- 1Department of Veterinary Medicine, University of Alaska Fairbanks, Fairbanks, AK, United States
- 2Department of Chemistry and Biochemistry, University of Alaska Fairbanks, Fairbanks, AK, United States
- 3Center for Wildlife Health, The University of Tennessee, Knoxville, Knoxville, TN, United States
- 4Kronotsky State Nature Biosphere Reserve, Kamchatka Krai, Russia
- 5ExxonMobil Upstream Research Company, Houston, TX, United States
- 6Shell Global Solutions International BV, The Hague, Netherlands
- 7The Institute of Environmental and Human Health, Texas Tech University, Lubbock, TX, United States
- 8Veterinary Integrative Biosciences, College of Veterinary Medicine & Biomedical Sciences, Texas A&M University, College Station, TX, United States
The gray whale (Eschrichtius robustus) is recognized as two geographically isolated and somewhat genetically distinct populations, with recent evidence showing exchange that questions the degree of isolation. The western gray whale subpopulation is currently listed as critically endangered, as designated by the IUCN, whereas the eastern North Pacific population is listed by the IUCN as “Least Concern.” The International Whaling Commission has stressed the value of studies on feeding ecology and contaminants for the western subpopulation. Our objective was to test for differences among epidermal tissue strata as part of an optimization effort to best use and interpret data from small biopsy samples. Fresh to moderately decomposed full thickness samples of epidermis and dermis were collected from stranded eastern gray whales along the California coast, United States (N: 14), and biopsy samples (N: 10) were collected from free-ranging western gray whales. We determined 13C and 15N stable isotope ratios (δ13C, δ15N); and total Hg concentrations ([THg]) from histologically different strata of epidermal tissue samples from the eastern gray whale population The information from these assessments (stranded whale samples) were directly applied for determining δ13C and δ15N from biopsies collected remotely from free-ranging western gray whales, as well as evaluating [THg]. We found a significant difference in [THg] values between the different strata. This has implications for analyzing Hg, and possibly other elements, from an epidermal biopsy from a free-ranging gray whale, and we recommend identifying which stratum/strata the biopsy represents. There were no significant differences in δ13C and δ15N values between the different strata, making epidermal biopsies from free-ranging gray whales an ideal sampling method to investigate δ13C and δ15N ratios regardless of strata representation.
Introduction
Gray whales (Eschrichtius robustus) inhabit shallow coastal waters in the eastern and western coastlines of the North Pacific and are recognized as two geographically isolated and somewhat genetically distinct populations (Bradford et al., 2008; Bickham et al., 2013; Brüniche-Olsen et al., 2018a), with recent evidence showing some exchange (Weller et al., 2012; Lang et al., 2014; Mate et al., 2015; Brüniche-Olsen et al., 2018a). The western gray whale subpopulation is currently listed as critically endangered, as designated by the IUCN, whereas the eastern North Pacific population is listed by the IUCN as “Least Concern” (IUCN, 2012). The International Whaling Commission (IWC) and the IUCN have stressed the value of studies on factors that could impact recovery of the western subpopulation (Bickham et al., 2013). A research program to evaluate the western population’s biology was initiated in 1997 as a collaboration between Russian and United States scientists. The study is focused around the primary western gray whale feeding ground, which is located off the northeastern coast of Sakhalin Island, Russia, in the Okhotsk Sea, and is considered to provide access to most of the western gray whale population (Bradford et al., 2008; Bickham et al., 2013; Brüniche-Olsen et al., 2018b).
Some contaminants are known to bioaccumulate and biomagnify in marine mammals in the North Pacific and Arctic (Dietz et al., 2013). Hg concentrations have increased in Arctic environments during the past 150 years, leading to more than 92% of Hg body burden in higher trophic level species possibly originating from anthropogenic activities (Dietz et al., 2009). The relative longevity of gray whales sets the stage for bioaccumulation, but gray whales primarily feed on lower trophic levels of benthic invertebrates (Dehn et al., 2006a, b; Horstmann-Dehn et al., 2012) as compared to piscivorous cetaceans well known to have much higher Hg tissue concentrations. It is challenging to measure adverse effects from Hg in free-ranging species, but reports from pinnipeds (Van-Hoomissen et al., 2015; Kennedy et al., 2019) suggests an increase in abnormal behavior and a decrease in haptoglobin levels, associated with increased Hg concentrations. Previous reports have documented Hg concentrations in subsistence hunted gray whales (Tilbury et al., 2002; Dehn et al., 2006a), and from stranded gray whales (Varanasi et al., 1994). In contrast, there is no documentation of Hg concentrations sampled from living free-ranging gray whales, possibly biasing the reported Hg concentrations for this species. Biopsies collected remotely by boat from live animals will typically provide only a small sample of skin (epidermis and dermis). Thus, it is important to know difference in Hg concentration for the different epidermal layers as the proportion of strata may vary biologically based on sample collection dynamics (e.g., full thickness epidermis or not, angle of biopsy through the epidermis). The structure of cetacean epidermis is different from other mammals. The epidermis consists of three strata, in contrast to five strata in most terrestrial mammals. Strata granulosum and lucidum which are found in most mammals are absent in cetaceans (Sokolov, 1962; Reeb et al., 2007). Evidence shows that epidermal conditions alter Hg deposition in bottlenose dolphin (Tursiops truncatus), with a positive relationship between Hg concentrations and age, and a negative relationship with height of stratum spinosum (Miller et al., 2011). Hg analysis in different epidermal strata was previously done in beluga (Delphinapterus leucas) and narwhal (Monodon monoceros) (Wagemann and Kozlowska, 2005), but to our knowledge it has not been previously analyzed in gray whales.
Assessments of feeding ecology are also important in long-lived cetaceans. Ratios of stable isotopes of carbon-13 and nitrogen-15 have been used extensively to trace pathways of organic matter and complexity of trophic levels (Rounick and Winterbourn, 1986; Caraveo-Patino and Soto, 2005; Dehn et al., 2006b; Horstmann-Dehn et al., 2012) as well as movement (migration) between regions with varying isotopic signatures (Hoekstra et al., 2002). The eastern gray whale population migrates from their summer feeding grounds in Alaska to their winter grounds in Baja California Sur, Mexico. The western gray whale population has its summer feeding grounds in the Okhotsk Sea, and migrates south along the eastern coast of northern Asia to their winter breeding grounds thought to be along the coast of southern China (Bradford et al., 2008; Weller et al., 2012; Brüniche-Olsen et al., 2018b). Gray whales are benthic feeders filtering sediment for their primary prey of ampeliscid amphipods (Ampelisca macrocephala) (Caraveo-Patino and Soto, 2005; Dehn et al., 2006a; Caraveo-Patino et al., 2007). It had been generally accepted that gray whales only feed during summer, but δ13C and δ15N values have shown large variability in stable isotope composition during the winter months (Caraveo-Patino et al., 2007) indicating feeding behavior may be more complicated and worthy of temporal and spatial assessment for both eastern and western gray whales.
The critically endangered western gray whale population is dangerous to approach and difficult to study in the remote marine environment of the subarctic and Arctic. Biological studies of this species rarely provide robust sample materials unless a stranding occurs near an established network of responders (e.g., central California) or from well-coordinated sampling of subsistence harvested whales (Russia). Biopsy samples collected by remote sampling from boats often result in small samples of epidermal tissue with varying amounts of underlying tissue (“blubber”). Gray whale epidermis samples were morphologically and functionally divided into three distinct layers for this study: stratum corneum, stratum spinosum, and stratum basale. The epidermis and dermis interdigitate for structural support resulting in mixing of the stratum spinosum and stratum basale (Reeb et al., 2007).
In this study, we determined 13C and 15N stable isotope ratio values (δ13C, δ15N) and total Hg concentrations ([THg]) from histologically different strata of epidermal tissue samples from the eastern gray whale population where sample mass was generous. Our aim was to assess if obtaining a full depth proportionally representative biopsy sample is critical in preventing biases for Hg, and δ13C and δ15N measurements that may be introduced from a partial depth epidermis sample. In addition, these represent background data for this region and time of year. Previous studies on gray whales found that lipid extractions changes δ13C ratios in the epidermis (Horstmann-Dehn et al., 2012), and we conducted our stable isotope analysis both before and after lipid extraction for the eastern gray whale samples. The information gained from assessing full depth biopsies from stranded gray whales, is directly applied when we report δ13C and δ15N from biopsies collected remotely from free-ranging western gray whales of expected extremely limited epidermal mass.
Materials and Methods
Collection and Storage: Eastern Gray Whale Samples
From 2000–2011 fresh to moderately decomposed (code 2–3, Raverty et al., 2018) full thickness samples of epidermis and dermis were collected from stranded eastern gray whales along the California coast, United States (N = 15). The animals ranged from calves to adults, with 9 males, 5 females and one unknown sex. The samples were obtained through a collaboration with The Marine Mammal Center (TMMC, Sausalito, CA, United States; MMPA permit No. 932-1905-00/MA-009526 to Frances Gulland). Samples were stored at −80°C prior to and after shipping and shipped on dry ice. Total mercury concentration ([THg]) and carbon (C) and nitrogen (N) stable isotope analyses were conducted at the University of Alaska Fairbanks (UAF), Fairbanks, Alaska (United States). Histologic examination was conducted at the University of Tennessee (UT). Samples and specifically targeted subsamples (e.g., individual layers) provided ample amounts (mass) with full thickness epidermis, dermis and hypodermis abundantly available from these stranded animals relative to biopsies. Thus, this investigation allowed for sharing among investigators, validation (repeated analyses), and archiving that are not possible with valuable and hard to obtain smaller biopsy samples (described next). Sample collection site on whales could not be standardized because of variation in access based on position of the stranded whales.
Collection and Storage: Western Gray Whale Biopsies (Russia)
Biopsy samples (N = 10) were collected from free-ranging western gray whales off the northeast coast of Sakhalin Island, Russia. Briefly, biopsies were obtained using a 150 lb. draw weight compound crossbow (Barnett RC-150) with 40 mm by 7 mm internal diameter tips arrows (Brüniche-Olsen et al., 2018b). Samples collected in 2011 were obtained through a collaboration with the IWC, Russian Academy of Sciences Severtsov Institute of Ecology and Evolution (IPEE RAS), and National Scientific Center of Marine Biology of the Far East Branch of the Russian Academy of Science (NNTsMB DVO RAN). The 2011 samples were imported frozen from Russia to the NOAA Southwest Fisheries Science Center, La Jolla, CA by the IWC and then to Texas Tech University under CITES permit 11US774223/9. Samples collected in 2013 were obtained through a collaboration with the Western Gray Whale Monitoring Program that Exxon Neftegas Limited and Sakhalin Energy Investment Company have conducted since 2002. These samples were imported frozen from Russia to Texas Tech University under CITES permit 13US082589/9. All frozen subsamples were subsequently shipped from Texas Tech University on dry ice to UAF for analyses. Frozen samples were stored at −80°C until analysis. Research was completed under NMFS permits No. 932-1905-00/MA-009526 and No. 932-1905-01/MA-009526-01.
Histology
Previously frozen tissues from the eastern population (stranded animals) were fixed in 10% formalin, routinely processed, sectioned at approximately 5 μm, placed on glass slides, stained with Hematoxylin and Eosin (H&E), and viewed using light microscopy. Photomicrographs of the sections were taken using an Olympus dp71 camera (Olympus America, Inc., 3500 Corporate Parkway, Center Valley, PA, United States), layers were identified as stratum corneum, stratum spinosum, and stratum basale, with significant interdigitation between stratum spinosum and stratum basale near the base of the epidermis (Figure 1).
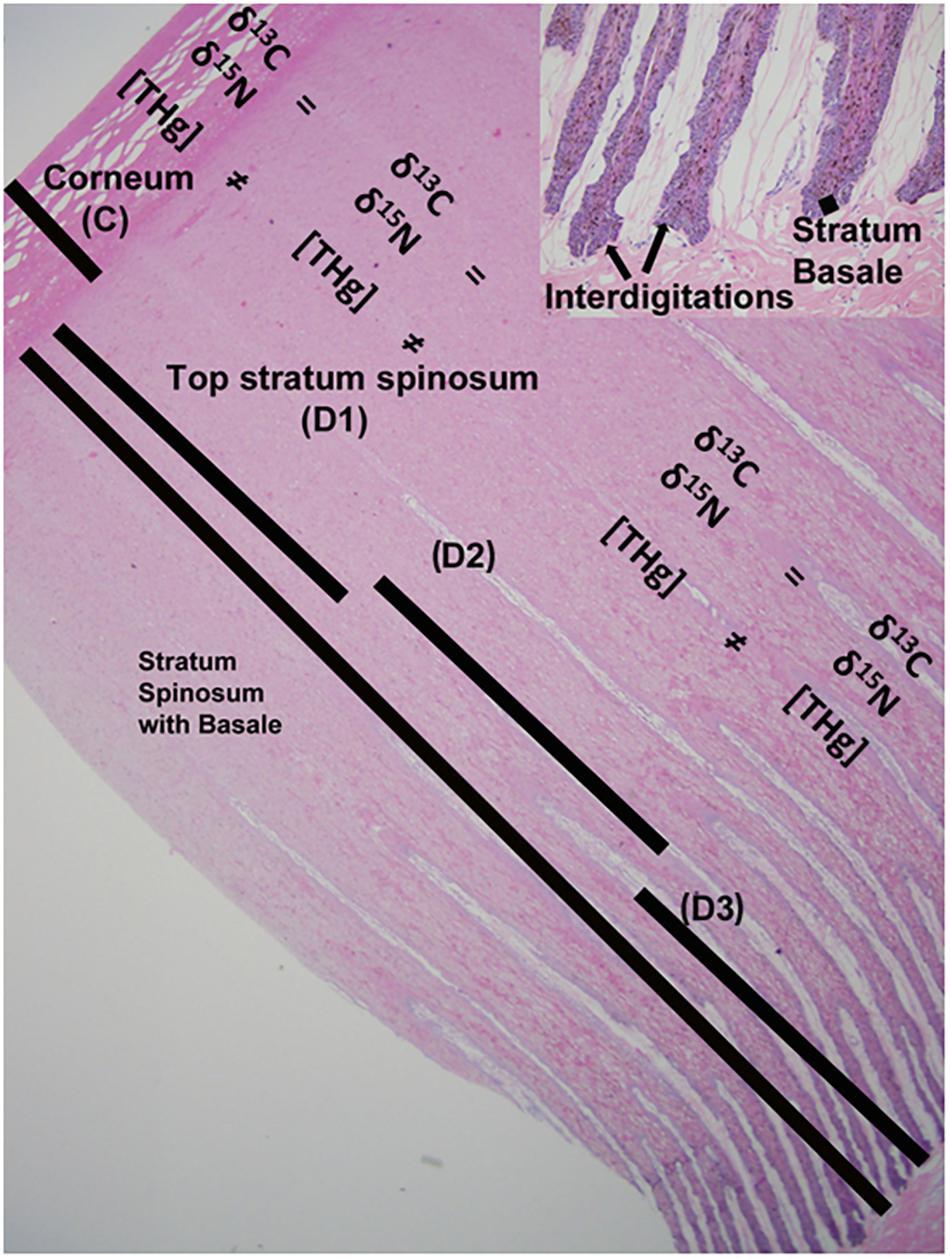
Figure 1. Gray whale epidermis is morphologically and functionally divided into three distinct layers for this study: stratum corneum (C), stratum spinosum, and stratum basale. Depth 1 (D1) includes only stratum spinosum and is equal to the top layer of the spinosum. Depth 2 (D2) is the distal portion of the epidermal-dermal interdigitation area and is composed of primarily stratum spinosum, with little stratum basale. Depth 3 (D3) is the proximal portion of the epidermal-dermal interdigitation area and is composed of primarily stratum basale, with little stratum spinosum.
Chemical Analyses; Mercury
Total mercury (THg) analysis was only conducted for the eastern gray whales due to limitation in biopsy sample size from the western gray whales. Determination of THg concentration ([THg]) was performed at the Wildlife Toxicology Laboratory (WTL) at UAF. Gray whale epidermis has three distinct morphological and functional layers for our purposes: the protective outer layer, stratum corneum (C); the middle layer, stratum spinosum; and the regenerative layer, stratum basale (Reeb et al., 2007). Based on structural analysis (histologic), samples of epidermis from 15 stranded whales were sectioned to provide the corneum and 3 equal depths for the rest of the epidermis – C (stratum corneum), D1 (only stratum spinosum), D2 (primarily stratum spinosum) and D3 (primarily stratum basale, Figure 1). The stratum corneum was easily identified visually and was removed with a clean scalpel. The remaining epidermis was then divided into three equal layers (D1–D3), removing all traces of underlying hypodermis from D3.
Subsamples from each depth were freeze-dried for 48 h and water content was calculated by subtraction of dry mass from wet mass. Dried samples were ground to a fine, homogenous powder with a cryomill. The [THg] was measured in triplicate using a direct mercury analyzer (DMA80; Milestone Inc., 25 Controls Drive, Shelton, CT, United States) according to EPA method 7473 (Castellini et al., 2012; Rea et al., 2013). Data for [THg] are reported on both a wet weight (ww) and dry weight (dw) basis. Quality control included measurement of blanks, liquid standards (3 ng THg at 0.100 μg/g) and certified reference materials IAEA 086 Hair (0.573 μg/g) and DORM 3 (0.355 μg/g) with each analytical run. Percent recoveries (mean ± SD) for liquid standards, IAEA 086, and DORM 3 were 96.7 ± 2.7, 102.4 ± 3.5 and 114.5 ± 2.6, respectively. Detection limit for [THg]dw was 0.008 μg/g for a 30 mg dry sample (0.25 ng THg). Three individuals had [THg] below the detection limit and have not been included in comparisons of epidermal layers. Thus, [THg] data represents 12 of 15 whales.
Chemical Analyses; C and N Stable Isotope Ratios
Isotopic ratios of carbon (δ13C) and nitrogen (δ15N) were analyzed at the Alaska Stable Isotope Facility at UAF by combustion using a continuous flow isotope ratio mass spectrometer according to methods described in Rea et al. (2013). Isotopic analyses are expressed in delta (δ) notation relative to an international standard (Vienna PeeDee Belemnite for δ13C and atmospheric air for δ15N) in parts per thousand (‰, Bond and Hobson, 2012). The mean analytical precision was determined using working laboratory standards (Sigma Chemical Co., Highland, IL, United States; mean δ13C = −15.82 ‰ and mean δ15N = 7.02 ‰) across separate sample runs and instrument precision was 0.1 ‰ for δ13C and 0.2 ‰ for δ15N. Enrichment of the heavy isotope of a particular element was reported using the following notation and equation:
Where the differential notation (δR) represents the relative difference between isotopic ratios of the sample and standard gases (i.e., 13C/12C, 15N/14N).
Due to the limited amount of tissue available from biopsy samples (western gray whales), full thickness epidermis samples from stranded eastern gray whales were used to evaluate potential variability of δ13C and δ15N values based on epidermal depth in gray whale populations. Distinct sampling layers for C and N stable isotope analysis were the same as those used for THg analysis described above.
Biopsies from epidermis of western gray whales (frozen, not stored in ethanol) were very small (4–40 mg). Most samples had a small amount of hypodermis attached which was removed with a clean scalpel, being careful to preserve as much epidermis as possible. Enough tissue was recovered from 10 individuals (3 from 2011, 7 from 2013) to measure C and N stable isotopes from the D3 layer (based on presence of hypodermis). Epidermal samples from both eastern gray whales (subsampled by layer) and western gray whales (biopsies) were freeze-dried for 48 h and homogenized using a tissue mill or Wig-L-BugTM (depending on sample size) in the WTL. Stable isotope ratios of C and N were measured before and after lipid extractions for eastern gray whales as sample mass was abundant. For western gray whales, tissue samples were too small and stable isotope analysis could only be done once without lipid extractions. Lipid extractions followed the method described in Cyr et al. (2019), where 1.0–2.0 g of freeze-dried sample muscle was rinsed three times in a mixture of 2:1 chloroform: methanol for 15 min, air dried overnight, then freeze-dried for 24 h.
Statistical Analysis
All statistical analyses were performed as linear models in the statistical software R, version 3.1.1 (R Development Core Team, 2014) and SigmaPlot 11.0 (Systat Software, Inc.). Tissue variables analyzed included [THg]dw, [THg]ww, δ13C and δ15N from the three different epidermal strata, sectioned in four different layers (C, D1, D2, and D3), both before and after lipid extraction. We tested for normality with histogram, fitted values and theoretical quantiles (Normal Q-Q plot). Water content, [THg]dw and [THg]ww from different epidermal layers were analyzed using Friedman repeated measures ANOVA on ranks followed by post hoc Tukey multiple pairwise comparison tests. Comparisons of C and N stable isotope ratios between the two populations were made with ANOVA. Comparison of 13C and 15N stable isotopes before and after lipid extractions were made using repeated measures ANOVA followed by post hoc Holm-Sidak multiple pairwise comparison tests. Summary statistics for all variables were calculated with Microsoft Excel®. For all statistical analysis, we considered p values less than 0.05 significant.
Results
Total Mercury
Total mercury concentration was measured in four different epidermal depths for the eastern gray whale samples (Figure 2). The mean and standard deviation (SD) of [THg] measured in wet weight and dry weight, water content and lipid content, from four different layers (for the eastern population) are reported in Table 1. There was a significant difference in water content between sectioned epidermal layers (Õ2 = 25.10, 3DF, p < 0.001), with corneum having significantly lower water content than D2 or D3 (p < 0.05). There was a significant difference in [THg] represented as both dry weight (dw) and wet weight (ww) concentrations from the different epidermal layers (X2 = 25.2, 3DF, p < 0.001 and X2 = 19.6, 3DF, p < 0.001, respectively). The pattern of significant differences (p < 0.05) between layers was different for [THg]dw compared to [THg]ww, as illustrated in Figures 2A,B, respectively.
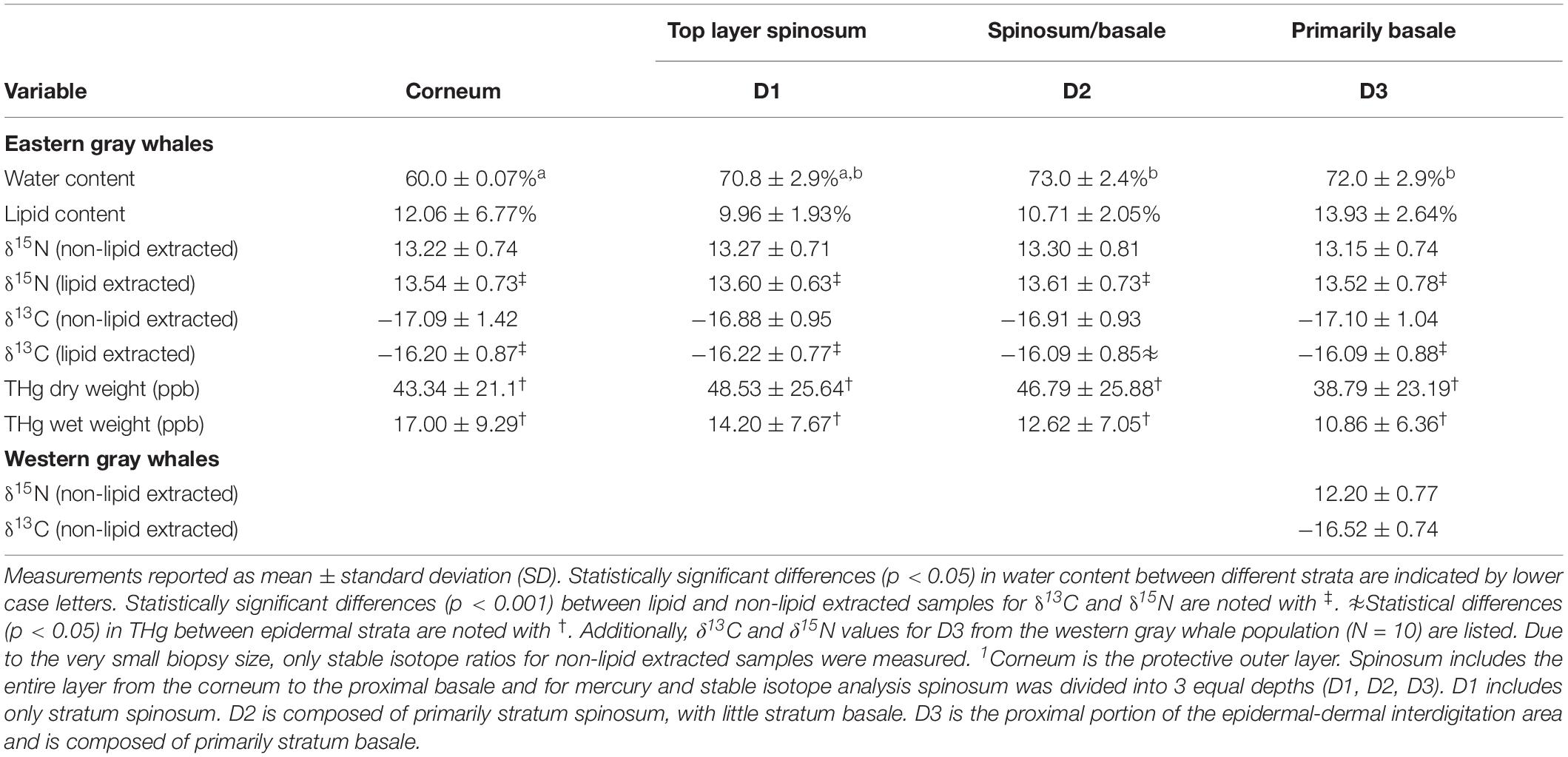
Table 1. Water and lipid content and values of carbon and nitrogen stable isotopes (δ13C and δ15N) from different epidermal strata; corneum, spinosum (Depth (D) 1 and 2) and basale (D3)1 of eastern and western gray whales.
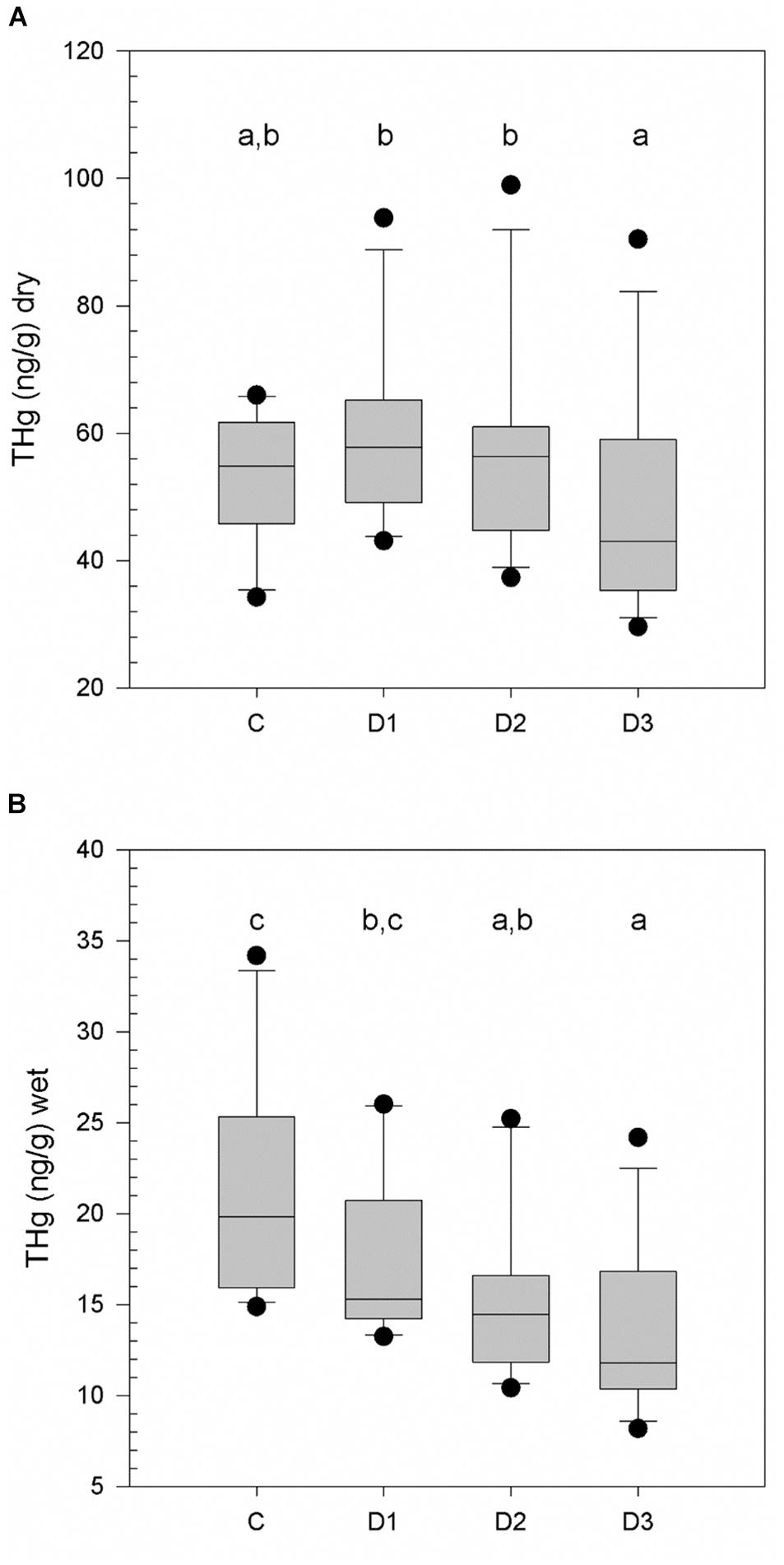
Figure 2. Total mercury concentrations ([THg]) in dry weight (A) and wet weight (B) for eastern gray whales (N = 15) from different epidermal strata; corneum, spinosum (Depth (D) 1 and 2) and basale (D3). Statistically significant differences in [THg] between strata (p < 0.05) are indicated by different lower case letters.
The greatest individual [THg]dw measured was 98.92 ng/g (0.098 μg/g) in D2. There were three calves in the data set, and all had [THg]dw < 8.0 ng/g. In contrast, the lowest value measured in an adult was 29.58 ng/g measured in D3.
Stable Isotopes (Carbon-13 and Nitrogen-15; δ13C and δ15N)
For the eastern population the mean and SD of δ13C and δ15N measured from four different layers are reported in Table 1. The chemical analyses for δ13C and δ15N in eastern gray whales were conducted with and without lipid extraction. For samples that were not lipid extracted there was no significant difference in δ15N (F3,42 = 1.84, p = 0.15) or δ13C (F3,42 = 1.77, p = 0.17) values measured from the different epidermal layers for the eastern gray whale samples. These findings were consistent after lipid extraction with no significant difference in δ15N values (F3,41 = 0.652, p = 0.586) or δ13C values (F3,41 = 1.838, p = 0.155) measured from the different epidermal layers. As expected, there was a significant association between both δ15N (slope = 1.017, R2 = 0.93, p < 0.01) and δ13C (slope = 1.22, R2 = 0.86, p < 0.01) before and after lipid extraction (Figure 3) and both δ15N and δ13C were significantly enriched in the heavy isotope in lipid extracted versus non-lipid extracted samples in all epidermal layers (p < 0.001 for all pairwise comparisons). The absolute difference between lipid and non-lipid extracted samples was relatively small for δ15N (0.34 ± 0.20 ‰, maximum = 1.08 ‰), while effect of lipid extraction on δ13C was more variable (0.44 ± 0.58 ‰, maximum = 3.16 ‰).
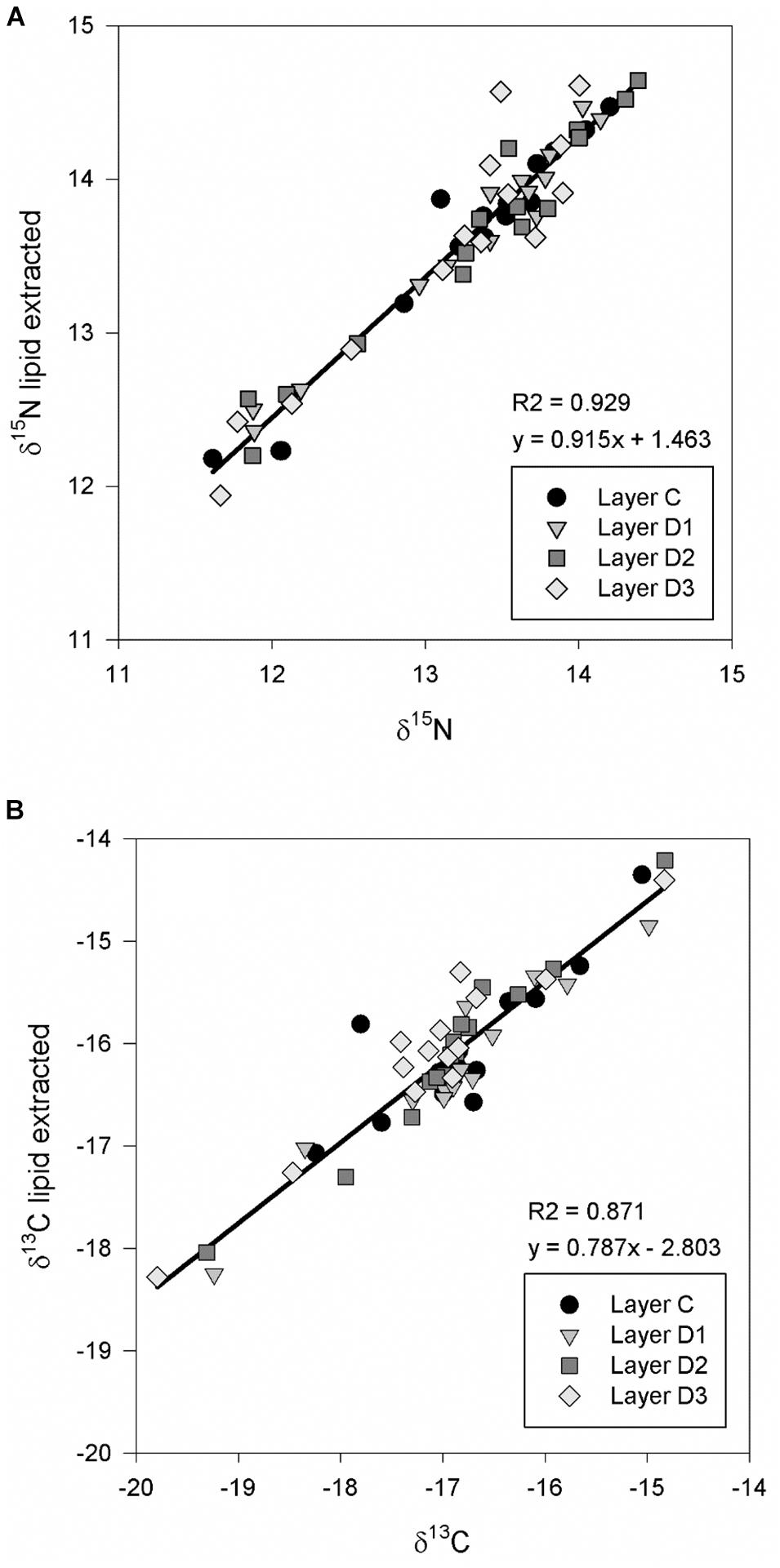
Figure 3. Comparison of nitrogen-15 (A) and carbon-13 (B) stable isotopes ratios (δ13C and δ15N) from different epidermal strata from eastern gray whales (N = 15); corneum (Layer C), spinosum (Layers D1 and D2) and basale (Layer D3) with and without lipid extraction.
Due to very small tissue volume the δ13C and δ15N values for the western gray whale population were only measured in the D3 as non-lipid extracted samples (Table 1). We found no differences in δ15N or δ13C values as measured in D3 (F1,8 = 1.24, p = 0.29 and F1,8 = 0.86, p = 0.39, respectively) when comparing eastern and western gray whale populations (non-lipid extracted samples).
Discussion
We report [THg], δ15N and δ13C values from stranded animals from the eastern gray whale population; and δ15N and δ13C values from the biopsy sampled western population. The analyses were performed on the histologically distinct epidermal strata to investigate differences between epidermal layers in [THg] and δ15N and δ13C for the eastern population. This evaluation was directly applied to assist with analyzing and reporting δ15N and δ13C values from biopsies of living free-ranging gray whales from the western population as we considered use of the small but valuable biopsy material.
Mercury levels in arctic marine habitats have increased 92% in the last 150 years, and it continues to be important to conduct biomonitoring for Hg in long-lived marine mammals (Dietz et al., 2013; Peterson et al., 2016). The [THg] found in epidermal tissues in this study are higher than reported by Dehn et al. (2006a), but lower than reported by Varanasi et al. (1994) and Tilbury et al. (2002). As expected, calves had the lowest values. Using a small epidermal biopsy collected from a free-ranging animal for Hg biomonitoring in cetaceans is a valuable approach. This is analogous to use of hair and fur in pinnipeds to monitor Hg exposure (Rea et al., 2013; Van-Hoomissen et al., 2015; Peterson et al., 2016). However, as we have shown here, there are significant differences in [THg] between the different epidermal strata, with greatest [THg]dw occurring in D1 and D2. The relatively high [THg]ww in the corneum reflects lower water content in that layer (60.0 ± 0.07% water compared to 70.8 ± 2.9, 73.0 ± 2.4, and 72.0 ± 2.9% water in layers D1–D3 of the spinosum, respectively) and demonstrates the utility of comparing [THg] on both a wet and dry mass basis. Thus, determining which strata and representation of the concentration (wet or dry weight) best correlates with other tissues would be a logical next step. A previous study found that epidermal levels of Hg were weakly correlated with blubber, kidney, muscle, and liver concentrations in bowhead whales (Balaena mysticetus) (O’Hara et al., 2008). In bottlenose dolphins, epidermal Hg was closely correlated with whole blood making epidermal samples valuable for biomonitoring in this species (Woshner et al., 2008), and epidermal condition drives [THg] deposition in this tissue (Miller et al., 2011). For gray whales, further sampling should be conducted to establish correlations between epidermis and other tissues and among populations and cohorts (calves, subadults, adults) where access to stranded and subsistence harvested (Russia) whales allows a multiple tissue study design to improve interpretation of epidermal biopsies. These proposed studies could include other elements of interest such as zinc, cadmium, selenium, and lead when tissue sample weight allows.
When the distinct epidermal strata were compared in samples collected from stranded whales, we found no difference in δ13C or δ15N ratios. We do not expect the stable isotope ratios for carbon-13 and nitrogen-15 to change between fresh to moderately decomposed samples and living animals (Payo-Payo et al., 2013). This indicates biopsy sampling, allowing only for marginal tissue size, from free-ranging gray whales to study these isotopes does not require consideration of the anatomic structure of epidermis for δ13C and δ15N. We also found similar significant associations between lipid extracted and non-lipid extracted samples, although the lipid extracted samples were significantly enriched in both the 15Nitrogen and 13Carbon across strata compared to non-lipid extracted samples.
Gray whales have higher δ13C values compared to other baleen whales, likely due to their feeding habit of filtering out invertebrates from sediment in the benthic ecosystems (Dehn et al., 2006b; Horstmann-Dehn et al., 2012). The δ15N values were similar to values reported previously (Dehn et al., 2006a; Caraveo-Patino et al., 2007; Horstmann-Dehn et al., 2012), demonstrating a low trophic level and prey based on benthic gammaridean amphipods (Dehn et al., 2006a). Hoekstra et al. (2002), Gendron et al. (2015) demonstrated how tissue measures of δ13C were seasonally variable related to the migration of bowhead whales, and studies of δ13C in eastern gray whales have shown that this population likely feed during their time at the winter breeding grounds (Caraveo-Patino and Soto, 2005; Caraveo-Patino et al., 2007). Future studies might elucidate the western population of gray whales’ geographical winter range and possible temporal and spatial changes with extended monitoring and research using C and N stable isotope ratios. Our finding that δ13C and δ15N ratios do not vary in the different epidermal strata, makes a small biopsy from the endangered free-ranging western gray whales suitable for such analyses.
To maximize information obtained from each small biopsy sample from the free-ranging gray whales, histologic analyses were performed on samples collected from stranded eastern gray whale epidermis. We found that the protective corneum layer was about one tenth of the spinosum layer. In the D3 layer rete pegs interdigitate with papillae from the dermis for support, much like other cetacean epidermal tissues (Reeb et al., 2007; Miller et al., 2011). Structural studies of cetacean epidermal tissues are important for studies of free-ranging whale biopsies to assess such things as contaminants, skin lesions/disease, body condition, etc (Wagemann and Kozlowska, 2005; Woshner et al., 2008; Miller et al., 2011). Epidermal biopsies are difficult to obtain and very valuable, and the information gained helps determine whether biopsy testing may target specific strata to minimize waste of tissue and possibly enhance comparisons among whales (reduced variability in values that may be driven by strata type).
Conclusion
Epidermal tissues collected from stranded eastern gray whales were used to evaluate [THg] measures, and δ13C and δ15N ratios between the corneum, stratum spinosum and stratum basale. We found a significant difference in [THg] between the different strata, represented as both ww and dw. This has implications for analyzing Hg from an epidermal biopsy from a free-ranging gray whale, and we recommend identifying which stratum the biopsy represents. For these comparisons of Hg concentrations between strata it is important the comparison is done on a wet mass basis as% water is one of the main factors driving the [THg] differences between the epidermal layers. There were, however, no significant difference in δ13C and δ15N values between the different strata, making epidermal biopsies from free-ranging whales an ideal sampling method to investigate δ13C and δ15N relationships that can be conducted independent of strata identification.
Data Availability Statement
The datasets generated for this study are available on request to the corresponding author.
Ethics Statement
This animal study was reviewed and approved by the International Whaling Commission and then to Texas Tech University under CITES permit 11US774223/9. NMFS permits Nos. 932-1905-00/MA-009526 and 932-1905-01/MA-009526-01.
Author Contributions
All authors contributed to manuscript review and preparation. Additionally, TO’H, CG-C, DM, JD, and KB designed the study. TO’H and DM supervised experiments. ML conducted stable isotope analyses, statistical analyses and prepared the first draft of the manuscript. JC performed the Hg analyses. DM and BG prepared and analyzed the histological analyses. VV conducted fieldwork and sample collection. CG-C and VV supervised sample processing and distribution of samples to collaborators and secured all permits. All authors contributed to the writing, critically revised the manuscript, approved the final version and agreed to be held accountable for the content herein.
Funding
This study was funded by the Exxon Neftegas Limited (ENL), the Sakhalin Energy Investment Company (SEIC), and the TTU Center for Biotechnology and Genomics. The content is solely the responsibility of the authors and does not necessarily represent the official views of the funding parties.
Conflict of Interest
JD was employed by the company ExxonMobil Upstream Research Company. KB was employed by the company Shell Global Solutions.
The remaining authors declare that the research was conducted in the absence of any commercial or financial relationships that could be construed as a potential conflict of interest.
Acknowledgments
Aimée Lang (Southwest Fisheries Science Center, National Marine Fisheries Service, National Oceanic and Atmospheric Administration, La Jolla, CA, United States) provided methods used previously for sexing gray whales and curating the eastern gray whale samples provided via the IWC. John Patton (Purdue University, West Lafayette, IN, United States) shared methods used previously for sexing bowhead whales. This work was conducted under NMFS Office of Protected Resources’ Marine Mammal Health and Stranding Response Program (MMHSRP) permits 932-1905-MA-009526 and 18786. We thank Teri Rowles (National Marine Fisheries Service, Silver spring, MD, United States) for assistance with the project. We acknowledge the support from Frances Gulland (The Marine Mammal Center, Sausalito, CA, United States) who provided eastern gray whale samples, the National Scientific Center of Marine Biology of the Far East Branch of the Russian Academy of Science (NNTsMB DVO RAN) and Oregon State University for the collection of the eastern gray whale samples, and the University of Tennessee College of Veterinary Medicine Histology Laboratory for preparation of histological sections. We thank Azivy Aziz and Lucie N’Guessan (ExxonMobil Upstream Research Company), Mike Swindoll and Mike Scott (Exxon Neftegas Limited) for invaluable assistance in implementing this research project.
References
Bickham, J. W., Doyle, J., DeWoody, A., Pezdek, R., Wooten, K., Kellar, N., et al. (2013). Genetics and preliminary hormone analyses in Western Gray whale biopsy samples collected off Sakhalin Island in 2011. Paper SC/65a/BRG23 presented to the IWC Scientific Committee, (Cambridge, MA: International Whaling Commission).
Bond, A. L., and Hobson, K. A. (2012). Reporting stable-isotope ratios in ecology: recommended terminology, guidelines and best practices. Waterbirds 35, 324–331. doi: 10.1675/063.035.0213
Bradford, A., Weller, D., Wade, P., Burdin, A., and Brownell, R. Jr. (2008). Population abundance; and growth rate of western gray whales Eschrichtius robustus. Endang Species Res 6, 1–14. doi: 10.3354/esr00106
Brüniche-Olsen, A., Urban, R. J., Vertyankin, V. V., Godard-Codding, C. A. J., Bickham, J. W., and DeWoody, J. A. (2018b). Genetic data reveal mixed stock aggregations of gray whales in the North Pacific Ocean. Biol. Lett. 14:20180399. doi: 10.1098/rsbl.2018.0399
Brüniche-Olsen, A., Westerman, R., Kazmierczyk, Z., Vertyankin, V. V., Godard-Codding, C., Bickham, J. W., et al. (2018a). The inference of gray whale (Eschrichtius robustus) historical population attributes from whole-genome sequences. BMC Evol. Biol. 18:87. doi: 10.1186/s12862-018-1204-3
Caraveo-Patino, J., Hobson, K., and Soto, L. (2007). Feeding ecology of gray whales inferred from stable-carbon and nitrogen isotopic analysis of baleen plates. Hydrobiologia 586, 17–25. doi: 10.1007/s10750-006-0477-5
Caraveo-Patino, J., and Soto, L. (2005). Stable carbon isotope ratios for the gray whale (Eschrichtius robustus) in the breeding grounds of Baja California Sur, Mexico. Hydrobiologia 539, 99–107.
Castellini, J. M., Rea, L. D., Lieske, C. L., Beckmen, K. B., Fadely, B. S., Maniscalco, J. M., et al. (2012). Mercury concentrations in hair from neonatal and juvenile Stellar sea lions (Eumetopias jubatus):implications based on age and region in this Northern Pacific marine sentinel piscivore. Ecohealth 9, 267–277. doi: 10.1007/s10393-012-0784-4
Cyr, A., López, J. A., Rea, L., Wooler, M. J., Loomis, T., McDermott, S., et al. (2019). Mercury concentrations in marine species from the Aleutian Islands: spatial and biological determinants. Sci. Total Environ. 664, 761–770. doi: 10.1016/j.scitotenv.2019.01.387
Dehn, L., Follmann, E., Rosa, C., Duffy, L., Thomas, D., Bratton, G., et al. (2006a). Stable isotope and trace element status of subsistence-hunted bowhead and beluga whales in Alaska and gray whales in Chukotka. Mar. Pollut. Bull. 52, 301–319. doi: 10.1016/j.marpolbul.2005.09.001
Dehn, L., Follmann, E., Thomas, D., Sheffield, G., Rosa, C., Duffy, L., et al. (2006b). Trophic relationships in an Arctic food web and implications for trace metal transfer. Sci. Total Environ. 362, 103–123. doi: 10.1016/j.scitotenv.2005.11.012
Dietz, R., Outridge, P., and Hobson, K. (2009). Anthropogenic contributions to mercury levels in present-day Arctic animals—A review. Sci. Total Environ. 407, 6120–6131. doi: 10.1016/j.scitotenv.2009.08.036
Dietz, R., Sonne, C., Basu, N., Braune, B., O’Hara, T. M., Letcher, R., et al. (2013). What are the toxicological effects of mercury in Arctic biota? Sci. Total Environ. 443, 775–790. doi: 10.1016/j.scitotenv.2012.11.046
Gendron, M., Castellini, J. M., O’Hara, T., Klein, D., Wooten, K., Pezdek, R. J., et al. (2015). Hormone and stable isotope of C and N analyses in Western Gray Whale (Eschrichtius robustus) biopsy samples collected off Sakhalin Island in 2011, 2012 and 2013. Paper SC/66a/BRG/10 (Rev1) presented to the International Whaling Commission, Scientific Committee, San Diego, CA.
Hoekstra, P. F., Dehn, L. A., George, J. C., Solomon, K. R., Muir, D. C. G., and O’Hara, T. M. (2002). Trophic ecology of bowhead whales (Balaena mysticetus) compared with that of other arctic marine biota as interpreted from carbon-, nitrogen-, and sulfur-isotope signatures. Can. J. Zool. 80, 223–231. doi: 10.1139/Z01-229
Horstmann-Dehn, L., Follmann, E. H., Rosa, C., Zelensky, G., and George, C. (2012). Stable carbon and nitrogen isotope ratios in muscle and epidermis of arctic whales. Mar. Mam. Sci. 28, E173–E190.
Kennedy, S. N., Castellini, J. M., Hayden, A. B., Fadely, B. S., Burkanov, V. N., Dajles, A., et al. (2019). Regional and age-related variations in haptoglobin concentrations in Steller sea lions (Eumetopias jubatus) from Alaska, USA. J. Wildl Dis. 55, 91–104. doi: 10.7589/2017-10-257
Lang, A., Calambokidis, J., Scordino, J., Pease, V., Klimek, A., Burkanov, V., et al. (2014). Assessment of genetic structure among eastern North Pacific gray whales on their feeding grounds. Mar. Mam. Sci. 30, 1473–1493. doi: 10.1111/mms.12129
Mate, B. R., Ilyashenko, V. Y., Bradford, A. L., Vertyankin, V. V., Tsidulko, G. A., Rozhnov, V. V., et al. (2015). Critically endangered western gray whales migrate to the eastern North Pacific. Biol. Lett. 11:20150071. doi: 10.1098/rsbl.2015.0071
Miller, D. L., Woshner, V., Styer, E. L., Ferguson, S., Knott, K. K., Gray, M. J., et al. (2011). Histologic findings in free-ranging sarasota bay bottlenose dolphin (Tursiops truncatus) skin: mercury, selenium, and seasonal factors. J. Wildl Dis. 47, 1012–1018. doi: 10.7589/0090-3558-47.4.1012
O’Hara, T. M., Hanns, C., Woshner, V., Zeh, J., Bratton, G., and Taylor, R. (2008). Essential and non-essential elements in the bowhead whale: epidermis-based predictions of blubber, kidney, liver and muscle tissue concentrations. J. Cetacean Res. Manag. 10, 107–117.
Payo-Payo, A., Ruiz, B., Cardona, L., and Borrell, A. (2013). Effect of tissue decomposition on stable isotope signatures of striped dolphins Stenella coeruleoalba and loggerhead sea turtles Caretta caretta. Aquat. Biol. 18, 141–147. doi: 10.3354/ab00497
Peterson, S. H., McHuron, E. A., Kennedy, S. N., Ackerman, J. T., Rea, L. D., Castellini, J. M., et al. (2016). Evaluating hair as a predictor of blood mercury: the influence of ontogenetic phase and life history in pinnipeds. Arch. Environ. Contam. Toxicol. 70, 28–45. doi: 10.1007/s00244-015-0174-3
Raverty, S., Duignan, P. J., Jepson, P. D., and Morell, M. (2018). “Marine Mammal Gross Necropsy,” in CRC Handbook of Marine Mammal Medicine, eds F. M. D. Gulland, L. A. Dierauf, and K. L. Whitman (Boca Raton, FL: CRC Press), 249–265.
R Development Core Team (2014). R: A Language and Environment for Statistical Computing. Vienna: R Foundation for Statistical Computing. Available online at: http://www.R-project.org/ (accessed 2016).
Rea, L. D., Castellini, J. M., Correa, L., Fadely, B. S., and O’Hara, T. M. (2013). Maternal Stellar sea lion diets elevate fetal mercury concentrations in an area of population decline. Sci. Total Environ. 454-455, 277–282. doi: 10.1016/j.scitotenv.2013.02.095
Reeb, D., Best, P., and Kidson, S. (2007). Structure of the integument of southern right whales, Eubalaena australis. Anat. Rec. 290, 596–613. doi: 10.1002/ar.20535
Rounick, J., and Winterbourn, M. (1986). Stable carbon isotopes and carbon flow in ecosystems. Bioscience 36, 171–177. doi: 10.2307/1310304
Sokolov, V. (1962). Adaptations of the mammalian skin to the aquatic mode of life. Nature 195, 464–466. doi: 10.1038/195464a0
Tilbury, K., Stein, J., Krone, C., Brownell, R. Jr., Blokhin, S., Bolton, J., et al. (2002). Chemical contaminants in juvenile gray whales (Eschrichtius robustus) from a subsistence harvest in Arctic feeding grounds. Chemosphere 47, 555–564. doi: 10.1016/s0045-6535(02)00061-9
Van-Hoomissen, S., Gulland, F. M. D., Greig, D. J., Castellini, J. M., and O’Hara, T. M. (2015). Blood and hair mercury concentrations in the Pacific Harbor Seal (Phoca vitulina richardii) Pup: associations with neurodevelopmental outcomes. Ecohealth 12, 490–500. doi: 10.1007/s10393-015-1021-8
Varanasi, U., Stein, J., Tilbury, K., Meador, J., Sloan, C., Clark, R., et al. (1994). Chemical contaminants in gray whales (Eschrichtius robustus) stranded along the west coast of North America. Sci. Total Environ. 145, 29–53. doi: 10.1016/0048-9697(94)90296-8
Wagemann, R., and Kozlowska, H. (2005). Mercury distribution in the skin of beluga (Delphinapterus leucas) and narwhal (Monodon monoceros) from the Canadian Arctic and mercury burdens and excretion by moulting. Sci. Total Environ. 351-352, 333–343. doi: 10.1016/j.scitotenv.2004.06.028
Weller, D., Klimek, A., Bradford, A., Calambokidis, J., Lang, A., Gisborne, B., et al. (2012). Movements of gray whales between the western and eastern North Pacific. Endang Species Res. 18, 193–199. doi: 10.3354/esr00447
Woshner, V., Knott, K. K., Wells, R., Willerro, C., Swor, R., and O’Hara, T. M. (2008). Mercury and selenium in blood and epidermis of bottlenose dolphins (Tursiops truncatus) from Sarasota Bay, FL: interaction and relevance to life history and hematologic parameters. Ecohealth 5, 360–370. doi: 10.1007/s10393-008-0164-2
Keywords: biopsy, stranded marine mammal, contaminant, epidermis, gray whale, mercury, stable isotopes
Citation: Lian M, Castellini JM, Miller D, Griff B, Vertyankin VV, Dupont J, Broker K, Godard-Codding CAJ and O’Hara TM (2020) Assessing δ13C, δ15N and Total Mercury Measures in Epidermal Biopsies From Gray Whales. Front. Mar. Sci. 7:133. doi: 10.3389/fmars.2020.00133
Received: 16 October 2019; Accepted: 18 February 2020;
Published: 06 March 2020.
Edited by:
Rob Harcourt, Macquarie University, AustraliaReviewed by:
Luis Cardona, University of Barcelona, SpainRichard Reina, Monash University, Australia
Copyright © 2020 Lian, Castellini, Miller, Griff, Vertyankin, Dupont, Broker, Godard-Codding and O’Hara. This is an open-access article distributed under the terms of the Creative Commons Attribution License (CC BY). The use, distribution or reproduction in other forums is permitted, provided the original author(s) and the copyright owner(s) are credited and that the original publication in this journal is cited, in accordance with accepted academic practice. No use, distribution or reproduction is permitted which does not comply with these terms.
*Correspondence: Marianne Lian, bWxpYW5AYWxhc2thLmVkdQ==