- 1Halmos College of Natural Sciences and Oceanography, Nova Southeastern University, Dania Beach, FL, United States
- 2South Carolina Department of Natural Resources, Marine Resources Research Institute, Charleston, SC, United States
The most abundant fishes on Earth live in the meso- and bathypelagic (deep-pelagic, collectively) zones of the open ocean, where they play a key role in deep-sea food webs by mediating energy flow from surface waters to great depth. Of these fishes, the most speciose taxon is the family Stomiidae (dragonfishes). Despite being the numerically dominant predators of the global mesopelagic zone, stomiid reproductive ecology is poorly known. Research surveys rarely catch larger adults, impeding reproductive ecology studies. Between 2010 and 2011, the Offshore Nekton Sampling and Analysis Program sampled the Gulf of Mexico using a research-sized, opening/closing trawl (10-m2 MOCNESS) and a commercial-sized, high-speed rope trawl (HSRT). Size-distribution analysis by gear type revealed: the HSRT caught more specimens per species, and the HSRT caught significantly larger specimens, whereas the MOCNESS sampled more juveniles. Gonads were dissected from 714 individuals representing 47 species, and the 12 dominant species were analyzed in further detail. Gonadal histology assessment indicated that stomiids are gonochoristic and exhibit asynchronous oocyte development and batch spawning. A total of 11 of the 12 species had sex ratios that did not significantly differ from a 1:1 (male:female) ratio (P < 0.05). Histological analysis indicated that females mature at larger sizes than males. Given the lack of age and growth data for this family, these data are critical for estimating stomiid production rates, a key element for quantifying the role of stomiids in the transfer of organic matter within the deep-pelagic zone, the planet's largest cumulative ecosystem.
Introduction
Mesopelagic (200–1,000 m depth) fishes comprise the majority of Earth's fish biomass (Irigoien et al., 2014; Nelson et al., 2016). A study by Gjøsaeter and Kawaguchi (1980) used trawl catch data to estimate the global aggregate biomass of mesopelagic fishes to be 1 × 109 tons. However, the authors also indicated that this number was likely an underestimate due to trawl avoidance (most research-sized nets are relatively small compared to commercial nets) and extrusion (specimens passing through the meshes of larger, commercial-sized trawls) (Kashkin and Parin, 1983). More recent studies based on modeling and acoustic data (Koslow et al., 1997; Kaartvedt et al., 2012) suggest the global biomass to be significantly higher than earlier estimates; for example, the biomass of mesopelagic fishes between 40°N and 40°S alone is estimated to be at least an order of magnitude higher, between 7 and 10 billion metric tons (Koslow et al., 1997; Kaartvedt et al., 2012; Irigoien et al., 2014). Given their high abundance and biomass, mesopelagic fishes likely play a significant role in global, deep-pelagic food webs.
The most speciose family of mesopelagic fishes (305 valid species; Fricke et al., 2018a) is the Stomiidae (sensu Fink, 1985), collectively including the snaggletooths (Astronesthinae), viperfishes (Chauliodontinae), black dragonfishes (Idiacanthinae), loosejaws (Malacosteinae), scaleless black dragonfishes (Melanostomiinae), and scaly dragonfishes (Stomiinae). These predatory fishes inhabit all oceans, including the Southern Ocean (Gibbs, 1969; Fink, 1985; Kenaley, 2007), and while they predominantly occupy the mesopelagic zone, evidence indicates that some species thrive in the deeper waters of the bathypelagic (1,000–4,000 m depth) zone (Gibbs, 1969; Childress et al., 1980). Ecologically, stomiids play an important role in deep-sea ecosystems because they are trophic mediators and link surface waters to those of the deep-pelagic. Most stomiids are vertical migrators and migrate to the epipelagic (0–200 m depth) zone at night to feed on the heightened influx of lanternfishes (Myctophidae), the primary fish zooplanktivores in most oceanic food webs (Clarke, 1974; Hopkins and Gartner, 1992). By bringing carbon fixed in the surface waters to their deeper daytime depth ranges, stomiids regulate deep-sea energy flow and play a vital role in the interzonal transfer of energy between the epipelagic, mesopelagic, and bathypelagic zones (Sutton and Hopkins, 1996a).
Quantifying carbon flow through mesopelagic systems requires an estimate of fish biomass and production, which in turn requires data on reproduction. Although aspects of stomiid feeding ecology have been quantified (Clarke, 1982; Sutton and Hopkins, 1996a; Butler et al., 2001; Kenaley, 2012), the data gap regarding their reproductive ecology remains, a consequence of insufficient sample sizes of adults due to the type of gear commonly used to collect specimens. Larger, sexually mature stomiids are likely more adept at net avoidance than smaller juveniles (Clarke, 1974; Fisher and Pearcy, 1983; Sutton and Hopkins, 1996b), thereby impeding synoptic studies of reproductive biology. However, hermaphroditism is known to occur in Gonostomatidae, a sister taxon in the Stomiiformes. Reproductive information is a key component of bioenergetics modeling, which takes into account the productivity of species and is frequently applied to several areas of study such as predator-prey interactions and ecosystem modeling (Hansen et al., 1993). Each of these areas requires knowledge about the reproduction of its component species. In this paper, we utilize one of the largest sets of deep-pelagic samples, one that utilized two complementary gear types to sample both juveniles and large adults. By using samples obtained with a Multiple Opening/Closing Net and Environmental Sensing System (MOCNESS) midwater trawl, as well as a high-speed rope trawl (HSRT), we had an unprecedented opportunity to investigate stomiid reproductive ecology.
Anthropogenic events may significantly hinder the capacity for mesopelagic fishes to regulate energy flow in the deep sea because such events can create unfavorable conditions for growth and survival. For example, repurcussions of hydrocarbon toxicity on larval fishes due to catastrophes such as oil spills have been widely documented, including declines in productivity at the population level due to decreases in reproductive health (Brown et al., 1996; Short, 2003). The Gulf of Mexico is particularly relevant in this regard given the location, size, and duration of the Deepwater Horizon oil spill (DWHOS), which lasted from April–September 2010. In order to understand the impact of the DWHOS on deep-pelagic fishes and expand our knowledge base of important predators, the focus of this study was to quantify reproductive parameters and investigate reproductive life history characteristics of stomiids in the Gulf of Mexico. The following questions relating to stomiid reproductive ecology were investigated: (1) Are stomiids gonochoristic, like most teleosts, or hermaphroditic, like sister taxa in the order Stomiiformes? (2) What type of oocyte development and spawning pattern is exhibited by stomiids? (3) What are the sex ratios of stomiid species? and (4) What are the sizes at maturity for stomiids in the Gulf of Mexico? These results can be used to further investigations of the impact of anthropogenic events to deep-pelagic assemblages, as well as general studies on mesopelagic ecosystems and carbon flow.
Materials and Methods
Sample Collection
A series of seven cruises was conducted in the Gulf of Mexico between 2010 and 2011 as part of the DWHOS Offshore Nekton Sampling and Analysis Program. A total of 1,600 trawl samples were collected at stations arranged in a grid pattern across the northern gulf, most of which were seaward of the 1,000-m isobath (Figure 1). All sampling occurred both day and night from the surface to 1,500 m depth using two gear types in order to catch a broad assortment of individual sizes and species.
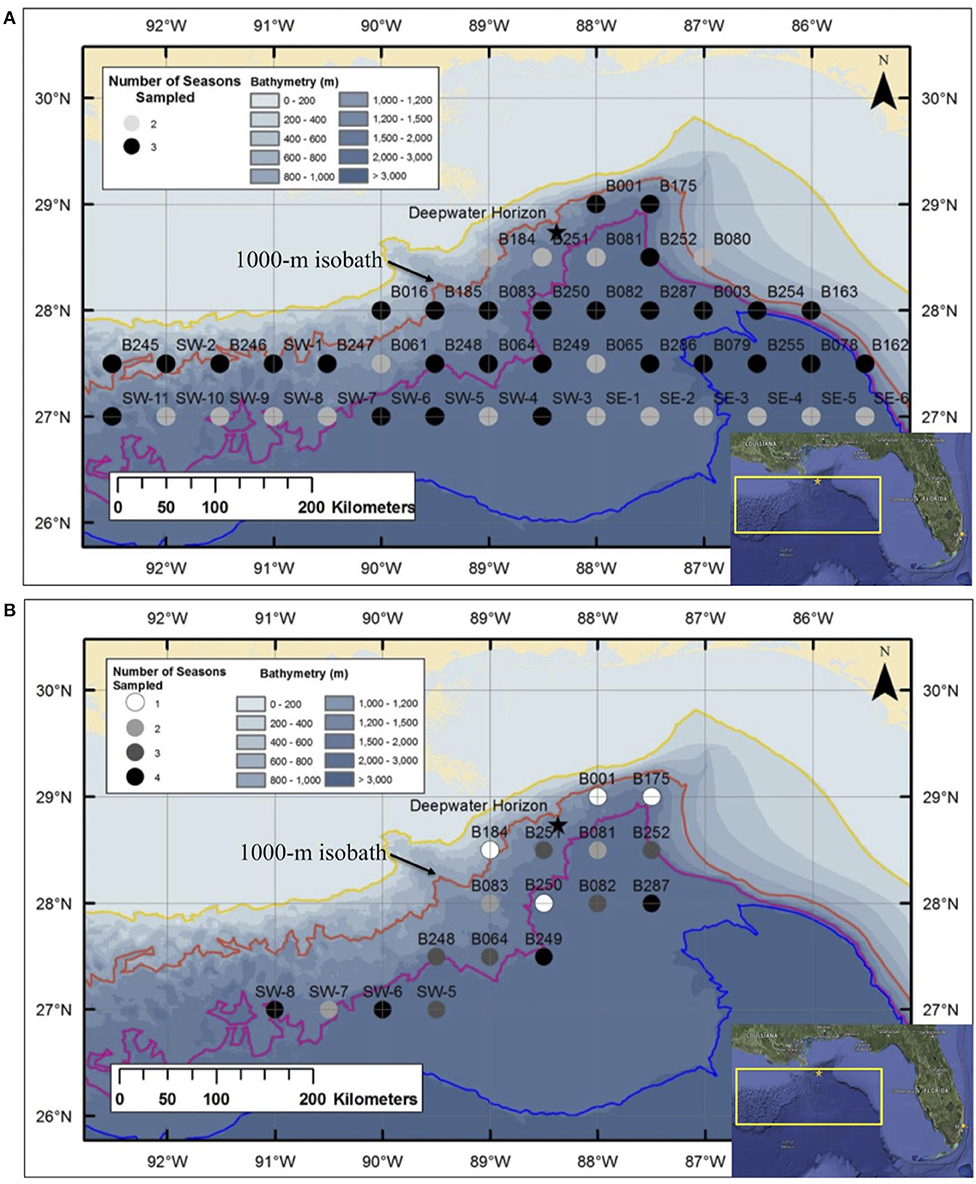
Figure 1. Deepwater stations sampled in the Gulf of Mexico during: (A) Meg Skansi 6, Meg Skansi 7, and Meg Skansi 8 cruises between January and September 2011; and (B) Pisces 8, Pisces 9, Pisces 10, and Pisces 12 cruises between December 2010 and September 2011. All adjacent sampling stations were equidistant from each other by 30 nautical miles (55.6 km). Isobaths, from top to bottom, indicate depths of 200, 1,000, 2,000, and 3,000 m, respectively. The 1,000-m isobath is individually labeled for reference. The black star marks the site of the Deepwater Horizon oil spill that occurred between April and September 2010.
During three cruise series, sampling was conducted with a six-net, 10-m2 mouth area MOCNESS midwater trawl (Wiebe et al., 1985), with each net having a uniform mesh size of 3 mm (stretched). Each deployment produced up to five discrete-depth, quantitative samples; the sixth net was an oblique tow from the surface to 1,500 m and catches in this net were not processed quantitatively. Sampling occurred in each month from January–September 2011, and a total of 46 stations were sampled either twice (17 stations) or three times (29 stations) (Figure 1A). Additional sampling details can be found in Burdett et al. (2017) and (Sutton et al., 2020). On four additional cruises, specimens were collected using a HSRT (Dotson and Griffith, 1996) with an effective mouth area of 165.47 m2 (Sutton & Mercier unpubl. data) and a graded mesh size (stretched) of 3.2 m at the mouth which tapered to 19 mm at the cod end. A total of 17 stations were sampled one to four times (Figure 1B), with each cruise lasting 3 weeks, between December 2010 and September 2011. Specimens used in this study were opportunistically selected from both gears to increase the size spectrum from juveniles to spawning-sized adults.
After capture and subsequent identification and data logging, all stomiid specimens were fixed in a 10% (v:v) formalin:seawater solution. Specimens were stored in formalin until sample processing began back in the laboratory and were never transferred to alcohol after fixation. Just before they were processed, specimens were transferred to water to de-gas under a fume hood. Specimens for this study were selected during sequential processing of trawl samples at the Nova Southeastern University Oceanic Ecology Laboratory. When practical, all specimens for each species collected on the seven cruises (Table 1) were used in this study. In cases of superfluous specimens, a subsample spanning the available size spectrum was chosen for the species.
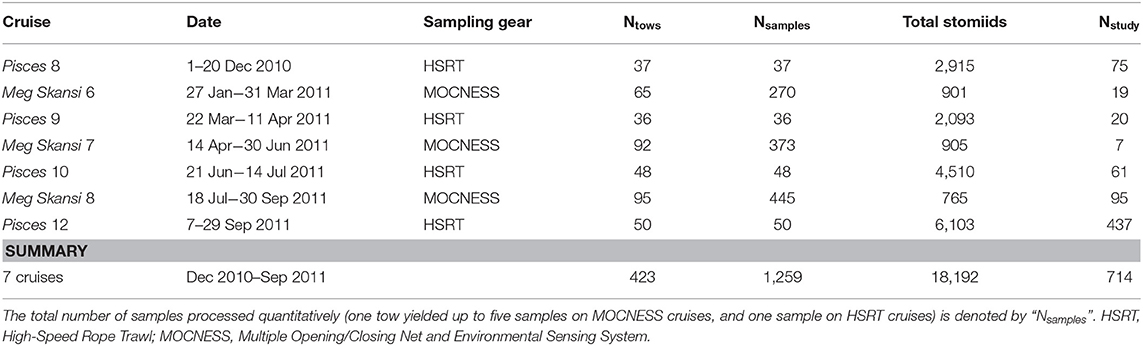
Table 1. Cruise details from the 2010 to 2011 expeditions in the Gulf of Mexico and the total number of stomiid specimens from each cruise used in this study (Nstudy).
Specimen Processing
Stomiid specimens were selected for reproductive analysis as they became available during a large-scale, quantitative taxonomic analysis program. Specimens were identified using the keys of Gibbs (1964a,b, 1969), Morrow (1964a,b,c), Morrow and Gibbs (1964), Barnett and Gibbs (1968), Goodyear and Gibbs (1969), Gibbs et al. (1983), Gomon and Gibbs (1985), Gibbs and McKinney (1988), Sutton and Hartel (2004), Kenaley and Hartel (2005), Kenaley (2007), and Flynn and Klepadlo (2012). Species were measured to the nearest 0.1 mm standard length (SL) and total wet weights were recorded to the nearest 0.01 g. Entire gonads were removed and the wet weight recorded to the nearest 0.001 g. All gonads were stored in 70% ethanol for at least 1 week prior to histological preparation.
Histology
Transverse subsections of 1–2 cm were removed from the middle of one lobe of the preserved gonads and dehydrated using an automated tissue processor before being embedded in paraffin. Gonadal tissue was cross-sectioned twice at 5 μm using a microtome, then mounted on glass microscope slides, rehydrated, stained with Harris's hematoxylin, and counterstained with eosin-y with phloxine following routine histological procedures (Avwioro, 2011).
Histological sections were examined with a compound microscope as an aid in determining sex, as well as one of five reproductive phases. Without reference to body length or date of capture, tissue sections were classified as “immature,” “developing,” “spawning capable,” “regressing,” or “regenerating,” following the criteria of Brown-Peterson et al. (2011). Based on key histological markers (type of spermatogenic cells present in males, type and size of oocytes in females), samples were classified as “mature” if they were in one of the four latter reproductive phases. Gonads that were underdeveloped and unable to be identified as male or female with certainty were classified as “undifferentiated.” Photomicrographs were taken of demonstrative samples to show key structures involved in the reproductive development of males (Figure 2) and females (Figure 3). Specimens (N = 41) in which the reproductive phase could not be determined unequivocally were not assigned a maturity phase and were excluded from analyses.
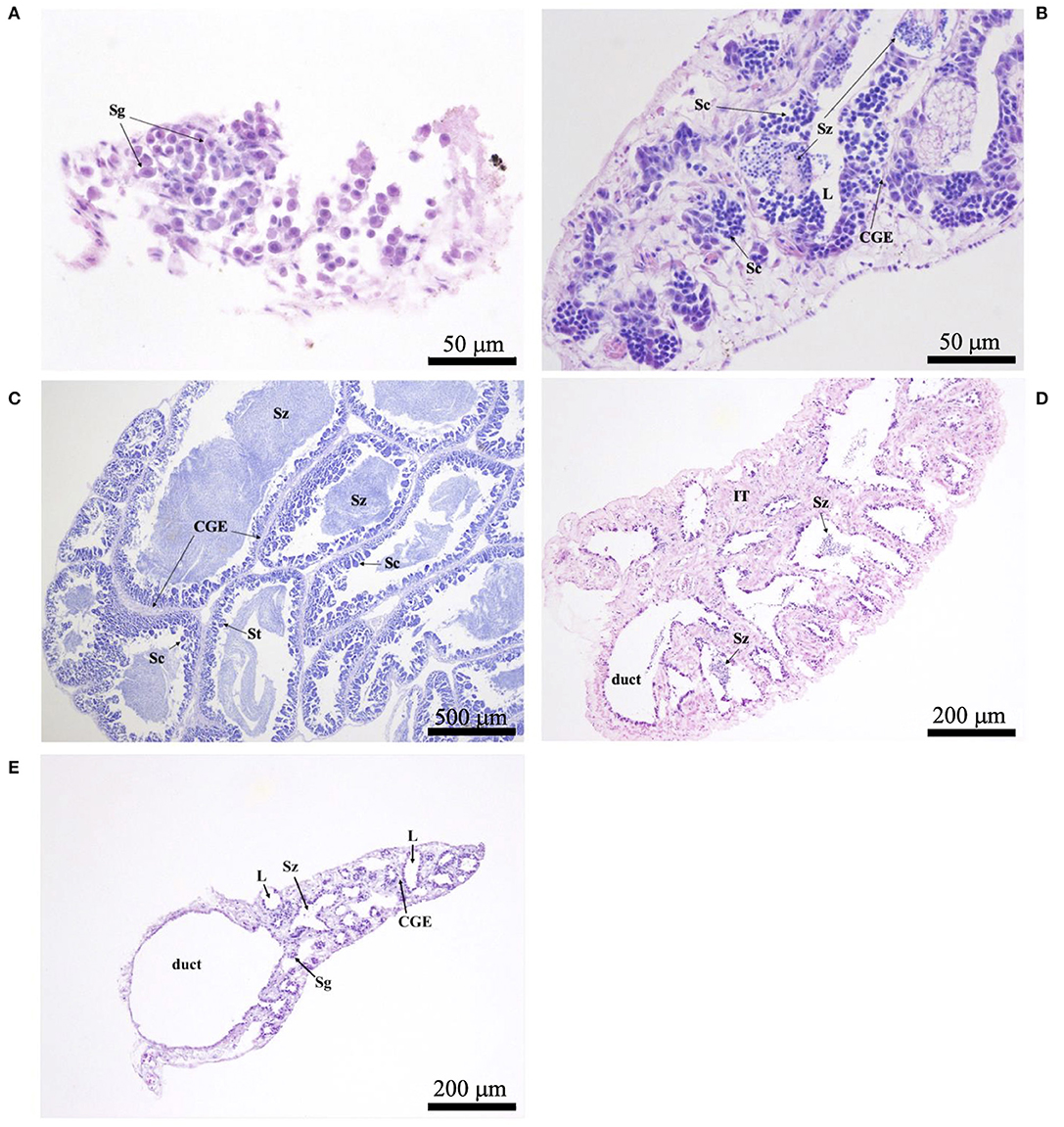
Figure 2. Photomicrographs of testicular histology, depicting: (A) Astronesthes atlanticus in the immature phase, with only spermatogonia (Sg) in the testes; scale bar = 50 μm; (B) Melanostomias melanops in the developing phase, with spermatocysts of spermatocytes (Sc), and cysts of spermatozoa (Sz) that have not yet entered the lobular lumina (L). Continuous germinal epithelium (CGE) present; scale bar = 50 μm; (C) peripheral tissue of Echiostoma barbatum in the spawning capable phase, with Sc, spermatids (St), Sz, and CGE; scale bar = 500 μm; (D) Melanostomias melanops in the regressing phase, with residual Sz in the lumina and duct; note the expanded interstitial (connective) tissue (IT); scale bar = 200 μm; (E) Echiostoma barbatum in the regenerating phase, with residual Sz in some lobules, empty lumina (L), CGE, and Sg proliferation; scale bar = 200 μm.
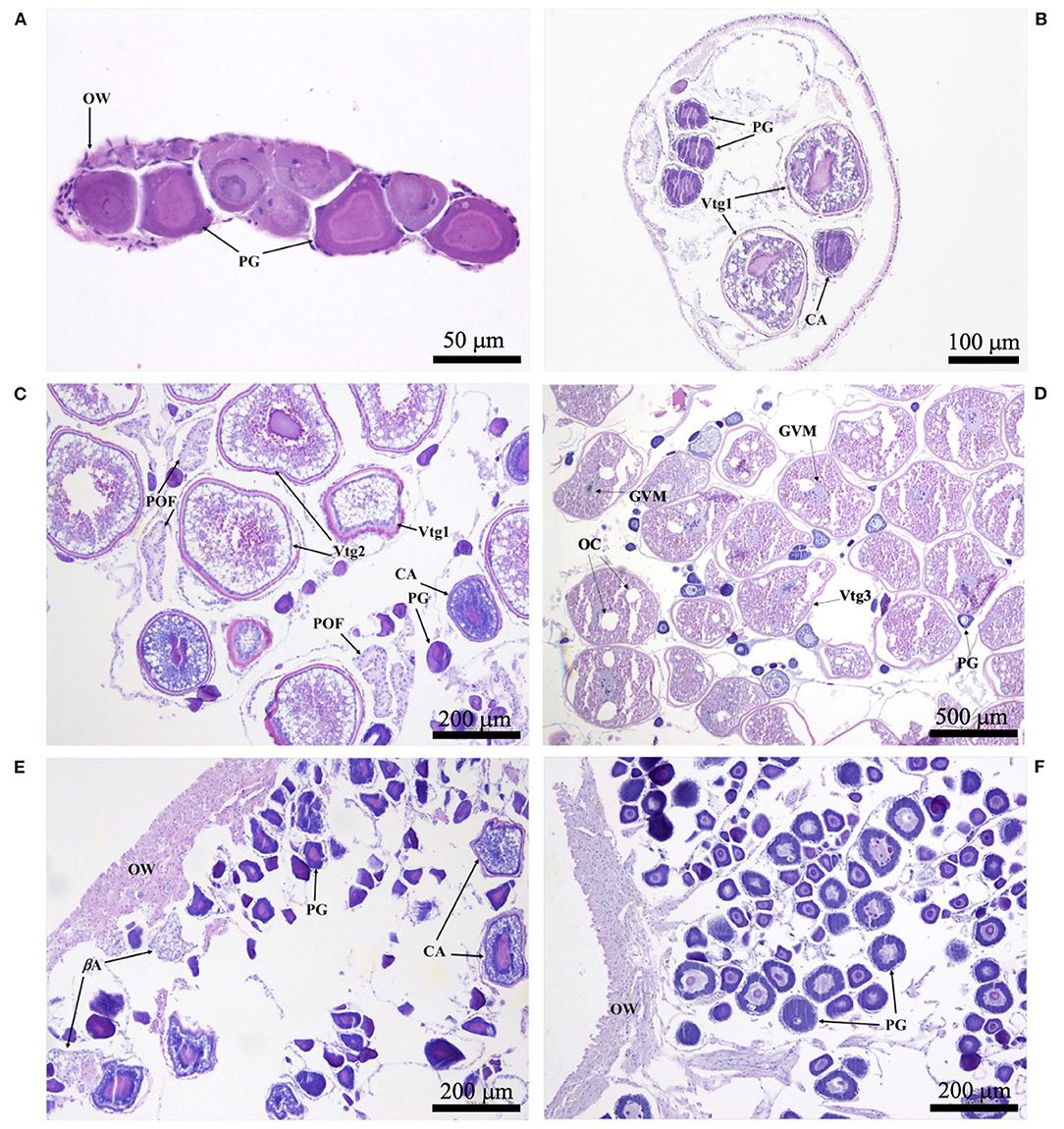
Figure 3. Photomicrographs of ovarian histology, depicting: (A) Photostomias guernei in the immature phase, with only primary growth oocytes (PG) and a thin ovarian wall (OW); scale bar = 50 μm; (B) Eustomias hypopsilus in the developing phase, with PG, cortical alveolar oocytes (CA), and primary vitellogenic oocytes (Vtg1); scale bar = 100 μm; (C) Aristostomias xenostoma in the spawning capable phase, with PG, CA, primary and secondary vitellogenic oocytes (Vtg1 & Vtg2), and postovulatory follicles (POF); scale bar = 200 μm; (D) Echiostoma barbatum in the actively spawning subphase undergoing oocyte maturation, with PG, tertiary vitellogenic oocytes (Vtg3), oil coalescence (OC), and germinal vesicle migration (GVM); scale bar = 500 μm; (E) Aristostomias xenostoma in the regressing phase, with beta atresia (βA), PG, and few CA; scale bar = 200 μm; (F) Eustomias schmidti in the regenerating phase, with larger PG and a thicker OW; scale bar = 200 μm.
Statistical Analysis
All statistical analysis was performed using R 0.98.1062 (R Development Core Team, 2013) for the 12 dominant (N ≥ 19 individuals) species, and results were considered significant at P < 0.05. Sex ratios were calculated using the total number of males to females, both immature and mature. Sex ratios were also calculated for only the mature males and females of each species. A Chi-Square Goodness of Fit Test was performed using the chisq.test() function to determine if the sex ratio of each species diverged from the expected ratio of 1:1 (male: female). All specimens identified as “undifferentiated” were incorporated only in size distribution analyses.
The size at 50% maturity (L50) was estimated using the “MASS” package (Venables and Ripley, 2002) glm() and dose.p() functions for binomial regression to show the percentage of mature specimens as a function of 10-mm size class. In cases where complete model separation occurred (i.e., each size class had only immature specimens, or only mature specimens), size at maturity was determined to be the smallest mature specimen. Size at maturity, based on histological results, was used in conjunction with size distributions by gear type to extrapolate what portion of all measured specimens of each species caught during the seven cruises was immature or mature. Additionally, a t-test was used to assess gear selectivity by comparing the mean sizes of specimens collected with the MOCNESS and HSRT.
Results
Sampling
In total, 714 specimens representing 47 stomiid species were examined, the majority of which (61%) were collected in the fall of 2011. Of the 714 specimens, 364 females, 318 males, and 32 undifferentiated specimens were identified. For the 12 dominant species, the size and weight compositions of female, male, and undifferentiated specimens sampled are shown in Table 2. Morphometric data for the less-abundant 35 species are presented in Supplementary Table 1.
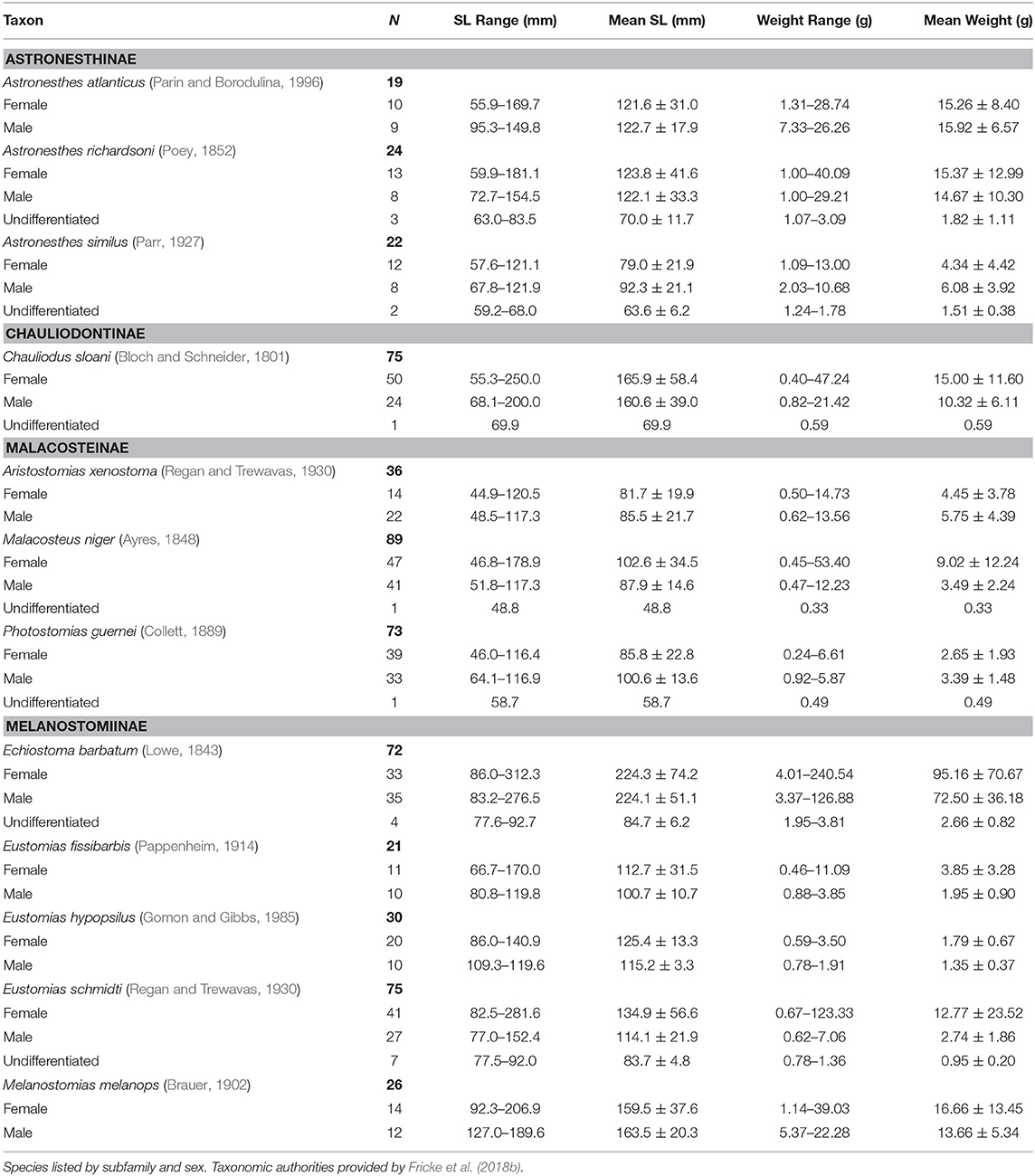
Table 2. Count, size range, mean standard length (SL), and standard deviation, range of wet weight, and mean wet weight and standard deviation of 12 dominant stomiid species collected in the Gulf of Mexico between 2010 and 2011.
Reproductive Strategy
No histological evidence of testicular and ovarian tissue was observed simultaneously in any stomiid gonad, indicating that stomiids are gonochoristic. Following the terminology of Grier and Uribe (2009), male stomiids possess an unrestricted, lobular testicular structure. Histological assessment of the testes also indicates that males are able to spawn continuously, as evidenced by the preponderance of spawning capable individuals (83%) and scarcity of individuals in the regressing and regenerating phases. In females, 25% of all specimens were spawning capable. Oocytes in all developmental stages, without dominant populations, were observed to be simultaneously present in the ovary, indicative of asynchronous oocyte development and batch spawning. Through this type of oocyte development, stomiids either spawn more than once during each spawning season, or continuously throughout the year with no discrete spawning season.
Sex Ratio
Chauliodus sloani was the only species in which the overall sex ratio (juveniles included) significantly differed from the expected 1:1 ratio; this species exhibited a sex ratio of 1:2.08, indicating a significant female bias (P = 0.003) (Table 3). When considering mature (adult) fish only, the observed sex ratio significantly favored males in the species Aristostomias xenostoma, Eustomias fissibarbis, Eustomias schmidti, and Malacosteus niger, presumably because males mature earlier than females (Table 3).
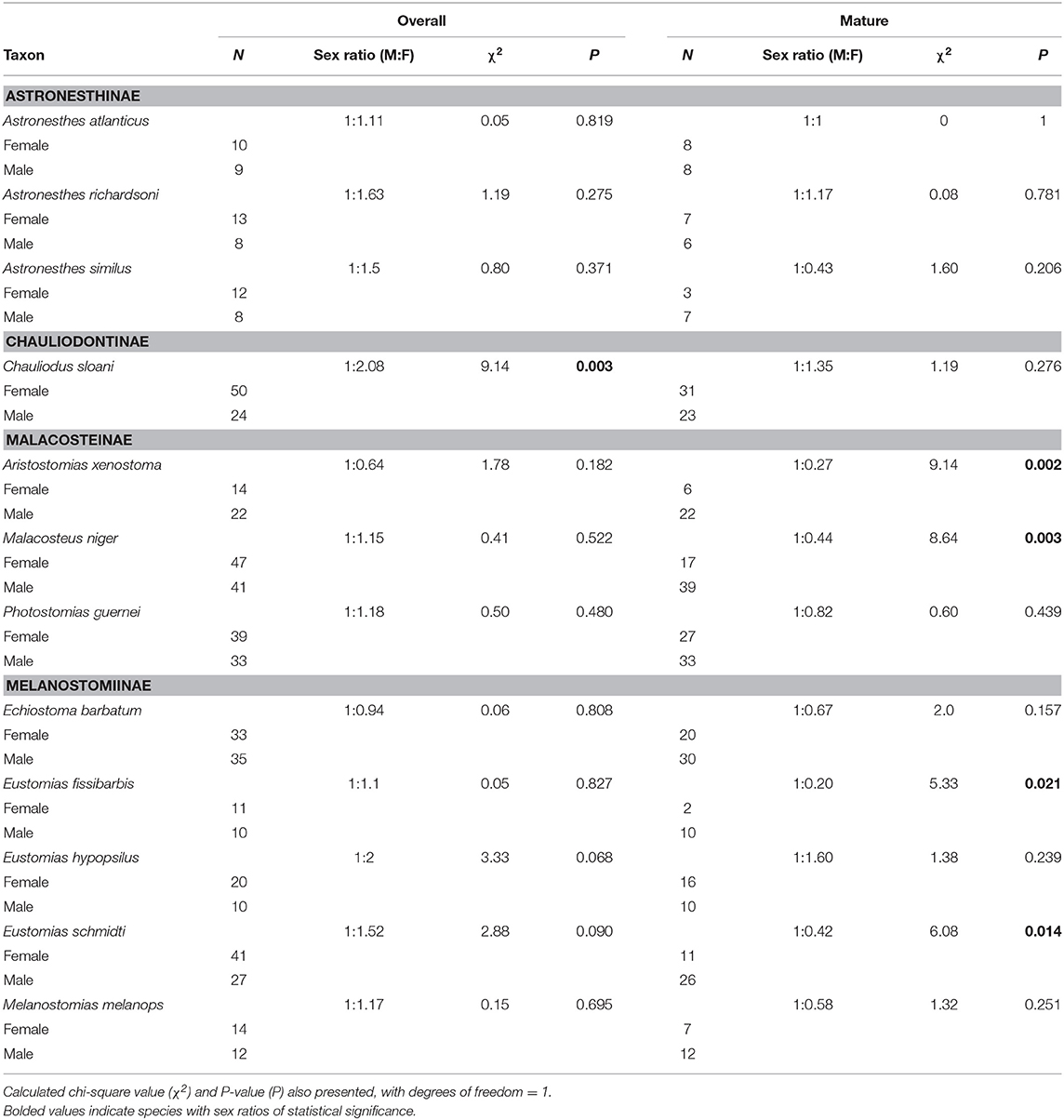
Table 3. Overall and mature sex ratios of 12 dominant stomiids in the Gulf of Mexico, listed by subfamily.
Size at Maturity
Maturity data were sufficient to estimate size at 50% maturity of five species using binomial regression. In increasing order, female size (SL) at 50% maturity was 106.4 mm in Eustomias hypopsilus, 110.9 mm in Malacosteus niger, 151.9 mm in Chauliodus sloani, 166.6 mm in Eustomias schmidti, and 200.5 mm in Echiostoma barbatum (Figure 4). In females of E. hypopsilus, the smallest mature specimen was larger than the estimated size at 50% maturity (Table 4) due to small sample size, especially in intermediate size classes.
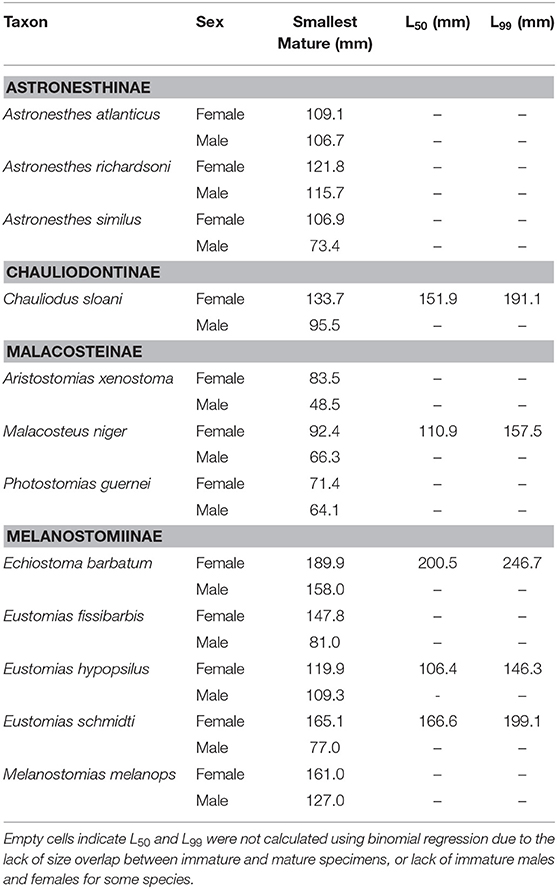
Table 4. Smallest size (standard length) at maturity, length at 50% maturity (L50), and length at 99% maturity (L99) of 12 dominant stomiids in the Gulf of Mexico, listed by subfamily, and sex.
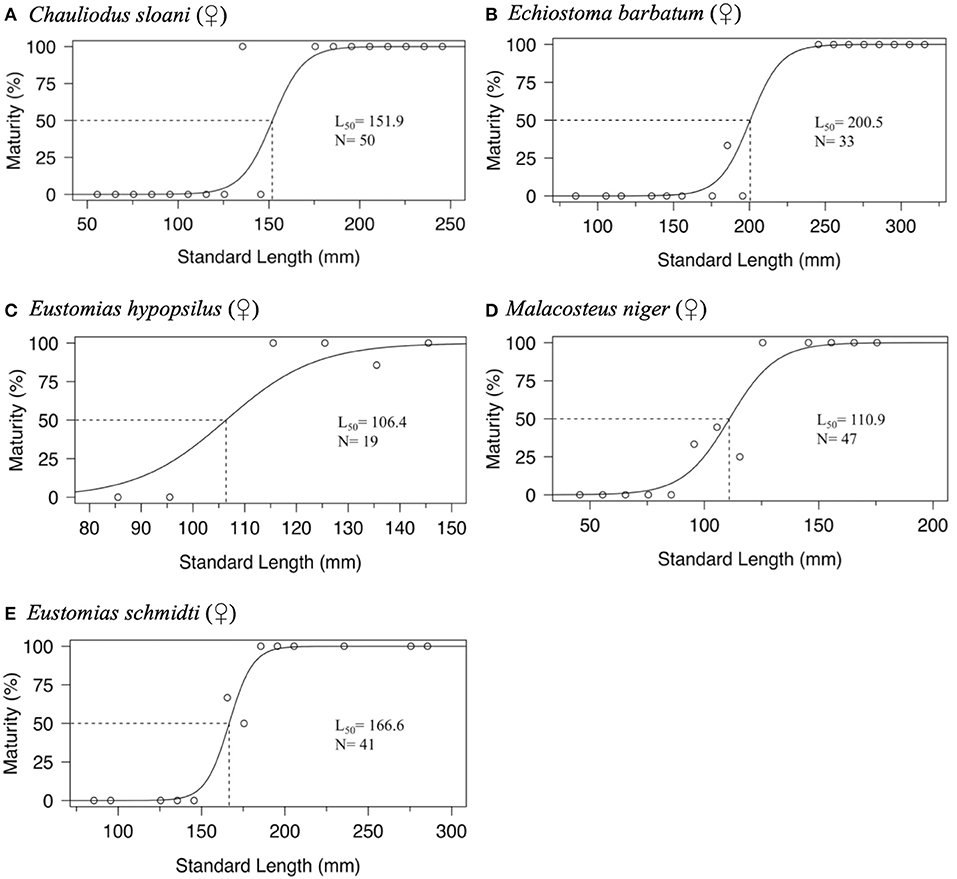
Figure 4. Binomial regression of size at maturity of: (A) female Chauliodus sloani, (B) female Echiostoma barbatum, (C) female Eustomias hypopsilus, (D) female Malacosteus niger, and (E) female Eustomias schmidti. Dotted lines indicate standard length at median sexual maturity (L50). N = sample size.
Binomial regression was not appropriate for the remaining species either because there was not enough variation in the ratio of immature to mature specimens per size class, or because size classes were perfect predictors of maturity state (all specimens were either immature or mature in a given size class). No immature males of Aristostomias xenostoma, Eustomias fissibarbis, Eustomias hypopsilus, Melanostomias melanops, and Photostomias guernei were identified, and less than four specimens were classified as immature for Astronesthes atlanticus, Astronesthes richardsoni, Astronesthes similus, Chauliodus sloani, and Malacosteus niger. The length of the smallest mature specimen of each sex was considered the size at maturity in species for which L50 could not be estimated. Specimen size at which the smallest mature individual was identified, as well as L50 and L99, is presented in Table 4. Males reached maturity at smaller lengths than their female conspecifics in all 12 species.
Size Distributions
Size-frequency distributions are shown in Figure 5 for each of the 12 dominant species. Distributions were separated by gear type to demonstrate the differing size selectivity of the MOCNESS and HSRT. Two patterns emerged, both of which were observed for every species: the MOCNESS captured smaller juveniles more effectively than the HSRT (Figure 6; two sample t-test, t = 42.3, P << 0.001), and the HSRT caught more specimens per species. Superimposing the size at maturity based on histological data revealed a third pattern: the majority of specimens collected with the HSRT were mature.
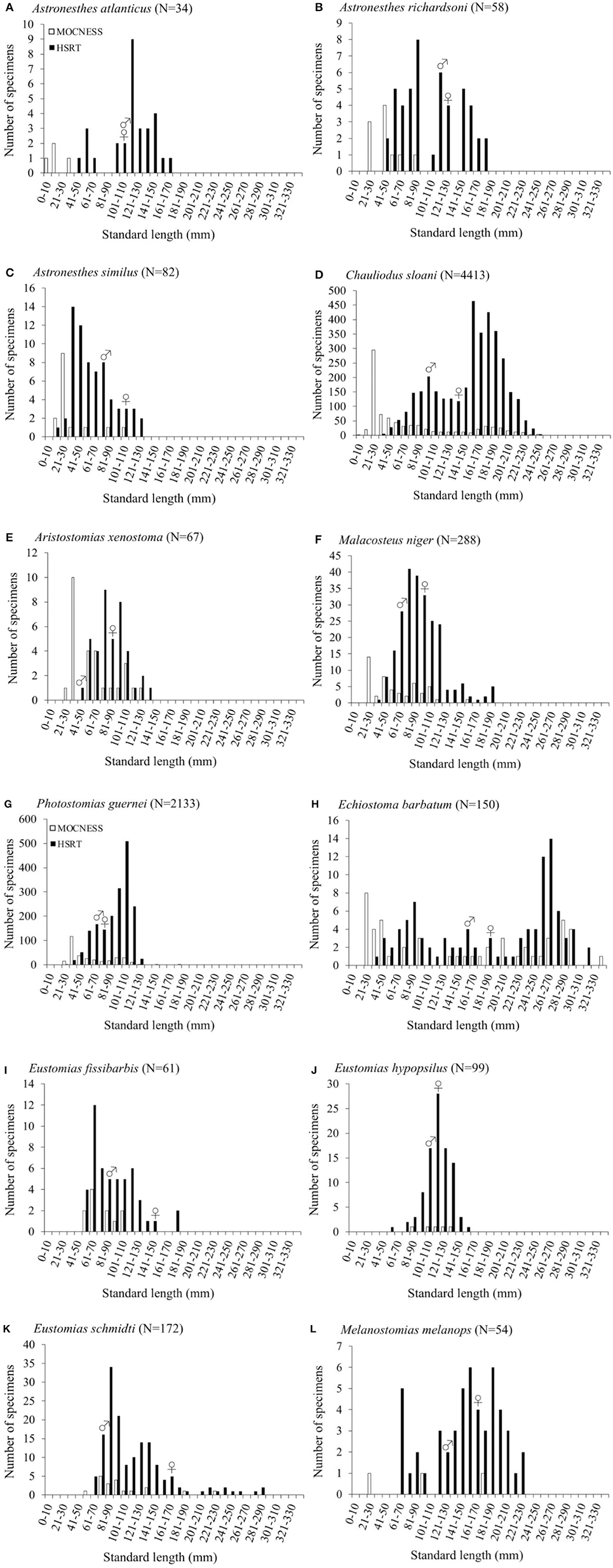
Figure 5. Size distributions as a function of gear type of (A) Astronesthes atlanticus, (B) Astronesthes richardsoni, (C) Astronesthes similus, (D) Chauliodus sloani, (E) Aristostomias xenostoma, (F) Malacosteus niger, (G) Photostomias guernei, (H) Echiostoma barbatum, (I) Eustomias fissibarbis, (J) Eustomias hypopsilus, (K) Eustomias schmidti, and (L) Melanostomias melanops from the northern Gulf of Mexico.
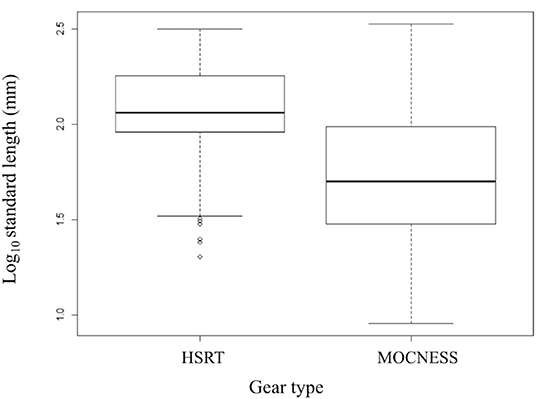
Figure 6. Boxplot comparing the size distributions of the 12 dominant stomiid species collected with the HSRT and MOCNESS in the Gulf of Mexico. Bolded horizontal lines indicate the median, and boxes represent the interquartile range (IQR), with the top and bottom lines indicating the 75th and 25th percentile, respectively. Whiskers represent minimum and maximum values no more than 1.5 times the IQR (upper value = 75th percentile + 1.5 × IQR, lower value = 25th percentile – 1.5 × IQR). Open circles represent outliers and indicate smaller fish were caught. HSRT, High-Speed Rope Trawl; MOCNESS, Multiple Opening/Closing Net and Environmental Sensing System.
Discussion
Histology and Reproductive Strategy
This study is the first extensive examination of the reproductive ecology of stomiids, the top predators of the mesopelagic zone, collected using multiple gear types that sample the full known size range of the taxa. No histological evidence of testicular and ovarian tissue was observed simultaneously in the gonad, indicating that stomiids are gonochoristic, unlike other species within the Stomiiformes. For example, protandric hermaphroditism has been documented in species of the family Gonostomatidae, including Cyclothone atraria (Gilbert, 1905); Sigmops bathyphilus [sensu Miya and Nishida (2000), formerly Gonostoma bathyphilum (Vaillant, 1884)]; S. elongatus [sensu Miya and Nishida (2000), formerly Gonostoma elongatum (Günther, 1878)]; and S. gracilis [sensu Miya and Nishida (2000), formerly Gonostoma gracile (Günther, 1878)] (Kawaguchi and Marumo, 1967; Fisher, 1983; Miya and Nemoto, 1985; Badcock, 1986). Considering that the deep-pelagic habitat harbors a lower abundance (per volume) of fishes and larger nearest-neighbor distances between conspecifics than coastal seas (Marshall, 1954), the chance of finding a mate is lower, and thus hermaphroditism could confer benefits through population-level “bet-hedging.” Typically, the terminal sex is female, meaning the bulk of the biomass of these species is found within the egg-producing component. However, maintaining separate sexes can increase reproductive fitness relative to hermaphroditism in two ways: energy can be focused on gamete production instead of reconfiguring the reproductive system, and reproductive opportunities will not be forfeited while undergoing the change (Warner, 1975; Helfman et al., 2009).
Gonochorism may benefit longer-lived predators during large-scale disturbances by preserving genetic diversity. If stomiids were protandrous hermaphrodites like other hermaphroditic stomiiform fishes, a sudden decline in smaller fishes due to a large-scale disturbance (DWHOS) could hypothetically cause a large-scale cohort loss of spawning females some length of time after the event. Smaller fishes may be more vulnerable to oil toxicity than larger fishes (Barron et al., 2004), so the loss of this cohort, which would never have been able to spawn, could result in a loss of genetic diversity at the population level. As genetic diversity is a primary attribute of resilience to disturbance, the finding of gonochorism in this keystone predator group (Sutton and Hopkins, 1996a) is significant.
Sex Ratio
Departure from the expected 1:1 sex ratio is not common in most fishes (Conover and Van Voorhees, 1990). For Chauliodus sloani, we found there was a significant bias in favor of females among juveniles. One hypothesis that can explain this is that females reach maturity at a larger size than males (Table 4). Overall, we found the higher ratio of females to males to be the exception; in most cases where sex ratio significantly favored one sex over the other, males were favored. This pattern held for Aristostomias xenostoma, Malacosteus niger, Eustomias fissibarbis, and Eustomias schmidti. One possible explanation for this pattern is that males mature faster because development of spermatozoa is energetically less costly than development of ova. If the assemblage of these species actually favors males, having a higher ratio of males to females would increase the probability of females finding mates. We believe that female location of a mate may be facilitated by dimorphisms in light organs; many stomiids have males that possess enlarged postorbital photophores in contrast to those of females, which are smaller or absent (Gibbs, 1964a; Morrow and Gibbs, 1964; Krueger and Gibbs, 1966; Borodulina, 1994, 2009). The benefit of larger postorbital photophores in males may be to aid females in finding a mate by increasing male detectability over greater distances in an environment with diminished light levels (Herring, 2000). The larger postorbital photophore in males can be seen as an evolutionary trade-off to small size, and thus restricted mobility, in male stomiids. Alternatively, divergence from a 1:1 sex ratio can be attributed to other factors such as differential growth rates and differences in spatial distributions between sexes (Trindade-Santos and Freire, 2015), or a genetic basis for sex determination. Little is known about the mechanism of sex determination in stomiids, but Chen (1969) presented evidence of heterogamety in the form of the XO sex type in Sternoptyx diaphana (Family Sternoptychidae), a family in the same order as the stomiids.
Previous studies on stomiid sex ratios are limited, so comparisons are not possible for most of the species studied. Based on 100 specimens taken with 40 and 60-ft midwater trawls, Krueger and Gibbs (1966) found the overall sex ratio of Echiostoma barbatum from the Mississippi Delta to be significantly female-biased, at 2:1, which differed from this study. Specimens ranged in size from 51.0 to 291.0 mm SL, with the largest male being 252 mm SL and the largest female at 291 mm. Krueger and Gibbs (1966) indicated that E. barbatum has dimorphic postorbital photophores and suggested that high male mortality is responsible for a female-biased sex ratio. While larger photophores may increase detection by females, the reasoned drawback is the simultaneous attraction of potential predators due to a more conspicuous display signal. Stomiids have been found in the diets of epipelagic fishes and mammals (Robison and Craddock, 1983; Flynn and Paxton, 2013), but no sex determination was made of those predated-upon individuals. If males experience higher rates of mortality due to increased detection by predators, sex ratios might favor females. However, the data presented in this study do not support this hypothesis.
Size at Maturity
The current data support the conclusion that, in general, female stomiids mature at larger sizes than males, which is a common life-history pattern in deep-sea fishes (Herring, 2002). In this study, Echiostoma barbatum was found to reach 50% maturity at much smaller lengths (158.0 mm SL for males, 189.9 mm SL for females) than those determined by Krueger and Gibbs (1966); they reported that males and females were mature at 220 and 271 mm SL, respectively. Differences in size at maturity of E. barbatum may be attributed to contrasting methodologies between studies. While we used histology to classify maturity state, Krueger and Gibbs (1966) relied upon oocyte size and macroscopic observations of gonads. Histology is a more accurate method to classify internal gonadal development and ultimately reveals more information on the reproductive development of fishes (West, 1990).
Size at maturity has also been reported in studies from other waters. For example, two species of Photostomias collected near Hawaii are reported to mature at 60 and 120 mm SL (Clarke, 1982), which is similar to the minimum length at maturity found in our study. However, Clarke did not report the species due to taxonomic uncertainty of the genus. Kenaley (2009) clarified the taxonomy of the unidentified Photostomias species collected by Clarke (1982). Clarke (2000) mentioned that female Eustomias fissibarbis and Eustomias schmidti from the North Atlantic Ocean mature at larger sizes than males, but no specific lengths were provided for comparison. The differences in size at maturity of E. barbatum and Photostomias guernei between this study and previous studies may be a result of the number and size of specimens available for histological analysis, or differences in environmental conditions, or gear types between the studies. For P. guernei, this difference may even be attributed to species; Kenaley (2009) described the two unidentified species from Clarke (1982) as new to science.
Binomial regression was not used to estimate minimum size at maturity in males and females of most species due to complete separation of maturity state. Small sample size, especially for male stomiids, likely contributed to complete separation. Only 11 (4%) of 239 male specimens were identified as immature. In males of Aristostomias xenostoma, Eustomias fissibarbis, Eustomias hypopsilus, Eustomias schmidti, and Melanostomias melanops, the smallest mature individual identified was also the smallest individual overall, suggesting that males of these species may mature at even smaller sizes. Alternatively, it could also suggest that immaturity is a relatively short-lived phase in the reproductive cycle of male stomiids, and reaching maturity is a rapid process. The example of the ribbon sawtail fish Idiacanthus fasciola Peters 1877, a species of barbeled stomiid, lends credence to this hypothesis. Males are no more than 10–20% the standard length of mature females, and are mature immediately following transformation from the larval stage (Marshall, 1954; Clarke, 1983). A larger sample of specimens representative of smaller size classes would likely improve the estimate of size of maturity in stomiids.
Size Distributions
While an advantage to using individual gear types for sampling midwater fishes is the targeting of specific sizes (Kashkin and Parin, 1983; Millar, 1992), it is also a potential hindrance, especially when assessing the size distributions of species assemblages. Using one type of gear can bias results and produce spurious conclusions because of size-specific selectivity (Willis et al., 2000). Gear selectivity in trawls is largely related to mesh size. In this study, the MOCNESS had a 3-mm mesh size and primarily caught smaller specimens. MacLennan (1992) reported that with smaller mesh sizes, the chance of smaller fishes escaping through the net is reduced. Larger nets, such as the rope trawl, effectively collect larger fishes, and yield a larger catch per unit effort (e.g., time) (Kashkin and Parin, 1983). Larger, mature stomiids would have been greatly underrepresented from the sample set and true size distributions would be poorly reflected if the only specimens used in this study were collected with the 10-m2 MOCNESS. The reproductive component of the assemblage would have been missed. From a sampling standpoint, the benefits of using a large rope trawl only apply to larger predatory species, not for smaller taxa. Using the larger gear was crucial to effectively sample the reproductive component of stomiid assemblages, and, in conjunction with the MOCNESS, depict a more accurate representation of stomiid size distributions in the Gulf of Mexico.
The Link Between Reproductive Ecology and Oil Contamination in Pelagic Ecosystems
A recent paper by Romero et al. (2018) chronicled the exposure and contamination of mesopelagic fishes by the DWHOS, using polycyclic aromatic hydrocarbon (PAH) signatures as a proxy to identify DWHOS hydrocarbons. These authors presented baseline (pre-spill) PAH levels, along with levels shortly after (2010–2011) and 5–7 years after the DWHOS. The authors reported a dramatic increase in PAH levels in muscle tissues of vertically migrating fishes from the families Gonostomatidae, Sternoptychidae, and Myctophidae (all primary prey of Stomiidae) in 2010–2011, then a reduction in PAH muscle tissue loads in 2015–2017 (but still above pre-spill levels). The primary pathway of incorporation of PAHs was identified as contaminated prey consumption. The findings most relevant to this study, however, were the heightened levels of PAHs in ovaries of fishes collected between 2015 and 2017, with eggs containing 13× more PAHs than other tissues. Furthermore, PAH levels from the eggs of fishes collected during this period were above levels known to cause abnormalities in developing fishes (Sundberg et al., 2006; Sørhus et al., 2017). An explanation for the differences in PAH concentration between eggs and somatic tissue is the maternal transfer of contaminants to offspring. Lipophilic contaminants such as PAHs are transferred to the egg during vitellogenesis, when maternal resources for embryo development are stored in the yolk (Lubzens et al., 2017). This mechanism of oil contaminant retention is important for future risk assessment and monitoring studies in the deep-pelagic ocean, where biological (e.g., egg production), chemical (e.g., oil composition), physical (e.g., animal and oil dispersion), and behavioral (e.g., vertical migration) factors are key processes with respect to exposure to contaminants, with bioaccumulation of organic chemicals in mesopelagic fishes potentially higher than their shallower counterparts (Romero et al., 2018).
Continued threats of major contamination events in the Gulf of Mexico are likely, given the trajectory of oil extraction going deeper (Sutton et al., 2020) and the increased likelihood of accidents with greater platform depth (8.5% for every 30 m; Muehlenbachs et al., 2013). The average depth of ultra-deep drilling in the Gulf of Mexico is now over 500 m deeper than Deepwater Horizon (Murawski et al., 2020). Therefore, given the link between oil contamination, egg production, and bioaccumulation, it is becoming increasingly important to understand the dynamics of deep-pelagic fish reproduction to understand the full effects of oil spills in the deep-pelagic environment.
In conclusion, given the link between increasing petrogenic contamination of the deep ocean and fish reproduction, the key questions for which we have urgent need for information include: (1) how often do fishes spawn (e.g., iteroparous vs. semelparous, total vs. batch spawning); (2) what proportion of the fish population spawns; and (3) when do females produce eggs. In this study, the first extensive study on the reproductive ecology of stomiids in the Atlantic Ocean, as well as the largest study on the reproductive ecology of this family globally, we provide evidence that the 12 stomiids investigated maintain separate sexes, exhibit asynchronous oocyte development and batch spawning, and generally have even sex ratios, with females reaching maturity at larger sizes than males. Further studies of the deep-pelagic domain in other regions will allow comparisons of stomiid reproduction on a global basis, though future investigators must carefully consider gear type due to the inherent avoidance capacity of larger fishes. Our findings provide critical keystone predator data for oceanic ecosystem and bioenergetics modeling and suggest that stomiid reproductive tactics enhance the taxon's resilience to point-source disturbances such as DWHOS, which ideally would serve to maintain carbon flow and the reproductive health of the deep-pelagic Gulf of Mexico.
Data Availability Statement
The datasets generated for this study are available on request to the corresponding author.
Ethics Statement
Ethical review and approval was not required for the animal study because the vertebrate animals we worked with for this study were all dead before research began.
Author Contributions
AM conducted the research, analyzed the data, and wrote the manuscript with input from DK, DW, and TS. DK also provided statistical guidance and DW assisted in reading histology slides and analyzing histological data. TS oversaw all aspects of this research, including the collection, and identification of specimens. All authors have agreed to being listed as such and approve of the submitted version of this manuscript.
Funding
This research was made possible in part by a grant from The Gulf of Mexico Research Initiative. Data are publicly available through the Gulf of Mexico Research Initiative Information & Data Cooperative (GRIIDC) at https://data.gulfresearchinitiative.org (doi: 10.7266/N7ZS2V04).
Conflict of Interest
TS, one of the editors for the research topic in which this manuscript will be submitted, is also a coauthor on this manuscript. However, he did not review this paper.
The remaining authors declare that the research was conducted in the absence of any commercial or financial relationships that could be construed as a potential conflict of interest.
Acknowledgments
We are indebted to the captains and crews of the M/V Meg Skansi and NOAA FRV Pisces, as well as the scientific team for their shiptime services during sample collection and processing. Similarly, we thank Katie Bowen, Kendall Lord, Lacey Malarky, Michael Novotny, and Nina Pruzinsky of the Oceanic Ecology Laboratory at Nova Southeastern University for their assistance with quantitative sample processing. We especially thank April Cook for her assistance with database management and Rosanna Milligan for statistical support. This is contribution number 820 of the South Carolina Marine Resources Center.
Supplementary Material
The Supplementary Material for this article can be found online at: https://www.frontiersin.org/articles/10.3389/fmars.2020.00101/full#supplementary-material
References
Ayres, W. O. (1848). Description of a new genus of fishes, Malacosteus. Proc. Boston. Soc. Nat. Hist. 3, 69–70.
Badcock, J. (1986). Aspects of the reproductive biology of Gonostoma bathyphilum (Gonostomatidae). J. Fish. Biol. 29, 589–603. doi: 10.1111/j.1095-8649.1986.tb04975.x
Barnett, M. A., and Gibbs, R. H. (1968). Four new stomiatoid fishes of the genus Bathophilus with a revised key to the species of Bathophilus. Copeia 1968, 826–832. doi: 10.2307/1441850
Barron, M. G., Carls, M. G., Heintz, R., and Rice, S. D. (2004). Evaluation of fish early life-stage toxicity models of chronic embryonic exposures to complex polycyclic aromatic hydrocarbon mixtures. Toxicol. Sci. 78, 60–67. doi: 10.1093/toxsci/kfh051
Bloch, M. E., and Schneider, J. G. (1801). Systema Ichthyologiae: Iconibus CX Ilustratum, Vol. 1. Berolini: Sumtibus Auctoris Impressum et Bibliopolio Sanderiano Commissum.
Borodulina, O. (1994). The external structure of the postorbital organ in species of the genus Astronesthes (Astronesthidae). J. Ichthyol. 34, 142–150.
Borodulina, O. (2009). External structure of the postorbital organ in some representatives of the family Melanostomiidae (Stomiiformes). J. Ichthyol. 49, 698–701. doi: 10.1134/S0032945209080165
Brauer, A. (1902). Diagnosen von neuen Tiefseefischen welche von der Valdivia-Expedition gesammelt sind. Zool. Anz. 25, 277–298.
Brown, E. D., Baker, T., Hose, J., Kocan, R., Marty, G., McGurk, M., et al. (1996). “Injury to the early life history stages of Pacific herring in Prince William Sound after the Exxon Valdez oil spill,” in Proc 18 Am Fish Soc Symp (Anchorage, AK), 448–462.
Brown-Peterson, N. J., Wyanski, D. M., Saborido-Rey, F., Macewicz, B. J., and Lowerre-Barbieri, S. K. (2011). A standardized terminology for describing reproductive development in fishes. Mar. Coast. Fish. 3, 52–70. doi: 10.1080/19425120.2011.555724
Burdett, E. A., Fine, C. D., Sutton, T. T., Cook, A. B., and Frank, T. M. (2017). Geographic and depth distributions, ontogeny, and reproductive seasonality of decapod shrimps (Caridea: Oplophoridae) from the northeastern Gulf of Mexico. Bull. Mar. Sci. 93, 743–767. doi: 10.5343/bms.2016.1083
Butler, M., Bollens, S. M., Burkhalter, B., Madin, L. P., and Horgan, E. (2001). Mesopelagic fishes of the Arabian Sea: distribution, abundance and diet of Chauliodus pammelas, Chauliodus sloani, Stomias Affinis, and Stomias Nebulosus. Deep Sea Res. Topical Stud. Oceanogr. 48, 1369–1383. doi: 10.1016/S0967-0645(00)00143-0
Childress, J., Taylor, S., Cailliet, G. M., and Price, M. (1980). Patterns of growth, energy utilization and reproduction in some meso-and bathypelagic fishes off southern California. Mar. Biol. 61, 27–40. doi: 10.1007/BF00410339
Clarke, T. A. (1974). Some aspects of the ecology of stomiatoid fishes in the Pacific Ocean near Hawaii. Fish. Bull. 72, 337–351.
Clarke, T. A. (1982). Feeding habits of stomiatoid fishes from Hawaiian waters. Fish. Bull. 80, 287–304.
Clarke, T. A. (1983). Sex ratios and sexual differences in size among mesopelagic fishes from the central Pacific Ocean. Mar. Biol. 73, 203–209. doi: 10.1007/BF00406889
Clarke, T. A. (2000). Review of nine species of North Atlantic Eustomias, subgenus Dinematochirus (Pisces: Stomiidae), with the description of two new species. Copeia 2000, 96–111. doi: 10.1643/0045-8511(2000)2000[0096:RONSON]2.0.CO;2
Collett, R. (1889). Diagnoses de poissons nouveaux provenant des campagnes de “L'Hirondelle.” II. Sur un genre nouveau de la famille des Stomiatidae. Bull. Soc. Zool. Fr. 14, 291–293.
Conover, D. O., and Van Voorhees, D. A. (1990). Evolution of a balanced sex ratio by frequency-dependent selection in a fish. Science 250, 1556–1558. doi: 10.1126/science.250.4987.1556
Dotson, R. C., and Griffith, D. A. (1996). A high-speed midwater rope trawl for collecting coastal pelagic fishes. CalCOFI Rep. 37, 134–139.
Fink, W. L. (1985). Phylogenetic interrelationships of the stomiid fishes (Teleostei: Stomiiformes). Misc. Publ. Mus. Zool. Univ. Mich. 171, 1–127.
Fisher, J. P., and Pearcy, W. G. (1983). Reproduction, growth and feeding of the mesopelagic fish Tactostoma macropus (Melanostomiatidae). Mar. Biol. 74, 257–267. doi: 10.1007/BF00403449
Fisher, R. A. (1983). Protrandric sex reversal in Gonostoma elongatum (Pisces: Gonostomatidae) from the eastern Gulf of Mexico. Copeia 1983, 554–557. doi: 10.2307/1444411
Flynn, A., and Klepadlo, C. (2012). Two new species of Photonectes (Teleostei: Stomiidae) from the Indo-Pacific, and a re-examination of P Achirus. Mem. Mus. Victoria 69, 259–267. doi: 10.24199/j.mmv.2012.69.04
Flynn, A. J., and Paxton, J. R. (2013). Spawning aggregation of the lanternfish Diaphus danae (family Myctophidae) in the north-western Coral Sea and associations with tuna aggregations. Mar. Freshw. Res. 63, 1255–1271. doi: 10.1071/MF12185
Fricke, R., Eschmeyer, W. N., and Fong, J. D. (2018a). Species by Family/Subfamily. Available online at: http://researcharchive.calacademy.org/research/ichthyology/catalog/SpeciesByFamily.asp (accessed Jun 12, 2018).
Fricke, R., Eschmeyer, W. N., and van der Laan, R. (2018b). Catalog of Fishes: Genera, Species, References. Avaliable online at: http://researcharchive.calacademy.org/research/ichthyology/catalog/fishcatmain.asp (accessed Nov 19, 2018).
Gibbs, R. H. (1964a). “Family Astronesthidae,” in Fishes of the Western North Atlantic, eds H. B. Bigelow, D. M. Cohen, M. M. Dick, R. H. Gibbs, M. Grey, J. E. Morrow, L. P. Schultz, and V. Walters (New Haven, CT: Yale University Press), 311–350. doi: 10.2307/j.ctvbcd0k1.11
Gibbs, R. H. (1964b). “Family Idiacanthidae,” in Fishes of the Western North Atlantic, Vol. 4, eds H. B. Bigelow, C. M. Breder, D. M. Cohen, G. W. Mead, D. Merriman, Y. H. Olsen, et al. (New Haven, CT: Yale University Press), 512–522. doi: 10.2307/j.ctvbcd0k1.13
Gibbs, R. H. (1969). Taxonomy, sexual dimorphism, vertical distribution, and evolutionary zoogeography of the bathypelagic fish genus Stomias (Stomiatidae). Smithson Contrib. Zool. 31, 1–25. doi: 10.5479/si.00810282.31
Gibbs, R. H., Clarke, T. A., and Gomon, J. R. (1983). Taxonomy and Distribution of the Stomioid Fish Genus Eustomias (Melanostomiidae), I: Subgenus Nominostomias. Washington, DC: Smithsonian Institution Press.
Gibbs, R. H., and McKinney, J. F. (1988). High-count species of the stomiid fish genus Astronesthes from Southern subtropical convergence region: two new species and redescription of Cryptostomias (Astronesthes) psychrolutes. Smithson Contrib. Zool. 460, 1–25. doi: 10.5479/si.00810282.460
Gilbert, C. H. (1905). The deep-sea fishes of the Hawaiian Islands. U.S. Fish. Comm. Bull. 23, 577–713.
Gjøsaeter, J., and Kawaguchi, K. (1980). A review of the world resources of mesopelagic fish. FAO Fish. Tech. Pap. 193, 1–151.
Gomon, J. R., and Gibbs, R. H. (1985). Taxonomy and distribution of the stomioid fish genus Eustomias (Melanostomiidae), II: Biradiostomias, new subgenus. Smithson Contrib. Zool. 409, 1–58. doi: 10.5479/si.00810282.409
Goodyear, R. H., and Gibbs, R. H. (1969). Systematics and zoogeography of stomiatoid fishes of the Astronesthes cyaneus species group (Family Astronesthidae) with descriptions of three new species. Arch. Fischereiwiss 20, 107–131.
Grier, H. J., and Uribe, M. C. (2009). “The testes and spermatogenesis in teleosts,” in Reproductive Biology and Phylogeny of Fishes (Agnathans and Bony Fishes), ed B. G. Jamieson (Enfield, NH: Science Publishers), 119–142.
Günther, A. (1878). II.-Preliminary notices of deep-sea fishes collected during the voyage of H.M.S ‘Challenger’. J. Nat. Hist. 2, 17–28.
Hansen, M. J., Boisclair, D., Brandt, S. B., Hewett, S. W., Kitchell, J. F., Lucas, M. C., et al. (1993). Applications of bioenergetics models to fish ecology and management: where do we go from here? Trans. Am. Fish. Soc. 122, 1019–1030. doi: 10.1577/1548-8659(1993)122<1019:AOBMTF>2.3.CO;2
Helfman, G., Collette, B. B., Facey, D. E., and Bowen, B. W. (2009). The Diversity of Fishes: Biology, Evolution, and Ecology. Sussex: John Wiley & Sons.
Herring, P. J. (2000). Species abundance, sexual encounter and bioluminescent signalling in the deep sea. Philos. Trans. R. Soc. 355, 1273–1276. doi: 10.1098/rstb.2000.0682
Hopkins, T. L., and Gartner, J. V. (1992). Resource-partitioning and predation impact of a low-latitude myctophid community. Mar. Biol. 114, 185–197. doi: 10.1007/BF00349518
Irigoien, X., Klevjer, T. A., Røstad, A., Martinez, U., Boyra, G., Acuña, J., et al. (2014). Large mesopelagic fishes biomass and trophic efficiency in the open ocean. Nat. Commun. 5:3271. doi: 10.1038/ncomms4271
Kaartvedt, S., Staby, A., and Aksnes, D. L. (2012). Efficient trawl avoidance by mesopelagic fishes causes large underestimation of their biomass. Mar. Ecol. Prog. Ser. 456, 1–6. doi: 10.3354/meps09785
Kashkin, N. I., and Parin, N. V. (1983). Quantitative assessment of micronektonic fishes by nonclosing gear (a review). Biol. Oceanogr. 2, 263–287.
Kawaguchi, K., and Marumo, R. (1967). Biology of Gonostoma gracile (Gonostomatidae). I. Morphology, life history and sex reversal. Inform. Bull. Plankt. Jpn. 1967, 53–69.
Kenaley, C. P. (2007). Revision of the stoplight loosejaw genus Malacosteus (Teleostei: Stomiidae: Malacosteinae), with description of a new species from the temperate southern hemisphere and Indian Ocean. Copeia 2007, 886–900. doi: 10.1643/0045-8511(2007)7[886:ROTSLG]2.0.CO;2
Kenaley, C. P. (2009). Revision of indo-pacific species of the loosejaw dragonfish genus Photostomias (Teleostei: Stomiidae: Malacosteinae). Copeia 2009, 175–189. doi: 10.1643/CI-07-224
Kenaley, C. P. (2012). Exploring feeding behaviour in deep-sea dragonfishes (Teleostei: Stomiidae): jaw biomechanics and functional significance of a loosejaw. Biol. J. Linn. Soc. 106, 224–240. doi: 10.1111/j.1095-8312.2012.01854.x
Kenaley, C. P., and Hartel, K. E. (2005). A revision of Atlantic species of Photostomias (Teleostei: Stomiidae: Malacosteinae), with a description of a new species. Ichthyol. Res. 52, 251–263. doi: 10.1007/s10228-005-0281-7
Koslow, J. A., Kloser, R. J., and Williams, A. (1997). Pelagic biomass and community structure over the mid-continental slope off southeastern Australia based upon acoustic and midwater trawl sampling. Mar. Ecol. Prog. Ser. 30, 21–35. doi: 10.3354/meps146021
Krueger, W. H., and Gibbs, R. H. (1966). Growth changes and sexual dimorphism in the stomiatoid fish Echiostoma barbatum. Copeia 1966, 43–49. doi: 10.2307/1440759
Lowe, R. (1843). Notices of fishes newly observed or discovered in Madeira during the years 1840, 1841, and 1842. Proc. Zool. Soc. Lond. 11, 81–95.
Lubzens, E., Bobe, J., Young, G., and Sullivan, C. V. (2017). Maternal investment in fish oocytes and eggs: the molecular cargo and its contributions to fertility and early development. Aquaculture 472, 107–143. doi: 10.1016/j.aquaculture.2016.10.029
MacLennan, D. N. (1992). Fishing gear selectivity: an overview. Fish. Res. 13, 201–204. doi: 10.1016/0165-7836(92)90076-6
Millar, R. B. (1992). Estimating the size-selectivity of fishing gear by conditioning on the total catch. J. Am. Stat. Assoc. 87, 962–968. doi: 10.1080/01621459.1992.10476250
Miya, M., and Nemoto, T. (1985). Protandrous sex reversal in Cyclothone atraria (Family Gonostomatidae). Jpn. J. Ichthyol. 31, 438–440.
Miya, M., and Nishida, M. (2000). Molecular systematics of the deep-sea fish genus Gonostoma (Stomiiformes: Gonostomatidae): two paraphyletic clades and resurrection of Sigmops. Copeia 2000, 378–389. doi: 10.1643/0045-8511(2000)000[0378:MSOTDS]2.0.CO;2
Morrow, J. E. (1964a). “Family Chauliodontidae,” in Fishes of the Western North Atlantic, eds H. B. Bigelow, D. M. Cohen, M. M. Dick, R. H. Gibbs, M. Grey, J. E. Morrow, L. P. Schultz, and V. Walters (New Haven, CT: Yale University Press), 274–289. doi: 10.2307/j.ctvbcd0k1.9
Morrow, J. E. (1964b). “Family Stomiatidae,” in Fishes of the Western North Atlantic, eds H. B. Bigelow, D. M. Cohen, M. M. Dick, R. H. Gibbs, M. Grey, J. E. Morrow, L. P. Schultz, and V. Walters (New Haven, CT: Yale University Press), 290–310. doi: 10.2307/j.ctvbcd0k1.10
Morrow, J. E. (1964c). “Family Malacosteidae,” in Fishes of the Western North Atlantic, eds H. B. Bigelow, C. M. Breder, D. M. Cohen, G. W. Mead, D. Merriman, Y. H. Olsen, et al. (New Haven, CT: Yale University Press), 523–549. doi: 10.2307/j.ctvbcd0k1.14
Morrow, J. E., and Gibbs, R. H. (1964). “Family Melanostomiatidae,” in Fishes of the Western North Atlantic, eds H. B. Bigelow, C. M. Breder, D. M. Cohen, G. W. Mead, D. Merriman, Y. H. Olsen, et al. (New Haven, CT: Yale University Press), 351–511. doi: 10.2307/j.ctvbcd0k1.12
Muehlenbachs, L., Cohen, M. A., and Gerarden, T. (2013). The impact of water depth on safety and environmental performance in offshore oil and gas production. Energy Policy 55, 699–705. doi: 10.1016/j.enpol.2012.12.074
Murawski, S., Hollander, D. J., Gilbert, S., and Gracia, A. (2020) “Deep-water oil gas production in the Gulf of Mexico, related global trends,” in Scenarios Responses to Future Deep Oil Spills, eds S. Murawski, C. Ainsworth, S. Gilbert, D. Hollander, C. Paris, M. Schlüter (Cham: Springer), 16–32. doi: 10.1007/978-3-030-12963-7_2
Nelson, J. S., Grande, T. C., and Wilson, M. V. (2016). Fishes of the World. Hoboken, NJ: John Wiley & Sons.
Pappenheim, P. (1914). Die Fische der Deutschen Südpolar-Expedition 1901–1903. II. Die Tiefseefische. Deutschen Südpolar-Expedition. 15, 161–200.
Parin, N., and Borodulina, O. (1996). Revision of the Astronesthes indicus species group (Astronesthidae), with descriptions of five new species. J. Ichthyol. 36, 551–565.
Parr, A. E. (1927). The stomiatoid fishes of the suborder Gymnophotodermi (Astronesthidae, Melanostomiatidae, Idiacanthidae) with a complete review of the species. Bull. Bingham Oceanogr. Coll. 3, 1–123.
Poey, F. (1852). Memorias sobre la Historia Natural de la Isla de Cuba: Accompañadas de Sumarios Latinos y Extractos en Frances, Vol. 1. La Habana: Barcina.
R Development Core Team (2013). A Language and Environment for Statistical Computing. Vienna: R Foundation for Statistical Computing.
Regan, C. T., and Trewavas, E. (1930). The fishes of the families Stomiatidae and Malacosteidae. Danish Dana Expedition 1920–22. 6, 1–143.
Robison, B. H., and Craddock, J. E. (1983). Mesopelagic fishes eaten by Fraser's dolphin, Lagenodelphis hosei. Fish. Bull. 81, 283–289.
Romero, I. C., Sutton, T., Carr, B., Quintana-Rizzo, E., Ross, S. W., Hollander, D. J., et al. (2018). Decadal assessment of polycyclic aromatic hydrocarbons in mesopelagic fishes from the Gulf of Mexico reveals exposure to oil-derived sources. Environ. Sci. Technol. 52, 10985–10996. doi: 10.1021/acs.est.8b02243
Short, J. (2003). Long-term effects of crude oil on developing fish: lessons from the Exxon Valdez oil spill. Energy Sources 25, 509–517. doi: 10.1080/00908310390195589
Sørhus, E., Incardona, J. P., Furmanek, T., Goetz, G. W., Scholz, N. L., Meier, S., et al. (2017). Novel adverse outcome pathways revealed by chemical genetics in a developing marine fish. Elife 6, 1–30. doi: 10.7554/eLife.20707
Sundberg, H., Ishaq, R., Tjärnlund, U., Åkerman, G., Grunder, K., Bandh, C., et al. (2006). Contribution of commonly analyzed polycyclic aromatic hydrocarbons (PAHs) to potential toxicity in early life stages of rainbow trout (Oncorhynchus mykiss). Can. J. Fish. Aquat. Sci. 63, 1320–1333. doi: 10.1139/f06-034
Sutton, T. T., Frank, T., Judkins, H., and Romero, I. C. (2020) “As oil extraction goes deeper, who is at risk? community structure, distribution connectivity of the deep-pelagic fauna,” in Scenarios Responses to Future Deep Oil Spills, eds S. Murawski, C. Ainsworth, S. Gilbert, D. Hollander, C. Paris, M. Schlüter (Cham: Springer), 403–418. doi: 10.1007/978-3-030-12963-7_24
Sutton, T. T., and Hartel, K. E. (2004). New species of Eustomias (Teleostei: Stomiidae) from the western North Atlantic, with a review of the subgenus Neostomias. Copeia 2004, 116–121. doi: 10.1643/CI-03-120R1
Sutton, T. T., and Hopkins, T. L. (1996a). Trophic ecology of the stomiid (Pisces: Stomiidae) fish assemblage of the eastern Gulf of Mexico: strategies, selectivity and impact of a top mesopelagic predator group. Mar. Biol. 127, 179–192. doi: 10.1007/BF00942102
Sutton, T. T., and Hopkins, T. L. (1996b). Species composition, abundance, and vertical distribution of the stomiid (Pisces: Stomiiformes) fish assemblage of the Gulf of Mexico. Bull. Mar. Sci. 59, 530–542.
Trindade-Santos, I., and Freire, K. M. F. (2015). Analysis of reproductive patterns of fishes from three large marine ecosystems. Front. Mar. Sci. 2:38. doi: 10.3389/fmars.2015.00038
Vaillant, L. L. (1884). In Filhol, H. Explorations sous-marines. Voyage du “Talisman”. La Nat. 559. 182–186.
Venables, W. N., and Ripley, B. D. (2002). Modern Applied Statistics With S, 4th Edn. Berlin, Heidelberg: Springer-Verlag.
Warner, R. R. (1975). The adaptive significance of sequential hermaphroditism in animals. Am. Nat. 109, 61–82. doi: 10.1086/282974
West, G. (1990). Methods of assessing ovarian development in fishes: a review. Mar. Freshw. Res. 41, 199–222. doi: 10.1071/MF9900199
Wiebe, P. H., Morton, A. W., Bradley, A. M., Backus, R. H., Craddock, J. E., Barber, V., et al. (1985). New development in the MOCNESS, an apparatus for sampling zooplankton and micronekton. Mar. Biol. 87, 313–323. doi: 10.1007/BF00397811
Keywords: Stomiidae, reproduction, mesopelagic, sex ratio, gonad histology, maturity, size distributions
Citation: Marks AD, Kerstetter DW, Wyanski DM and Sutton TT (2020) Reproductive Ecology of Dragonfishes (Stomiiformes: Stomiidae) in the Gulf of Mexico. Front. Mar. Sci. 7:101. doi: 10.3389/fmars.2020.00101
Received: 30 August 2019; Accepted: 07 February 2020;
Published: 03 March 2020.
Edited by:
Michael Vecchione, National Oceanic and Atmospheric Administration (NOAA), United StatesReviewed by:
Shannon Colleen DeVaney, Pierce College, United StatesMackenzie E. Gerringer, The State University of New York College Geneseo, United States
Copyright © 2020 Marks, Kerstetter, Wyanski and Sutton. This is an open-access article distributed under the terms of the Creative Commons Attribution License (CC BY). The use, distribution or reproduction in other forums is permitted, provided the original author(s) and the copyright owner(s) are credited and that the original publication in this journal is cited, in accordance with accepted academic practice. No use, distribution or reproduction is permitted which does not comply with these terms.
*Correspondence: Alex D. Marks, am2919@nova.edu