- 1Institute of Marine Environment and Ecology, National Taiwan Ocean University, Keelung City, Taiwan
- 2Center of Excellence for the Oceans, National Taiwan Ocean University, Keelung City, Taiwan
- 3Graduate Institute of Marine Biology, National Dong Hwa University, Pingtung, Taiwan
- 4National Museum of Marine Biology and Aquarium, Pingtung, Taiwan
- 5Department of Oceanography, National Sun Yat-sen University, Kaohsiung City, Taiwan
Previous studies have found that calcification in coral reefs is generally stronger during the day, whereas dissolution is prevalent at night. On the basis of these contrasting patterns, the diel variations of net community calcification (NCC) were monitored to examine the relative sensitivity of CaCO3 production (calcification) and dissolution in coral reefs to ocean acidification (OA), using two mesocosms that replicated a typical subtropical coral reef ecosystem in southern Taiwan. The results revealed that the daytime NCC remained unchanged, whereas the nighttime NCC decreased between the control (ambient) and treatment (OA) conditions, suggesting that carbonate dissolution could be more sensitive to OA than coral calcification. The average sensitivity of the integrated daily NCC to changes in the seawater saturation state (Ωa) was estimated to be a reduction of 54% in NCC per unit change in Ωa, which is consistent with the global average. In summary, our results support the prevailing anticipation that OA would lead to a reduction in the overall accretion of coral reef ecosystems. However, increased CaCO3 dissolution rather than decreased coral calcification could be the dominant driving force responsible for this OA-induced reduction in NCC.
Introduction
Anthropogenic activities, such as the burning of fossil fuels, land-use changes, and cement production, have led to a rapid rise in atmospheric carbon dioxide (CO2) levels, which have increased from 280 ppm in preindustrial times to a present-day level of 400 ppm (Le Quere et al., 2016). As the atmospheric CO2 concentration increases, the CO2 level in the surface ocean also rises, which reduces the seawater pH, carbonate ion concentration, and calcium carbonate saturation state (Ω), a process known as “ocean acidification” (OA) (Caldeira and Wickett, 2005; Doney et al., 2009). Early experimental evidence has shown a positive correlation between seawater Ω and the calcification rates of coral reef organisms (e.g., scleractinia and coralline algae) (Gattuso et al., 1998; Kleypas et al., 1999; Langdon et al., 2000), which has raised concerns that coral reefs could transition from net accretion to net erosion due to OA-induced reductions in calcification (Kleypas and Yates, 2009; Silverman et al., 2009). Recent in situ CO2 enrichment and OA reversal experiments on a natural reef flat further confirm that OA will compromise the ecosystem function of coral reefs (Albright et al., 2016, 2018).
The CaCO3 accretion of a coral reef is determined by the physical balance between CaCO3 transport onto and off the reef, and the chemical balance between CaCO3 production (calcification) and dissolution, which is referred to as net community calcification (i.e., NCC = calcification – CaCO3 dissolution). Although OA-associated changes are expected to adversely affect the chemical accretion of coral reefs, previous predictions are mostly based on the relationship between Ω and the calcification rates of individual corals or coral reef communities (Pandolfi et al., 2011; van Hooidonk et al., 2014). Nonetheless, the impact of OA on coral reef NCC, and thus chemical accretion, is also dependent on the effect of OA on CaCO3 dissolution, which has received less attention than the effect on calcification. Several studies, including modeling simulations and laboratory, field and mesocosm experiments, have revealed that OA may accelerate CaCO3 dissolution in coral reefs (Andersson et al., 2009; Cyronak et al., 2013; Comeau et al., 2014). Several recent studies have even shown evidence that carbonate dissolution may be more sensitive to OA than coral calcification (Cyronak and Eyre, 2016; Eyre et al., 2018). Therefore, understanding the effect of OA on CaCO3 dissolution could be as important as understanding its effect on biogenic calcification.
Clearly, to improve our ability to predict the future evolution of CaCO3 chemical accretion rates of coral reefs, it is necessary to better understand the relative sensitivities of calcification and dissolution in response to OA. However, we currently know little about this, mainly due to the fact that the alkalinity anomaly technique, which is the prevailing technique used to date, only measures the net effect of gross calcification and dissolution; thus, it is challenging to quantify these two processes independently (Andersson and Mackenzie, 2012). Nevertheless, previous studies have found that the diel variations of gross calcification and dissolution in coral reef communities may have contrasting patterns (Yates and Halley, 2006; Schneider et al., 2009; Albright et al., 2015). During the day, photosynthesizing symbiotic algae consume CO2, resulting in high Ω levels that consequently facilitate calcification (Cohen et al., 2016). In addition, the oxygen and energy produced by photosynthesis can be utilized to support active transport of inorganic carbon to the calcification site and the active removal of protons from the calcification site, both of which would also favor calcification (Jokiel, 2011; Ries, 2011). As a result, higher daytime calcification rates than nighttime rates are well documented (Gattuso et al., 1999); this is also known as light-enhanced calcification (Schneider et al., 2009). In contrast, unlike biologically mediated calcification, CaCO3 dissolution is mostly a thermodynamically constrained geochemical response to changes in seawater chemistry (Eyre et al., 2014). CO2 produced from respiration at night decreases Ω levels and thus facilitates CaCO3 dissolution. Observations from the natural environment and experiments conducted in mesocosms have shown evidence that net dissolution primarily takes place at night (Leclercq et al., 2002; Langdon et al., 2003; Yates and Halley, 2006; Andersson et al., 2009; Comeau et al., 2014). Therefore, the contrasting patterns in the diel variations of calcification and dissolution in coral reefs have the potential to be utilized to examine their relative sensitivities to OA.
In this study, in order to verify if carbonate dissolution could be more sensitive to OA than biological calcification, we investigated the effects of OA on the diel variations of NCC in a typical subtropical coral reef community, which was replicated and incubated in mesocosms over 5 weeks. We hypothesized that if the calcification is more sensitive to OA than dissolution, the daytime NCC would be depressed more than the nighttime NCC under OA conditions. In contrast, if dissolution is more sensitive to OA than calcification, the nighttime NCC would decrease more than the daytime NCC under OA conditions.
Materials and Methods
Coral Reef Mesocosm Facility
The coral reef mesocosm facility is located at the National Museum of Marine Biology and Aquarium (NMMBA, Figure 1). Two mesocosms (5.14 m2 × 1 m depth) were designed to simulate the fringing reefs in southern Taiwan (Liu et al., 2009). The bottom of each tank was covered by a 3 cm layer of coral sand, and six artificial rocks were distributed on the sand. A plastic plate (180 cm × 120 cm) was placed on six artificial rocks, and two square baskets (81.5 cm × 81.5 cm × 6.5 cm high) covered with coral sand were placed on the plastic plate to simulate a reef flat. Sand-filtered seawater pumped directly from the adjacent Houwan Bay was added to each mesocosm at an exchange rate of 10% d–1 of the total volume. Each tank contained two pumps: one was used to generate cyclical currents (250 W, 7,200 L h–1, Trundean, Taiwan), and the other was set up at the front of each tank to produce waves (SE200, WAVEMAKER, Taiwan). The water temperatures were maintained at 25°C via a heat-exchanger cooling system (GREAT HELPER System & XT130C, Dixell, Italy), and the precision was 0.3°C. For each of the two mesocosms, LED lamps (wavelength 380∼780 nm, XLamp XT-E LEDs, Cree, Taiwan) were used from 7:00 to 17:00 (photoperiod: light:dark = 10:14). The photosynthetically active radiation (PAR) at a depth of 0.5 m in the tank was maintained at 258 ± 16.7 μmol photons m–2 s–1 as detected by a photometer (LI-1400, LI-COR, Germany). The Apex AquaController system (Neptune System, United States) was used to monitor the pH and water temperature in real time and to control the on-off switch of the electromagnetic valve to release CO2. The release of CO2 was initiated when the pH meter sensed a pH value >7.80 and stopped when the pH was <7.70.
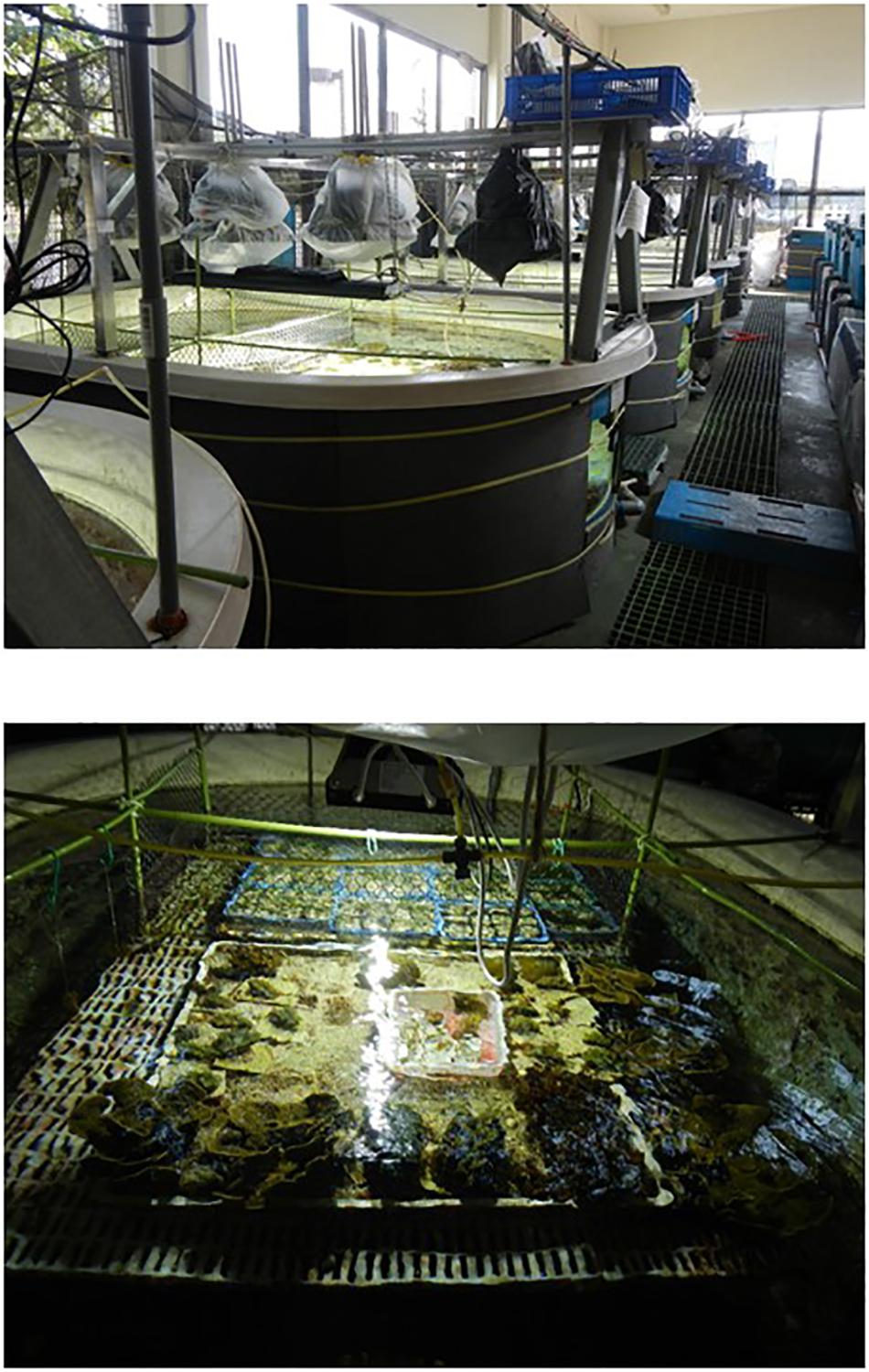
Figure 1. Continuous-flow of coral reef mesocosm facility at the National Museum of Marine Biology and Aquarium, southern Taiwan. Upper panel: outside view; lower panel: inside view.
The biological components of these tanks were prepared from parent colonies originating from coral reefs in Nanwan Bay, and were cultured at the NMMBA for several years prior to the experiment. The trophic levels of these organisms ranged from trophic level I to level III. These coral reef organisms were added in each tank, including 4 sea cucumbers (Holothuria leucospilota), 2 shrimps (Stenopus hispidus), 14 fishes (2 Neoglyphidodon melas, 1 Istigobius decoratus, 3 Salarias fasciatus, and 8 Monodactylus argenteus), 20 corals (1 Millepora sp., 1 Porites spp., 1 Fungiid spp., 1 Heliopora coerulea, 1 Faviid spp., 3 Pavona cactus, 1 Montipora sp., 8 Pocillopora damicornis, and 3 Turbinaria spp.), 20 gastropods (Trochus hanleyanus), 2 sea urchins (Tripneustes gratilla), 14 giant clams (Tridacna sp.), 27 calcifying macroalgae (9 Halimeda opuntia, 9 Mastophora rosea, and 9 Mesophyllum sp.). Coverage of uncolonized sand in the mesocosms was 58% of the bottom area, which was comparable to the 56% in Nanwan Bay (Liu et al., 2009). At the front, where the wave maker was set up to simulate the reef front, the corals Montipora, Turbinaria, Fungiidae and Millepora were deposited. On the front side of the reef flat, the giant clam Tridacna and the corals Turbinaria and Pavona cactus were deposited. The corals Porites, Faviidae, Heliopora coerulea and Pocillopora damicornis were placed in the middle.
After 10 days of acclimation, stage I of the experiment was conducted for 14 days without CO2 treatment (27 March to 9 April 2017), representing ambient (control) conditions. Then, CO2 began to be released into the water to maintain the pH at 7.70–7.80 with the other environmental factors remaining the same. After 1 week of acclimation, Stage II of experiment was conducted for 14 days (17–30 April 2017), representing acidification (treatment) conditions.
Carbonate Chemistry Measurements and Calculations
During the control and treatment periods, discrete seawater samples for TA, DIC, and pH analyses were collected at 6:30 am and 17:30 pm every day. Measurements of TA, DIC, and pH have been detailed in our previous studies (Chou et al., 2016, 2018). Briefly, the TA and DIC were determined using Gran titration on an automatic TA titrator (AS-ALK2, Apollo SciTech Inc.) and the non-dispersive infrared method on a DIC analyzer (AS-C3, Apollo SciTech Inc.), respectively. Certified reference materials (CRMs) obtained from Dr. A. Dickson’s lab at the Scripps Institution of Oceanography were used to calibrate and ensure the performance of both the alkalinity titrator and the DIC analyzer. The analytical TA and DIC measurements both had accuracies of ±0.15% or better. The pH was spectrophotometrically determined at 25°C with a precision of 0.001 (Chou et al., 2016) on the total hydrogen ion concentration scale based on the method described in Clayton and Byrne (1993). The seawater pCO2 and Ωa were calculated from the DIC, TA, temperature, and salinity using the CO2SYS program (Pierrot et al., 2006). For this calculation, the dissociation constants for carbonic acid were obtained from the data of Mehrbach et al. (1973) that were refit by Dickson and Millero (1987). The concentration of calcium ([Ca2+]) was determined from the salinity according to Riley and Tongudai (1967). The Ksp of aragonite was calculated as described by Mucci (1983).
Calculations of Net Community Calcification, Production, and Respiration
For every 1 mol of CaCO3 that precipitates or dissolves, 2 mol of TA is produced or removed. Assuming that the changes in TA in the mesocosms are uniquely attributed to calcification and/or carbonate dissolution, the NCC can be easily calculated using the following equation (Andersson et al., 2009):
where and represent the TA inflow and outflow fluxes of the mesocosms, respectively; and is the rate of change of seawater TA in the mesocosms between consecutive sampling times.
Over the course of the control and treatment periods, we have conducted 8 times TA measurements for the inflowing seawater (3/27 6:30 and 17:30, 4/9 6:30 and 17:30, 4/17 6:30 and 17:30, and 4/30 6:30 and 17:30), which ranged from 2257 to 2280 μmol kg–1 with an average of 2268 ± 8 μmol kg–1 and from 2254 to 2281 μmol kg–1 with an average of 2271 ± 9 μmol kg–1 for mesocosms I and II, respectively. The average TA values of 2268 and 2271 μmol kg–1 were taken constantly to represent the inflowing seawater TA concentrations for mesocosms I and II, respectively. The standard deviation of ±9 μmol kg–1 was used to represent the potential TA fluctuation range of the inflowing seawater, which may produce an uncertainty of ±0.02 μmol CaCO3 m–2 h–1 in NCC calculation. The average TA of the dawn (6:30 am) and dusk (17:30 pm) measurements and that of the dusk and next dawn measurements were used to represent the outflowing TA concentrations for the daytime and nighttime NCC calculations, respectively. The TA difference between the dawn and dusk measurements was used to calculate the daytime NCC, whereas the difference between the dusk and dawn measurements of the next morning was used to calculate the nighttime NCC. The net daily NCC was then calculated by integrating the hourly daytime NCC (11 h) and nighttime NCC (13 h) over 24 h. The flow rate and the volume of the tank was 0.021 m3 h–1 and 5 m3, respectively.
Similarly, the net community production (NCP) and respiration (R) can be calculated from DO data using the following equation:
where is the rate of change of seawater DO in the mesocosms between consecutive sampling times; and and represent the DO inflow and outflow fluxes of the mesocosms, respectively. The DO difference between the dawn and dusk measurements was used to calculate the daytime NCP, whereas the difference between the dusk and dawn measurements of the next morning was used to calculate the nighttime R. The saturated DO values, calculated from the temperature and salinity data, were taken to represent the inflowing seawater DO concentrations. The average DO of the dawn and dusk measurements and that of the dusk and next dawn measurements were used to represent the outflowing DO concentrations for the daytime NCP and nighttime R calculations, respectively.
Statistical Analysis
Differences in all of the parameters’ means between the control and treatment periods in each mesocosm and between the two mesocosms during each period, including DIC, TA, pCO2, pH, Ωa, DO, salinity, and daytime, nighttime, and daily NCC, daytime NCP, and nighttime R were assessed using an analysis of variance (f-test) followed by a comparison of the mean values (t-test), and a significance level (p-value) of 0.05 was used to determine significant statistical differences, which were consistent with those used in our previous studies (Chou et al., 2016, 2018). All of the statistical analyses were performed with Excel 2016.
Results
Variations in Seawater Carbonate Chemistry, Dissolved Oxygen, and Salinity During the Control and Treatment Periods
The variation ranges, means and standard deviations (1σ) of the salinity-normalized dissolved inorganic carbon (NDIC) and total alkalinity (NTA), partial pressure of CO2 (pCO2), aragonite saturation state (Ωa), dissolved oxygen (DO), and salinity in the two mesocosms during the control and treatment periods are summarized in Table 1. During the initial control period, all parameters did not significantly differ between the two mesocosms (except salinity, p < 0.05; Table 2), which provided confidence to move forward with an OA treatment. In contrast to the initial control period, all carbonate chemistry parameters showed a significant difference between the control and treatment periods in both mesocosms (p < 0.05; Table 2), confirming OA treatment indeed have caused changes in carbonate chemistry. Salinity varied within a relatively narrow range from 34.5–35.6 and 34.6–35.7 in mesocosms I and II, respectively, and did not show an increasing trend over time (Figure 2G). Statistically, salinity during the treatment period was slightly lower than that during the control period (34.8 vs. 35.2 and 34.8 vs. 35.3 in mesocosms I and II, respectively; Table 1), suggesting that changes in source water could be more important than evaporation on the variation of salinity over the time frame of the experiments.
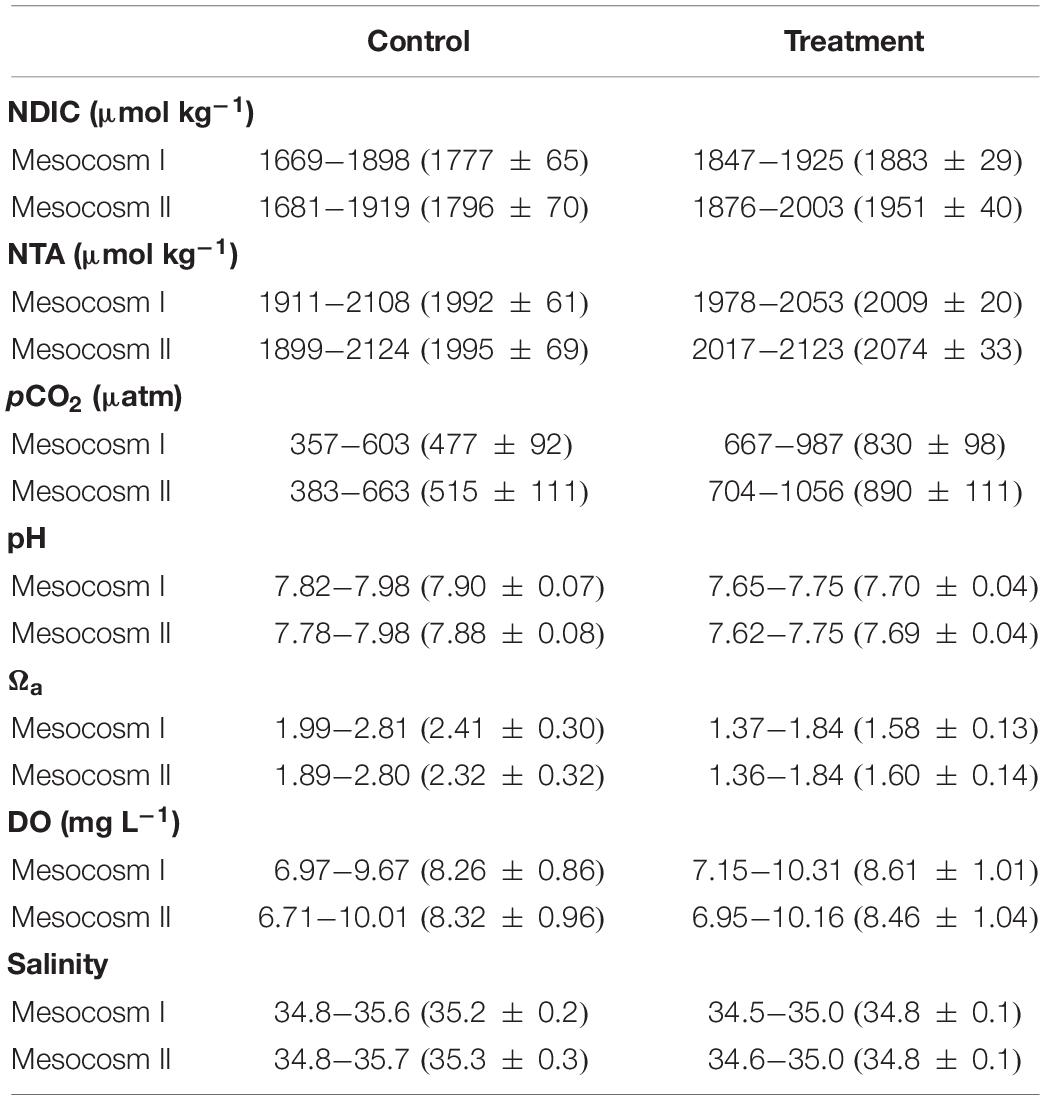
Table 1. Summary of the variation ranges and means ± standard deviations of the salinity-normalized DIC (NDIC) and TA (NTA), pCO2, pH, Ωa, DO and salinity in the replicate mesocosms over the course of the control and treatment periods.
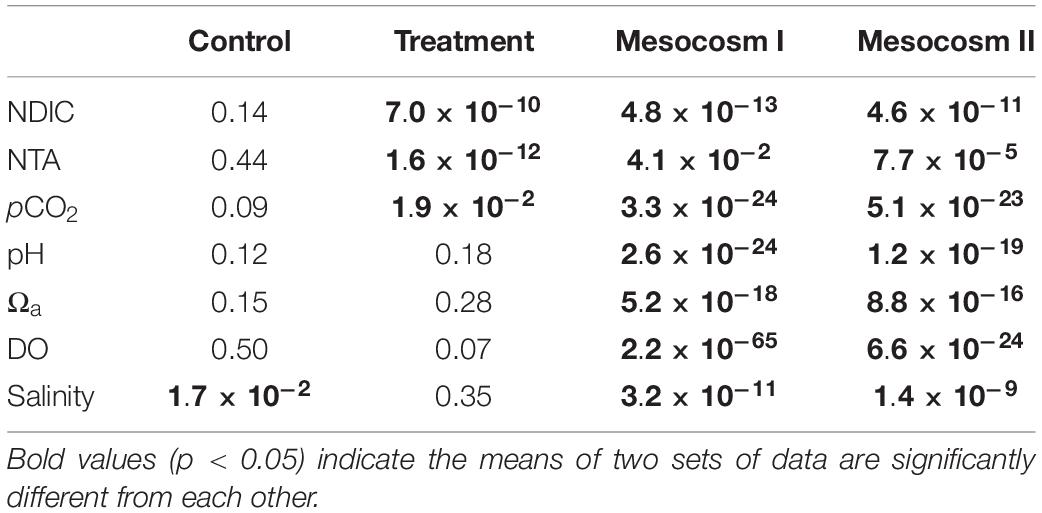
Table 2. Student’s t-test results (p-value) comparing the difference in salinity normalized DIC (NDIC) and TA (NTA), pCO2, pH, Ωa, DO and salinity between the replicate mesocosms during the control and treatment periods, and the difference between the control and treatment periods in the replicate mesocosms.
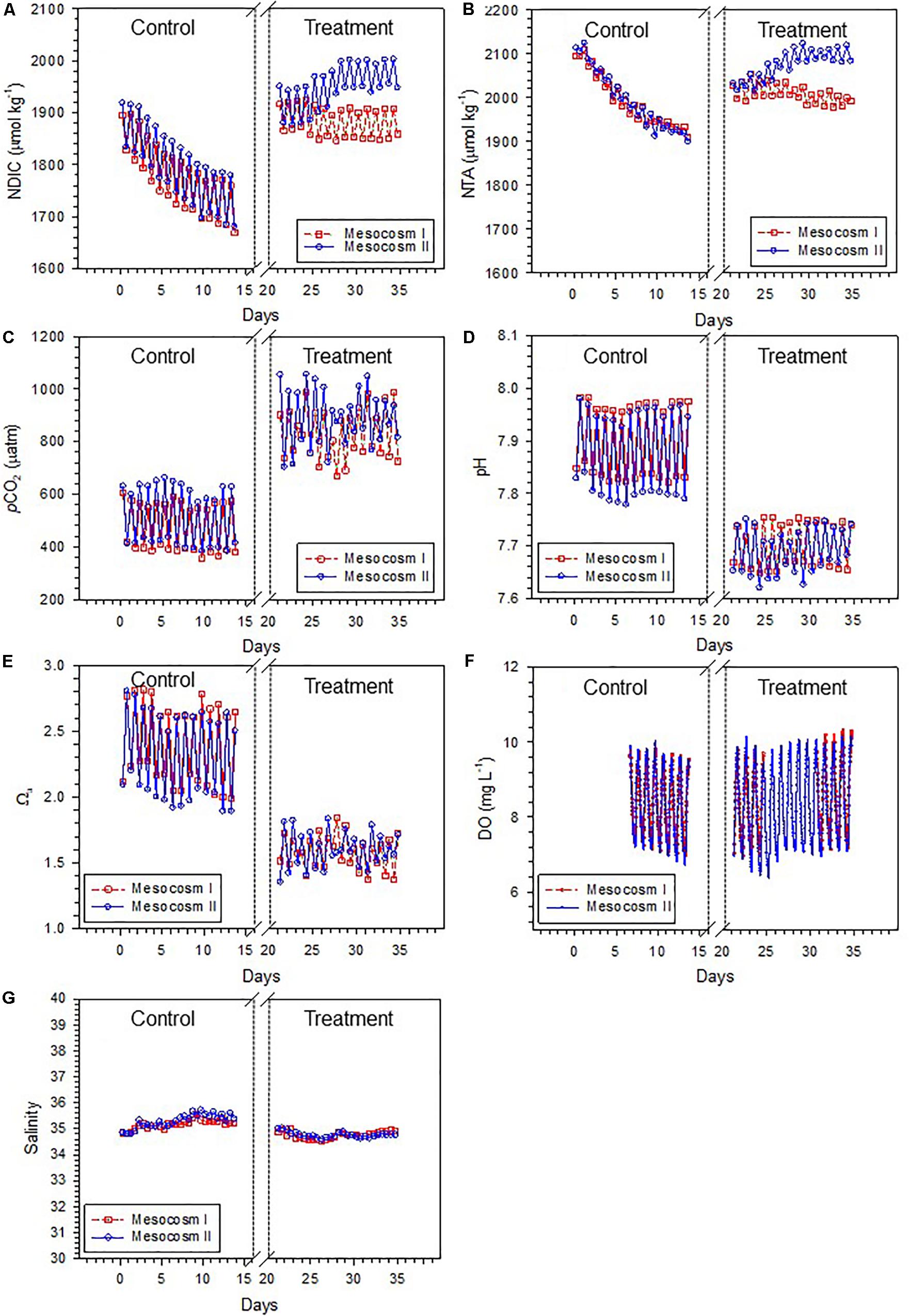
Figure 2. Temporal variations of (A) salinity-normalized dissolved inorganic carbon (NDIC), (B) salinity-normalized total alkalinity (NTA), (C) partial pressure of CO2 (pCO2), (D) pH, (E) aragonite saturation state (Ωa), (F) dissolved oxygen (DO), and (G) salinity in mesocosms I and II during the control and treatment periods.
The acidification of seawater during the treatment period was designed to mimic the chemical conditions with respect to pCO2, pH and Ωa that could occur by the end of this century as a result of continued emissions of anthropogenic CO2. As expected, the results showed that pCO2 during the treatment period exceeded that in the control period by 353 μatm (830 ± 98 vs. 477 ± 92) and 375 μatm (890 ± 111 vs. 515 ± 111); the pH was lower by −0.20 (7.70 ± 0.04 vs. 7.90 ± 0.07) and −0.19 (7.69 ± 0.04 vs. 7.88 ± 0.08); and Ωa was lower by −0.83 (1.58 ± 0.13 vs. 2.41 ± 0.30) and −0.72 (1.60 ± 0.14 vs. 2.32 ± 0.32) in mesocosms I and II, respectively. The observed differences in pCO2, pH and Ωa between the two periods in both mesocosms generally agree with the projected changes for the year 2100 under emission scenario A2 (IPCC, 2001). However, because CO2 injection would not result in changes in TA and DO, the observed significant difference in NTA and DO between the two periods (p < 0.05; Table 2) imply that NCC and NCP may have noticeable differences between the two periods, which will be further examined in the following section.
In terms of the differences between mesocosm I and II during the treatment period, pH (7.70 ± 0.04 vs. 7.69 ± 0.04), Ωa (1.58 ± 0.13 vs. 1.60 ± 0.14) and DO (8.61 ± 1.01 vs. 8.46 ± 1.04 mg L–1) remained no significant difference between the two mesocosms (Table 1), but NDIC (1883 ± 29 vs. 1951 ± 40 μmol kg–1), NTA (2009 ± 20 vs. 2074 ± 33 μmol kg–1) and pCO2 (830 ± 98 vs. 890 ± 111 μatm) in mesocosm I was significantly lower by 68 μmol kg–1, 65 μmol kg–1, and 60 μatm than those in mesocosm II, respectively (p < 0.05; Table 2), implying that the extent of responses to OA treatment in each mesocosm could be variable.
Due to metabolic processes, seawater carbonate chemistry generally shows natural variations on diel time scales in coral reefs (Shamberger et al., 2011; Shaw et al., 2015). In this study, the carbonate chemistry conditions of neither the control nor the treatment periods remained at constant levels but, as anticipated, varied throughout the diel cycle. NDIC, NTA, and pCO2 were high just before dawn and low just before dusk with a decreasing trend during the day and an increasing trend at night in both mesocosms (Figures 2A–C). The patterns of pH, Ωa, and DO were similar to those of NDIC, NTA, and pCO2, with lows before dawn and highs before dusk (Figures 2D–F). As a result of CO2 injection during the treatment period, which would depress the daytime increases in pH and Ωa, these parameters generally showed a weaker degree of diel variability during the treatment period than during the control period.
Variations in Daytime, Nighttime and Daily NCC, Daytime NCP, and Nighttime Respiration During the Control and Treatment Periods
The variation ranges, means and standard deviations (1σ) of the daytime, nighttime, and daily NCC, daytime NCP, and nighttime respiration (R) in the two mesocosms during the control and treatment periods are summarized in Table 3. The Student’s t-test results (p-value) comparing the difference in these parameters between the two mesocosms during the control and treatment periods, and the difference between the control and treatment periods in the two mesocosms are given in Table 4.
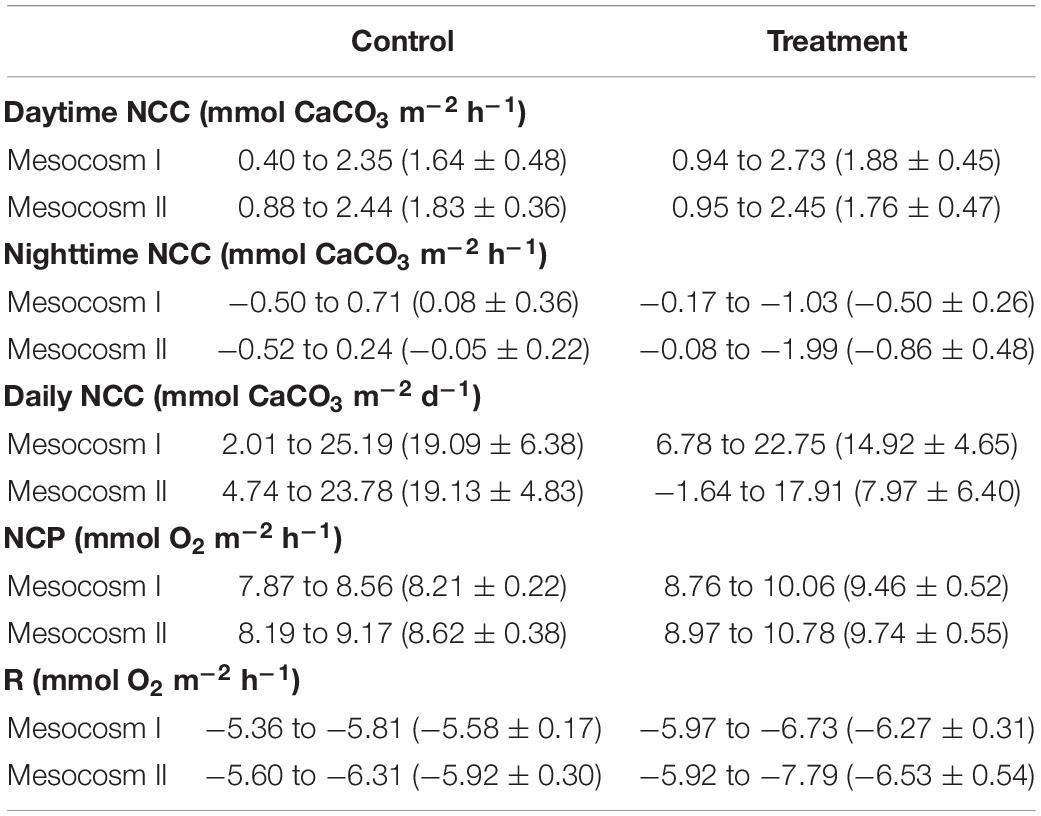
Table 3. Summary of the variation ranges and mean ± standard deviations of the daytime, nighttime, and daily net community calcification (NCC), net community production (NCP), and respiration (R) in the replicate mesocosms over the course of the control and treatment periods.
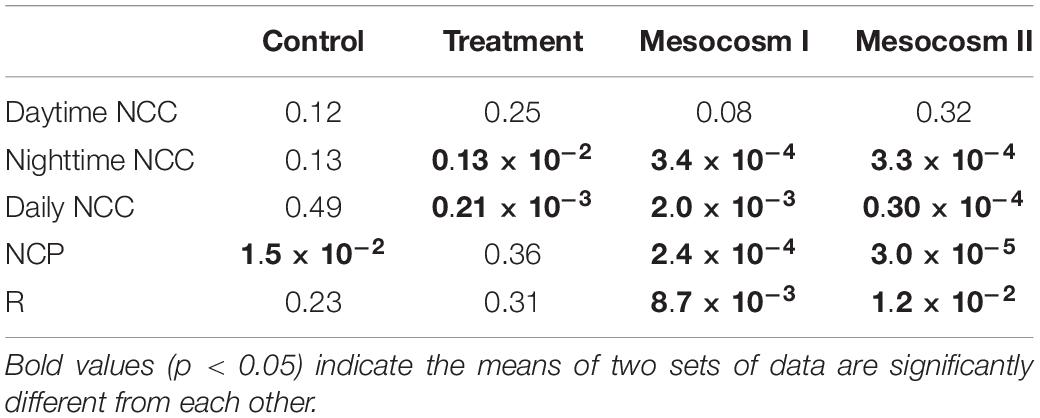
Table 4. Student’s t-test results (p-value) comparing the difference in daytime, nighttime and daily net community calcification (NCC), net community production (NCP), and respiration (R) between the replicate mesocosms during the control and treatment periods, and the difference between the control and treatment periods in the replicate mesocosms.
The daytime NCC was higher than the nighttime NCC during both periods but had a larger diel variation during the treatment period than during the control period (Figures 3A,B) in both mesocosms. The daytime and nighttime NCC showed clearly divergent responses to OA treatment. The daytime NCC remained in a net accretion state (positive NCC) and did not show significant differences between the control and treatment periods (p = 0.08 and 0.32 for mesocosm I and II, respectively; Figures 3E,F). In contrast, the nighttime NCC transitioned from a nearly balance state (NCC close to 0) to a net dissolution state (negative NCC) and decreased significantly between the control and treatment periods in both mesocosms (p < 0.05; Figures 3E,F). Because the daytime NCC was much higher than the nighttime NCC and because it remained nearly unchanged during both the control and treatment periods, the integrated daily NCC remained in a net accretion state during both periods in the two mesocosms (Figures 3C,D), despite the fact that the nighttime NCC transitioned to a net dissolution state during the treatment period. The average daily NCC was also significantly lower during the treatment period than in the control period in both mesocosms (p < 0.05; Figures 3G,H), which mainly reflects the reduction of the nighttime NCC due to the influence of OA.
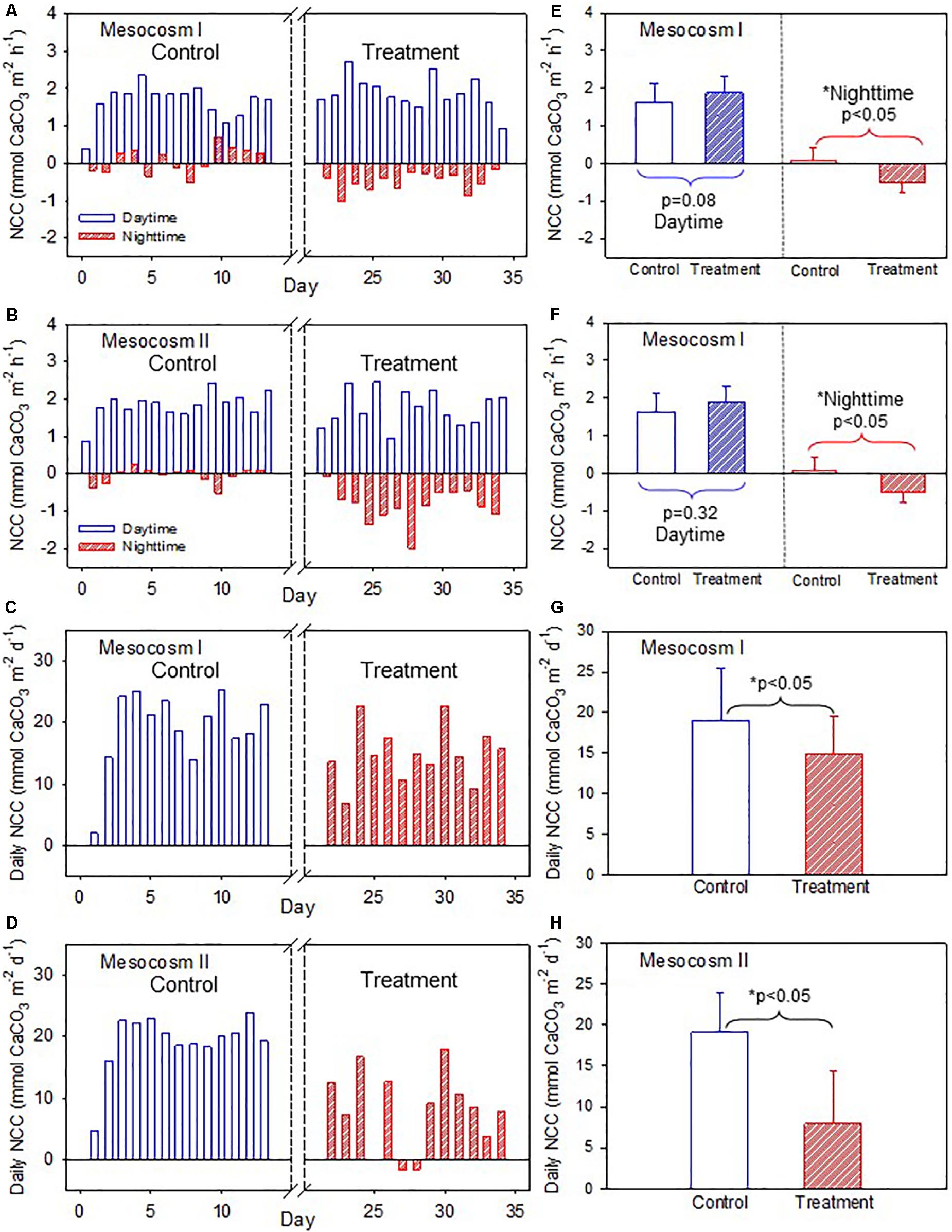
Figure 3. (A,B) Temporal variations of daytime and nighttime net community calcification (NCC), and (C,D) daily NCC in mesocosms I and II during the control and treatment periods. (E,F) Comparisons of average daytime and nighttime NCC, and (G,H) average daily NCC in mesocosms I and II between the control and treatment periods by the Student’s t-test. The asterisks indicate that the difference is statistically significant (p-value < 0.05).
The temporal variations of daytime NCP and nighttime R in the two mesocosms are shown in Figures 4A,B. Daytime NCP was generally higher than nighttime R during both periods. In contrast to the deviating responses of the daytime and nighttime NCC, the daytime NCP and nighttime R revealed similar responses to OA treatment: both the daytime NCP and nighttime R increased significantly between the control and treatment periods in the two mesocosms (p < 0.05; Figures 4C,D).
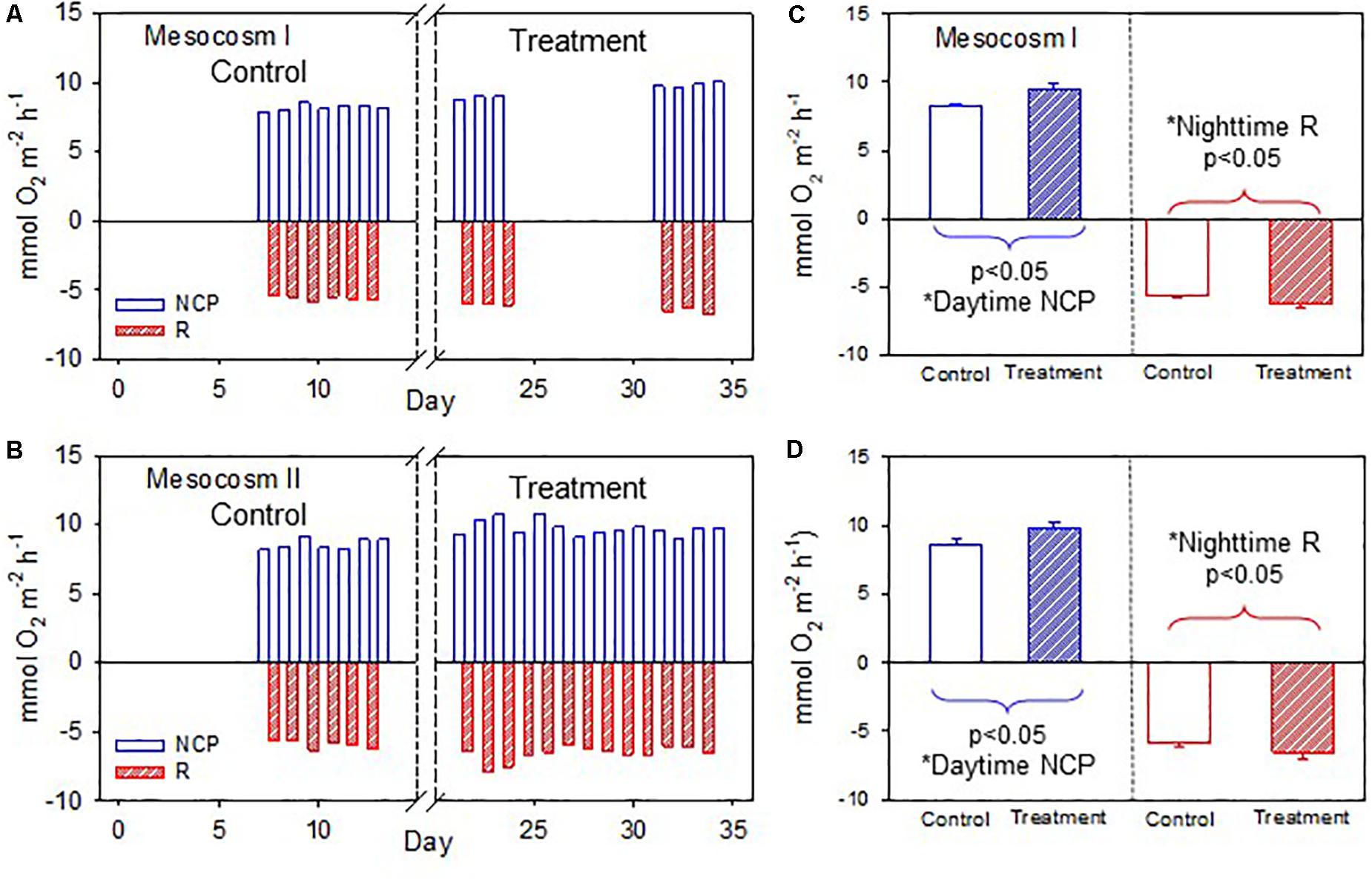
Figure 4. (A,B) Temporal variations of daytime net community production (NCP) and nighttime respiration (R) in mesocosms I and II during the control and treatment periods. (C,D) Comparisons of average daytime NCP and nighttime R in mesocosms I and II between the control and treatment periods by the Student’s t-test. The asterisks indicate that the difference is statistically significant (p-value < 0.05).
Discussion
Most previous studies investigating the effect of OA on coral reef ecosystems have shown a consistent decrease in NCC. However, in this study, we found that the daytime NCC did not follow this expected trend. As shown in Figure 5A, although Ωa during the treatment period was significantly lower than during the control period by approximately 0.8, the daytime NCC did not show a significant difference between the two periods in the both mesocosms. This result implies that a reduction in seawater Ωa may not necessarily lead to a decrease in calcification provided that calcification is the dominant process that determines the daytime NCC. In fact, several recent studies have indicated that corals can actively control the carbonate chemistry at the site of calcification (Venn et al., 2011; McCulloch et al., 2012) and have described species-specific responses of some corals and calcifying algae that are resistant to OA (Comeau et al., 2013; Takahashi and Kurihara, 2013). Additionally, there is mounting evidence across organisms and ecosystems demonstrating that biologically mediated calcification in an acidifying ocean is not controlled by the bulk seawater Ωa (Cyronak et al., 2013). Alternatively, light availability, temperature, and NCP have been proposed to be more important in driving future changes in calcification rates (McNeil et al., 2004; Venti et al., 2014; Albright et al., 2015). In this study, the light availability and temperature were kept at constant levels, and the daytime NCP shows a significant increase between the control and treatment periods; therefore, the observed insignificant response of the daytime NCC to OA treatment also could be explained as a result of that the negative effect of Ωa decrease has been partially compensated by the positive effect of NCP increase on NCC. This result also suggests that more studies are needed to elucidate the potential feedbacks between NCC and NCP in response to OA.
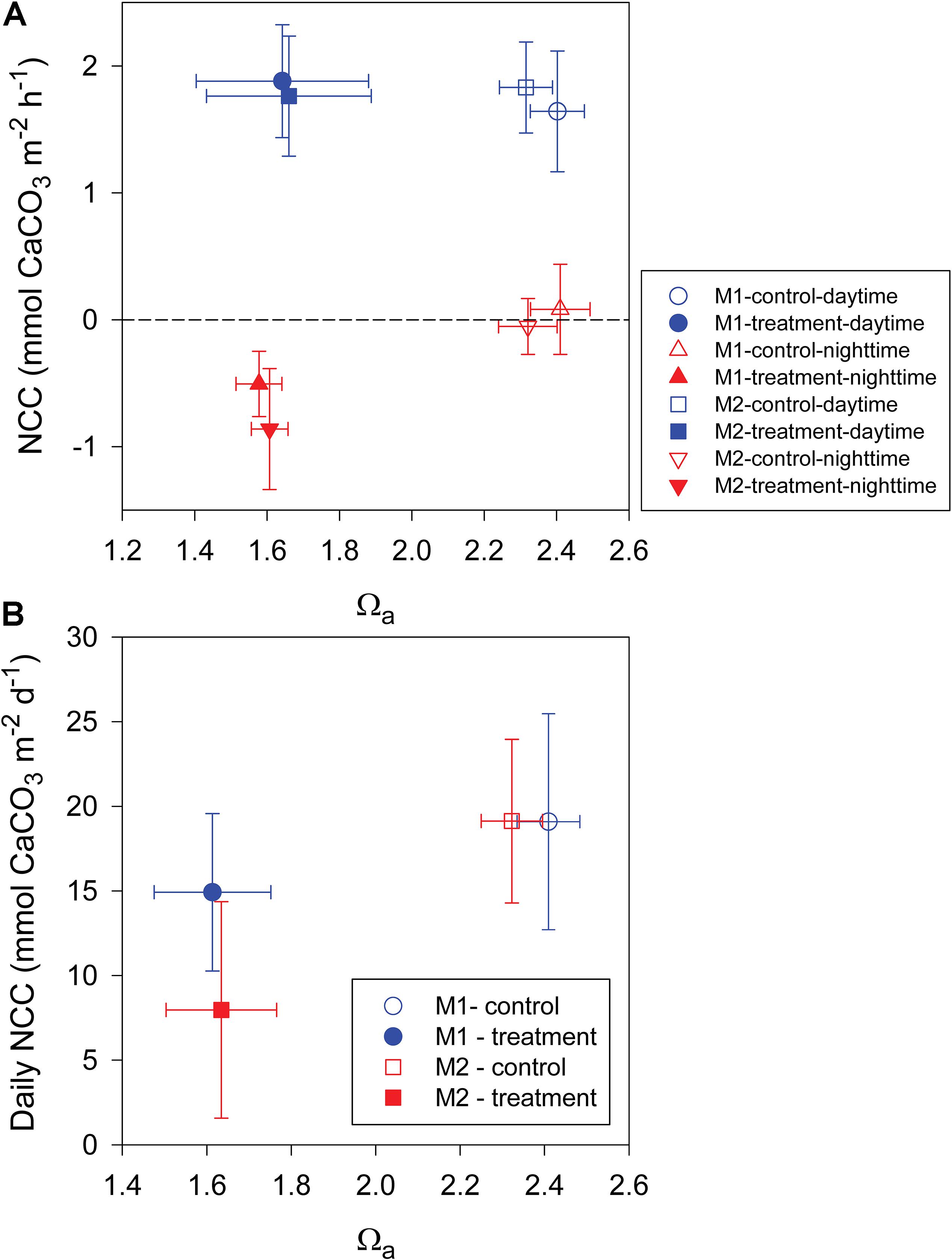
Figure 5. (A) Average daytime and nighttime net community calcification (NCC) and (B) average daily NCC versus seawater aragonite saturation state (Ωa) in mesocosms I (M1) and II (M2) during the control and treatment periods.
In contrast to the unchanged daytime NCC, the average nighttime NCC decreased dramatically between the control and treatment periods. Provided that CaCO3 dissolution tends to occur at night, the observed decrease in nighttime NCC during the OA period thus more likely resulted from increased CaCO3 dissolution than from decreased calcification. Previous studies have indicated that CaCO3 dissolution is an ongoing process in all coral reef environments due to the metabolic activity of microbes (Tribollet et al., 2009; Andersson and Gledhill, 2013), despite the fact that seawater is supersaturated with respect to the general group of carbonate mineral phases (i.e., aragonite, Mg-calcite and calcite). Microbial metabolic activity can produce CO2 and thus decrease the carbonate saturation state in microenvironments and sediment pore waters, thereby creating corrosive conditions with respect to carbonate minerals that subsequently could undergo dissolution (Andersson, 2015). Accordingly, a decrease in the seawater saturation state will result in increasingly corrosive conditions with respect to carbonate mineral phases and subsequently increasing rates of dissolution. In fact, the nighttime respiration in the present study shows a significant increase between the control and treatment periods, which can further enhance the reduction of Ωa during nighttime of the treatment period. Therefore, the observed significant decrease in nighttime NCC during the OA period supports the notion that OA may accelerate CaCO3 dissolution in coral reef environments. Moreover, several studies have hypothesized that CaCO3 dissolution may be more sensitive to OA than coral calcification (Andersson et al., 2009; Eyre et al., 2014, 2018). For example, a recent in situ study at Heron Island, Australia, reported that CaCO3 sediment dissolution increased by an order of magnitude more than the decrease in calcification per unit decrease in Ωa (Cyronak and Eyre, 2016). Our finding that the daytime NCC remained unchanged but the nighttime NCC decreased significantly in response to OA treatment thus adds to the growing evidence of the sensitivity of CaCO3 dissolution to OA.
As a combined result of the unchanged daytime NCC and the decreased nighttime NCC, the integrated daily NCC also decreased significantly between the control and treatment periods. This finding is also supported by the observed divergent variation trends in NTA and NDIC between the control and treatment periods (Figures 2A,B). During the control period, the higher daily NCC could consume more TA and DIC, which could be larger than TA and DIC supply from the inflowing water, and thus TA and DIC would decrease over time. In contrast, during the treatment period, the reduced NCC could consume less TA and DIC, which could be close to TA and DIC supply from the inflowing water, and thus the similar decreasing trend could not be found anymore.
Based on the present data set, the sensitivity of the daily NCC to changes in seawater Ωa was estimated to be a reduction in seawater Ωa by 1 unit resulting in a 26% and 81% decrease in NCC for mesocosms I and II, respectively (Figure 5B). The average value of this study (54%) is generally consistent with the global average of 60% reported by Eyre et al. (2018). However, it is worth noting that the sensitivity of NCC to OA has been found to be significantly lower than that of CaCO3 sediment dissolution but higher than biologically mediated coral calcification (Eyre et al., 2018). For example, a recent in situ study across five globally distributed reef sites showed that CaCO3 sediment is very sensitive to OA, with a 170% change per unit change in seawater Ωa, whereas a recent meta-analysis of biologically mediated coral calcification only showed a 15% reduction per unit change in seawater Ωa (Chan and Connolly, 2013). The sensitivity of the nighttime NCC in this study was 140 and 132% change per unit change in seawater Ωa for mesocosms I and II, respectively, which is slightly lower than the sensitivity of 170% for sediment dissolution reported by Eyre et al. (2018), but it is much higher than that of daily NCC found in this study. Thus, our observation that the nighttime NCC responded more strongly than the daily NCC to OA treatment is consistent with these recent findings. These results demonstrate again that increased CaCO3 dissolution could contribute more than decreased biological calcification to the observed decline in NCC under OA conditions. The different sensitivities of biological calcification and CaCO3 sediment dissolution to OA have been suggested to be associated with organisms that are able to upregulate pH at the site of calcification (Venn et al., 2011; McCulloch et al., 2012) and thus may have the potential to adapt to the effects of OA, whereas CaCO3 dissolution is mostly a geochemical response to changes in seawater chemistry and thus will increase according to thermodynamic and kinetic constraints (Eyre et al., 2018).
In summary, our results reveal contrasting responses of daytime (unchanged) and nighttime (decreased) NCC to OA, suggesting that carbonate dissolution can be more sensitive to OA than biological calcification. Adding on the mounting evidences, the present study supports the prevailing expectation that OA would lead to a reduction in the overall chemical accretion of coral reef ecosystems. However, increased CaCO3 dissolution in response to OA may pose a more serious threat to this reduction than decreased coral calcification. Therefore, although recent studies have found that calcification by some corals may be relatively tolerant to OA, the persistence of coral reefs is still at risk due to enhanced CaCO3 dissolution. Finally, it is worth noting that the present work and other earlier studies on this topic were conducted only during short-term experiments (1 day to weeks), more long-term OA experiments (several months to years) are still needed to rule out the potential short-term responses to treatment and to assess whether coral reef organisms can acclimatize to changes in seawater carbonate chemistry (Comeau et al., 2019).
Data Availability Statement
The raw data supporting the conclusions of this article will be made available by the authors, without undue reservation, to any qualified researcher.
Author Contributions
W-CC and P-JL designed the experiments and wrote the manuscript. Y-HC performed the experiments and participated in discussions about the manuscript. W-JH participated in discussions and revised the manuscript. All authors reviewed the manuscript.
Funding
Funding was provided by the Ministry of Science and Technology of Taiwan grants #107-2611-M-019-001-MY3 awarded to W-CC and #107-2611-M-259-001 awarded to P-JL.
Conflict of Interest
The authors declare that the research was conducted in the absence of any commercial or financial relationships that could be construed as a potential conflict of interest.
Acknowledgments
We thank Rong-Wei Syu, Hui-Chuan Chu, and Kuan-Chieh Wu for help with laboratory work.
References
Albright, R., Benthuysen, J., Cantin, N., Caldeira, K., and Anthony, K. (2015). Coral reef metabolism and carbon chemistry dynamics of a coral reef flat. Geophys. Res. Lett. 42, 3980–3988. doi: 10.1002/2015GL063488
Albright, R., Caldeira, L., Hosfelt, J., Kwiatkowski, L., Maclaren, J. K., Mason, B. M., et al. (2016). Reversal of ocean acidification enhances net coral reef calcification. Nature 531, 362–365. doi: 10.1038/nature17155
Albright, R., Takeshita, Y., Koweek, D. A., Ninokawa, A., Wolfe, K., Rivlin, T., et al. (2018). Carbon dioxide addition to coral reef waters suppresses net community calcification. Nature 555, 516–519. doi: 10.1038/nature25968
Andersson, A. J. (2015). A fundamental paradigm for coral reef carbonate sediment dissolution. Front. Mar. Sci. 2:52. doi: 10.3389/fmars.2015.00052
Andersson, A. J., and Gledhill, D. (2013). Ocean acidification and coral reefs: effects on breakdown, dissolution, and net ecosystem calcification. Ann. Rev. Mar. Sci. 5, 321–348. doi: 10.1146/annurev-marine-121211-172241
Andersson, A. J., Kuffner, I. B., Mackenzie, F. T., Jokiel, P. L., Rodgers, K. S., and Tan, A. (2009). Net loss of CaCO3 from a subtropical calcifying community due to seawater acidification: mesocosm-scale experimental evidence. Biogeosciences 6, 1811–1823. doi: 10.5194/bg-6-1811-2009
Andersson, A. J., and Mackenzie, F. T. (2012). Revisiting four scientific debates in ocean acidification research. Biogeosciences 9, 893–905. doi: 10.5194/bg-9-893-2012
Caldeira, K., and Wickett, M. E. (2005). Ocean model predictions of chemistry changes from carbon dioxide emissions to the atmosphere and ocean. J. Geophys. Res. 110:C09S04. doi: 10.1029/2004JC002671
Chan, N. C. S., and Connolly, S. R. (2013). Sensitivity of coral calcification to ocean acidification: a meta-analysis. Glob. Chang. Biol. 19, 282–290. doi: 10.1111/gcb.12011
Chou, W.-C., Chu, H.-C., Chen, Y.-H., Syu, R.-W., Hung, C.-C., and Soong, K. (2018). Short-term variability of carbon chemistry in two contrasting seagrass meadows at Dongsha Island: implications for pH buffering and CO2 sequestration. Estuar. Coast. Mar. Sci. 210, 36–44. doi: 10.1016/j.ecss.2018.06.006
Chou, W.-C., Gong, G.-C., Yang, C.-Y., and Chuang, K.-Y. (2016). A comparison between field and laboratory pH measurements for seawater on the East China Sea shelf. Limnol. Oceanogr. Methods 14, 315–322. doi: 10.1002/lom3.10091
Clayton, T. D., and Byrne, R. H. (1993). Spectrophotometric seawater pH measurements: total hydrogen ion concentration scale calibration of m-cresol purple and at-sea results. Deep Sea Res. Part I Oceanogr. Res. Pap. 40, 2115–2129. doi: 10.1016/0967-0637(93)90048-90048
Cohen, I., Dubinsky, Z., and Erez, J. (2016). Light enhanced calcification in hermatypic corals: new insights from light spectral responses. Front. Mar. Sci. 2:122. doi: 10.3389/fmars.2015.00122
Comeau, S., Cornwall, C. E., DeCarlo, T. M., Doo, S. S., Carpenter, R. C., and McCulloch, M. T. (2019). Resistance to ocean acidification in coral reef taxa is not gained by acclimatization. Nat. Clim. Chang. 9, 477–483. doi: 10.1038/s41558-019-0486-489
Comeau, S., Edmunds, P. J., Spindel, N. B., and Carpenter, R. C. (2013). The responses of eight coral reef calcifiers to increasing partial pressure of CO2 do not exhibit a tipping point. Limnol. Oceanogr. 58, 388–398. doi: 10.4319/lo.2013.58.1.0388
Comeau, S., Edmunds, P. J., Spindel, N. B., and Carpenter, R. C. (2014). Fast coral reef calcifiers are more sensitive to ocean acidification in short-term laboratory incubations. Limnol. Oceanogr. 59, 1081–1091. doi: 10.4319/lo.2014.59.3.1081
Cyronak, T., and Eyre, B. D. (2016). The synergistic effects of ocean acidification and organic metabolism on calcium carbonate (CaCO3) dissolution in coral reef sediments. Mar. Chem. 183, 1–12. doi: 10.1016/j.marchem.2016.05.001
Cyronak, T., Santos, I. R., and Eyre, B. D. (2013). Permeable coral reef sediment dissolution driven by elevated p CO 2 and pore water advection. Geophys. Res. Lett. 40, 4876–4881. doi: 10.1002/grl.50948
Dickson, A. G., and Millero, F. J. (1987). A comparison of the equilibrium constants for the dissociation of carbonic acid in seawater media. Deep Sea Res. Part A. Oceanogr. Res. Pap. 34, 1733–1743. doi: 10.1016/0198-0149(87)90021-90025
Doney, S. C., Fabry, V. J., Feely, R. A., and Kleypas, J. A. (2009). Ocean acidification: the other CO 2 problem. Ann. Rev. Mar. Sci. 1, 169–192. doi: 10.1146/annurev.marine.010908.163834
Eyre, B. D., Andersson, A. J., and Cyronak, T. (2014). Benthic coral reef calcium carbonate dissolution in an acidifying ocean. Nat. Clim. Chang. 4, 969–976. doi: 10.1038/NCLIMATE2380
Eyre, B. D., Cyronak, T., Drupp, P., DeCarlo, E. H., Sachs, J. P., and Andersson, A. J. (2018). Coral reefs will transition to net dissolving before end of century. Science 359, 908–911. doi: 10.1126/science.aao1118
Gattuso, J. P., Allemand, D., and Frankignoulle, M. (1999). Photosynthesis and calcification at cellular, organismal and community levels in coral reefs: a review on interactions and control by carbonate chemistry. Am. Zool. 39, 160–183. doi: 10.1093/icb/39.1.160
Gattuso, J. P., Frankignoulle, M., Bourge, I., Romaine, S., and Buddemeier, R. W. (1998). Effect of calcium carbonate saturation of seawater on coral calcification. Glob. Planet. Change 18, 37–46. doi: 10.1016/s0921-8181(98)00035-6
IPCC, (2001). Climate Change 2001: The Scientific Basis, Contribution of Working Group I to the Third Assessment Report of the Intergovernmental Panel on Climate Change, eds J. T. Houghton, Y. Ding, D. J. Griggs, M. Noguer, P. J. van der Linden, X. Dai, et al. (New York, NY: Cambridge University Press).
Jokiel, P. L. (2011). Ocean acidification and control of reef coral calcification by boundary layer limitation of proton flux. Bull. Mar. Sci. 87, 639–657. doi: 10.5343/bms.2010.1107
Kleypas, J. A., Buddemeier, R. W., Archer, D., Gattuso, J. P., Langdon, C., and Opdyke, B. N. (1999). Geochemical consequences of increased atmospheric carbon dioxide on coral reefs. Science 284, 118–120. doi: 10.1126/science.284.5411.118
Kleypas, J. A., and Yates, K. K. (2009). Coral reefs and ocean acidification. Oceanography 22, 108–117. doi: 10.5670/oceanog.2009.101
Langdon, C., Broecker, W. S., Hammond, D. E., Glenn, E., Fitzsimmons, K., Nelson, S. G., et al. (2003). Effect of elevated CO2 on the community metabolism of an experimental coral reef. Glob. Biogeochem. Cycles 17:1011. doi: 10.1029/2002GB001941
Langdon, C., Takahashi, T., Sweeney, C., Chipman, D., Goddard, J., Marubini, F., et al. (2000). Effect of calcium carbonate saturation state on the calcification rate of an experimental coral reef. Glob. Biogeochem. Cycles 14, 639–654. doi: 10.1029/1999GB001195
Le Quere, C., Andrew, R. M., Canadell, J. G., Sitch, S., Korsbakken, J. I., Peters, G. P., et al. (2016). Global carbon budget 2016. Earth Syst. Sci. Data 8, 605–649. doi: 10.5194/essd-8-605-2016
Leclercq, N., Gattuso, J.-P., and Jaubert, J. (2002). Primary production, respiration, and calcification of a coral reef mesocosm under increased CO2 partial pressure. Limnol. Oceanogr. 47, 558–564. doi: 10.4319/lo.2002.47.2.0558
Liu, P.-J., Lin, S.-M., Fan, T.-Y., Meng, P.-J., Shao, K.-T., and Lina, H.-J. (2009). Rates of overgrowth by macroalgae and attack by sea anemones are greater for live coral than dead coral under conditions of nutrient enrichment. Limnol. Oceanogr. 54, 1167–1175. doi: 10.4319/lo.2009.54.4.1167
McCulloch, M., Falter, J., Trotter, J., and Montagna, P. (2012). Coral resilience to ocean acidification and global warming through pH up-regulation. Nat. Clim. Chang. 2, 623–627. doi: 10.1038/nclimate1473
McNeil, B. I., Matear, R. J., and Barnes, D. J. (2004). Coral reef calcification and climate change: the effect of ocean warming. Geophys. Res. Lett. 31:L22309. doi: 10.1029/2004GL021541
Mehrbach, C., Culberson, C. H., Hawley, J. E., and Pytkowicx, R. M. (1973). Measurement of the apparent dissociation constants of carbonic acid in seawater at atmospheric pressure. Limnol. Oceanogr. 18, 897–907. doi: 10.4319/lo.1973.18.6.0897
Mucci, A. (1983). The solubility of calcite and aragonite in seawater at various salinities, temperatures, and one atmosphere total pressure. Am. J. Sci. 283, 780–799. doi: 10.2475/ajs.283.7.780
Pandolfi, J. M., Connolly, S. R., Marshall, D. J., and Cohen, A. L. (2011). Projecting coral reef futures under global warming and ocean acidification. Science 333, 418–422. doi: 10.1126/science.1204794
Pierrot, D., Lewis, E., and Wallace, D. W. R. (2006). MS Excel Program Developed for CO2 System Calculations. ORNL/CDIAC-105a. Oak Ridge: U.S. Department of Energy, doi: 10.3334/CDIAC/otg.CO2SYS_XLS_CDIAC105a
Ries, J. B. (2011). A physicochemical framework for interpreting the biological calcification response to CO2-induced ocean acidification. Geochim. Cosmochim. Acta 75, 4053–4064. doi: 10.1016/j.gca.2011.04.025
Riley, J. P., and Tongudai, M. (1967). The major cation/chlorinity ratios in sea water. Chem. Geol. 2, 263–269. doi: 10.1016/0009-2541(67)90026-90025
Schneider, K., Levy, O., Dubinsky, Z., and Erez, J. (2009). In situ diel cycles of photosynthesis and calcification in hermatypic corals. Limnol. Oceanogr. 54, 1995–2002. doi: 10.4319/lo.2009.54.6.1995
Shamberger, K. E. F., Feely, R. A., Sabine, C. L., Atkinson, M. J., DeCarlo, E. H., Mackenzie, F. T., et al. (2011). Calcification and organic production on a Hawaiian coral reef. Mar. Chem. 127, 64–75. doi: 10.1016/j.marchem.2011.08.003
Shaw, E. C., Phinn, S. R., Tilbrook, B., and Steven, A. (2015). Natural in situ relationships suggest coral reef calcium carbonate production will decline with ocean acidification. Limnol. Oceanogr. 60, 777–788. doi: 10.1002/lno.10048
Silverman, J., Lazar, B., Cao, L., Caldeira, K., and Erez, J. (2009). Coral reefs may start dissolving when atmospheric CO 2 doubles. Geophys. Res. Lett. 36:L05606. doi: 10.1029/2008GL036282
Takahashi, A., and Kurihara, H. (2013). Ocean acidification does not affect the physiology of the tropical coral Acropora digitifera during a 5-week experiment. Coral Reefs 32, 305–314. doi: 10.1007/s00338-012-0979-978
Tribollet, A., Godinot, C., Atkinson, M., and Langdon, C. (2009). Effects of elevated pCO2 on dissolution of coral carbonates by microbial euendoliths. Glob. Biogeochem. Cycles 23:GB3008. doi: 10.1029/2008GB003286
van Hooidonk, R., Maynard, J. A., Manzello, D., and Planes, S. (2014). Opposite latitudinal gradients in projected ocean acidification and bleaching impacts on coral reefs. Glob. Chang. Biol. 20, 103–112. doi: 10.1111/gcb.12394
Venn, A., Tambutté, E., Holcomb, M., Allemand, D., and Tambutté, S. (2011). Live tissue imaging shows reef corals elevate ph under their calcifying tissue relative to seawater. PLoS One 6:e20013. doi: 10.1371/journal.pone.0020013
Venti, A., Andersson, A., and Langdon, C. (2014). Multiple driving factors explain spatial and temporal variability in coral calcification rates on the Bermuda platform. Coral Reefs 33, 979–997. doi: 10.1007/s00338-014-1191-1199
Keywords: ocean acidification, coral reef, calcification, carbonate dissolution, diel variation, mesocosm
Citation: Chou W-C, Liu P-J, Chen Y-H and Huang W-J (2020) Contrasting Changes in Diel Variations of Net Community Calcification Support That Carbonate Dissolution Can Be More Sensitive to Ocean Acidification Than Coral Calcification. Front. Mar. Sci. 7:3. doi: 10.3389/fmars.2020.00003
Received: 28 August 2019; Accepted: 07 January 2020;
Published: 23 January 2020.
Edited by:
Jianfang Chen, Second Institute of Oceanography, Ministry of Natural Resources, ChinaReviewed by:
Tyler Cyronak, Nova Southeastern University, United StatesChenhua Han, The Second Institute of Oceanography, MENR, China
Copyright © 2020 Chou, Liu, Chen and Huang. This is an open-access article distributed under the terms of the Creative Commons Attribution License (CC BY). The use, distribution or reproduction in other forums is permitted, provided the original author(s) and the copyright owner(s) are credited and that the original publication in this journal is cited, in accordance with accepted academic practice. No use, distribution or reproduction is permitted which does not comply with these terms.
*Correspondence: Wen-Chen Chou, d2NjaG91QG1haWwubnRvdS5lZHUudHc=