- 1U.S. Geological Survey, Louisiana Fish and Wildlife Cooperative Research Unit, School of Renewable Natural Resources, Louisiana State University Agricultural Center, Baton Rouge, LA, United States
- 2School of Renewable Natural Resources, Louisiana State University Agricultural Center, Baton Rouge, LA, United States
- 3Department of Fisheries, Animal and Veterinary Sciences, University of Rhode Island, Kingston, RI, United States
- 4Graduate School of Oceanography, University of Rhode Island, Narragansett, RI, United States
Oyster reefs provide habitat for numerous fish and decapod crustacean species that mediate ecosystem functioning and support vibrant fisheries. Recent focus on the restoration of eastern oyster (Crassostrea virginica) reefs stems from this role as a critical ecosystem engineer. Within the shallow estuaries of the northern Gulf of Mexico (nGoM), the eastern oyster is the dominant reef building organism. This study synthesizes data on fish and decapod crustacean occupancy of oyster reefs across nGoM with the goal of providing management and restoration benchmarks, something that is currently lacking for the region. Relevant data from 23 studies were identified, representing data from all five U.S. nGoM states over the last 28 years. Cumulatively, these studies documented over 120,000 individuals from 115 fish and 41 decapod crustacean species. Densities as high as 2,800 ind m−2 were reported, with individual reef assemblages composed of as many as 52 species. Small, cryptic organisms that occupy interstitial spaces within the reefs, and sampled using trays, were found at an average density of 647 and 20 ind m−2 for decapod crustaceans and fishes, respectively. Both groups of organisms were comprised, on average, of 8 species. Larger-bodied fishes captured adjacent to the reef using gill nets were found at an average density of 6 ind m−2, which came from 23 species. Decapod crustaceans sampled with gill nets had a much lower average density, <1 ind m−2, and only contained 2 species. On average, seines captured the greatest number of fish species (n = 33), which were made up of both facultative residents and transients. These data provide general gear-specific benchmarks, based on values currently found in the region, to assist managers in assessing nekton occupancy of oyster reefs, and assessing trends or changes in status of oyster reef associated nekton support. More explicit reef descriptions (e.g., rugosity, height, area, adjacent habitat) would allow for more precise benchmarks as these factors are important in determining nekton assemblages, and sampling efficiency.
Introduction
Understanding the impacts of habitat change on natural resources remains a key component for informing restoration and management policy (Barbier et al., 2011; Bennett et al., 2015). Fisheries policy in particular, through the 1996 amendment to the U.S. Magnuson-Stevens Act (passed 1976), introduced a mandate on defining and protecting Essential Fish Habitat, which include “all waters and substrate necessary to fish for spawning, breeding, feeding, or growth to maturity” (Magnuson Stevens Fishery Management Conservation Act, 1996, 16 U.S.C. section 1801–1804). This placed high importance on species-habitat associations (Magnuson Stevens Fishery Management Conservation Act, 1996: 50 CFR, sections 600.805–930). Since then, data on habitat-specific fisheries and associated species have been used to justify, inform, and guide policy and management activities (Rondinini and Chiozza, 2010; Vasconcelos et al., 2015; NRC, 2017). Thus, documenting species richness and assemblages within a habitat type is a first crucial step to defining habitat support and ensuring collection of proper baseline data. These baseline data can be used to support modeling, evaluate restoration outcomes (Dufrêne and Legendre, 1997; NRC, 2017), and, as highlighted recently, to evaluate resource injury (i.e., under the U.S. Oil Pollution Act, 19901, section 1006I(1) for coastal resources).
Reefs built by the eastern oyster, Crassostrea virginica, have been recognized as Essential Fish Habitat (Coen and Grizzle, 2007). Historically valued for their economic impact as a direct food commodity, oysters also create reefs, providing valuable three-dimensional habitat within coastal environments. In recent decades, significant efforts to conserve, and restore oyster reefs have been justified based on the valuable ecosystem services they provide, including water quality improvements, shoreline protection, and habitat creation for commercially and recreationally important fisheries (Coen and Grizzle, 2007; Grabowski et al., 2012). Importantly, for fisheries and restoration policy, oyster reefs are recognized as key biogenic habitat for a diverse assemblage of fishes and decapod crustaceans (e.g., Mobius, 1877; Frey, 1946; Wells, 1961; Coen et al., 1999; Coen and Grizzle, 2007, 2016). The reported functional decline in oyster reefs (Beck et al., 2011; Zu Ermgassen et al., 2012) is likely to have broad consequences for habitat provision, and thus biodiversity and fisheries production (Peterson et al., 2003; Humphries and La Peyre, 2015; Zu Ermgassen et al., 2015), but a lack of established benchmarks hinders our ability to assess impacts.
Recent guidelines for monitoring oyster reefs suggest setting explicit goals for assessing habitat support (Baggett et al., 2015; NRC, 2017). Goals for restoration projects may focus simply on provision of habitat (i.e., La Peyre et al., 2014a; Baggett et al., 2015), or, they may be expanded to include local enhancement of ecosystem services (i.e., Coen and Luckenbach, 2000). Central to either of these goals, however, is an understanding of what the nekton assemblages look like on the desired habitat in terms of expected assemblages, abundance, and biomass of species. As such, many oyster reef restoration planning documents call for the use of project-specific reference sites (Coen et al., 2004; SER Society for Ecological Restoration, 2004; Baggett et al., 2015; NRC, 2017); however, such sites are often not available, or monitoring efforts fail to collect these data. An alternative is to establish desirable conditions, or benchmarks to measure changes in resource status (Ehrenfeld, 2000; Kentula, 2000).
Benchmarks provide a tool to assess the status of natural, managed, or restored ecosystems (Angermeier and Karr, 1994; McClanahan et al., 2019). Ideally, benchmarks are set using baseline data derived from natural or pristine systems, which are defined by functional and evolutionary limits of the ecosystem (Pickett et al., 1992). For many resources and regions, effects of altered landscapes (e.g., river management, climate change), and a lack of historical data confound efforts to set benchmarks representing pristine conditions (Toledo et al., 2011). Lacking these historical data, useful benchmarks can still be established for current ecosystem status against which future assessments of natural resource condition or management effects can be measured. Across the northern Gulf of Mexico (nGoM), significant coastal restoration, river management, and climate change impacts have affected local hydrology, water quality, and landscape configurations (Pendleton et al., 2010; Montagna et al., 2011). These changes alter oyster population dynamics and reef characteristics which ultimately impacts the oyster reef's provision of ecosystem services, including nekton habitat. Benchmarks representing reef-resident and transient assemblages based on the current range or means of values from recent assessments would provide useful tools to assess changes in status of natural reefs, as well as managed and restored reefs.
The nGoM supports extensive natural oyster reefs (Kilgen and Dugas, 1989), and recently has experienced significant restoration activities related to oyster reefs, with a goal of providing fisheries habitat (La Peyre et al., 2014a). Reports from the Atlantic coast of the United States document occupancy of eastern oyster reefs by 50 to over 300 species, with the wide range of reported species depending on whether the surveys include fish, decapod crustaceans, molluscs or other organisms, such as protozoans, sponges and flatworms (e.g., Frey, 1946; Wells, 1961; Coen and Grizzle, 2007). Similar data do not exist for the nGoM, despite the exceptional fisheries support and habitat characteristics of the region. For example, the nGoM is characterized by a microtidal regime (<0.5 m) where water level changes are driven mostly by winds, precipitation, and runoff (Kim and Park, 2012). As such, depth and exposure of Gulf coast oyster reefs can vary and be difficult to predict (Kilgen and Dugas, 1989). Importantly, water depth and substrate or reef exposure influence reef formation, which impacts oyster population dynamics (Ridge et al., 2015). Oysters in the nGoM have higher growth rates and reduced mortality rates compared to other regions, despite experiencing lower salinity, and higher temperatures than other regions (Lowe et al., 2017). These differences in reef formation, water quality, and growth characteristics likely influence the associated nekton communities, suggesting a need for region specific benchmarks.
This study aims to quantify fish and decapod crustacean assemblages associated with nGoM oyster reefs. The goal is to provide benchmarks using available data collected on nekton occupancy across a broad spectrum of oyster reefs. These benchmarks will be useful as a guideline to assess policy and restoration outcomes in the nGoM, as well as inform future injury assessments (Geist and Hawkins, 2016). These needs have been identified in previous studies and planning documents and represent a critical information gap for assessing restoration and policy goals (Baggett et al., 2015; Coen and Humphries, 2017; NRC, 2017).
Methods
Peer Reviewed Study Selection
A literature search for fish and decapod crustacean sampling on oyster reefs across the nGoM was conducted by searching Google Scholar using the following search terms: “oyster reef” AND (Texas OR Louisiana OR Mississippi OR Alabama OR Florida) AND (macrofauna OR nekton), for all papers dated through April 2019. Only peer-reviewed, regional nGoM shallow water (<3 m) studies that provided oyster reef location, sample size, sample methods (i.e., gear), species identities, and density information were included in the final database. Also, only studies targeting oyster reef fish and/or decapod crustacean communities (and not individual species focused studies) were included for the targeted evaluation of community composition, and not specific species.
Nekton Variables
Nekton variables were collected by gear type, and standardized across studies. To address the issue of unequal sampling effort, all nekton density data were standardized to individuals m−2 for all gear types, except gill net, which was standardized to individuals hr−1 of net soak time. Sampling effort was calculated by multiplying the number of samples taken by either the total area sampled, or total hours fished.
Reef and Water Quality Characteristics
Variables describing reef characteristics and water quality, where available, were added to the database. Data availability was examined for tidal height, reef elevation, exposure, and water quality for selected study reefs and locations. Water quality data for salinity, temperature (°C), and dissolved oxygen (mg L−1) were the only three variables consistently available. We recorded reef type (natural vs. constructed) as there were few data sets for natural reefs, and using data from only natural reefs resulted in a very limited database. For the purposes of this study, we define natural reefs as reefs that had not, to the best of our knowledge, been anthropogenically enhanced, restored, or created in any way. We define “constructed” reefs as those that were anthropogenically enhanced, restored, or created using shell or other materials such as rock or cement with the goal of reef restoration and not oyster production for human consumption. Reefs were classified as intertidal or subtidal based on information from the original studies. Any reef not explicitly identified as intertidal was classified as subtidal, although we acknowledge that some subtidal reefs in this region are occasionally exposed from weather events (i.e., La Peyre et al., 2017).
Final Database
The final database included density or catch per unit effort (CPUE) of fish and decapod crustaceans, number of fish and decapod crustacean species, list of species collected, sample size, sampling gear, reef type (natural, constructed), tidal status (intertidal vs. subtidal), and a few discrete environmental parameters (water temperature, salinity, dissolved oxygen).
Data are presented and discussed using statistical means and distributions by gear type. We use the means for fish and decapod crustacean density and species number separately, by dominant gear type quantifying “on-reef” and “near reef” values to suggest potential benchmarks based on the range and means of values currently found across the nGoM (R 3.5.1; R Core Team, 2018).
Results
From a total of 1,072 search results, 23 articles were selected for this analysis based on their overall goals and data availability (Figure 1; Table 1). The majority of studies were excluded because they focused on a region other than the Gulf of Mexico, the study was not related to oyster reefs, or data were not reported for all species. Within the Gulf of Mexico, there were many studies examining various aspects of fisheries, nekton production, and trophic changes on oyster reefs, but they failed to meet our criteria for inclusion in the final dataset. A number of studies focused exclusively on indicator species, economically important species, a subset of the reef community, or failed to report all catch data. While valuable, such studies were not included.
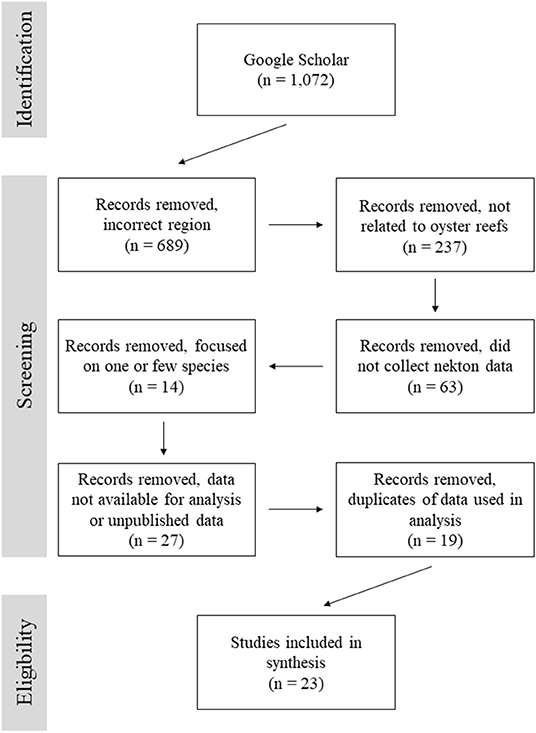
Figure 1. Flow diagram for records search documenting number of records identified and screened for inclusion in this data review.
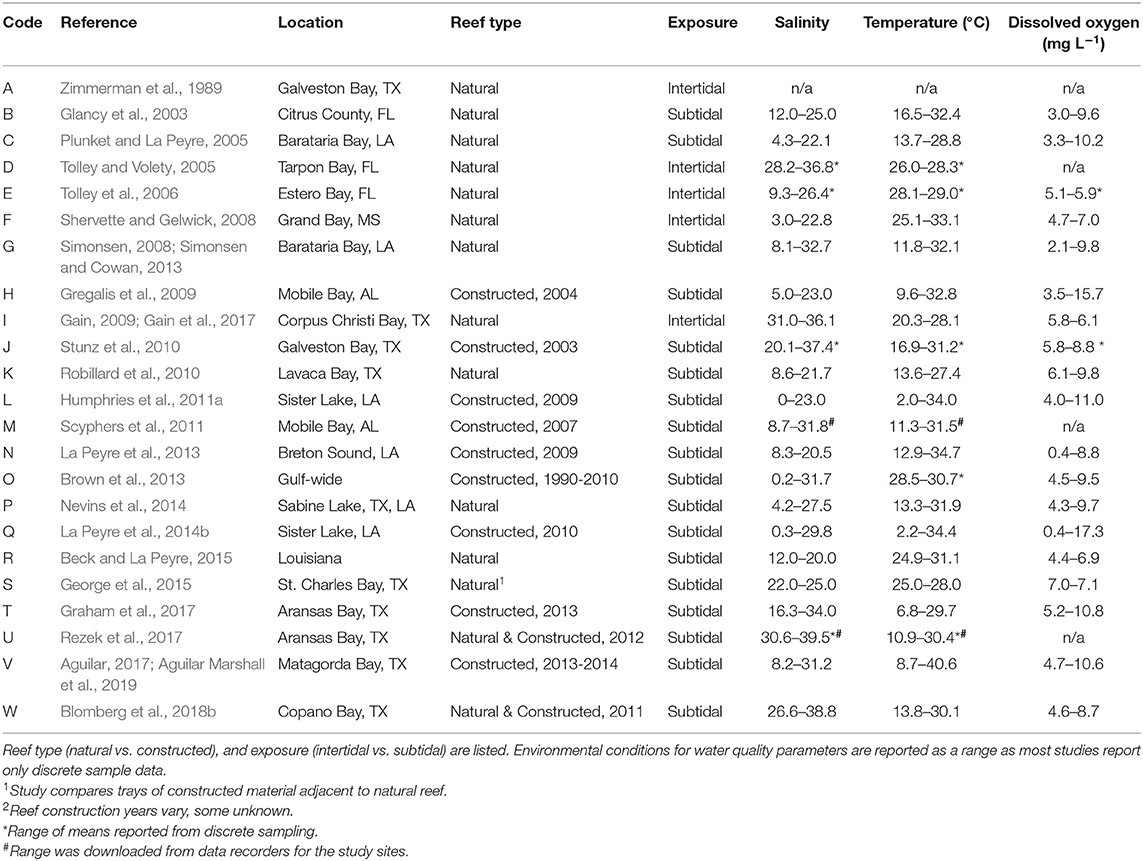
Table 1. List of studies identified as sampling for nekton assemblages on oyster reefs within estuaries across the northern Gulf of Mexico.
The studies selected for analyses covered all five Gulf Coast states and used multiple gear types. Texas had the most data (9 studies), followed by Louisiana (6 studies), Florida (3 studies), Alabama (2 studies), and Mississippi (1 study), and 2 studies covered multiple states (Figure 2). Across the 23 studies, seven different gear types were used with most studies using more than one gear type on a single oyster reef. Trays (TR) were the most frequently used gear type (n = 12). Gill nets (GN) were the next most used gear type (n = 8), although the lengths, mesh sizes and soak times varied. Drop-samplers (DS), throw traps (TT), and lift nets (LN) were grouped in one category (DS/TT/LN; n = 8). Seines (SN; n = 4) and epibenthic sleds (ES; n = 3) represented the remainder of sampling gear included in these analyses. We classified TR, DS/TT/LN and ES as sampling “on-reef,” and GN and SN as sampling “near-reef” assemblages.
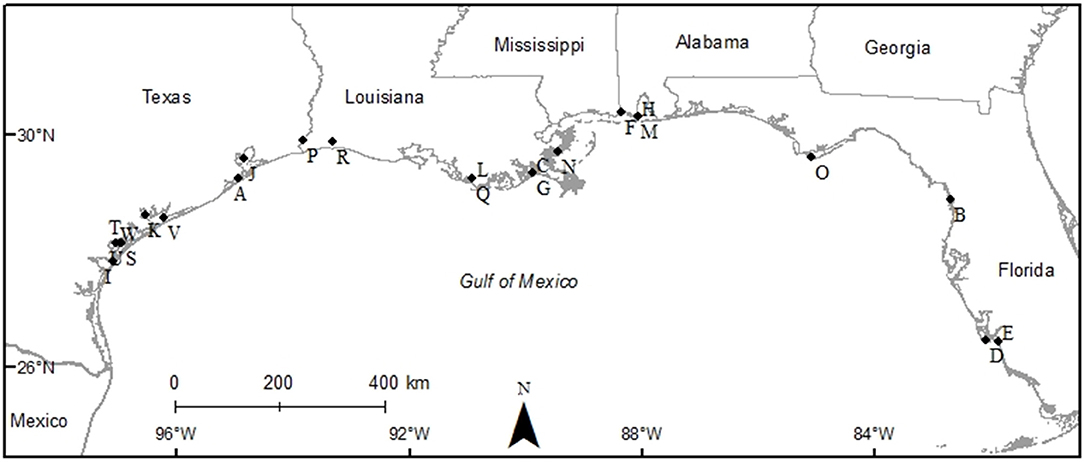
Figure 2. Map of study sites used to examine the diversity and abundance of fish and decapod crustacean communities on oyster reefs across the northern coast of the Gulf of Mexico (Texas, Louisiana, Mississippi, Alabama, and Florida Gulf coast). See Table 1 for explanation of study codes.
Reefs sampled across the selected studies included a mix of reef types, tidal status, and location. Studies sampled either natural reefs (n = 12) or constructed reefs (n = 9), with two studies comparing natural and constructed. Constructed reef habitats (n = 9) were built at different times (1990–2014) using different base materials that included shell, limestone, and concrete base materials. There was a mix of intertidal and subtidal reefs (intertidal = 5; subtidal = 18). Due to lack of site-specific water level data, we were unable to determine exposure levels of reefs or exposure time. Intertidal reefs were all located nearshore (identified as < 25 m from shore), along with a few of the subtidal reefs (n = 3); the remainder of reefs were located offshore (>25 m from shore).
Water quality parameters commonly reported included salinity, temperature, and dissolved oxygen. Oyster reefs were sampled through a broad range of salinities (0–39.5) from Florida to Texas with most studies reporting ranges of salinities >20, reflecting the highly variable nature of the estuarine environments where many oysters are found in the nGoM (Table 1). Temperature reflected the annual range in the region, from 2.0° to 40.6°C, encompassing winter and summer samples, while dissolved oxygen conditions ranged from 0.4 to 17.3 mg L−1. No other environmental parameters were reported consistently across studies.
A total of 115 fish species, based on over 32,000 individual fish, were reported on or near oyster reefs (Table 2, Supplementary Table 1). Of the 115 reported fish species, 22 species (~19%) were collected in eight or more of the studies (Table 3), and 40 species were only reported in one study (Supplementary Table 1). The number of fish species collected ranged from 4 to 46 per study (Table 2; Figure 3; Supplementary Table 1). Species assemblages varied among gear types with TR and DS/TT/LN capturing more known reef residents, such as gobies and blennies, which included 15 species found only in these gear types. Gill nets captured larger and more mobile transient fish species, such as sheepshead (Archosargus probatocephalus) and red drum (Sciaenops ocellatus), while seines captured a mix of both the transient fish species and some of the smaller more ubiquitous resident species (Table 3; Supplementary Table 1). Seine and gill net data included 51 fish species not captured with any of the other methods. Of these, 23 species were captured exclusively by gill net, including many of the shark species, and 15 species were captured exclusively by seine. Combined, the SN and GN gear types uniquely captured 44% of the fish species (Supplementary Table 1). Densities reported were gear-dependent, with TR and TT/DS/LN having mean densities of 20.2 ± 4.3 ind m−2 (mean ± SE) and 15.6 ± 2.8 ind m−2, respectively (Figure 4). GN, capturing larger bodied fish, reported mean densities of 6.7 ± 8.4 ind m−2. SN and ES reported low densities (< 1 ind m−2).
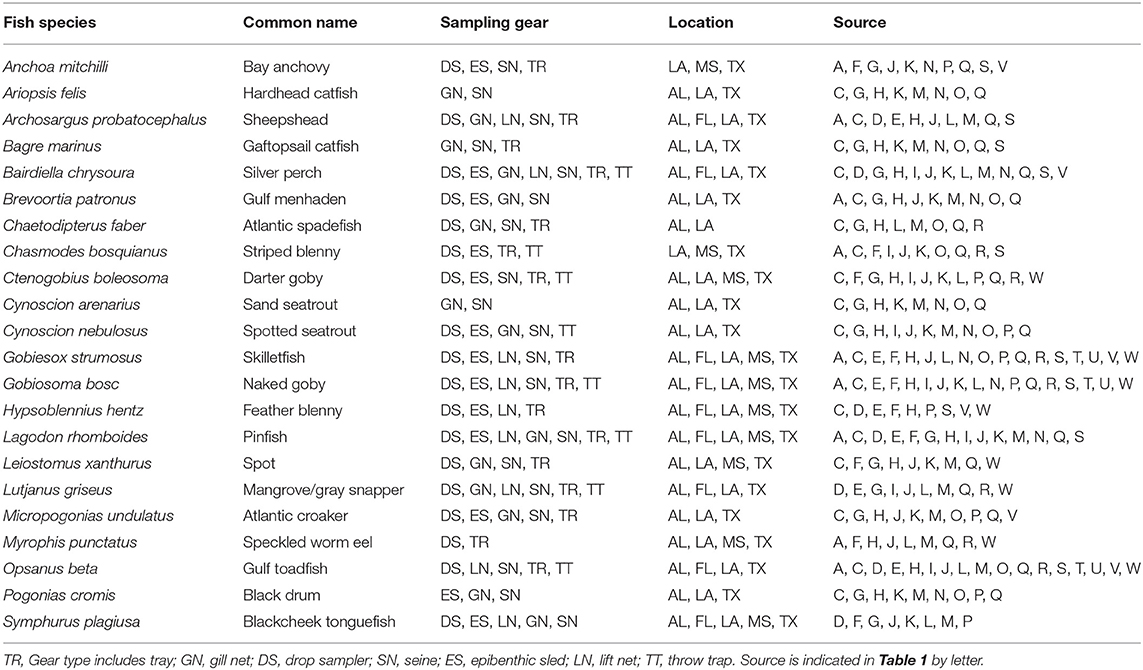
Table 3. List of fish species reported in 8 studies or more, with sampling gear, location, and source.
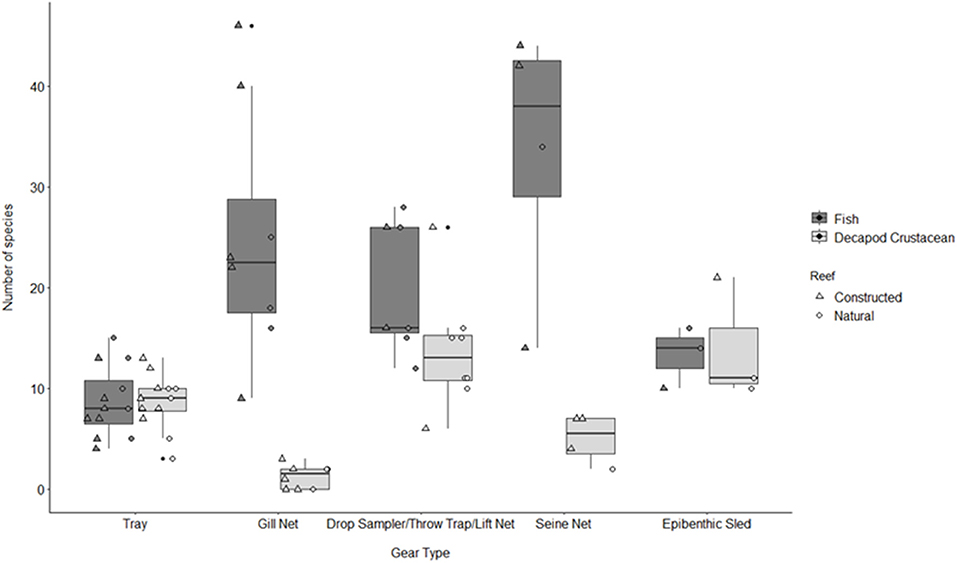
Figure 3. Boxplot of number of fish and decapod crustacean species presented by gear type TR, tray; GN, gill net; DS, drop sampler; LN, lift net; TT, throw trap; SN, seine; ES, epibenthic sled. Box represents 25, 50, and 75% quantilets, with whiskers at ±1.5*IQR.
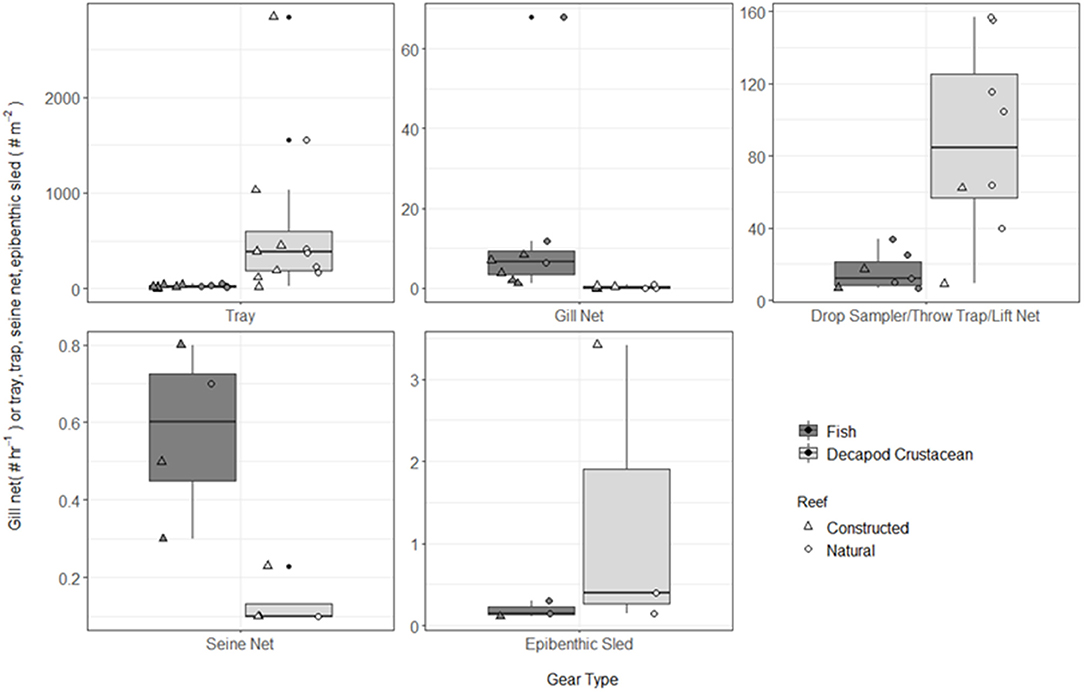
Figure 4. Boxplot of fish and decapod crustacean density presented by gear type TR, tray; GN, gill net; DS, drop sampler; LN, lift net; TT, throw trap; SN, seine; ES, epibenthic sled. Note different y-axes for each gear type. Box represents 25, 50, and 75% quantilets, with whiskers at ±1.5*IQR.
A total of 56 decapod crustacean species, based on over 90,000 individuals were identified (Table 2, Supplementary Table 2). The number of decapod crustacean species ranged up to 26 per study. Of the 56 reported crustacean species, 11 species (snapping shrimp, blue crabs, depressed mud crabs, brown shrimp, white shrimp, Gulf stone crabs, Palaemonetes pugio and P. vulgaris grass shrimp, Panopeid mud crabs, green porcelain crabs, Harris mud crabs) were listed in eight or more of the studies (Table 4) while 27 species were only listed in one study (Supplementary Table 2). Species composition differed between gear types, with TR, TT/DS/LN, and ES capturing the most species. TR consistently captured highest densities (mean ± SE: 647.9 ± 245.9; Figure 4), while GN and SN captured few decapod crustaceans (Figure 4, Supplementary Table 2).
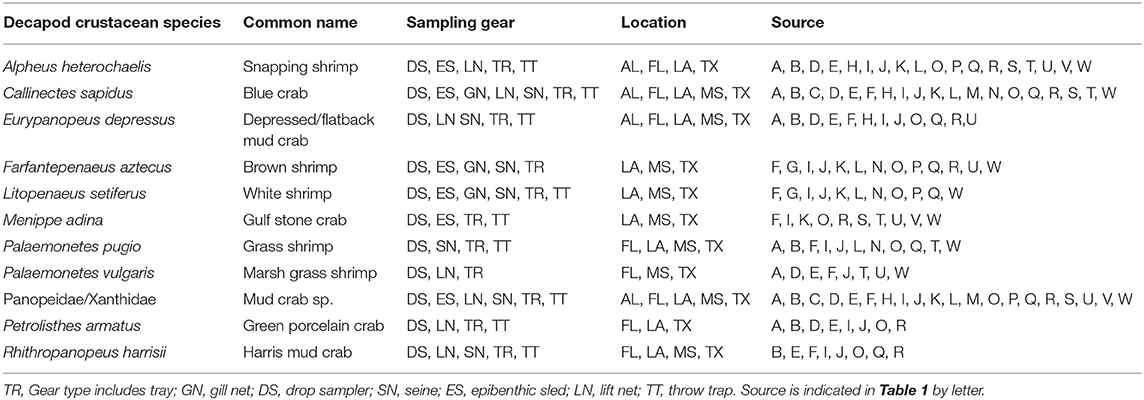
Table 4. List of decapod crustacean species reported in 8 studies or more, with sampling gear, location, and source.
Discussion
Modern day natural and constructed reefs across the nGoM support diverse and dense assemblages of fishes and decapod crustaceans. Nekton density was as high as 2,800 ind m−2 and some reefs had >50 species of fish and decapod crustaceans. Using the available published data, gear-dependent means for density and species richness of dominant “on-reef” sampling (tray) and “near reef” sampling (gill net) serve as useful benchmarks. These benchmarks provide quantitative measures that can be used to assess changes in the status of existing and constructed oyster reefs. Management of coastal habitat and fisheries requires data on species-habitat associations to delineate Essential Fish Habitat, better implement restoration, perform injury assessments, and set policy goals. These suggested benchmarks, based on current reef status, may be used to assess trends in oyster reef habitat support of fisheries and ecosystem functioning for this region.
Relating specific assemblages or densities to reef characteristics or across locations was problematic due to a lack of gear standardization, established gear conversion factors for oyster reefs, and reef habitat metrics. For example, some studies only used gill nets and seines to sample whereas others used drop samplers; given differences in catch efficiencies based on species' identity and size, and variable efficiency of different gear types across reefs, results from different gear types are not directly comparable (Zimmerman et al., 1984). As more data are collected, methodologies are standardized, or conversion factors developed, these fish and decapod crustacean benchmarks will become more targeted to specific locations, reef complexity or reef type.
Reef Characteristics and Complexity
Reef characteristics, such as adjacent habitats, connectivity, habitat redundancy, and water quality all affect fish and decapod crustacean assemblages, and are thus vital metrics for interpreting benchmark data. In general, data synthesized for this study lacked consistent reporting of reef location, in terms of adjacent habitats, connectivity or habitat redundancy, or complexity even though these factors influence nekton assemblages. For example, in Texas, Nevins et al. (2014) hypothesized that low faunal densities observed on natural oyster reefs may be a result of adjacent habitats, as well as difficulties sampling the complex habitat. Tolley et al. (2006) highlighted the impacts of salinity and freshwater flow on reef assemblages identifying flow rates and salinity as key location characteristics influencing reef communities. For constructed reefs, Gregalis et al. (2009) demonstrated that reef height affected fish abundance, while resident species abundance and transient fish assemblages varied by reef location. In some cases, reefs have been proposed as potentially redundant habitat due to their location adjacent to or near other high-quality habitat (i.e., Geraldi et al., 2009; La Peyre et al., 2014a; Heck et al., 2017). Reporting distance to adjacent habitats, reef exposure, and water quality/level at sampling would be useful for studies to better understand drivers of fish and decapod crustacean occupancy of oyster reefs.
Habitat complexity may also affect nekton assemblages through direct and indirect effects on trophic cascades, predation and habitat availability (e.g., Grabowski et al., 2008; Humphries et al., 2011a). Quantifying complexity, particularly across various constructed reef materials remains challenging. In particular, while habitat complexity may be important, some studies have suggested a potential complexity threshold due to failure of finding increasing nekton numbers with increased complexity (e.g., Humphries et al., 2011b; George et al., 2015). For example, George et al. (2015) found no difference in nekton assemblages or prey mortality in experimental studies comparing five possible substrate materials. Similar densities of resident nekton despite increasing oyster density led the authors of one study to hypothesize that there might be a low threshold for habitat complexity (Beck and La Peyre, 2015). These studies highlight the difficulty of relating nekton density and assemblage composition to habitat complexity; some of the differences, however, might also relate to faulty comparisons across studies as a result of sampling gear issues.
Impact of Sampling Gear
Sampling gear are selective for specific size ranges or species, and are not equally effective across, or within, complex habitats (Rozas and Minello, 1997). For oyster reefs in particular, the natural and constructed reefs include a wide range of reef complexity, heterogeneity, reef sizes, reef history, and reef locations. All of these factors influence the assemblages found on or around oyster reefs (Grabowski et al., 2005; Luckenbach et al., 2005; Geraldi et al., 2009; Nevins et al., 2014; Beck and La Peyre, 2015), as well as the effectiveness of gear types. While gear-dependent benchmarks for fish and decapod crustacean density and species number provide general region-wide values, the effects of the interaction of gear type with reef complexity, type, and location remain unknown.
Our study highlighted that different gear types capture different assemblages and numbers of nekton. The highest densities of fish and decapod crustaceans were consistently quantified using methods sampling “on-reef” despite reporting the lowest sampling effort. Specifically, trays, and/or enclosure samplers (lift nets, throw traps, drop-samplers), report sampling “on-reef,” where they capture species generally occupying interstitial spaces within the reef structure. Some variance between these gear types likely reflects differences in location as they cannot be used across all reef types (i.e., throw traps and drop-samplers require water depth < 1. 5 m), or require small patches of reefs where the sampler can fully enclose the reef (i.e., Stunz et al., 2010; Humphries et al., 2011a). Substrate trays sampled similar resident faunal assemblages as other “on-reef” gear, but can be used at greater depths and on larger reef patches. Trays are often criticized for allowing organisms to escape during retrieval in deeper waters, although modifications including nets that can be drawn closed have been suggested (Beck and La Peyre, 2015). Overall, resident decapod crustaceans (e.g., panopeid crabs) and resident fishes (e.g., gobies, blennies) were ubiquitous on the nGoM oyster reefs. In coral reef systems, these cryptobenthic fauna have been shown to provide as much as 70% of the energy consumed in the ecosystem (Brandl et al., 2019). However, many of these species were captured only with tray sampling, suggesting that without these sampling approaches, an important part of the oyster reef community would not be captured.
In contrast, lower numbers were generally captured using gear sampling adjacent to the reef; specifically, by seines and gill nets. Due to the nature of the reef, these techniques limit sampling to near the reef. These approaches however captured the larger commercially and recreationally important fish species of interest, such as red drum (Sciaenops ocellatus), and Spanish mackerel (Scomberomorus maculatus). For both gill net and seine sampling, variation in mesh size, time of day, water flow, and distance covered all influence catch rates (e.g., Vandergroot et al., 2011; Hubert et al., 2012). While techniques exist for standardizing data for some of these differences (i.e., mesh size; Shoup and Ryswky, 2016), other details may be more difficult to reconcile (i.e., interaction with hydrology, reef characteristics), and are often not fully reported.
Gear conversion factors have been developed for comparing different sampling gear, using a variety of statistical techniques (i.e., Pelletier, 1998; Gibson-Reinemer et al., 2016). This might be a useful technique to standardize reported densities and species' numbers for oyster reefs, if effects of habitat location and complexity can be reconciled. For example, even when similar gears can be used, their catch efficiencies and deployment techniques can vary dramatically across conditions. Drop samplers were found to have over 90% catch efficiency at small Texas oyster reefs (Zimmerman et al., 1984; Stunz et al., 2010), but this rate is highly correlated to water clarity and wave action (Rozas and Minello, 1997), two variables that are not frequently reported. Improved reporting of full environmental conditions, standardization of gear type for specific habitats, and, as data permit, exploring possible gear conversion factors could help in developing more targeted reef benchmarks than what we present here.
Reef Restoration, Monitoring, and Benchmarks
If we consider the current state of oyster reefs to be the desired reference condition for assessing changes in status and setting goals and policy for restoration (Coen and Luckenbach, 2000; NRC, 2017), this synthesis provides a set of benchmarks based on means and ranges of current values found across the region. These benchmarks help provide an abundance, density and composition of fish and decapod crustaceans expected to occupy a reef. We suggest these benchmarks as gear-dependent goals, using trays and gill nets, the two most versatile gear types used to sample “on-reefs” (tray), and “near-reefs” (gill nets). To effectively develop and use this approach, habitat characteristics that may be driving the differences between reef assemblages, densities, and species richness need to be better documented. Specifically, habitat characteristics, including reef location, complexity, water quality, and reef exposure critically influence occupancy of reefs by nekton, and benchmarks should be developed to reflect these variations. These same habitat characteristics, along with sampling conditions (i.e., winds, currents, waves, tidal conditions) may also impact sampling gear efficiency. Suggested benchmarks should be treated as a general guide for this region, with adjustments made based on knowledge of local reef habitat characteristics and conditions during sampling. Ideally, future data collection will provide for more targeted benchmarks for this region.
Over the last decade, the oyster reef restoration community has developed and increasingly follows detailed guidance for selection of restoration sites (Coen et al., 2004, and further discussed in Coen and Humphries, 2017) and monitoring reef restoration (i.e., Baggett et al., 2015). However, the difference between these original criteria for guiding site selection, and assessing occupancy by fish and decapod crustaceans, is that the characteristics of the species assemblage using a reef may be dependent on variables that do not necessarily limit oyster reef development (e.g., structural complexity). As a result, better quantification of occupancy of oyster reefs by fish and decapod crustaceans would significantly help in predicting effects of reef complexity, or location (Gilby et al., 2018).
With enormous investments targeted for habitat restoration in the nGoM, and continued emphasis on habitat-species linkages through Essential Fish Habitat policy, quantitative and standardized baseline data to establish benchmarks are increasingly important for managers and policy-makers (Baggett et al., 2015; NRC, 2017; Blomberg et al., 2018a). In the nGoM, over 6 billion USD has been designated for restoration of ecosystems (Environmental Law Institute, 2016) as a result of injury settlements. To ensure effective use of these funds, and to support existing policies related to fisheries and habitat management, generation of standardized monitoring metrics and clear benchmarks to help assess restoration and policy outcomes remains critical.
Data Availability Statement
Datasets were taken from the published literature identified in Table 1.
Author Contributions
ML, DA, and LM reviewed the literature, analyzed and graphed the data. ML and AH conceived the idea, and led the writing. DA and LM contributed to writing of the manuscript.
Funding
This work was supported by funding from the Louisiana Department of Wildlife and Fisheries through support for the U.S. Geological Survey, Louisiana Fish and Wildlife Cooperative Research Unit.
Conflict of Interest
The authors declare that the research was conducted in the absence of any commercial or financial relationships that could be construed as a potential conflict of interest.
Acknowledgments
We thank the many researchers who answered questions, and shared (and dug up) old datasets summarized in this work. This work benefitted from discussions, provision of raw data, and input from numerous researchers including Drs. Steven Scyphers, Kevin Gregalis, and Thomas Glancy, as well as Steve Beck. Drs. Shaye Sable, Brittany Blomberg, and Loren Coen provided comments on an earlier version of this manuscript as well as a number of reviewers. We thank constructive reviews from two reviewers for significant improvements to this manuscript. Any use of trade, firm, or product names is for descriptive purposes only and does not imply endorsement by the U.S. Government.
Supplementary Material
The Supplementary Material for this article can be found online at: https://www.frontiersin.org/articles/10.3389/fmars.2019.00666/full#supplementary-material
Footnotes
1. ^Oil Pollution Act of 1990 (OPA) (101 H.R.1465, P.L. 101–380).
References
Aguilar Marshall, D., Lebreton, B., Palmer, T., De Santiago, K., and Beseres Pollack, J. (2019) Salinity disturbance affects faunal community composition organic matter on a restored Crassostrea virginica oyster reef. Estuarine, Coastal Shelface Sci. 226:106267. doi: 10.1016/j.ecss.2019.106267
Aguilar, D. (2017). Salinity Disturbance Affects Community Structure and Organic Matter on a Restored Crassostrea virginica Oyster Reef in Matagorda Bay, Texas. Master's thesis. Corpus Christi, TX: A&M University–Corpus Christi.
Angermeier, P. L., and Karr, J. R. (1994) Biological integrity versus biological diversity as policy directives. BioScience 44, 690–697. doi: 10.2307/1312512
Baggett, L. D., Powers, S. P., Brumbaugh, R. D., Coen, L. D., DeAngelis, B. M., Greene, J. K., et al. (2015). Guidelines for evaluating performance of oyster habitat restoration. Restor. Ecol. 23, 737–745. doi: 10.1111/rec.12262
Barbier, E. B., Hacker, S. D., Kennedy, C., Koch, E. W., Stier, A. C., and Silliman, B. R. (2011). The value of estuarine and coastal ecosystem services. Ecol. Mongr. 81, 169–193. doi: 10.1890/10-1510.1
Beck, M. W., Brumbaugh, R. D., Airoldi, L., Carranza, A., Coen, L. D., Crawford, C., et al. (2011). Oyster reefs at risk and recommendations for conservation, restoration, and management. BioScience 61, 107–116. doi: 10.1525/bio.2011.61.2.5
Beck, S. L., and La Peyre, M. (2015). Effects of oyster harvest activities on Louisiana reef habitat and resident nekton communities. Fish. Bull. 113, 327–340. doi: 10.7755/FB.113.3.8
Bennett, E. M., Cramer, W., Begossi, A., Cundill, G., Diaz, S., Egoh, B. N., Geijzendorffer, I. R., et al. (2015). Linking biodiversity, ecosystem services and human well-being: three challenges for designing research for sustainability. Curr. Opin. Enviorn. Sustain. 14, 76–85. doi: 10.1016/j.cosust.2015.03.007
Blomberg, B. N., Beseres Pollack, J., Montagna, P. A., and Yoskowitz, D. W. (2018a). Evaluating the U.S. Estuary Restoration Act to inform restoration policy implementation: A case study focusing on oyster reef projects. Marine Policy 91, 161–166. doi: 10.1016/j.marpol.2018.02.014
Blomberg, B. N., Palmer, T. A., Montagna, P. A., and Beseres Pollack, J. (2018b). Habitat assessment of a restored oyster reef in South Texas. Ecol. Eng. 122, 48–61. doi: 10.1016/j.ecoleng.2018.07.012
Brandl, S. J., Tornabene, L., Goatley, C. H., Casey, J. M., Morais, R. A., Côté, I. M., et al. (2019). Demographic dynamics of the smallest marine vertebrates fuel coral-reef ecosystem functioning. Science 364:peaav3384. doi: 10.1126/science.aav3384
Brown, L., Furlong, J., Brown, K. M., and La Peyre, M. K. (2013). Oyster reef restoration in the northern Gulf of Mexico: effect of artificial substrate and age on nekton and benthic macroinvertebrate assemblage use. Restor. Ecol. 22, 214–222. doi: 10.1111/rec.12071
Coen, L. D., and Grizzle, R. (2007). The Importance of Habitat Created by Molluscan Shellfish to Managed Species Along the Atlantic Coast of the United States. Atlantic States Marine Fisheries Commission Habitat Management Series #8.
Coen, L. D., and Grizzle, R. (2016). “Bivalve molluscs,” in Encyclopedia of Estuaries, ed M. Kennish (Dordrecht: Springer, 89–109.
Coen, L. D., and Humphries, A. T. (2017). “Chapter 19. Oyster-generated marine habitats: their services, enhancement and monitoring.” in Routledge Handbook of Ecological and Environmental Restoration, eds S. Stuart and S. Murphy (New York, NY: Routledge), 274–294. doi: 10.4324/9781315685977-19
Coen, L. D., and Luckenbach, M. W. (2000). Developing success criteria and goals for evaluating oyster reef restoration: ecological function or resource exploitation? Ecol. Eng. 15, 323–343. doi: 10.1016/S0925-8574(00)00084-7
Coen, L. D., Luckenbach, M. W., and Breitburg, D. L. (1999). “The role of oyster reefs as an essential fish habitat: a review of current knowledge and some new perspectives,” in Fish Habitat: Essential Fish Habitat and Rehabilitation, ed L. R. Benaka (Bethesda, MD: American Fisheries Society), 438–454.
Coen, L. D., Walters, K., Wilber, D., and Hadley, N. (2004). A South Carolina Sea Grant Report of a 2004 Workshop to Examine and Evaluate Oyster Restoration Metrics to Assess Ecological Function, Sustainability and Success: Results and Related Information. Sea Grant, 27. Available online at: http://www.oyster-restoration.org/wp-content/uploads/2012/06/SCSG04.pdf (accessed September 15, 2017).
Dufrêne, M., and Legendre, P. (1997). Species assemblages and indicator species: the need for a flexible asymmetrical approach. Ecol. Monogr. 67, 345–366. doi: 10.2307/2963459
Ehrenfeld, J. G. (2000). Defining the limits of restoration: the need for realistic goals. Restor. Ecol. 8, 2–9. doi: 10.1046/j.1526-100x.2000.80002.x
Environmental Law Institute (2016). BP Oil Spill Main Funding Process. Available online at: http://eli-ocean.org/gulf/files/Overview-Educational-Material-4-21-16.pdf (accessed March 15, 2018).
Frey, D. G. (1946). Oyster Bars of the Potomac River. Fish and Wildlife Service Special Scientific Report. 32, 1–93.
Gain, I. (2009). Oyster reefs as Nekton Habitat in Estuarine Ecosystems. Master's thesis. Corpus Christi, TX: Texas A&M University – Corpus Christi.
Gain, I. E., Brewton, R. A., Reese Robillard, M. M., Johnson, K. D., Smee, D. L., and Stunz, G. W. (2017). Macrofauna using intertidal oyster reef varies in relation to position within the estuarine mosaic. Mar. Biol. 164:8. doi: 10.1007/s00227-016-3033-5
Geist, J., and Hawkins, S. J. (2016). Habitat recovery and restoration in aquatic ecosystems: current progress and future challenges. Aquat. Conserv. 26, 942–962. doi: 10.1002/aqc.2702
George, L. M., De Santiago, K., Palmer, T. A., and Beseres Pollack, J. (2015). Oyster reef restoration: effect of alternative substrates on oyster recruitment and nekton habitat use. J. Coastal Conserv. 19, 13–22. doi: 10.1007/s11852-014-0351-y
Geraldi, N. R., Powers, S. P., Heck, K. L., and Cebrian, J. (2009). Can habitat restoration be redundant? Response of mobile fishes and crustaceans to oyster reef restoration in marsh tidal creeks. Mar. Ecol. Prog. Seri. 389, 171–180. doi: 10.3354/meps08224
Gibson-Reinemer, D. K., Ickes, B. S., and Chick, J. H. (2016) Development assessment of a new method for combining catch per unit effort data from different fish sampling gears: multigear mean standardization (MGMS). Can. J. Fish. Aquat. Sci. 74, 8–14. doi: 10.1139/cjfas-2016-0003
Gilby, B. L., Olds, A. D., Peterson, C. H., Connolly, R. M., Voss, C. M., Bishop, M. J., et al. (2018). Maximizing the benefits of oyster ref restoration for finfish and their fisheries. Fish Fish. 19:931–947. doi: 10.1111/faf.12301
Glancy, T., Frazer, T., Cichra, C., and Lindberg, W. (2003). Comparative patterns of occupancy by decapod crustaceans in seagrass, oyster, and marsh-edge habitats in a northeast Gulf of Mexico estuary. Estuaries 26, 1291–1301. doi: 10.1007/BF02803631
Grabowski, J. H., Brumbaugh, R. D., Conrad, R. F., Keller, A. G., Opaluch, J., Peterson, C. H., et al. (2012). Economic valuation of ecosystem services provided by oyster reefs. BioScience 62, 900–909. doi: 10.1525/bio.2012.62.10.10
Grabowski, J. H., Hughes, A. R., and Kimbro, D. L. (2008). Habitat complexity influences cascading effects of multiple predators. Ecology 89, 3413–3422. doi: 10.1890/07-1057.1
Grabowski, J. H., Hughes, A. R., Kimbro, D. L., and Dolan, M. A. (2005). How habitat setting influences restored oyster reef communities. Ecology 86, 1926–1935. doi: 10.1890/04-0690
Graham, P. M., Palmer, T. A., and Beseres Pollack, J. (2017). Oyster reef restoration: substrate suitability may depend on specific restoration goals. Restor. Ecol. 25, 459–470. doi: 10.1111/rec.12449
Gregalis, K., Johnson, M., and Powers, S. (2009). Restored oyster reef location and design affect responses of resident and transient fish, crab, and shellfish species in Mobile Bay, Alabama. Transac. Am. Fish. Soc. 138, 314–327. doi: 10.1577/T08-041.1
Heck, K. L. Jr., Cebrian, J., Powers, S. P., Geraldi, N., Plutchak, R., Byron, D., et al. (2017). “Ecosystem services provided by shoreline reefs in the Gulf of Mexico: an experimental assessment using live oysters.” in Living Shorelines: The Science and Management of Nature-based Coastal Protecion, eds D. Bilkovic, M. Roggero, J. Toft, and M. La Peyre (Boca Raton, FL: CRC Press), 401–420. doi: 10.1201/9781315151465-24
Hubert, W. A., Pope, K. L., and Dettmers, J. M. (2012). “Passive capture techniques.” in Fisheries Techniques, 3rd Edn, eds. A. V. Zale, D. L. Parrish, and T. M. Sutton (Bethesda, MD: American Fisheries Society), 223–265.
Humphries, A., La Peyre, M. K., Kimball, M., and Rozas, L. (2011a). Testing the effect of habitat structure and complexity on nekton assemblages using experimental oyster reefs. J. Exp. Mar. Biol. Ecol. 409, 172–179. doi: 10.1016/j.jembe.2011.08.017
Humphries, A. T., and La Peyre, M. K. (2015). Oyster reef restoration supports increased nekton biomass and potential commercial fishery value. PeerJ 3:e1111. doi: 10.7717/peerj.1111
Humphries, A. T., La Peyre, M. K., and Decossas, G. A. (2011b). The effect of structural complexity, prey density, and predator-free space on prey survivorship at oyster reef mesocosms. PLoS ONE 6:e28339. doi: 10.1371/journal.pone.0028339
Kentula, M. E. (2000). Perspectives on setting success criteria for wetland restoration. Ecol. Eng. 15, 199–209. doi: 10.1016/S0925-8574(00)00076-8
Kilgen, R., and Dugas, R. (1989). The Ecology of Oyster Reefs of the Northern Gulf of Mexico: An Open File Report. NWRC-open file report 89-02, Washington, DC: US Dept of the Interior.
Kim, C., and Park, K. (2012) A modeling study of water salt exchange for a micro-tidal, stratified northern Gulf of Mexico estuary. J. Mar. Syst. 96, 103–115. doi: 10.1016/j.jmarsys.2012.02.008
La Peyre, M., Schwarting, L., and Miller, S. (2013). Preliminary Assessment of Bioengineered Fringing Shoreline Reefs in Grand Isle and Breton Sound. Louisiana: US Geological Survey Open-File Report 2013–1040. 34p. doi: 10.3133/ofr20131040
La Peyre, M. K., Furlong, J., Brown, L. A., Piazza, B. P., and Brown., K. (2014a). Oyster reef restoration in the northern Gulf of Mexico: extent, methods and outcomes. Ocean Coast. Manag. 89, 20–28. doi: 10.1016/j.ocecoaman.2013.12.002
La Peyre, M. K., Humphries, A., Casas, S., and La Peyre, J. F. (2014b). Temporal variation in development of ecosystem services from oyster reef restoration. Ecol. Eng. 63, 34–44. doi: 10.1016/j.ecoleng.2013.12.001
La Peyre, M. K., Schwarting-Miller, L., Miller, S., and Melancon, E. (2017). “Chapter 20: Comparison of oyster populations, shoreline protection service and site characteristics at seven created fringing reefs in Louisiana: key parameters and responses to consider.” in Living Shorelines: The Science and Management of Nature-based Coastal Protecion, eds D. Bilkovic, M. Roggero, J. Toft, and M. La Peyre (Boca Raton, FL: CRC Press), 363–382. doi: 10.1201/9781315151465-22
Lowe, M. R., Sehlinger, T., Soniat, T. M., and La Peyre, M. K. (2017). Interactive effects of water temperature and salinity on growth and mortality of eastern oysters, Crassostrea virginica: a meta-analysis using 40 years of monitoring data. J. Shellf. Res. 36, 683–697. doi: 10.2983/035.036.0318
Luckenbach, M. W., Coen, L. D., Ross, P. G. Jr., and Stephen, J. A. (2005). Oyster reef habitat restoration: relationship between oyster abundance and community development based on two studies in Virginia and South Carolina. J. Coast. Res. 40, 64–78.
Magnuson Stevens Fishery Management Conservation Act (1996). Available online at: https://www.gpo.gov/fdsys/pkg/STATUTE-110/pdf/STATUTE-110-Pg3559.pdf. (accessed March 15, 2018).
McClanahan, T. R., Schroeder, R. E., Friedlander, A. M., Vigliola, L., Wantiez, L., Caselle, J. E., et al. (2019). Global baselines and benchmarks for fish biomass: comparing remote reefs and fisheries closures. Mar. Ecol. Prog. Series 612, 167–192. doi: 10.3354/meps12874
Mobius, K. (1877). The Oyster and Oyster-Culture. Reprinted in U.S. Commission of Fish and Fisheries Annual Reports. 1880. Available online at: http://penbay.org/cof/uscof.html (accessed March 22, 2018).
Montagna, P. A., Brenner, J., Gibeaut, J., and Morehead, S. (2011). “Coastal impacts,” in The Impact of Global Warming in Texas, 2nd Edn, eds J Schmandt, G. R. North, and J. Clarkson (Austin, TX: University of Texas Press), 96–123.
Nevins, J., Beseres Pollack, J., and Stunz, G. (2014). Characterizing nekton use of the largest unfished oyster reef in the United States compared with adjacent estuarine habitats. J. Shellfish Res. 33, 227–238. doi: 10.2983/035.033.0122
NRC (2017). National Research Council and National Academies of Sciences Engineering and Medicine. (2017). Effective Monitoring to Evaluate Ecological Restoration in the Gulf of Mexico. Washington, DC: The National Academies Press.
Pelletier, D. (1998) Intercalibration of research survey vessels in fisheries: a review and an application. Can. J. Fish Aquat. Sci. 55, 2672–2690. doi: 10.1139/f98-151.
Pendleton, E. A., Barras, J. A., Williams, S. J., and Twichell, D. C. (2010). Coastal Vulnerability Assessment of the Northern Gulf of Mexico to Sea-Level Rise and Coastal Change. U.S. Geological Survey Open-File Report 2010–1146. doi: 10.3133/ofr20101146
Peterson, C. H., Grabowski, J. H., and Powers, S. P. (2003). Estimated enhancement of fish production resulting from restoring oyster reef habitat: quantitative valuation. Mar. Ecol. Prog. Seri. 264, 249–264. doi: 10.3354/meps264249
Pickett, S. T. A., Parker, V. T., and Fiedler, P. L. (1992). “The new paradigm in ecology: implications for conservation biology above the species level,” in Conservation Biology, eds P. L. Fielder and S. K. Jain (New York, NY: Chapman & Hall), 65–88. doi: 10.1007/978-1-4684-6426-9_4
Plunket, J., and La Peyre, M. (2005). Oyster beds as fish and macroinvertebrate habitat in Barataria Bay, Louisiana. Bull. Mar. Sci. 77, 155–164.
R Core Team (2018). R: A Language and Environment for Statistical Computing. R Foundation for Statistical Computing, Vienna Austria. Available online at: http://www.R-project.org
Rezek, R. J., Lebreton, B., Roark, E. B., Palmer, T. A., and Beseres Pollack, J. (2017). How does a restored oyster reef develop? An assessment based on stable isotopes and community metrics. Mar. Biol. 164:54. doi: 10.1007/s00227-017-3084-2
Ridge, J. T., Rodriguez, A. B., Fodrie, F. J., Lindquist, N. L., Brodeur, M. C., and Coleman, S. E. (2015) Maximizing oyster-reef growth supports green infrastructure with accelerating sea-level rise. Sci. Rep. 5:14785. doi: 10.1038/srep14785.
Robillard, M., Stunz, G., and Simons, J. (2010). Relative value of deep subtidal oyster reefs to other estuarine habitat types using a novel sampling method. J. Shellf. Res. 29, 291–302. doi: 10.2983/035.029.0203
Rondinini, C., and Chiozza, F. (2010). Quantitative methods for defining percentage area targets for habitat types in conservation planning. Biol. Conserv. 143, 1646–1653. doi: 10.1016/j.biocon.2010.03.037
Rozas, L., and Minello, T. (1997). Estimating densities of small fishes and decapod crustaceans in shallow estuarine habitats: a review of sampling design with focus on gear selection. Estuaries 20, 199–213. doi: 10.2307/1352731
Scyphers, S., Powers, S., Heck, K., and Byron, D. (2011). Oyster reefs as natural breakwaters mitigate shoreline loss and facilitate fisheries. PLoS ONE 6:e22396. doi: 10.1371/journal.pone.0022396
SER Society for Ecological Restoration (2004). The SER International Primer on Ecological Restoration. Tucson, AZ: Society for Ecological Restoration International. Available online at: http://c.ymcdn.com/sites/www.ser.org/resource/resmgr/custompages/publications/SER_Primer/ser_primer.pdf (accessed Septeber 01, 2017).
Shervette, V., and Gelwick, F. (2008). Seasonal and spatial variations in fish and macroinvertebrate communities of oyster and adjacent habitats in a Mississippi estuary. Estuar. Coasts 31, 584–596. doi: 10.1007/s12237-008-9049-4
Shoup, D. E., and Ryswky, R. G. (2016). Length selectivity and size-bias correction for the North American standard gill net. North Am. J. Fish. Manag. 36, 485–496. doi: 10.1080/02755947.2016.1141809
Simonsen, K. (2008). The Effect of an Inshore Artificial Reef on the Community Structure and Feeding Ecology of Estuarine Fishes in Barataria Bay, Louisiana. master's thesis (Baton Rouge: LA: Louisiana State University).
Simonsen, K. A., Cowan, J. H. Jr, and Fischer, J. (2013). Examination of an estuarine fish assemblage over an inshore artificial reef. Open Fish Sci. J. 6, 48–57. doi: 10.2174/1874401X01306010048
Stunz, G., Minello, T., and Rozas, L. (2010). Relative value of oyster reef as habitat for estuarine nekton in Galveston Bay, TX. Mar. Ecol. Prog. 406, 147–159. doi: 10.3354/meps08556
Toledo, D., Agudelo, M. S., and Bentley, A. L. (2011). The shifting of ecological restoration benchmarks and their social impacts: digging deeper into pleistocene re-wilding. Restor. Ecol. 19, 564–568. doi: 10.1111/j.1526-100X.2011.00798.x
Tolley, S. G., and Volety, A. K. (2005). The role of oysters in habitat use of oyster reefs by resident fishes and decapod crustaceans. J. Shellfish Res. 24, 1007–1012. doi: 10.2983/0730-8000(2005)24[1007:TROOIH]2.0.CO;2
Tolley, S. G., Volety, A. K., and Savarese, M. (2006). Influence of salinity on the habitat use of oyster reefs in three southwest Florida estuaries. J. Shellfish Res. 24, 127–137. doi: 10.2983/0730-8000(2005)24[127:IOSOTH]2.0.CO;2
Vandergroot, C. S., Kocovsky, P. M., Brenden, T. O., and Liu, W. (2011). Selectivity evaluation for two experimental gill-net configurations used to sample Lake Erie walleyes. North Am. J. Fish. Manag. 21, 660–665. doi: 10.1080/02755947.2011.623758
Vasconcelos, R. P., Henriques, S., Franca, S., Pasquaud, S., Cardoso, I., Laborde, M., et al. (2015). Global patterns and predictors of fish species richness in estuaries. J. Anim. Ecol. 84, 1331–1341. doi: 10.1111/1365-2656.12372
Wells, H. W. (1961). The fauna of oyster beds, with special reference to the salinity factor. Ecol. Monogr. 31, 266–329. doi: 10.2307/1948554
Zimmerman, R., Minello, T., Baumer, T., and Castiglione, M. (1989). Oyster Reef as Habitat for Estuarine Macrofauna. NOAA Technical Memorandum, NMFS-SEFC-249.
Zimmerman, R. J., Minello, T. J., and Zamora, G. (1984). Selection of vegetated habitat by brown shrimp, Penaeus aztecus, in a Galveston Bay salt marsh. Fish. Bull. 82, 325–336.
Zu Ermgassen, P. S. E., Grabowski, J. H., Gair, J. R., and Powers, S. P. (2015). Quantifying fish and mobile invertebrate production from a threatened nursery habitat. J. Appl. Ecol. 53, 596–606. doi: 10.1111/1365-2664.12576
Keywords: essential fish habitat, estuary, fisheries, restoration, Crassostrea virginica
Citation: La Peyre MK, Aguilar Marshall D, Miller LS and Humphries AT (2019) Oyster Reefs in Northern Gulf of Mexico Estuaries Harbor Diverse Fish and Decapod Crustacean Assemblages: A Meta-Synthesis. Front. Mar. Sci. 6:666. doi: 10.3389/fmars.2019.00666
Received: 18 June 2019; Accepted: 10 October 2019;
Published: 25 October 2019.
Edited by:
Nancy Knowlton, Smithsonian Institution, United StatesReviewed by:
Charles Alan Jacoby, St. Johns River Water Management District, United StatesAnya Leard Brown, University of Georgia, United States
Copyright © 2019 La Peyre, Aguilar Marshall, Miller and Humphries. This is an open-access article distributed under the terms of the Creative Commons Attribution License (CC BY). The use, distribution or reproduction in other forums is permitted, provided the original author(s) and the copyright owner(s) are credited and that the original publication in this journal is cited, in accordance with accepted academic practice. No use, distribution or reproduction is permitted which does not comply with these terms.
*Correspondence: Megan K. La Peyre, bWxhcGV5cmVAYWdjZW50ZXIubHN1LmVkdQ==