- 1Marine Mammal Research, Department of Bioscience, Aarhus University, Roskilde, Denmark
- 2Total E&P Denmark A/S, Health, Safety and Environment, Esbjerg, Denmark
The most common cetacean in the North Sea is the harbor porpoise (Phocoena phocoena). Underwater noise is increasingly recognized as a source of impact on the marine environment and seismic airguns were one of the first man-made high intensity sound source to receive attention with respect to potential impact on marine mammals. In this study, we investigate the effects of a 3D seismic survey on harbor porpoise echolocation activity in the Danish sector of the North Sea. This was achieved by deploying porpoise click detectors (C-PODs) and sound recorders (SM2M and SM3M) both inside and adjacent to the seismic survey area, before, during and after the survey over a total duration of 9 months. Three echolocation parameters were analyzed: number of clicks per minute, minutes with porpoise echolocation click trains and feeding buzz frequency in relation to all minutes with click trains. Decreases in echolocation signals were detected up to 8–12 km from the active airguns, which may indicate temporary displacement of porpoises or a change in porpoise echolocation behavior. However, no general displacement of harbor porpoises away from the seismic survey area could be detected when comparing to reference stations 15 km away from any seismic activity. Our results add to the understanding that underwater noise has the potential to affect temporarily foraging efficiency in porpoises. While the effect of seismic surveys on harbor porpoise behavior was smaller than what has been found for pile-driving, the cumulative effect of anthropogenic impacts could be assessed by evaluation of potential population level consequences.
Introduction
Underwater noise is increasingly recognized as a source of impact on the marine environment (Jones, 2019). Airgun arrays used during marine seismic surveys to map the local geology were some of the first man-made high intensity sound sources to receive attention with respect to potential impact on marine mammals (Gales, 1982; Richardson et al., 1986). Most studies have primarily been focused on the effects of seismic surveys on baleen whales, as they are presumed to have the best hearing in the low frequency range where most of the energy in airgun pulses is also found (Gordon et al., 2003; Castellote et al., 2012; Blackwell et al., 2015; Dunlop et al., 2017). Toothed whales, however, are much more abundant than baleen whales in the North Sea, a region with extensive and mature offshore oil and gas exploration and extraction. This region is also a core habitat for harbor porpoises (Phocoena phocoena, Hammond et al., 2013; Delefosse et al., 2017). Despite this, only a few field-based studies on the effects of seismic activities on small toothed whales are available. Stone and Tasker (2006) showed reduced sighting rates of small odontocetes from seismic vessels with firing airguns, but had too few observations to detect any specific effect on harbor porpoises. Another study using acoustic data loggers showed that a seismic survey (11 days with a 470 cubic inch airgun array) had short-term effects on porpoise occurrence up to 5–10 km from the survey vessel with a reduction in acoustic activity of animals inside the surveyed area, but with no long-term effect on porpoise abundance after the activities (Thompson et al., 2013; Pirotta et al., 2014). van Beest et al. (2018b) demonstrated variable behavioral reactions in movement and diving for up to 8 h when individual tagged porpoises were exposed for 1 min to a single small airgun (10 cubic inch) at a range of about 1 km.
Reaction of porpoises to underwater noise from pile driving during installation of offshore wind farms has been studied more extensively than reaction to seismic surveys. Passive acoustic monitoring and aerial surveys have demonstrated a significant gradual decrease in harbor porpoise echolocation activity and visual observations from about 20 km from the piling site and toward the sound source (Tougaard et al., 2009; Brandt et al., 2011; Dähne et al., 2013a). Source levels generated by percussive piling of steel monopiles and jacket foundations for offshore wind turbines are high, e.g., 250 dB re. 1 μPa, Lpk–pk back calculated to 1 m for a 1.8 m diameter jacket pile (Bailey et al., 2010), comparable to back-calculated source levels from large airgun arrays (e.g., Richardson et al., 1995). However, noise pulses from airguns and percussive pile driving are not identical. First, pile driving pulses contain more energy at higher frequencies than airgun pulses. Second, the geometry of the sources is different, although this mainly affects the sound in the near field. Whereas the sound from pile driving originates from a Mach-wave propagating down the length of the pile, the airgun array is a cluster of multiple sound sources spaced several meters apart. Additionally, large airgun arrays typically fire with a pulse rate of one pulse every 5–10 s and operate for many days (intermitted by line change); alternatively, a pile driving of a monopole is more intense, typically one pulse per 1–2 s, but only lasts for a period of 1–3 h per pile and usually only one pile is typically completed per day. Finally, the noise source in pile driving is stationary, whereas the sound source continuously moves in a seismic survey. This means that a porpoise can only distance itself from pile driving noise by swimming away, whereas a porpoise exposed to a seismic survey will first experience a source approaching and then moving away. While this suggests that porpoises could also react to airgun noise at considerable distances from the source vessel, the highlighted differences imply that one cannot simply extrapolate porpoise reaction distances from pile driving to seismic surveys. The reaction of harbor porpoises to sounds produced during seismic surveys may seem counterintuitive, as most of the energy in airgun pulses is well below 500 Hz and thus in a range where porpoises have poor hearing (Kastelein et al., 2010). However, there is still substantial energy above ambient noise, up to 10s of kHz, even if the contribution to the total energy of an airgun pulse is negligible (Goold and Fish, 1998; Hermannsen et al., 2015; Kyhn et al., 2019).
There is evidence that energy at higher frequencies is more important for eliciting behavioral reactions of porpoises than energy at lower frequencies. Dyndo et al. (2015) showed that fast swimming and porpoising was consistently observed by captive porpoises in response to ship noise, and that this behavior correlated better with energy from ships above 1 kHz than below. Additionally, the compilation of reactions of wild porpoises to a range of different sounds indicate that received sound levels above the hearing threshold (sensation level, or loudness) correlates better with behavior than does the absolute sound pressure level, i.e., the relative noise level above the hearing curve is most important to the animal (Tougaard et al., 2015). If this is a general relationship, porpoise reaction distances to airgun noise are predicted to be shorter than to pile driving noise at the same energy, because the perceived loudness of the airgun pulses is predicted to be lower than for pile driving noise due to less energy at the higher frequencies where porpoise hearing is better.
Here, we report on the effects of a large 3D seismic survey conducted in the central North Sea on harbor porpoise echolocation activity. This is the first time a full size seismic survey has been studied in relation to harbor porpoises by means of acoustic data loggers recording received noise levels and echolocation behavior. Clear effect on echolocation activity was found within 12 km of the survey vessel, while no overall displacement could be detected.
Materials and Methods
Study Area and Survey Details
The 3D seismic survey was conducted between 26 July and 5 November 2016 in the Danish sector of the central North Sea in water depth ranging from 36 to 49 m (Figure 1). The seismic source was comprised of two alternating airgun arrays towed 5 m below the sea surface and approximately 200 m behind either the main seismic vessel or, in the case when data below one of the present oil and gas platforms was collected, a secondary smaller seismic vessel.
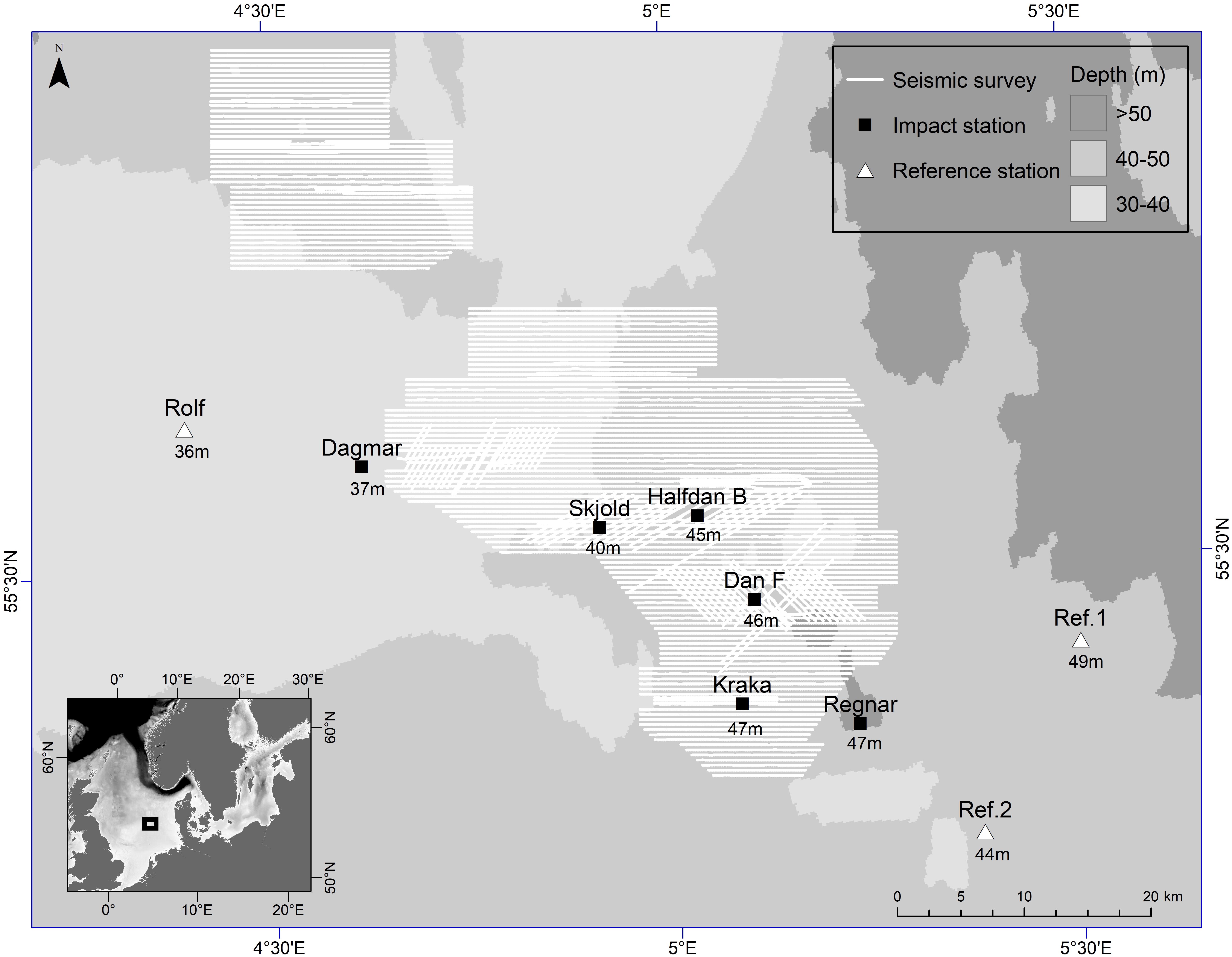
Figure 1. Map of the study area in the Danish sector of the central North Sea (insert) with the seismic survey transect lines highlighted in white, and the nine recording stations, divided into impact stations (squares) and reference stations (triangles). C-PODs were deployed at all nine stations and six full band acoustic recorders were placed at Rolf, Dagmar, DanF, Kraka, Regnar and Ref.1. Numbers indicate water depth at each deployment site (m). Projection UTM zone 31N, ED50. Source: Total E&P Denmark A/S.
The airgun array on the streamer seismic vessel was made up of three subarrays, consisting of 21 active airguns in total, with a combined volume of 58.8 l (3570 cubic inches) and which operated at an air pressure of 14 MPa (2000 psi). This vessel also towed eight 3.5 km long passive seismic streamers containing hydrophones. The streamers were separated by 100 m giving a total spread width of 700 m. The secondary seismic source vessel towed an airgun array of 3360 cubic-inches.
The survey lines were closely spaced, about 400 m apart, and the objective was to replicate, as closely as possible, a previously conducted 3D survey on the same lines (making it a so-called 4D survey). The average speed of the seismic vessel was 8.6 km/h (4.6 knots). The initial airgun pulse lasted less than 0.1 s and was repeated approximately every 10 s (5.7 shots/min).
The survey lasted 103 days and acquired data from 3600 km transect lines distributed over almost 300 line segments. The surveyed area was 1121 km2. All movements of the seismic vessel as well as the airgun shooting were continuously logged in a database by the ship. Seismic shooting took place on all but 17 days, during which weather prohibited operation. Total data acquisition time was 481 h, therefore airguns were active at full output for approximately 20% of the survey time. Additional time was spent testing equipment, seismic source ramp up before line starts, and other preparations.
Monitoring Stations
Nine recording stations (Figure 1) were established on 8 June, 2016 and data collection continued until 22 March, 2017. Six of these stations were placed 100–200 m from oil and gas platforms, one station was placed 200 m from an inactive subsurface wellhead placed on the seafloor (7.5 m × 6.5 m × 4.5 m), and two reference stations placed at least 15 km away from any seismic activity on the bare seabed. Four stations were marked with surface buoys (Rolf, Dagmar, Ref.1 and Ref.2). The remaining stations were deployed with natural fiber bags filled with gravel (2 × 15 kg) as anchors and recorders were recovered by means of an acoustic release system (SubSeaSonics, AR-60-E, San Diego, CA, United States).
All nine recording stations were equipped with a porpoise click detector (C-PODs, Chelonia, Penzance, Cornwall). C-PODs are self-contained dataloggers designed to detect echolocation pulses (clicks) and store summary information about the detected clicks. They were calibrated prior to deployment to make sure their sensitivity were comparable (Dähne et al., 2013b). Detection thresholds can be found in Supplementary Table S1. C-PODs were placed approximately 2 m above the seabed. The maximum detection radius for a T-POD (predecessor of the C-POD) is some 100 m depending on external environmental conditions, like variation in background noise, salinity, and water column stratification (Kyhn et al., 2012). These detection distances likely also apply to the C-POD, as the two types of instruments have comparable detection thresholds (Kyhn et al., 2012; Supplementary Table S1). Background noise variations were not taken into consideration as the seismic survey was done over a relatively long period in relatively good weather. We therefore believe that changes in background noise would be averaged out over the scale of the survey and that the background noise was relatively constant during the short term analysis. Furthermore, if anything, the background noise would be greater outside the survey period where poor weather conditions would have increased background noise and thereby less porpoises would be detected. We therefore consider our results reliable and conservative. Thus, as stations were more than 7.5 km apart, any echolocation signal recorded would only represent the presence of porpoises around that particular station. C-PODs are fitted with a 20 kHz high-pass filter, which effectively prevents the detection of airgun pulses.
Two models of acoustic broad-band recorders were used to detect the airgun pulses (SM2M and SM3M; Wildlife Acoustics, Boston) and deployed at six of the nine stations (Rolf, Dagmar, DanF, Kraka, Regnar and Ref.1). The two logger versions are conceptually similar and consist of a hydrophone (HTI96-min, sensitivity −165 dB re. 1 V/μPa), an amplifier, recording electronics and storage on SD-memory cards. Recordings were conducted at a sampling rate of 48 kHz (16-bit, 0 dB gain, providing a recording bandwidth of 20 Hz–24 kHz) and duty cycled to save battery life (30 min of recordings, 30 min pause). Sound recorders were also calibrated prior to deployment. Hydrophones were placed inside a custom-built coupler on a pistonphone (42AC, GRAS Sound and Vibration, Holte, Denmark) and the 250 Hz reference signal was recorded on the datalogger. The pistonphone coupler itself was calibrated against a calibrated pressure microphone (46AO, GRAS Sound and Vibration, Holte, Denmark).
Equipment was exchanged twice during the monitoring period. Detailed information about individual deployments can be found in Supplementary Table S2.
Porpoise Detections
The recorded data was processed off-line by the associated software package CPOD.exe (v 2.044), which groups clicks into clusters (trains) and classifies the trains based on parameters such as peak sound pressure, mean instantaneous frequency and inter-click intervals. The selection criteria were custom set to exclude all trains classified by the built-in KERNO classifier to have originated from dolphins and sonars/echo-sounders and to include only click trains found by KERNO with at least five consecutive clicks with a mean instantaneous frequency between 100 and 160 kHz and a minimum duration of 10 cycles (equal to 77 μs for a 130 kHz pure tone signal). Harbor porpoises produce narrow-band pulses around 130 kHz, with a 50–100 μs duration, and essentially without any energy below 110 kHz (Møhl and Andersen, 1973; Kyhn et al., 2013). This procedure follows the study of Clausen et al. (2018), which showed that these filter settings performed slightly better than standard KERNO settings (CetHigh and CetMod) in the noise environment near offshore installations of the central North Sea.
Porpoise detections were aggregated into hourly values of; (i) number of porpoise clicks per minute (CPM), (ii) porpoise positive minutes (PPMs) in binary output (i.e., either no click train or at least one click train containing at least five clicks within a given minute, as defined by Clausen et al., 2018) and (iii) the ratio of minutes with high repetition rate click trains called buzzes (i.e., <15 ms between clicks) to minutes with any click train calculated per hour (BPM/PPM). Buzzing is presumed to be indicative of foraging behavior (Carlström, 2005; Todd et al., 2009; Wisniewska et al., 2016, 2018) However, social communication is similar to buzzes and may be interpreted as feeding buzzes. One study has estimated that about 26% of all high-repetition rate click trains may be social calls and therefore not related to feeding (Sørensen et al., 2018). In the present analysis we are not able to distinguish between feeding buzzes and social communication. Changes in the occurrence of high-repetition rate click trains can thus be either a change in feeding activity, in social communication, or both.
Sound Recordings
The sound files from the six stations with full bandwidth recorders were analyzed using Matlab (v. R2014b, Mathworks, Inc.) with custom-written routines for airgun pulse detection and noise level analysis. Airgun pulses were detected by an envelope level detector with variable thresholds, and detections were manually verified by visual inspection. Only recordings for which the active seismic source vessel was known to be less than 20 km from the recorder were analyzed as the signal to noise ratio at larger distances was not sufficiently high to allow for automatic detection. Sound signals were frequency weighted according to recommendations by National Marine Fisheries Service (2016) using the HF-cetacean weighting function. The weighted 90% pulse energy was computed for each pulse (Madsen, 2005). Furthermore, the running rms-average with a time constant of 125 ms (Leq–fastHF, Tougaard et al., 2015) were computed for all detected and frequency weighted airgun signals (Tougaard and Beedholm, 2018). The broadband sound exposure level of a single shot (SELSS) was calculated for each airgun pulse.
Statistical Analyses
Links between the seismic survey and porpoise acoustic activity were explored at a small spatiotemporal scale of minutes and at a large spatiotemporal scale comparing periods before, during and after the survey. In the small scale analysis, variation in PPM (a binary variable on the minute level), CPM and BPM/PPM were modeled as a function of either distance from the seismic vessel with an active airgun, received SELSS, or received Leq–fastHF. Preliminary data analyses revealed non-linear relationships between the response variables and the covariates and we therefore analyzed the data using generalized additive mixed models (GAMMs). PPM (binomial), BPM/PPM (ratio 0–1) and CPM (Poisson) were included as response variables in separate models with Station ID as a random variable to account for unbalanced data over space and time and a first order autoregressive correlation structure was used to account for serial dependence between observations. AIC and diagnostic plots of model residuals were used to verify whether the inclusion of the correlation function and random effect improved both the model fit and the associated statistical assumptions. Distance to seismic vessel, SELSS, and Leq–fastHF were fitted as fixed effect smooth terms, in separate models, with the optimal curve estimated by generalized cross-validation procedure (Wood, 2006). Through this approach, a total of nine generalized additive mixed models were created. To assess which covariate best predicted small-scale responses, we report for each GAMM the amount of variation in the data explained (R2adj). All statistical analyses were carried out with the software package R version 3.4.1 (R Development Core Team, 2015).
In the large-scale analysis, stations were divided into groups to test for differences in CPM, PPM, and BPM/PPM using a Before-After Control-Impact (BACI) style design (Green, 1979; Carstensen et al., 2006). One group consisting of six stations was designated as “impact” stations, as they are inside or immediately outside (2–3 km) the seismic survey area (Dagmar, DanF, Halfdan, Kraka, Regnar, and Skjold). The three remaining stations, more than 15 km from the seismic source vessel at any time, were designated as “control” stations (Ref.1, Ref.2 and Rolf, Figure 1). This was coupled with the separation of time periods into before (prior to the survey start), during-off (during the survey with inactive airguns), during-on (during survey with active airguns active) and after (after the end of the survey). Insufficient usable data was recovered from the reference stations in the post-survey period (approximately only 1.5 months of data from Rolf and no data from Ref.1 or Ref.2 were collected, likely due to trawling of the equipment when deployed at the stations) to justify inclusion of the combination “after-control” in the BACI analysis. For each of the remaining seven combinations of area and period the hourly values were aggregated for CPM, PPM and calculated for BPM/PPM per hour as statistical models based on 1-min resolution data were too large and did not converge. Class (combinations of area and period) was fitted as an explanatory variable with hourly CPM, PPM, and BPM/PPM as response variables in separate generalized mixed effect models. In addition to class, Julian day, hour of the day and the resulting second order polynomials were included as explanatory variables to account for any variation in the seasonal and diurnal activity of porpoises (Figure 2). PPM were summed for each hour (maximum value of 60) and then divided by 60 to get a 0–1 range value, which was then analyzed in binomial models with Station ID as a random effect to account for unbalanced data over space and time. The same model formulation was used to estimate variation in number of clicks, i.e., CPM h–1 though here we employed a generalized mixed model with a Poisson distribution. Differences in the modeled means based on the output of the large-scale BACI analysis between the seven classes were estimated using a post hoc Tukey honest significant difference (HSD) tests for mixed models. We used a threshold of p < 0.05 to assign significant differences in mean CPM, PPM, and BPM/PPM h–1.
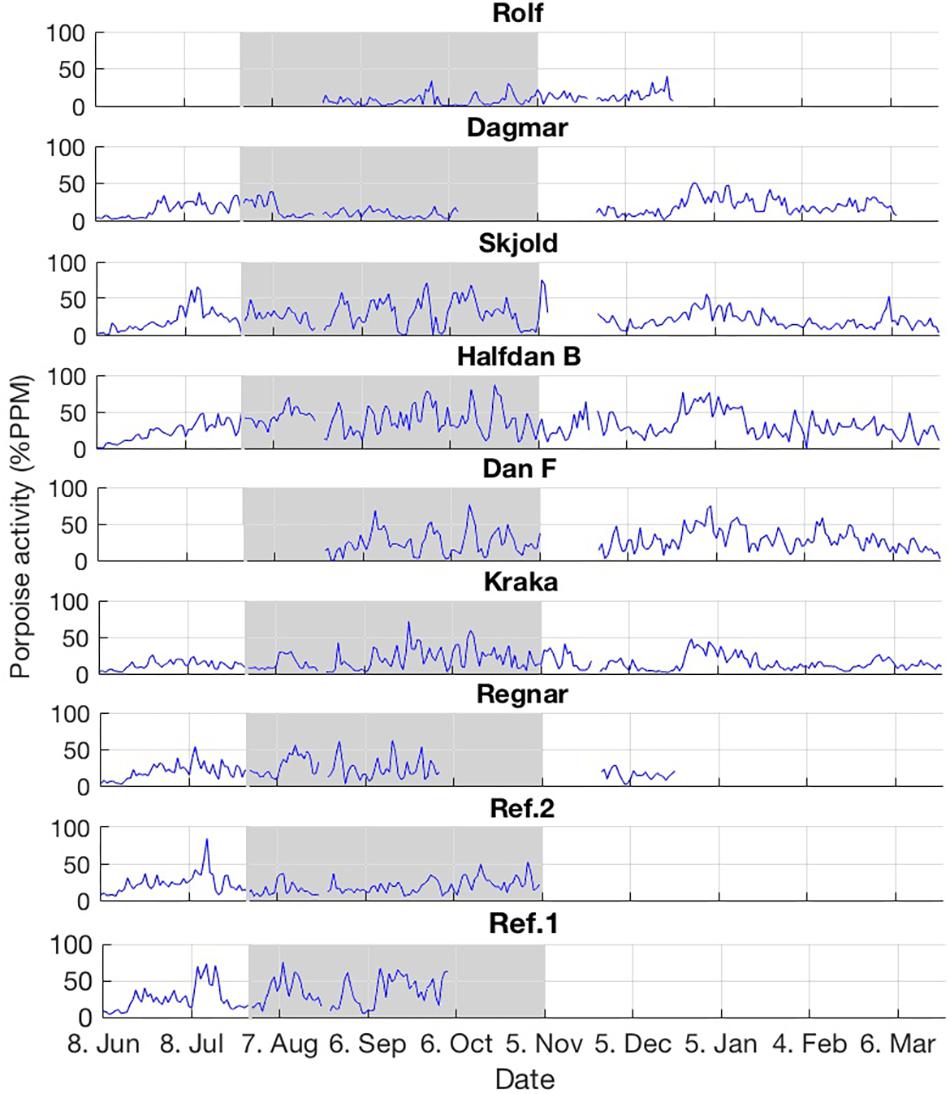
Figure 2. Porpoise acoustic activity expressed as percent porpoise positive minutes per day (% PPM) for the nine stations during the entire study period June 2016–March 2017. The shaded gray time period indicates when the seismic survey was conducted.
Results
Harbor porpoises were detected at all stations throughout the study period from June 2017 to March 2018 (Figure 2 and Supplementary Figure S1). Often, high porpoise acoustic activity levels were observed with more than 50% porpoise positive minutes per day. An overall natural seasonal pattern was also present, with increasing levels of activity from mid June through October and gradually declining toward mid December when increasing activity was seen until mid January and then gradually declining toward March (Figure 2). These overall natural variations are probably related to prey availability movements followed by harbor porpoises in the general Norths Sea area. We are only beginning to understand what drives movements and what habitats are important to porpoises in the North Sea (van Beest et al., 2018a, Figure 2).
Small Scale Responses
At the smallest spatiotemporal scale, porpoise acoustic activity varied non-linearly with distance to the seismic source vessel (Figure 3A). All three indicators of porpoise acoustic activity (CPM, PPM, and BPM/PPM) showed a dose-response effect with lowest activity closest to the source vessel and increased up to a range of 8–12 km after which baseline acoustic activity was attained beyond a range of approximately 12 km. Although there was considerable variation in the received noise level from single pulse SELSS and Leq–fastHF, the noise level correlated well with distance to the source vessel (Figure 4).
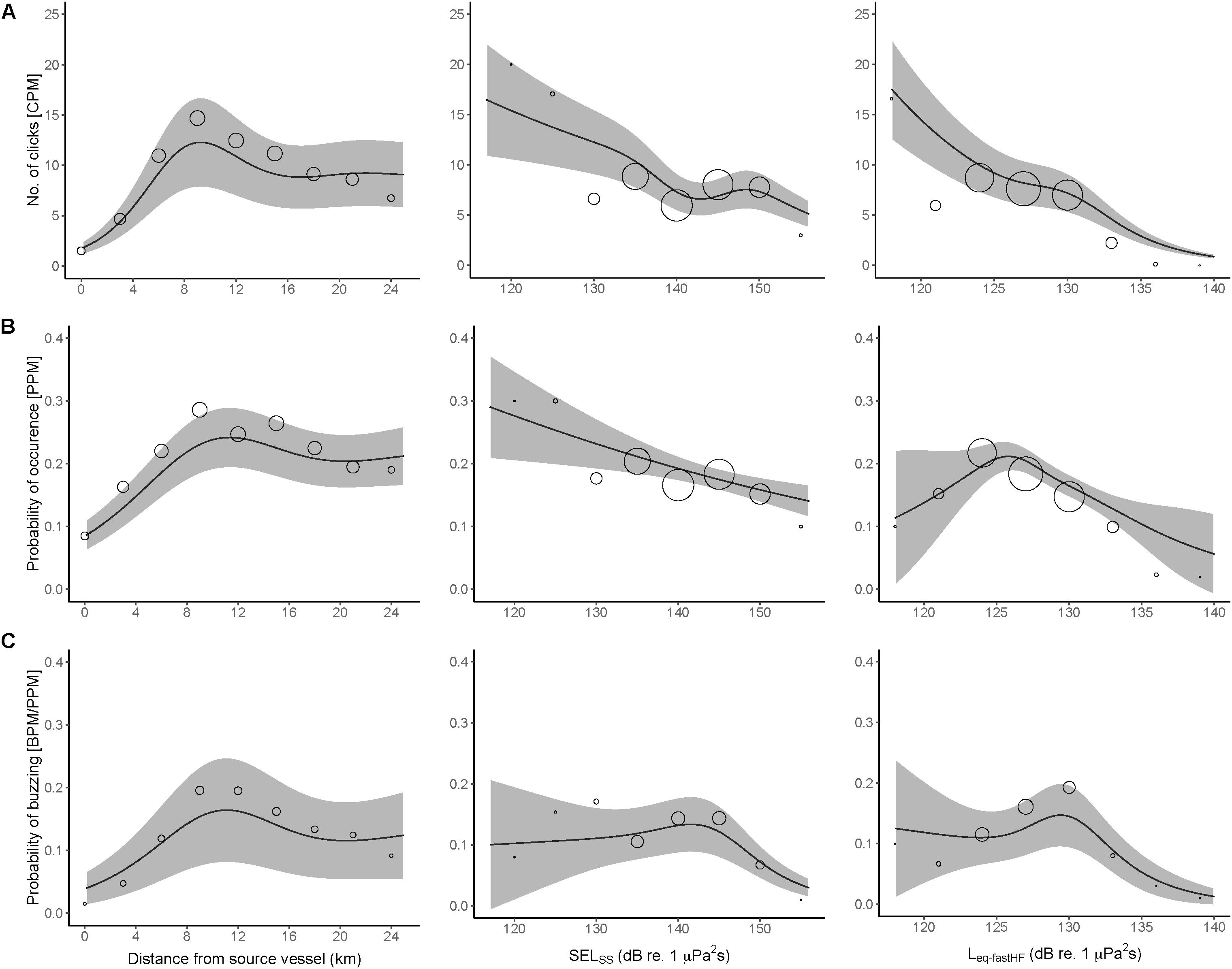
Figure 3. (A) Predicted effect of distance to source vessel with an active airgun, left; SELSS, center; Leq–fastHF, right. The number of porpoise clicks (CPM), (B) porpoise positive minutes (PPM), and (C) the probability of porpoise buzzing (BPM/PPM). Each panel is the result of a separate GAMM (see text for details). Circles indicate the modeled mean of CPM, PPM, and BPM/PPM for each model and 95% CI in gray shading. The size of the circles is proportional to the amount of data in each interval, i.e., the larger the circle the more precise the estimate is.
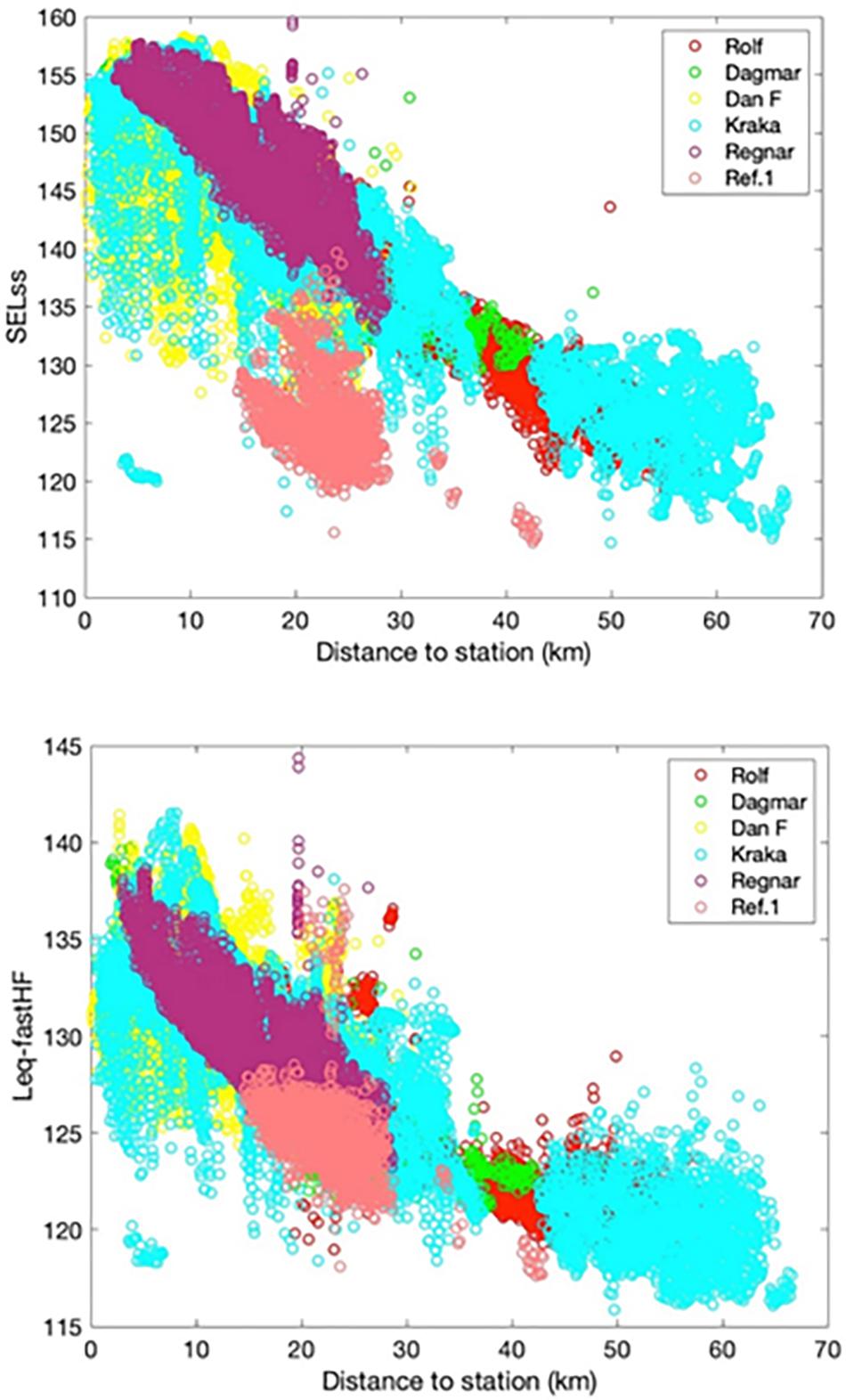
Figure 4. Measured noise level for single pulse SELSS, and Leq–fastHF with distance to source vessel. Different colors indicate the different recording stations shown in the legend. Each pulse is thus included up to six times at different ranges.
The small scale response was characterized by a similar response for CPM, PPM and BPM/PPM where activity decreased as SELSS increased, with the lowest porpoise acoustic activity recorded at SELSS equal to 155 dB re. 1 μPa2s (Figure 3B).
Similar patterns were seen for Leq–fastHF, where only PPM showed an unexpected hump-shape curve with highest porpoise activity at Leq–fastHF = 126 dB re. 1 μPa. All three parameters approached 0 activity at Leq–fastHF = 140 dB re. 1 μPa (Figure 3C). Statistical results of each GAMM can be found in Supplementary Tables S3–S5. Models based on distance from the source vessel as the predictor variable explained more of the variation in the data (for CPM, PPM, and BPM/PPM) than models with SELSS and Leq–fastHF as predictor variables (Supplementary Table S6).
Large Scale Responses
Not all stations provided data over the entire survey period because of equipment loss or technical errors. Overall the dataset covered 1829 station days (one station day corresponds to 24 h of usable data from one station) out of a maximum of 2610 possible days for C-PODs, and 1012 station days out of 1740 maximum possible days for the broad-band sound recorders, which corresponds to 70 and 58% of maximum possible days, respectively. The largest gap in data was in the third deployment period (after the seismic survey), when the data loggers were lost, likely as a result of trawling.
The large scale results show that before the seismic survey started, the mean CPM, PPM, and BPM/PPM did not differ between the “control” and “impact” stations (Tukey HSD: p > 0.05; Figure 5). During the seismic survey, mean CPM h–1 and PPM h–1 increased at both the “control” and “impact” stations. The “impact” stations increase in CPM h–1 with 366% (Tukey HSD: p < 0.001) while PPM h–1 increased 190% (Tukey HSD: p < 0.001) compared to the “before” period (Figures 5A,B). By contrast, the mean BPM/PPM h–1 was lower during the seismic survey compared to the “before” period. This was the case at both “control” and “impact” stations (Tukey HSD: p < 0.05; Figure 5C). There were no statistical differences detected in mean CPM h–1, PPM h–1, and BPM/PPM h–1 during the seismic survey between “control” and “impact” stations (Tukey HSD: p > 0.05 for all comparisons). After the seismic survey, the mean CPM, PPM, and BPM/PPM h–1 at “impact” stations remained stable and similar to levels detected during the seismic survey period (Tukey HSD: p > 0.05 for all comparisons; Figure 5). Model outputs of the generalized mixed models are shown in the Supplementary Table S7.
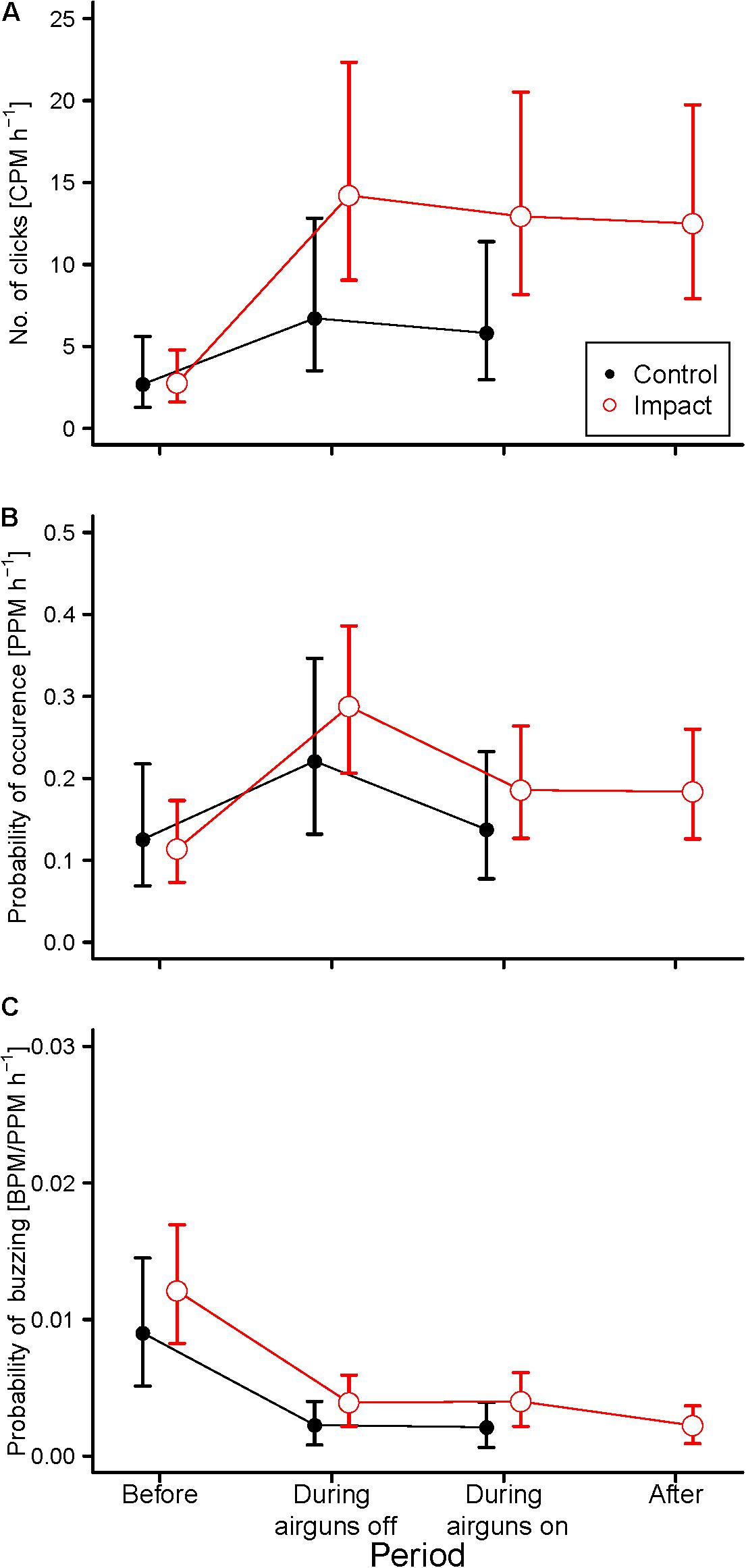
Figure 5. Predicted (circles: mean and whiskers: 95% CI) porpoise activity at the “control” and “impact” stations, before, during (with airguns off or on) and after the seismic survey, expressed as, (A) the number of porpoise clicks (CPM h−1), (B) probability of occurrence (PPM h−1), (C) probability of buzzing (BPM/PPM h−1). Each panel is the result of a separate generalized mixed model (see text for details) (Supplementary Table S7). Each model included Julian day and hour of the day as covariates to accommodate variation in seasonal and diurnal activity of porpoises.
Discussion
The reaction of harbor porpoises to the seismic survey showed a decrease in recorded CPM (a measure of how intensely echolocation was used), PPM (proxy for porpoise presence) and BPM/PPM (measure of foraging buzzes and social calls) up to 8–12 km away from the source vessel. This suggests that the density of porpoises is reduced in a circle of 8–12 km around the moving seismic vessel. This effect has a shorter range than the reaction observed in response to pile driving of wind farm foundations, where significantly fewer porpoises were observed out to approximately 20 km (Tougaard et al., 2009; Brandt et al., 2011; Dähne et al., 2013a). The reaction of harbor porpoises to seismic surveys are thus comparable to reactions to pile driving with the use of attenuating air bubble curtains (Dähne et al., 2017). A plausible explanation for the small-scale responses that occurred out to 8–12 km from the source vessel compared to the stronger responses to pile driving could be related to the difference between a moving sound source (as in seismic surveys), and a stationary sound source (as in pile driving). The moving seismic vessel may encounter many animals on its path. The sound level will increase steadily and, after passing the point of closest approach to the porpoise, the sound level decreases quickly, which in turn helps mitigate the sound effect. Additionally, porpoises may be able to hear the seismic shooting faintly over the whole survey period, potentially initiating habituation. Alternatively, in pile driving the animal has no choice but to move away from the noise if the noise affects them significantly, which can take hours and thus extend the duration of the impact.
Results of the large scale responses analyzed by the BACI analysis, showed no significant change between the control and impact stations. BACI also take natural fluctuations in porpoise density into account, by pairing results from the reference stations at the same time, and thereby, only the relative changes in porpoise activity was used to measure the effect of the seismic survey. This means that porpoises used the general seismic survey area at any time to a similar degree as the reference stations placed 15 km away from any survey activities. This suggests that there were no long-term and large-scale displacements of porpoises during the 103 days of seismic shooting. These results correspond well to the findings reported by Pirotta et al. (2014) for a 12-day survey with a smaller airgun array of 470 cubic-inch. However, it is not known whether it was the same animals that remained in the area during the survey or displaced animals were continuously replaced by new animals moving into the area during the seismic survey. Some tagged porpoises exposed to a single small airgun (10 cubic-inch) for only 1 min showed strong responses for up to 8 h and moved 10s of km away from the sound source indicating that some animals leave an area with seismic activity, while others may be more resistant to noise disturbance or move into the area from elsewhere (van Beest et al., 2018b). Marine mammal observers were on board the seismic vessels, but the very few and short encounters with porpoises did not allow for any inference about behavior of individuals. The general increase in porpoise activity in the whole study area over time suggests a seasonal porpoise migration into or through the area. Such seasonal changes have also been observed in the Dutch North Sea (e.g., Scheidat et al., 2011) and are likely related to annual changes in prey availability.
The performance of any detector is influenced by noise. The effect of noise on detecting porpoises with stationary acoustic monitoring devices has recently been quantified in the same area as the present study (Clausen et al., 2018). The airgun pulses themselves are short, with little energy above 20 kHz (the lower bandwidth limit of the C-POD) and with a low duty cycle (pulse interval 5–10 s). Thus, they have limited capacity to mask detection of the ultrasonic clicks of porpoises (around 130 kHz). Moreover, the aggregation of clicks into detection-positive minutes is robust to the effects of seismic noise, as the absolute number of clicks detected within 1 min is collapsed into a single, binary value of clicks either present or absent. The airgun pulses are therefore unlikely to have affected the detection of porpoise clicks. Furthermore, background noise was not included in this study as there is no straight forward way to correct echolocation clicks based on variations in background noise. Also, during the small scale response noise may be seen as a constant, the fact that no significant change in the large scale overall distribution of porpoises was seen does not suggest that variations in ambient noise would impact the outcome of the results.
A decrease in the detection rate of echolocation clicks and buzzing can be interpreted in several ways. First, an actual deterrence can occur, in which porpoises swim away from the seismic source vessel. Secondly a cessation of echolocation activity can occur, either in CPM which is indicative of the porpoises’ exploratory behavior (i.e., more clicks in a minute indicate exploring objects a shorter distances), PPM which is an indicator of porpoise presence and general use of echolocation or BPM/PPM indicative of feeding and social communication. A lowering in buzzing has been observed during the passing of noisy vessels (Wisniewska et al., 2018), while CPM and PPM was significantly reduced during wind farm construction and operation (Teilmann and Carstensen, 2012). Changes in behavior may lead to a reduction in echolocation parameters, for example when swimming at the surface, where sound propagation is reduced (van Beest et al., 2018b), if source level of the emitted echolocation clicks are reduced by the animal (Teilmann et al., 2002), or a change from pelagic to bottom feeding occur (Schaffeld et al., 2016). In any case, the reduction of recorded echolocation activity is indicative of a change in behavior, meaning that porpoises were affected by this disturbance. So, except for the unlikely event that animals changed to surface feeding where they may be less acoustically detectable, the animals had less time available for foraging, communicating or any other behaviors they were engaged in when they were disturbed. The decrease in the buzz ratio found here, is in line with previous findings of Pirotta et al. (2014) and indicates that underwater noise can reduce foraging activity or social calling of porpoises. These similarities were found even though the magnitude of the two surveys differed markedly, both in terms of airgun array size and in the duration of the survey.
Three different explanatory variables (distance, SELSS, and Leq–fastHF) were tested against the response variables. Although distance was the overall best predictor of response, each variable correlated well with the response. This is to be expected, as the two noise measurements and distance are highly correlated for stereotypical signals such as airgun pulses. It remains an open question whether the relevant parameter for the porpoises were the perceived loudness of the signal (approximated by the weighted Leq–fastHF), the signal energy (SELSS), the distance per se, or a combination of two or all of these parameters. The fact that the parameters correlate so well in this study, due to the stereotypical nature of the signals, means that the variables’ effects are confounding and cannot easily be separated. To do so, a much more variable sound source would be required.
A few disturbance events are likely insignificant to the energetic status of a porpoise, but these disturbances may have fitness consequences when repeated frequently (Wisniewska et al., 2018). Porpoises are small cetaceans living in a relatively cold environment, resulting in a high demand for energy (Rojano-DonÞate et al., 2018). Indeed, harbor porpoises have been shown to forage almost continuously in some areas; therefore, the disturbance of foraging activities is important to quantify and assess (Wisniewska et al., 2016). The magnitude of these effects on the population level are impossible to quantify at present, due to the lack of data available to track the energy expenditure and intake of individuals with sufficient precision that could be translated directly into impact by the disturbance on the fitness of individuals. Presently, the best alternative to predict the disturbance effects on the population-scale is by means of agent-based models. Such models, including the DEPONS model for harbor porpoises (Nabe-Nielsen et al., 2018), are currently under development. Agent-based models requires a high level of detailed knowledge about the energetics of the animals and, in particular, the energetic consequences of disturbance, which is still in its infancy and should be recommended as a future priority research area.
Data Availability Statement
Scripts used to analyze the data and datasets have been deposited at Dryad upon acceptance of the paper: https://doi.org/10.5061/dryad.7sqv9s4pg.
Ethics Statement
The study took advantage of a seismic survey planned and permitted independently from the research project. The study in itself did not result in any additional exposure to the animals and thus did not require a separate permission under Danish law.
Author Contributions
JTo, JTe, MD, and JB conceived and designed the study. JTo and JB prepared and programed the recording instruments. JTe and JB collected the data. JS, JB, and JTo processed and analyzed the data. FVB performed statistical analysis. JS, JTo, and JTe drafted the manuscript. All authors aided in finalizing it.
Funding
The study was initially funded by Maersk Oil which has now been acquired by Total. Total E&P Denmark A/S funded the work, through a contract with the authors and by means of logistical support in connection with fieldwork (vessel charter and equipment).
Conflict of Interest
MD was employed by company Total E&P Denmark A/S.
The remaining authors declare that the research was conducted in the absence of any commercial or financial relationships that could be construed as a potential conflict of interest.
Acknowledgments
Peter Hickman and Hanno Klemm provided constructive input and support during the planning of the study and the analysis of the results. We thank Signe Sveegaard for creating the map. FOGA ApS crew service vessels are thanked for expert assistance in deployment and recovery of instruments. We also thank Kathrine Whitman for correcting the language and the reviewers for constructive criticism.
Supplementary Material
The Supplementary Material for this article can be found online at: https://www.frontiersin.org/articles/10.3389/fmars.2019.00824/full#supplementary-material
References
Bailey, H., Senior, B., Simmons, D., Rusin, J., Picken, G., and Thompson, P. M. (2010). Assessing underwater noise levels during pile-driving at an offshore windfarm and its potential effects on marine mammals. Mar. Pollut. Bull. 60, 888–897. doi: 10.1016/j.marpolbul.2010.01.003
Blackwell, S. B., Nations, C. S., Mcdonald, T. L., Thode, A. M., Mathias, D., Kim, K. H., et al. (2015). Effects of airgun sounds on bowhead whale calling rates: evidence for two behavioral thresholds. PLoS One 10:e0125720. doi: 10.1371/journal.pone.0125720
Brandt, M. J., Diederichs, A., Betke, K., and Nehls, G. (2011). Responses of harbour porpoises to pile driving at the horns rev II offshore wind farm in the Danish North Sea. Mar. Ecol. Prog. Ser. 421, 205–216. doi: 10.3354/meps08888
Carlström, J. (2005). Diel variation in echolocation behavior of wild harbor porpoises. Mar. Mamm. Sci. 21, 1–12. doi: 10.1111/j.1748-7692.2005.tb01204.x
Carstensen, J., Henriksen, O. D., and Teilmann, J. (2006). Impacts on harbour porpoises from offshore wind farm construction: acoustic monitoring of echolocation activity using porpoise detectors (T-PODs). Mar. Ecol. Prog. Ser. 321, 295–308. doi: 10.3354/meps321295
Castellote, M., Clark, C. W., and Lammers, M. O. (2012). Acoustic and behavioural changes by fin whales (Balaenoptera physalus) in response to shipping and airgun noise. Biol. Conserv. 147, 115–122. doi: 10.1016/j.biocon.2011.12.021
Clausen, K. T., Tougaard, J., Carstensen, J., Delefosse, M., and Teilmann, J. (2018). Noise affects porpoise click detections – the magnitude of the effect depends on logger type and detection filter settings. Bioacoustics 28, 443–458. doi: 10.1080/09524622.2018.1477071
Dähne, M., Gilles, A., Lucke, K., Peschko, V., Adler, S., Kruegel, K., et al. (2013a). Effects of pile-driving on harbour porpoises (Phocoena phocoena) at the first offshore wind farm in Germany. Environ. Res. Lett. 8:025002. doi: 10.1088/1748-9326/8/2/025002
Dähne, M., Verfuss, U. K., Brandecker, A., Siebert, U., and Benke, H. (2013b). Methodology and results of calibration of tonal click detectors for small odontocetes (C-PODs). J. Acoust. Soc. Am. 134, 2514–2522. doi: 10.1121/1.4816578
Dähne, M., Tougaard, J., Carstensen, J., Rose, A., and Nabe-Nielsen, J. (2017). Bubble curtains attenuate noise from offshore wind farm construction and reduce temporary habitat loss for harbour porpoises. Mar. Ecol. Prog. Ser. 580, 221–237. doi: 10.3354/meps12257
Delefosse, M., Rahbek, L., Roesen, L., and Tubbert Clausen, K. (2017). Marine mammal sightings around oil and gas installations in the central North Sea. J. Mar. Biol. Assoc. 98, 993–1001. doi: 10.1017/S0025315417000406
Dunlop, R. A., Noad, M. J., Mccauley, R. D., Kniest, E., Slade, R., Paton, D., et al. (2017). The behavioural response of migrating humpback whales to a full seismic airgun array. Proc. Biol. Sci. 284:20171901. doi: 10.1098/rspb.2017.1901
Dyndo, M., Wiśniewska, D. M., Rojano-Doñate, L., and Madsen, P. T. (2015). Harbour porpoises react to low levels of high frequency vessel noise. Sci. Rep. 5:11083. doi: 10.1038/srep11083
Gales, R. S. (1982). Effects of Noise of Offshore Oil and Gas Operations on Marine Mammals - An Introductory Assessment. NOSC Technical Report 844 to the Bureau of Land Management. Washington, D.C: Department of Interior.
Goold, J. C., and Fish, P. J. (1998). Broadband spectra of seismic survey air-gun emissions, with reference to dolphin auditory thresholds. J. Acoust. Soc. Am. 103, 2177–2184. doi: 10.1121/1.421363
Gordon, J., Gillespie, D. C., Potter, J., Frantzis, A., Simmonds, M. P., Swift, R., et al. (2003). A review of the effects of seismic surveys on marine mammals. Mar. Technol. Soc. J. 37, 16–34. doi: 10.1007/978-1-4939-2981-8_16
Green, R. H. (1979). Sampling Design and Statistical Methods for Environmental Biologists. New York, NY: Wiley.
Hammond, P. S., Macleod, K., Berggren, P., Borchers, D. L., Louise, B., Ana, C., et al. (2013). Cetacean abundance and distribution in European Atlantic shelf waters to inform conservation and management. Biol. Conserv. 164, 107–122. doi: 10.1016/j.biocon.2013.04.010
Hermannsen, L., Tougaard, J., Beedholm, K., Nabe-Nielsen, J., and Madsen, P. T. (2015). Characteristics and propagation of Airgun pulses in shallow water with implications for effects on small marine mammals. PLoS One 10:e0133436. doi: 10.1371/journal.pone.0133436
Kastelein, R. A., Hoek, L., De Jong, C. A. F., and Wensveen, P. J. (2010). The effect of signal duration on the underwater detection thresholds of a harbor porpoise (Phocoena phocoena) for single frequency-modulated tonal signals between 0.25 and 160 kHz. J. Acoust. Soc. Am. 128, 3211–3222. doi: 10.1121/1.3493435
Kyhn, L. A., Tougaard, J., Beedholm, K., Jensen, F. H., Ashe, E., Williams, R., et al. (2013). Clicking in a killer whale habitat: narrow-band, high-frequency biosonar clicks of harbour porpoise (Phocoena phocoena) and Dall’s porpoise (Phocoenoides dalli). PLoS One 8:e63763. doi: 10.1371/journal.pone.0063763
Kyhn, L. A., Tougaard, J., Thomas, L., Poulsen, L. R., Steinbæk, J., Desportes, G., et al. (2012). From echolocation clicks to animal density – acoustic sampling of harbour porpoises with static dataloggers. J. Acoust. Soc. Am. 131, 550–560. doi: 10.1121/1.3662070
Kyhn, L. A., Wisniewska, D. M., Beedholm, K., Tougaard, J., Simon, M., Mosbech, A., et al. (2019). Basin-wide contributions to the underwater soundscape by multiple seismic surveys with implications for marine mammals in Baffin Bay, Greenland. Mar. Pollut. Bull. 138, 474–490. doi: 10.1016/j.marpolbul.2018.11.038
Madsen, P. T. (2005). Marine mammals and noise: problems with root mean square sound pressure levels for transients. J. Acoust. Soc. Am. 117, 3952–3957. doi: 10.1121/1.1921508
Møhl, B., and Andersen, S. (1973). Echolocation: high-frequency component in the click of the harbour porpoise (Phocoena ph. L.). J. Acoust. Soc. Am. 54, 1368–1372. doi: 10.1121/1.1914435
Nabe-Nielsen, J., van Beest, F. M., Grimm, V., Sibly, R. M., Teilmann, J., and Thompson, P. M. (2018). Predicting the impacts of anthropogenic disturbances on marine populations. Conserv. Lett. 11:e12563. doi: 10.1111/conl.12563
National Marine Fisheries Service, (2016). Technical Guidance for Assessing the Effects of Anthropogenic Sound on Marine Mammal Hearing Underwater Acoustic Thresholds for Onset of Permanent and Temporary Threshold Shifts. NOAA Technical Memorandum NMFS-OPR-55. Silver Spring, MA: National Marine Fisheries Service.
Pirotta, E., Brookes, K. L., Graham, I. M., and Thompson, P. M. (2014). Variation in harbour porpoise activity in response to seismic survey noise. Biol. Lett. 10:4. doi: 10.1098/rsbl.2013.1090
R Development Core Team (2015). R: A Language and Environment for Statistical Computing. Vienna: R Foundation for Statistical Computing.
Richardson, W. J., Greene, C. R. Jr., Malme, C. I., and Thomson, D. H. (1995). Marine Mammals and Noise. London: Academic Press, 547.
Richardson, W. J., Würsig, B., and Greene, C. R. (1986). Reactions of bowhead whales, Balaena mysticetus, to seismic exploration in the Canadian Beaufort Sea. J. Acoust. Soc. Am. 79, 1117–1128. doi: 10.1121/1.393384
Rojano-DonÞate, L., McDonald, B. I., Wisniewska, D. M., Johnson, M., Teilmann, J., Wahlberg, M., et al. (2018). High field metabolic rates of wild harbour porpoises. J. Exp. Biol. 221:185827. doi: 10.1242/jeb.185827
Schaffeld, T., Bräger, S., Gallus, A., Dähne, M., Krügel, K., Herrmann, A., et al. (2016). Diel and seasonal patterns in acoustic presence and foraging behaviour of free-ranging harbour porpoises. Mar. Ecol. Prog. Ser. 547, 257–272. doi: 10.3354/meps11627
Scheidat, M., Tougaard, J., Brasseur, S., Carstensen, J., Petel, T. V. P., Teilmann, J., et al. (2011). Harbour porpoises (Phocoena phocoena) and wind farms: a case study in the Dutch North Sea. Environ. Res. Lett. 6:025102. doi: 10.1088/1748-9326/6/2/025102
Sørensen, P. M., Wisniewska, D. M., Jensen, F. H., Johnson, M., Teilmann, J., and Madsen, P. T. (2018). Click communication in wild harbour porpoises (Phocoena phocoena). Sci. Rep. 8:9702. doi: 10.1038/s41598-018-28022-8
Stone, C. J., and Tasker, M. L. (2006). The effects of seismic airguns on cetaceans in UK waters. J. Cetacean Res. Manag. 8, 255–263.
Teilmann, J., and Carstensen, J. (2012). Negative long term effects on harbour porpoises from a large scale offshore wind farm in the Baltic - evidence of slow recovery. Environ. Res. Lett. 7:045101. doi: 10.1088/1748-9326/7/4/045101
Teilmann, J., Miller, L., Kirketerp, T., Kastelein, R., Madsen, P. T., Nielsen, B. K., et al. (2002). Characteristics of echolocation signals used by a harbour porpoise (Phocoena phocoena) during a target detection experiment. Aqua. Mamm. 28, 275–284.
Thompson, P. M., Brookes, K. L., Graham, I. M., Barton, T. R., Needham, K., Bradbury, G., et al. (2013). Short-term disturbance by a commercial two-dimensional seismic survey does not lead to long-term displacement of harbour porpoises. Proc. R. Soc. B Biol. Sci. 280:8. doi: 10.1098/rspb.2013.2001
Todd, V. L. G., Pearse, W. D., Tregenza, N. J. C., Lepper, P. A., and Todd, I. B. (2009). Diel echolocation activity of harbour porpoises (Phocoena phocoena) around North Sea offshore gas installations. ICES J. Mar. Sci. 66, 734–745. doi: 10.1093/icesjms/fsp035
Tougaard, J., and Beedholm, K. (2018). A practical implementation of auditory time and frequency weighting in marine bioacoustics. Appl. Acoust. 145, 137–143. doi: 10.1016/j.apacoust.2018.09.022
Tougaard, J., Carstensen, J., Teilmann, J., Skov, H., and Rasmussen, P. (2009). Pile driving zone of responsiveness extends beyond 20 km for harbour porpoises (Phocoena phocoena, (L.)). J. Acoust. Soc. Am. 126, 11–14. doi: 10.1121/1.3132523
Tougaard, J., Wright, A. J., and Madsen, P. T. (2015). Cetacean noise criteria revisited in the light of proposed exposure limits for harbour porpoises. Mar. Pollut. Bull. 90, 196–208. doi: 10.1016/j.marpolbul.2014.10.051
van Beest, F. M., Teilmann, J., Dietz, R., Galatius, A., Mikkelsen, L., Stalder, D., et al. (2018a). Environmental drivers of harbour porpoise fine-scale movements. Mar. Biol. 165:95. doi: 10.1007/s00227-018-3346-7
van Beest, F. M., Teilmann, J., Hermannsen, L., Galatius, A., Mikkelsen, L., Sveegaard, S., et al. (2018b). Fine-scale movement responses of free-ranging harbour porpoises to capture, tagging and short-term noise pulses from a single airgun. R. Soc. Open Sci. 5:170110. doi: 10.1098/rsos.170110
Wisniewska, D. M., Johnson, M., Teilmann, J., Rojano-Doñate, L., Shearer, J., Sveegaard, S., et al. (2016). Ultra-High Foraging Rates of Harbor Porpoises Make Them Vulnerable to Anthropogenic Disturbance. Curr. Biol. 26, 1441–1446. doi: 10.1016/j.cub.2016.03.069
Wisniewska, D. M., Johnson, M., Teilmann, J., Rojano-Donate, L., Shearer, J., Sveegaard, S., et al. (2018). Response to resilience of harbor porpoises to anthropogenic disturbance: must they really feed continuously? Mar. Mamm. Sci. 34, 265–270. doi: 10.1111/mms.12463
Keywords: small odontocete, underwater noise, behavioral response, passive acoustic monitoring, C-POD, frequency weighting, noise logger
Citation: Sarnocińska J, Teilmann J, Balle JD, van Beest FM, Delefosse M and Tougaard J (2020) Harbor Porpoise (Phocoena phocoena) Reaction to a 3D Seismic Airgun Survey in the North Sea. Front. Mar. Sci. 6:824. doi: 10.3389/fmars.2019.00824
Received: 18 March 2019; Accepted: 19 December 2019;
Published: 17 January 2020.
Edited by:
Jeremy Kiszka, Florida International University, United StatesReviewed by:
Ursula Katharina Verfuss, SMRU Consulting, United KingdomRebecca Dunlop, The University of Queensland, Australia
Copyright © 2020 Sarnocińska, Teilmann, Balle, van Beest, Delefosse and Tougaard. This is an open-access article distributed under the terms of the Creative Commons Attribution License (CC BY). The use, distribution or reproduction in other forums is permitted, provided the original author(s) and the copyright owner(s) are credited and that the original publication in this journal is cited, in accordance with accepted academic practice. No use, distribution or reproduction is permitted which does not comply with these terms.
*Correspondence: Jonas Teilmann, anRlQGJpb3MuYXUuZGs=