- 1Centre for Marine Science and Technology, Curtin University, Perth, WA, Australia
- 2German Oceanographic Museum, Stralsund, Germany
- 3Sea Mammal Research Unit, Scottish Oceans Institute, University of St Andrews, St Andrews, United Kingdom
- 4German Environment Agency, Dessau-Roßlau, Germany
- 5National Marine Mammal Foundation, San Diego, CA, United States
- 6Meereszoologie, Nehmten, Germany
- 7International Fund for Animal Welfare, London, United Kingdom
- 8Australian Marine Mammal Centre, Australian Antarctic Division, Kingston, TAS, Australia
- 9NOAA Fisheries, Office of Protected Resources, Silver Spring, MD, United States
- 10DW-ShipConsult GmbH, Schwentinental, Germany
- 11Ocean Acoustics Lab, Alfred Wegener Institute (AWI), Helmholtz Centre for Polar and Marine Research, Bremerhaven, Germany
- 12Helmholtz Institute for Functional Marine Biodiversity (HIFMB), Carl von Ossietzky University of Oldenburg, Oldenburg, Germany
The Protocol on Environmental Protection of the Antarctic Treaty stipulates that the protection of the Antarctic environment and associated ecosystems be fundamentally considered in the planning and conducting of all activities in the Antarctic Treaty area. One of the key pollutants created by human activities in the Antarctic is noise, which is primarily caused by ship traffic (from tourism, fisheries, and research), but also by geophysical research (e.g., seismic surveys) and by research station support activities (including construction). Arguably, amongst the species most vulnerable to noise are marine mammals since they specialize in using sound for communication, navigation and foraging, and therefore have evolved the highest auditory sensitivity among marine organisms. Reported effects of noise on marine mammals in lower-latitude oceans include stress, behavioral changes such as avoidance, auditory masking, hearing threshold shifts, and—in extreme cases—death. Eight mysticete species, 10 odontocete species, and six pinniped species occur south of 60°S (i.e., in the Southern or Antarctic Ocean). For many of these, the Southern Ocean is a key area for foraging and reproduction. Yet, little is known about how these species are affected by noise. We review the current prevalence of anthropogenic noise and the distribution of marine mammals in the Southern Ocean, and the current research gaps that prevent us from accurately assessing noise impacts on Antarctic marine mammals. A questionnaire given to 29 international experts on marine mammals revealed a variety of research needs. Those that received the highest rankings were (1) improved data on abundance and distribution of Antarctic marine mammals, (2) hearing data for Antarctic marine mammals, in particular a mysticete audiogram, and (3) an assessment of the effectiveness of various noise mitigation options. The management need with the highest score was a refinement of noise exposure criteria. Environmental evaluations are a requirement before conducting activities in the Antarctic. Because of a lack of scientific data on impacts, requirements and noise thresholds often vary between countries that conduct these evaluations, leading to different standards across countries. Addressing the identified research needs will help to implement informed and reasonable thresholds for noise production in the Antarctic and help to protect the Antarctic environment.
Introduction
The Antarctic Treaty was established for the protection of the Antarctic, allowing scientific research but prohibiting military activity. It entered into force in 1961 and has since been signed by 53 Parties. Its Protocol on Environmental Protection (the Protocol) entered into force in 1998, stipulating that the protection of the Antarctic environment and associated ecosystems be fundamentally considered in the planning and conducting of all activities in the Antarctic Treaty area (area south of 60°S, i.e., approximately south of the Antarctic Convergence, including all ice shelves). While fishing was deemed allowable by the Convention on the Conservation of Antarctic Marine Living Resources (CCAMLR) in 1982, the Protocol prohibits all activities relating to Antarctic mineral and hydrocarbon resources, except for scientific research.
Parties implement the Protocol via national acts and laws. For example, in Germany, the Act Implementing the Protocol on Environmental Protection to the Antarctic Treaty (AIEP, 1998) identifies the German Environment Agency (Umweltbundesamt, UBA) as the competent authority for assessing and permitting German activities in the Antarctic. The AIEP and the Protocol protect native animals at individual and population levels. Activities that molest, handle, capture, injure or kill a native mammal or bird are prohibited (Annex II to the Protocol). However, exceptions can be granted for scientific or educational purposes. A permit cannot be issued if the activity is suspected to cause (a) harmful changes to the distribution, abundance or productivity of an animal species or its populations, (b) threats to endangered species or populations, or (c) significant detrimental effects on the environment and associated ecosystems. Any scientific research that is deemed by UBA to have the potential to create at least a minor or transitory impact is also evaluated by an independent committee of scientific experts (Sachverständigenkommission Antarktis, SV-KOM).
Underwater noise is part of almost all anthropogenic activities in the Antarctic, ranging from ship traffic to construction and scientific seismic surveys (Figure 1). Such noise can have profound effects on marine organisms and has been identified as a major stressor in the marine environment (see the collection of articles covering a diversity of species in Popper and Hawkins, 2016). Yet, no specific guidelines for noise production in the Antarctic have been established and noise has only once been considered at the Meetings of the Committee for Environmental Protection (CEP) since 2012.1 The CEP normally meets once a year in conjunction with the Antarctic Treaty Consultative Meeting (ATCM) and (a) addresses matters relating to environmental protection and management, (b) provides advice to the ATCM, and (c) formulates measures or resolutions in furtherance of the principles and objectives of the Treaty for the adoption through the ATCM. The Scientific Committee on Antarctic Research (SCAR) is an inter-disciplinary committee of the International Science Council (ISC) and provides scientific advice to the Parties at the ATCM.
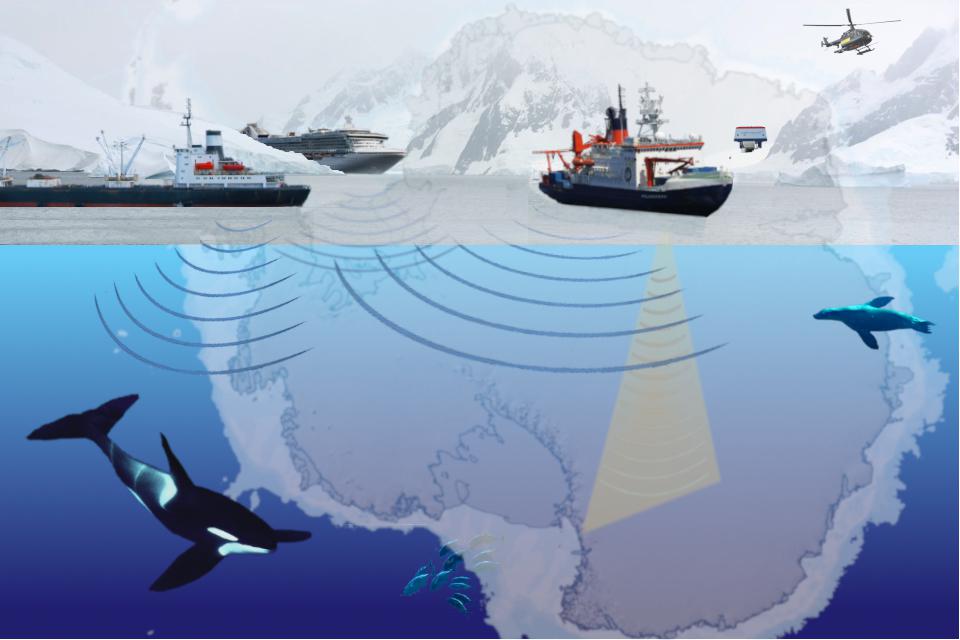
Figure 1. Sketch of sources of underwater noise in the Antarctic. All vessels (fishing vessels, cruise ships, research vessels, etc.) produce underwater noise in a nearly omni-directional pattern (indicated by circular sound wavefronts). Ships use echosounders that scan the sea floor with a narrow swatch of sound (indicated in yellow). Research station infrastructure and support includes construction activities, vessels as well as aircraft—all of which may be detected under water.
Arguably, amongst the species most vulnerable to noise are marine mammals since they specialize in using sound for communication, navigation and foraging, and therefore have evolved sensitive auditory systems (Au et al., 2000). The effects of ship noise on marine mammals have recently been reviewed (Erbe et al., 2019). Knowledge about the effects of noise on marine mammals is mostly based on studies from regions other than the Southern Ocean. Documented effects include potential increases in stress (Rolland et al., 2012), behavioral changes such as short- and long-term avoidance of affected areas (Nowacek et al., 2007; Götz and Janik, 2013), auditory masking (Erbe et al., 2016), hearing threshold shifts (Finneran, 2015), and—in extreme cases—death (Schrope, 2002). Studies conducted outside of the Antarctic have shown that reactions to noise differ widely between marine mammal species (Ellison et al., 2012).
The Southern Ocean is in many ways not comparable to other ocean basins. In terms of biodiversity, the Antarctic is home to a range of marine species that cannot be found elsewhere on the globe. Some species are year-round residents of Antarctic waters, such as the ice-breeding pinniped species. Other species migrate to the Antarctic annually to forage. In fact, the Antarctic is of critical importance to migrating mysticete whales, which come here during the austral summer for feeding. During this time, they take in a large proportion (possibly up to 80%) of their annual energy requirements and store substantial amounts of lipids (some grow their body weight by 30–100%; Brodie, 1975; Lockyer, 1981; Reilly et al., 2004). In terms of acoustics, the marine soundscape of the Southern Ocean is a unique combination of sounds from Antarctic fauna, weather events and ice (plus anthropogenic sounds). Underwater sound propagation is strongly influenced by the low water temperature and ice cover around the Antarctic continent. Thus, we set out to determine the current state of knowledge on the effects of underwater noise on marine mammals in the Antarctic, to identify knowledge gaps, and to discuss research needs.
Marine Mammals in the Antarctic
Eight mysticete species (and subspecies), 10 odontocete species, and six pinniped species have been observed south of 60°S (Table 1). Out of these, the Antarctic blue whale is listed by the International Union for Conservation of Nature and Natural Resources (IUCN; iucnredlist.org) as “critically endangered,” the pygmy blue whale, fin whale, and sei whale are listed as “endangered,” the Antarctic minke whale is “near threatened,” and the sperm whale is “vulnerable.” Arnoux’s, Gray’s, and strap-toothed beaked whales, as well as the killer whale are data deficient; so their conservation status cannot be determined. Other Antarctic marine mammals are currently listed as “least concern.”
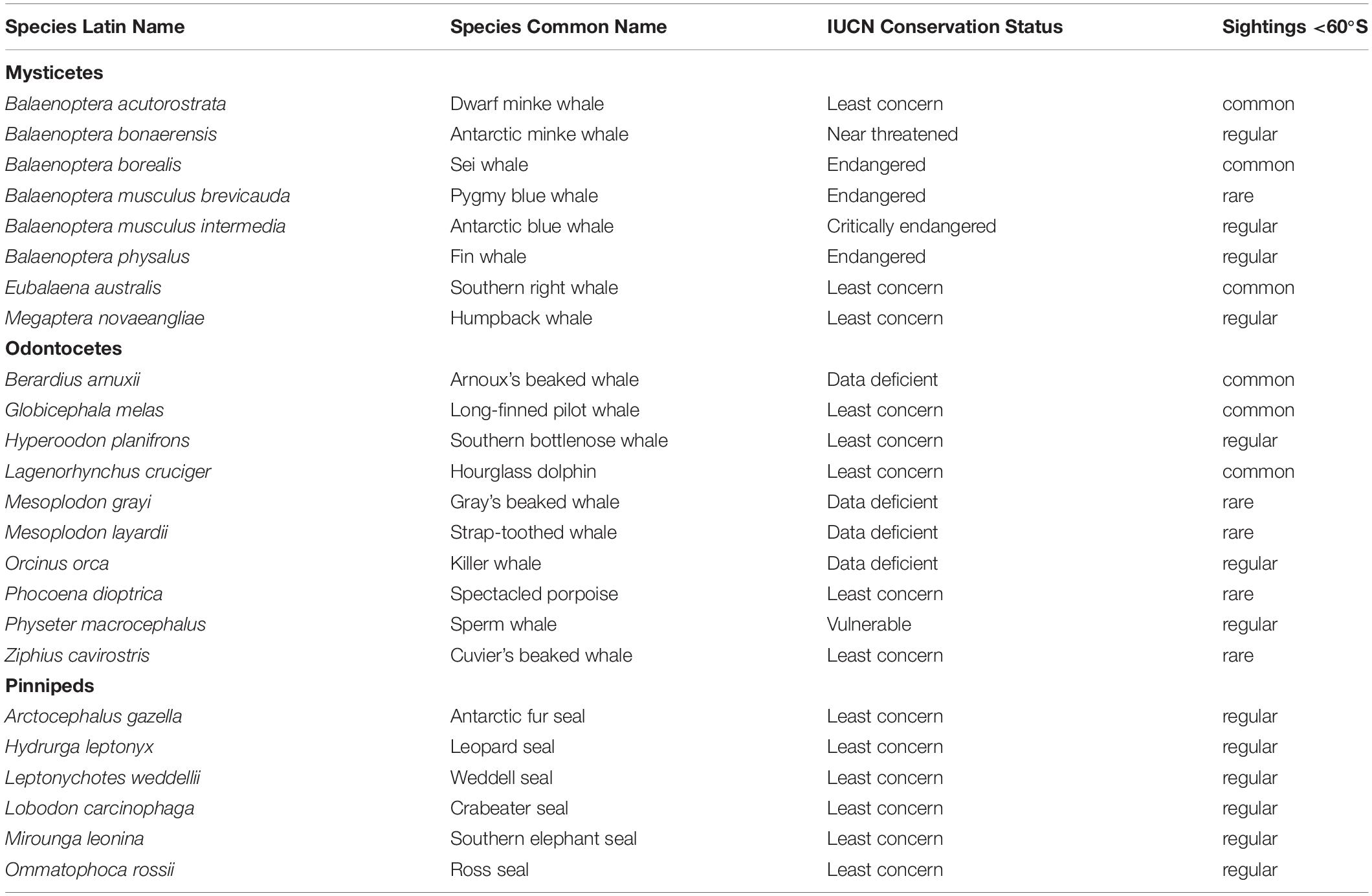
Table 1. Marine mammal species in the Antarctic, based on Ropert-Coudert et al. (2014).
With regard to the application and interpretation of the legal regulations relating to the Antarctic Treaty area, it is important to ascertain which marine species are relevant: The Environmental Protocol protects individual members of “native” mammal species and also protects the populations of all animal species, including sporadically occurring species. In this context, the word “native,” which is used in the Environmental Protocol, has the same meaning as the notion of “true” Antarctic species, as defined in Boyd et al. (2002): “those species whose populations rely on the Southern Ocean as a habitat, i.e., critical to a part of their life history, either through the provision of habitat for breeding or through the provision of the major source of food.” For the Protocol, however, the “native” criterion is only applied to individual members of a species. With regard to populations, protection is extended to both native and non-native animal species, including those that occur only rarely, such as Phocoena dioptrica.
Information on distribution and abundance of Antarctic marine mammals is mostly scarce, although annual surveys were conducted as part of the International Whaling Commission (IWC) circumpolar IDCR/SOWER programs between 1978/79 and 2003/04. These programs surveyed a sector of roughly 60° longitude each year, from the ice edge to 60°S, generating abundance estimates for a number of species including the Antarctic blue whale (Branch, 2007), humpback whale (Branch, 2011), and Antarctic minke whale (IWC, 2013). These and most other visual surveys have been generally confined to ice-free areas and undertaken during the brief austral summer. Information on migrations, spatial distribution, and abundance in ice-covered areas (e.g., Herr et al., 2019) or during other times of the year is limited though growing—for example, as a result of autonomous passive acoustic monitoring, which can collect information on acoustic presence year-round (e.g., Van Opzeeland et al., 2008; Van Parijs et al., 2009). Field research in the Antarctic is expensive and limited in space and time, resulting in numerous data gaps (Table 2).
The available information indicates that blue, fin, humpback, and minke whales are found all the way to the ice edge throughout the austral summer season, with the peak of fin and humpback whale encounters tending to be further away from the ice edge than the highest densities of Antarctic blue and Antarctic minke whales (e.g., Tynan, 1998; Williams et al., 2014b). Passive acoustic observations have shown that Antarctic blue, Antarctic minke, and humpback whale distributions are, however, not limited by ice (van Opzeeland et al., 2013; Dominello and Širović, 2016; Thomisch et al., 2016). Observations of Antarctic minke whales show this species predominantly occurs in areas with dense ice cover (Williams et al., 2014b; Herr et al., 2019). Fin whales are acoustically present year-round in some areas (E. Burkhardt pers. comm.), although in other areas they seem to avoid ice cover (Sirovic et al., 2004; Herr et al., 2016). Sei and southern right whales are typically not encountered at the ice edge (Kasamatsu et al., 1996; Best, 2007). Killer whales occurring in Antarctic waters comprise four different ecotypes, which all occur beyond the ice edge in pack-ice areas (see de Bruyn et al., 2013 for a review). Southern bottlenose and Arnoux’s beaked whales occur in open water south of 60°S up to the ice edge. Arnoux’s beaked whales have furthermore during summer been observed to occur in pack-ice areas (Best, 2007). Sperm whales, other beaked whales, and the smaller odontocetes are found further away from the ice edge (Kasamatsu and Joyce, 1995). The Antarctic fur seal and the southern elephant seal are ice-tolerant, but open-water species, that generally depend on land for breeding (Boyd et al., 1998; Bornemann et al., 2004; Hindell et al., 2016). The crabeater, leopard, Ross, and Weddell seals also have pelagic phases, but are bound to the presence of sea-ice for breeding and molt, with each species exhibiting different sea-ice habitat requirements (Ropert-Coudert et al., 2014; Siniff, 2015). Distribution maps for marine mammals occurring around Antarctica are shown in the Biogeographic Atlas of the Southern Ocean (Ropert-Coudert et al., 2014).
Reported encounter rates for mysticetes and odontocetes peak in January and February (Kasamatsu and Joyce, 1995; Kasamatsu et al., 1996). Many mysticetes migrate to the Antarctic in the austral summer to feed before they migrate to warmer waters where they breed in the austral winter (Lowther, 2018). There is increasing evidence of mysticete presence in the Antarctic throughout the austral winter from passive acoustic recordings (Sirovic et al., 2009; van Opzeeland et al., 2013; Thomisch et al., 2016). Of odontocetes, some killer whale ecotypes are resident in the Antarctic all year-round (Pitman and Ensor, 2003). Of sperm whales, only males venture this far south and stay over the winter (Kasamatsu and Joyce, 1995). Winter surveys indicate that 20 or more cetacean species have regular, potentially year-round presence in the Antarctic (Thiele and Gill, 1999; Thiele et al., 2004; van Waerebeek et al., 2010). Amongst pinnipeds, elephant seals and Antarctic fur seals forage in the Antarctic in the austral winter, but breed on subantarctic islands like the Kerguelen Islands, Macquarie Island, or South Georgia during the summer (Boyd et al., 2002; Rodríguez et al., 2017). All other Antarctic seal species are ice breeding and are resident in Antarctic waters south of the Antarctic Convergence year-round. However, some of these species, in particular leopard seals, can also be found on subantarctic islands (Lowther, 2018).
Information on the diet of some Antarctic marine mammals is scarce, though data are available for some whale species from whaling records, and for other species diet can be inferred from the same or related species in other geographic regions (see Pauly et al., 1998 for an overview). The mysticetes feed primarily on krill, but may also take small fish, zooplankton, and possibly squid. The odontocetes eat fish and squid, with certain killer whale ecotypes also hunting penguins and other marine mammals (both cetaceans and pinnipeds). Antarctic pinnipeds forage on krill, fish, zooplankton, and squid, with leopard seals also taking other seals and seabirds. Lowther (2018) provides a recent summary of the diets of Antarctic marine mammals.
Given the potential for anthropogenic activities occurring in Antarctic waters to affect critical life functions of marine mammals, it is imperative for environmental impact assessments to consider impacts on the acoustic habitat of marine mammals. Marine mammals actively and passively use sound in support of their various life functions, as do at least some of their prey species. Sound plays a role in marine mammal behavioral contexts, comprising social encounters, feeding, mother-offspring recognition, and mating (van Opzeeland et al., 2010; Janik, 2014; Reichmuth and Casey, 2014). Odontocetes use active biosonar for navigating and foraging (Au, 1993). All marine mammals likely listen to environmental sounds, as well as the sounds of predators and prey (Gannon et al., 2005; Janik, 2005). Interfering with sound usage and sensing while marine mammals undergo critical life functions in the Antarctic can affect individuals and possibly populations in the Antarctic and beyond.
Underwater Antarctic Noise
Ambient noise in the Antarctic can be of abiotic, biotic, and anthropogenic origin. Wind over open ocean leads to the entrainment of bubbles, which produce a broad spectrum of sound (Knudsen et al., 1948). Wind blowing over ice produces a different spectrum of sound. Wind and currents move ice flows and push icebergs together or against the seabed, resulting in distinct rubbing and cracking sounds, with the former being quite tonal in character (e.g., Gavrilov and Li, 2007). Temperature changes lead to ice cracking, which is typically impulsive and broadband.
Polar waters can be both noisier and quieter than the open ocean. The ice edge typically is an active acoustic zone with high sound levels due to ice breaking, colliding, and shearing (Haver et al., 2017). Conversely, it is quieter under the ice fields during stable conditions (Mikhalevsky, 2001). Marine mammals, fish, and crustaceans produce sound, often prolifically, resulting in continuous choruses in characteristic frequency bands. A multi-year recording at 0°E, 66°S was analyzed to present a statistical analysis of biotic and abiotic ambient noise, as a function of wind speed and ice cover, showing that whale and seal choruses generated distinct peaks in the ambient noise spectra (e.g., Antarctic blue whale chorus at 15–30 Hz, fin whales at 95–105 Hz, minke whales at 90–200 Hz, and leopard seals at 320–350 Hz; Menze et al., 2017).
Anthropogenic underwater noise in the Southern Ocean originates from ships—mostly research vessels, cruise ships, and fishing vessels. During the 2017/2018 austral summer, 98 research stations and 51 research or research support ships were registered with the Council of Managers of National Antarctic Programs (COMNAP)2, 53 tourism ships were registered with the International Association of Antarctica Tour Operators (IAATO)3, and 46 fishing vessels reported to the Commission for the Conservation of Antarctic Marine Living Resources (CCAMLR)4.
The latest season where data from COMNAP, IAATO, and CCAMLR were available for a more detailed analysis was the austral summer of 2016/2017. In terms of the cumulative amount of time spent by these types of vessels, IAATO tourist vessels contributed 3,200 ship-days,5 CCAMLR fishing vessels contributed 1,400 ship-days6, and COMNAP research vessels contributed 1,100 ship-days7 in the 2016/2017 season. In terms of the total number of people carried into the Antarctic during the 2016/2017 season, cruise ships (73,400 people incl. staff and crew) surpassed research vessels (3,300 people incl. crew) and fishing vessels (2,100 people). In terms of person-days (i.e., the cumulative sum of the number of persons multiplied by the time each spends), research (797,000 person-days at fixed stations plus approximately 100,000 person-days on COMNAP ships) outweighed tourism (730,000 person-days) and fisheries (63,000 person-days).
Since 2015, CCAMLR has required an automated vessel monitoring system (VMS) for all fishing vessels (Commission for the Conservation of Antarctic Marine Living Resources, 2015). While detailed positions of the CCAMLR fishing fleet have been collected since 2015, they are treated as commercially confidential information by the CCAMLR secretariat; so positions for each nation’s vessels are only disclosed to the appropriate authority for that contracting nation. Thus, only an aggregate list of vessels licensed by CCAMLR for fishing in the Antarctic is generally available, rather than their precise locations and tracks (Commission for the Conservation of Antarctic Marine Living Resources, 2018). Automatic Identification System (AIS) data are also available for some regions and times. Vessels from nations outside of the Antarctic Treaty, CCAMLR, and IAATO are missing from the corresponding databases; however, it is unlikely that there are a substantial number of such ships. Most private yachts do not report either. An extrapolated number of 95 non-IAATO yachts compared to 18 IAATO-yachts8 entered Antarctic waters in the 2017/2018 austral summer.9
Antarctic tourism has increased since the 1950s (Enzenbacher, 1992). Cruise ships are present from October through March, peaking in January. While the number of operators, number of ships, number of voyages and number of passengers increased between 1992/1993 and 2018/2019, the number of operators and ships has leveled off; yet the number of voyages and passengers keeps rising (Bender et al., 2016; International Association of Antarctica Tour Operators [IAATO], 2018; Figure 2). Research vessels are present all year-round, peaking in January and February. The number of research vessels south of 60°S has doubled from about 12 in 2011/2012 to 25 in 2016/2017.10 The number of licensed fishing vessels (46 in 2017/2018), the number of licensing periods (52 in 2017/2018), and the number of licensed areas (119 in 2017/2018) have remained fairly constant from 2011/2012 to 2017/201811. Ships are not evenly distributed. Rather, the Antarctic Peninsula and the Ross Sea experience the most ship traffic of all types.
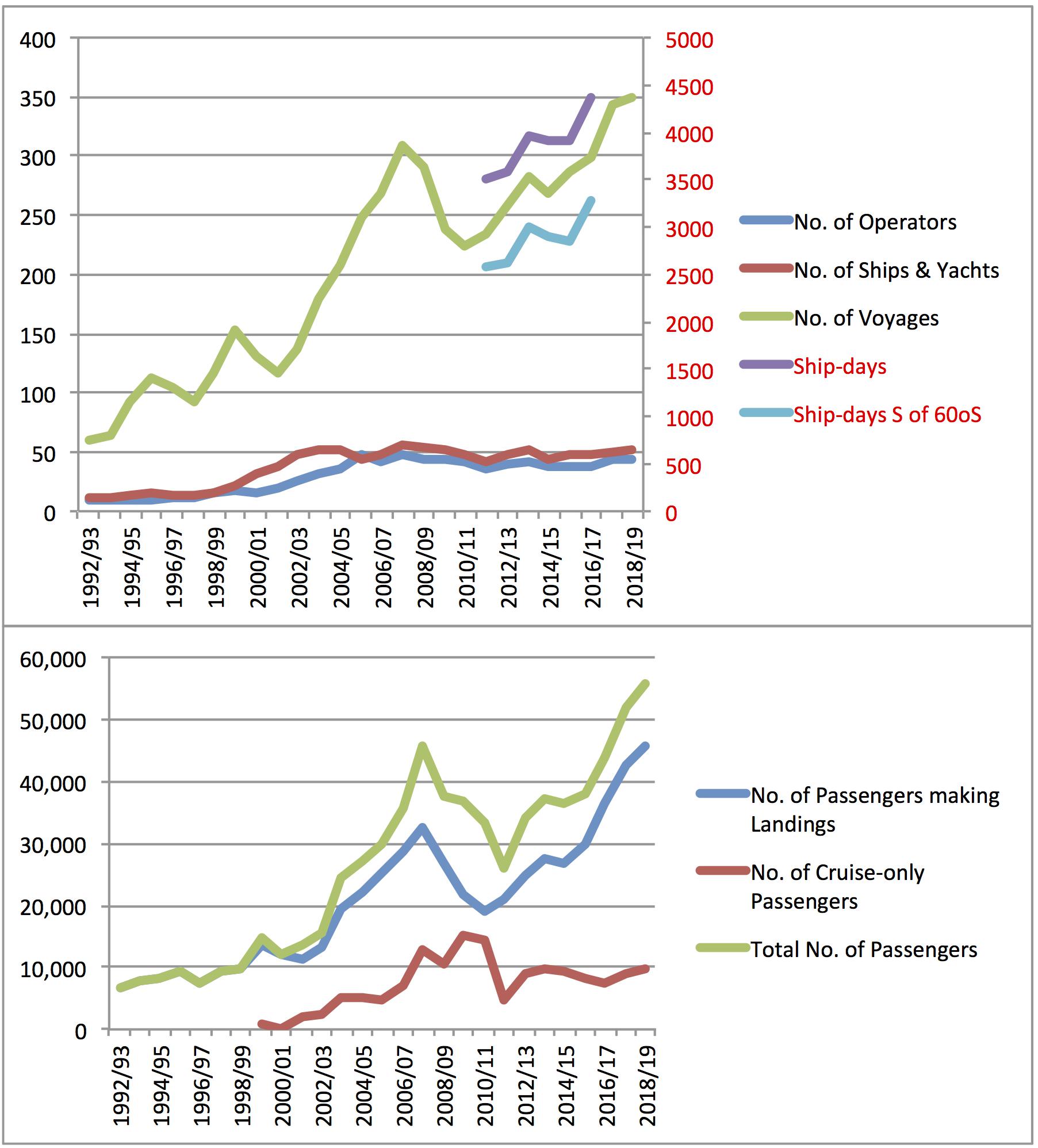
Figure 2. Trends in Antarctic ship-based tourism. For more information on these data, see International Association of Antarctica Tour Operators [IAATO] (2018).
Ship noise is continuous and consists of a broadband (10 Hz–20 kHz) cavitation spectrum overlain with distinct propeller and engine tones and harmonics (5–200 Hz) (e.g., Arveson and Vendittis, 2000; Kipple, 2002; Wales and Heitmeyer, 2002). In addition, icebreakers produce sounds related to pushing and crushing ice (Erbe and Farmer, 2000; Roth et al., 2013). Broadband radiated noise levels of large ships including icebreakers can be as high as 200 dB re 1 μPa m (Allen et al., 2012; Roth et al., 2013). Ships typically run echosounders for depth-ranging, and the ATCM has produced Resolution H (2014) “Strengthening Cooperation in Hydrographic Surveying and Charting of Antarctic Waters,” by which all ships of national Antarctic programs (and all other ships) are encouraged to collect hydrographic and bathymetric data using powerful echosounders while in the Antarctic Treaty area. Such echosounders repeatedly (every few seconds) emit pings at multiple frequencies (typically above 10 kHz) with source levels up to 240 dB re 1 μPa peak-to-peak (pk-pk) and 200 dB re 1 μPa2m2s (Crocker and Fratantonio, 2016; Crocker et al., 2018).
Research in the Antarctic is carried out from ships, land-based platforms, and air. Research station and wharf construction may involve geotechnical work, rock breaking, and pile driving—all of which generate noise underwater (e.g., Soloway and Dahl, 2014; Erbe and McPherson, 2017). Driving piles into the seafloor with a vibrator creates underwater noise at 10–1000 Hz with distinct tonal structure and levels up to 170 dB re 1 μPa rms at close range (Dahl et al., 2015). Percussive pile driving creates impulsive underwater noise of up to 227 dB re 1 μPa pk-pk and 201 dB re 1 μPa2s at close range (Hastings and Popper, 2005; Illinworth and Rodkin Inc, 2007). Aircraft produce noise in air, however, noise transmits into water directly below (e.g., Erbe et al., 2017b, 2018b). Additionally, some countries, such as Germany, Italy, Spain, Poland, China, South Korea, and Russia, have been undertaking marine seismic surveys for research in the Antarctic (Breitzke, 2014). Germany alone acquired 59,621 km of multichannel seismic survey lines between 1976 and 2011 (Boebel et al., 2009; Breitzke, 2014). Seismic airgun arrays emit broadband (5 Hz–20 kHz) pulses repeatedly (every 5–20 s) at levels up to 224 dB re 1 μPa2m2s (Ainslie et al., 2016; Li and Bayly, 2017).
Sound propagation in Antarctic waters differs from that at lower latitudes due to low surface temperatures and the possible presence of ice. In polar water, the sound speed increases with depth, which leads to upward refracting sound propagation paths and the establishment of a so-called surface duct. Sound trapped in the surface duct can travel over long ranges. Sound emitted near the surface will follow a refracted propagation path where it travels to some depth and then bends upward without interacting with the seafloor and thus without the associated reflection loss that occurs at the seafloor. Reflection occurs at the sea surface, and the associated loss depends on whether the surface is open or ice-covered, and on its roughness. First-year ice is typically smooth underwater and hence very little scattering loss occurs here, resulting in very effective sound propagation under such ice. Furthermore, given the deep bathymetry around Antarctica, there is no low-frequency mode stripping, meaning that low-frequency noise from ships or seismic airguns can travel over very long ranges (hundreds to thousands of kilometers; Siebert et al., 2014; Gavrilov, 2018). With such long-range propagation, the spectral and temporal features of sound change, because energy at different frequencies travels at slightly different speeds and along slightly different paths (termed “dispersion”; Horton Sr, 1974; Dushaw et al., 1993). Brief (100 ms), broadband (<20 kHz), high-amplitude pulses as emitted by seismic airguns turn into longer-duration (several seconds), narrower-band (<200 Hz), lower-amplitude, frequency-modulated sounds at distances of tens of kilometers (Yang, 1984; Siebert et al., 2014; Hastie et al., 2019). Such spectro-temporal changes in noise characteristics yield different types of noise impacts as a function of range.
Noise Impacts
The effects of noise on marine mammals range from individual, short-term responses to population-level, long-term impacts (see Erbe et al., 2018a). In terms of severity, they also range from cases which might not result in any consequences, to those that prompt behavioral changes, mask communication, induce hearing loss, increase stress, or lead to death (e.g., in the case of tactical mid-frequency sonar affecting beaked whales; Fernández et al., 2013). Mortality can also occur in close proximity to underwater explosions (Ketten, 1995; Danil and St. Leger, 2011). These types of noise impacts have been reported not only for marine mammals but also for fishes and other taxa (e.g., Day et al., 2017, 2019; McCauley et al., 2017; Hawkins and Popper, 2018), which are preyed upon by marine mammals. Noise impacts on these taxa can thus indirectly affect marine mammals if noise leads to a physical reduction in prey availability or to a change in prey behavior that affects its availability to predators. Examples for each type of effect of noise on Antarctic marine mammals or their closely related northern species are summarized in Table 3.
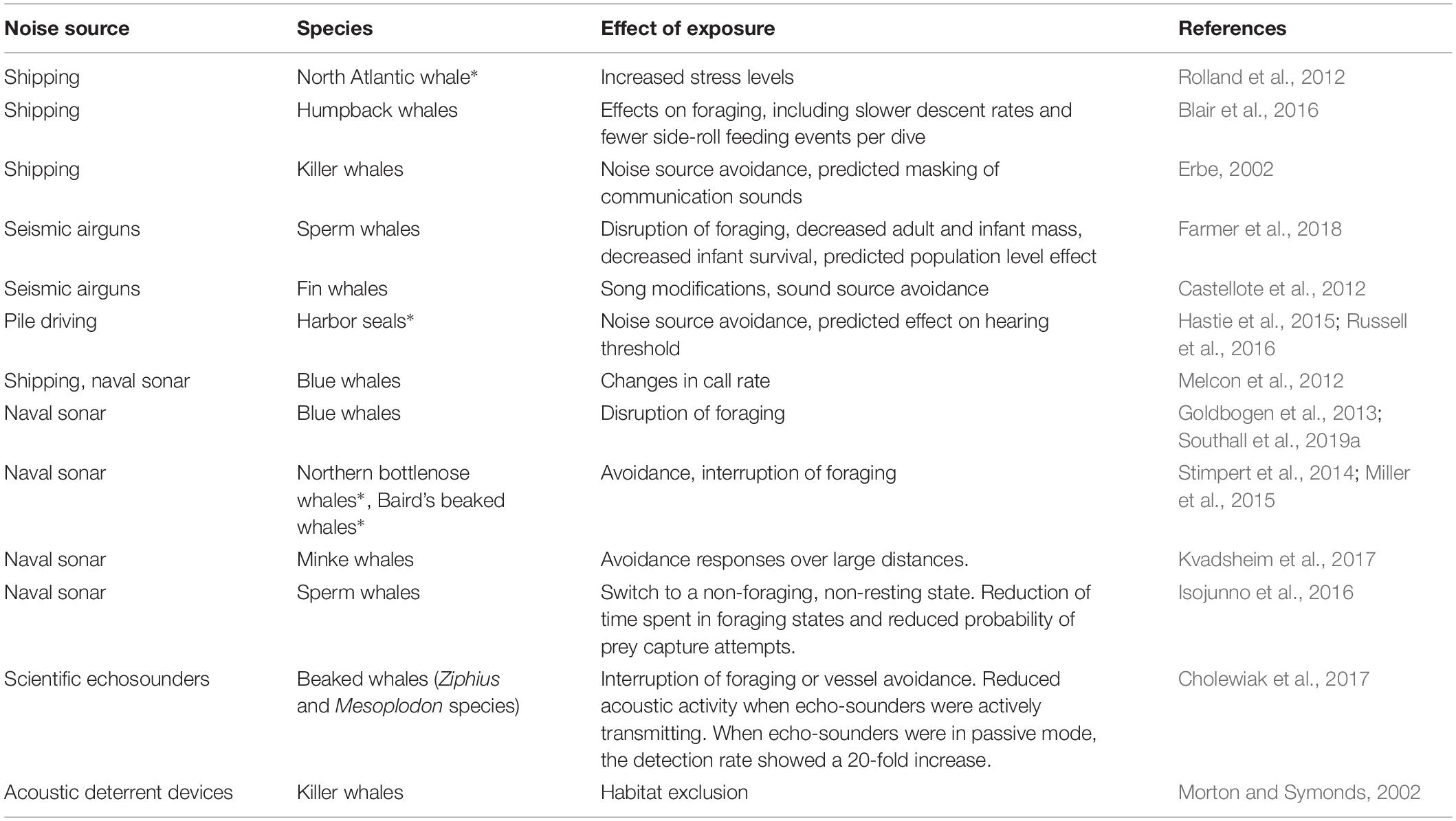
Table 3. Examples of reported effects of noise on Antarctic marine mammals (∗ or closely related species).
While the above impacts are experienced by individual animals, they can lead to population-level impacts. Animals might be displaced from preferred habitats into areas with higher predation risk, lower prey abundance, or poorer prey quality. They might suffer reduced energy intake while expending more energy. Malnutrition, stress and hearing loss might compromise health and lead to shortened life span. If enough individuals in a population are affected, then population dynamics may change. The Population Consequences of Acoustic Disturbance (PCAD) and Population Consequences of Disturbance (PCoD) models provide a conceptual framework that link short-term individual impacts to population consequences (National Research Council, 2005; Harwood et al., 2014; National Academies of Sciences, Engineeering and Medicine, 2017). A well-studied example species is the elephant seal (both northern and southern), where disrupted foraging behavior due to noise leads to predictions of reduced foraging success in mothers; then a reduced maternal mass leads to reduced pup mass at weaning, which is predicted to negatively impact pup survival and lead to changes in population dynamics (New et al., 2014; Costa et al., 2016). Population consequences of disturbance can potentially be significant, in particular when noise-making activities occur in high-priority areas for a population.
Research on hearing abilities and the effects of noise on Antarctic marine mammals has been sparse and little data are available to assess the potential impacts of noise on their hearing. Out of the 23 marine mammal species that occur south of the Antarctic Convergence, a behavioral audiogram is only available for the killer whale (Branstetter et al., 2017), with some hearing information from auditory evoked potential measurements on a stranded long-finned pilot whale (Pacini et al., 2010). Behavioral audiograms remain the standard for hearing tests and provide a whole-animal response (including decision making by the animal); in contrast, auditory evoked potential audiograms reflect the averaged response of the auditory brainstem to acoustic stimuli only. The audiogram of the northern elephant seal could possibly be used as a surrogate for the southern elephant seal (Kastak and Schusterman, 1999). Although several anatomical predictions of the frequency range of hearing have been produced for mysticetes (Houser et al., 2001; Parks et al., 2007; Tubelli et al., 2012; Cranford and Krysl, 2015), no empirically measured audiogram exists for any mysticete species globally. Data on noise-induced hearing loss or impacts of stress in Antarctic marine mammals do not exist, although a fair amount of work has been performed on the endocrine response to stress in the southern elephant seal’s close relative, the northern elephant seal (e.g., Ensminger et al., 2014; Jelincic et al., 2017). While the sounds made by Antarctic marine mammals have been documented (e.g., Erbe et al., 2017a), there is no information (such as critical ratios) to assess masking of those sounds by noise, except for four studies indicating anti-masking processes in humpback, killer, and long-finned pilot whales elsewhere (see Erbe et al., 2016). There have been no dedicated studies on the behavioral responses of marine mammals to noise in the Antarctic, though some studies have been undertaken on the same species in other regions (Table 2). It is uncertain how results from other regions (where animals potentially undergo different life functions; e.g., feeding in the Southern Ocean versus breeding at low latitudes) and other populations or species relate to Antarctic marine mammals, especially in light of the modulating influence that behavioral and exposure context can have on reactions to noise (Harris et al., 2018).
Noise Management
Annex I to the Protocol and the Guidelines for Environmental Impact Assessment in Antarctica (Resolution 1, 2016) outline the Environmental Impact Assessment (EIA) process for activities in the Antarctic. The proponent prepares the EIA document, which describes the project and the environment, identifies potential interactions and consequences, determines the significance of predicted impacts, considers alternatives, and designs mitigation and monitoring programs (Figure 3). Monitoring is (a) required for activities expected to have more than a minor or transitory impact, (b) suggested for those of minor or transitory impacts, and (c) not required for those of less than minor or transitory impacts. The EIA is reviewed and assessed by national authorities. Projects with environmental impacts that are less than minor or transitory are allowed to proceed—potentially with conditions imposed. Projects with environmental impacts that are minor or transitory require that the proponent prepare an Initial Environmental Evaluation (IEE). Projects with environmental impacts that are more than minor or transitory require that the proponent prepare a Comprehensive Environmental Evaluation (CEE). The CEE is reviewed by all the Antarctic Treaty Parties, by the CEP, and at the ATCM. The final CEE addresses comments from this review process. The national authorities eventually make a decision to either reject the project or allow the project to proceed—likely with conditions imposed.
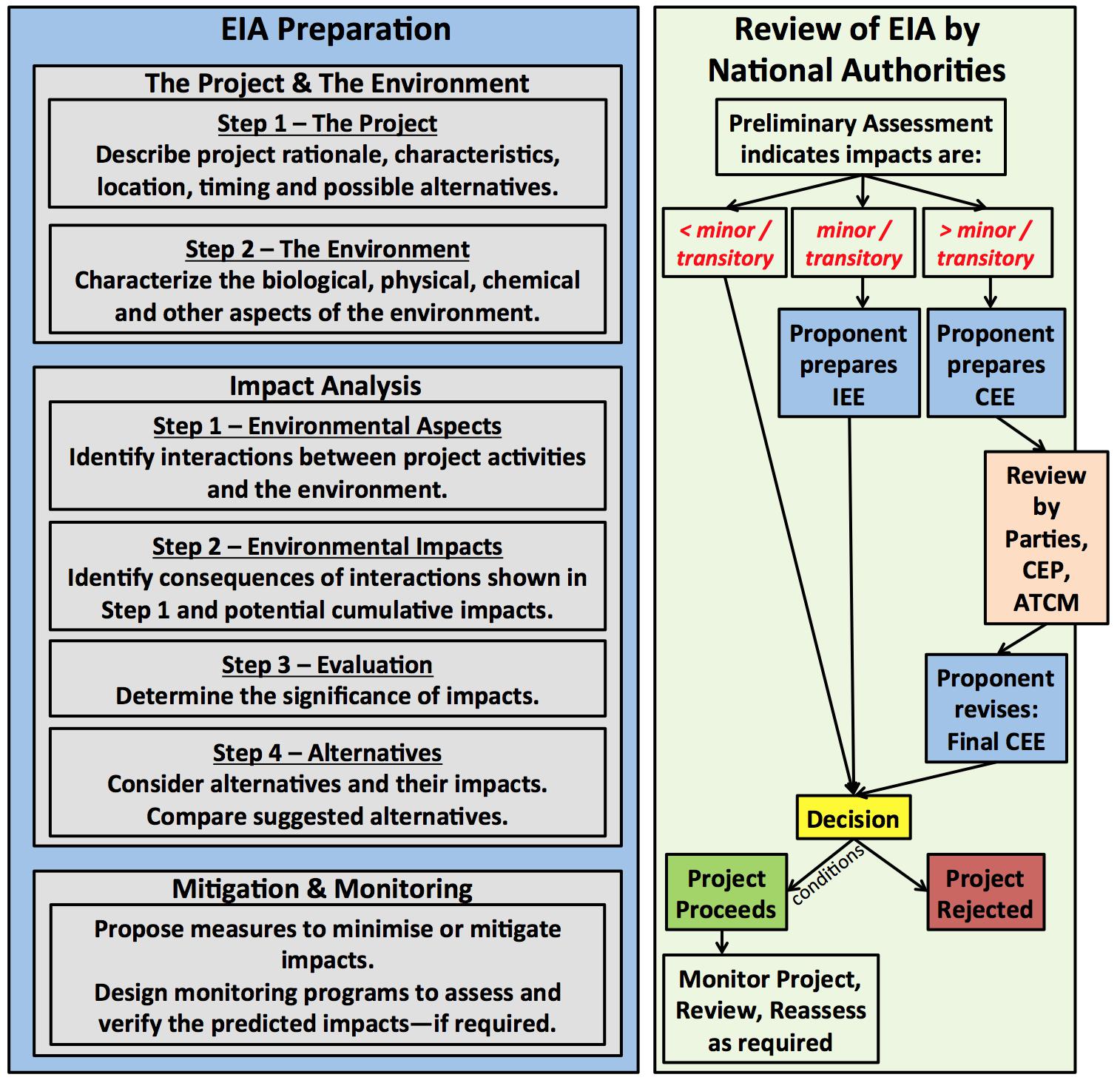
Figure 3. A schematic of the EIA process outlined in Annex I of the Protocol. The Proponent prepares the EIA, which includes sections on the project, the environment, potential impacts, and mitigation and monitoring measures. The EIA is reviewed by national authorities. If impacts are expected to be greater than minor or transitory, the Parties of the Antarctic Treaty, the CEP and ATCM get to review the proposal as well. National authorities make the final decision whether a project goes ahead (potentially with imposed conditions) or not.
Since the EIA process is conducted at a national level, several countries and jurisdictions have developed guidelines and regulations for the management of underwater noise (Erbe, 2013; Lucke et al., 2016). Typically these involve exposure modeling and impact prediction, mitigation, and sometimes in situ monitoring related to intense sources such as seismic airguns or pile driving.
Impact prediction requires knowledge of sound characteristics and levels at which different types of impact occur. While the regulations in different countries often aim to protect the same or similar species from the same types of impact, the metrics, thresholds, and management procedures that are applied differ. One reason for these differences is that the impact of sound on marine mammals is an active field of science, and new knowledge is being delivered gradually. There is a general acceptance that hearing damage can result from either an instantaneous exposure to very high sound pressure levels or from the accumulated exposure to acoustic energy over an extended period of time. This requires management with dual criteria and thresholds to address the different types of sound sources in the ocean, one sound pressure based, the other energy based (Southall et al., 2019b). Regulators aim to ensure that any exceedance of these thresholds does not have significant impacts to the noise-exposed populations. Energy-based criteria present particular practical challenges in that the animals’ behavior, and in particular how they move in three dimensions with respect to a sound source, affects the received acoustic exposure. Often this is the least known and most uncertain component of a risk assessment.
For example, for high-frequency cetaceans such as porpoises and exposure from impulsive noise such as impact pile driving, the United States. uses a dual criterion (i.e., applies the one resulting in the largest effect distance) of 196 dB re 1 μPa zero-to-peak pressure and 140 dB re 1 μPa2s cumulative sound exposure (weighted and integrated over 24 h) as the onset of temporary threshold shift (TTS), and a dual criterion of 202 dB re 1 μPa zero-to-peak pressure and 155 dB re 1 μPa2s cumulative sound exposure (weighted and integrated over 24 h) as the onset of permanent threshold shift (PTS) (National Marine Fisheries Service, 2018). Germany applies a dual criterion of an unweighted single-impulse (i.e., not cumulative) sound exposure level of 160 dB re 1 μPa2s and 190 dB re 1 μPa peak-to-peak pressure at a range of 750 m from the pile in order to avoid TTS (Bundesministerium für Umwelt Naturschutz und Reaktorsicherheit, 2014). While the United States criteria are applied at the receiving animal, which can be anywhere around the source, the German criteria are referenced to the exact distance of 750 m from the source.
The criteria employed by the two countries are difficult to compare, and it is not possible to generalize which country uses stricter regulations, because the criteria apply at different ranges, and the site-specific sound propagation environment will affect at what range certain levels are exceeded. Furthermore, Germany uses unweighted sound exposure, while the United States weights exposures according to categorization to a defined functional hearing group. Germany uses single exposures, while the United States integrates over 24 h. Different regulators also vary in the degree of precaution they are minded to apply, given the high levels of uncertainty in so many aspects of this topic. Germany, for example, considers TTS as the beginning of injury, whereas the United States only considers the onset of PTS auditory injury under the Marine Mammal Protection Act (MMPA) (National Marine Fisheries Service, 2016).
There are a number of mitigation procedures that can be applied to reduce noise exposures from various sources (Weir and Dolman, 2007; Dolman et al., 2009; Merck et al., 2014; Verfuss et al., 2016). Some methods can be applied at or close to the source. This might involve using a quieter source or one that produces a different type of signal that might reduce specific impacts (e.g., marine vibroseis versus seismic airguns; Duncan et al., 2017). Sound barriers (e.g., bubble curtains) may be installed near a fixed source to reduce sound propagation into the wider environment. Operations might be scheduled to occur at times when marine mammal abundance is expected to be lower or to avoid times of particular vulnerability, such as calving seasons (Van Opzeeland and Boebel, 2018). During operations, safety zones might be searched for marine mammals (e.g., using visual, infrared, and/or passive acoustic methods). A delay in initiating activities or a shut-down might result if animals are detected within mitigation zones. The effectiveness of this as a mitigation approach depends on the ability to detect animals and in many cases results in little reduction of risk (Leaper et al., 2015).
Mitigation effectiveness and practicality depend on the activities to be mitigated, the environment, the target species, and the approach taken. Multiple mitigation approaches are sometimes applied. Generally, mitigation can reduce the risks to marine mammals, but not eliminate them. Impacts such as behavioral disturbance and masking are particularly difficult to minimize except by reducing sound at the source. In the presence of knowledge gaps and uncertainty on noise impacts, regulators are expected to take a conservative approach, following the precautionary principle. What level of mitigation is reasonably practicable is debatable amongst proponents and regulators.
International Responsibility
Concern about the effects of anthropogenic underwater noise on marine life is widespread and increasing (as evidenced by, e.g., publication patterns; Williams et al., 2015; Shannon et al., 2016). In some countries, underwater noise is considered a form of water pollution, alongside chemical pollution (e.g., in the European Union; van der Graaf et al., 2012). Sound underwater travels much faster and farther than it does in air. Depending on the sound propagation conditions, sound can travel hundreds of kilometers and traverse entire ocean basins. Noise therefore crosses legal boundaries and the noise received in one jurisdiction might originate in a region that is under different jurisdiction, making noise regulation and ultimately conservation management an international responsibility.
The Convention on Environmental Impact Assessment in a Transboundary Context (Espoo Convention, United Nations Economic Commission for Europe [UNECE], 1991) requires that member states undertake environmental impact assessments of planned activities, and then inform and consult other member states if the impacts are expected to occur in other states as well. There are several examples where countries that border the same body of water have reached international agreements to manage noise and other stressors. This is the case for some European seas (Agreement on the Conservation of Cetaceans of the Black Sea, Mediterranean Sea and Contiguous Atlantic Area - ACCOBAMS; Pavan, 2006; Authier et al., 2017), Baltic (Helsinki Commission - HELCOM; Backer et al., 2010), European Union (Marine Strategy Framework Directive - MSFD; van der Graaf et al., 2012), and other regions.
Other international organizations that recognize underwater noise as a threat to marine mammals (including in the Antarctic) are the IWC, the Convention on Biological Diversity (CBD), the Convention on Migratory Species (CMS), the International Maritime Organization (IMO), and the United Nations General Assembly. Anthropogenic underwater noise was the focus topic for the UN Informal Consultative Process on Oceans and the Law of the Sea in June 201812.
The IWC supported a workshop on global soundscape mapping in 2014 and a workshop on acoustic masking in 2016, and continues to discuss underwater noise at annual meetings of its Scientific Committee. In 2018, the IWC passed a Resolution on Anthropogenic Underwater Noise13 by consensus, recognizing that chronic anthropogenic underwater noise is affecting the marine acoustic environment in many regions, and that there is emerging evidence that compromised acoustic habitat may adversely affect some cetacean populations.
In 2014, the Conference of the Parties to the CBD (Decision XII/23) encouraged parties to take appropriate measures to avoid, minimize, and mitigate the potential significant adverse impacts of anthropogenic underwater sound on marine and coastal biodiversity. It also encouraged governments to require environmental impact assessments (EIAs) for sound-generating offshore activities, and to combine mapping of the acoustic footprints of activities with habitat mapping to identify areas of risk.
The Conference of the Parties to the CMS adopted a Resolution on “Adverse impacts of anthropogenic noise on cetaceans and other migratory species” in 2017, which urges parties, whose flagged vessels travel beyond national jurisdictional limits, to undertake EIAs and manage the impact of anthropogenic noise on CMS-listed marine species and their prey. Guidelines for EIAs of underwater noise were also published in 2017 under this Convention.
The IMO stated that uncertainty as to the effects of noise should not preclude efforts toward developing quieting technologies for commercial ships (International Maritime Organization, 2009). The IMO developed guidelines on underwater noise reduction (MEPC.1/Circ.833) in 2014 acknowledging that noise from commercial ships may have both short- and long-term negative consequences on marine life. The International Convention for the Prevention of Pollution from Ships (MARPOL 1973/1978) and the International Code for Ships Operating in Polar Waters (Polar Code) were both developed by the IMO and have implications for ship noise in the Antarctic. MARPOL bans heavy fuel oil, both as fuel and cargo, from south of 60°S, thus limiting older vessels, which may emit more noise due to older, less efficient propulsion and design. The Polar Code requires that vessel masters consider marine mammal aggregation and migration areas when planning routes.
The International Association of Antarctic Tour Operators (IAATO) promotes environmentally responsible travel to Antarctica and could be an organization to also address underwater noise. However, some commercial operators are not members of the IAATO.
The Commission for the Conservation of Antarctic Marine Living Resources (CCAMLR) has the objective of conserving Antarctic marine life and focuses on commercial fisheries species (e.g., krill and toothfish). Impacts of anthropogenic noise on marine mammal prey species may thus need to be considered by CCAMLR.
Research and Management Needs
In November 2018, we held an international workshop on the possible effects of noise on Antarctic marine mammals in Berlin, Germany. Twenty-nine workshop participants (15 biological scientists, 5 regulators, and 9 Antarctic seismic and ship noise producers) were asked what they saw as key research and management needs for this topic in the Antarctic. We then asked all participants to rate the importance of each topic on a scale from 1 (low importance) to 5 (high importance). The complete list of topics and their scores can be found in the Supplementary Material. The research needs that received the highest rankings by workshop participants were (1) better data on abundance and distribution of Antarctic marine mammals with particular urgency to identify areas of high abundance (“hotspots”) and low abundance (“coldspots”), (2) hearing data for Antarctic marine mammals, in particular a mysticete audiogram, and (3) an assessment of the effectiveness of various noise mitigation options.
Management needs with the three highest scores overall were a refinement of noise exposure criteria, clear application guidance for environmental impact assessments, and transparency in regulatory decisions. Transparency was the highest-ranking need for proponents (4.67/5), though ranked lower by regulators (3.25/5). Regulators ranked the refinement of noise exposure criteria highest (4.5/5) and proponents agreed this was important (4.17/5). Biological scientists prioritized the need for agencies with an Antarctic interest (e.g., SCAR and CCAMLR) to join forces on noise management (4.4/5), establishing a public database on marine mammal distribution (4.2/5) and the sharing of research and ancillary data amongst users (e.g., of seismic data and echosounders data) (4.1/5). There were a few very specific research needs that directly relate to management and regulation requirements, such as (1) the allowance of hearing impairment recovery in cumulative exposure calculations, (2) justification and modification of the 24 h integration period for cumulative exposure calculations, and (3) choosing an appropriate metric and weighting to predict behavioral disturbance. These three needs and studies on responses to natural ambient noise were ranked of very high importance by potential noise producers, yet low by regulators.
Assessing and managing underwater noise and its potential impacts on marine mammals in the Antarctic is complex and difficult. Multiple countries operate in Antarctica, and many stakeholders and sectors have an interest in the Antarctic (tourism, fisheries, shipping, research, and conservation). In addition to the complicated management framework, there are significant scientific knowledge gaps. Antarctic species are understudied. Some undergo critical life functions while in the Antarctic (such as feeding by mysticetes before migration to breeding grounds at lower latitudes) and it can only be speculated how impacts potentially incurred in the Antarctic will affect the fitness of these animals when in other areas. Applying data on noise impacts from other areas or species should be avoided until similarities are proven. The unique aspects of the Southern Ocean (i.e., the species and their life functions in this environment, the ambient noise, and the sound propagation characteristics) constitute a critical need for research on the effects of anthropogenic noise.
Many of the research needs we present here have been internationally recognized and some are at least partially addressed by other entities. For example, the urgent need to identify marine mammal hotspots has also recently (October 2018) been recognized by the IUCN Marine Mammal Protected Areas Task Force, proposing 15 candidate Important Marine Mammal Areas (IMMAs) for the Southern Ocean and Sub-Antarctic Islands14. The Subcommittee on Ocean Science and Technology (SOST), which is a partnership between the United States Office of Naval Research, Chief of Naval Operations N45, the Bureau of Ocean Energy Management, the National Oceanic and Atmospheric Administration, and the Marine Mammal Commission, called for proposals for mysticete audiograms in mid-2018. The Joint Industry Program of Oil and Gas Producers currently include a study on masking in marine mammals. Some research needs, such as the effectiveness of certain mitigation methods, behavioral responses, and prey responses (e.g., availability of krill), could potentially be developed as proposals for future voyages to Antarctica.
Data sharing is one aspect of international collaboration and efforts are underway to make seismic and hydrographic data publicly available. There appear to be significant delays of several years in this process, but the complexity, effort, and costs of data preparation, warehousing, and support are considerable.
It is encouraging that the international scientific community is coming together to review current knowledge as reported here and that efforts are underway to fill some of the research gaps that we recognized. The Antarctic is unusual in its sound transmission characteristics, its species community, and the way in which humans use its waters. Not all findings from other areas of the world are necessarily applicable to this environment, and studies addressing the effects of noise specifically in the Antarctic are often lacking. Furthermore, different nations operate around the continent without necessarily coordinating their efforts. The effects of multiple stressors and multiple sound sources have been recognized as a high research priority in the marine science community in general (Rudd, 2014). In the Arctic, an integrated approach in the management of noise sources has been called for Moore et al. (2012). A similar approach would be prudent in the Antarctic. In 2048, the Protocol on Environmental Protection of the Antarctic Treaty may be reviewed if one of the Parties requests it. Additional anthropogenic activities such as mining may be considered. Such activities would lead to an increase of noise in the Antarctic. We hope that our review here will contribute to identifying and steering where research and management actions are most needed to protect the Antarctic environment from anthropogenic noise as much as possible.
Author Contributions
All of the authors participated in the workshop on Antarctic noise and effects on marine mammals, collaborated on the data gap table, and wrote sections of the report and/or manuscript. At the workshop, the following authors reviewed, summarized and presented the main topics. HH and AS-S: regulation. MM: human activities in Antarctica. MD: Antarctic marine mammal ecology. DH: hearing and hearing impairment. CE: acoustic masking. SK: behavior. JO: population consequences. RM: marine mammal prey impacts. JG: mitigation and monitoring. MS: ambient noise and propagation. MM, AM, and CE drew the figures. CE and VJ organized the workshop and produced the first draft of the overall manuscript.
Funding
The workshop on Antarctic noise and effects on marine mammals was funded by the German Environment Agency.
Conflict of Interest
MS was employed by company DW-ShipConsult.
The remaining authors declare that the research was conducted in the absence of any commercial or financial relationships that could be construed as a potential conflict of interest.
Acknowledgments
We thank all of the workshop participants.
Supplementary Material
The Supplementary Material for this article can be found online at: https://www.frontiersin.org/articles/10.3389/fmars.2019.00647/full#supplementary-material
Footnotes
- ^ ATCM 2019, WP 68, “Anthropogenic Noise in the Southern Ocean: an Update,” submitted by SCAR.
- ^ COMNAP Antarctic Facilities Master List v 2.0.0, dated 08.12.2017; https://github.com/PolarGeospatialCenter/comnap-antarctic-facilities/raw/73f28e19f7e93f9e9e8b2c4dfb620b510e5eb256/dist/COMNAP_Antarctic_Facilities_Master.xls
- ^ ATCM XLI, IP 71: IAATO Overview of Antarctic Tourism: 2017–2018 Season and Preliminary Estimates for 2018–2019 Season. Data from Appendix 1; https://iaato.org/documents/10157/2398215/IAATO+overview/bc34db24-e1dc-4eab-997a-4401836b7033
- ^ CCAMLR List of authorized vessel for season 2017/2018; https://www.ccamlr.org/en/compliance/list-authorised-vessels
- ^ Based on IAATO 2017 statistics: 2016–2017 Summary of Seaborne, Airborne and Land-Based Antarctic Tourism.
- ^ Assuming 30 days/ship; CCAMLR List of authorized vessel for season 2016/2017: https://www.ccamlr.org/en/compliance/list-authorised-vessels
- ^ Data provided by COMNAP based on COMNAP’s Ship Position Reporting System (SPRS). Ships are requested to report once per day.
- ^ ATCM XLI, IP 71: IAATO Overview of Antarctic Tourism: 2017–2018 Season and Preliminary Estimates for 2018–2019 Season. Data from Appendix 1.
- ^ ATCM XXXIX, IP 36: Antarctic Tourism Study: Analysis and Enhancement of the Legal Framework, submitted by Germany. The German Environment Agency commissioned a study that showed, that of the >200 known yachts that sailed in the Antarctic Treaty area between 1997 and 2013, only 16% were IAATO-members at the time of their Antarctic Voyage.
- ^ Data provided by COMNAP based on COMNAP’s Ship Position Reporting System (SPRS).
- ^ data from https://www.ccamlr.org/en/compliance/list-authorised-vessels
- ^ http://www.un.org/depts/los/consultative_process/ICP-19_information_for_participants.pdf
- ^ https://iwc.int/document_3685.download
- ^ https://www.iucn.org/news/marine-and-polar/201810/fourth-important-marine-mammal-areas-workshop-adds-15-candidate-immas-southern-ocean-and-sub-antarctic-islands
References
Aguilar Soto, N., Johnson, M., Madsen, P. T., Tyack, P. L., Bocconcelli, A., and Borsani, J. F. (2006). Does intense ship noise disrupt foraging in deep-diving Cuvier’s beaked whales (Ziphius cavirostris)? Mar. Mam. Sci. 22, 690–699. doi: 10.1111/j.1748-7692.2006.00044.x
Aguilar, A. (2009). “Fin whale - Balaenoptera physalus,” in Encyclopedia of Marine Mammals, eds W. Perrin, B. Würsig, and J. Thewissen (Amsterdam, NL: Academic Press), 433–437.
Ainslie, M. A., Halvorsen, M. B., Dekeling, R. P. A., Laws, R. M., Duncan, A. J., Frankel, A. S., et al. (2016). Verification of airgun sound field models for environmental impact assessment. Proc. Meet. Acoust. 27:070018. doi: 10.1121/2.0000339
Allen, K., Peterson, M., Sharrard, G., Wright, D., and Todd, S. (2012). Radiated noise from commercial ships in the gulf of maine: implications for whale/vessel collisions. J. Acoust. Soc. Am. 132, EL229–EL235.
Antunes, R., Kvadsheim, P. H., Lam, F. P. A., Tyack, P. L., Thomas, L., Wensveen, P. J., et al. (2014). High thresholds for avoidance of sonar by free-ranging long-finned pilot whales (Globicephala melas). Mar. Pollut. Bull. 83, 165–180. doi: 10.1016/j.marpolbul.2014.03.056
Armstrong, A. J., and Siegfried, W. R. (1991). Consumption of antarctic krill by minke whales. Antarct. Sci. 3, 13–18. doi: 10.1017/S0954102091000044
Arveson, P. T., and Vendittis, D. J. (2000). Radiated noise characteristics of a modern cargo ship. J. Acoust. Soc. Am. 107, 118–129. doi: 10.1121/1.428344
Au, W. W. L., Popper, A. N., and Fay, R. R. (eds) (2000). Hearing by Whales and Dolphins. New York, NY: Springer.
Authier, M., Commanducci, F. D., Genov, T., Holcer, D., Ridoux, V., Salivas, M., et al. (2017). Cetacean conservation in the Mediterranean and Black Seas: fostering transboundary collaboration through the european marine strategy framework directive. Mar. Policy 82, 98–103. doi: 10.1016/j.marpol.2017.05.012
Ayres, K. L., Booth, R. K., Hempelmann, J. A., Koski, K. L., Emmons, C. K., Baird, R. W., et al. (2012). Distinguishing the impacts of inadequate prey and vessel traffic on an endangered killer whale (Orcinus orca) population. PLoS One 7:e36842. doi: 10.1371/journal.pone.0036842
Backer, H., Leppänen, J.-M., Brusendorff, A. C., Forsius, K., Stankiewicz, M., Mehtonen, J., et al. (2010). HELCOM Baltic Sea action plan – a regional programme of measures for the marine environment based on the ecosystem approach. Mar. Poll. Bull. 60, 642–649. doi: 10.1016/j.marpolbul.2009.11.016
Bain, D., and Dahlheim, M. (1994). “Effects of masking noise on detection thresholds of killer whales,” in Marine Mammals and the Exxon Valdez, ed. T. Loughlin (San Diego, CA: Academic Press), 243–256.
Baraff, L. S., Clapham, P. J., Mattila, D. K., and Bowman, R. S. (1991). Feeding behavior of a humpback whale in low latitude waters. Mar. Mamm. Sci. 7, 197–202. doi: 10.1111/j.1748-7692.1991.tb00567.x
Baumgartner, M. F., and Fratantoni, D. M. (2008). Diel periodicity in both sei whale vocalization rates and the vertical migration of their copepod prey observed from ocean gliders. Limnol. Oceanogr. 53, 2197–2209. doi: 10.4319/lo.2008.53.5_part_2.2197
Bender, N. A., Crosbie, K., and Lynch, H. J. (2016). Patterns of tourism in the antarctic peninsula region: a 20-year analysis. Antarcs. Sci. 28, 194–203. doi: 10.1017/s0954102016000031
Berman-Kowalewski, M., Gulland, F. M., Wilkin, S., Calambokidis, J., Mate, B., Cordaro, J., et al. (2010). Association between blue whale (Balaenoptera musculus) mortality and ship strikes along the california coast. Aqua. Mamm. 36, 59–66. doi: 10.1578/AM.36.1.2010.59
Best, P. B. (2007). Whales and Dolphins of the Southern African Subregion. Cambridge: Cambridge University Press.
Bester, M. N. (1988). Marking and monitoring studies of the Kerguelen stock of southern elephant seals Mirounga leonina and their bearing on biological research in the vestfold hills. Hydrobiologia 165, 269–277. doi: 10.1007/bf00025596
Bester, M. N., and Hofmeyr, G. J. G. (2005). Numbers of elephant seals at Prince Edward Island. Southern Ocean. South Afr. J. Wildlife Res. 35, 85–88.
Blair, H. B., Merchant, N. D., Friedlaender, A. S., Wiley, D. N., and Parks, S. E. (2016). Evidence for ship noise impacts on humpback whale foraging behaviour. Biol. Lett. 12:20160005. doi: 10.1098/rsbl.2016.0005
Boebel, O., Breitzke, M., Burkhardt, E., and Bornemann, H. (2009). Strategic Assessment of the Risk Posed to Marine Mammals by the use of Airguns in the Antarctic Treaty Area. Bremerhaven: Alfred Wegener Institute for Polar and Marine Research.
Bornemann, H., Kreyscher, M., Ramdohr, S., Martin, T., Carlini, A., Sellmann, L., et al. (2004). Southern elephant seal movements and antarctic sea ice. Antarcs. Sci. 12, 3–15. doi: 10.1017/S095410200000002X
Borsa, P. (1990). Seasonal occurrence of the leopard seal, Hydrurga leptonyx, in the Kerguelen Islands. Can. J. Zool. 68, 405–408. doi: 10.1139/z90-059
Boyd, I. L., McCafferty, D. J., Reid, K., Taylor, R., and Walker, T. R. (1998). Dispersal of male and female antarctic fur seals (Arctocephalus gazella). Can. J. Fish. Aquatics. Sci. 55, 845–852. doi: 10.1139/f97-314
Boyd, I. L., Staniland, I. J., and Martin, A. R. (2002). Distribution of foraging by female antarctic fur seals. Mar. Ecol. Prog. Ser. 242, 285–294. doi: 10.3354/meps242285
Bradshaw, C. J. A., Hindell, M. A., Best, N. J., Phillips, K. L., Wilson, G., and Nichols, P. D. (2003). You are what you eat: describing the foraging ecology of southern elephant seals (Mirounga leonina) using blubber fatty acids. Proc. R. Soc. Lond. Ser. B Biol. Sci. 270:1283. doi: 10.1098/rspb.2003.2371
Branch, T. A. (2006). Humpback abundance south of 60 oS from three completed sets of IDCR/SOWER circumpolar surveys. Available at: http://hdl.handle.net/11427/17255 (accessed October 18, 2019).
Branch, T. A. (2007). Abundance of antarctic blue whales south of 60 oS from three complete circumpolar sets of surveys. J. Cetacean Res. Manag. 9, 253–262.
Branch, T. A. (2011). Humpback whale abundance south of 60oS from three complete circumpolar sets of surveys. J. Cetacean Res. Manag. 3, 53–69.
Branch, T. A., and Butterworth, D. S. (2001). Estimates of abundance south of 60oS for cetacean species sighted frequently on the 1978/79 to 1997/98 IWC/IDCR-SOWER sighting surveys. J. Cetacean Res. Manag. 3, 251–270.
Branch, T. A., Monnahan, C. C., and Sirovic, A. (2018). Separating Pygmy Blue Whale Catches by Population. Impington: International Whaling Commission.
Branstetter, B. K., Leger, J. S., Acton, D., Stewart, J., Houser, D., Finneran, J. J., et al. (2017). Killer whale (Orcinus orca) behavioral audiograms. J. Acoust. Soc. Am. 141, 2387–2398. doi: 10.1121/1.4979116
Braulik, G. (2018). Lagenorhynchus cruciger. The IUCN Red List of Threatened Species, e.T11144A50361701.
Bravington, M. V., and Hedley, S. L. (2012). Abundance Estimates of Antarctic Minke Whales From the IWC IDCR/SOWER Surveys, 1986–2002. Impington: International Whaling Commission.
Breitzke, M. (2014). Overview of seismic research activities in the southern ocean-quantifying the environmental impact. Antarcs. Sci. 26, 80–92. doi: 10.1017/s095410201300031x
Brodie, P. F. (1975). Cetacean energetics, an overview of intraspecific size variation. Ecology 56, 152–161. doi: 10.2307/1935307
Brown, D. J., Boyd, I. L., Cripps, G. C., and Butler, P. J. (1999). Fatty acid signature analysis from the milk of antarctic fur seals and southern elephant seals from south georgia: implications for diet determination. Mar. Ecol. Prog. Ser. 187, 251–263. doi: 10.3354/meps187251
Bundesministerium für Umwelt Naturschutz und Reaktorsicherheit (2014). “Concept for the protection of harbour porpoises from sound exposures during the construction of offshore wind farms in the german north sea (sound protection concept),” in Paper Presented at the 21st ASCOBANS Advisory Committee Meeting, Gothenburg.
Burns, J. M., Trumble, S. J., Castellini, M. A., and Testa, J. W. (1998). The diet of Weddell seals in McMurdo Sound, Antarctica as determined from scat collections and stable isotope analysis. Pol. Biol. 19, 272–282. doi: 10.1007/s003000050245
Castellote, M., Clark, C., and Lammers, M. (2012). Acoustic and behavioural changes by fin whales (Balaenoptera physalus) in response to shipping and airgun noise. Biol. Conserv. 147, 115–122. doi: 10.1016/j.biocon.2011.12.021
Cherel, Y., Ducatez, S., Fontaine, C., Richard, P., and Guinet, C. (2008). Stable isotopes reveal the trophic position and mesopelagic fish diet of female southern elephant seals breeding on the Kerguelen Islands. Mar Ecol. Prog. Ser. 370, 239–247. doi: 10.3354/meps07673
Cholewiak, D., DeAngelis, A. I., Palka, D., Corkeron, P. J., and Van Parijs, S. M. (2017). Beaked whales demonstrate a marked acoustic response to the use of shipboard echosounders. [10.1098/rsos.170940]. R. Soc. Open Sci. 4:170940. doi: 10.1098/rsos.170940
Clapham, P. (2009). “Humpback whale - Megaptera novaeangliae,” in Encyclopedia of Marine Mammals, eds W. Perrin, B. Würsig, and J. Thewissen (Amsterdam, NL: Academic Press), 582–585.
Commission for the Conservation of Antarctic Marine Living Resources (2015). Conservation Measure 10-04: Automated satellite-linked Vessel Monitoring Systems (VMS) (Conservation Measure No. 10-04). Hobart, TAS: Commission for the Conservation of Antarctic Marine Living Resources.
Commission for the Conservation of Antarctic Marine Living Resources (2018). CCAMLR Statistical Bulletin, Vol. 30, Data Files. Hobart, TAS: Commission for the Conservation of Antarctic Marine Living Resources.
Cooke, J. G. (2018a). Balaenoptera acutorostrata. The IUCN Red List of Threatened Species 2018 e.T2474A50348265. Available at: http://dx.doi.org/10.2305/IUCN.UK.2018-2.RLTS.T2474A50348265.en (accessed September 08, 2019).
Cooke, J. G. (2018b). Balaenoptera musculus. The IUCN Red List of Threatened Species, e.T2477A50226195. Available at: http://dx.doi.org/10.2305/IUCN.UK.2018-2.RLTS.T2477A50226195.en (accessed October 18, 2019).
Costa, D. P., Schwarz, L., Robinson, P., Schick, R. S., Morris, P. A., Condit, R., et al. (2016). “A bioenergetics approach to understanding the population consequences of disturbance: elephant seals as a model system,” in The Effects of Noise on Aquatic Life II 161-169, eds A. N. Popper and T. Hawkins (New York, NY: Springer Verlag).
Cranford, T. W., and Krysl, P. (2015). Fin whale sound reception mechanisms: skull vibration enables low-frequency hearing. PLoS One 10:e0116222. doi: 10.1371/journal.pone.0116222
Crocker, S. E., and Fratantonio, F. D. (2016). Characteristics of Sounds Emitted During High-Resolution Marine Geophysical Surveys. Report no. TR 12,203. Newport, RI: Naval Undersea Warfare Center Division.
Crocker, S. E., Fratantonio, F. D., Hart, P. E., Foster, D. S., Thomas, F. O. B., and Labak, S. (2018). Measurement of sounds emitted by certain high-resolution geophysical survey systems. Piscataway, NJ: IEEE.
Cure, C., Antunes, R., Alves, A. C., Visser, F., Kvadsheim, P. H., and Miller, P. J. O. (2013). Responses of male sperm whales (Physeter macrocephalus) to killer whale sounds: implications for anti-predator strategies. Sci. Rep. 3:1579. doi: 10.1038/srep01579
Curé, C., Isojunno, S., Visser, F., Wensveen, P. J., Sivle, L. D., Kvadsheim, P. H., et al. (2016). Biological significance of sperm whale responses to sonar: comparison with anti-predator responses. Endanger Spec. Res. 31, 89–102. doi: 10.3354/esr00748
Dahl, P. H., Dall’Osto, D. R., and Farrell, D. M. (2015). The underwater sound field from vibratory pile driving. J. Acoust. Soc. Am. 137, 3544–3554. doi: 10.1121/1.4921288
Danil, K., and St. Leger, J. A. (2011). Seabird and dolphin mortality associated with underwater detonation exercises. Mar. Technol. Soc. J. 45, 89–95. doi: 10.4031/MTSJ.45.6.5
Day, R. D., McCauley, R. D., Fitzgibbon, Q. P., Hartmann, K., and Semmens, J. M. (2017). Exposure to seismic air gun signals causes physiological harm and alters behavior in the scallop Pecten fumatus. Proc. Natl. Acad. Sci. 114:E8537. doi: 10.1073/pnas.1700564114
Day, R. D., McCauley, R. D., Fitzgibbon, Q. P., Hartmann, K., and Semmens, J. M. (2019). Proc. R. Soc. B Biol. Sci. 286:20191424.
de Bruyn, P. J. N., Tosh, C. A., and Terauds, A. (2013). Killer whale ecotypes: is there a global model? Biol. Rev. 88, 62–80. doi: 10.1111/j.1469-185X.2012.00239.x
DeRuiter, S. L., Southall, B. L., Calambokidis, J., Zimmer, W. M. X., Sadykova, D., Falcone, E. A., et al. (2013). First direct measurements of behavioural responses by Cuvier’s beaked whales to mid-frequency active sonar. Biol. Lett. 9:20130223. doi: 10.1098/rsbl.2013.0223
Dolman, S. J., Weir, C. R., and Jasny, M. (2009). Comparative review of marine mammal guidance implemented during naval exercises. Mar. Poll. Bull. 58, 465–477. doi: 10.1016/j.marpolbul.2008.11.013
Dominello, T., and Širović, A. (2016). Seasonality of Antarctic minke whale (Balaenoptera bonaerensis) calls off the western Antarctic Peninsula. Mar. Mamm. Sci. 32, 826–838. doi: 10.1111/mms.12302
Duncan, A. J., Weilgart, L. S., Leaper, R., Jasny, M., and Livermore, S. (2017). A modelling comparison between received sound levels produced by a marine vibroseis array and those from an airgun array for some typical seismic survey scenarios. Mar. Poll. Bull. 119, 277–288. doi: 10.1016/j.marpolbul.2017.04.001
Dunlop, R. A., Cato, D. H., and Noad, M. J. (2010). Your attention please: increasing ambient noise levels elicits a change in communication behaviour in humpback whales (Megaptera novaeangliae). Proc. R. Soc. B Biol. Sci. 277, 2521–2529. doi: 10.1098/rspb.2009.2319
Dunlop, R. A., Noad, M. J., McCauley, R. D., Scott-Hayward, L., Kniest, E., Slade, R., et al. (2017). Determining the behavioural dose–response relationship of marine mammals to air gun noise and source proximity. J. Exp. Biol. 220, 2878–2886. doi: 10.1242/jeb.160192
Dushaw, B. D., Worcester, P. F., Cornuelle, B. D., and Howe, B. M. (1993). On equations for the speed of sound in seawater. J. Acoust. Soc. Am. 93, 255–275. doi: 10.1121/1.405660
Ellison, W., Southall, B., Clark, C., and Frankel, A. (2012). A new context-based approach to assess marine mammal behavioral responses to anthropogenic sounds. Conserv. Biol. 26, 21–28. doi: 10.1111/j.1523-1739.2011.01803.x
Ensminger, D. C., Somo, D. A., Houser, D. S., and Crocker, D. E. (2014). Metabolic responses to adrenocorticotropic hormone (ACTH) vary with life-history stage in adult male northern elephant seals. Gen. Comp. Endocrinol. 204, 150–157. doi: 10.1016/j.ygcen.2014.04.024
Enzenbacher, D. J. (1992). Tourists in Antarctica: numbers and trends. Pol. Record. 28, 17–22. doi: 10.1017/S0032247400020210
Erbe, C. (2002). Underwater noise of whale-watching boats and its effects on killer whales (Orcinus orca). Mar. Mamm. Sci. 18, 394–418. doi: 10.1111/j.1748-7692.2002.tb01045.x
Erbe, C., and Farmer, D. M. (2000). Zones of impact around icebreakers affecting beluga whales in the Beaufort Sea. J. Acoust. Soc. Am. 108, 1332–1340. doi: 10.1121/1.1288938
Erbe, C., Marley, S., Schoeman, R. P., Smith, J. N., Trigg, L. E., and Embling, C. B. (2019). The effects of ship noise on marine mammals – a review. Front. Mar. Sci. doi: 10.3389/fmars.2019.00606
Erbe, C., and McPherson, C. (2017). Underwater noise from geotechnical drilling and standard penetration testing. J. Acoust. Soc. Am. 142, EL281–EL285. doi: 10.1121/1.5003328
Erbe, C., Dunlop, R., and Dolman, S. (2018a). “Effects of noise on marine mammals,” in Effects of Anthropogenic Noise on Animals, eds H. Slabbekoorn, R. J. Dooling, A. N. Popper, and R. R. Fay (New York, NY: Springer), 277–309.
Erbe, C., Dunlop, R., Jenner, K. C. S., Jenner, M.-N. M., McCauley, R. D., Parnum, I., et al. (2017a). Review of underwater and in-air sounds emitted by Australian and Antarctic marine mammals. Acoust. Aust. 45, 179–241. doi: 10.1007/s40857-017-0101-z
Erbe, C., Parsons, M., Duncan, A. J., Osterrieder, S., and Allen, K. (2017b). Aerial and underwater sound of unmanned aerial vehicles (UAV, drones). J. Unmann. Vehicle Syst. 5, 92–101. doi: 10.1139/juvs-2016-0018
Erbe, C., Reichmuth, C., Cunningham, K. C., Lucke, K., and Dooling, R. J. (2016). Communication masking in marine mammals: a review and research strategy. Mar. Poll. Bull. 103, 15–38. doi: 10.1016/j.marpolbul.2015.12.007
Erbe, C., Williams, R., Parsons, M., Parsons, S. K., Hendrawan, I. G., and Dewantama, I. M. I. (2018b). Underwater noise from airplanes: an overlooked source of ocean noise. Mar. Poll. Bull. 137, 656–661. doi: 10.1016/j.marpolbul.2018.10.064
Falcone, E. A., Schorr, G. S., Watwood, S. L., DeRuiter, S. L., Zerbini, A. N., Andrews, R. D., et al. (2017). Diving behaviour of Cuvier’s beaked whales exposed to two types of military sonar. R. Soc. Open Sci. 4:170629. doi: 10.1098/rsos.170629
Farmer, N. A., Baker, K., Zeddies, D. G., Denes, S. L., Noren, D. P., Garrison, L. P., et al. (2018). Population consequences of disturbance by offshore oil and gas activity for endangered sperm whales (Physeter macrocephalus). Biol. Conserv. 227, 189–204. doi: 10.1016/j.biocon.2018.09.006
Fernández, A., Arbelo, M., and Martín, V. (2013). Whales: no mass stranding since sonar ban. Nature 497:317. doi: 10.1038/497317d
Fernandez, A., Edwards, J. F., Rodriguez, F., de los Monteros, A. E., Herraez, P., Castro, P., et al. (2005). “Gas and fat embolic syndrome” involving a mass stranding of beaked whales (Family Ziphiidae) exposed to anthropogenic sonar signals. Veterinary Pathology 42, 446–457. doi: 10.1354/vp.42-4-446
Fernández, M., Berón-Vera, B., García, N. A., Raga, J. A., and Crespo, E. A. (2003). Notes: Food and parasites from two hourglass dolphins Lagenorhynchus cruciger (Quoy and Gaimard, 1824) from Patagonian waters. Mar. Mamm. Sci. 19, 832–836. doi: 10.1111/j.1748-7692.2003.tb01133.x
Field, I. C., Cherel, Y., Guinet, C., Bradshaw, C. J., McMahon, C. R., and Hindell, M. A. (2011). Diet of juvenile southern elephant seals reappraised by stable isotopes in whiskers. Mar. Ecol. Prog. Ser. 424, 247–258. doi: 10.3354/meps08769
Finneran, J. J. (2015). Noise-induced hearing loss in marine mammals: a review of temporary threshold shift studies from 1996 to 2015. J. Acoust. Soc. Am. 138, 1702–1726. doi: 10.1121/1.4927418
Ford, J. (2009). “Killer whale - Orcinus orca,” in Encyclopedia of Marine Mammals, eds W. Perrin, B. Würsig, and J. Thewissen (Amsterdam: Academic Press), 650–657.
Friedlaender, A. S., Nowacek, D. P., Johnston, D. W., Read, A. J., Tyson, R. B., Peavey, L., et al. (2010). Multiple sightings of large groups of Arnoux’s beaked whales (Berardius arnouxii) in the Gerlache Strait, Antarctica. Mar. Mamm. Sci. 26, 246–250. doi: 10.1111/j.1748-7692.2009.00346.x
Gambell, R. (1985). “Fin whale Balaenoptera physalus (Linnaeus, 1758),” in Handbook of Marine Mammals, eds S. Ridgeway and H. R. Harrison (London: Academic Press), 171–192.
Gannon, D. P., Barros, N. B., Nowacek, D. P., Read, A. J., Waples, D. M., and Wells, R. S. (2005). Prey detection by bottlenose dolphins, Tursiops truncatus: an experimental test of the passive listening hypothesis. Anim. Behav. 69, 709–720. doi: 10.1016/j.anbehav.2004.06.020
Gannon, D. P., Read, A. J., Craddock, J. E., Fristrup, K. M., and Nicolas, J. R. (1997). Feeding ecology of long-finned pilot whales Globicephala melas in the western north atlantic. Mar. Ecol. Prog. Ser. 148, 1–10. doi: 10.3354/meps148001
Gavrilov, A. (2018). Propagation of underwater noise from an offshore seismic survey in Australia to Antarctica: measurements and modelling. Acoust. Aust. 46, 143–149. doi: 10.1007/s40857-018-0131-1
Gavrilov, A., and Li, B. (2007). “Antarctica as one of the major sources of noise in the ocean,” in Proceedings of the Underwater Acoustic Measurements: Technologies & Results, 2nd International Conference and Exhibition, 25-29 June 2007, Heraklion.
Gill, P. C., and Thiele, D. (1997). A winter sighting of killer whales (Orcinus orca) in antarctic sea ice. Pol. Biol. 17, 401–404. doi: 10.1007/s003000050
Goldbogen, J. A., Southall, B. L., DeRuiter, S. L., Calambokidis, J., Friedlaender, A. S., Hazen, E. L., et al. (2013). Blue whales respond to simulated mid-frequency military sonar. Proc. R. Soc. B 280:20130657. doi: 10.1098/rspb.2013.0657
Goodall, R. N. P. (1997). Review of Sightings of the Hourglass Dolphin, Lagenorhynchus cruciger, in the South American Sector of the Antarctic and Sub-Antarctic. Impington: International Whaling Commission.
Götz, T., and Janik, V. M. (2013). Acoustic deterrent devices to prevent pinniped depredation: efficiency, conservation concerns and possible solutions. Mar. Ecol. Prog. Ser. 492, 285–302. doi: 10.3354/meps10482
Hall, J. D., and Johnson, C. S. (1972). Auditory thresholds of a killer whale Orcinus orca linnaeus. J. Acoust. Soc. Am. 51, 515–517. doi: 10.1121/1.1912871
Harris, C. M., Thomas, L., Falcone, E. A., Hildebrand, J., Houser, D., Kvadsheim, P. H., et al. (2018). Marine mammals and sonar: dose-response studies, the risk-disturbance hypothesis and the role of exposure context. J. Appl. Ecol. 55, 396–404. doi: 10.1111/1365-2664.12955
Harwood, J., King, S., Schick, R., Donovan, C., and Booth, C. (2014). A protocol for implementing the interim population consequences of disturbance (PCoD) approach: quantifying and assessing the effects of UK offshore renewable energy developments on marine mammal populations. Scott. Mar. Freshwater Sci. 5:97.
Hastie, G. D., Russell, D. J. F., Mcconnell, B., Moss, S., Thompson, D., and Janik, V. M. (2015). Sound exposure in harbour seals during the installation of an offshore wind farm: predictions of auditory damage. J. Appl. Ecol. 52, 631–640. doi: 10.1111/1365-2664.12403
Hastie, G., Merchant, N. D., Götz, T., Russell, D. J. F., Thompson, P. M., and Janik, V. M. (2019). Effects of impulsive noise on marine mammals: investigating range-dependent risk. Ecol. App. 29:e01906.
Hastings, M., and Popper, A. N. (2005). Effects of Sound on Fish (Report for California Department of Transportation). Sacramento, CA: Jones & Stokes.
Haver, S. M., Klinck, H., Nieukirk, S. L., Matsumoto, H., Dziak, R. P., and Miksis-Olds, J. L. (2017). The not-so-silent world: measuring arctic, equatorial, and antarctic soundscapes in the Atlantic Ocean. Deep Sea Res. Part I Oceanogr. Res. Papers 122, 95–104. doi: 10.1016/j.dsr.2017.03.002
Hawkins, A. D., and Popper, A. N. (2018). “Effects of man-made sound on fishes,” in Effects of Anthropogenic Noise on Animals, eds H. Slabbekoorn, R. J. Dooling, A. N. Popper, and R. R. Fay (New York, NY: Springer), 145–177. doi: 10.1007/978-1-4939-8574-6_6
Heimark, R. J., and Heimark, G. M. (1986). Southern elephant seal pupping at Palmer Station, Antarctica. J. Mammal. 67, 189–190. doi: 10.2307/1381022
Herr, H., Kelly, N., Dorschel, B., Huntemann, M., Kock, K.-H., Lehnert, L. S., et al. (2019). Aerial surveys for antarctic minke whales (Balaenoptera bonaerensis) reveal sea ice dependent distribution patterns. Ecol. Evol. 9, 5664–5682. doi: 10.1002/ece3.5149
Herr, H., Viquerat, S., Siegel, V., Kock, K.-H., Dorschel, B., Huneke, W. G. C., et al. (2016). Horizontal niche partitioning of humpback and fin whales around the west antarctic peninsula: evidence from a concurrent whale and krill survey. Pol. Biol. 39, 799–818. doi: 10.1007/s00300-016-1927-9
Hindell, M. A., McMahon, C. R., Bester, M. N., Boehme, L., Costa, D., Fedak, M. A., et al. (2016). Circumpolar habitat use in the southern elephant seal: implications for foraging success and population trajectories. Ecosphere 7:e01213. doi: 10.1002/ecs2.1213
Hofmeyr, G. J. G. (2015). Mirounga leonina. The IUCN Red List of Threatened Species, e.T13583A45227247. Available at: http://dx.doi.org/10.2305/IUCN.UK.2015-4.RLTS.T13583A45227247.en (accessed October 15, 2019).
Hofmeyr, G. J. G. (2016). Arctocephalus gazella. The IUCN Red List of Threatened Species e.T2058A66993062. Gland: IUCN Red List.
Holt, M. M., Noren, D. P., Veirs, V., Emmons, C. K., and Veirs, S. (2009). Speaking up: killer whales (Orcinus orca) increase their call amplitude in response to vessel noise. J. Acoust. Soc. Am. 125, El27–El32. doi: 10.1121/1.3040028
Holt, M., Noren, D., and Emmons, C. (2011). Effects of noise levels and call types on the source levels of killer whale calls. J. Acoust. Soc. Am. 130, 3100–3106. doi: 10.1121/1.3641446
Horton Sr, C. W. (1974). Dispersion relationships in sediments and sea water. J. Acoust. Soc. Am. 55, 547–549. doi: 10.1121/1.1914534
Horwood, J. W. (2009). “Sei whale - Balaenoptera borealis,” in Encyclopedia of Marine Mammals eds W. Perrin, B. Würsig, and J. Thewissen (Amsterdam, NL: Academic Press), 1001–1003.
Houser, D. S., Helweg, D. A., and Moore, P. W. B. (2001). A bandpass filter-bank model of auditory sensitivity in the humpback whale. Aqua. Mamm. 27, 82–91.
Hückstädt, L. (2015a). Lobodon carcinophaga. The IUCN Red List of Threatened Species, e.T12246A45226918. Gland: IUCN Red List.
Hückstädt, L. (2015b). Ommatophoca rossii. The IUCN Red List of Threatened Species, e.T15269A45228952. Available at: http://dx.doi.org/10.2305/IUCN.UK.2015-4.RLTS.T15269A45228952.en (accesssed October 15, 2019).
Hückstädt, L. (2015c). Leptonychotes weddellii. The IUCN Red List of Threatened Species, e.T11696A45226713. Gland: IUCN Red List.
Hückstädt, L., Burns, J., Koch, P., McDonald, B., Crocker, D. E., and Costa, D. (2012). Diet of a specialist in a changing environment: the crabeater seal along the western antarctic peninsula. Mar. Ecol. Prog. Ser. 455, 287–301. doi: 10.3354/meps09601
Illinworth and Rodkin Inc (2007). Compendium of Pile Driving Sound Data (Report for the California Department of Transportation). Cotati, CA: Illinworth and Rodkin Inc.
International Association of Antarctica Tour Operators [IAATO] (2018). “IAATO overview of antarctic tourism: 2017-18 season and preliminary estimates for 2018-19 season,” in Paper Presented at the XLI Antarctic Treaty Consultative Meeting, 13-18 May 2018, Buenos Aires.
International Maritime Organization (2009). Report of the 59th Session of IMO Marine Environment Protection Committee MEPC 59/24. London: International Maritime Organization.
International Whaling Commission (2001). Report of the workshop on the comprehensive assessment of right whales: a worldwide comparison. J. Cetacean Res. Manag. 2, 1–35.
Isojunno, S., Curé, C., Kvadsheim, P. H., Lam, F.-P. A., Tyack, P. L., Wensveen, P. J., et al. (2016). Sperm whales reduce foraging effort during exposure to 1–2 kHz sonar and killer whale sounds. Ecol. App. 26, 77–93. doi: 10.1890/15-0040
Janik, V. M. (2005). “Underwater acoustic communication networks in marine mammals,” in Animal Communication Networks, ed. P. K. McGregor (Cambridge, NY: Cambridge University Press), 390–415. doi: 10.1017/cbo9780511610363.022
Janik, V. M. (2014). Cetacean vocal learning and communication. Curr. Opin. Neurobiol. 28, 60–65. doi: 10.1016/j.conb.2014.06.010
Jelincic, J. A., Tift, M. S., Houser, D. S., and Crocker, D. E. (2017). Variation in adrenal and thyroid hormones with life-history stage in juvenile northern elephant seals (Mirounga angustirostris). Gen. Comp. Endocrinol. 252, 111–118. doi: 10.1016/j.ygcen.2017.08.001
Kasamatsu, F., and Joyce, G. G. (1995). Current status of odontocetes in the antarctic. Antarct. Sci. 7, 365–379. doi: 10.1017/S0954102095000514
Kasamatsu, F., Joyce, G. G., Ensor, P., and Mermoz, J. (1996). Current Occurrence of Baleen Whales in Antarctic Waters. Impington: International Whaling Commission.
Kastak, D., and Schusterman, R. J. (1999). In-air and underwater hearing sensitivity of a northern elephant seal (Mirounga angustirostris). Can. J. Zool. 77, 1751–1758. doi: 10.1139/z99-151
Kato, H., and Fujise, Y. (2000). Dwarf Minke Whales: Morphology, Growth and Life History with Some Analyses on Morphometric Variation Among the Different Forms and Regions. Impington: International Whaling Commission
Kenney, R. (2009). “Right whales - Eubalaena glacialis,” in Encyclopedia of Marine Mammals, eds E. Japonica, E. Australis, W. Perrin, B. Würsig, and J. Thewissen (Amsterdam, NL: Academic Press), 962–972.
Ketten, D. R. (1995). “Estimates of blast injury and acoustic trauma zones for marine mammals from underwater explosions,” in Sensory Systems of Aquatic Mammals, eds R. A. Kastelein, J. A. Thomas, and P. E. Nachtigall (Woerden: De Spil Publishing), 391–407.
Kipple, B. (2002). Southeast Alaska Cruise Ship Underwater Acoustic Noise. Report no. NSWCCD-71-TR-2002/574. Bremerton, WA: Naval Surface Warfare Center.
Knudsen, V. O., Alford, R. S., and Emling, J. W. (1948). Underwater ambient noise. J. Mar. Res. 7, 410–429.
Krysl, P., Cranford, T. W., Wiggins, S. M., and Hildebrand, J. A. (2006). Simulating the effect of high-intensity sound on cetaceans: modeling, approach and a case study for cuvier’s beaked whale (Ziphius cavirostris). J. Acoust. Soc. Am. 120, 2328–2339. doi: 10.1121/1.2257988
Kvadsheim, P. H., DeRuiter, S., Sivle, L. D., Goldbogen, J., Roland-Hansen, R., Miller, P. J. O., et al. (2017). Avoidance responses of minke whales to 1-4 kHz naval sonar. Mar. Pollut. Bull. 121, 60–68. doi: 10.1016/j.marpolbul.2017.05.037
Laist, D. W., Knowlton, A. R., Mead, J. G., Collet, A. S., and Podesta, M. (2001). Collisions between ships and whales. Mar. Mamm. Sci. 17, 35–75. doi: 10.1111/j.1748-7692.2001.tb00980.x
Lauriano, G., Fortuna, C. M., and Vacchi, M. (2007). Observation of killer whale (Orcinus orca) possibly eating penguins in terra nova bay. Antarct. Antarct. Sci. 19, 95–96. doi: 10.1017/S0954102007000132
Leaper, R., Bannister, J. L., Branch, T. A., Clapham, P. J., Donovan, G. P., Matsuoka, K., et al. (2008a). A Review of Abundance, Trends and Foraging Parameters of Baleen Whales in the Southern Hemisphere. Impington: International Whaling Commission.
Leaper, R., Calderan, S., and Cooke, J. (2015). A simulation framework to evaluate the efficiency of using visual observers to reduce the risk of injury from loud sound sources. Aqua. Mamm. 41, 375–387. doi: 10.1578/AM.41.4.2015.375
Leaper, R., Best, P., Branch, T., Donovan, G., Murase, H., and Waerebeek, K. (2008b). Report of Review Group of Data Sources on Odontocetes in the Southern Ocean in Preparation for IWC/CCAMLR Workshop in August 2008. Impington: International Whaling Commission.
Li, B., and Bayly, M. (2017). “Quantitative analysis on the environmental impact benefits from the bandwidth-controlled marine seismic source technology,” in Paper Presented at the Acoustics 2017, Perth, WA.
Lockyer, C. (1981). Growth and Energy Budgets of Large Baleen Whales From the Southern Hemisphere Mammals in the Seas (Vol. 3 - General Papers and Large Cetaceans. Rome: Food and Agriculture Organization of the United Nations.
Lowther, A. D. (2018). “Antarctic marine mammals,” in Encyclopedia of Marine Mammals, eds B. Würsig, J. G. M. Thewissen, and K. M. Kovacs (London: Academic Press), 27–32. doi: 10.1016/b978-0-12-804327-1.00047-9
Lucke, K., Scowcroft, G., Winter, H. V., Knowlton, C., Lam, F.-P. A., Hawkins, A., et al. (2016). International harmonization of approaches to define underwater noise exposure criteria and needs of the international regulatory community. Proc. Meet. Acoust. 27:070010. doi: 10.1121/2.0000287
MacLeod, C. D., Santos, M. B., and Pierce, G. J. (2003). Review of data on diets of beaked whales: evidence of niche separation and geographic segregation. J. Mar. Biol. Assoc. U K. 83, 651–665. doi: 10.1017/S0025315403007616h
Matsuoka, K., Hakamada, T., Kiwada, H., Murase, H., and Nishiwaki, S. (2006). Distribution and Standardized Abundance Estimates for Humpback, Fin and Blue Whales in the Antarctic Areas IIIE, IV, V and VIW (35 oE–145 oW), south of 60 oS. Impington: International Whaling Commission.
McCann, T. S., and Rothery, P. (1988). Population size and status of the southern elephant seal (Mirounga leonina) at South Georgia, 1951–1985. Pol. Biol. 8, 305–309. doi: 10.1007/bf00263179
McCauley, R. D., Day, R. D., Swadling, K. M., Fitzgibbon, Q. P., Watson, R. A., and Semmens, J. M. (2017). Widely used marine seismic survey air gun operations negatively impact zooplankton. Nat. Ecol. Evol. 1:0195. doi: 10.1038/s41559-017-0195
McCauley, R. D., Gavrilov, A. N., Jolliffe, C. D., Ward, R., and Gill, P. C. (2018). Pygmy blue and antarctic blue whale presence, distribution and population parameters in southern Australia based on passive acoustics. Deep Sea Res. Part II Top. Stud. Oceanogr. 154–168. doi: 10.1016/j.dsr2.2018.09.006
McGarry, T., Boisseau, O., Stephenson, S., and Compton, R. (2017). Understanding the Effectiveness of Acoustic Deterrent Devices (ADDs) on Minke Whale (Balaenoptera acutorostrata), a Low-Frequency Cetacean. Chepstow: The Carbon Trust.
Melcon, M., Cummins, A., Kerosky, S., Roche, L., Wiggins, S., and Hildebrand, J. (2012). Blue whales respond to anthropogenic noise. PLoS One 7:e32681. doi: 10.1371/journal.pone.0032681
Menze, S., Zitterbart, D. P., van Opzeeland, I., and Boebel, O. (2017). The influence of sea ice, wind speed and marine mammals on southern ocean ambient sound. R. Soc. Open Sci. 4:160370. doi: 10.1098/rsos.160370
Merck, T., Werner, S., Koschinski, S., and Lüdemann, K. (2014). OSPAR Inventory of Measures to Mitigate the Emission and Environmental Impact of Underwater Noise. London: OSPAR Commission.
Mikhalevsky, P. N. (2001). “Acoustics, arctic,” in Encyclopedia of Ocean Sciences, eds J. H. Steele, S. A. Thorpe, and K. K. Turekian (Amsterdam: Elsevier Science), 53–61. doi: 10.1006/rwos.2001.0314
Miller, P. J. O., Antunes, R. N., Wensveen, P. J., Samarra, F. I. P., Alves, A. C., Tyack, P. L., et al. (2014). Dose-response relationships for the onset of avoidance of sonar by free-ranging killer whales. J. Acoust. Soc. Am. 135:975. doi: 10.1121/1.4861346
Miller, P. J. O., Johnson, M. P., Madsen, P. T., Biassoni, N., Quero, M., and Tyack, P. L. (2009). Using at-sea experiments to study the effects of airguns on the foraging behavior of sperm whales in the Gulf of Mexico. Deep Sea Res. Part I Oceanogr. Res. Papers 56, 1168–1181. doi: 10.1016/j.dsr.2009.02.008
Miller, P. J. O., Kvadsheim, P. H., Lam, F.-P. A., Tyack, P. L., Cure, C., Deruiter, S. L., et al. (2015). First indications that northern bottlenose whales are sensitive to behavioural disturbance from anthropogenic noise. R. Soc. Open Sci. 2:140484. doi: 10.1098/rsos.140484
Monteiro, S., Ferreira, M., Vingada, J. V., López, A., Brownlow, A., and Méndez-Fernandez, P. (2015). Application of stable isotopes to assess the feeding ecology of long-finned pilot whale (Globicephala melas) in the Northeast Atlantic Ocean. J. Exp. Mar. Biol. Ecol. 465, 56–63. doi: 10.1016/j.jembe.2015.01.007
Moore, S., Reeves, R., Southall, B., Ragen, T., Suydam, R., and Clark, C. (2012). A new framework for assessing the effects of anthropogenic sound on marine mammals in a rapidly changing arctic. Biosience 62, 289–295. doi: 10.1525/bio.2012.62.3.10
Morton, A. B., and Symonds, H. K. (2002). Displacement of Orcinus orca (L.) by high amplitude sound in British Columbia. Can. ICES J. Mar. Sci. 59, 71–80. doi: 10.1006/jmsc.2001.1136
Murray, D. (1981). The breeding of the southern elephant seal, Mirounga leonina L, on the antartic continent. Pol. Record. 20, 370–371. doi: 10.1017/S0032247400018854
Natalie, R., Goodall, P., and Brownell, R. L. (2018). “Spectacled porpoise: Phocoena dioptrica,” in Encyclopedia of Marine Mammals, eds B. Würsig, J. G. M. Thewissen, and K. M. Kovacs (Cambridge, MA: Academic Press), 912–916. doi: 10.1016/B978-0-12-804327-1.00240-5
National Academies of Sciences, Engineeering and Medicine (2017). Approaches to Understanding the Cumulative Effects of Stressors on Marine Mammals. Washington, DC: National Academies of Sciences, Engineeering and Medicine
National Marine Fisheries Service (2016). Technical Guidance for Assessing the Effects of Anthropogenic Sound on Marine Mammal Hearing: Underwater Acoustic Thresholds for Onset of Permanent and Temporary Threshold Shifts (Technical Memorandum No. NMFS-OPR-55). Silver Spring, MD: U.S. Department of Commerce, National Oceanic and Atmospheric Administration (NOAA).
National Marine Fisheries Service (2018). 2018 Revisions to: Technical Guidance for Assessing the Effects of Anthropogenic Sound on Marine Mammal Hearing (Version 2.0): Underwater Thresholds for Onset of Permanent and Temporary Threshold Shifts (Technical Memorandum No. NMFS-OPR-59). Silver Spring, MD: U.S. Department of Commerce, National Oceanic and Atmospheric Administration.
National Research Council (2005). Marine Mammal Populations and Ocean Noise: Determining When Noise Causes Biologically Significant Effects. Washington, DC: National Academies Press.
New, L. F., Clark, J. S., Costa, D. P., Fleishman, E., Hindell, M. A., Klanjcek, T., et al. (2014). Using short-term measures of behaviour to estimate long-term fitness of southern elephant seals. Mar. Ecol. Prog. Ser. 496, 99–108. doi: 10.3354/meps10547
Nowacek, D. P., Thorne, L. H., Johnston, D. W., and Tyack, P. L. (2007). Responses of cetaceans to anthropogenic noise. Mamm. Rev. 37, 81–115. doi: 10.1111/j.1365-2907.2007.00104.x
Pacini, A. F., Nachtigall, P. E., Kloepper, L. N., Linnenschmidt, M., Sogorb, A., and Matias, S. (2010). Audiogram of a formerly stranded long-finned pilot whale (Globicephala melas) measured using auditory evoked potentials. J. Exp. Biol. 213, 3138–3143. doi: 10.1242/Jeb.044636
Parks, S. E., Ketten, D. R., O’Malley, J. T., and Arruda, J. (2007). Anatomical predictions of hearing in the north Atlantic right whale. Anat. Rec. Adv. Integr. Anat. Evol. Biol. 290, 734–744. doi: 10.1002/Ar.20527
Pastene, L. A., Goto, M., Nishiwaki, S., Yoshida, H., and Kanda, N. (2006). Genetic Characteristics and Population Structure of Humpback Whales in the Antarctic Feeding Ground as Revealed by Mitochondrial DNA Control Region Sequencing and Microsatellite Analyses. Impington: International Whaling Commission.
Pauly, D., Trites, A. W., Capuli, E., and Christensen, V. (1998). Diet composition and trophic levels of marine mammals. ICES J. Mar. Sci. 55, 467–481. doi: 10.1006/jmsc.1997.0280
Pavan, G. (2006). Guidelines to Address the Issue of the Impact of Anthropogenic Noise on Marine Mammals in the ACCOBAMS area (Report to the 3rd meeting of the ACCOBAMS Scientific Committee). Monaco: ACCOBAMS Secretariat.
Perrin, W. F., and Brownell, R. L. (2009). “Minke whales - Balaenoptera acutorostrata and bonaerensis,” in Encyclopedia of Marine Mammals, eds B. Perrin, W. B. Würsig, and J. Thewissen (Amsterdam, NL: Academic Press), 733–735. doi: 10.1016/b978-0-12-373553-9.00170-x
Piatkowski, U., Vergani, D. F., and Stanganelli, Z. B. (2002). Changes in the cephalopod diet of southern elephant seal females at King George Island, during El Niño-La Niña events. J. Mar. Biol. Assoc. U.K. 82, 913–916. doi: 10.1017/S0025315402006343
Pitman, R. L., and Durban, J. W. (2010). Killer whale predation on penguins in antarctica. Pol. Biol. 33, 1589–1594. doi: 10.1007/s00300-010-0853-5
Pitman, R. L., and Durban, J. W. (2012). Cooperative hunting behavior, prey selectivity and prey handling by pack ice killer whales (Orcinus orca), type B, in antarctic peninsula waters. Mar. Mamm. Sci. 28, 16–36. doi: 10.1111/j.1748-7692.2010.00453.x
Pitman, R. L., and Ensor, P. (2003). Three forms of killer whales (Orcinus orca) in Antarctic waters. J. Cetacean Res. Manag. 5, 131–140. doi: 10.1098/rsbl.2008.0168
Popper, A. N., and Hawkins, A. (eds) (2016). The Effects of Noise on Aquatic Life II. New York, NY: Springer Verlag.
Reichmuth, C., and Casey, C. (2014). Vocal learning in seals, sea lions, and walruses. Curr. Opin. Neurobiol. 28, 66–71. doi: 10.1016/j.conb.2014.06.011
Reilly, S., Hedley, S., Borberg, J., Hewitt, R., Thiele, D., Watkins, J., et al. (2004). Biomass and energy transfer to baleen whales in the South Atlantic sector of the Southern Ocean. Deep Sea Res. Part II Top. Stud. Oceanogr. 51, 1397–1409. doi: 10.1016/j.dsr2.2004.06.008
Rodríguez, J. P., Fernández-Gracia, J., Thums, M., Hindell, M. A., Sequeira, A. M. M., Meekan, M. G., et al. (2017). Big data analyses reveal patterns and drivers of the movements of southern elephant seals. Sci. Rep. 7:112. doi: 10.1038/s41598-017-00165-0
Rogers, T. L. (2009). “Leopard seal - Hydrurga leptonyx,” in Encyclopedia of Marine Mammals, eds W. Perrin, B. Würsig, and J. Thewissen (Amsterdam: Academic Press), 673–674.
Rolland, R. M., Parks, S. E., Hunt, K. E., Castellote, M., Corkeron, P. J., Nowacek, D. P., et al. (2012). Evidence that ship noise increases stress in right whales. Proc. R. Soc. Lond. Ser. B Biol. Sci. 279, 2363–2368. doi: 10.1098/rspb.2011.2429
Ropert-Coudert, Y., Hindell, M. A., Phillips, R., Charrassin, J. B., Trudelle, L., and Raymond, B. (2014). “Biogeographic patterns of birds and mammals,” in Biogeographic Atlas of the Southern Ocean, eds C. de Broyer, P. Koubbi, H. Griffiths, B. Raymond, C. D. d’Udekem d’Acoz, A. van de Putte, et al. (Cambridge: The Scientific Committee on Antarctic Research), 364–387.
Roth, E. H., Schmidt, V., Hildebrand, J. A., and Wiggins, S. M. (2013). Underwater radiated noise levels of a research icebreaker in the central Arctic Ocean. J. Acoust. Soc. Am. 133, 1971–1980. doi: 10.1121/1.4790356
Rounsevell, D., and Eberhard, I. (1980). Leopard Seals, Hydrurga leptonyx (Pinnipedia), at Macquarie Island from 1949 to 1979. Wildlife Res. 7, 403–415. doi: 10.1071/WR9800403
Rudd, M. A. (2014). Scientists’ perspectives on global ocean research priorities. Front. Mar. Sci. 1:36. doi: 10.3389/fmars.2014.00036
Russell, D. J. F., Hastie, G. D., Thompson, D., Janik, V. M., Hammond, P. S., Scott-Hayward, L. A. S., et al. (2016). Avoidance of wind farms by harbour seals is limited to pile driving activities. J. Appl. Ecol. 53, 1642–1652. doi: 10.1111/1365-2664.12678
Santos, M. B., Monteiro, S. S., Vingada, J. V., Ferreira, M., López, A., Martinez Cedeira, J. A., et al. (2014). Patterns and trends in the diet of long-finned pilot whales (Globicephala melas) in the northeast atlantic. Mar. Mamm. Sci. 30, 1–19. doi: 10.1111/mms.12015
Secchi, E. R., Barcellos, L., Zerbini, A. N., and Rosa, L. D. (2003). Biological obeservations on a dwarf minke whale, Balaenoptera acutorostrata, caught in southern Brazilian waters, with a new record of prey for the species. Latin Am. J. Aqua. Mamm. 2, 109–115.
Sekiguchi, K., Klages, N. T. W., and Best, P. B. (1996). The diet of strap-toothed whales (Mesoplodon layardii). J. Zool. 239, 453–463. doi: 10.1111/j.1469-7998.1996.tb05935.x
Sekiguchi, K., Olavarria, C., Morse, L., Olson, P., Ensor, P., Matsuoka, K., et al. (2006). The spectacled porpoise (Phocoena dioptrica) in antarctic waters. J. Cetacean Res. Manag. 8, 265–271.
Shannon, G., McKenna, M. F., Angeloni, L. M., Crooks, K. R., Fristrup, K. M., Brown, E., et al. (2016). A synthesis of two decades of research documenting the effects of noise on wildlife. Biol. Rev. 91, 982–1005. doi: 10.1111/brv.12207
Siebert, U., Dähne, M., Danehl, S., Meyer-Klaeden, O., van Neer, A., Wittekind, D., et al. (2014). Assessment of Potential for Masking in Marine Mammals of the Antarctic Exposed to Underwater Sound From Airguns (Report by the Institute for Terrestrial and Aquatic Wildlife Research, University of Veterinary Medicine Hannover). Dessau: Umweltbundesamt Deutschland.
Siniff, D. B. (2015). An overview of the ecology of antarctic seals. Am. Zool. 31, 143–149. doi: 10.1093/icb/31.1.143
Sirovic, A., Hildebrand, J. A., Wiggins, S. M., and Thiele, D. (2009). Blue and fin whale acoustic presence around Antarctica during 2003 and 2004. Mar. Mamm. Sci. 25, 125–136. doi: 10.1111/j.1748-7692.2008.00239.x
Sirovic, A., Hildebrand, J. A., Wiggins, S. M., McDonald, M. A., Moore, S. E., and Thiele, D. (2004). Seasonality of blue and fin whale calls and the influence of sea ice in the western antarctic peninsula. Deep Sea Res. Part II Top. Stud. Oceanogr. 51, 2327–2344. doi: 10.1016/j.dsr2.2004.08.005
Sivle, L. D., Kvadsheim, P. H., Cure, C., Isojunno, S., Wensveen, P. J., Lam, P. A., et al. (2015). Severity of expert-identified behavioural responses of humpback whale, minke whale, and northern bottlenose whale to naval sonar. Aqua. Mamm. 41, 469–502. doi: 10.1578/AM.41.4.2015.469
Sivle, L. D., Wensveen, P. J., Kvadsheim, P. H., Lam, F. P. A., Visser, F., Curé, C., et al. (2016). Naval sonar disrupts foraging in humpback whales. Mar. Ecol. Prog. Ser. 562, 211–220. doi: 10.3354/meps11969
Skaug, H. J., Gjøsaeter, H., Haug, T., Lindstrøm, U., and Nilssen, K. (1997). Do minke whales (Balaenoptera acutorostrata) exhibit particular prey preferences? J. Northwest Atlantic Fish. Sci. 22, 91–104. doi: 10.2960/j.v22.a8
Soloway, A. G., and Dahl, P. H. (2014). Peak sound pressure and sound exposure level from underwater explosions in shallow water. J. Acoust. Soc. Am. 136, EL218–EL223. doi: 10.1121/1.4892668
Southall, B. L., DeRuiter, S. L., Friedlaender, A., Stimpert, A. K., Goldbogen, J. A., Hazen, E., et al. (2019a). Behavioral responses of individual blue whales (Balaenoptera musculus) to mid-frequency military sonar. J. Exp. Biol. 222:jeb190637. doi: 10.1242/jeb.190637
Southall, B. L., Finneran, J. J., Reichmuth, C., Nachtigall, P. E., Ketten, D. R., Bowles, A. E., et al. (2019b). Marine mammal noise exposure criteria: updated scientific recommendations for residual hearing effects. Aqua. Mamm. 45, 125–232. doi: 10.1578/AM.45.2.2019.125
Southwell, C., Bengtson, J., Bester, M., Schytte-Blix, A., Bornemann, H., Boveng, P., et al. (2012). A review of data on abundance, trends in abundance, habitat utilisation and diet for southern ocean ice-breeding seals. CCAMLR Sci. 19, 1–49.
Stimpert, A. K., Deruiter, S. L., Southall, B. L., Moretti, D. J., Falcone, E. A., Goldbogen, J. A., et al. (2014). Acoustic and foraging behavior of a Baird’s beaked whale, Berardius bairdii, exposed to simulated sonar. Sci. Rep. 4:7031. doi: 10.1038/srep07031
Stone, C. J., and Tasker, M. L. (2006). The effects of seismic airguns on cetaceans in UK waters. J. Cetacean Res. Manag. 8, 255–263.
Szymanski, M. D., Bain, D. E., Kiehl, K., Pennington, S., Wong, S., and Henry, K. R. (1999). Killer whale (Orcinus orca) hearing: auditory brainstem response and behavioral audiograms. J. Acoust. Soc. Am. 106, 1134–1141. doi: 10.1121/1.427121
Thiele, D., and Gill, P. C. (1999). Cetacean observations during a winter voyage into antarctic sea ice south of Australia. Antarct. Sci. 11, 48–53. doi: 10.1017/S0954102099000073
Thiele, D., Chester, E. T., Moore, S. E., Širovic, A., Hildebrand, J. A., and Friedlaender, A. S. (2004). Seasonal variability in whale encounters in the western antarctic peninsula. Deep Sea Res. Part II Top. Stud. Oceanogr. 51, 2311–2325. doi: 10.1016/j.dsr2.2004.07.007
Thomisch, K., Boebel, O., Clark, C. W., Hagen, W., Spiesecke, S., Zitterbart, D. P., et al. (2016). Spatio-temporal patterns in acoustic presence and distribution of antarctic blue whales Balaenoptera musculus intermedia in the Weddell Sea. Endanger. Spec. Res. 30, 239–253. doi: 10.3354/esr00739
Tubelli, A., Zosuls, A., Ketten, D., Yamato, M., and Mountain, D. (2012). A prediction of the minke whale (Balaenoptera acutorostrata) middle-ear transfer function. J. Acoust. Soc. Am. 132, 3263–3272. doi: 10.1121/1.4756950
Tynan, C. T. (1998). Ecological importance of the southern boundary of the antarctic circumpolar current. Nature 392, 708–710. doi: 10.1038/33675
United Nations Economic Commission for Europe [UNECE] (1991). Convention on Environmental Impact Assessment in a Transboundary Context. Espoo: United Nations Economic Commission for Europe.
van den Hoff, J., Burton, H., and Raymond, B. (2007). The population trend of southern elephant seals (Mirounga leonina L.) at Macquarie Island (1952–2004). Pol. Biol. 30, 1275–1283. doi: 10.1007/s00300-007-0288-9
van der Graaf, A. J., Ainslie, M. A., Andre, M., Brensing, K., Dalen, J., Dekeling, R. P. A., et al. (2012). European Marine Strategy Framework Directive–Good Environmental Status (MSFD GES): Report of the Technical Subgroup on Underwater noise and other forms of energy (JRC Scientific and Technical Report). Brussels: TSG Noise & Milieu Ltd.
Van Opzeeland, I., and Boebel, O. (2018). Marine soundscape planning: seeking acoustic niches for anthropogenic sound. J. Ecoacous. 2:5GSNT8. doi: 10.22261/JEA.5GSNT8
Van Opzeeland, I., Kindermann, L., Boebel, O., and Van Parijs, S. (2008). “Insights into the acoustic behaviour of polar pinnnipeds – current knowledge and emerging techniques of study,” in Animal Behaviour: New Research, eds E. A. Weber and L. H. Krause (Hauppage, NY: Nova Science Publishers).
van Opzeeland, I., van Parijs, S., Bornemann, H., Frickenhaus, S., Kindermann, L., Klinck, H., et al. (2010). Acoustic ecology of Antarctic pinnipeds. Mar. Ecol. Prog. Ser. 414, 267–291. doi: 10.3354/Meps08683
van Opzeeland, I., van Parijs, S., Kindermann, L., Burkhardt, E., and Boebel, O. (2013). Calling in the cold: pervasive acoustic presence of humpback whales (Megaptera novaeangliae) in antarctic coastal waters. PLoS One 8:e73007. doi: 10.1371/journal.pone.0073007
Van Parijs, S. M., Clark, C. W., Sousa-Lima, R. S., Parks, S. E., Rankin, S., Risch, D., et al. (2009). Management and research applications of real-time and archival passive acoustic sensors over varying temporal and spatial scales. Mar. Ecol. Prog. Ser. 395, 21–36. doi: 10.3354/Meps08123
van Waerebeek, K., Leaper, R., Baker, A. N., Papastavrou, V., and Thiele, D. (2010). Odontocetes of the southern ocean sanctuary. J. Cetacean Res. Manag. 11, 315–346.
Verfuss, U. K., Sparling, C. E., Arnot, C., Judd, A., and Coyle, M. (2016). “Review of offshore wind farm impact monitoring and mitigation with regard to marine mammals,” in The Effects of Noise on Aquatic Life II, eds A. N. Popper and A. Hawkins (New York, NY: Springer Verlag), 1175–1182. doi: 10.1007/978-1-4939-2981-8_147
Visser, F., Curé, C., Kvadsheim, P. H., Lam, F.-P. A., Tyack, P. L., and Miller, P. J. O. (2016). Disturbance-specific social responses in long-finned pilot whales, Globicephala melas. Sci. Rep. 6:28641. doi: 10.1038/srep28641
Wales, S. C., and Heitmeyer, R. M. (2002). An ensemble source spectra model for merchant ship-radiated noise. J. Acoust. Soc. Am. 111, 1211–1231. doi: 10.1121/1.1427355
Weir, C. R., and Dolman, S. J. (2007). Comparative review of the regional marine mammal mitigation guidelines implemented during industrial seismic surveys, and guidance towards a worldwide standard. J. Int. Wildlife Law Policy 10, 1–27. doi: 10.1080/13880290701229838
Whitehead, H. (2002). Estimates of the current global population size and historical trajectory for sperm whales. Mar. Ecol. Prog. Ser. 242, 295–304. doi: 10.3354/meps242295
Whitehead, H. (2009). “Sperm whale - Physeter macrocephalus,” in Encyclopedia of Marine Mammals, eds W. Perrin, B. Würsig, and J. Thewissen (Amsterdam: Academic Press), 1091–1097.
Williams, R., Erbe, C., Ashe, E., Beerman, A., and Smith, J. (2014a). Severity of killer whale behavioural responses to ship noise: a dose-response study. Mar. Pollut. Bull. 79, 254–260. doi: 10.1016/j.marpolbul.2013.12.004
Williams, R., Kelly, N., Boebel, O., Friedlaender, A. S., Herr, H., Kock, K. H., et al. (2014b). Counting whales in a challenging, changing environment. Sci.Rep. 4:4170. doi: 10.1038/srep04170
Williams, R., Wright, A. J., Ashe, E., Blight, L. K., Bruintjes, R., Canessa, R., et al. (2015). Impacts of anthropogenic noise on marine life: publication patterns, new discoveries, and future directions in research and management. Ocean Coastal Manag. 115, 17–24. doi: 10.1016/j.ocecoaman.2015.05.021
Yang, T. C. (1984). Dispersion and ranging of transient signals in the Arctic Ocean. J. Acoust. Soc. Am. 76, 262–273. doi: 10.1121/1.391103
Zerbini, A. N., Ward, E., Engel, M. H., Andriolo, A., and Kinas, P. G. (2006). A Bayesian Assessment of the Conservation Status of Humpback Whales (Megaptera novaeangliae) in the Western South Atlantic Ocean (Breeding Stock A). Reports, No. SC/58/SH52, 01-25. Cambridge: International Whaling Commission.
Keywords: underwater noise, Antarctica, marine mammal, Antarctic Treaty, ship, seismic survey, noise management
Citation: Erbe C, Dähne M, Gordon J, Herata H, Houser DS, Koschinski S, Leaper R, McCauley R, Miller B, Müller M, Murray A, Oswald JN, Scholik-Schlomer AR, Schuster M, Van Opzeeland IC and Janik VM (2019) Managing the Effects of Noise From Ship Traffic, Seismic Surveying and Construction on Marine Mammals in Antarctica. Front. Mar. Sci. 6:647. doi: 10.3389/fmars.2019.00647
Received: 08 April 2019; Accepted: 02 October 2019;
Published: 06 November 2019.
Edited by:
Lyne Morissette, M – Expertise Marine, CanadaReviewed by:
Benjamin de Montgolfier, Independent Researcher, Sainte-Luce, MartiniqueChristopher W. Clark, Cornell University, United States
Copyright © 2019 Erbe, Dähne, Gordon, Herata, Houser, Koschinski, Leaper, McCauley, Miller, Müller, Murray, Oswald, Scholik-Schlomer, Schuster, Van Opzeeland and Janik. This is an open-access article distributed under the terms of the Creative Commons Attribution License (CC BY). The use, distribution or reproduction in other forums is permitted, provided the original author(s) and the copyright owner(s) are credited and that the original publication in this journal is cited, in accordance with accepted academic practice. No use, distribution or reproduction is permitted which does not comply with these terms.
*Correspondence: Christine Erbe, Yy5lcmJlQGN1cnRpbi5lZHUuYXU=