- 1Programa de Pós-Graduação em Biologia Animal, Departamento de Biologia, Universidade Federal de Pernambuco, Recife, Brazil
- 2Laboratório de Dinâmica de Populações Marinhas (DIMAR), Departamento de Pesca e Aquicultura, Universidade Federal Rural de Pernambuco, Recife, Brazil
- 3Laboratório de Espectrometria Atômica, Departamento de Química, Universidade Federal de Santa Maria, Santa Maria, Brazil
Sharks are among the most endangered and data poor vertebrates in the world. The lack of information regarding their habitat use is especially concerning since these are crucial for the establishment of priority areas for species conservation. Investigating the trace elements present in shark vertebrae has become an interesting tool to analyze species habitat use over individuals’ lifetimes. Therefore, we applied vertebrae microchemistry to investigate habitat use of Carcharhinus porosus in Brazil’s Northern Coast (BNC). We also discuss methodological issues that must be addressed in the future to make microchemistry studies with elasmobranchs at low latitudes more robust. Vertebrae from seventeen individuals sampled in the 1980s (n = 8), and in 2017 and 2018 (n = 9) were evaluated through laser ablation inductively coupled plasma mass spectrometry. We analyzed five elements known to reflect environmental characteristics (Ba, Ca, Mg, Mn, and Sr) by sex, seasons, decades of capture, and life stages. Since Ca is the most abundant element in the vertebrae, we calculated element:Ca ratios and employed these proportions for all statistical analysis. We also used fisheries-independent catch data from the 1980s to test if the BNC is a nursery area for C. porosus. We found significant differences in element concentrations between sexes in both multi and single-element analysis, while decades differed only in the multi-element and Sr:Ca. Furthermore, seasons differed in both multi-element and Mg:Ca and Ba:Ca ratios. We did not find differences between life stages. Neonate multi-element signatures yielded three major groups, thus suggesting that the species has at least three birthing grounds in the area. Despite the occurrence of individuals of all sizes, adults show a more distinct occurrence pattern. Our results point toward the hypothesis that the BNC is an essential habitat for this species since its whole life cycle occurs in this area. Therefore, the BNC is a critical area for its conservation. We reinforce that future studies with strictly tropical species should investigate the effects of metabolism, the species’ growth rate, and the validation of other elements capable of demonstrating fine-scale changes in habitat to reduce the inherent noise in microchemistry studies.
Introduction
Basic biological data such as demographic parameters, life history traits, reproductive patterns, and habitat use are crucial for species management and conservation (Cochrane, 2002). However, sharks are among the taxonomic groups with less basic information available, thus hampering proper conservation actions in all geographical scales (Dulvy et al., 2014). Furthermore, most shark populations are subjected to overfishing and an overall decreasing habitat quality due to water pollution, which raises concern for their sustainability in the near future.
Sharks in Brazil are facing similar problems, and legislation aiming at mitigating them has not followed along (Barreto et al., 2017). In addition, several nationally and internationally endangered species are consistently caught as bycatch in the shrimp and teleost targeted fisheries (Palmeira et al., 2013; Almerón-Souza et al., 2018; Feitosa et al., 2018). Brazil’s Northern Coast (BNC) is the main region in the country where sharks experience bycatch (Oliver et al., 2015). The region comprises Maranhão, Pará, and Amapá states, thus including the Amazon estuary, and is one of the main fishing grounds of the country, harboring a great diversity of sharks, including endemic species (Lessa et al., 1999b). Furthermore, it is considered a global conservation hotspot for these taxa and several portions are or should be protected areas (Dulvy et al., 2014; Davidson and Dulvy, 2017).
Nevertheless, artisanal and semi-industrial gillnet fisheries targeting large teleost species such as the Acoupa Weakfish Cynoscion acoupa and the Brazilian Spanish Mackerel Scomberomorus brasiliensis have caused severe population collapses in endemic shark species such as Isogomphodon oxyrhynchus in Brazil’s northern coast (Lessa et al., 2016). These fisheries use drift gillnets soaked for up to 12 h with 170 mm meshes between knots, 4 to 6 m in height extending for at least 3 km for Cynoscion acoupa (de Almeida et al., 2014) and 60 mm meshes for S. brasiliensis reaching more than 5 km in extension (Mourão et al., 2014). The smalltail shark Carcharhinus porosus is another shark species that has suffered severe declines, but its collapse has been under documented. The only evidences of collapse are an 85% decrease in the biomass captured in 2004 in the BNC, which is considered its global center of abundance, and its extremely low genetic diversity in the same area (Lessa et al., 2006; Tavares et al., 2013).
Since this species corresponded to 43% of the sharks caught by gillnets in the region during the 1980s and 1990s (Lessa, 1997), this dramatic decline is of particular concern. Despite its small size (150 cm estimated maximum length), its life history traits make it easily susceptible to overfishing since its fecundity is low (average of 6 pups per gestation), the reproductive cycle is biannual, and both sexes reach sexual maturity with 6 years old (Lessa and Santana, 1998). It is highly associated to turbid and dynamic areas with mangrove rich coasts (Feitosa et al., 2020), and juveniles were consistently caught near estuaries in Maranhão state’s coast (Menni and Lessa, 1998). Nevertheless, its habitat use patterns are still poorly understood.
Several techniques such as acoustic and satellite telemetry have been recently applied to study habitat use of several shark species (Hazin et al., 2013; Taylor et al., 2017). However, these methods are expensive, require the capture, tagging, and release of live specimens, and only gather data within a limited timeframe of the animal’s life (Fraser et al., 2018). As a counterpart, the evaluation of trace element composition in fish hard parts provides a glimpse of how an animal uses its habitat during its whole lifetime (Walther, 2019). Therefore, it is a promising technique that has been widely used for teleost fishes (de Pontual and Geffen, 2002; Gillanders, 2005; Elsdon et al., 2008; Paillon et al., 2014), and is being increasingly applied on sharks and rays (Tillett et al., 2011; Izzo et al., 2016; McMillan et al., 2017, 2018). Several elements such as Strontium (Sr), Barium (Ba), Manganese (Mn), and Magnesium (Mg) have been considered to be reliable proxies for environmental tracers of species habitat use, since their concentration in the vertebrae has been shown to reflect their environmental concentrations (McMillan et al., 2017).
More specifically, Sr and Ba are related to changes in salinity, in which the former is more abundant in saltwater and the latter in freshwater (McMillan et al., 2017). On the other hand, both Mn and Mg are associated with large variations in water temperature, but Mn concentration changes are also believed to reflect proximity of mangrove areas (Paillon et al., 2014; Smith et al., 2016). Since these trace element concentrations are marked in the vertebrae and no evidence of element reabsorption by the body exists, evaluating their variations in hard parts enables the understanding of changes in trace element composition throughout an individual’s lifetime. With these, key information on the type of habitat a species uses during each life stage (McMillan et al., 2017), and even potential nursery areas (Tillett et al., 2011; Lewis et al., 2016; Smith et al., 2016; Heupel et al., 2018) can be obtained. However, several aspects of this methodology need to be addressed to improve the robustness of conclusions, especially in areas with little environmental variation such as the BNC.
Unraveling the habitat use patterns of a species according to age, together with other biological information can provide enough information to establish key areas for a species conservation such as nurseries. Thus, this study aimed at investigating these patterns across life stages and between sexes for one of the most heavily fished shark species in the Brazilian northern coast through vertebrae trace elements. We also used the vertebrae microchemistry data to investigate eventual changes in habitat use when the species was abundant (1980s) and after population collapse (2010s), as well as investigating eventual chemical differences between seasons. Furthermore, we tested the data obtained to the criteria developed by Heupel et al. (2007), which define the assumptions for an area to be considered an elasmobranch nursery, and discuss the existence of an essential habitat for the species in the area. Finally, we briefly discuss knowledge gaps that need to be filled by future elasmobranch vertebrae microchemistry studies carried out with species inhabiting low latitude areas.
Materials and Methods
Sampling Area
Brazil’s northern coast (4°11′40.58′′N to 2°18′45.53′′S) is a highly indented area subjected to the Amazon River’s estuary of which Maranhão state is part (Figure 1). Temperatures suffer little variation during the year (average water temperature 28°C), but the region experiences two marked seasonal pluviometry cycles. The rainy season extends from January to June and the dry season from August to December, with a transition period in July. In addition, daily tidal cycles pose a great level of dynamism to the coastal areas with spring tides reaching up to 7 m in amplitude from high to low. The vast amount of estuaries and the tropical climate grant the region the unique characteristics of its mangrove forests, with trees reaching 30 m in height and extending for over 5,000 km2 (Souza-Filho, 2005). Due to its highly productive and turbid waters, diversity and endemism levels are high, thus making it one of the most important areas for shark conservation in the world.
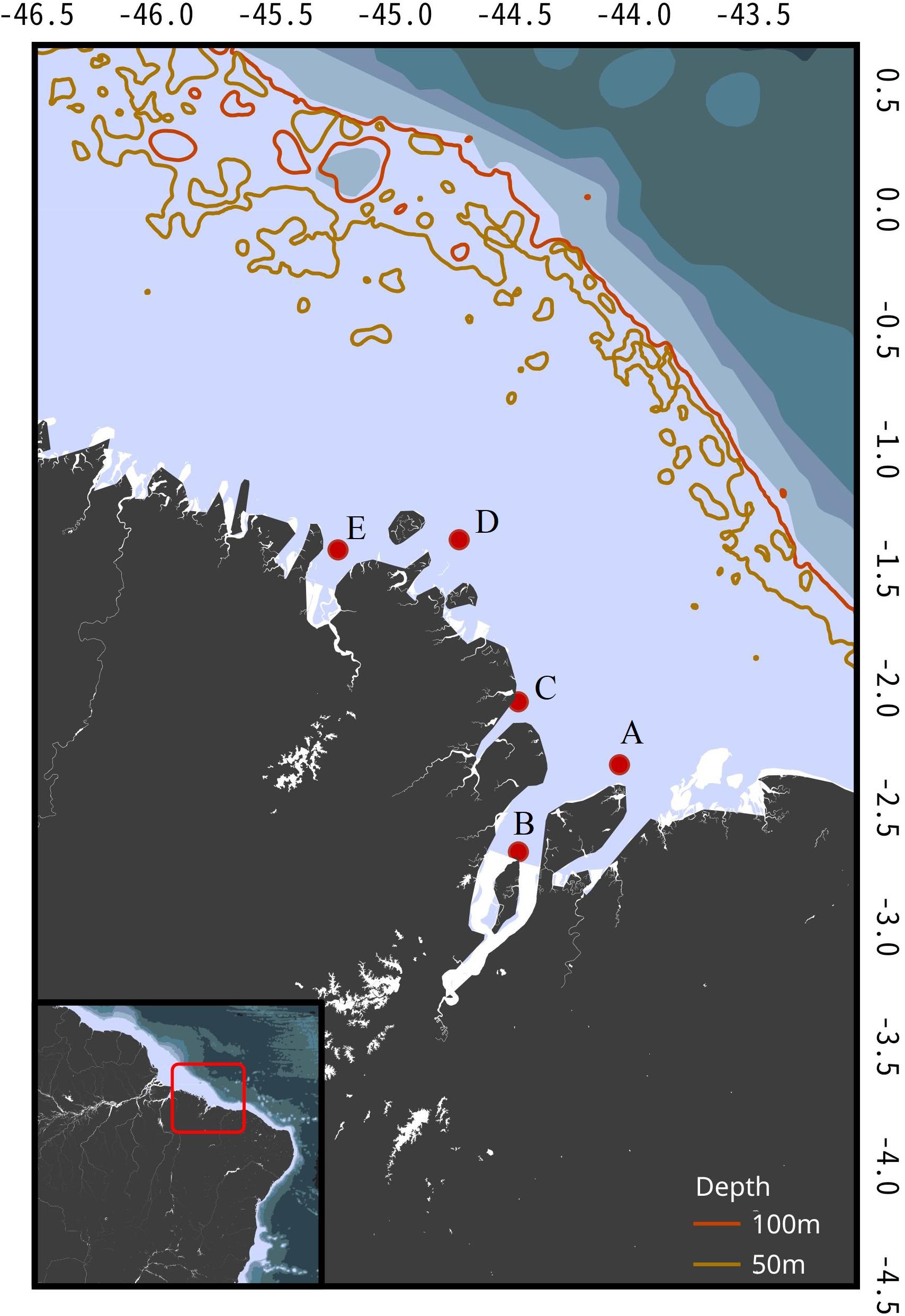
Figure 1. Brazil’s Northern Coast (area within the red square) with sampling locations, and 50 and 100 m depth isobaths. A, Canal do Navio; B, Ilha dos Caranguejos; C, Araoca; D, Farol São João; E, Turiaçu.
Sampling Design
Smalltail shark vertebrae were sampled from specimens landed in the Raposa municipality in Maranhão state (0°59′0.88′′S to 2°18′45.53′′S). Blocks of five vertebrae were retrieved from seventeen specimens. Since carcasses were already processed (headed and gutted), identification followed the field remarks pointed by Compagno (1984), such as the origin of the second dorsal fin over the midbase of a strongly notched anal fin. Samples were collected according to the Brazilian environmental laws under the license (License SISBIO, 49663-1). Since samples obtained in 2017 and 2018 were collected from dead specimens landed and traded in local markets, no ethics committee approval is necessary. For the samples collected in the 1980s, no ethics committee existed at the time.
For the vertebrae microchemistry analysis, frozen samples were thawed and two vertebrae from each block were retrieved. The connecting tissue was removed and vertebrae were air dried for 48 h (n = 9). Samples collected by R. Lessa and V. Batista in the 1980s were also included in the analysis (n = 8) (see Lessa and Santana, 1998 for sampling locations). Although these were subjected to formaldehyde 4% treatment, it does not impair our analysis since there is no evidence of this kind of procedure affecting microchemistry results – other than sodium concentration (Mohan et al., 2017). Furthermore, although we acknowledge that the sample size used was somewhat small, other studies using trace elements for habitat use, and even age and growth analysis, used smaller sample sizes for critically endangered, vulnerable, and near threatened shark and teleost species, including with a single hard part sample (Scharer et al., 2012; Hermann et al., 2016; Mohan et al., 2018). Indeed, collecting new vertebrae samples from the smalltail shark is difficult due to their rarity.
Vertebrae Processing
For processing, all vertebrae were embedded in polyester resin and air-dried for 48 h. Vertebrae were transversely sectioned with a low speed diamond IsometTM (Buehler) saw (Figure 2). Translucent and opaque ring pairs were considered to be formed annually (Lessa and Santana, 1998). Prior to analysis by laser ablation inductively coupled plasma mass spectrometry (LA-ICP-MS), vertebrae sections were polished with silicon carbide paper (no 8000), washed ultrasonically with ultrapure water (Milli-Q, Millipore), and air-dried in sterilized plastic vials for 48 h before analysis. Cleaned samples were stored individually in hermetic plastic bags until laser ablation.
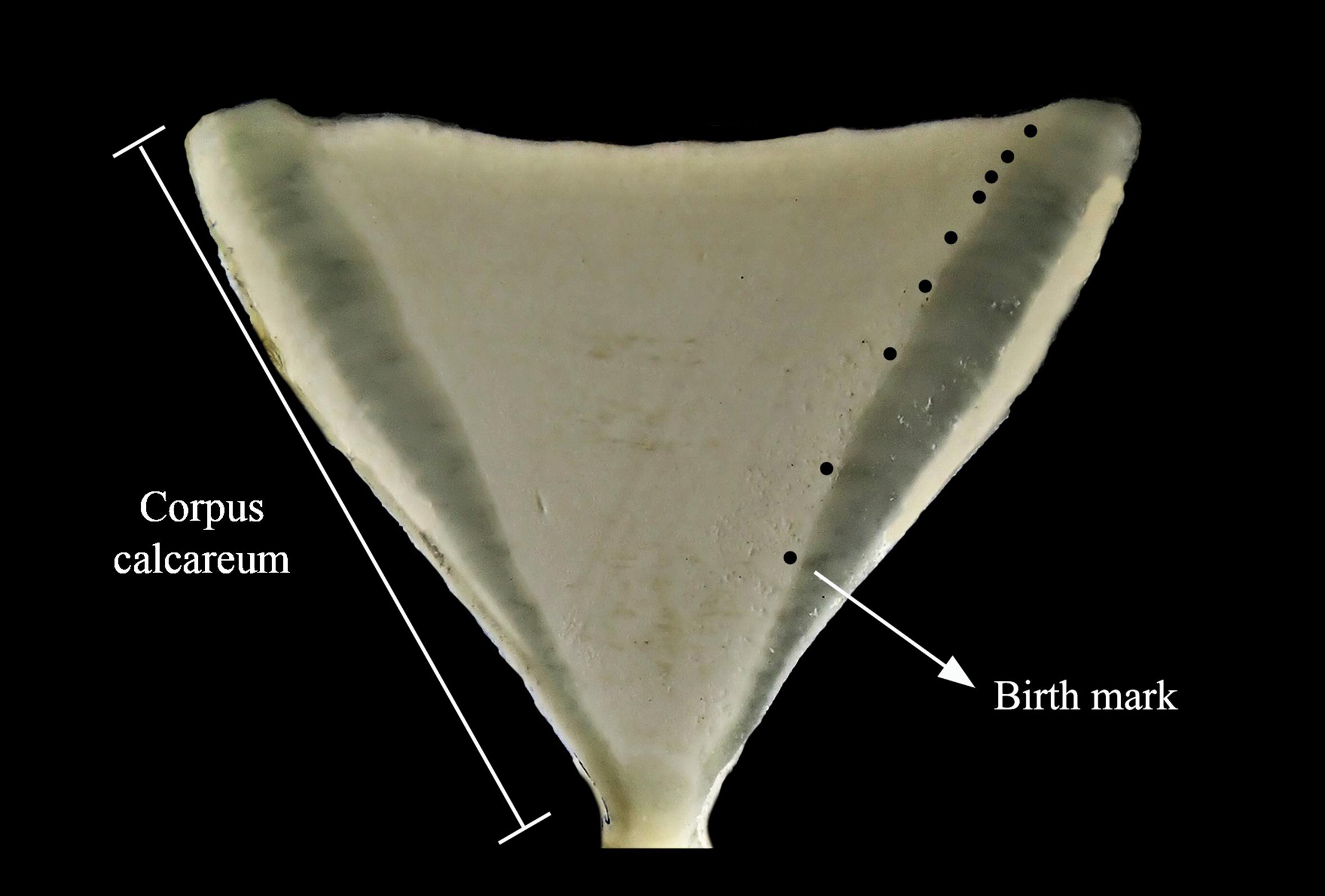
Figure 2. Carcharhinus porosus vertebrae section. Black dots represent translucent bands counted as part of the ring pairs.
Analytical measurements were taken at the Laboratório de Espectrometria Atômica - Departamento de Química (UFSM) with a Q-switched pulse 213 nm Nd:YAG laser ablation system (NWR 213, ESI – New Wave Research) coupled to an Agilent 7500 inductively coupled plasma mass spectrometer. The laser was operated with a pulse frequency of 20 Hz, a scan speed of 30 μm s–1, and an energy output of 0.2 to 0.3 mJ per pulse. Under these conditions, each crater width was approximately 25 μm. The ablated material was conducted through a Teflon-coated tube into the ICP-MS using Argon as a carrier gas (0.85 dm3 min–1). The ICP was operated at 1,300 W nominal powers with outer and intermediate gas flows of 15.0 and 1.1 dm3 min–1, respectively. Quantification was performed using element:Calcium ratios as internal standard for each element. The NIST 612 certified reference material was employed to obtain counts per second (CPS). The limit of detection (LOD) was calculated following Longerich et al. (1996) and elements that did not meet this standard were excluded from the analysis.
Experimental Fisheries Data
We also used the catch dataset from the specimens captured between 1984 and 1987 and that was the basis for the Lessa and Santana (1998) study. The inverted von Bertalanffy growth function was calculated to obtain an age estimate based on the total size of each individual. All specimens sampled (n = 930) were computed, together with the sampling location, month, year, total length, and depth. However, we only used the season, month, and depth information to evaluate eventual occurrence patterns based on such abiotic factors (Table 1).
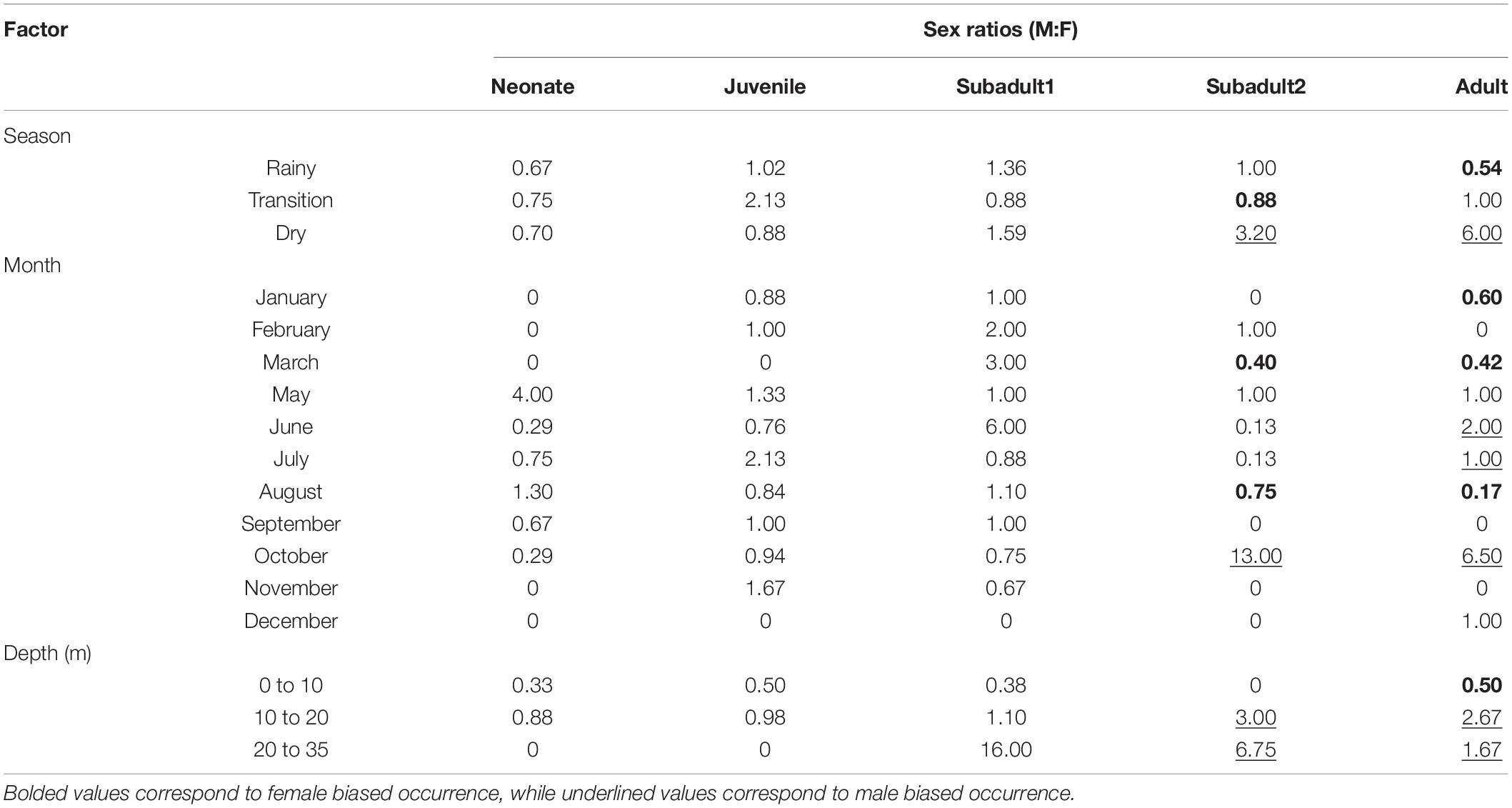
Table 1. Sex ratio (M:F) of catch data published by Lessa et al. (1999a) discriminated by season, month, and depth range.
Data Analysis
The distance for each growth band from the nucleus was measured to obtain the exact positioning of the element concentration counts provided by the LA-ICP-MS. This enabled the establishment of the profile for each element analyzed by year of life. Since specimens had different ages and the information on habitat use per life stage is much more meaningful than on an annual basis, life stages were chosen following the age and growth data for both sexes (Lessa and Santana, 1998). The neonate portion of vertebrae was considered to range from the birthmark to the end of the first year of life, followed by the juvenile phase between the start of the second year of life to the end of the third. The sub-adult stage was subdivided in two groups to avoid misrepresenting habitat use patterns of larger sub-adults: sub-adult1 ranging from the third year to the end of the fourth, and sub-adult2 ranging from the start of the fifth to the end of the sixth year of life. Adult reads were considered from the sixth year to the end of the vertebra.
Seventeen individuals (Table 2) were analyzed in a multi-element LA-ICP-MS for 24Mg, 43Ca, 55Mn,86Sr, 138Ba,112Cd,206Pb, and 208Pb. Element:Ca ratios were calculated for each element with raw concentration counts later transformed into concentrations (ppm) by the equation formulated by Longerich et al. (1996). A Ca concentration of 35% was considered in the present study to convert element reads from CPS to ppm. These data were then either log(x + 1) transformed (for the PERMANOVA and SIMPER analyses) or scaled with the Z transformation (for the cluster analysis) to obtain results on the same scale for all elements analyzed. Only elements that have already been validated to represent proxies for environmental features such as salinity and temperature changes, and that remained above the LOD were used in further analysis. Average reads for each life stage, element, and sample were calculated and used on the subsequent statistical analysis. We performed multi and single-element PERMANOVAs with an Euclidian distance resemblance matrix, a 1000 permutations, and a significance level of 0.05 to evaluate multi and single-elemental differences in habitat use for the species. Sexes, life stages, seasons, and decades of capture were considered fixed factors.
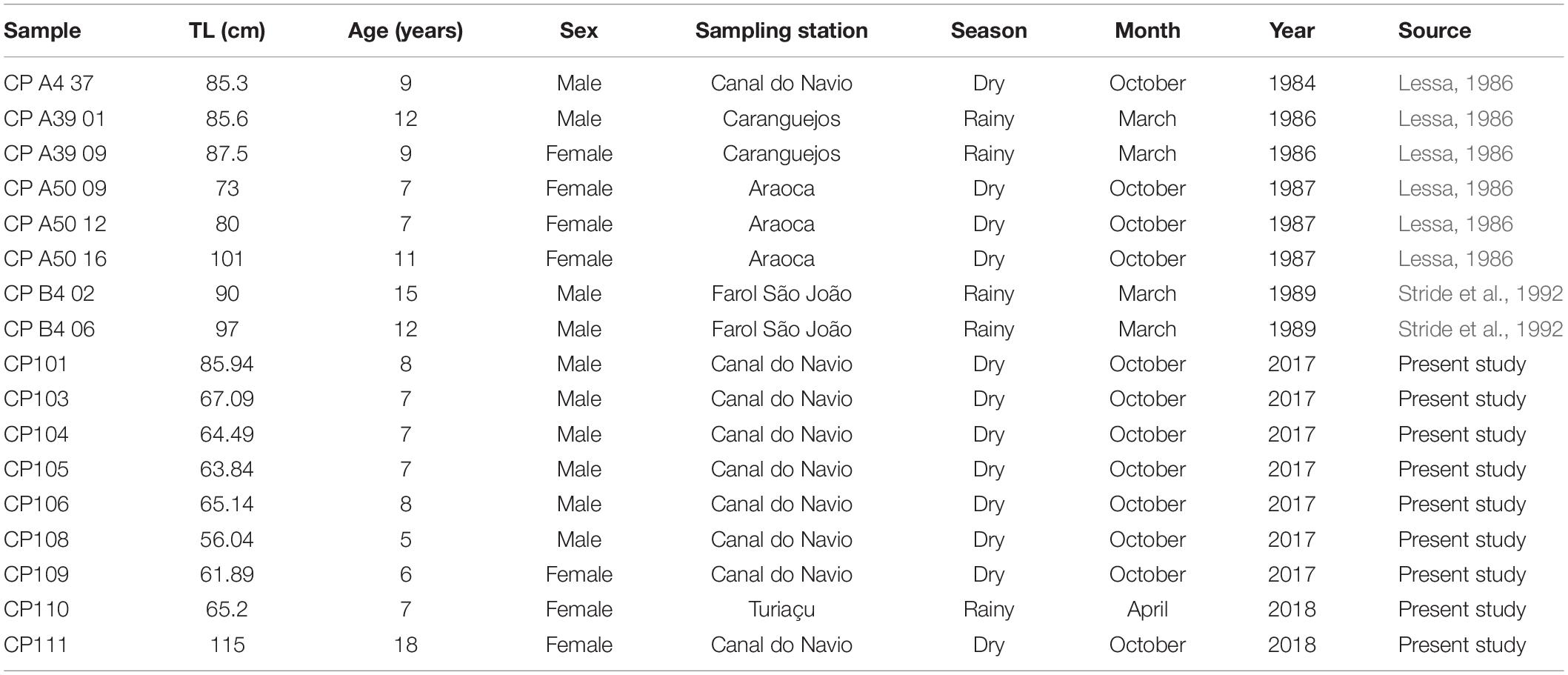
Table 2. Total length (TL), sex, location, date of capture, and source for each individual sampled and analyzed with LA-ICP-MS.
We also performed similarity percentages analysis (SIMPER) for each factor using Euclidian distance. This analysis aimed at evidencing which elements were most important for the chemical signature of the sexes, seasons, and decades. It is important to stress that SIMPER results are not related to the actual concentration of each element in the vertebrae, but to the percentage of contribution that each element has on explaining the total distance calculated through the distance matrix. Therefore, an element with high percentage in the SIMPER results is not necessarily present in high concentrations in the vertebrae. Finally, we did a cluster analysis with average distances to check if groups would be formed based on their multi-element chemical signature exclusively with the data from the neonate phase. All statistical analysis were performed in R version 3.5.1 (R Core Team, 2013) except for the SIMPER which was performed in PRIMER7 software.
Results
Out of the elements analyzed, only Ca, Mg, Mn, Sr, and Ba were used for the habitat use analysis due to their reliability as proxies for environmental tracers in elasmobranchs. Cd and Pb isotopes were excluded from the final analysis because they were below the limit of detection (LOD). No significant statistical differences between life stages were found in any of the PERMANOVAs performed. However, significant differences were found between sexes, seasons, and decades in the multi-element analysis, as well as for sexes in all element:Ca analysis. Furthermore, significant differences were found in the single-element PERMANOVAs between decades for Sr:Ca, and between seasons for Mg:Ca and Ba:Ca (Table 3).
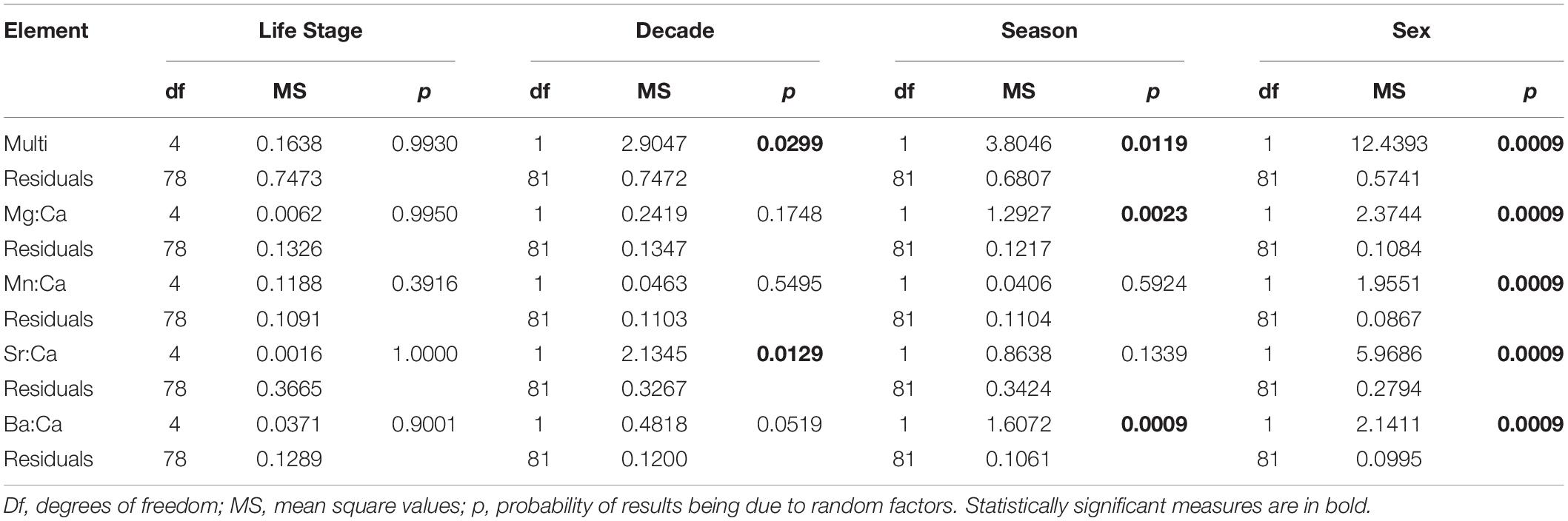
Table 3. Results for the one-step PERMANOVAs calculated for the multi and single-element analysis with life stage, decade, season, and sex of individuals as factors.
As expected based on the PERMANOVA results, SIMPER demonstrated that the chemical signatures were strongly different for sexes, with Sr being much more important in females than in males, and Ba following the inverse pattern in males (Table 4). Furthermore, SIMPER results demonstrate the clear signal of Ba in the rainy season and elevated percentage of Sr importance in the dry season (Figure 3). Regarding the decades of the samples analyzed, the major differences were found between Sr and Mn, with the former being much more significant in the 1980s and the latter being sharply more important in the 2010s. Overall, the element with the smallest variation in all factors evaluated was Mn (SIMPER whole Av. Value = 1.04; Standard Deviation = 0.102) (Table 4). Sr had the highest variation among factors (Av. Value = 2.47; Standard Deviation = 0.217), followed by Ba (Av. Value = 1.02; Standard Deviation = 0.157), and Mg (Av. Value = 2.94; Standard Deviation = 0.149). As demonstrated, Mg and Sr average values are two-fold higher than Mn and Ba.

Table 4. SIMPER results for sexes, seasons, and decades evaluated. Av., average; SD, squared distance.
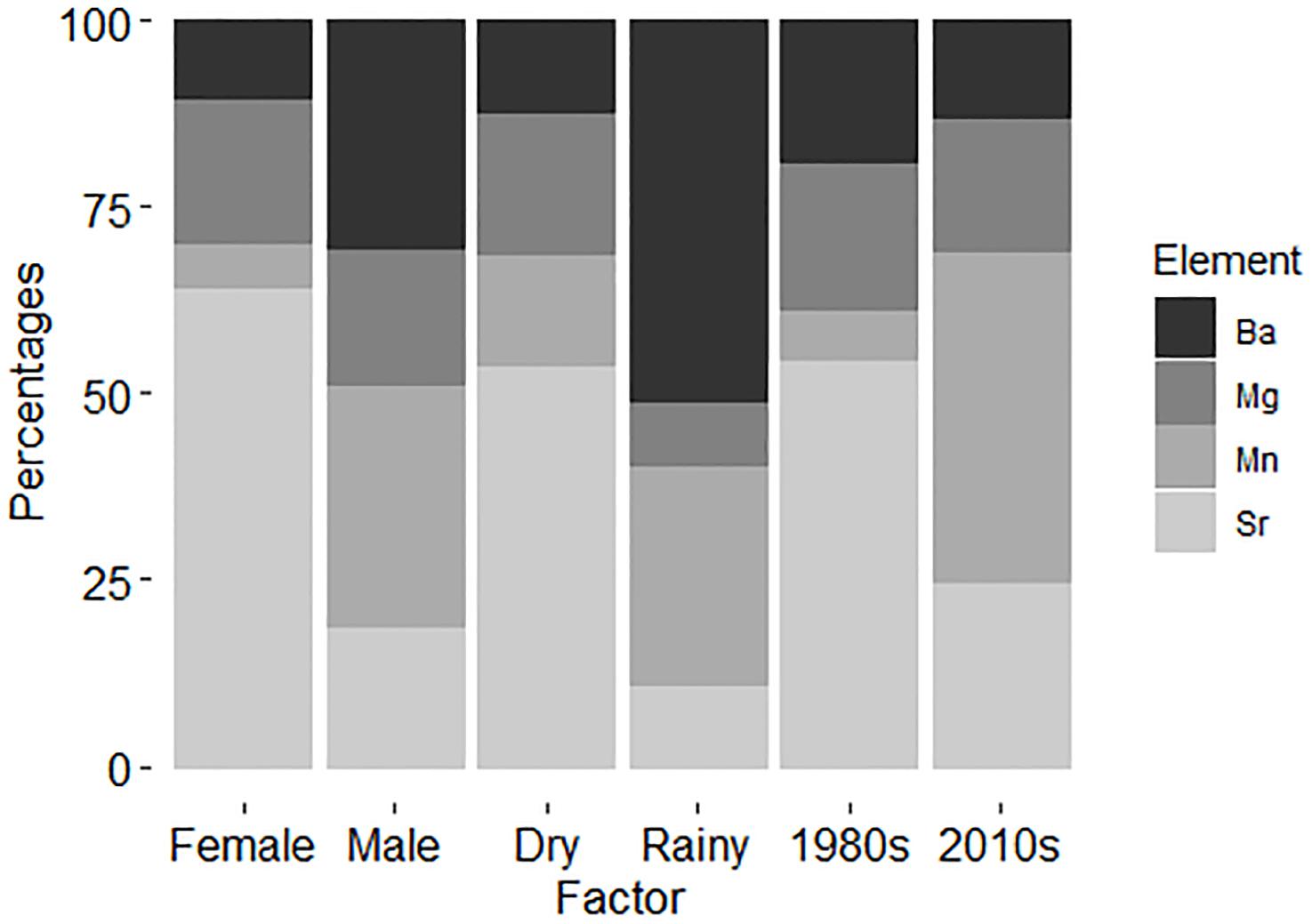
Figure 3. Similarity percentages (SIMPER) demonstrating the chemical signatures for the factors analyzed. Total n = 17 (sex – female: n = 7; male: n = 10), (seasons – dry: n = 12; rainy: n = 5), (sampling decades – 1980: n = 8; 2010: n = 9).
Cluster results with the multi-element signatures for the neonate phase demonstrated that individuals have different birthplaces. Indeed, the cluster analysis evidenced at least three birthing grounds based on the average chemical similarities between individuals with a cophenetic distance of 0.952 (Figure 4). Furthermore, since the locations in Figure 4 reflect the collection places of the adults instead of the actual birth areas, and the localities are intermingled among the clusters, the birthing grounds seem to be specific, but adults use the same larger area. Finally, the cluster shows the use of the same birthing grounds across decades since reads from samples collected in the 1980s and in 2017 and 2018 were gathered in the clusters (Table 2). Sample CP105 has a different chemical signature than all the other samples and likely represents an individual born in a different area.
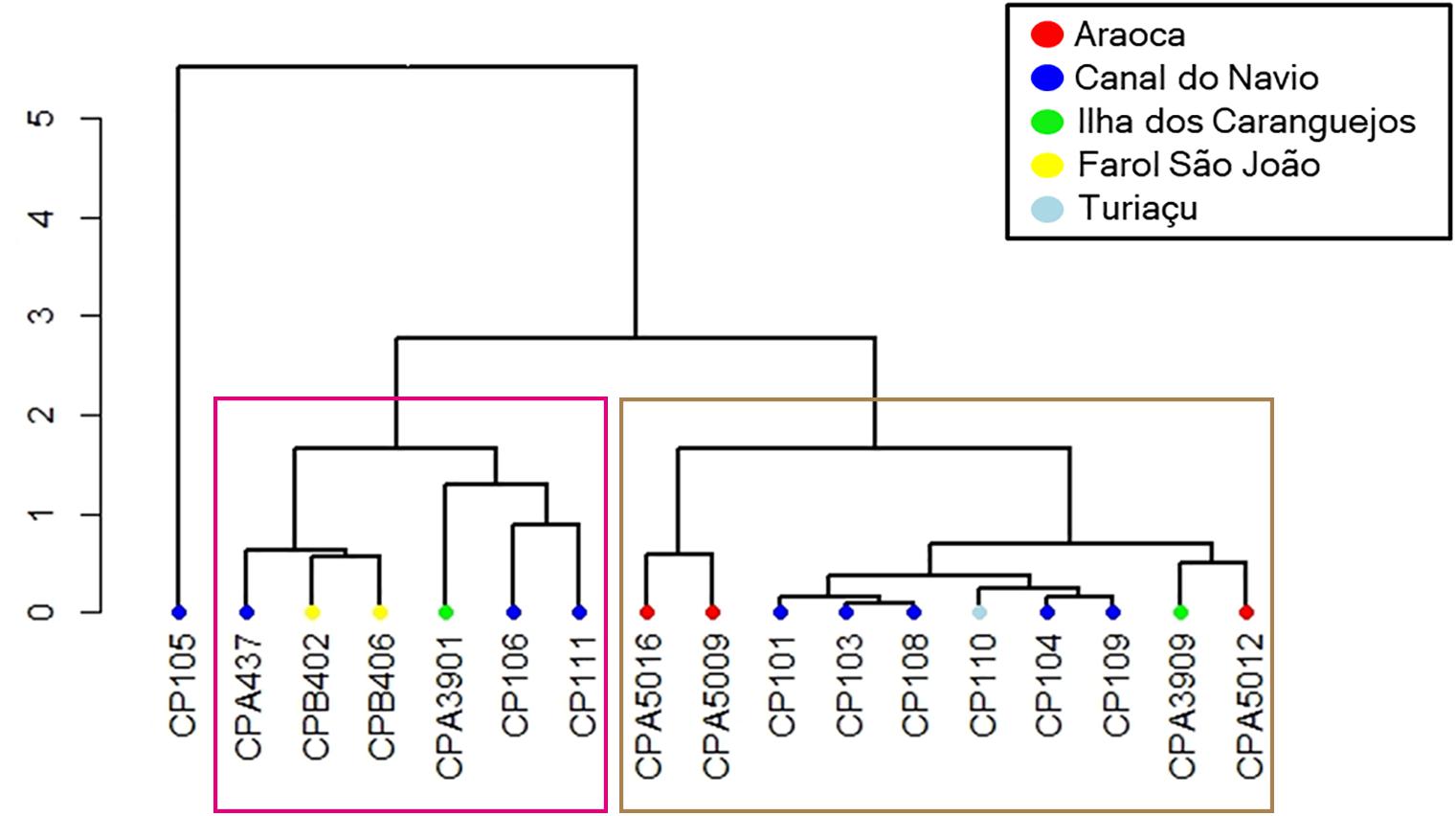
Figure 4. Cluster analysis for neonate multi-element chemical signatures. Sample codes are in the x-axis. Sites are the sampling points where adults were captured. Each branch tip color represents a sampling location: red, Araoca, blue, Canal do Navio, light blue, Turiaçu, green, Ilha dos Caranguejos, yellow, Farol São João. The two major clusters found are highlighted by the pink and brown rectangles.
Discussion
Patterns of Habitat Use Inferred From Vertebrae Microchemistry Data
Results demonstrated that the smalltail shark likely does not undertake habitat partitioning throughout its ontogeny (Table 3). However, multi and single-element PERMANOVAs separately testing differences between seasons, sexes, and decades yielded statistically significant differences. Overall, the PERMANOVA results suggest that all life stages of the C. porosus population use the whole area in a constant manner. However, the differences obtained demonstrate that the smalltail shark likely presents sex segregation, which is a somewhat widespread behavior among shark species (Wearmouth and Sims, 2010). Nevertheless, it is not clear if the specimens move further away from the coast or to deeper areas where salinity is expected to be higher in the rainy seasons due to predominance of freshwater in the surface.
Seasonality in the region is strong and the sex differences in multi-element signatures shown by the SIMPER analysis (Figure 3) seem to reinforce this argument, since Ba is much more representative for males. Indeed, Ba is an element known as a surrogate for the proximity to freshwater runoff areas (Smith et al., 2013). However, experimental fisheries catch data point to a greater proportion of females near the coast on the rainy season instead of males. Indeed, Ba has a greater importance for designating the typical chemical pattern in males than in females, as it does in the rainy season when compared to the dry season (Table 4). It is important to notice that, despite this higher importance, Sr is more abundant than Ba in both sexes and seasons (Table 4). Regardless, no evidence was obtained that C. porosus enters freshwater systems. In fact, geochemical data from within the Amazon River point toward similar levels of Sr and Ba on the edge of the saltwater intrusion area near Óbidos (Seyler and Boaventura, 2003). Although we found no information on the geochemical characteristics of the rivers that compose the larger Gulf of Maranhão area from where the specimens were collected, they have similar physical characteristics to the Amazon River, are subjected to the same weather pattern, and could have similar trace element compositions. However, further research to describe such chemical patterns in both the rivers and their estuaries is necessary.
Even though the SIMPER results demonstrate a drastic change in Sr percentages of contribution between the dry (higher) and rainy (lower) seasons, its single-element results yielded no significant differences between them. In fact, the incongruence between analyses is likely related to the higher variance for Ba, which was indeed statistically significant in the single-element analysis between seasons (Table 2). On the other hand, Mg was also significantly different between seasons in the single-element analysis. Since its concentration is considered to be negatively affected by temperature variations (Smith et al., 2013), we tested it to investigate potential dives for deeper areas in which water temperature would be significantly lower than in coastal waters. This behavior has been shown for several shark species (Afonso and Hazin, 2015), but never for a small coastal one. Indeed, no evidence of such dives were obtained for C. porosus, which is in accordance with the known biology of the species, thus reinforcing that its distribution is likely restricted to the coastal waters within the continental platform (Lessa et al., 1999a). However, there is also evidence pointing that Mg may suffer biological influences from diet and physiology on a species-specific basis (McMillan et al., 2017). Therefore, its results must be taken cautiously and further laboratory validation needs to be done.
Results from the Mn single-element analysis likely indicate that the species remains near the coast subjected to influences from mangrove habitat, since Mn is considered to be more abundant in coastal areas with mangrove forests nearby and no differences were obtained between life stages (Paillon et al., 2014). This result is also in accordance with the average values computed for Mn in Table 4. Furthermore, we obtained statistically significant differences between sexes for the Mn:Ca ratios as for the other elements in both single and multi-element analysis. Although manganese is also known to be a physiologically active element participating on the activation of reproductive hormones, as well as protein production and cellular signals (Smith et al., 2013), no differences in growth and age at maturity exists between sexes for C. porosus (Lessa et al., 1999a). While the hypothesis that Mn results may be affected by physiological features requires further testing, its results presented herein are evaluated on a more comprehensive array, especially in the cluster analysis discussed below.
Evaluation of Nursery Criteria and Suggestions for Effective Species Management
Nurseries have long been considered as one of the most critical areas for elasmobranch conservation since they provide key habitat for neonates and juveniles to grow and thus guarantee recruitment for the next generations (Lessa et al., 1999b). However, there have been several attempts to define what would be considered as a nursery, since these areas should be preserved to guarantee population recruitment (Beck et al., 2001; Heupel et al., 2007, 2018). Therefore, Heupel et al. (2007) developed three testable criteria to designate a given area as a nursery, which are: (1) newborns and young-of-year specimens must be more commonly found in the studied area than elsewhere, (2) must remain in that area for longer periods of time, and (3) the area must be repeatedly used across years. Furthermore, Heupel et al. (2018) have considered that the statement by Beck et al. (2001) that juveniles and adults should not coexist in a nursery to be true.
Recently, Heupel et al. (2018) revised the existing literature testing the criteria and stated that small-bodied coastal sharks tend not to have specific nursery areas. Indeed, applying those criteria to the gillnet capture and microchemistry data presented herein, the smalltail shark seems to follow this statement for four reasons. First, although the BNC has the highest occurrence of newborns and young-of-year specimens known in any other area along its geographical distribution (Lessa et al., 1999a, 2006), it is not the only area where they are found. In fact, other areas with known neonate and young-of-year captures in Brazil are Sergipe and São Paulo state’s coasts (Sadowsky, 1967; Meneses et al., 2005), and other such areas are likely to exist throughout its distribution. However, their numbers are still proportionately much lower than in the BNC.
Second, they seem to stay in the same area until reaching sexual maturity as shown by the experimental fisheries data. Even though these data (Table 1) show a somewhat sex segregation behavior, the lack of differences between life stages shown by the microchemistry data reinforce the idea that individuals remain in the same area throughout their lives. Therefore, the species whole life cycle is likely fulfilled in the nearshore waters of the BNC. Regardless, adult females are more abundant during the rainy season, especially in March when they correspond to twice the number of adult males caught in the area. On the other hand, the sex ratio is skewed toward adult males in the dry season, especially October (Table 1). Interestingly, the sex ratio between adult males and females becomes less skewed during June and July when the transition between the rainy and dry seasons occur. We argue that these differences in occurrence between adult individuals, together with the microchemistry results, are strong evidence that a seasonal habitat partitioning between sexes likely occurs in the BNC population.
However, fishing gear might also be responsible for these catch patterns. Experimental fisheries surveys carried out in the BNC with different gillnet sizes during the 1980s and 1990s have conflicting results. Lessa et al. (1999a), using gillnets 900 m long (7.5 m in height and 80 mm mesh size between knots) caught 1,128 specimens between 1984 and 1987 from which ∼80% comprised neonates or juveniles. On the other hand, Stride et al. (1992) only caught adult individuals (N = 78) on the same areas using gillnets with larger mesh sizes (200 mm between knots, 400 m long, and 6 m height) than the ones used by Lessa et al. (1999a). Furthermore, increasing mesh sizes resulted in a decrease in capture of C. porosus from 14% in the 200 mm mesh to 0.8% in the 300 mm mesh size (Stride et al., 1992). Thus, gear selectivity is an important source of bias for the life stage occurrences observed. In addition, specimens were most commonly caught in areas where salinity tended to be higher, but no information on seasonal changes in captures are provided (Stride et al., 1992).
Regardless, Stride et al. (1992) caught a large number of pregnant females (n = 10). Among those samples, six were caught in May and most had near-term embryos (size at birth at 30 cm TL). This information, together with the existing reproductive, catch, and habitat use data for the species led us to argue that most C. porosus females likely give birth in the second trimester (rainy season), and copulation likely takes place in October with females that are resting from gestation from the previous year. Further evidence for that is the capture of an adult female used in the present study (individual CP111) with fresh mating scars in October 2018 (Supplementary Figure S1).
Third, the existence of at least two groups in the cluster analysis (Figure 4), as well as the grouping of samples captured in the 1980s and 2017/2018 point toward the existence of reused birthing grounds and possible philopatric behavior. Future research should focus on catching neonates and juveniles and evaluating the vertebral edge microchemistry signature to compare with the ones obtained in the present study to assign accurately each one to a specific birthing ground. Molecular studies through genotype reconstruction or long-term telemetry of adult females are also effective tools to prove this hypothesis. Furthermore, the coexistence of adults born in areas with diverging chemical signatures (Figure 4) reinforces the hypothesis that the BNC’s smalltail sharks comprise a single population and thus should be managed as a single unit.
Fourth, young-of-year, juveniles, subadults, and adults coexist. Despite the neonates and juveniles being completely absent from areas deeper than 20 m, individuals from all life stages inhabit the shallow coastal waters of the BNC (Lessa and Santana, 1998). Therefore, we argue that the BNC, especially the eastern Amazon coast in Maranhão state, does not fulfill the elasmobranch nursery criteria. Additionally, the species likely spends its whole life cycle within the continental platform, but with a small degree of habitat partitioning between sexes and several different birthing grounds. Furthermore, recent analysis estimated that the Northern coast of South America (defined as the coastal extension between Guyana and Maranhão state) is actually the region with the smallest decline in catch probability along the species distribution (Feitosa et al., 2020). The last IUCN species assessment also considers this area as the center of abundance for this species (Lessa et al., 2006). Therefore, based on the evidence herein presented and the published information for this species, we argue that the BNC is actually an Essential Fish Habitat (EFH) area for C. porosus, especially the shallow coastal banks and estuarine areas of the BNC as noted by Lessa et al. (1999a).
While the BNC is likely not a nursery for the C. porosus, it seems to be a much more important area for this species. Knip et al. (2010) developed a hypothesis of how small-sized sharks, such as C. porosus, use their habitats. The authors argue that such species tend to be highly dependent on nearshore areas and typically do not roam very far, especially for the high abundance of prey. This was the case for C. sorrah, another small-sized tropical shark inhabiting nearshore waters off Australia, which showed some degree of site-fidelity but some individuals did roam to further areas (Knip et al., 2012a). Further evidence supporting this conclusion is that C. porosus is an opportunistic species that feeds mainly on small teleosts such as Stellifer naso and Macrodon ancylodon, which are highly abundant in the area regardless of season (Lessa and Almeida, 1997). Therefore, C. porosus seems to fit the theoretical model for small-sized sharks developed by Knip et al. (2010).
Since C. porosus seems to have a restricted dispersion capacity due to its small size, it is reasonable to assume that individuals in the BNC are a single population, but connectivity with adjacent areas such as the Guyanas and Suriname need to be further investigated. Regardless, evidence points to a low genetic diversity scenario in the BNC population (Tavares et al., 2013), which is in agreement with both the critically endangered status in Brazil and the lack of connectivity between the BNC population and individuals from other areas. In fact, this pattern is likely a life history feature for this species, since several local extinctions have been reported throughout its range in Brazil (Lessa et al., 2018). Nevertheless, roaming capacity for this species needs to be further investigated. This is especially important due to the fisheries interactions that populations may experience. If individuals have little roaming behavior, localized populations are more likely to be severely impacted by fisheries in areas where fishing pressure is strong, such as the BNC (Knip et al., 2010, 2012b).
Improvement Suggestions for Future Microchemistry Studies at Low Latitudes
Although elasmobranch vertebrae microchemistry has proven to be a robust technique to evaluate habitat use patterns (McMillan et al., 2017), there is not enough information on the physiological processes involved in element uptake by the vertebrae. Indeed, few studies have been carried out in controlled environments evaluating the effects of biotic (diet, physiology) and abiotic factors (salinity, temperature, pH) on element concentrations (Smith et al., 2013; Pistevos et al., 2019). In addition, elasmobranch microchemistry studies are mostly carried out with temperate species subjected to a much more variable environment with strong seasonality. Therefore, the published literature is not always in accordance with the environmental characteristics of the tropics. Consequently, it is a lot harder for researchers studying tropical species to draw strong conclusions about their results based on literature specialized in species from regions subjected to temperate climate.
Regardless, it is clear that a lot more research in both tropical and temperate areas is necessary to fill the major elasmobranch microchemistry knowledge gaps pointed by McMillan et al. (2017). Therefore, we discuss what we consider the next frontiers for elasmobranch vertebrae microchemistry research carried out with tropical sharks and rays. We also raise two questions regarding the main knowledge gaps for elasmobranch microchemistry based on the published literature and our data.
First, which elements are capable of assessing finer-scale changes in habitat use for species subjected to little environmental variation? The smalltail shark might indeed have little variation in habitat use and, since the habitat environmental characteristics are generally stable over time, the trace elements we analyzed were not capable of detecting fine scale changes, such as salinity differences detected by Tillett et al. (2011). Therefore, we reinforce the need to study element uptake physiology in depth and to validate the environmental signal each element provides, especially for species occurring in low latitudes. A potential candidate might be sulfur, which could be a successful element to evaluate horizontal migrations and distance from the coast (see Rubenstein and Hobson, 2004; Doubleday et al., 2018 for further information), since it seems to reflect organic matter concentration in the water.
Second, what is the effect of metabolic rate on the time it takes for a given water chemical signature to be marked on the vertebrae? To our knowledge, the only study ever carried out investigating how growth and environmental history affected elemental concentration in the vertebrae was done by Smith et al. (2013). As expected, specimens in higher temperatures had a significantly higher growth rate when compared to those at lower temperatures, as well as the deposition rate. However, growth rates did not have a significant effect on elemental composition (Smith et al., 2013). Therefore, it is expected that specimens occurring in low latitudes have higher growth and vertebrae deposition rates. Nevertheless, few conclusions regarding the effects of temperature on vertebrae growth and element composition on adults can be drawn, since this experiment was performed with juvenile individuals of Urobatis halleri. Thus, the question of how much time is necessary for the chemistry of a given water mass to have a distinct signature on the vertebrae remains. In fact, this is such a difficult topic that the same question exists for otoliths, even though some data point to a timeframe between 25 and 40 days for a signature to be formed (Walsh and Gillanders, 2018).
Another important factor to consider is the difference in growth rate during ontogenetic development (Walther and Limburg, 2012). Indeed, C. porosus is expected to grow 7 cm.year–1 on the first 4 years of life, and decreasing to 4 cm.year–1 from then on (Lessa et al., 1999a). Therefore, it is likely that the chemical signals from the subadult2 phase and further are subjected to different metabolic and growth rates. Furthermore, this pattern is likely to be species-specific, since each has different growth rates and life histories. Perhaps a good alternative to overcome this problem would be to choose a model species of relatively easy rearing in controlled environments for each major taxonomic group of sharks (family Carcharhinidae, Lamnidae, etc.). Studying these models would potentially enable researchers to extrapolate the results obtained to the other species from the same group, thus obtaining stronger interpretations from the data.
Conclusion
In general, our vertebrae microchemistry results suggest that the smalltail shark population occurring in the BNC is a single stock with different birthing grounds. This was the first study employing vertebrae microchemistry on an elasmobranch in the Neotropical region, and we recommend a few methodological advancements based on both our data and knowledge gaps we identified in the published literature. Since C. porosus spends its whole life cycle in the coastal waters and abundances seem to be much higher in the BNC than any other area of its known distribution, the region is a crucial area for its conservation on a global scale. A short-term feasible conservation strategy to mitigate its bycatch in fisheries targeting two of the most important Brazilian fishing resources could be the release of live specimens, especially those under 70 cm TL and hence sexually immature. It is worth noting that, even though their productivity has been declining quickly, it is impossible to ban the target fisheries, since several communities depend on them for subsistence. Therefore, managing the target fisheries will likely have a positive effect on C. porosus populations. However, since elasmobranchs have a longer life cycle, these management strategies would require a longer time to rebuild its stock. Another feasible and perhaps more crucial action would be to implement effectively the existing conservation areas throughout the BNC, notably in Maranhão state. Finally, the legal framework, especially to enable in situ inspections, adequate deterrent measures for prohibited catches, and the existence of fisheries statistic programs along the BNC are crucial to monitor trends in geographical occurrence and stock size.
Data Availability Statement
The datasets generated for this study are available on request to the corresponding author.
Ethics Statement
Ethical review and approval was not required for the animal study because sampling involved only already fished specimens. Other animals used in this study were sampled between 1984 and 1989, when no Ethical standards existed in Brazil, thus no Ethics Committee approval exists for them.
Author Contributions
LF participated on the study design, field sampling, vertebrae processing, aging, and preparation for the LA-ICP-MS analysis, as well as analyzed the data, and wrote the manuscript. VD participated on the LA-ICP-MS laboratory work, provided key insights on data analysis, and reviewed the manuscript. RL participated on the study design, sample preparation and analysis on the LA-ICP-MS, data analysis, provided the experimental fisheries catch data used, and reviewed the manuscript.
Funding
This study was financed in part by the Coordenação de Aperfeiçoamento de Pessoal de Nivel Superior – Brazil (CAPES) – Finance code 001. Comissão Interministerial para os Recursos do Mar (CIRM) funded the data collection along the Maranhão Coast (1984/1988) and Conselho Nacional de Desenvolvimento Científico e Tecnológico – CNPq provided Productivity Research Grants (306672/2015-4 and 302276/2017-3) to RL and VD, respectively. In addition, we acknowledge the help of Vinicius M. Neves in the LA-ICP-MS processing, and Mariana Bonfim in the data analysis.
Conflict of Interest
The authors declare that the research was conducted in the absence of any commercial or financial relationships that could be construed as a potential conflict of interest.
Acknowledgments
We thank FACEPE and CNPq under the projects APQ 10.11-2015 and 306672/15, respectively, for covering the costs of the LA-ICP-MS analysis, FACEPE for providing a scholarship to LF under the process IBPG 0089-2.05/17. We also thank Dr. Francisco Marcante Santana for the help with reading growth rings and aging specimens, and the thorough discussions about the results, Dr. Ana Paula Barbosa Martins for her thoughts on the contents of this manuscript. In addition, we acknowledge the help of Vinicius M. Neves in the LA-ICP-MS 1099 processing, and Mariana Bonfim and Dr. Jonas Vasconcelos in the data analysis. Furthermore, we thank Leandro Augusto de Souza Junior, Dr. Leandro Manzoni Vieira, Clarisse Éleres Figueiredo, and Rafael Antonio Brandão for helping with figure editing and elaboration, and Maylis Labonne (IRD, France) for critical readings. Finally, we thank Dr. Jorge Nunes, Dr. Getúlio Rincon, and the fishers from Raposa for the help with sample collection.
Supplementary Material
The Supplementary Material for this article can be found online at: https://www.frontiersin.org/articles/10.3389/fmars.2020.00125/full#supplementary-material
FIGURE S1 | Mating scars on the left pectoral fin of individual CP111.
References
Afonso, A. S., and Hazin, F. H. V. (2015). Vertical movement patterns and ontogenetic niche expansion in the tiger shark, Galeocerdo cuvier. PLoS One 10:e0116720. doi: 10.1371/journal.pone.0116720
Almerón-Souza, F., Sperb, C., Castilho, C. L., Figueiredo, P. I. C. C., Gonçalves, L. T., Machado, R., et al. (2018). Molecular identification of shark meat from local markets in Southern Brazil based on DNA barcoding: evidence for mislabeling and trade of endangered species. Front. Genet. 9:138. doi: 10.3389/fgene.2018.00138
Barreto, R. R., Bornatowski, H., Motta, F. S., Santander-Neto, J., Vianna, G. M. S., and Lessa, R. (2017). Rethinking use and trade of pelagic sharks from Brazil. Mar. Policy 85, 114–122. doi: 10.1016/j.marpol.2017.08.016
Beck, M. W., Heck, K. L., Able, K. W., Childers, D. L., Eggleston, D. B., Gillanders, B. M., et al. (2001). The identification, conservation, and management of estuarine and marine nurseries for fish and invertebrates. BioScience 51, 633–641.
Compagno, L. J. V. (1984). FAO Species Catalogue. Sharks of the World, Part 2 – Carcharhiniformes, Vol. 4. Rome: Food and Agriculture Organization of the United Nations, 251–633.
Cochrane, K. L. (2002). A Fishery Manager’s Guidebook: Management Measures and Their Applications, ed. K. L. Cochrane (Rome: Food and Agriculture Organization).
de Almeida, Z. S., de Santos, N. B., Carvalho-Neta, R. N. F., and de Pinheiro, A. L. R. (2014). “Análise multidisciplinar das pescarias de emalhe da pescada-amarela, de camarão de puçá de muruada e da catação de caranguejo uçá em três municípios costeiros do Maranhão,” in A Pesca Marinha e Estuarina no Brasil: Estudos de Caso Multidisciplinares, eds M. Haimovici, J. M. Sunye, and P. S. Andriguetto Filho (Rio Grande: Editora da FURG), 161–170.
Davidson, L. N. K., and Dulvy, N. K. (2017). Global marine protected areas to prevent extinctions. Nat. Ecol. Evol. 1:0040. doi: 10.1038/s41559-016-0040
de Pontual, H., and Geffen, A. J. (2002). “Otolith microchemistry,” in Manual of Fish Sclerochronology, eds J. Panfili, H. Pontual, H. Troadec, and P. J. Wright (Brest: Ifremer), 245–307. doi: 10.1643/OT-03-266
Doubleday, Z. A., Cliff, J., Izzo, C., and Gillanders, B. M. (2018). Untapping the potential of sulfur isotope analysis in biominerals. Mar. Ecol. Prog. Ser. 598, 159–166. doi: 10.3354/meps12605
Dulvy, N. K., Fowler, S. L., Musick, J. A., Cavanagh, R. D., Kyne, P. M., Harrison, L. R., et al. (2014). Extinction risk and conservation of the world’s sharks and rays. eLife 3:e00590. doi: 10.7554/eLife.00590
Elsdon, T., Wells, B., Campana, S., Gillanders, B., Jones, C., Limburg, K., et al. (2008). “Otolith chemistry to describe movements and life-history parameters of fishes,” in Oceanography and Marine Biology: An Annual Review, 46th Edn, eds R. N. Gibson, R. J. A. Atkinson, and J. D. M. Gordon (Boca Raton, FL: CRC Press), 297–330. doi: 10.1201/9781420065756.ch7
Feitosa, L. M., Martins, A. P. B., Giarrizzo, T., Macedo, W., Monteiro, I. L., Gemaque, R., et al. (2018). DNA-based identification reveals illegal trade of threatened shark species in a global elasmobranch conservation hotspot. Sci. Rep. 8:3347. doi: 10.1038/s41598-018-21683-5
Feitosa, L. M., Martins, L. P. M., de Souza-Junior, L. A., and Lessa, R. P. (2020). Potential distribution and population trends of the smalltail shark Carcharhinus porosus inferred from species distribution models and historical catch data. Aquat. Conserv. doi: 10.1002/aqc.3293
Fraser, K. C., Davies, K. T., Davy, C. M., Ford, A. T., Flockhart, D. T. T., and Martins, E. G. (2018). Tracking the conservation promise of movement ecology. Front. Ecol. Evol. 6:150. doi: 10.3389/FEVO.2018.00150
Gillanders, B. M. (2005). Otolith chemistry to determine movements of diadromous and freshwater fish. Aquat. Living Resour. 18, 291–300. doi: 10.1051/alr:2005033
Hazin, F. H. V., Afonso, A. S., De Castilho, P. C., Ferreira, L. C., and Rocha, B. C. L. M. (2013). Regional movements of the tiger shark, Galeocerdo cuvier, off Northeastern Brazil: inferences regarding shark attack hazard. An. Acad. Bras. Ciênc. 85, 1053–1062. doi: 10.1590/S0001-37652013005000055
Hermann, T. W., Stewart, D. J., Limburg, K. E., Castello, L., and Hermann, T. W. (2016). Unravelling the life history of Amazonian fishes through otolith microchemistry. R. Soc. Open Sci. 3:160206. doi: 10.1098/rsos.160206
Heupel, M. R., Carlson, J. K., and Simpfendorfer, C. A. (2007). Shark nursery areas: concepts, definition, characterization and assumptions. Mar. Ecol. Prog. Ser. 337 287–297. doi: 10.3354/meps337287
Heupel, M. R., Kanno, S., Martins, A. P. B., and Simpfendorfer, C. A. (2018). Advances in understanding the roles and benefits of nursery areas for elasmobranch populations. Mar. Freshw. Res. 70, 897–907. doi: 10.1071/MF18081
Izzo, C., Huveneers, C., Drew, M., Bradshaw, C. J. A., Donnellan, S. C., and Gillanders, B. M. (2016). Vertebral chemistry demonstrates movement and population structure of bronze whaler. Mar. Ecol. Prog. Ser. 556, 195–207. doi: 10.3354/meps11840
Knip, D. M., Heupel, M. R., and Simpfendorfer, C. A. (2010). Sharks in nearshore environments: models, importance, and consequences. Mar. Ecol. Prog. Ser. 402, 1–11. doi: 10.3354/meps08498
Knip, D. M., Heupel, M. R., and Simpfendorfer, C. A. (2012a). Evaluating marine protected areas for the conservation of tropical coastal sharks. Biol. Conserv. 148, 200–209. doi: 10.1016/j.biocon.2012.01.008
Knip, D. M., Heupel, M. R., and Simpfendorfer, C. A. (2012b). Habitat use and spatial segregation of adult spottail sharks Carcharhinus sorrah in tropical nearshore waters. J. Fish Biol. 80, 767–784. doi: 10.1111/j.1095-8649.2012.03223.x
Lessa, R., and Almeida, Z. (1997). Analysis of stomach contents of the smalltail shark Carcharhinus porosus from northern Brazil. Cybium 21, 123–133.
Lessa, R., Almeida, Z., Santana, F. M., Siu, S., and Perez, M. (2006). Carcharhinus Porosus Smalltail Shark. The IUCN Red List of Threatened Species, 8235. Available online at: https://dx.doi.org/10.2305/IUCN.UK.2006.RLTS.T60220A12324372.en (accessed July 26, 2017).
Lessa, R., Batista, V. S., and Santana, F. M. (2016). Close to extinction? The collapse of the endemic daggernose shark (Isogomphodon oxyrhynchus) off Brazil. Glob. Ecol. Conserv. 7, 70–81. doi: 10.1016/j.gecco.2016.04.003
Lessa, R., and Santana, F. M. (1998). Age determination and growth of the smalltail shark, Carcharhinus porosus, from northern Brazil. Mar. Freshw. Res. 49, 705–711. doi: 10.1071/MF98019
Lessa, R., Santana, F., Menni, R., and Almeida, Z. (1999a). Population structure and reproductive biology of the smalltail shark (Carcharhinus porosus) off Maranhão (Brazil). Mar. Freshw. Res. 50, 383–388.
Lessa, R., Santana, F. M., Rincón, G., Gadig, O. B. F., and El-Deir, A. C. D. (1999b). Biodiversidade de Elasmobrânquios do Brasil. Recife: Ministério do Meio Ambiente.
Lessa, R. P. (1986). Contribuição ao conhecimento da biologia de carcharhinus porosus ranzani, 1839 das reentrâncias maranhenses. Acta Amaz. 16/17, 73–86.
Lessa, R. P. (1997). Sinopse dos estudos sobre elasmobrânquios da costa do Maranhão. Bol. Lab. Hidrobiol. 10, 19–36.
Lessa, R. P. T., Repinaldo-Filho, F. P. M., Moro, G., Charvet, P., and Santana, F. M. (2018). “Carcharhinus porosus,” in Livro Vermelho da Fauna Brasileira Ameaçada de Extinção, Vol. 6Peixes (Brasília: ICMBio), 950–953.
Lewis, J. P., Patterson, W. F., Carlson, J. K., and McLachlin, K. (2016). Do vertebral chemical signatures distinguish juvenile blacktip shark (Carcharhinus limbatus) nursery regions in the northern Gulf of Mexico? Mar. Freshw. Res. 67, 1014–1022. doi: 10.1071/MF15088
Longerich, H. P., Jackson, S. E., and Günther, D. (1996). Inter-laboratory note. Laser ablation inductively coupled plasma mass spectrometric transient signal data acquisition and analyte concentration calculation. J. Anal. At. Spectrom. 11, 899–904. doi: 10.1039/JA9961100899
McMillan, M. N., Huveneers, C., Semmens, J. M., and Gillanders, B. M. (2018). Natural tags reveal populations of conservation dependent school shark use different pupping areas. Mar. Ecol. Prog. Ser. 599, 147–156. doi: 10.3354/meps12626
McMillan, M. N., Izzo, C., Wade, B., and Gillanders, B. M. (2017). Elements and elasmobranchs: hypotheses, assumptions and limitations of elemental analysis. J. Fish Biol. 90, 559–594. doi: 10.1111/jfb.13189
Meneses, T. S., Santos, F. N., and Pereira, C. W. (2005). Fauna De Elasmobrânquios Do Litoral Do Estado De Sergipe, Brasil. Arq. Ciênc. Mar. 38, 79–83. doi: 10.2312/ActaFish.2017.5.1.33-42
Menni, R. C., and Lessa, R. P. (1998). The chondrichthyan community off Maranhão (northeastern Brazil) II. Biology of species. Acta Zool. Lilloana 44, 69–89.
Mohan, J. A., Miller, N. R., Herzka, S. Z., Sosa-nishizaki, O., Kohin, S., Dewar, H., et al. (2018). Elements of time and place: manganese and barium in shark vertebrae reflect age and upwelling histories. Proc. R. Soc. B Biol. Sci. 285:20181760. doi: 10.1098/rspb.2018.1760
Mohan, J. A., TinHan, T. C., Miller, N. R., and David Wells, R. J. (2017). Effects of sample cleaning and storage on the elemental composition of shark vertebrae. Rapid Commun. Mass Spectrom. 31, 2073–2080. doi: 10.1002/rcm.7998
Mourão, K. R. M., do Espírito-Santo, R. V., Silva, B. B., Almeida, M. C., Isaac, V. J., Frédou, T., et al. (2014). “A pesca de scomberomorus brasiliensis e alternativas para o seu manejo no litoral nordeste do Pará – Brasil,” in A Pesca Marinha e Estuarina no Brasil: Estudos de Caso Multidisciplinares, eds M. Haimovici, J. M. Andriguetto, and P. S. Filho Sunye (Rio Grande: Editora da FURG), 171–180.
Oliver, S., Braccini, M., Newman, S. J., and Harvey, E. S. (2015). Global patterns in the bycatch of sharks and rays. Mar. Policy 54, 86–97. doi: 10.1016/j.marpol.2014.12.017
Paillon, C., Wantiez, L., Kulbicki, M., Labonne, M., and Vigliola, L. (2014). Extent of mangrove nursery habitats determines the geographic distribution of a coral reef fish in a South-Pacific Archipelago. PLoS One 9:e105158. doi: 10.1371/journal.pone.0105158
Palmeira, C. A. M., da Rodrigues-Filho, L. F. S., de Sales, J. B. L., Vallinoto, M., Schneider, H., and Sampaio, I. (2013). Commercialization of a critically endangered species (largetooth sawfish, Pristis perotteti) in fish markets of northern Brazil: authenticity by DNA analysis. Food Control 34, 249–252. doi: 10.1016/j.foodcont.2013.04.017
Pistevos, J. C. A., Reis-Santos, P., Izzo, C., and Gillanders, B. M. (2019). Element composition of shark vertebrae shows promise as a natural tag. Mar. Freshw. Res. 70, 1722–1733. doi: 10.1071/MF18423
R Core Team (2013). R: A Language and Environment for Statistical Computing. Vienna: R Foundation for Statistical Computing.
Rubenstein, D. R., and Hobson, K. A. (2004). From birds to butterflies: animal movement patterns and stable isotopes. Trends Ecol. Evol. 19, 256–263. doi: 10.1016/j.tree.2004.03.017
Sadowsky, V. (1967). Selachier aus dem Litoral von Sao Paulo, Brasilien. Beitr. Neotropischen Fauna 5, 71–88. doi: 10.1080/01650526709360398
Scharer, R. M., Patterson, W. F., Carlson, J. K., and Poulakis, G. R. (2012). Age and growth of endangered smalltooth sawfish (Pristis pectinata) verified with LA-ICP-MS analysis of vertebrae. PLoS One 7:e47850. doi: 10.1371/journal.pone.0047850
Seyler, P. T., and Boaventura, G. R. (2003). Distribution and partition of trace metals in the Amazon basin. Hydrol. Process. 17, 1345–1361. doi: 10.1002/hyp.1288
Smith, W. D., Miller, J. A., and Heppell, S. S. (2013). Elemental markers in elasmobranchs: effects of environmental history and growth on vertebral chemistry. PLoS One 8:e62423. doi: 10.1371/journal.pone.0062423
Smith, W. D., Miller, J. A., Márquez-Farías, J. F., and Heppell, S. S. (2016). Elemental signatures reveal the geographic origins of a highly migratory shark: prospects for measuring population connectivity. Mar. Ecol. Prog. Ser. 556, 173–193. doi: 10.3354/meps11844
Souza-Filho, P. W. M. (2005). Costa de Manguezais de Macromaré da Amazônia: cenários morfológicos, mapeamento e quantificação de áreas usando dados de sensores remotos. Rev. Bras. Geofís. 23, 427–435. doi: 10.1590/s0102-261x2005000400006
Stride, R. K., da Batista, V. S., and Raposo, L. A. B. (1992). Pesca Experimental de Tubarão com Redes de Emalhar no Litoral Maranhense, 3rd Edn. São Luís: CORSUP/EDUFMA.
Tavares, W., da Silva Rodrigues-Filho, L. F., Sodré, D., Souza, R. F. C., Schneider, H., Sampaio, I., et al. (2013). Multiple substitutions and reduced genetic variability insharks. Biochem. Syst. Ecol. 49, 21–29. doi: 10.1016/j.bse.2013.02.004
Taylor, M. D., Babcock, R. C., Simpfendorfer, C. A., and Crook, D. A. (2017). Where technology meets ecology: acoustic telemetry in contemporary Australian aquatic research and management. Mar. Freshw. Res. 68, 1397–1402. doi: 10.1071/MF17054
Tillett, B. J., Meekan, M. G., Parry, D., Munksgaard, N., Field, I. C., Thorburn, D., et al. (2011). Decoding fingerprints: elemental composition of vertebrae correlates to age-related habitat use in two morphologically similar sharks. Mar. Ecol. Prog. Ser. 434, 133–142. doi: 10.3354/meps09222
Walsh, C. T., and Gillanders, B. M. (2018). Extrinsic factors affecting otolith chemistry – implications for interpreting migration patterns in a diadromous fish. Environ. Biol. Fishes 101, 905–916. doi: 10.1007/s10641-018-0746-y
Walther, B. D. (2019). The art of otolith chemistry: interpreting patterns by integrating perspectives. Mar. Freshw. Res. 70, 1643–1658. doi: 10.1071/MF18270
Walther, B. D., and Limburg, K. E. (2012). The use of otolith chemistry to characterize diadromous migrations. J. Fish Biol. 81, 796–825. doi: 10.1111/j.1095-8649.2012.03371.x
Keywords: Carcharhinidae, Brazil’s Northern Coast, movement ecology, vertebrae trace elements, tropical coastal shark
Citation: Feitosa LM, Dressler V and Lessa RP (2020) Habitat Use Patterns and Identification of Essential Habitat for an Endangered Coastal Shark With Vertebrae Microchemistry: The Case Study of Carcharhinus porosus. Front. Mar. Sci. 7:125. doi: 10.3389/fmars.2020.00125
Received: 28 September 2019; Accepted: 17 February 2020;
Published: 06 March 2020.
Edited by:
Alejandra Vanina Volpedo, University of Buenos Aires, ArgentinaReviewed by:
Oscar Sosa-Nishizaki, Ensenada Center for Scientific Research and Higher Education (CICESE), MexicoBree J. Tillett, The University of Queensland, Australia
Copyright © 2020 Feitosa, Dressler and Lessa. This is an open-access article distributed under the terms of the Creative Commons Attribution License (CC BY). The use, distribution or reproduction in other forums is permitted, provided the original author(s) and the copyright owner(s) are credited and that the original publication in this journal is cited, in accordance with accepted academic practice. No use, distribution or reproduction is permitted which does not comply with these terms.
*Correspondence: Leonardo Manir Feitosa, lmfeitos@gmail.com