- 1CCMAR, Centro de Ciências do Mar do Algarve, Universidade do Algarve, Faro, Portugal
- 2CBQF - Centro de Biotecnologia e Química Fina e Laboratório Associado, Escola Superior de Biotecnologia, Rua Arquiteto Lobão Vital, Universidade Católica Portuguesa, Porto, Portugal
- 3Lucta S.A., Innovation Division, UAB Research Park, Edifici Eureka - MRB, Barcelona, Spain
Cephalopods are gaining momentum as an alternate group for aquaculture species diversification, not only because they are a good food source (highly appreciated in some worldwide markets) but they also have the potential to quickly reach a market size. However, there are some bottlenecks impeding the transition of culture technology from the laboratory to industry. One is related to control over reproduction in captivity. The objective of the present experiment was to verify the effects of tanks with different bottom areas/volumes on the reproduction performance of S. officinalis breeding stocks, when sex ratios were controlled a priori; and the food cost associated with such performance when individuals are fed a natural frozen diet. One hundred and ninety two juvenile cuttlefish were used to compare three different round-shaped tanks: one type with 3000L volume and two types with 9000L volume (with differences in bottom areas and water column). Individuals had their sex and maturity stage determined to establish a sexual ratio of 2♀:1♂ per tank and assure that cuttlefish were still immature. Biological data was collected during both growth and reproduction stages and until the death of all females in each tank. The experiment lasted nearly 300 days. Temperature differences between tank types were registered during both stages. The optimizing of rearing conditions has allowed for higher growth and a higher amount of cuttlefish available for breeding purposes. A total of 123,751 eggs (in 85 batches) was obtained during this experiment, which is a number that may meet a small scale cuttlefish commercial hatchery facility requirements. The present conditions contributed to a better and predictable reproduction performance in specific 9000L tanks, with values reaching pre-industrial numbers (≈ 24,000 eggs/tank). Moreover, both the amount of eggs per batch and the overall quality of eggs has increased. Three of these 9000L tanks have an overall consumption of ≈ 38.64 Kg tank–1, which translates in an investment in feed of ≈ 193 € tank–1, 8.40 € per cuttlefish and an overall daily tank expense of 1.76 € d–1.
Introduction
The stagnation of global capture fishery production has led to the fast growth of the aquaculture industry as the world’s fastest-growing source of animal protein. In addition, the recent societal challenge to feed 9 billion people by 2050 (Béné et al., 2015) puts additional pressure to the rapid rise in the number of aquatic species under domestication (Duarte et al., 2007), due on the needs of diversification in production. An important step toward species domestication is control over reproduction in captivity; being essential for obtaining high quality eggs and offspring, which promote the sustainability of commercial aquaculture production (Mylonas et al., 2010; Migaud et al., 2013). In this sense, unpredictable reproduction performance is seen as a major bottleneck for the successful industrial production of finfish juveniles (Izquierdo et al., 2001) and cephalopods (Vidal et al., 2014; Villanueva et al., 2014).
Cephalopods, namely species as the cuttlefish (Sepia officinalis) and the common octopus (Octopus vulgaris) have long been pointed with high potential for European aquaculture diversification because they are highly valued and appreciated worldwide (e.g., Southern European and Asian countries); and have the potential to quickly reach a marketable size (Barnabé, 1996; Iglesias et al., 2014). The species within this class display diverse reproduction strategies but most share a single reproduction period over its life cycle (Rocha et al., 2001; Boletzky and Villanueva, 2014). This means that the broodstock management of cephalopods will be different to that of most finfish species currently under production. Despite multiple consecutive generations of S. officinalis having been achieved in captivity (Forsythe et al., 1994; Sykes et al., 2006a), reproduction performance results are inconsistent (in terms of replication) and do not meet the occurring quantity and/or quality observed in the wild (Laptikhovsky et al., 2003; Sykes et al., 2006b). Recently, Sykes et al. (2013) have hypothesized that this unpredictability is probably due to insufficient knowledge on the variables that affect directly or indirectly the captive breeding stock. Comparatively, this issue is more important in S. officinalis than with O. vulgaris since the first species displays natural low fecundity and there are reports of the eventual existence of inbreeding after 6 consecutive generations of culture (Sykes et al., 2006a), while the second species can lay up to 500,000 eggs/female in nature (Mangold, 1983) and ≈100,000 eggs/female are obtained in captivity (Iglesias and Fuentes, 2014).
Like in finfish (Migaud et al., 2013), reproduction performance of S. officinalis will rely on optimal broodstock management practices based on extensive knowledge of the nutritional (Izquierdo et al., 2001) and environmental requirements (Mylonas et al., 2010) of the species in captivity. However, both knowledge on nutrition and digestive physiology of cuttlefish, and in general of cephalopods, is still currently low (Navarro et al., 2014; Sykes et al., 2017a). Therefore, until now the current management of the cuttlefish breeding stock has included feeding on natural frozen food (Sykes et al., 2006a, 2013, 2014). It is known that tank size (water volume and/or depth) and bottom areas (Sykes et al., 2013), as well as stocking density (Correia et al., 2005), influence cuttlefish reproduction performance. However, cuttlefish behavior and eventual stress in captivity (in both growth and reproduction phases) should not be overlooked when setting up the breeding stocks, as Conte (2004) reported that rearing/housing conditions influence finfish welfare translating into lower female quality and reproduction performance. In finfish, spontaneous spawning behavior (Mylonas et al., 2010) and reproduction performance is usually attained with moderate to large holding volumes, low stocking densities (Okumura et al., 2003; Buchet et al., 2008) and 1 female: 1 male sex ratios (Mylonas et al., 2011). Moreover, it has been recently reported that, similar to cultured sea bream (Sparus aurata) (Castanheira et al., 2013, 2016), S. officinalis display personality traits (Carere et al., 2015; Zoratto et al., 2018) which will also influence growth and reproduction. Hence, the use of circular tanks for the breeding stock allow for enough space for cuttlefish in the bottom (growth stage) and water column (reproduction stage) and promote increased interaction between individuals during growth and reproduction (Sykes et al., 2013). Moreover, these interactions seem to promote an increased duration of the reproduction period, egg batches and fecundity when the number of females is higher than that of males in a breeding stock/tank. Recently, new technology that allows for in vivo identification of sex and state of maturity was reported by Sykes et al. (2017b), which allows for the proper establishment of sex ratios and assurance of an immature state of the cuttlefish individuals used in reproduction studies. This is a pre-requisite for any studies of reproduction performance involving this due to its capacity of retaining active sperm for months (Hanlon et al., 1999).
The main objective of the present study was to verify the effects of tanks with different bottom areas/volumes on the reproduction performance of S. officinalis breeding stocks, when sex ratios were controlled a priori and established as 2♀:1♂. A secondary objective was to determine the food consumption and cost associated with such performance when individuals are fed a natural frozen diet.
Materials and Methods
Culture Methodology
A total of 192 juvenile cuttlefish with a mean wet weight (MWW) of 32.7 ± 4.0 g were used. All cuttlefish used in the experiment hatched in captivity two and a half months earlier (23/06/2014) from eggs obtained from a F5 captive population. Prior to the start of the experiment, 1000 cuttlefish were reared in group since hatching and were fed live grass shrimp (Palaemonetes varians) ad libitum until this mean weight was attained (Sykes et al., 2014). Both the initial rearing of the animals and the experiment were performed at the Ramalhete Experimental Aquaculture Station (Faro, Portugal – 37°00′22.39″N; 7°58′02.69″W; Figure 1).
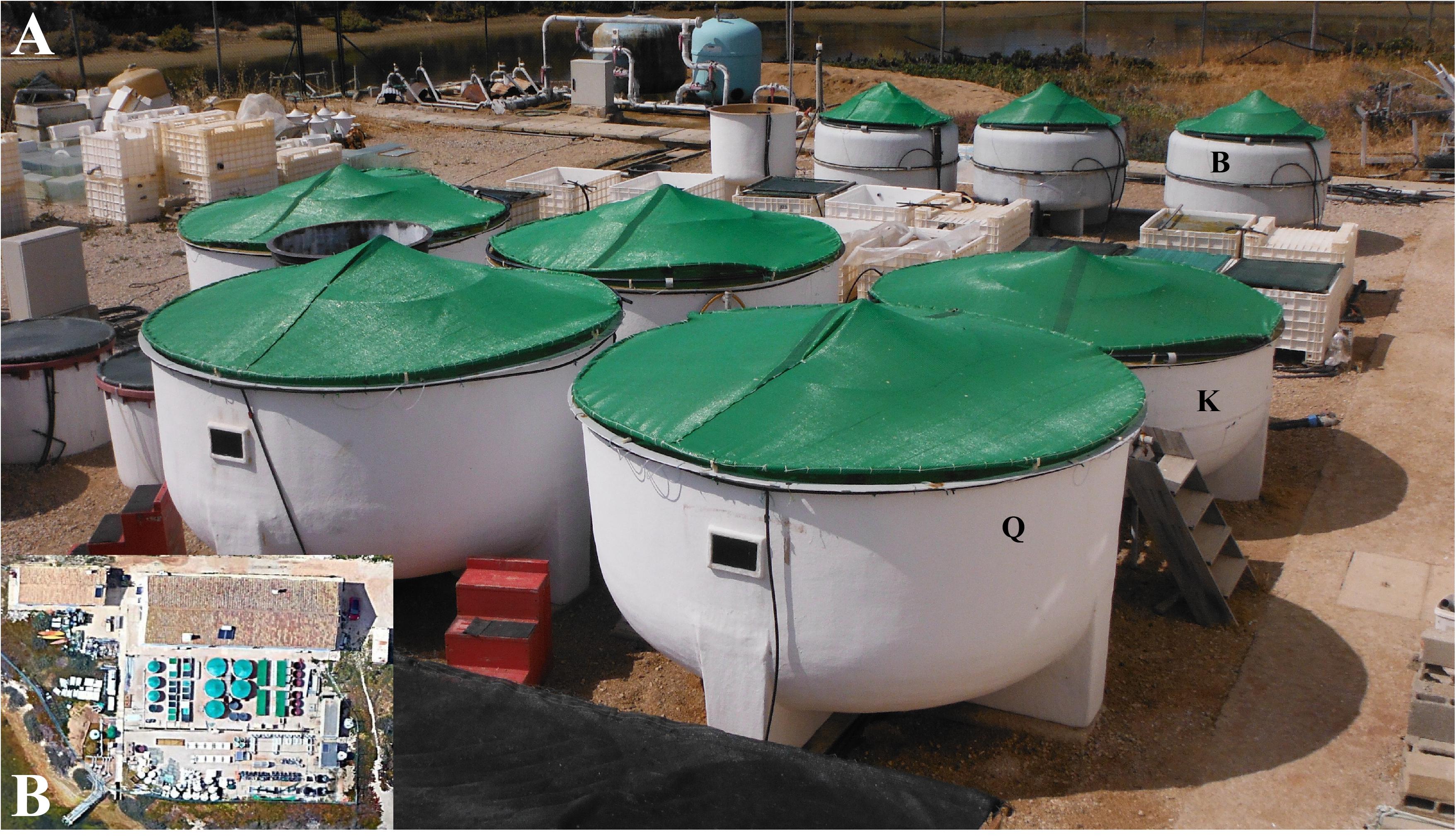
Figure 1. (A) - Experimental tanks of 3000 L (3.24 m2 area; B), 9000 L (7.07 m2 area; K), and 9000 L (6.67 m2 area; Q); (B) Aerial view of the Ramalhete Station, where the experimental tanks can be seen.
A flow-through system with eight tanks (Figure 1) was used: three 3000 L (3.24 m2 area, a height of 1.35 m and a water column of 1.20 m; B), three 9000 L (7.07 m2 area, a height of 1.50 m and a water column of 1.35 m; K), and two 9000 L (6.67 m2 area, a height of 1.70 m and a water column of 1.55 m; Q). The Q 9000 L tanks were the same as used previously by Sykes et al. (2013) and should be considered the control of the experiment. Each tank was set with three airlifts, two air diffusers placed in the middle, and an outer filter (1 cm mesh), high enough to surpass the seawater level of the tank. All tanks were covered with a green plastic net to prevent an excess of incident light (>200 lux). Natural daylight cycle corresponded from autumn to summer conditions for the location (Figure 2). Tank water flow was of 1020 L h–1. Water was pre-filtered through an industrial sand filter (0.3–0.8 mm) and then decanted (30–60 min).
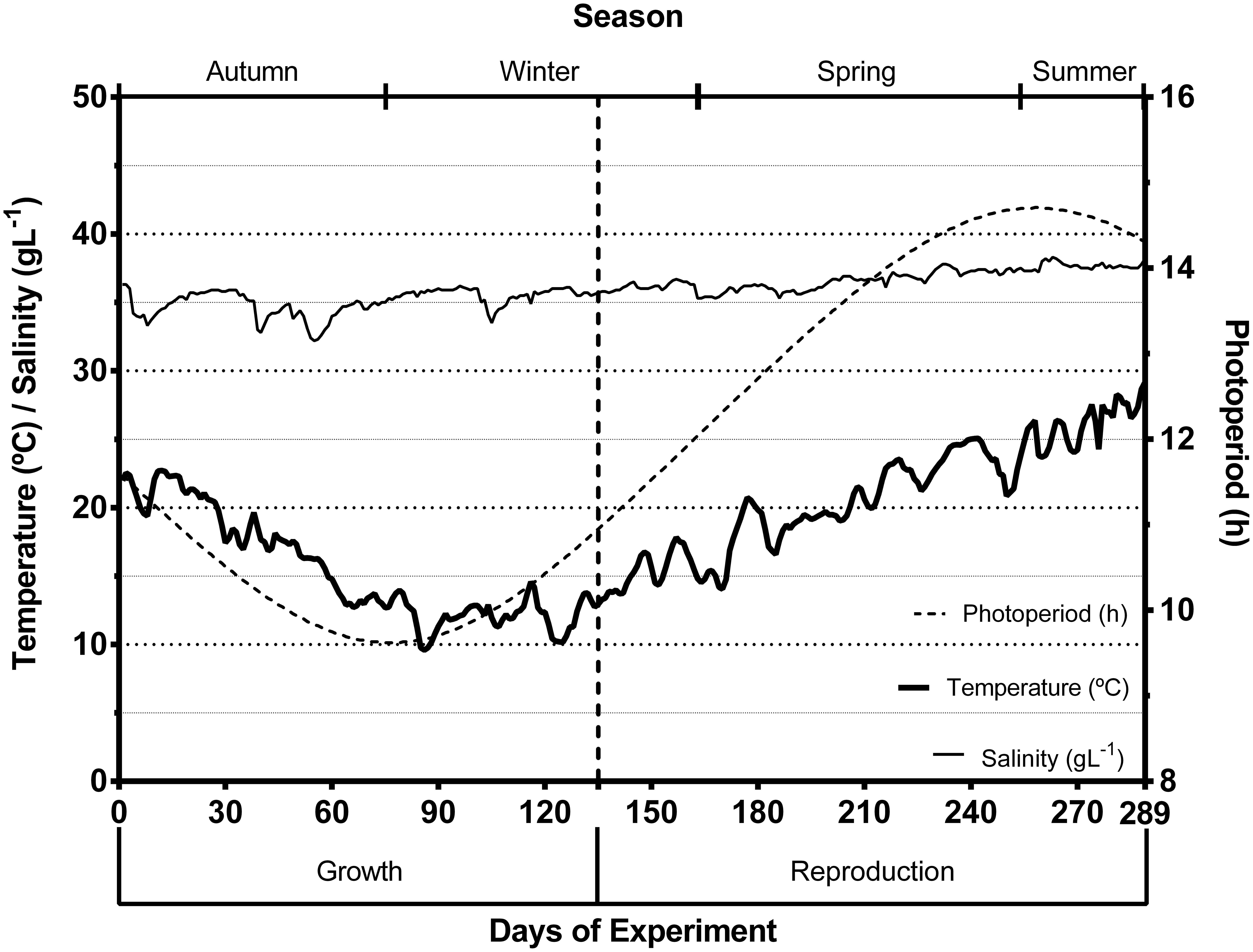
Figure 2. Temperature (°C), salinity (gL– 1) and photoperiod (hours of light) throughout the different stages (bold vertical dash line) and seasons of the breeding stocks of cuttlefish S. officinalis. Temperature and salinity values are a mean of all tanks under study.
At the beginning of the experiment, each replicate/tank was set with 24 juvenile cuttlefish (which corresponded to densities of 7, 3, and 4 cuttlefish m–2, respectively for B’s, K’s and Q’s tanks) and a sex ratio of 2♀:1♂. To attain that, cuttlefish was first anesthetized with magnesium chloride (hexahydrate) at 27 mgL–1 (Gonçalves et al., 2012). Afterward, individuals were weighed in a Kern EW6000 (KERN and SOHN GmbH, Germany). To attain this sexual proportion, females and males sex and state of maturity were determined, DNA samples taken (for comparison in future studies related to reproduction in captivity), and cuttlefish tagged according to the protocols described in Sykes et al. (2017b). Photos of individuals and of marking details were taken at that time for later identification. Animals were randomly placed in given tanks after sexual determination and until the sex ratio and total amount of animals was reached. During both growth and reproduction stages of the experiment, animals were fed daily a quantified ad libitum of thawed grass shrimp to determine the total amount used and costs of feeding each tank.
Data and Sample Collection
Temperature (°C), salinity (g L–1) and dissolved oxygen (%) were measured every day, at 9 h30, in all experimental and egg hatching tanks. Both temperature and dissolved oxygen were measured with an OxiGuard Handy Gamma probe while salinity was measured with a VWR EC300 salinity meter. In addition, a Maxim-Dallas temperature acquisition system network was established by placing one High-Precision 1-Wire Digital Thermometer (DS18S20) in each tank to register temperature every 15 min. These data was used to establish an overall mean temperature evolution throughout the experiment (Figure 2).
All cuttlefish in every tank were weighed, every 15 days, until the start of reproduction. All individuals were carefully removed from the tank with the help of a hand net of proper size (Decathlon Caperlan PRF 4 × 4 240) and placed in 10L black buckets. Afterward, these buckets were moved by hand to the sampling room inside the facilities and cuttlefish was individually weighed. Animals were returned to the original tanks after. Data collected was used to calculate: (1) Mean Wet Weight (MWW; g); (2) Mean Absolute Instantaneous Growth Rate (MAIGR;% BW d–1) = (LnW2−LnW1)/t × 100, where W1 and W2 are the initial and final weight, respectively, Ln the natural logarithm and t the number of days of the experiment; (3) Total Absolute Mortality (TAM) which express all dead animals for the tank volume; (4) Mean Cumulative Mortality (MCM;%) = (Σ dead/initial live animals) × 100; (5) Biomass (Bm; g) = mean Σ of MWW of all cuttlefish for each tank volume; (6) Mean Biomass Relative Increase index (B%;% BW d–1) = [(Bf-Bi)/(Bi × t)] × 100, where Bi and Bf represent the initial and final Biomass, respectively, and t the number of days of the experiment (Sykes et al., 2011); (7) Tank Feeding Rate (FR;% BW d–1) = [FI/Average B(t)] × 100, where FI is the food ingested and average B(t) is the biomass of the cuttlefish of a given tank every 15 days or during the whole growth stage (t); and (8) Tank Food Conversion (FCR) = FI/(Bf−Bi), every 15 days and during the whole growth stage.
Data regarding cuttlefish reproduction was collected and used to calculate: (1) Duration of Growth and Reproduction Stage, the first representing the time from the beginning of the experiment and until the first batch of eggs in each tank (DGS, days) and the second from the end of DGS until the last female died (DRS, days), respectively; (2) Life Cycle Temperature (LCT, °C) temperature for a given tank for the whole experiment; (3) Fecundity (F; eggs) = Total eggs laid per tank; (4) Total Egg Biomass (EB; g) = Σ of egg weight accounted for a given tank; (5) Oocytes (n) = Σ of total number of oocytes in all females gonads after death, per tank; (6) Individual Expected Fecundity (IFep; eggs female–1) = F/number of ♀, set per tank at the beginning of the experiment; (7) Individual Effective Fecundity (IFe; eggs female–1) = F/effective number of ♀ that have reached the reproduction stage per tank; (8) Individual Potential Fecundity (IFp; eggs female–1) = IFe + mean oocytes per female in a given tank; (9) Eggs sampled (n) = amount of eggs sampled per tank; (10) Mean egg weight (MEW; g) per tank; (11) Maximum and Minimum Individual Egg Weight (MaxIEW and MinIEW; g) per tank; (12) Mean female and male weight (MW♀, MW♂; g) at time of death; (13) Female and Male Gonadosomatic index (GI ♀, GI ♂) = Females or males gonads mean weight/MW♀ or MW♂, per tank; (14) Female and Male Digestive Gland Index (DGI ♀, DGI ♂) = Females or males digestive gland mean weight/MW♀ or MW♂, per tank; (15) Mean Female or Male Maturation Stage [MMS ♀, MMS ♂; according to ICES (2010)] = Predominant maturation stage of females or males at death; (16) Sex ratio (♀/♂); (17) Number of Egg Batches (Ba; n) = Σ of batches for a given tank; (18) Number of Eggs per Batch (EBa; eggs batch–1) = Mean number of eggs per number of occurring batches; (19) Viable and non-viable eggs (n) per tank, determined by external morphology and color, according to table 11.2 and fig. 11.3 of Sykes et al. (2014). Eggs that did not present a flask shape and black color were considered non-viable; (20) Hatching Rate (HR;%) = hatching rate of three replicates of 100 eggs, collected from different egg batches in each tank (beginning, middle and end); (21) Mean Hatching Rate (MHR;%) = calculated from mean of HR per tank; (22) Mean Hatchling Weight (MHW; g) = mean weight of hatching cuttlefish from HR.
Sex ratios were verified for confirmation at the end of the experiment. The eggs weight was determined in a KERN 0.001g scale. Eggs were individualized, then placed inside a small tray filled with seawater and weighed one by one. Depending on the amount, a given percentage of a batch was weighed, as follows: 100% of eggs were sampled for batches with up to 100 eggs; 50% of eggs from batches containing up to 300 eggs; 40% of eggs from batches up to 500 eggs; 30% from batches with 500–1000 eggs; and 20% from batches with more than 1000 eggs. Viability of given tanks (HR and MHR) was determined in separated 2.6 L hatching tanks (22.0 cm × 14.5 cm × 8.0cm) of a semi-open seawater system (250 L) with 56 hatching tanks. The embryonic culture conditions used were as described by Sykes et al. (2014).
Data regarding the costs of operation (only food related) was calculated for both growth and reproduction stages as follows: (1) Food Supplied (FS; Kg); (2) Food Cost (FC; €) = FS x price per kg (€5.00); (3) Individual Cuttlefish Cost (ICC; €) = FC/number of cuttlefish in the tank; (4) Tank Cost per Day (TCD; €) = FC/DRS; (5) Egg Cost (EC; €) = FC/F; and (6) Viable Egg Cost (VEC; €) = FC/Viable eggs.
Data Analysis
Results are given as means ± standard deviation (SD) from replicate tanks of a particular tank type, unless stated otherwise. Data was treated in a blind way by assigning non-consecutive numbers to variables and tank treatment before the analysis. All data were tested for both normal distribution and homogeneity of variances using the Shapiro-Wilk test and the Levene’s test (Zar, 1999), respectively. Arcsin square root transformation was applied to all data expressed as percentage (Fowler et al., 1998) and to other not achieving normality and/or homocedasticity. When a normal distribution and/or homogeneity of the variances were not achieved after transformation, non-parametric or robust tests were used, according to the variance similarity criteria (Quinn and Keough, 2002). Statistical difference was considered for p < 0.05.
Data analysis was performed considering the division of the experiment into two stages: growth and reproduction. At the beginning of the experiment, every cuttlefish was individually weighed and a Nested ANOVA test (Zar, 1999) was performed to assure that no significant differences (p < 0.05; data not shown) were found in MWW among all tanks.
One-way ANOVA was used to determine differences in LCT, MEW, MW♀, MW♂, and MHW between tanks. Nested ANOVA (Zar, 1999) was used to determine differences in temperature, salinity, dissolved oxygen saturation, and MWW between tank types. Kruskal-Wallis ANOVA (Zar, 1999) was used to determined differences in MCM, DGS, GI♀, and DGI♂. Welch Robust ANOVA tests (Quinn and Keough, 2002) were performed to determine differences in MAIGR, Bm, B%, FR, FCR, FS, FC, ICC, TCD, DRS, F, EB, Oocytes, IFep, IFe, IFp, MaxIEW, MinIEW, GI♀, DGI♂, Ba, EBa, viable eggs, non-viable eggs, MHR, FS, FC, ICC, TCD, EC, and VEC; between different tanks types. When differences were found, Tukey post hoc tests (Zar, 1999) were used to determine multiple comparisons between tank type or individual tanks, depending on the factor chosen to analyze data.
Results
Statistical tables that support the results are available as Supplementary Material.
Water Quality
The experiment lasted up to 289 days, where seawater parameters and photoperiod varied according to given local environmental conditions (Figure 2). Salinity and dissolved oxygen saturation present similar fluctuations between tanks throughout the whole experiment (p > 0.05; Table 1). As regards temperature, B and Q tanks displayed a higher mean temperature throughout the growth stage, while K and Q displayed the highest mean temperature during the reproduction stage (p < 0.05; Table 1).
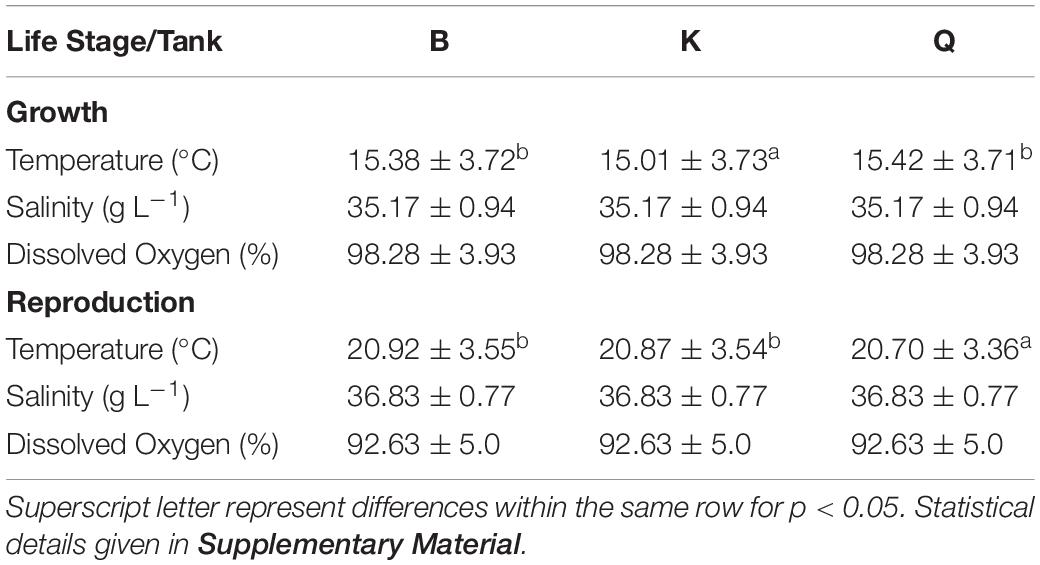
Table 1. Mean water temperature, salinity and dissolved oxygen registered during the growth and reproduction stages of cuttlefish life cycle.
Growth
Table 2 presents the biological and economical results obtained at the end of the growth stage. Cuttlefish reared in tanks K and Q displayed a higher MWW than those stocked in the B tanks (Figure 3; p < 0.05). This difference was not, however, reflected in MAIGR, Bm or B%, whose values were similar amongst tank types (Figures 3, 4; p > 0.05). Growth and biomass of all tank types was fitted to a second and third order polynomial regression, respectively (Figure 3). Despite the differences verified between tank types in the overall amount of cuttlefish that died (TAM), no differences in MCM were found among tank types (Figure 5; p > 0.05). This was due to the mortality expressed in given tanks, namely in one replicate of the B tanks, which lead to the high standard deviation. No differences were also shown in FR, FCR or FS (Table 2; Figure 6; p > 0.05). At the end of this stage, from the original 72 animals of the B and K tanks, only 53 (5, 13 and 1 dead cuttlefish in B1, B2, and B3, respectively) and 69 (2 and 1 dead cuttlefish in K2 and K3, respectively) animals were, respectively, available to contribute to reproduction; whereas all cuttlefish were available in the Q tanks.
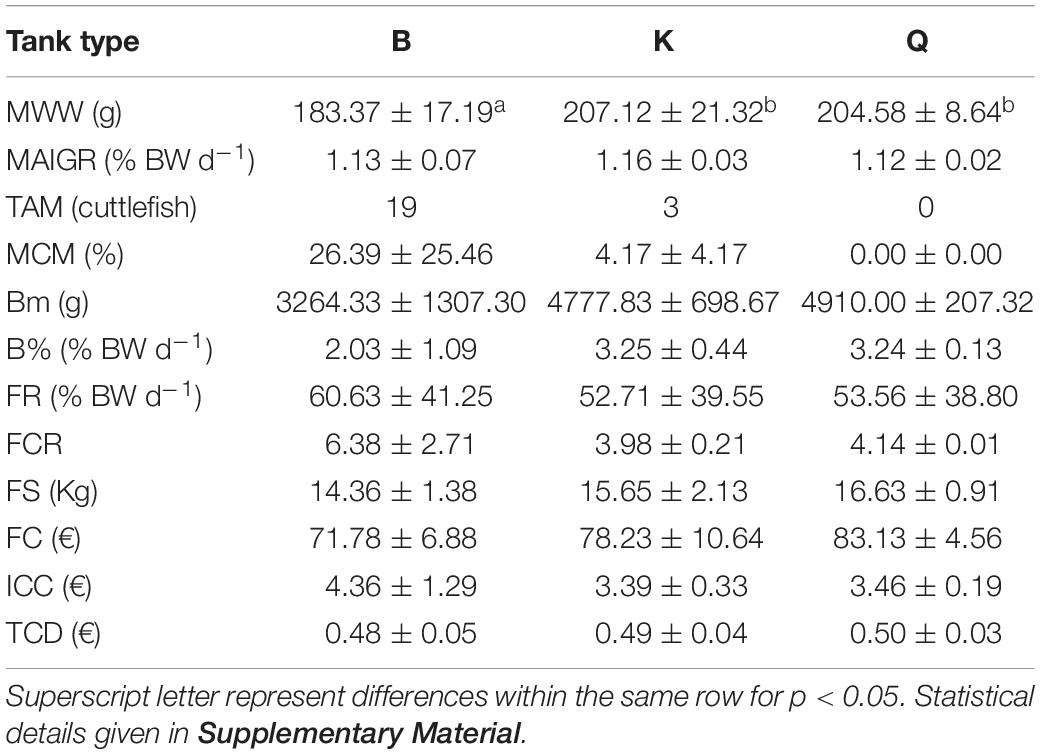
Table 2. Mean Wet Weight (MWW), Mean Absolute IGR (MAIGR), Total Absolute Mortality (TAM), Mean Cumulative Mortality (MCM), Biomass (Bm), Mean Biomass Increase (B%), Tank Feeding Rate (FR), Food Conversion Rate (FCR), Food Supplied (FS), Food Cost per Tank type (FC), Individual Cuttlefish Cost per Tank type (ICC) and Tank type Cost per Day (TCD) values for cuttlefish at the end of growth stage.
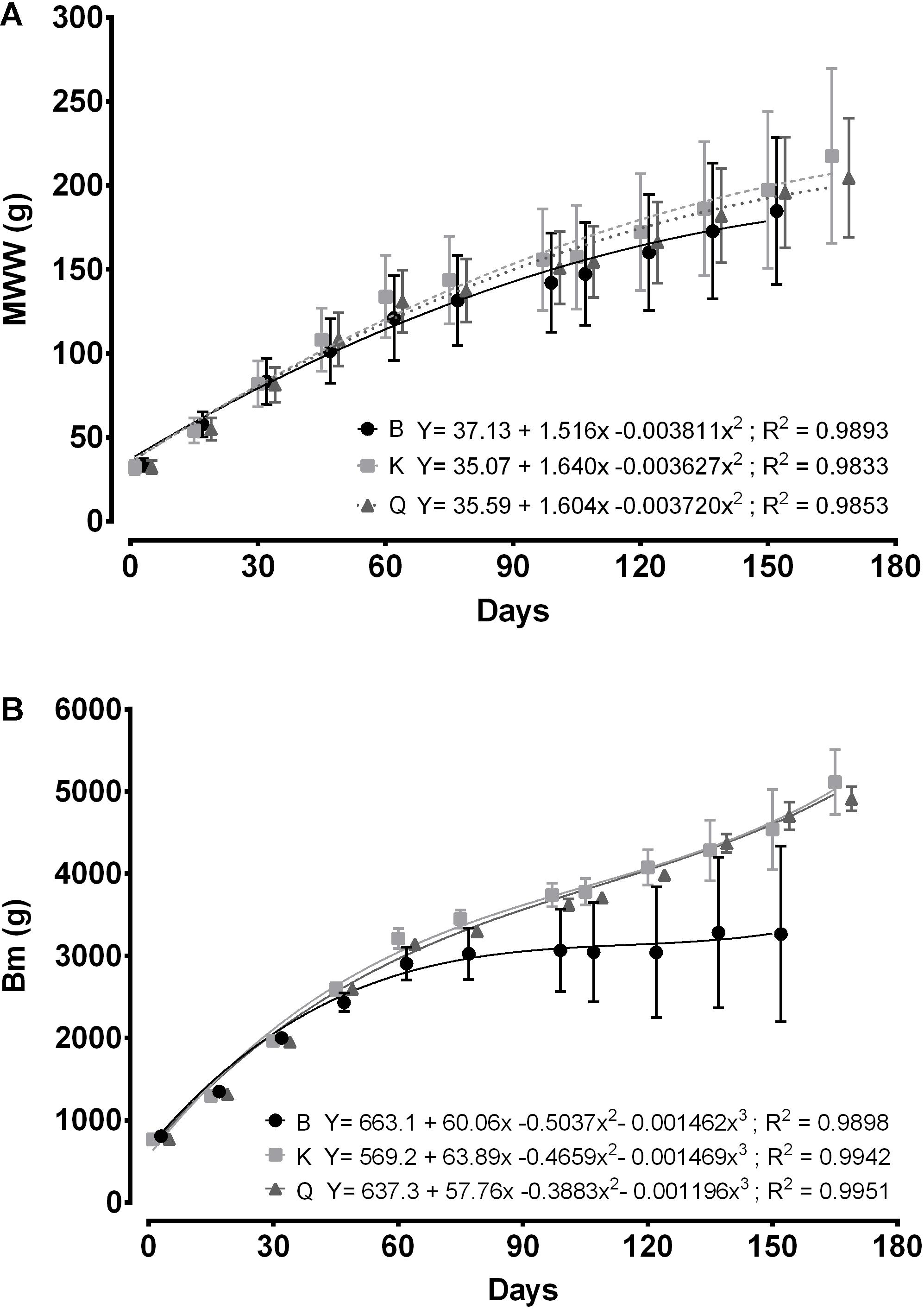
Figure 3. Mean wet weight (g; A) and biomass (g; B) of cuttlefish breeders stocked in different tank types during the growth stage. Data are presented in offset. Bars represent standard deviation.
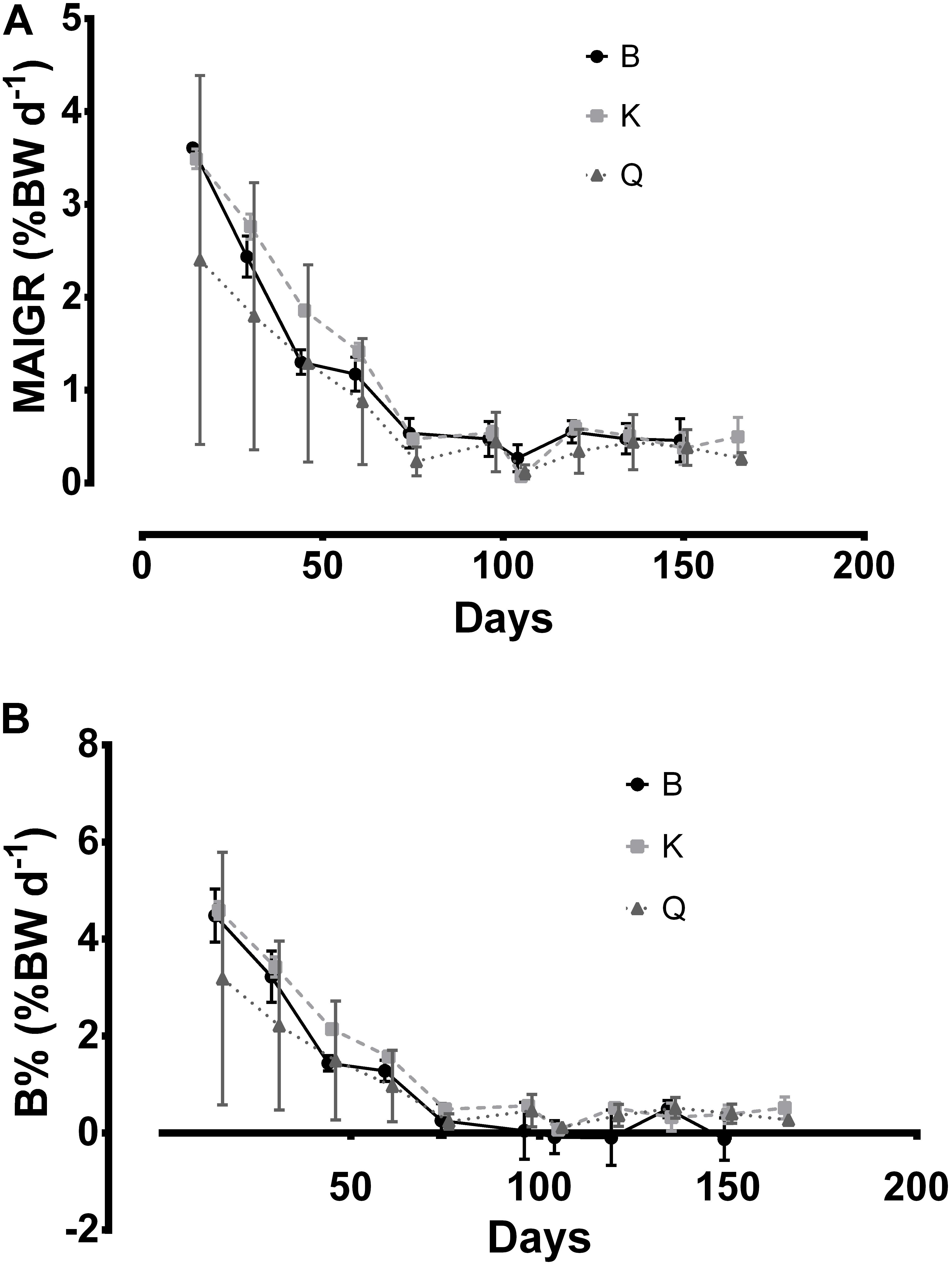
Figure 4. Mean absolute instantaneous growth rate (MAIGR,%BW d– 1; A) and mean biomass relative increase index (B%,%BW d– 1; B) values for cuttlefish breeders reared in different tank types during the growth stage. Data are presented in offset. Bars represent standard deviation.
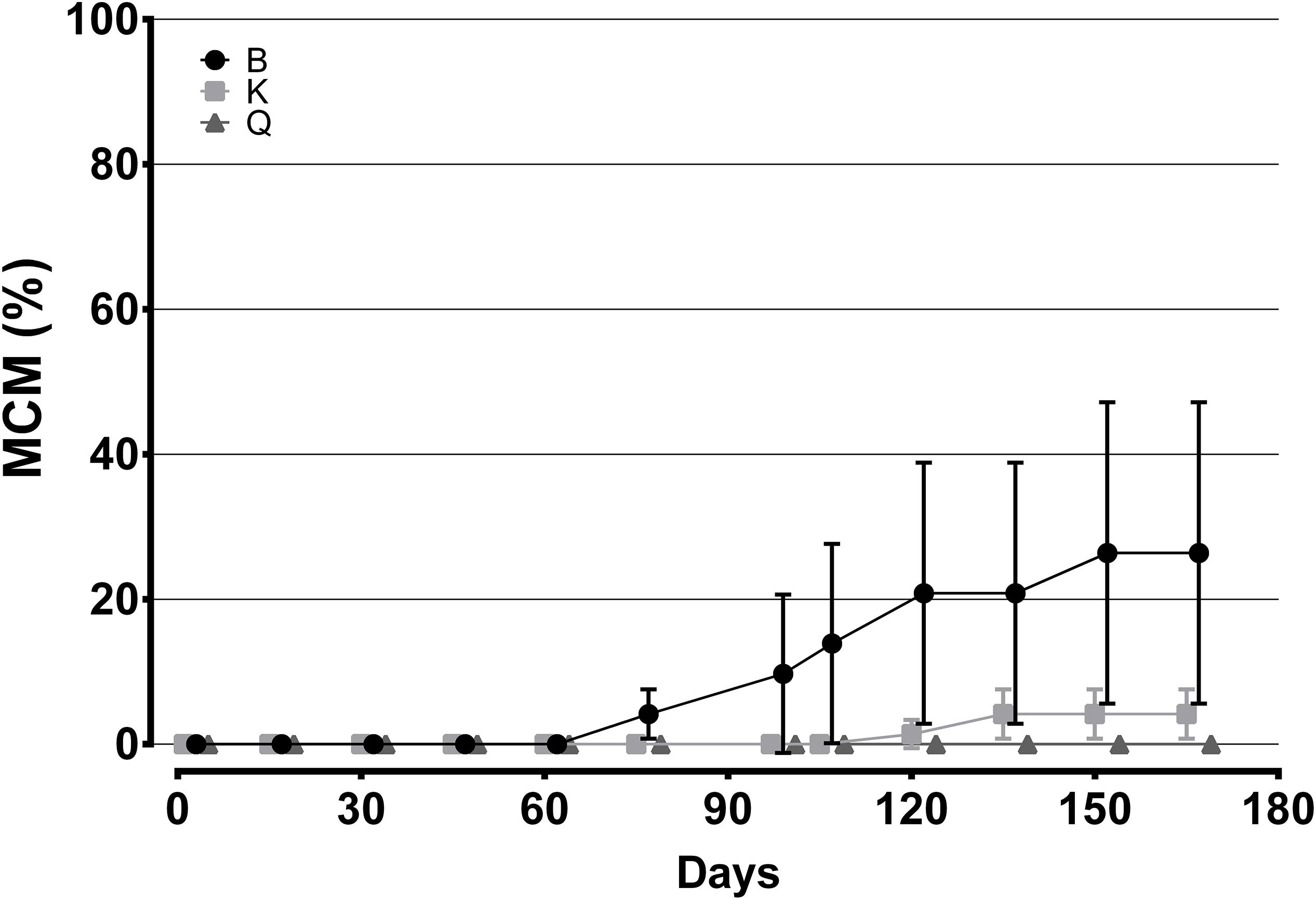
Figure 5. Variation in mean cumulative mortality (MCM,%) for cuttlefish reared in different tank types during the growth stage. Data are presented in offset. Bars represent standard deviation.
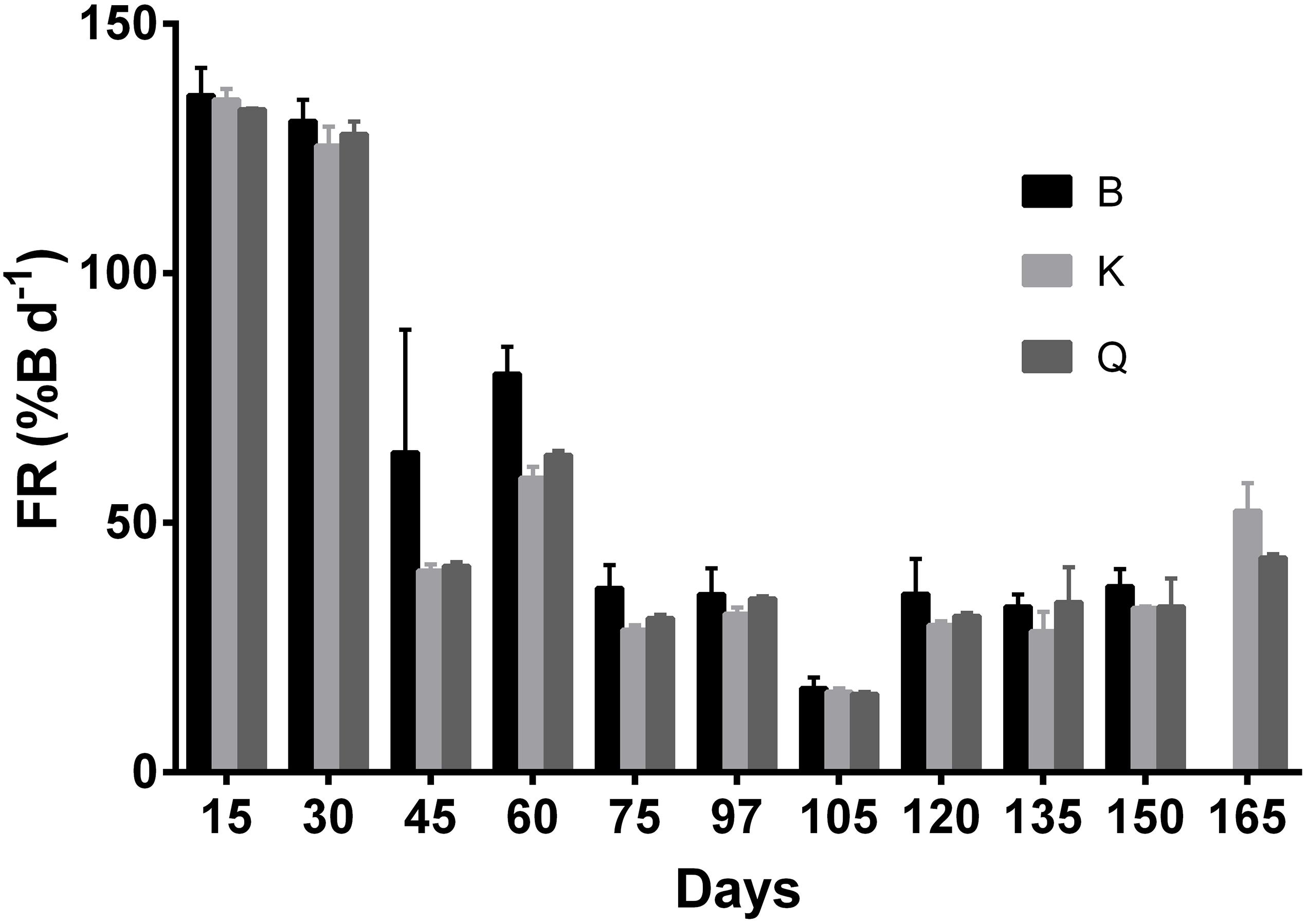
Figure 6. Tank Feeding Rate (FR,% BW d– 1) values for cuttlefish reared in different tank types during the growth stage. Data are presented in offset. Vertical bars represent standard deviation.
Reproduction
Table 3 shows both the biological and economical results obtained for the reproduction stage. The duration of reproduction had a lower time span when compared with the duration of the growth stage. Lifecycle temperature (LCT) depended not only of the values registered in each tank but also on the duration of the experiment in each tank (dependent on the last female to die). Differences in LCT were found between tanks, being the highest mean registered in Q1 and the lowest in K1 (p < 0.05). Fecundity was the highest in two K type tanks (K2 and K3) with a value of ≈ 24000 eggs in each tank and the lowest in one of the B type tanks (B2) with only 369 eggs. The EB showed similar results, with the highest biomasses recorded in K type tanks and the lowest in one B type tank. No differences were found in the amount of oocytes found in each tank nor in IFep, IFe and IFp (p > 0.05). The MEW was different between tanks, with the highest value registered in B2 and the lowest in B3, K1, K2, and K3 (p < 0.05), and minimum and maximum individual egg weights (MinIEW and MaxIEW) varied between 0.137 and 1.138 g registered in K3 and Q1, respectively, but no difference was found between tank types (p < 0.05). The mean weight of the breeders and GI only showed differences between tanks regarding females (MW ♀ and GI ♂; p < 0.05). Most individuals in every tank reached a 3A maturity except for the females in tank B2, where only 25% had attained that degree of maturity at time of death. The number of egg batches (Ba) (Figure 7), eggs per batch (EBa), viable and non-viable eggs (Figures 8, 9) was similar between tank types (p > 0.05).
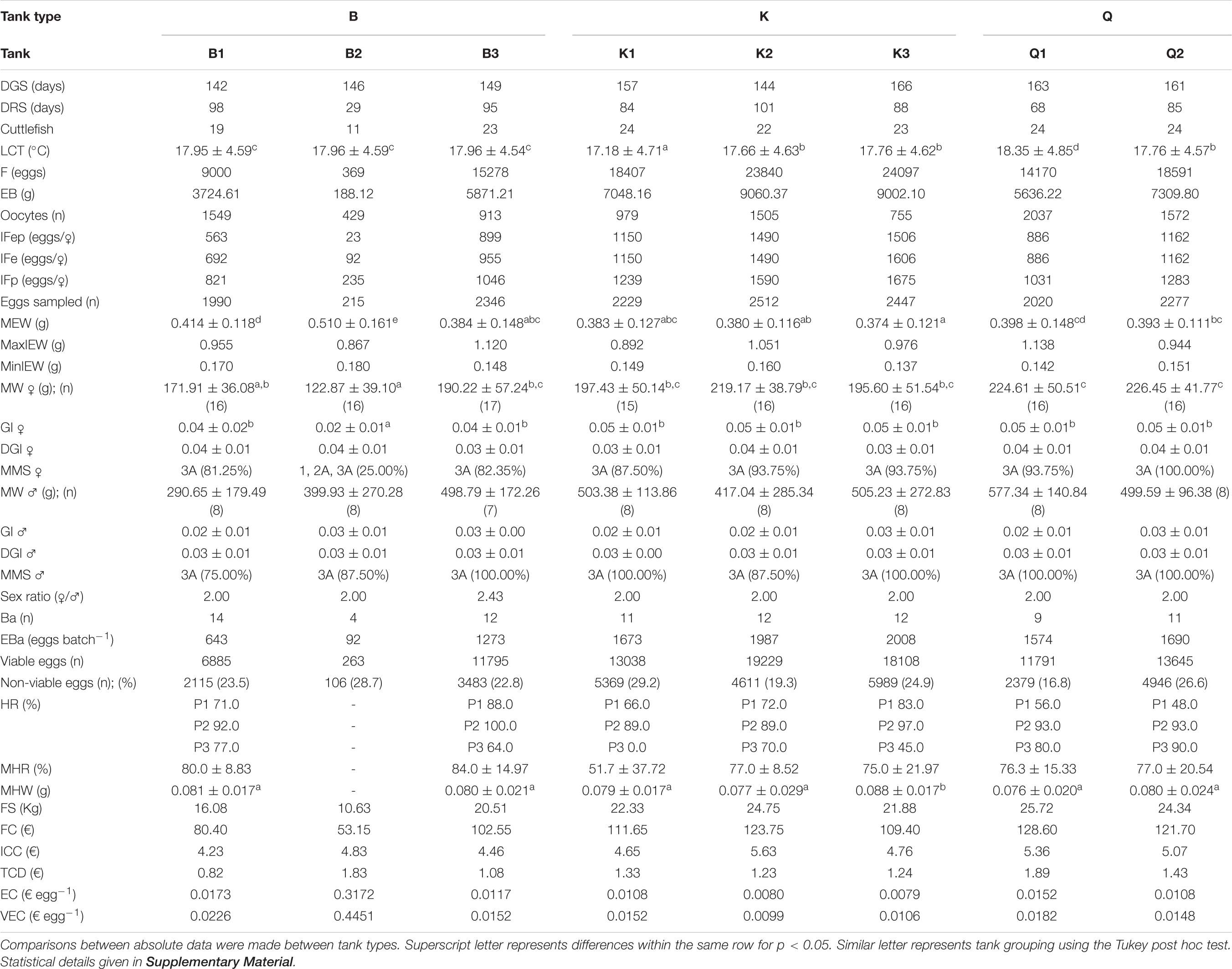
Table 3. Duration of Growth and Reproduction Stages (DGS and DRS), Cuttlefish (available animals at the start of the reproduction stage), Life Cycle Temperature (LCT), Fecundity (F), Egg Biomass (EB), Oocytes, Individual Expected Fecundity (IFep), Individual Effective Fecundity (IFe), Individual Potential Fecundity (IFp), Eggs Sampled, Mean Egg Weight (MEW), Maximum and Minimum Individual Egg Weight (MaxIEW and MinIEW, respectively), Mean Female and Male Weight (MW ♀ and MW ♂, respectively), Female and Male Gonadosomatic index (GI ♀ and GI ♂, respectively), Female and Male Digestive Gland Index (DGI ♀ and DGI ♂, respectively), Mean Female and Male Maturation Stage [MMS ♀ and MMS ♂, respectively; according to ICES (2010)], Sex Ratio, Number of Batches (Ba), Eggs per Batch (EBa), Viable and Non-viable Eggs, Hatching Rate (HR), Mean Hatching Rate (MHR), Mean Hatchling Weight (MHW), Food Supplied (FS), Food Cost per tank (FC), Individual Cuttlefish Cost per tank (ICC), Tank Cost per Day (TCD), Egg Cost (EC) values and Viable Egg Cost (VEC); for cuttlefish at the end of reproduction stage.
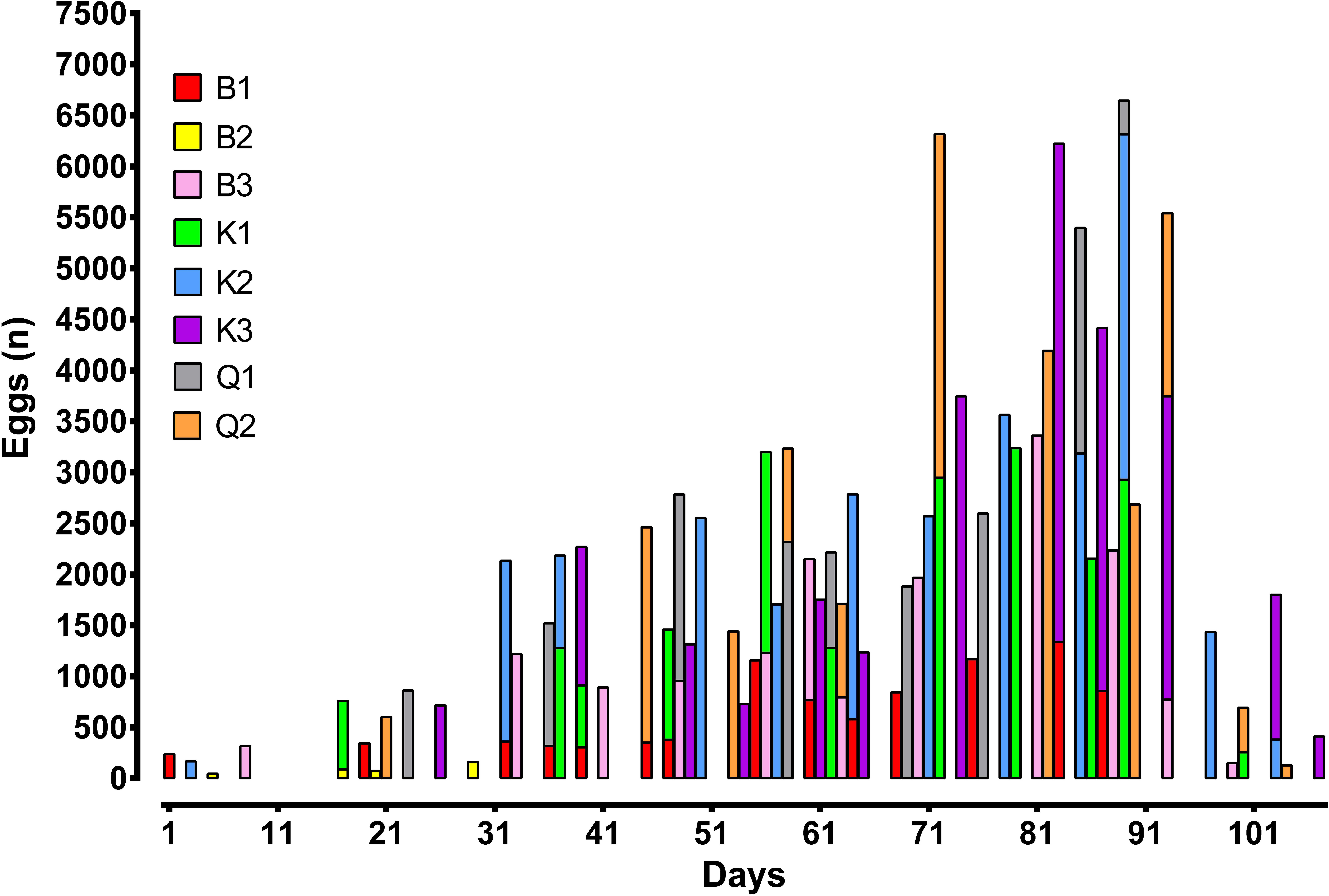
Figure 7. Number of eggs obtained in each batch and in each tank during the reproduction stage of experiment.
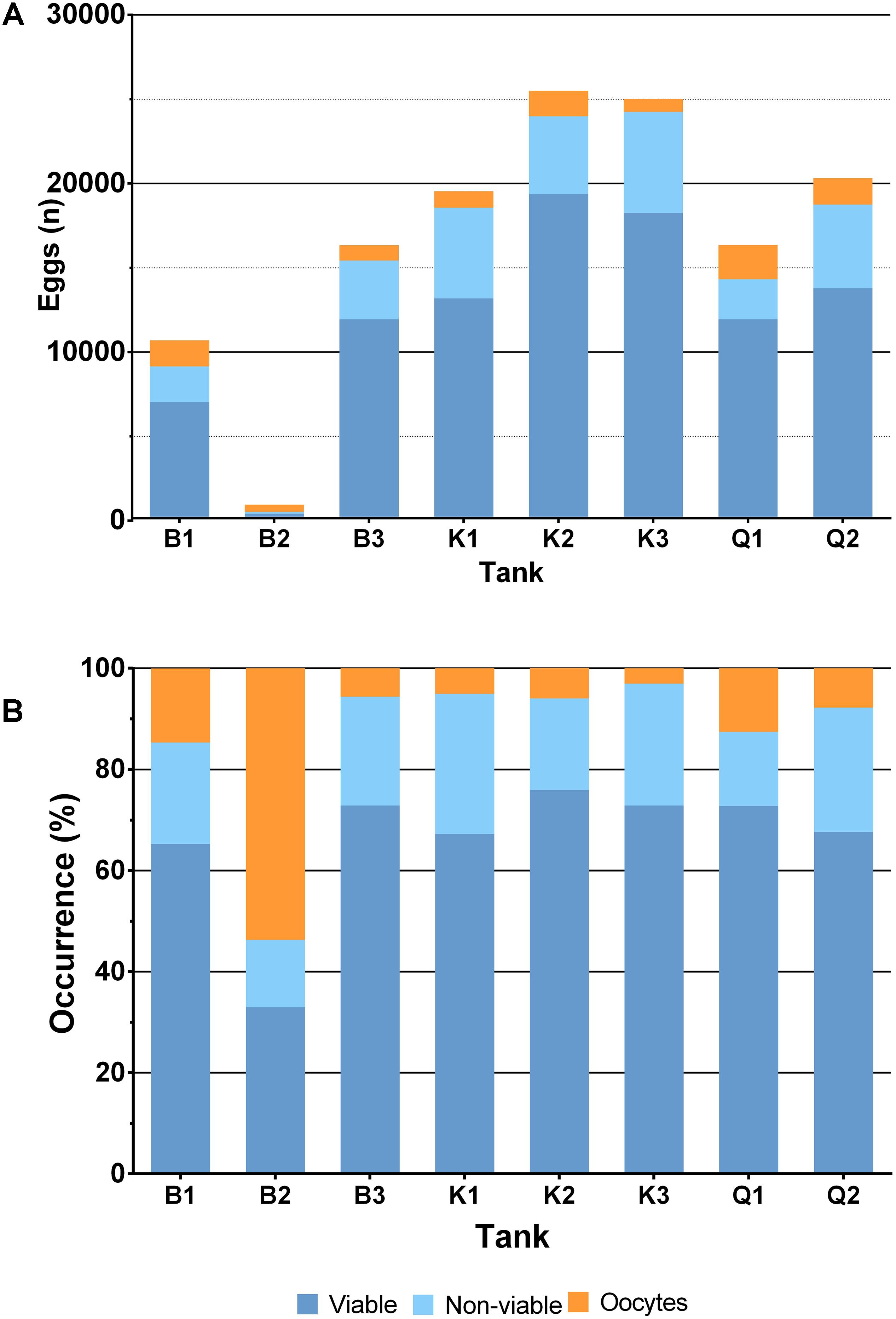
Figure 8. Total amount (A) and percentage of occurrence (B) of eggs (viable and non-viable) plus oocytes (observed in post mortem females) obtained per tank.
Since cuttlefish from tank B2 cuttlefish never laid a batch with at least 100 eggs, no samples for HR were collected nor determined. Despite MHR did not varied between tanks, MHW was higher in K3 when compared with the remaining (p < 0.05).
Food Cost of Breeding Stocks
During the growth stage, all tanks displayed similar costs per tank type (FC) and for individual reared cuttlefish (ICC), which translated in similar individual daily costs (TCD) (p > 0.05; Table 2) of ≈ € 77, € 3.77 and € 0.5 d–1, respectively. Likewise, no differences were verified during the reproduction stage regarding FC, ICC, and TCD between tank types (≈ € 104, € 4.87 and € 1.36 d–1, respectively; p > 0.05; Table 3). EC and VEC also showed no differences between tank types (p > 0.05; Table 3) being of ≈ 0.499 and 0.0689 € egg–1, respectively.
Discussion
Water Quality
Temperature has a profound effect on growth rate, particularly during the juvenile stage (Forsythe, 1993; Sykes et al., 2006a), which corresponds in the present study to the growth stage. In this sense temperature was verified continuously during both stages of the present experiment. Both B and Q tanks presented marginal higher mean temperatures throughout the growth stage (Table 1), which could be explained by the location of the tanks in the facilities (Figure 1). These tanks were more exposed to sun during autumn and winter than the K tanks. On the other hand, the K and B tanks displayed marginal higher mean temperatures than the Q tanks during spring and summer. While in the first, this was probably due to the higher area of exposure to sun in terms of radius and water column, in the second this was for surely due to the lower volume (3000 L). Nonetheless, the differences found in temperature and between tanks were lower than 1°C and they should have not had an effect in the present results.
Growth
The different tanks setups influenced this part of the life stage of cuttlefish development. The main differences were found in MWW but the observed mortality should not be disregarded (Table 2). MWW was higher in tanks with 9000L volume and higher area when compared with 3000L, but no difference was seen regarding the effects of available bottom area in the 9000L tanks.
Cephalopod growth is highly variable and influenced by biotic and abiotic factors such as temperature, diet, age, gender and level of maturity (Forsythe, 1984, 1993; Forsythe and Van Heukelem, 1987; Forsythe and Hanlon, 1988). Individual growth variation can impact many facets of a cephalopod population including size and age structure, reproductive dynamics, and survival rate of hatchlings (Leporati et al., 2007).
Sykes et al. (2013) used the same 9000 L Q tanks plus another two smaller tanks of 750 L and 250 L for rearing 23 cuttlefish juveniles of ≈ 45 g, at a similar temperature, and found no effects of volume in MWW. Interestingly, the growth stage increased from a mean of 105 days of that study to a mean of 150 days observed in the current study. Moreover, despite the animals used in the present study had a lower weight at the beginning (≈ 30 g), they displayed almost the double weight and tank biomass at time of maturation when compared with the previous study (≈ 110 g and ≈ 2500 g vs. ≈ 200 g and ≈ 5000 g, respectively). These data plus the lower mortality observed in higher volumes verify the assumption drawn by these authors that cuttlefish reproduction in captivity should be performed in higher volume tanks. However, since there were no differences regarding Bm and MCM of different tanks types, it cannot be concluded that “cuttlefish, in space stressful conditions, might have the ability to adjust the amount of biomass/animals present in each tank by competition,” as previously suggested by these authors. Despite the differences verified between tank types in the overall amount of cuttlefish that died (TAM), no differences in MCM were found among tank types (p > 0.05). This was due to the mortality expressed in given tanks, namely in one replicate of the B tanks, which lead to the high standard deviation of both Bm and MCM (Figures 3, 6, respectively).
Domingues and Marquez (2010) observed that when cuttlefish juveniles of ≈ 10 g were cultured at similar bottom areas (3 m2), but considerably different culture densities (16 and 33 cuttlefish m2), growth was affected by the culture density, but survival was the same in the two treatments (96%). This result indicates that bottom areas are clearly vital for the proper growth of this species in captivity and that the present density of 24 cuttlefish breeders per tank is suitable. On the other hand, considering a retrospective severity analysis (Smith D. et al., 2018), the non-natural mortality observed during the growth stage raises issues related to the welfare of cuttlefish in this kind of studies. Thus, despite Directive 2010/63/EU provide exemption for studies carried out for veterinary and zootechnical purposes, we believe that the cephalopod community should consider prospective severity assessment analysis to be carried out in all instances (Smith D. et al., 2018; Cooke et al., 2019) and the application of the PREPARE guidelines (Smith A.J. et al., 2018).
Although MAIGR was similar between the three tank types (Figure 4), it was a bit higher than that reported by Sykes et al. (2013). The values of biomass per tank (Bm) attained in the present study almost duplicate for the 9000 L tanks, when compared with that previous study; with values of B% of the present study surpassing in 2-fold those of the previous.
The optimization on the rearing protocol of cuttlefish breeders has allowed for enhanced values of FR and FCR in the present study comparatively with those reported by Domingues and Marquez (2010).
In a general way, the optimization of rearing conditions (increased volumes and bottom areas) has allowed for higher growth (MWW) and a higher number of cuttlefish available for breeding.
Reproduction
According to Sykes et al. (2017b), sexual discrimination and maturity stage determination in cuttlefish used for reproduction performance studies should be performed through an endoscopy internal examination of the animals. This methodology was applied in the present study, being highly effective (only failed in one tank and displayed an overall accuracy of 99%, similar to that reported by these authors), and allowing for the proper establishment of the sex ratio of 2♀:1♂.
The K tank type tanks showed the best overall reproduction performance results and consistency between replicate tanks.
The first reports by Forsythe et al. (1994) on the species life duration in captivity and in a recirculating seawater system indicated a life span of up to nearly 18 months in six consecutive generations. More recently, Sykes et al. (2006a) reported smaller life spans in several consecutive generations cultured in captivity and a possible relation of this smaller life cycle with seawater temperature. Interestingly, the values of duration of the reproduction stage (DRS) could not be related with the increasing tank volumes (Sykes et al., 2013), nor with different sizes of bottom areas (Sykes et al., 2017b), or both (the present paper data). In fact, all the DRS of the present experiment were smaller than the ≈ 150 days reported by Boletzky (1987). On the other hand, despite the lower average weight of the cuttlefish used in the present study, when compared with that of the Sykes et al. (2013) and Sykes et al. (2017b) experiments (≈ 33g in the first when compared with ≈ 46 g in the remaining two studies; F1 and F3 captive generations, respectively), a higher mean duration of the growth stage (DGS) was observed in the present study (≈ 150 days vs. 105 days and 23 days, respectively). Unfortunately, we only have records of cuttlefish age prior to their use in the present experiment (75 days after hatching) and different values might related to the age of animals at the start of each study. Nevertheless, considering only the 9000L volume tanks in all studies, the overall life span as part of the breeding stock (sum of both DGS and DRS days) was 169, 175, and 243 days, respectively for the Sykes et al. (2013, 2017b), and the present study.
Cuttlefish displayed intermittent spawning (Boletzky, 1987; Rocha et al., 2001), which is considered to be a normal process either in captive or wild conditions (Laptikhovsky et al., 2003), where females laid more than one egg batch and probably had successive egg maturation cycles. Older reports (Boletzky, 1983; Forsythe et al., 1991) describe the egg-laying as a typically lengthy process in cuttlefish, where most females produce eggs (200–500 eggs/♀) over a 2–4 week period and occasionally up to 10 weeks while continuing to feed daily. More recently, Laptikhovsky et al. (2003) reported that S. officinalis individual potential fecundity (IFp) can reach nearly 6000 oocytes in the wild, but individual effective fecundity (IFe) is lower by 1000–3000 eggs. Forsythe et al. (1994) and Hanley et al. (1998) reported values of IFe up to 3000 eggs in captivity (F3 generation for the Forsythe et al. study and no generation reported in the Hanley et al. study). Interestingly, the best overall and IFe obtained by Sykes et al. (2017b) was in a K tank (≈ 1800 eggs/♀ and ≈ 14,500 eggs tank–1; F3 generation), where DRS was 224 days (the highest of that study) contrasts with the previous values of fecundity (≈ 1400 eggs/♀ and ≈ 16,600 eggs tank–1; F1 generation) reported by Sykes et al. (2013). On the other hand, the highest overall and IFe of the present study (≈ 1600 eggs/♀ and ≈ 24,000 eggs tank–1) was also observed in two K tanks, where the mean DRS was only 95 days. Moreover, the values of both DGS and DRS were inverted in magnitude and sex ratios were also different (1:1 in the previous study with a F3 generation). While the individual fecundity did not surpass the previous maximum values of Sykes et al. (2017b), the overall tank production almost doubled to pre-industrial numbers. Furthermore, for the first time, these values were similar within replicates of the K tanks and the prospective egg viability and mean hatching rate (MHR) was the highest attained until now (78 and 75%, respectively). The mean weight of eggs and hatchlings as well of minimum and maximum individual egg weights (MEW, MinIEW, MaxIEW, and MHW, respectively) were similar to those reported previously (Sykes et al., 2006a, 2013) and differences are related to the size/weight of females - larger females lay larger eggs (Boletzky, 1983). Both mean female and male maturation stages (MMS♀ and MMS♂, respectively) results of the present study were similar to those observed previously by Sykes et al. (2017b).
When comparing the weights of both mature female and male cuttlefish (MW♀ and MW♂, respectively) of the tanks with the best reproduction performance at time of death in the present study with that of previous studies (Sykes et al., 2013, 2017b) (namely, individuals from tanks K2 and K3, T8 (which is Q2 in the present study), and K2, respectively; F1 and F3 generations, respectively), the weight ratio (MW♀/MW♂) increased from the first study to the present one (1.31, 1.67, and 2.25, respectively).
The changes applied to the breeders protocol in the present study, compared to those previously reported (Sykes et al., 2013, 2017b), promoted a lower amount of egg batches (Ba; 12, 23, and 40, respectively) and an increased number of eggs per batch (EBa; ≈ 2000, ≈ 700, and ≈ 360 eggs Ba–1) in the tanks that displayed the highest reproduction performance (namely, K2 and K3, T8 (which is Q2 in the present study), and K2, chronologically for the three studies). The laying of egg batches increased from one day in the mentioned previous two studies (Table 4 and Table 3, respectively) to several days in the present one. Moreover, several pairs of cuttlefish were seen repeatedly copulating and laying eggs at the same time.
Both gonadossomatic and digestive gland indexes (GI and DGI, respectively) of ♀ of the K2 and K3 (present study) displayed approximately half the values reported by Sykes et al. (2017b) (Table 3 in both manuscripts), reflecting the higher investment made by the females in the production of gametes. On the other hand, the slightly lower GI and DGI of ♂ verified in the present study might reflect the increased copulation activity by males due to the increased sex ratios/availability of females.
Estimation of Food Cost of Breeding Stocks
To the best of our knowledge, this is the first time ever that a study involving the development of breeding protocols in cephalopods reported the expense value in terms of food. The amount of food supplied (FS) to cuttlefish during the growth stage was similar among tanks (≈ 15 kg) for ≈ 150 days, which translated in a cost of ≈ 77 € tank–1, 3.77 € cuttlefish–1, and an overall daily tank expense of € 0.5 d–1 (Table 2).
In the reproduction stage, FS was similar among the B3, K and Q tanks (≈ 24 kg) for ≈ 85 days, but different from B1 and B2 (≈ 14 kg) for the same experimental period (Table 3). This may be explained by the difference in cuttlefish available for breeding purposes when reaching maturity in the latter tanks. In these two tanks, the FC reached ≈ 67 € tank–1, 4.53 € cuttlefish–1 and a daily tank expense of € 1.33 d–1. On the other hand, the remaining tanks displayed a FC of ≈ 116 € tank–1, 4.99 € cuttlefish–1 and a daily tank expense of € 1.37 d–1. The individual cuttlefish cost per tank (ICC) was similar among different tank types, as well as the tank cost per day (TCD). Interestingly, both ICC and TCD displayed similar values concerning the costs during growth and reproduction, but FC was higher in the reproduction stage. This is justified by the increase of the cuttlefish biomass when entering this stage (Table 2).
Despite the higher variability found in the values of egg cost (EC) and viable egg cost (VEC), which led to the lack of statistical differences between tank types, both K2 and K3 tanks displayed the lowest VEC (Table 3). K type tanks were the more productive with an EC of ≈ 0.0089 € egg–1 and a VEC of ≈ 0.0119 € egg–1. On the other hand, the Q tank type had increased values of EC and VEC (≈ 0.0130 € egg–1 and ≈ 0.0165 € egg–1, respectively; Table 3).
Overall, considering the better reproduction performance of the K type tanks throughout the growth and reproduction stages (all the experimental life cycle), it can be estimated that 3 tanks have a total consumption of ≈ 38.64 Kg tank–1 for ≈ 247 days, which translates in an investment in feed of ≈ 193 € tank–1, 8.40 € cuttlefish–1 and an overall daily tank expense of 1.76 € d–1.
Importance and Applicability for Cephalopod and Finfish Aquaculture
The zootechnical changes applied to the protocol of the breeding stock of S. officinalis reared in the K type tanks have promoted overall better welfare conditions that led to the availability of a proper amount of animals for copula and a higher emission of gametes after maturation was reached. The present results confirmed that cuttlefish will spawn spontaneous and increases reproduction performance in large tanks (9000L) and low stocking densities (3 cuttlefish m–2), in a similar fashion to what is described in literature for finfish (Okumura et al., 2003; Buchet et al., 2008; Mylonas et al., 2010). However, we have verified that having a sex ratio of 2♀:1♂ in these conditions will increase reproduction performance to pre-industrial levels.
The present results show that the reported bottleneck concerning control over reproduction in captivity (Sykes et al., 2014; Villanueva et al., 2014) was surpassed as reproduction performance results of the K tank type tanks were for the first time consistent in terms of replication Moreover, values of fecundity and fertility surpassed those reported by Forsythe et al. (1994) for a F5 generation and were similar to those obtained previously in a F1 population (eggs collected from the wild and individuals cultured in captivity through the life cycle) cultured in a Q tank (Sykes et al., 2013).
More space/volume for housing the breeding stock promoted a more natural environment and better reproduction performance, with values nearer to those estimated to happen in nature. This was attained just by increasing welfare conditions under a captive environment.
Despite that one of the B tanks contributed with only 369 eggs, the eight tanks generated a total of 123,751 eggs (in 85 batches), which is not only the highest number of eggs ever obtained at CCMAR’s facilities but also a number of eggs that may meet the requirements of a small scale cuttlefish commercial hatchery facility. We must emphasize that these results were obtained in a F5 generation of captive cuttlefish breeders and that the experimental tanks were placed outside the premises (less control and more natural/wild type). Overall, the present conditions contributed to a better and predictable reproduction performance in specific tanks (K), with values reaching pre-industrial numbers (≈ 24,000 eggs tank–1). Moreover, both the amount of eggs per batch and the overall quality of eggs has increased. Batches were laid in about 100 days, which allows for not releasing a high amount of cuttlefish in to the market at the same time, therefore allowing for maintaining the whole sale price. The present results should be considered a 3Rs refinement to the current existing protocol for cuttlefish reproduction. In this sense, we must stress that any studies on cuttlefish reproduction should be carried using similar conditions of round-shape tanks with a volume equal or higher to 9000L.
There was an interesting result that may point to an optimization of the species breeding protocol. One of the B type tanks (B3) had a higher sex ratio, due to an error of sexual determination, and displayed F values above 15000 eggs. This leads to the question that reproduction performance may be eventually enhanced by increasing the sex ratios to 3♀:1♂or even to 5♀:1♂. The higher amount of available females will probably promote less competition between males and the establishment of pairs instead of having dominant alpha males (1–3 individuals), which promote increased stress in the tank social interaction and especially to females. However, a compromise between reproduction performance and inbreeding needs to be found so that the protocol can be applied by the industry.
Concerning other cephalopod species with potential for the aquaculture industry, the present results are highly relevant as there is little done concerning control over reproduction in captivity. Mainly, animals are collected from the wild and they are just placed in tanks and eggs collected later. For instance, to the best of our knowledge, reports on O. vulgaris description of reproduction methods are not consistent [i.e., Iglesias et al. (2000), Iglesias et al. (2007), and Iglesias et al. (2014)] or are in its infancy in terms of control for other cephalopods [e.g., Rosas et al. (2014), Nabhitabhata (2014), and Nabhitabhata and Ikeda (2014)]. Moreover, values of fecundity, fertility or even hatching rates are not reported in most studies made with octopus paralarvae and this is a setback while understanding the quality of these. This means little or no control over reproduction at all in most if not all cephalopods which are kept in captivity with any purpose; a constraint for the aquaculture industry, where estimates of egg biomass and when these are laid are crucial for the proper management of the stocks.
The present results show that domestication of cephalopods can be done in a natural way, having a welfare-based approach in an attempt to maximize the numbers for production. We believe that the existing results are even more important since personality traits have been reported already for S. officinalis (Carere et al., 2015; Zoratto et al., 2018) and we believe that these will be soon also reported in other cephalopods.
On the other hand, the welfare-based approach of broodstock management applied in the present study has not even been considered for finfish. There are some limitations on a direct comparison of the present results obtained in a cephalopod due to the different physiology and biology and the reproductive diversity of teleost fish (Smith and Wootton, 2015), which sees some parallels in cephalopods (Rocha et al., 2001). Both fish and cephalopods are poikilotherms with growth being determined by environment cues, which influence the energy balance and the existing trade-offs between growth and reproduction that optimize reproduction performance (Forsythe and Van Heukelem, 1987; Forsythe et al., 1994; Lee et al., 1998). In both, these trade-offs have paramount influence in the pattern of reproduction (Wootton and Smith, 2014), namely on timing of sexual maturation and life cycle (e.g., semelparity of both cuttlefish and the salmon, Salmo salar). However, in fish, these also affect the periodicity of the reproductive cycle and spawning seasonality in Sparidae (Mylonas et al., 2011), such as the sea bream (Sparus aurata), red porgy (Pagrus pagrus), the common dentex (Dentex dentex) or the sea bass (Dicentrarchus labrax) (Devauchelle and Coves, 1988; Barnabé, 1996).
The main achievement of the present study is the optimization of the broodstock rearing conditions, considering a more natural way and avoiding the stress related to handling and hormonal induction of gametogenesis. Hormonal manipulations are used as a management tool and in some cases are reported as the only way to produce fertilized eggs (Mylonas et al., 2010). This normally leads to stress and the loss of natural and spontaneous spawning in captive conditions, promoting the need to employ artificial gamete collection and fertilization (Conte, 2004; Migaud et al., 2013).
Data Availability Statement
The datasets generated for this study are available on request to the corresponding author.
Ethics Statement
The experiments included in this study have been carried out for the sole purpose of zootechnical purposes and with very limited interference and handling with animals. This study has been performed in 2014–2015, as part and extension of projects SEPIABREED (FCT PTDC/MAR/120876/2010) and SEPIATECH (PROMAR 31.03.05.FEP.002). Both projects started before the entry into force of Directive 2010/63/EU as national legislation in Portugal, and in this sense were exempt of approval by the Portuguese NCA.
Author Contributions
AS and JA obtained funding for the experiments. AS conceptualized and designed the experiments. JC, RG, PF, AA, and AC were responsible for animal husbandry. JC, DH-B, SB, AS, AC, and AA performed the experiments. JC and AS analyzed the data and prepared both figures and tables. AS and JC wrote the manuscript. All authors provided editorial input.
Funding
AS wish to thank Fundação para a Ciência e a Tecnologia (FCT) for funding (SFRH/BPD/36100/2007 grant, Invited Research grant - program Incentivo, and IF/00576/2014 contract). FCT [Project SEPIABREED PTDC/MAR/120876/2010 and Plurennial funding to CCMAR (UID/Multi/04326/2016)] and PROMAR (Project SEPIATECH 31.03.05.FEP.002) funded this work.
Conflict of Interest
RG was employed by Lucta, S.A.
The remaining authors declare that the research was conducted in the absence of any commercial or financial relationships that could be construed as a potential conflict of interest.
Acknowledgments
The authors thank Ana Rita Oliveira, Cátia Silva, Lauren Martin and Inma Ferrer Mateu for their assistance during the cuttlefish samplings. The authors also wish to thank Mr. João Reis, Miguel Viegas and Cristovão Nunes at Ramalhete Station for logistical assistance with the study. The authors also wish to thank Prof. William Driedzic for proofreading the manuscript regarding English grammatical errors. The authors would also like to thank the two reviewers for their valued criticism and suggestions.
Supplementary Material
The Supplementary Material for this article can be found online at: https://www.frontiersin.org/articles/10.3389/fmars.2020.00112/full#supplementary-material
References
Barnabé, G. (1996). Bases Biologiques et Écologiques de l’Aquaculture. Zaragoza: Spain Editorial Acribia, S.A.
Béné, C., Barange, M., Subasinghe, R., Pinstrup-Andersen, P., Merino, G., Hemre, G.-I., et al. (2015). Feeding 9 billion by 2050 – Putting fish back on the menu. Food Secur. 7, 261–274. doi: 10.1007/s12571-015-0427-z
Boletzky, S. V. (1983). “Sepia officinalis,” in Cephalopod Life Cycles, ed. P. R. Boyle, (London: Academic Press), 31–52.
Boletzky, S. V. (1987). Fecundity variation in relation to intermittent or chronic spawning in the cuttlefish, Sepia officinalis L. (Mollusca, Cephalopoda). Bull. Mar. Sci. 40, 382–387.
Boletzky, S. V., and Villanueva, R. (2014). “Cephalopod biology,” in Cephalopod Culture, eds J. Iglesias, L. Fuentes, and R. Villanueva, (Dordrecht: Springer), 3–16. doi: 10.1007/978-94-017-8648-5_1
Buchet, V., Coquard, E., Sévère, A., and Barone, H. (2008). Influence of tank volume on vitellogenesis and spawning performances in sea bass Dicentrarchus labrax L. Aquac. Res. 39, 420–426. doi: 10.1111/j.1365-2109.2007.01828.x
Carere, C., Grignani, G., Bonanni, R., Della Gala, M., Carlini, A., Angeletti, D., et al. (2015). Consistent individual differences in the behavioural responsiveness of adult male cuttlefish (Sepia officinalis). Appl. Anim. Behav. Sci. 167, 89–95. doi: 10.1016/j.applanim.2015.03.005
Castanheira, M. F., Cerqueira, M., Millot, S., Gonçalves, R. A., Oliveira, C. C. V., Conceição, L. E. C., et al. (2016). Are personality traits consistent in fish? The influence of social context. Appl. Anim. Behav. Sci. 178, 96–101. doi: 10.1016/j.applanim.2016.02.004
Castanheira, M. F., Herrera, M., Costas, B., Conceição, L. E. C., and Martins, C. I. M. (2013). Can we predict personality in fish? Searching for consistency over time and across contexts. PLoS ONE 8:e62037. doi: 10.1371/journal.pone.0062037
Conte, F. S. (2004). Stress and the welfare of cultured fish. Appl. Anim. Behav. Sci. 86, 205–223. doi: 10.1016/j.applanim.2004.02.003
Cooke, G. M., Anderson, D. B., Begout, M.-L., Dennison, N., Osorio, D., Tonkins, B., et al. (2019). Prospective severity classification of scientific procedures in cephalopods: report of a COST FA1301 Working Group survey. Lab. Anim. 53, 541–563. doi: 10.1177/0023677219864626
Correia, M., Domingues, P. M., Sykes, A., and Andrade, J. P. (2005). Effects of culture density on growth and broodstock management of the cuttlefish, Sepia officinalis (Linnaeus, 1758). Aquaculture 245, 163–173. doi: 10.1016/j.aquaculture.2004.12.017
Devauchelle, N., and Coves, D. (1988). Sea bass (Dicentrarchus labrax) reproduction in captivity: gametogenesis and spawning. Aquat. Living Resour. 1, 215–222. doi: 10.1051/alr:1988021
Domingues, P., and Marquez, L. (2010). Effects of culture density and bottom area on growth and survival of the cuttlefish Sepia officinalis (Linnaeus, 1758). Aquac. Int. 18, 361–369. doi: 10.1007/s10499-009-9249-3
Duarte, C. M., Marbá, N., and Holmer, M. (2007). Rapid domestication of marine species. Science 316, 382–383. doi: 10.1126/science.1138042
Forsythe, J. W. (1984). Octopus joubini (Mollusca: Cephalopoda): a detailed sutry of growth througth the full life cycle is a closed seawater system. J. Zool. 202, 393–417. doi: 10.1111/j.1469-7998.1984.tb05091.x
Forsythe, J. W. (1993). “A working hypothesis of how seasonal temperature change may impact the field growth of young cephalopods,” in Recent Advances in Fisheries Biology, eds T. Okutani, R. K. Odor, and T. Kubodera, (Tokyo: Tokai University Press), 133–143.
Forsythe, J. W., Derusha, R. H., and Hanlon, R. T. (1994). Growth, reproduction and life-span of Sepia officinalis (Cephalopoda, Mollusca) cultured through 7 consecutive generations. J. Zool. 233, 175–192. doi: 10.1111/j.1469-7998.1994.tb08582.x
Forsythe, J. W., and Hanlon, R. T. (1988). Effect of temperature on laboratory growth, reproduction and life span of Octopus bimaculoides. Mar. Biol. 98, 369–379. doi: 10.1007/bf00391113
Forsythe, J. W., Hanlon, R. T., and Derusha, R. (1991). “Pilot-Scale culture of Sepia in biomedical research,” in The Cuttlefish, ed. E. Boucaud-Camou, (Caen: Centre de Publications de l’Université de Caen), 313–323.
Forsythe, J. W., and Van Heukelem, W. F. (1987). “Growth,” in Cephalopod Life Cycles, ed. P. R. Boyle, (London: Academic Press, Inc.), 135–156.
Fowler, J., Cohen, L., and Jarvis, P. (1998). Practical Statistics for Field Biology. New York, NY: Wiley.
Gonçalves, R. A., Aragao, C., Frias, P. A., and Sykes, A. V. (2012). The use of different anaesthetics as welfare promoters during short-term human manipulation of European cuttlefish (Sepia officinalis) juveniles. Aquaculture 370, 130–135. doi: 10.1016/j.aquaculture.2012.10.014
Hanley, J. S., Shashar, N., Smolowitz, R., Bullis, R. A., Mebane, W. N., Gabr, H. R., et al. (1998). Modified laboratory culture techniques for the European cuttlefish Sepia officinalis. Biol. Bull. 195, 223–225. doi: 10.2307/1542850
Hanlon, R. T., Ament, S. A., and Gabr, H. (1999). Behavioral aspects of sperm competition in cuttlefish, Sepia officinalis (Sepioidea: Cephalopoda). Mar. Biol. 134, 719–728. doi: 10.1007/s002270050588
ICES (2010). “Report on the workshop on sexual maturity staging of cephalopods”, in ICES CM 2010/ACOM:49 (Livorno, Italy: ICES).
Iglesias, J., and Fuentes, L. (2014). “Octopus vulgaris. Paralarval culture,” in Cephalopod Culture, eds J. Iglesias, L. Fuentes, and R. Villanueva, (Dordrecht: Springer), 427–450. doi: 10.1007/978-94-017-8648-5_23
Iglesias, J., Fuentes, L., and Villanueva, R. (2014). Cephalopod Culture. Berlin: Springer, doi: 10.1007/978-94-017-8648-5
Iglesias, J., Sánchez, F. J., Bersano, J. G. F., Carrasco, J. F., Dhont, J., Fuentes, L., et al. (2007). Rearing of Octopus vulgaris paralarvae: present status, bottlenecks and trends. Aquaculture 266, 1–15. doi: 10.1016/j.aquaculture.2007.02.019
Iglesias, J., Sánchez, F. J., Otero, J. J., and Moxica, C. (2000). Culture of octopus (Octopus vulgaris, Cuvier): present knowledge, problems and perspectives. Cahiers Opt. Méditerranéennes 47, 313–321.
Izquierdo, M. S., Fernández-Palacios, H., and Tacon, A. G. J. (2001). Effect of broodstock nutrition on reproductive performance of fish. Aquaculture 197, 25–42. doi: 10.1016/s0044-8486(01)00581-6
Laptikhovsky, V., Salman, A., Onsoy, B., and Katagan, T. (2003). Fecundity of the common cuttlefish, Sepia officinalis L. (Cephalopoda, Sepiidae): a new look at an old problem. Sci. Marina 67, 279–284. doi: 10.3989/scimar.2003.67n3279
Lee, P. G., Turk, P. E., Forsythe, J. W., and Dimarco, F. P. (1998). Cephalopod culture: physiological, behavioral and environmental requirements. Suisanzoshoku 46, 417–422.
Leporati, S., Pecl, G., and Semmens, J. (2007). Cephalopod hatchling growth: the effects of initial size and seasonal temperatures. Mar. Biol. 151, 1375–1383. doi: 10.1007/s00227-006-0575-y
Mangold, K. (1983). “Octopus vulgaris,” in Cephalopod Life Cycles: Species Acounts, Vol. 1, ed. P. R. Boyle, (London: Academic Press), 475.
Migaud, H., Bell, G., Cabrita, E., Mcandrew, B., Davie, A., Bobe, J., et al. (2013). Gamete quality and broodstock management in temperate fish. Rev. Aquac. 5, S194–S223. doi: 10.1111/raq.12025
Mylonas, C. C., Fostier, A., and Zanuy, S. (2010). Broodstock management and hormonal manipulations of fish reproduction. Gen. Compar. Endocrinol. 165, 516–534. doi: 10.1016/j.ygcen.2009.03.007
Mylonas, C. C., Zohar, Y., Pankhurst, N., and Kagawa, H. (2011). “Reproduction and broodstock management,” in Sparidae, Biology and Aquaculture of Gilthead Sea Bream and other Species, eds M. A. Pavlidis and C. C. Mylonas, (New Delhi: Blackwell Publishing Ltd.), 95–131. doi: 10.1002/9781444392210.ch4
Nabhitabhata, J. (2014). “Sepia pharaonis,” in Cephalopod Culture, eds J. Iglesias, L. Fuentes, and R. Villanueva, (Dordrecht: Springer), 205–224. doi: 10.1007/978-94-017-8648-5_12
Nabhitabhata, J., and Ikeda, Y. (2014). “Sepioteuthis lessoniana,” in Cephalopod Culture, eds J. Iglesias, L. Fuentes, and R. Villanueva, (Dordrecht: Springer), 315–347. doi: 10.1007/978-94-017-8648-5_17
Navarro, J. C., and Monroig, Ó, and Sykes, A. V. (2014). “Nutrition as a key factor for cephalopod aquaculture,” in Cephalopod Culture, eds J. Iglesias, L. Fuentes, and R. Villanueva, (Dordrecht: Springer), 77–95. doi: 10.1007/978-94-017-8648-5_5
Okumura, S., Okamoto, K., Oomori, R., Sato, H., and Nakazono, A. (2003). Improved fertilization rates by using a large volume tank in red spotted grouper (Epinephelus akaara). Fish Physiol. Biochem. 28, 515–516. doi: 10.1023/B:FISH.0000030647.77596.c9
Quinn, G. P., and Keough, M. J. (eds) (2002). Experimental Design and Data Analysis for Biologists. Cambridge: Cambridge University Press.
Rocha, F., Guerra, A., and Gonzalez, A. F. (2001). A review of reproductive strategies in cephalopods. Biol. Rev. 76, 291–304. doi: 10.1017/S1464793101005681
Rosas, C., Gallardo, P., Mascaró, M., Caamal-Monsreal, C., and Pascual, C. (2014). “Octopus maya,” in Cephalopod Culture, eds J. Iglesias, L. Fuentes, and R. Villanueva, (Dordrecht: Springer), doi: 10.1007/978-94-017-8648-5_20
Smith, A. J., Clutton, R. E., Lilley, E., Hansen, K. E. A., and Brattelid, T. (2018). PREPARE: guidelines for planning animal research and testing. Lab. Anim. 52, 135–141. doi: 10.1177/0023677217724823
Smith, D., Anderson, D., Degryse, A.-D., Bol, C., Criado, A., Ferrara, A., et al. (2018). Classification and reporting of severity experienced by animals used in scientific procedures: FELASA/ECLAM/ESLAV Working Group report. Lab. Anim. 52, 5–57. doi: 10.1177/0023677217744587
Smith, C., and Wootton, R. J. (2015). The remarkable reproductive diversity of teleost fishes. Fish Fish. 12116, 1208–1215. doi: 10.1111/faf.12116
Sykes, A. V., Almansa, E., Cooke, G. M., Ponte, G., and Andrews, P. L. R. (2017a). The digestive tract of cephalopods: a neglected topic of relevance to animal welfare in the laboratory and aquaculture. Front. Physiol. 8:492. doi: 10.3389/fphys.2017.00492
Sykes, A. V., Alves, A., Capaz, J. C., Madeira, C., Couto, A. T., Goncalves, R. A., et al. (2017b). Refining tools for studying cuttlefish (Sepia officinalis) reproduction in captivity: In Vivo sexual determination, tagging and DNA collection. Aquaculture 479, 13–16. doi: 10.1016/j.aquaculture.2017.05.021
Sykes, A. V., Domingues, P., and Andrade, J. P. (2014). “Sepia officinalis,” in Cephalopod Culture, eds J. Iglesias, L. Fuentes, and R. Villanueva, (Dordrecht: Springer), 175–204. doi: 10.1007/978-94-017-8648-5_11
Sykes, A. V., Domingues, P. M., and Andrade, J. P. (2006a). Effects of using live grass shrimp (Palaemonetes varians) as the only source of food for the culture of cuttlefish, Sepia officinalis (Linnaeus, 1758). Aquac. Int. 14, 551–568. doi: 10.1007/s10499-006-9054-1
Sykes, A. V., Domingues, P. M., Correia, M., and Andrade, J. P. (2006b). Cuttlefish culture – State of the art and future trends. Vie et Milieu 56, 129–137.
Sykes, A. V., Domingues, P. M., Marquez, L., and Andrade, J. P. (2011). The effects of tank colours on the growth and survival of cuttlefish (Sepia officinalis, Linnaeus 1758) hatchlings and juveniles. Aquac. Res. 42, 441–449. doi: 10.1111/j.1365-2109.2010.02639.x
Sykes, A. V., Pereira, D., Rodríguez, C., Lorenzo, A., and Andrade, J. P. (2013). Effects of increased tank bottom areas on cuttlefish (Sepia officinalis, L.) reproduction performance. Aquac. Res. 44, 1017–1028. doi: 10.1111/j.1365-2109.2012.03106.x
Vidal, E. A. G., Villanueva, R., Andrade, J. P., Gleadail, I. G., Iglesias, J., Koueta, N., et al. (2014). Cephalopod culture: current status of main biological models and research priorities. Adv. Cephalopod Sci. 67, 1–98. doi: 10.1016/B978-0-12-800287-2.00001-9
Villanueva, R., Sykes, A. V., Vidal, ÉA. G., Rosas, C., Nabhitabhata, J., Fuentes, L., et al. (2014). “Current status and future challenges in cephalopod culture,” in Cephalopod Culture, eds J. Iglesias, L. Fuentes, and R. Villanueva, (Springer), 479–489. doi: 10.1007/978-94-017-8648-5_26
Wootton, R. J., and Smith, C. (2014). Reproductive Biology of Teleost Fishes. West Sussex. Hoboken, NJ: John Wiley & Sons, Ltd., doi: 10.1002/9781118891360
Keywords: broodstock, egg price, European cuttlefish (Sepia officinalis), fecundity, fertility, reproduction improvement, sex ratio, tank type
Citation: Capaz JC, Hernández-Brooke D, Balvet S, Couto AT, Alves AC, Gonçalves RA, Frias PA, Andrade JP and Sykes AV (2020) Control of Zootechnology Leads to Improved Cuttlefish (Sepia officinalis, L.) Reproduction Performance up to Pre-industrial Levels. Front. Mar. Sci. 7:112. doi: 10.3389/fmars.2020.00112
Received: 22 March 2019; Accepted: 11 February 2020;
Published: 26 February 2020.
Edited by:
JInghui Fang, Yellow Sea Fisheries Research Institute (CAFS), ChinaReviewed by:
Nawel Mezrai, Université de Caen Normandie, FranceGraziano Fiorito, Stazione Zoologica Anton Dohrn, Italy
Copyright © 2020 Capaz, Hernández-Brooke, Balvet, Couto, Alves, Gonçalves, Frias, Andrade and Sykes. This is an open-access article distributed under the terms of the Creative Commons Attribution License (CC BY). The use, distribution or reproduction in other forums is permitted, provided the original author(s) and the copyright owner(s) are credited and that the original publication in this journal is cited, in accordance with accepted academic practice. No use, distribution or reproduction is permitted which does not comply with these terms.
*Correspondence: António V. Sykes, YW50dnN5a2VzQGdtYWlsLmNvbQ==