vertebrates and Lophothrochozoa (e.g., mollusks), arthropod vitellogenesis is regulated by ecdysteroid and juvenile hormones, and not by estrogens. In arthropods, vitellogenesis is negatively regulated by ecdysteroids (Hannas et al., 2010). Upregulation of vitellogenesis, as confirmed in Verhaegen’s experiments (Verhaegen, 2012), would thus suggest an anti-ecdysteroidal effect of TBT (Verhaegen, 2012). Retarded molting, production of abnormalities in initial and final stages of limb regeneration and endocrine toxicity on ecdysis, vitellogenesis, and calcium resorption in a TBT environment were also proven in other studies on crustaceans (Nagabhushanam et al., 1990; Reddy et al., 1991, 1992; Wang et al., 2011; Hosamani et al., 2017). The interference of TBT in the calcium reabsorption inhibits the exoskeleton formation (Nagabhushanam et al., 1990).
The pathway of the TBT impact on metabolic stages of growth and reproduction in C. crangon seems to some extent identical to the deregulation in mollusks. This is not exceptional since the RXR, the fourth member of group B of the NR subfamily 2, is a highly conserved NR throughout the animal kingdom and mankind (Nishikawa et al., 2004). It is very likely that the mechanism of action of TBT on RXR is very similar in all examined taxa and occurs, presumably, in a thin line with natural ligands since Nishikawa et al. (2004) proved that the vertebrate natural RXR ligand 9-cis-RA, also induced imposex in rock shell Thais clavigera. This deterioration by natural ligands may be attributed to an important role of RXR in the differentiation and growth of male genital tracts in female gastropods (Nishikawa et al., 2004).
TBT Toxicity and Topical and Population-Relevant Endpoints
The highest sensitivity to TBT toxicity has long been assigned to gastropods. In the 80s and 90s, data on the TBT chemical status of the marine environment were commonly addressed via dose–response relationships in toxicity tests on mollusks in TBT-exposed water pathways. Lowest Observed Effect Concentrations as low as 1.0 to 2.0 ng TBT/l exposure water (Gibbs et al., 1987; Rainbow, 1995) brought imposex and intersex prevalence on the forefront as key indicators in monitoring programs in times of challenging developments of analytical techniques, sufficiently specific and sensitive enough to detect TBT concentrations at or below the ng/l (ppt) level in the water column for the reason that strong correlations were found between biological endpoints (e.g., LC50) and the BCF (Meador, 1997) while other studies reported high correlations with whole-body TBT residues as well (King et al., 1989; Hagger et al., 2006).
Intersex is a phenomenon seen in mollusks at much higher TBT contamination. E.g., intersex in the periwinkle Littorina littorea occurs at 100-fold higher TBT concentrations than imposex and is used as biomarker in mollusks in areas of high TBT exposure (Oehlmann et al., 1998). In laboratory tests on crustaceans TBT-induced macroscopic changes on the growth and reproduction morphology have been reported for population relevant endpoints, such as intersex, fecundity, % ovigerous females, reproduction, larval development. LOEC and NOEC in long-term and full life-cycle bioassays on several species ranged, respectively, from 10 to 190 ng TBT/l and 6.0 to 224 ng TBT/l (Johansen and Mohlenberg, 1987; Bushong et al., 1988, 1990; Kusk and Petersen, 1997; Hall et al., 1998; Oberdörster et al., 1998; Baer and Owens, 1999; Ohji et al., 2002; Schmidt et al., 2005; Huang et al., 2006; Lagadic et al., 2017). One outlier NOEC of 1250 ng TBT/l was reported for adult D. magna in a 21 days test with offspring as biological endpoint (Oberdörster et al., 1998).
The development of SSD models, based on the lowest NOEC and LOEC data from water-exposure pathways, have shown that many aquatic species from different taxa, including crustaceans, are in the range of sensitivity of mollusks and some fish species showed higher sensitivity (Lagadic et al., 2017). The accumulation of TBT in organisms as well as surroundings in most charged areas in the past were therefore undeniably transcending the vulnerability of crustaceans and species from other taxa as well for population relevant endpoints.
It should be noted that the in vivo experiments of Verhaegen et al. (2011) on expression capacity of CrcEcR/CrcRXR transcripts in C. crangon’s tissues were performed at (sub)lethal TBT concentrations because of the necessity for using short-term exposure tests since attempts to culture C. crangon in full life-cycle conditions still remain unsuccessful. However, the in vitro inhibition of CrcEcR/CrcRXR transcripts and affected expression of genes, disruption of calcium homeostasis and the acute lethality of C. crangon’s and H. americanus’ larvae demonstrated a higher toxicity at metabolic and topical endpoints (Laughlin and French, 1980; Verhaegen et al., 2011).
Knowledge Gaps
A critical gap in the toxicological research on TBT exposure is the need on information on the impact of the TBT body burdens on biological processes in affected organisms with the aim to more realistically assess the overall TBT impact in the field. It was postulated that the tissue residues reflect the bioavailability and effective target doses more accurately than the toxicity based on the water-exposure pathway (Meador, 2011) on standard laboratory-maintained organisms or their offspring. The variability in responses to toxicants in laboratory studies may occur over the natural, seasonal cycle of physiological variation that occurs in populations and organisms, held in laboratory, may become multi-fold more sensitive (Meador, 1993). Several studies have additionally found that when toxicity is expressed as a tissue residue, the variability between species, time periods and exposure conditions is greatly reduced (McCarty, 1991; van Wezel and Opperhuizen, 1995; Meador, 1997).
Unequal tissue distribution and TBT behavior may additionally influence the effective target dose to responsive tissue-specific biological endpoints. Several gastropod species concentrate up to 50% of the total TBT in the female gonads (Oehlmann et al., 1992; Stroben, 1994). The BCFs in the carp Carassius carassius grandoculis, obtained in exposure experiments, are also tissue-dependent and may vary almost 10-fold: 589 (muscle), 547 (vertebra), 5012 (liver), and 3162 (kidney) (Tsuda et al., 1986). Research on the chemical tissue partitioning of TBT in C. crangon was not a work package in Verhaegen (2012), however, in contrast to the absent responses in the hypodermis, hepatopancreas and muscle tissues, the exclusive and highly affected expression of CrcEcR/CrcRXR transcripts in vivo in the ovaries (Figure 6) may also indicate enhanced accumulation and disruption in that tissue.
TBT: A Putative Bottom–Up Regulator of C. crangon’s Population?
In contrast to the temporal and spatial trends in pernicious impacts on mollusks through female sterility associated with imposex and intersex, where the cause was rapidly attributed to TBT, and recovery following on the TBT restrictions, the assumption on TBT-mediated deregulation of C. crangon remained unclear and hypothetical since this species was commonly regarded as non-endangered during half a century. However, the underlying metabolic mechanism of action in both taxa was only unraveled until after 2000.
The question whether the TBT prevalence was an important indicator of the population health status of C. crangon is now no longer relevant as a result of the TBT bans. However, it remains a scientific necessity and societal obligation to be aware of the former threats on the population by the use of this chemical, in an ecosystem and economic perspective.
The most striking potential link between TBT and population-relevant endpoints in C. crangon may have happened before the TBT bans were introduced. Research on the seasonal spawning cycle and reproductive success in subareas of the German Bight in the North Sea in the period 1958–2005 linked reproductive impairment to low percentages of ovigerous females on the basis of morphological information (see review, Siegel et al., 2008). A decrease in the proportion of ovigerous females started in the western part of the German Bight (the Waddenzee) in the second half of the 1970s, where it dropped below 50% of the previous status. The absolute minimum was observed in the late 1980s with ovigerous female proportions below 10%. The subsequent low reproduction and recruitment caused dramatic drops in landings of consumption shrimp since the catches in the German Bight account for approximately 90% of the total European catch. Correlation analyses with common parameters, such as water temperature, river runoff, North Atlantic Oscillation climate index and the more obvious indicators predator and fishing mortality did not show any plausible proximate cause of this large-scale population impact (Siegel et al., 2008). Watermann and Dethlefsen (1983) postulated pollution-induced “dissolutions” of the shell and subsequent secondary infections. Unfortunately, a lack on data from other areas prevented larger geographical-scale comparisons and TBT-induced biological endpoints on growth and reproduction were at that time only extensively described in mollusks from TBT-charged areas. C. crangon is phylogeographically similar across its distribution range (Luttikhuizen et al., 2008; Hoffman, unpublished results). The whole population for management purposes is considered as one stock (ICES, 2014) which may also indicate similarity in sensitivity to TBT in a large geographical area.
The nature of the observed disorders in the German Bight survey, percentage affected ovigerous females and low recruitment were later diagnosed in conventional full life-cycle exposure tests on several crustacean species, e.g., at a LOEC of 10 ng/l in the copepod Schmackeria poplesia (Ohji et al., 2002; Huang et al., 2006, 2010; Lagadic et al., 2017). The described ‘dissolutions’ of the shell may in turn refer to ecdysis and vitellogenin disruption in the process of the endocrine toxicity on expression of cuticular proteins and eventually vitellogenin, as postulated by Verhaegen (2012). Further, Reddy et al. (1992) reported significant effects after the third molt in the prawn Caridina rajadhari. Pollution-induced impacts by a range of other priority chemicals seem not an issue in this incident since downward trends were general (OSPAR, 2010) and the severity of the toxicities of many of these chemicals compared to the toxic potential of TBT may be arguable.
A second indication on the putative deterioration by TBT is the stock rebound in the German Bight in the course of the 1990s (Siegel et al., 2008), shortly after the European 1989 TBT ban was introduced. A rapid recovery was remarkable in yachting areas while industrialized maritime areas showed delays until the implementation of the global TBT-ban (Verhaegen, 2012; Verhaegen et al., 2012).
The breakdown of the catches in 1990/1991 was in the 2014 WGCRAN report (ICES, 2014) suggested to be caused by a mass invasion of 0-group whiting (Merlangius merlangus). It is obvious that a decrease in commercial landings is linked to rebuilding stocks of the main predators cod (Gadus morhua) and whiting. Both cod and whiting can have high shares of C. crangon in their stomach (Temming and Hufnagl, 2014) although both species seem to prefer the co-occurring gobies in their diet (Jansen, 2002) and growth rates are smaller on homogeneous C. crangon diets (Temming, 1995). Events of extremely abundant cod and/or whiting 0-groups occurred in the years 1959, 1961, 1970, 1977, 1983, 1990, and 1998 (Tiews, 1961; Berghahn, 1996; ICES, 1998; Siegel et al., 2005). Increased predator invasions matched to drops in commercial shrimp landings in several years (1977, 1983, 1990, and to a lesser extent 1998). Increased predator abundances are, however, in the event of the German Bight incident and the disastrous 1990/91 breakdown of the catches not a justifiable argument for the population-level occurrence of overall growth, reproduction and recruitment failure. The German Bight incident is, in our opinion, fully attributable to endocrine disruption by the most prevalent chemical TBT and predation mortality at that time may have played a secondary lesser impact on the stock.
A different scenario manifested post 2000 till now complicating the distinction between the proportional influences of the different established population drivers. The gradual increase in landings of consumption shrimp maximized after 2003 (>32,000t) to 37,000t in 2014 (ICES, 2011, 2017). The clear relationship between the stepwise temporal and spatial rebound of the stock, the implementation of the 1989 partial ban, the 2003–2008 global ban and the fading out of the TBT prevalence to threshold values may have restored sustainable shrimp fisheries making the other drivers such as predation mortality, climate change and fishing effort more visibly accountable. Data on stock assessments and landings statistics of cod and whiting confirmed downward trends by, respectively, 58 and 25% in numbers between 2001 and 2010. Updates with predator distribution maps and effects of climate change revealed a shift in range of distribution of both cod and whiting, resulting in a reduced overlap with C. crangon (Kirby et al., 2009; Temming and Hufnagl, 2014).
Estimates on predator influences on the population dynamics also fluctuated by the differences in trends of predator abundances. ICES (2015a, b) informed on abundances a downward trend on whiting and an upward trend on cod. Cod abundances in the North Sea have increased because cod fishing mortality rates have been reduced since 2000, in combination with a stronger spawning stock biomass in 1999, 2005, and 2009. The North Sea cod spawning biomass had tripled in the years after the lowest estimated level of 21,000t in 2006 (ICES, 2015a). However, these changes in predation levels had seemingly no profound impact on the commercial shrimp catches in the southernmost areas of the North Sea.
So far, the fluctuating influences of the predation mortality on C. crangon’s population have interfered with a putative impact of TBT preventing a clear signal on a population relevant endpoint. The far-reaching elimination of TBT from the marine environment has ensured that TBT’s putative role on the health of the population has in the meantime minimized.
Recently, another stressor, fishing effort, which has never been regarded as a potential threat, has reached the highest efforts in 2013 and 2014 (ICES, 2015b). This impact resulted in an overall decrease in LPUE indicating an uncontrolled effort increase, overfishing and the need for a management plan to restore sustainable shrimp fisheries (ICES, 2013, 2014, 2016). 2015 was the turning year to again much lower commercial landings in 2016 (25,900t) and the lowest level since 1995 (ICES, 2017). In the years before 2000, Welleman and Daan (2001) quantified the yield of the total annual shrimp landings to only 5 to 10% of the total predation mortality. In 2012, an update of Welleman and Daan suggested fishing mortality beyond natural (predation) mortality (ICES, 2012). In 2015, the fishing pressure was estimated four times higher than natural mortality and the population was declared overfished (ICES, 2015b). Alarming is the U-turn to overfishing since, based on the findings of Welleman and Daan (2001), the yield of the landings could increase 10-fold or more, at zero predation mortality. It seems that the distinction between the influences of changes in fishing effort and predation are thin since the 2016 survey data again suggested a relationship between high abundances of whiting (Merlangius merlangus) and low shrimp abundances in the German areas (ICES, 2017). The suspected reason on the current overfishing can be found in the high shares of undersized shrimp compared to commercial sized shrimp in the catches, on average 0.85 L in the pulse gear and 0.68 L in the traditional gear per liter consumption shrimp (ICES, 2015b, 2016). These undersized shrimp are less discarded and landed nowadays causing recruitment overfishing despite a survival range of discarded shrimp between 75 and 80% (ICES, 2016). The impact of this change in fisheries attitude seems enormous and would be better assessed when expressed in numbers instead of liters.
Conclusion
A potential population-level endocrine disruption by TBT may have interfered unnoticed during the past 60 years of C. crangon’s life history and almost the entire history of its recorded fisheries. Attention to (sub-)chronic TBT toxicity in crustaceans was long not an issue of particular interest due to the initial moderate lethal toxicity compared to mollusks, and C. crangon’s long-term non-endangered status.
Most assessments on TBT impact on organisms in the marine environment were based on water exposure toxicity correlations between effects and TBT levels in water. The development of SSD models were also based on water exposure toxicity data in often full life-cycle tests on several taxa. These SSD models demonstrate more similarity in sensitivity to TBT among taxa.
Despite the lack on full life-cycle toxicity information on topical endpoints in C. crangon, the available information on biological endpoints in C. crangon and other crustacean species, delivers in our view, sufficient arguments to strengthen the hypothesis on population-relevant endocrine disruption to clarify the German bight incident in before and during 1990/91.
The high TBT accumulation in charged areas, especially in organisms lacking TBT catabolism, suggests that biomagnification played a higher distinctive role than could be expected from bioconcentration. The extremely high body residues of TBT in the past and our predictions on enhanced intracellular partitioning allow to suggest that the TBT toxicity in the marine environment has been underestimated, compared to the water exposure pathway toxicity.
The common mechanism of action on RXR throughout the animal kingdom and mankind at the lower ppt level provoked the implementation of stringent environmental assessment criteria and quality standards in the marine environment to secure the ecosystem and human health (EFSA, 2004; European Union, 2005; United States Environmental Protection Agency [EPA.US], 2007; Cole et al., 2015; HELCOM, 2018).
The historical contamination in hotspots, harbors and estuaries in the marine environment and the continued on-land use (Cole et al., 2015) has brought the TBT levels to thresholds which may remain long-lasting, especially in anoxic sediments.
Up-to-now no safe alternative to TBT is available. The antifouling industry was forced to fall back on toxic booster biocides such as copper, triazines and not at least tralopyril, all having their respective and often unknown and severe impacts. It is therefore strongly recommended to scrutinize the potential environmental impact of all new antifoulants prior to their acceptance in antifouling applications.
Author Contributions
GS and KC were the promotors and KP the daily mentor of co-author YV for his Ph.D. dissertation on Mechanism, Concentrations and Effects of Tributyltin in common shrimp Crangon crangon. The results of the research of YV and colleagues were frequently reviewed in the ICES Working Group on Biological Effects of Contaminants. This submitted manuscript (review) is a follow-up on the work of YV and colleagues on the potential detrimental role of tributyltin on the shrimp population in an integrated ecosystem assessment. All authors are or were actively involved in the tributyltin pollution research. ILVO was partner within the framework of the EU-project on ‘Sources, Consumer Exposure and Risks of Organotin contamination in Seafood.’
Conflict of Interest
The authors declare that the research was conducted in the absence of any commercial or financial relationships that could be construed as a potential conflict of interest.
Abbreviations
9-cis RA, 9-cis-retinoic acid; AFS, Convention on the Control of Harmful Antifouling Systems of IMO; BCF, bioconcentration factor; BSAF, biota to sediment accumulation factor; cDNA, copy- or complement-DNA; CrcEcR, C. crangon ecdysteroid receptor; CrcRXR, C. crangon retinoid X-receptor; DBT, dibutyltin; Dw, dry weight; ECHA, European Chemicals Agency; EcR, ecdysteroid receptor; EFSA, European Food Safety Authority; EU, European Union; ICES, International Council for the Exploration of the Sea; IMO, International Maritime Organization; Kow, Octanol-Water Partition Coefficient; LC50, median lethal concentration; LOEC, Lowest Observed Effect Concentration; LPUE, Landings per Unit Effort; MBT, monobutyltin; MEPC, Marine Environment Protection Committee of IMO; NOEC, No Observed Effect Concentration; NR, nuclear receptor; OT, organotin; OT-SAFE, Sources, consumer exposure and risks to organotins (OTs) accumulated in seafood; pHi, Intracellular pH; pKa, Acid-dissociation constant; RXR, retinoid-X-receptor; SSD, Species Sensitive Distribution; TBT, tributyltin; TBTO, Bis(tributyl)tin oxide.
References
Abele-Oeschger, D., Sartoris, F. J., and Pörtner, H.-O. (1997). Hydrogen peroxide causes a decrease in aerobic metabolic rate and in intracellular pH in the Shrimp Crangon crangon. Comp. Biochem. Physiol. 117C, 123–129. doi: 10.1016/s0742-8413(97)00059-5
Adelman, D., Hinga, K. R., and Pilson, M. E. Q. (1990). Biogeochemistry of butyltins in an enclosed marine ecosystem. Environ. Sci. Technol. 24, 1027–1032. doi: 10.1021/es00077a012
Alzieu, C., Heral, M., Thibaud, Y., Dardignac, M., and Feuillet, M. (1982). Influence des peintures antisalissures a base d’organostanniques sur la calcification de la coquille de l’huitre Crassostrea gigas. Revue des Travaux de l’Institut des pêches maritimes. 45, 101–116.
Ankley, G. T., Leonard, E. N., and Mattson, V. R. (1994). Prediction of bioaccumulation of metals from contaminated sediments by the oligochaete Lubriculus variegatus. Water Res. 28, 1071–1076. doi: 10.1016/0043-1354(94)90192-9
Antizar-Ladislao, B. (2008). Environmental levels, toxicity and human exposure to tributyltin (TBT)-contaminated marine environment. A review. Environ. Int. 34, 292–308. doi: 10.1016/j.envint.2007.09.005
Arena, G., Calì, R., Contino, A., Musumeci, A., Musumeci, S., and Purrello, R. (1995). Coordination properties of dialkyltin (IV) in aqueous solution. Thermodynamic study of dimethyltin (IV) complexes with l-amino acids. Inorgan. Chim. Acta 237, 187–191. doi: 10.1016/0020-1693(95)04655-S
Arnold, C. G., Weidenhaupt, A., David, M. M., Müller, S. R., Haderlein, S. B., and Schwarzenbach, R. P. (1997). Aqueous speciation and 1-octanol–water partitioning of tributyl- and triphenyltin: effect of pH and ion composition. Environ. Sci. Technol. 31, 2596–2602. doi: 10.1021/es970009
Baer, K. N., and Owens, K. D. (1999). Evaluation of selected endocrine disrupting compounds on sex determination in Daphnia magna using reduced photoperiod and different feeding rates. Environ. Contam. Toxicol. 62, 214–221. doi: 10.1007/s001289900862
Bailey, S. K., and Davies, I. M. (1988). Tributyltin contamination in the Firth of Forth (1975 – 1987). Sci. Total Environ. 76, 185–192. doi: 10.1016/0048-9697(88)90106-4
Bamber, R. N., and Henderson, P. A. (1994). Seasonality of caridean decapod and mysid distribution and movements within the Severn Estuary and Bristol Channel. Biol. J. Linnean Soc. 51, 83–91. doi: 10.1006/bijl.1994.1009
Bengtsson, H., and Wernersson, A. (2012). TBT, Koppar, Zink Och Irgarol I Dagvatten, Slam Och Mark I Småbåtshamnar. Göteborg: Länsstyrelsen i Västra Götaland, 16.
Berghahn, R. (1996). Episodic mass invasions of juvenile gadoids into the Wadden Sea and their consequences for the population dynamics of brown shrimp (Crangon crangon). Mar. Ecol. 17, 251–260. doi: 10.1111/j.1439-0485.1996.tb00506.x
Birchenough, A. C., Evans, S. M., Moss, C., and Welch, R. (2002). Re-colonisation and recovery of populations of dogwhelks Nucella lapillus (L) on shores formerly subject to severe TBT contamination. Mar. Pollut. Bull. 44, 652–659. doi: 10.1016/s0025-326x(01)00308-3
Bryan, G. W., Bright, D. A., Hummerstone, L. G., and Burt, G. R. (1993). Uptake, tissue distnbution and metabolism of 14C-labelled tributyltin (TBT) in the dog whelk, Nucella lapillus. J. Mar. Biol. Assoc. UK. 73, 889–912. doi: 10.1017/s0025315400034792
Bryan, G. W., Gibbs, P. E., Hugett, R. J., Curtis, L. A., Bailey, D. S., and Dauer, D. M. (1989). Effects of tributyltin pollution on the mud snail, Ilyanassa obsolete, from the York river and Sarah creek, Chesapeake bay. Mar. Pollut. Bull. 20, 458–462. doi: 10.1016/0025-326x(89)90067-2
Bryan, G. W., Gibbs, P. E., Hummerstone, L. G., and Burt, G. R. (1986). The decline of the gastropod Nucella lapillus around south-west England; evidence for the effect of tributyltin from antifouling paints. J. Mar. Biol. Assoc. UK. 66, 611–640. doi: 10.1017/s0025315400042247
Brändli, R. C., Breedveld, G. D., and Cornelissen, G. (2009). Tributyltin sorption to marine sedimentary black carbon and to amended activated carbon. Environ. Toxicol. Chem. 28, 503–508. doi: 10.1897/08-236.1
Bushong, S. J., Hall, L. W. Jr., Hall, W. S., Johnson, W. E., and Herman, R. L. (1988). Acute toxicity of tributyltin to selected chesapeake bay fish and invertebrates. Water Res. 22, 1027–1032. doi: 10.1016/0043-1354(88)90150-9
Bushong, S. J., Ziegenfuss, M. C., Unger, M. A., and Hall, L. W. (1990). Chronic tributyltin toxicity experiments with the Chesapeake bay copepod, Acartia tonsa. Environ. Toxicol. Chem. 9, 359–366. doi: 10.1002/etc.5620090311
Cattrijsse, A., Dankwa, H. R., and Mees, J. (1997). Nursery function of an estuarine tidal marsh for the brown shrimp Crangon crangon. J. Sea Res. 38, 109–121. doi: 10.1016/s1385-1101(97)00036-1
Champ, M. A. (1986). “Organotin symposium: introduction and review,” in Proceedings of the Conference Oceans 86, Vol. 4, ed. G. L. Maton, (Washington, DC: Marine Technology Society), 1093–1100.
Champ, M. A. and Seligman, P. F. (Eds) (1996). Organotin: Environmental fate and effects. Netherlands: Springer. 475–483.
Champ, M. A. (2000). A review of organotin regulatory strategies, pending actions, related costs and benefits. Sci. Total Environ. 258, 21–71. doi: 10.1016/s0048-9697(00)00506-4
Chang, E. S., and Mykles, D. L. (2011). Regulation of crustacean molting: a review and our perspectives. Gen. Comp. Endocrinol. 172, 323–330. doi: 10.1016/j.ygcen.2011.04.003
Cole, R. F., Mills, G. A., Parker, R., Bolam, T., Birchenough, A., Kröger, S., et al. (2015). Trends in the analysis and monitoring of organotins in the aquatic environment. Trends Environ. Anal. Chem. 8, 1–11. doi: 10.1016/j.teac.2015.05.001
Cooney, J. J. (1988). Microbial transformations of tin and tin compounds. J. Indust. Microbiol. 3, 195–204. doi: 10.1007/bf01569577
Doop, E., Hartmann, L. M., von Recklinghausen, U., Florea, A. M., Rabieh, S., Shokoushi, B., et al. (2007). The cyto- and genotoxicity of organotin compounds is dependent on the cellular uptake capability. Toxicology 232, 226–234. doi: 10.1016/j.tox.2007.01.014
ECHA (2008). Member State Committee Support Document for Identification of Bis(tributyltin)Oxide as a Substance of Very High Concern. Helsinki: European Chemicals Agency.
EFSA (2004). Opinion of the scientific panel on contaminants in the food chain on a request from the commission to assess the health risks to consumers associated with exposure to organotins in foodstuffs. EFSA J. 102, 1–119.
Eng, G., Bathersfield, O., and May, L. (1986). Mossbauer studies of the speciation of tributyltin compounds in seawater and sediment samples. Water Air Soil Pollut. 27, 191–197. doi: 10.1007/bf00464781
European Commission (1989). EU Council Directive 89/677/EEC of 21 December 1989 amending for the eighth time Directive 76/769/EEC on the approximation of the laws, regulations and administrative provisions of the member states relating to restrictions on the marketing and use of certain dangerous substances and preparations. Off. J. Eur. Union L398, 0019–0023.
European Union (2003). EU Regulation No 782/2003 of the European Parliament and of the Council of 14 April 2003 on the Prohibition of Organotin Compounds on Ships. Available at: http://eur-lex.europa.eu/LexUriServ/LexUriServ.do?uri=OJ:L:2003:115:0001:0011:EN:PDF (accessed October 11, 2019).
European Union (2005). Common Implementation Strategy for the Water Framework Directive: Environmental Quality Standards (EQS), Substance Data Sheet Priority Substance no 30: Tributyltin Compounds (TBT ion). Final Version. Brussels: European Union.
Evans, S. M., Evans, P. M., and Leksono, T. (1996). Widespread recovery of dogwhelks, Nucella lapillus (L) from tributyltin contamination in the North Sea and Clyde Sea. Mar. Pollut. Bull. 32, 263–269. doi: 10.1016/0025-326x(95)00127-9
Gadd, G. M. (2000). Microbial interactions with tributyltin compounds: detoxification, accumulation, and environmental fate. Sci. Total Environ. 258, 119–127. doi: 10.1016/s0048-9697(00)00512-x
Gibbs, P. E., Bryan, G. W., Pascoe, P. L., and Burt, G. R. (1987). The use of the dogwhelk, Nucella lapillus, as an indicator of tributyltin (TBT) contamination. J. Mar. Biol. Assoc. UK. 67, 507–523. doi: 10.1016/j.marpolbul.2015.08.038
Gibbs, P. E., Pascoe, P. L., and Burt, G. R. (1988). Sex change in the female dog-whelk, Nucella lapillus, induced by tributyltin from antifouling paints. J. Mar. Biol. Assoc. UK. 68, 715–731. doi: 10.1017/S0025315400028824
Hagger, J. A., Depledge, M. H., Oehlmann, J., Jobling, S., and Galloway, T. S. (2006). Is there a causal association between genotoxicity and the imposex effect? Environ. Health Perspect. 114, 20–26. doi: 10.1289/ehp.8048
Hall, L. W. Jr., Bushong, S. J., Hall, W. S., and Johnson, W. E. (1998). Acute chronic effects of tributyltin on a Chesapeake Bay copepod. Environ. Toxicol. Chem. 7, 41–46. doi: 10.1002/etc.5620070107
Hannas, B. R., Wang, Y. H., Thomson, S., Kwon, G., Li, H., and LeBlanc, G. A. (2010). Regulation and dysregulation of vitellogenin mRNA accumulation in daphnids (Daphnia magna). Aquat. Toxicol. 101, 351–357. doi: 10.1016/j.aquatox.2010.11.006
Hopkins, P. M. (2009). “Crustacean ecdysteroids and their receptors,” in Ecdysone: Structures and Functions, ed. G. Smagghe, (Houten: Springer Verlag), 73–97. doi: 10.1007/978-1-4020-9112-4_3
Hosamani, N., Reddy, S. B., and Reddy, R. P. (2017). Crustacean molting: regulation and effects of environmental toxicants. J. Mar. Sci. Res. Dev. 7:5. doi: 10.4172/2155-9910.1000236
Huang, Y., Zhu, L. Y., and Liu, G. X. (2006). The effects of bis(tributyltin) oxide on the development, reproduction and sex ratio of calanoid copepod Pseudodiaptomus marinus. Estuar. Coast. Shelf Sci. 69, 147–152. doi: 10.1016/j.ecss.2006.04.010
Huang, Y., Zhu, L. Y., Qiu, X. C., and Zhang, T. W. (2010). Effect of bis(tributyltin) oxide on reproduction and population growth rate of calanoid copepod Schmackeria poplesia. Chin. J. Oceanol. Limnol. 28, 280–287. doi: 10.1007/soo343-010-9002-9
Hunziker, R. W., Escher, B. I., and Schwarzenbach, R. P. (2001). pH dependence of the partitioning of triphenyltin and tributyltin between phosphatidylcholine liposomes and water. Environ. Sci. Toxicol. 35, 3899–3904. doi: 10.1021/es010043v
Hunziker, R. W., Escher, B. I., and Schwarzenbach, R. P. (2002). Acute toxicity of triorganotin compounds: different specific effects on the energy metabolism and role of pH. Environ. Toxicol. Chem. 21, 1191–1197. doi: 10.1002/etc.5620210611
ICES (1998). Report of the Working Group on Crangon Fisheries and Life History (WGCRAN) ICES CM 1998/G:8, Chap. ICES. Port-en-Bessin, 99.
ICES (2011). Report of the Working Group on Crangon Fisheries and Life History (WGCRAN) ICES CM 2011/SSGEF:11. Ijmuiden: ICES, 38.
ICES (2012). Report of the Working Group on Crangon Fisheries and Life History (WGCRAN) ICES CM 2012/SSGEF:09. Porto: ICES, 75.
ICES (2013). Report of the Working Group on Crangon Fisheries and Life History (WGCRAN) ICES CM 2013/SSGEF:12. Copenhagen: ICES HQ, 25.
ICES (2014). Report of the Working Group on Crangon Fisheries and Life History (WGCRAN) ICES CM 2014/SSGEF:08. Hamburg: ICES, 40.
ICES (2015a). Report of the Working Group for the Assessment of Demersal Stocks in the North Sea and Skagerrak (WGNSSK) ICES CM 2014/ACOM:13. Copenhagen: ICES HQ, 1493.
ICES (2015b). Report of the Working Group on Crangon Fisheries and Life History (WGCRAN) ICES CM 2015/SSGEPD:07. Ijmuiden: ICES, 58.
ICES (2016). Interim Report of the Working Group on Crangon Fisheries and Life History (WGCRAN) ICES CM 2016/SSGEPD:07. Oostende: ICES, 33.
ICES (2017). Interim Report of the Working Group on Crangon Fisheries and Life His-tory (WGCRAN) ICES CM 2017/SSGEPD:06. Hamburg: ICES, 37.
Ide, I., Witten, E. P., Fisher, J., Kalbfus, W., Zellner, A., Stroben, E., et al. (1997). Accumulation of organotin compounds in the common whelk Buccinum undatum and the red whelk Neptunea antiqua in association with imposex. Mar. Ecol. Prog. Ser. 152, 197–203. doi: 10.3354/meps152197
IMO resolution Mepc.195(61) (2010). Guidelines for Survey and Certification of Anti-fouling Systems on Ships. Available at: http://www.imo.org/blast/blastDataHelper.asp?data_id=29924&filename=195(61).pdf (accessed October 11, 2019).
IMO resolution Mepc.29(25) (1990). Amendments to the Annex of the Protocol of 1978 Relating to the International Convention for the Prevention of Pollution from Ships, 1973. Available at: http://www.imo.org/blast/blastDataHelper.asp?data_id=15603&filename=29(25).pdf (accessed October 11, 2019).
Jansen, S. (2002). Das Räuber-Beutesystem Juveniler Gadiden, Grundelnund Garnelen im Wattenmeer Nördlich Von Sylt. PhD Dissertation. University of Hamburg: Hamburg. 155.
Janssen, C., Vinckx, M., De Brabander, H., Roose, P., and Vethaak, D. (2007). Endocrine Disruption in the Scheldt Estuary Distribution. Exposure and effects (ENDIS-RISKS). D/2007/1191/45. Brussels: Belgian Science Policy.
Johansen, K., and Mohlenberg, F. (1987). Impairment of egg production in Acartia tonsa exposed to tributyltin oxide. Ophelia 27, 137–141. doi: 10.1080/00785236.1987.10422017
King, N., Miller, M., and de Mora, S. (1989). Tributyl tin levels for seawater, sediment, and selected species incoastal Northland and Auckland, New Zealand. N. Z. Mar. Fresh. 23, 287–294. doi: 10.1080/00288330.1989.9516365
Kirby, R., Beaugrand, G., and Lindley, J. A. (2009). Synergistic effects of climate change and fishing in a marine ecosystem. Ecosystems 12, 548–561. doi: 10.1007/s10021-009-9241-9
Kusk, K. O., and Petersen, S. (1997). Acute and chronic toxicity of tributyltin and linear alkylbenzene sulfonate to the marine copepod Acartia tonsa. Environ. Toxicol. Chem. 16, 1629–1633. doi: 10.1002/etc.5620160810
Lagadic, L., Katsiadaki, I., Biever, R., Guiney, P. D., Karouna-Renier, N., Schwarz, T., et al. (2017). “Tributyltin: advancing the science on assessing endocrine disruption with an unconventional endocrine-disrupting compound,” in Reviews of Environmental Contamination and Toxicology, Vol. 245, ed. P. De Voogt, (Cham: Springer), 65–127. doi: 10.1007/398_2017_8
Langston, W. J., and Pope, N. D. (1995). Determinants of TBT adsorption and desorption in estuarine sediments. Mar. Pollut. Bull. 31, 32–43. doi: 10.1016/0025-326x(95)91269-m
Langston, W. J., Pope, N. D., Davey, M., Langston, K. M., O’Hara, S. C. M., Gibbs, P. E., et al. (2015). Recovery from TBT pollution in english channel environments: a problem solved? Mar. Pollut. Bull. 95, 551–564. doi: 10.1016/j.marpolbul.2014.12.011
Laufer, H., Demir, N., Pan, X., Stuart, J. D., and Ahl, J. S. B. (2005). Methyl farnesoate controls adult male morphogenesis in the crayfish (Procambarus clarkia). J. Insect Physiol. 51, 379–384. doi: 10.1016/j.jinsphys.2005.02.007
Laughlin, R. B. Jr., and French, W. J. (1980). Comparative study of the acute toxicity of a homologous series of trialkyltins to larval shore crabs, Hemigrapsus nudus, and lobster, Homarus americanus. Bull. Environ. Contam. Toxicol. 25, 802–809. doi: 10.1007/bf01985611
Lee, C. C., Hsieh, C.-Y., and Tien, C.-J. (2006). Factors influencing organotin distribution in different marine environmental compartments, and their potential health risk. Chemosphere 65, 547–559. doi: 10.1016/j.chemosphere.2006.02.037
Luttikhuizen, P. C., Campos, J., van Bleijswijk, J., Peijnenburg, K. T. C. A., and van der Veer, H. W. (2008). Phylogeography of the common shrimp, Crangon crangon (L.) across its distribution range. Mol. Phylogenet. Evol. 46, 1015–1030. doi: 10.1016/j.ympev.2007.11.011
Ma, H. Z., Dai, S. G., and Huang, G. L. (2000). Distribution of tributyltin chloride in laboratory simulated estuarine microcosms. Water Res. 34, 2829–2841. doi: 10.1016/s0043-1354(00)00032-4
Massaro, E. J., Zucker, R. M., Elstein, K. H., Ting-Beall, H. P., and Easterling, R. E. (1989). Fixation of the plasma membrane/cytoplasm complex: a mechanism of toxic interaction of tributyltin. Biol. Trace Elem. Res. 21, 305–312. doi: 10.1007/bf02917268
Matthiessen, P. (2013). Detection, monitoring and control of tributyltin – An almost complete success story. Environ. Toxicol. Chem. 32, 487–489. doi: 10.1002/etc.2108
McCarty, L. S. (1991). “Toxicant body residues: implications for aquatic bioassays with some organic chemicals,” in Aquatic Toxicology and Risk Assessment. STP 1124, Vol. 14, eds M. A. Mayes, and M. G. Barron, (Philadelphia, PA: American Society for Testing and Materials), 183–192.
Meador, J. P. (1993). The effect of laboratory holding on the toxicity response of marine infaunal amphipods to cadmium and tributyltin. J. Exp. Mar. Biol. Ecol. 174, 227–242. doi: 10.1016/0022-0981(93)90019-k
Meador, J. P. (1997). Comparative toxicokinetics of tributyltin in five marine species and its utility in predicting bioaccumulation and toxicity. Aquat. Toxicol. 37, 307–326. doi: 10.1016/s0166-445x(96)00827-2
Meador, J. P. (2000). Predicting the fate and effects of tributyltin in marine systems. Rev. Environ. Contam. Toxicol. 166, 1–48.
Meador, J. P. (2011). Organotins in Aquatic Biota: Occurrence in Tissue and Toxicological Significance. Washington, DC: U.S. Department of Commerce, 552.
Mensink, B. P., ten Hallers-Tjabbes, C. C., Kralt, J., Freriks, I. L., and Boon, J. P. (1996a). Assessment of imposex in the common whelk, Buccinum undatum (L.) from the Eastern Scheldt, the Netherlands. Mar. Environ. Res. 41, 315–325. doi: 10.1016/0141-1136(95)00022-4
Mensink, B. P., van Hattum, B., Vethaak, A. D., and Boon, J. P. (1996b). The Development of Imposex in Relation to Organotin Contamination in the Common Whelk, Buccinum undatum. Field observations in the Eastern Sheldt and experimental exposure in a laboratory study. den Burg: Netherlands Institute for Sea Research (NIOZ).
Morton, B. (2009). Recovery from imposex by a population of the dog whelk, Nucella lapillus (Gastropoda: Caenogastropoda), on the southeastern coast of England since May 2004: a 52-month study. Mar. Pollut. Bull. 58, 1530–1538. doi: 10.1016/j.marpolbul.2009.05.012
Nagabhushanam, R., Reddy, P. S., and Sarojini, R. (1990). Tributyltin oxide induced alterations in exuvial weight and calcium content of the prawn, Caridina rajadhari. Proc. Anim. Sci. 99, 397–400. doi: 10.1007/bf03191873
Nicolaus, E. E. M., and Barry, J. (2015). Imposex in the dogwhelk (Nucella lapillus): 22 years monitoring around England and Wales. Environ. Monit. Assess. 187:736. doi: 10.1007/s10661-015-4961-0
Nishikawa, J., Mamiya, S., Kanayama, T., Nishikawa, T., Shiraishi, F., and Horiguchi, T. (2004). Involvement of the retinoid X receptor in the development of imposex caused by organotins in gastropods. Environ. Sci. Technol. 38, 6271–6276. doi: 10.1021/es049593u
Oberdörster, E., Rittschof, D., and LeBlanc, G. A. (1998). Alteration of [14C]-testosterone metabolism after chronic exposure of Daphnia magna to tributyltin. Arch. Environ. Contam. Toxicol. 34, 21–25. doi: 10.1007/s002449900281
Oehlmann, J., Bauer, B., Minchin, D., Schulte-Oehlmann, U., Fioroni, P., and Markert, B. (1998). Imposex in Nucella lapillus and intersex in Littorina littorea: interspecific comparison of two TBT-induced effects and their geographical uniformity. Hydrobiologia 378, 199–213. doi: 10.1007/978-94-011-5336-2_22
Oehlmann, J., Di Benedetto, P., Tilmann, M., Duft, M., Oetken, M., and Schulte-Oehlmann, U. (2007). Endocrine disruption in prosobranch molluscs: evidence and ecological relevance. Ecotoxicology 16, 29–43. doi: 10.1007/s10646-006-0109-x
Oehlmann, J., Stroben, E., and Fioroni, P. (1992). The rough tingle Ocenebra erinace (Gastropoda Murcidae): an exhibitor of imposex in comparison to Nucella lapillus. Helgoländer Meeresunters 46, 311–328. doi: 10.1007/bf02367102
Ohji, M., Arai, T., and Miyazaki, N. (2002). Effects of tributyltin exposure in the embryonic stage on sex ratio and survival rate in the caprellid amphipod Caprella danilevskii. MEPS 235, 171–176. doi: 10.3354/meps235171
Oliveira, I. B., Richardson, C. A., Sousa, A. C. A., Takahashi, S., Tanabe, S., and Barroso, C. M. (2009). Spatial and temporal evolution of imposex in dog whelk Nucella lapillus (L.) populations from North Wales, UK. J. Environ. Monit. 11, 1462–1468. doi: 10.1039/b906766c
OSPAR (2014). Imposex and TBT: Status, Trends and Effects in Marine Molluscs: an Improving Situation? London: OSPAR Commission.
OSPAR (2017a). Available at: https://oap.ospar.org/en/ospar-assessments/intermediate-assessment-2017/pressures-human-activities/contaminants/organotin-sediment/ (accessed October 11, 2019).
OSPAR (2017b). Available at: https://oap.ospar.org/en/ospar-assessments/intermediate-assessment-2017/pressures-human-activities/contaminants/imposex-gastropods/ (accessed October 11, 2019).
OSPAR Commission (2011). Background Document on Organic tin Compounds. London: OSPAR Commission, 33.
Pignatello, J. J., and Xing, B. (1996). Mechanisms of slow sorption of organic chemicals to natural particles. Environ. Sci. Technol. 30, 1–11. doi: 10.1021/es940683g
Pörtner, H. O., Boutilier, R. G., Tang, Y., and Toews, D. P. (1990). Determination of intracellular pH and PCO2 after metabolic inhibition by fluoride and nitrilotriacetic acid. Respir. Physiol. 81, 255–273. doi: 10.1016/0034-5687(90)90050-9
Rainbow, P. S. (1995). Biomonitoring of heavy metal availability in the marine environment. Mar. Pollut. Bull. 31, 183–192. doi: 10.1016/0025-326X(95)00116-5
Reddy, P. R., Nagaraju, G. P. C., and Reddy, P. S. (2004). Involvement of methyl farnesoate in the regulation of molting and reproduction in the freshwater crab Oziotelphusa senex senex. J. Crust. Biol. 24, 511–515. doi: 10.1651/C-2478
Reddy, P. S., Nagabhushanam, R., and Sarojini, R. (1992). Retardation of moulting in the prawn, Caridina rajadhari, exposed to tributyltin oxide (TBTO). Proc. Nat. Acad. Sci. India 62, 353–356.
Reddy, P. S., Sarojini, R., and Nagabhushanam, R. (1991). Impact of tributyltin oxide (TBTO) on limb regeneration of the prawn, Caridina rajadhari, after exposure to different time intervals of amputation. J. Tiss. Res. 1, 35–39.
Rice, S. D., Short, J. W., and Stickle, W. B. (1989). Uptake and catabolism of tributyltin by blue crabs fed TBT contaminated prey. Mar. Environ. Res. 27, 137–145. doi: 10.1016/0141-1136(89)90005-6
Rodríguez, J. G., Tueros, I., Borja, A., Franco, J., García Alonso, J. I., Garmendia, J. M., et al. (2009). Butyltin compounds, sterility and imposex assessment in Nassarius reticulates (Linneaus, 1758), prior to the 2008 European ban on TBT antifouling paints, within Basque ports and coastal areas. Cont. Shelf Res. 29, 1165–1173. doi: 10.1016/j.csr.2009.01.005
Schmidt, K., Steinberg, C. E. W., Staaks, G., and Pflugmacher, S. (2005). Influence of a xenobiotic mixture (PCB and TBT) compared to single substances on swimming behavior or reproduction of Daphnia magna. Acta Hydrochim. Hydrobiol. 33, 287–300. doi: 10.1002/aheh.200400579
Schøyen, M., Green, N. W., Hjermann, D. Ø., Tveiten, L., Beylich, B., Øxnevad, S., et al. (2018). Levels and trends of tributyltin (TBT) and imposex in dogwhelk (Nucella lapillus) along the Norwegian coastline from 1991 to 2017. Mar. Environ. Res. 144, 1–8. doi: 10.1016/j.marenvres.2018.11.011
Siegel, V., Damm, U., and Neudecker, T. (2008). Sex-ratio, seasonality and long-term variation in maturation and spawning of the brown shrimp Crangon crangon (L.) in the German Bight (North Sea). Helgoland Mar. Res. 62, 339–349. doi: 10.1007/s10152-008-0121-z
Siegel, V., Gröger, J., Neudecker, T., Damm, U., and Jansen, S. (2005). Longterm variation in the abundance of the brown shrimp Crangon crangon (L.) population of the German Bight and possible causes for its interannual variability. Fish Oceanogr. 14, 1–16. doi: 10.1111/j.1365-2419.2004.00301.x
Smith, B. S. (1981). Tributyltin compounds induce male characteristics on female mud snails Nassarius obsoletus = Ilyanassa obsolete. J. Appl. Toxicol. 1, 141–144. doi: 10.1002/jat.2550010302
Sousa, A. C. A., Barroso, C. M., Tanabe, S., and Horiguchi, T. (2010). “Involvement of retinoid X receptor in imposex development in Nucella lapillus and Nassarius reticulatus - Preliminary results,” in Interdisciplinary Studies on Environmental Chemistry - Biological Responses to Contaminants, eds N. Hamamura, S. Suzuki, S. Mendo, C. M. Barroso, H. Iwata, and S. Tanabe, (Tokyo: Terrapub), 189–196.
Sousa, A. C. A., Laranjeiro, F., Takahashi, S., Tanabe, S., and Barroso, C. M. (2009). Imposex and organotin prevalence in a European post-legislative scenario: temporal trends from 2003 to 2008. Chemosphere 77, 566–573. doi: 10.1016/j.chemosphere.2009.06.049
Sternberg, R. M., Gooding, M. P., Hotchkiss, A. K., and LeBlanc, G. A. (2010). Environmental-endocrine control of reproductive maturation in gastropods: implications for the mechanism of tributyltin induced imposex in prosobranchs. Ecotoxicology 19, 4–23. doi: 10.1007/s10646-009-0397-z
Strand, J., and Jacobsen, J. A. (2005). Accumulation and trophic transfer of organotins in a marine food web from the Danish coastal waters. Sci. Total Environ. 350, 72–85. doi: 10.1016/j.scitotenv.2005.02.039
Stroben, E. (1994). lmposex Und Weitere Effekte Von Chronischer TBT-Intoxikation bei Einigen Mesogastropoden und Bucciniden (Gastropoda, Prosobranchia). Göttingen: Cuvillier.
Stronckhorst, J., and van Hattum, B. (2003). Contaminants of concern in Dutch marine harbor sediments. Arch. Environ. Contam. Toxicol. 45, 306–316.
Sunday, A. O., Alafara, B. A., and Oladele, O. G. (2012). Toxicity and speciation analysis of organotin compounds. Chem. Spec. Bioavailab. 24, 216–226. doi: 10.3184/095422912X13491962881734
Takahashi, S., Tanabe, S., Takeuchi, I., and Miyazaki, N. (1999). Distribution and specific bioaccumulation of butyltin compounds in a marine ecosystem. Arch. Environ. Contam. Toxicol. 37, 50–61. doi: 10.1007/s002449900489
Tanabe, S. (1999). Butyltin contamination in marine mammals, a review. Mar. Pollut. Bull. 39, 62–72. doi: 10.1016/s0025-326x(99)00064-8
Temming, A. (1995). Die quantitative Bestimmung der Konsumtion von Fischen. Experimentelle, Methodische und theoretische Aspekte. Hamburg: Universität Hamburg.
Temming, A., and Hufnagl, M. (2014). Decreasing predation levels and increasing landings challenge the paradigm of non-management of North Sea brown shrimp (Crangon crangon). ICES J. Mar. Sci. 72, 804–823. doi: 10.1093/icesjms/fsu194
ten Hallers-Tjabbes, C. C., Mensink, B. P., van Hattum, B., and Boon, J. P. (1996). “Impact of traditional antifouling in open sea, risk and ecological consequences,” in Proceedings of the International Sysmposium on Directorate-General for Shipping and Marine Affairs and the Organotin Environmental Programme Association, (The Hague), 36–42.
Tiews, K. (1961). The role of whiting as an undesirable guest in German coastal waters. ICES Near Northern Seas Committee 28, 137–143.
Tsuda, T., Nakanishi, H., Aoki, S., and Takebayashi, J. (1986). Bioconcentration of butyltin compounds by round crucian carp. Toxicol. Environ. Chem. 12, 137–143. doi: 10.1080/02772248609357154
Tsuda, T. S., Aoki, S., Kojima, M., and Harada, H. (1990). The influence of pH on the accumulation of tri-n-butyltin chloride and triphenyltin chloride in carp. Comp. Biochem. Physiol. 95C, 151–153. doi: 10.1016/0742-8413(90)90096-r
Unger, M. A., MacIntyre, W. G., and Huggett, R. J. (1988). Sorption behaviour of tributyltin on estuarine and freshwater sediments. Environ. Toxicol. Chem. 7, 907–915. doi: 10.1002/etc.5620071107
United States Environmental Protection Agency [EPA. US], (1985). Office of Pesticide Programs. (1985). Tributyltin Support Document. Washington, D.C: United States Environmental Protection Agency.
United States Environmental Protection Agency [EPA.US], (2007). Ambient Aquatic Life Water Quality Criteria for Tributyltin (TBT). Washington, D.C: United States Environmental Protection Agency.
van Wezel, A. P., and Opperhuizen, A. (1995). Narcosis due to environmental pollutants in aquatic organisms: residue-based toxicity, mechanisms, and membrane burdens. Crit. Rev. Toxicol. 25, 255–279. doi: 10.3109/10408449509089890
Veltman, K., Huijbregts, M. A. J., van den Heuvel-Greve, M. J., Vethaak, A. D., and Hendriks, A. J. (2006). Organotin accumulation in an estuarine food chain: comparing field measurements with model estimations. Mar. Environ. Res. 61, 511–530. doi: 10.1016/j.marenvres.2006.02.005
Verhaegen, Y. (2012). Mechanism, Concentrations and Effects of Tributyltin in Common Shrimp Crangon crangon. PhD. Dissertation. Ghent University: Ghent.
Verhaegen, Y., Monteyne, E., Neudecker, T., Tulp, I., Smagghe, G., Cooreman, K., et al. (2012). Organotins in North Sea brown shrimp (Crangon crangon L.) after implementation of the TBT ban. Chemosphere 86, 979–984. doi: 10.1016/j.chemosphere.2011.11.028
Verhaegen, Y., Parmentier, K., Swevers, L., Renders, E., Rougé, P., De Coen, W., et al. (2011). The heterodimeric ecdysteroid receptor complex in the brown shrimp Crangon crangon: EcR and RXR isoform characteristics and sensitivity towards the marine pollutant tributyltin. Gen. Comp. Endocrinol. 172, 158–169. doi: 10.1016/j.ygcen.2011.02.019
Verhaegen, Y., Parmentier, K., Swevers, L., Rougé, P., Soin, T., De Coen, W., et al. (2010). The brown shrimp (Crangon crangon L.) ecdysteroid receptor complex: cloning, structural modeling of the ligand-binding domain and functional expression in an EcR-deficient Drosophila cell line. Gen. Comp. Endocrinol. 168, 415–423. doi: 10.1016/j.ygcen.2010.05.007
Waldock, M. J., Waite, M. E., and Thain, J. E. (1988). Inputs of TBT to the marine environment from shipping activity in the UK. Environ. Technol. Lett. 9, 999–1010. doi: 10.1080/09593338809384662
Wang, Y. H., Kwon, G., Li, H., and LeBlanc, G. A. (2011). Tributyltin synergizes with 20-hydroxyecdysone to produce endocrine toxicity. Toxicol. Sci. 123, 71–79. doi: 10.1093/toxsci/kfr154
Wang, Y. H., and LeBlanc, G. A. (2009). Interactions of methyl farnesoate and related compounds with a crustacean retinoid X receptor. Mol. Cell. Endocrinol. 309, 109–116. doi: 10.1016/j.mce.2009.05.016
Watermann, B., and Dethlefsen, V. (1983). Black spot disease of shrimps - an environmental problem, a disease or wounds. Fischwirtsch 30, 24–29.
Welleman, H. C., and Daan, N. (2001). Is the Dutch Shrimp Fishery Sustainable? Senckenbergiana Maritima 31, 321–328. doi: 10.1007/bf03043040
Wetzel, M. A., Winterscheid, A., and Wahrendorf, D.-S. (2013). Baseline of the butyltin distribution in surface sediments (0 – 20cm) of the Elbe estuary (Germany, 2011). Mar. Pollut. Bull. 77, 418–423. doi: 10.1016/j.marpolbul.2013.09.021
Willemsen, F., Wegener, J.-W., Morabito, R., and Pannier, F. (2004). Sources, Consumer Exposure and Risks of Organotin Contamination in Seafood. Final Report of the European Commission, 2004. Research Project OT-SAFE (QLK1-2001-01437).
Wilson, J. G., Minchin, D., McHugh, B., McGovern, E., Tanner, C. J., and Giltrap, M. (2015). Declines in TBT contamination in Irish coastal waters 1987 – 2011, using the dogwhelk (Nucella lapillus) as a biological indicator. Mar. Pollut. Bull. 100, 289–296. doi: 10.1016/j.marpolbul.2015.08.038
Wolf, G. (2006). Is 9-cis-retinoic acid the endogenous ligand for the retinoic acid-X receptor? Nutr. Rev. 64, 532–538. doi: 10.1111/j.1753-4887.2006.tb00186.x
Wu, X., Hopkins, P. M., Palli, S. R., and Durica, D. S. (2004). Crustacean retinoid-X receptor isoforms: distinctive DNA binding and receptor-receptor interaction with a cognate ecdysteroid receptor. Mol. Cell. Endocrinol. 218, 21–38. doi: 10.1016/j.mce.2003.12.013
Keywords: tributyltin, Crangon crangon, endocrine disruption, ecosystem impact, ecdysteroids, ecdysteroid receptor
Citation: Parmentier KFV, Verhaegen Y, De Witte BP, Hoffman S, Delbare DHR, Roose PM, Hylland KDE, Burgeot T, Smagghe GJ and Cooreman K (2019) Tributyltin: A Bottom–Up Regulator of the Crangon crangon Population? Front. Mar. Sci. 6:633. doi: 10.3389/fmars.2019.00633
Received: 10 May 2019; Accepted: 26 September 2019;
Published: 15 October 2019.
Edited by:
Julian Blasco, Spanish National Research Council (CSIC), SpainReviewed by:
Piero Giulio Giulianini, University of Trieste, ItalySumonto Mitra, Karolinska Institute (KI), Sweden
Copyright © 2019 Parmentier, Verhaegen, De Witte, Hoffman, Delbare, Roose, Hylland, Burgeot, Smagghe and Cooreman. This is an open-access article distributed under the terms of the Creative Commons Attribution License (CC BY). The use, distribution or reproduction in other forums is permitted, provided the original author(s) and the copyright owner(s) are credited and that the original publication in this journal is cited, in accordance with accepted academic practice. No use, distribution or reproduction is permitted which does not comply with these terms.
*Correspondence: Kris Cooreman, a3Jpcy5jb29yZW1hbkBpbHZvLnZsYWFuZGVyZW4uYmU=