Growing Up Down South: Spatial and Temporal Variability in Early Growth of Fuegian Sprat Sprattus fuegensis From the Southwest Atlantic Ocean
- 1Laboratorio de Zooplancton Marino, Departamento de Biodiversidad y Biología Experimental (DBBE), Facultad de Ciencias Exactas y Naturales, Universidad de Buenos Aires, Buenos Aires, Argentina
- 2Instituto de Biodiversidad y Biología Experimental Aplicada (IBBEA), CONICET-UBA, Facultad de Ciencias Exactas y Naturales, Universidad de Buenos Aires, Buenos Aires, Argentina
- 3Instituto Nacional de Investigación y Desarrollo Pesquero (INIDEP), Mar del Plata, Argentina
The Fuegian sprat Sprattus fuegensis represents one of the most important pelagic resources in the Southwest Atlantic Ocean (SWAO), exerting a crucial ecological role as an intermediary link in Patagonian food webs. Otolith microstructure of 217 age-0 sprats were analyzed aiming to characterize growth patterns and possible environmental effects over them. Samples were gathered during three oceanographic surveys (spring 2014; autumns 2016, 2017) in Tierra del Fuego (TDF) and the Marine Protected Area Namuncurá-Burdwood Bank (MPAN-BB), the first Argentinian open-sea marine protected area. Daily growths (DG) of larvae and post-larvae were estimated by individually modeling size and otolith radius relationships through back-calculation procedures using potential and linear functions, respectively. Increment widths (IW) and DG values and trajectories were assessed for older sprats (i.e., survivors) sampled in the autumn surveys considering the habitat, year sampled and sprats’ hatching seasons, and were additionally evaluated in relation to sea-surface temperature (SST). IW and DG trajectories differed primarily according to the habitat sampled and sprats’ hatching seasons. Overall, strong coupling was detected between IW and DG trajectories with SST in both habitats. However, statistical assessment on particular comparisons showed that this general trend is not strictly invariant. Even though several results pinpointed a positive correlation between IW and DG with SST, the highest DG were estimated for summer- and autumn-born sprats sampled in 2016 in the MPAN-BB, period and habitat with the lowest SST values. These results unveil a complex relationship between spatial and temporal variability during early growth of Fuegian sprats, supplying relevant information that could be used in the creation of adequate ecosystem based management strategies in the SWAO.
Introduction
The Fuegian sprat Sprattus fuegensis (i.e., Austral sprat, Patagonian sprat, Malvinas (Falkland) sprat) is a small clupeid that inhabits the coasts and shelves of southern South America (Cousseau, 1982; Aranis et al., 2007) and is usually referred to as the most important pelagic resource in the Southwest Atlantic Ocean (SWAO) south of 47°S (Bellisio et al., 1979). Despite representing a suitable target species, catches upon this clupeid are still incidental in the Patagonian Sea, with commercial extraction currently occurring only in Chile (Cerna et al., 2014). As many other small pelagic fishes, this zooplanktivorous sprat exerts an essential trophic role as an intermediary link between lower trophic levels and top predators of ecological and economical relevance (e.g., Ramírez, 1976; Bezzi, 1984; Schiavini et al., 1997; Ciancio et al., 2008; Belleggia et al., 2014; Scioscia et al., 2014). Furthermore, due to their high biomass schools and trophic position, S. fuegensis possibly controls the energy flux at the wasp-waist level (Cury et al., 2000; Bakun, 2006; Fauchald et al., 2011; Ricciardelli and Boy, unpublished data). Therefore, fluctuations in Fuegian sprat abundances could have major environmental and economic consequences.
Recruitment success in marine fishes is closely coupled to early stages’ growth and survival (e.g., Hjort, 1926; Houde, 1987; Anderson, 1988; Cushing, 1990; Leggett and Deblois, 1994; Sogard, 1997; Köster et al., 2003). In turn, several biotic and abiotic factors have been linked to early growth variability and survival for other sprats such as Sprattus sprattus, with food availability, density-dependent processes and temperature being among the most significant ones (Nissling, 2004; Voss et al., 2006; Baumann et al., 2006a,b,c, 2007, 2008; Hinrichsen et al., 2010; Peck et al., 2012). Combined with the high susceptibility of small pelagic fishes to ocean-atmosphere variations (Alheit and Hagen, 2001; Peck et al., 2013), this background pinpoint the importance of understanding Fuegian sprat early stages’ growth and the potential influence of environmental change over them, features poorly understood to the date.
Three different spawning/nursery grounds of S. fuegensis have been detected in the SWAO, the Malvinas Islands, the coasts of Tierra del Fuego (TDF) and the Marine Protected Area Namuncurá-Burdwood Bank (MPAN-BB), the first Argentinian open-sea marine protected area (Law no. 26,875) (Ciechomski and Weiss, 1974; Ciechomski et al., 1975; Sánchez and Ciechomski, 1995; Sánchez et al., 1995, 1997; Bruno et al., 2018; García Alonso et al., 2018). Located between 53°S and 55°S, the MPAN-BB and TDF encompass the southernmost extent inhabited by clupeids worldwide (Figure 1). Due to their similar latitudinal range and geographic proximity, these habitats exhibit shared environmental attributes: both of them are under the prevailing influence of intense westerly winds and high tidal variability, and their waters have a sub-Antarctic origin comprised mainly by the northernmost jets of the Antarctic Circumpolar Current and being partially fed by the Cape Horn Current (Piola et al., 2018). However, each habitat also possesses intrinsic physical characteristics, possibly imprinting environmentally induced variability over Fuegian sprats’ early development.
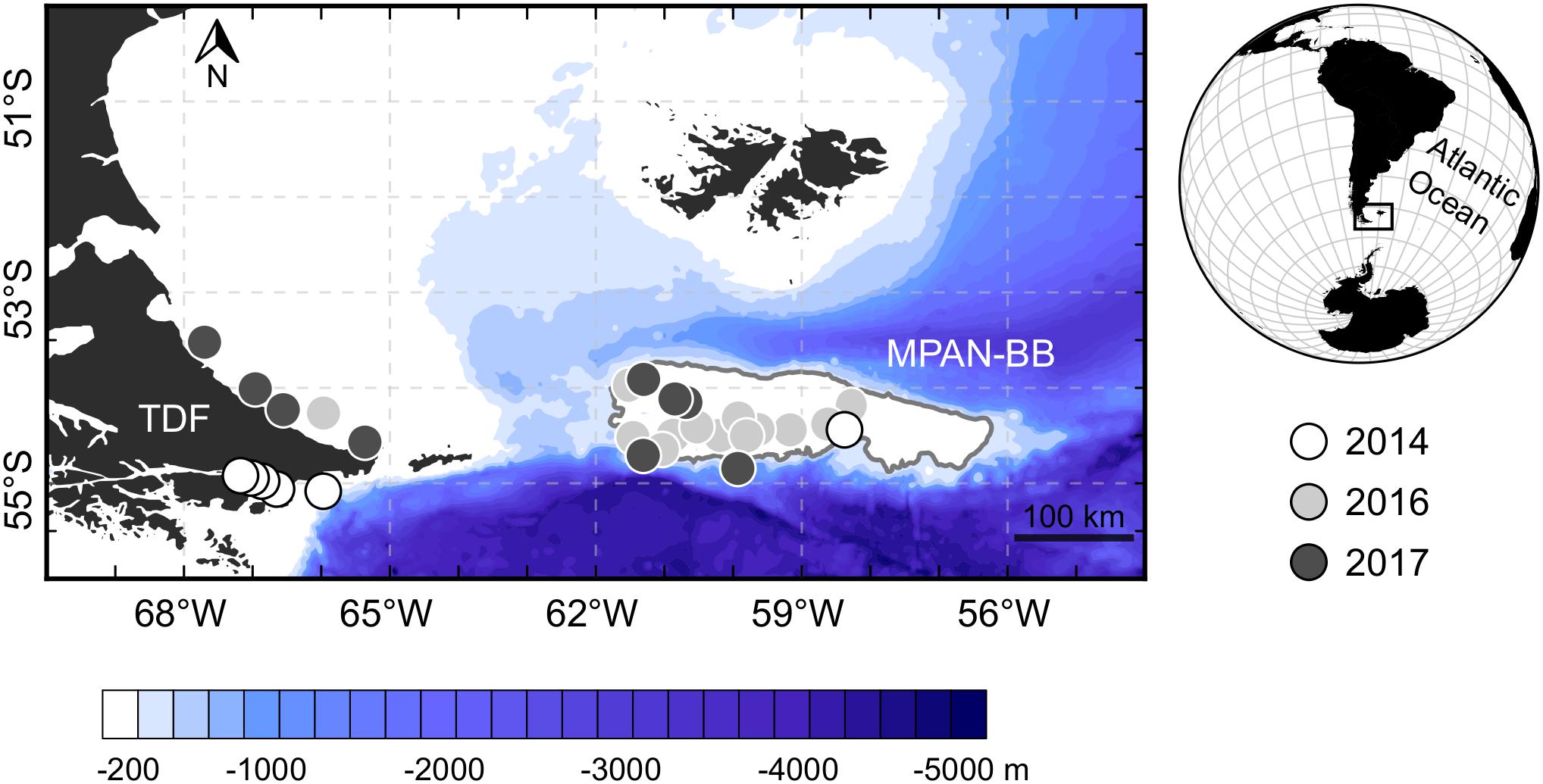
Figure 1. Location of the studied habitats in the Southwest Atlantic Ocean: Tierra del Fuego (TDF) and the Marine Protected Area Namuncurá-Burdwood Bank (MPAN-BB). Stations from which Fuegian sprat Sprattus fuegensis where collected for analyzes are indicated according to the year sampled. The MPAN-BB is delimited by a gray solid line. Bathymetry from ETOPO1 Global Relief Model (Amante and Eakins, 2009).
Otolith microstructure analysis has proven to be a powerful tool for fishes’ growth assessment, providing valuable growth information at an individual level (Stevenson and Campana, 1992). Daily increment counts and otolith growth trajectories enable not only age estimations, but also the detection of relevant life-history events and/or environmental influence over growth (Houde, 1989; Stevenson and Campana, 1992). Several studies have addressed the otolith microstructure analysis of young-of-the-year S. sprattus in the Baltic Sea, providing important information regarding growth and recruitment (e.g., Baumann et al., 2006a,b,c, 2007, 2008; Hinrichsen et al., 2010; Voss et al., 2012) and identifying ontogenetic variation in the relationship between fish length and otolith length (Günther et al., 2012). In contrast, few studies have addressed otolith analysis of early S. fuegensis in the Chilean Patagonia (Landaeta et al., 2012) or the SWAO (Sánchez et al., 1995, 1997; Brown and Sánchez, 2010; García Alonso et al., 2018), with growth being mainly assessed by modeling age-size relationships. However, habitat-induced variability over early stages’ growth was reported for the latter, with reduced larval growth in the MPAN-BB compared to TDF (García Alonso et al., 2018).
In the light of these recent findings and given the intrinsic environmental characteristics encountered in TDF and the MPAN-BB, these habitats emerge as suitable settings for evaluating how variation in physical factors can impact early growth of S. fuegensis. Therefore, the aims of the present study were to (1) characterize and compare otolith microstructure of age-0 Fuegian sprats from the MPAN-BB and TDF through the analysis of daily otolith and somatic growth trajectories and to (2) explore possible environmental influence over the early growth of this species by comparing mean growth trajectories with surface seawater temperature variability. Understanding such patterns, would not only provide vital information to further comprehend Fuegian sprat recruitment, but could also constitute pivotal evidence for the generation of new ecosystem-based management strategies policies at the MPAN-BB as well as be used as a baseline to sustainably manage potential extractive activities in the Patagonian Sea.
Materials and Methods
Study Area
The MPAN-BB is a shallow submarine plateau located 150 km east of Isla de los Estados where both spawning and nursery of early Fuegian sprats occur (Figure 1). Delimited by the 200 m isobath, the bank is surrounded by steep flanks of more than 3000 m depth through which strong currents circulate (Piola and Gordon, 1989; Reta et al., 2014; Matano et al., 2019). Intense upwelling and mixing occur over it, entraining deep nutrient-rich waters into the photic layer (Piola and Falabella, 2009; Matano et al., 2019) and resulting in a fairly homogeneous water column both spatially and temporally (Glorioso and Flather, 1995; Guerrero et al., 1999; Matano et al., 2019). Such features enhance the retention of eggs and early stages of Fuegian sprats along with abundant food (García Alonso et al., 2018). Moreover, physical features in the MPAN-BB are fairly stable, with salinity averaging 34 all year round and temperature ranging between 4 and 8°C overall (Guerrero et al., 1999; Acha et al., 2004; Piola and Falabella, 2009).
In contrast, TDF shows far more heterogeneous environmental characteristics. First, due to its shallowness and the proximity to the continent, the mass-heat exchange with the atmosphere is more pronounced than in the MPAN-BB, resulting not only in warmer temperatures, but also in significant seasonal stratification of the water column (Guerrero and Piola, 1997). Second, this habitat encompasses two different geographic regions, the Beagle Channel (BC) and the continental shelf. The BC is a 180 km strait where water is strongly diluted by intense precipitations occurring on the Southeast Pacific Ocean, continental run-offs and glacial melting (Guerrero and Piola, 1997; Piola and Rivas, 1997; Balestrini et al., 1998; Antezana, 1999; Isla et al., 1999). Eggs and early larvae found in the BC are energetically transported to warmer and more saline waters in the continental shelf where the transition to further developmental stages (i.e., transitional larvae/metamorphosing and juveniles) takes place (Sánchez et al., 1995, 1997; Acha et al., 1999).
Sample Collection
Fuegian sprats were collected during three oceanographic surveys carried out by the ARA “Puerto Deseado” oceanographic vessel in November 2014 (spring), March/April 2016 (autumn) and April/May 2017 (autumn) (Figure 1). Hauls were conducted through oblique tows for 5 min at 2–3 knots from 180 m to the surface or less, reaching bottom proximities when possible. Larvae were sampled using a 60 cm diameter Bongo net (300 μm), whereas post-larvae were collected using an Isaacs-Kidd Midwater Trawl (IKMT) employed only in 2017 in the same sampling positions surveyed with the Bongo net. Upon net recovery, several sprats per haul were sorted on board when available and stored frozen for biochemical analyses (article in preparation), while the remaining material was fixed in alcohol 80%. Since in 2014 unsorted sprats were fixed in 4% formaldehyde, they were excluded from this study.
Once in the laboratory, age-0 S. fuegensis from alcohol fixed samples were separated. Their developmental stages were determined distinguishing larval (yolk-sac, preflexion, flexion, and postflexion larvae) and post-larval sprats (transitional-larvae/metamorphosing) (Lebour, 1921; Kendall et al., 1984). Yolk-sac larvae were excluded from this study as they do not deposit daily increments (Alshuth, 1988). Standard lengths (SL) were measured from the tip of the snout to the end of the notochord and corrected for shrinkage (Fey, 1999). Larvae were measured to the nearest micrometer using a Carl Zeiss stereoscope equipped with Axio Vision software, while post-larvae were measured to the nearest millimeter with a scale. The same protocol was applied upon frozen sprats (previously thawed) without correcting SL (Petereit et al., 2008). Their heads were separated and preserved in 80% alcohol for posterior otolith microstructure analyzes.
Otolith Extraction and Microstructure Assessment
Sagittal otoliths were extracted from heads or complete specimens using fine dissection needles. They were cleaned, dried, placed on glass slides and covered with Pro-texx (transparent mounting medium).
Otolith’s daily deposition patterns were established following the recommendations of Campana (1992) and assumed based on observations for S. sprattus (Alshuth, 1988). Otoliths radius (OR) were measured from the core to the edge of the otolith along the longest axis. Daily increments were counted and their widths were measured to the nearest 0.01 μm under a binocular optical microscope (400× or 1,000×) with transmitted light connected to a computer equipped with the Kontron software for image analysis. Because of their thickness, otoliths from sprats larger than 30 mm had to be manually grounded with 12, 9, and 3 μm lapping film papers. A 90% coincidence in the number of increments was assured between the two otoliths from the same individual, and one of them was then randomly selected for posterior analyzes. When only one otolith was available/undamaged, its number of increments was considered.
Data Analyzes
Ages of S. fuegensis were estimated by adding 6 days to the number of increments to compensate for the yolk-sac stage (Alshuth, 1988). Their hatching dates were inferred by subtracting their ages to the corresponding sampling date. Mean SL and their standard errors were calculated according to the habitat, year sampled, developmental stage and hatching season. Differences in larval SL (dependent continuous variable) across habitats and years (independent categorical variables) were statistically compared. Since data did not meet the assumptions of normal distribution (Shapiro–Wilk-test, p < 0.05) and equal variances (Levene’s Test, p < 0.05), a linear model was fitted using generalized least squares (GLS) and variance was modeled with a constant variance function. This approach allowed for both assumptions to be met. The interaction between the independent variables was not significant (p = 0.485) and only the effect of the year was significant (p < 0.001). Therefore, a new model was fitted only considering the latter variable and Tukey HSD post hoc pairwise multiple comparisons were then performed.
Given the short life span of specimens sampled in the 2014 survey, sprats sampled in the autumn surveys (2016 and 2017, i.e., the survivors of the early larval stage which are closer to becoming recruits) were further assessed. The relationship between log-transformed larval SL and OR across different habitats (for each year) or years (within each habitat) was statistically tested through one-way ANCOVAs. A series of linear models were fitted considering SL as the dependent variable, OR and habitat/year as the independent variables. In order to standardize comparisons, only larvae with OR between 100 and 200 μm were included.
Daily growths (DG) were estimated for each individual sprat through proportional back-calculation procedures based on SL-OR relations (Francis, 1990). This approach was chosen due to the restricted ranges of SL shown by each cohort, allowing to estimate previous SL within a same cohort based on the corresponding otolith size. Preliminary assessment of the SL-OR relations evidenced distinct trajectories for different Fuegian sprats’ developmental stages, being curvilinear along the larval phase and linear across the post-larval stage. Thus, a segmented approach was adopted and different growth models were employed to model the larval and post-larval phases, respectively (Laidig et al., 1991; Günther et al., 2012). Given the allometric relationship observed along the larval phase, a power function was selected to fit OR-SL data of sprat larvae (Eq. 1):
where SLt is the standard length at a given time t, ORt the otolith radius at time t, and a and b the parameters of the power function. Parameters a and b of Eq. 1 were estimated for each larva by solving a system of two equations considering measures at capture (SLc and ORc) and at the first feeding day (SL0 and OR0), thus employing a biological intercept (Campana, 1990; Watanabe and Kuroki, 1997). OR0 corresponded to OR at first increment deposition and SL0 was established at 5.72 mm resembling the SL of the smaller larva sampled in its first feeding day (Age0). SL at each day (i.e., SL at each ORt) were back-calculated employing the individual estimated parameters with Eq. 1 and daily growth rates were then estimated as the difference in SL between two consecutive days (mm d–1).
On the other hand, a linear regression was chosen to fit OR-SL data during the post-larval phase (Eq. 2):
where c and d are the parameters of the linear equation. Given the different SL-OR relations evidenced by larval and post-larval stages, the growth trajectory of each post-larva was reconstructed by segmenting data and modeling each phase independently (Figure 2). To do so, we first estimated the point in which the growth trajectories shifted from curvilinear to linear by fitting general power and linear models to SL-OR data of larvae and post-larvae, respectively, by means of non-linear least squares. The interception between both models was estimated at ORi and SLi (Figure 2A) and the mean age at which ORi is attained (Agei) was interpolated (Figure 2B). Parameters c and d were then estimated by solving a system of two equations considering measures at capture (SLc and ORc) and at the interception (ORi and SLi) and SL during the post-larval phase were back-calculated with Eq. 2 from capture until Agei (Figure 2C). SL during the larval phase of these specimens were estimated with Eq. 1 from Agei to Age0 as described above for sprat larvae considering measures at the first feeding day (SL0 and OR0) and at the interception (ORi and SLi). Results for larval and post-larval phases per specimen were concatenated, and daily growth rates were calculated as the difference in SL between consecutive days.
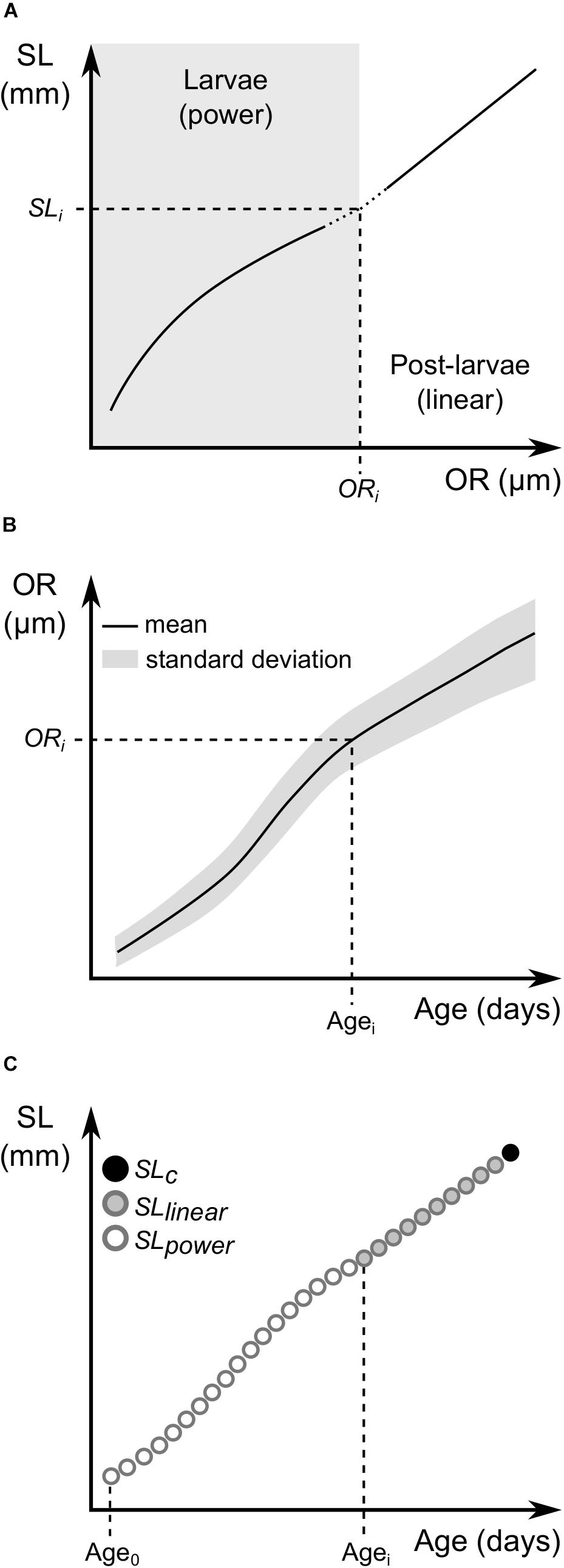
Figure 2. Schematic illustration of post-larval daily growth back-calculations. (A) General models were fitted to otolith radius (OR) and standard length (SL) relations according to developmental stage: a power relation for larvae (gray panel) and a linear function for post-larvae. The interception between both models was estimated at ORi and SLi. (B) The mean age at which ORi is attained (Agei) was interpolated. (C) SL back-calculations were performed from capture (SLc) to Agei applying a linear model (SLlinear) and from Agei to the first feeding day (Age0) with a power model (SLpower). SL of both stages from each specimen were concatenated and daily growth rates were estimated as the difference in SL between consecutive days.
Increment widths and DG data were pooled in different groups accounting for each combination of habitat, year sampled and hatching season, and mean values and their standard deviations were estimated. Otolith and somatic growth trajectories in relation to age were graphically assessed by averaging IW and DG of each group every 10 days. IW and DG values were statistically compared at 5 different ages (20, 40, 60, 80, and 100 days) across habitats, years or hatching seasons by means of repeated measure analyses. In order to comply with the requirement of identical record lengths, individuals with one or more missing values at these ages were excluded. Given the nature of the response variables, different approaches were applied for IW and DG data, respectively. In the case of IW, data was modeled using a Gaussian distributed GLS whereas for DG, generalized estimation equations (GEE) with a Gamma distribution were applied. Several models of covariance structure were tested for both IW and DG. The best model was identified using the Akaike’s information criterion (AIC; Akaike, 1974) for GLS models and the quasi-likelihood information criterion (QIC; Pan, 2001) for GEE models. Interaction between factors (habitat, year or season of hatching) and age was assessed in all the selected
models. When such interaction was significant, post hoc pair-wise comparisons for every combination of levels were carried out using least-squares and adjusting p-values with a Bonferroni correction. Otherwise, the main effect of each factor was assessed and a post hoc pair-wise comparison was performed across levels of the factors. Seasonal variability in the NMPA-BB was not assessed due to an insufficient number of observations.
Temperature variability was evaluated both spatially and temporally. Knowing that major abundances of Fuegian sprats usually occur in the sub-surface (Landaeta et al., 2013; Contreras et al., 2014), sea-surface temperature (SST) was assessed, thus allowing the acquisition of temperatures registered in periods not sampled. Time series were obtained through the Ocean Time Series Investigator App developed by Google Earth Engine (Gorelick et al., 2017). This platform computes level 3 sea surface temperature data from the MODIS-Aqua dataset (NASA, 2019). Five sites per habitat were chosen in order to cover the overall extension of the sampled areas. Data was downloaded, outliers were removed and smooth conditional means were displayed for each habitat using a “gam” smoothing function. Regression analyzes using generalized least squares were conducted to assess temperature variability (response continuous variable) across habitats and across years within each habitat separately (explanatory categorical variables), comparing equivalent date intervals in the latter. When detected, variance heterogeneity was modeled. The effect of temperature over patterns of growth trajectories was graphically assessed by aligning mean IW and DG trajectories of the groups considered to the corresponding SSTs registered. Pearson correlation coefficients between SST and IW and DG were estimated. To account for ontogenetic related variability, age-detrended IW and DG (i.e., residuals of the models of each variable in relation to age) models were used in the correlations.
Data analyzes were carried out within the R environment (R Core Team, 2019) with the stats (R Core Team, 2019), plyr (Wickham, 2011), tidyr (Wickham and Henry, 2018), dplyr (Wickham et al., 2019b), scales (Wickham, 2018), nlme (Pinheiro et al., 2018), geepack (Halekoh et al., 2006), lsmeans (Lenth, 2016), multcomp (Hothorn et al., 2008), modelr (Wickham, 2020), tidyverse (Wickham et al., 2019a), broom (Robinson and Hayes, 2020) and car (Fox and Weisberg, 2011) packages. Figures were visualized with ggplot2 (Wickham, 2016) and edited with the open-source software Inkscape1.
Results
A total of 217 individuals were analyzed in this study, with 134 specimens sampled in the MPAN-BB and 83 in TDF. Larval sprats were found in all surveys in both habitats, while post-larval specimens were only captured during the autumn survey of 2017 in TDF (Table 1). Larvae sampled in the spring survey (2014) were the smallest sprats analyzed overall (p < 0.001), displaying mean SL of 9.12 and 8.21 mm for the MPAN-BB and TDF, respectively. Their ages varied between 6 and 21 days. Due to their short life span, the hatching season estimated resembled that of the survey. On the other hand, larvae from the autumn surveys (2016 and 2017) evidenced higher mean SL values ranging from 15.61 to 26.73 mm. Values measured in 2017 where significantly higher than those measured in 2016 in both habitats (p < 0.001). In terms of age, a wider range was observed both between and within habitats in 2016 and 2017 compared to 2014, with a range of more than 90 days of age among larvae from the MPAN-BB in 2016. This variability was replicated by the estimated hatching seasons, registering larval hatching during summer-autumn (2016) and spring-summer (2017) in the MPAN-BB but exclusively in summer in TDF. Furthermore, larvae in the MPAN-BB attained older ages than in TDF in both autumns sampled. Finally, post-larvae found in TDF had hatched entirely in spring. SL varied between 33.00 and 51.00 mm while ages ranged from 155 to 202 days. The oldest larvae found in 2017 in TDF were at least 40 days younger than post-larvae. On the contrary, older summer-born larvae from the MPAN-BB were 3 days younger, with the only spring-born larva being even older (165 days) than the youngest post-larvae (155 days).
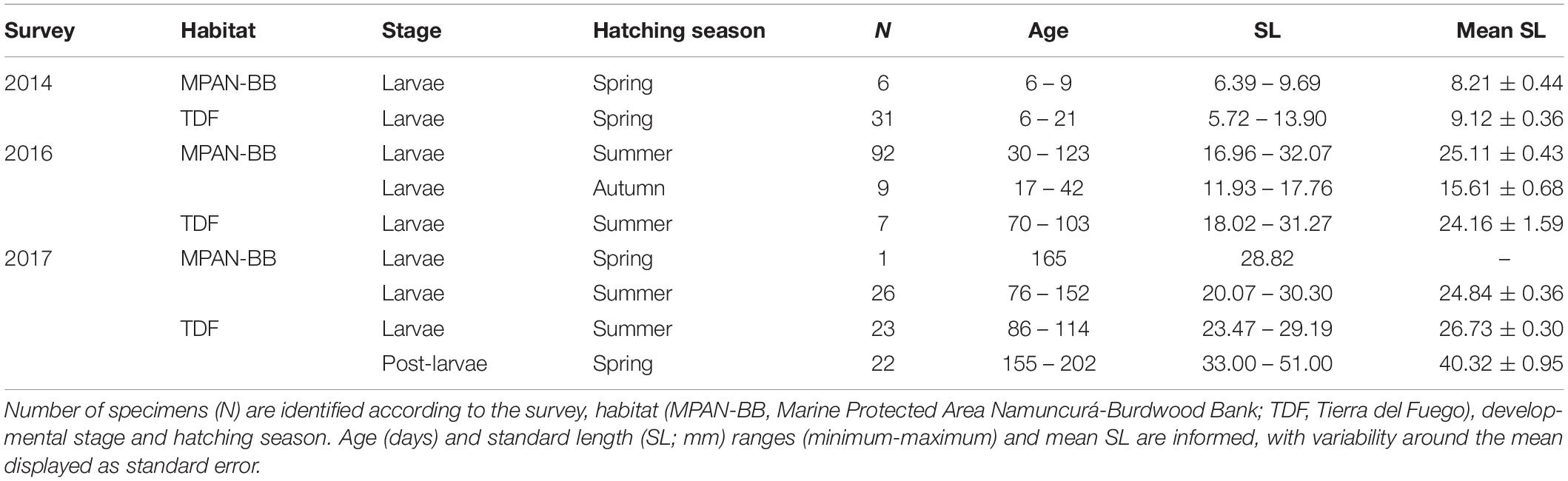
Table 1. General information of Fuegian sprats Sprattus fuegensis analyzed in the Southwest Atlantic Ocean.
The relationship between SL and OR revealed a marked ontogenetic variability (Figure 3). Regardless of the habitat and year sampled, larval SL-OR data displayed a distinct curvilinear relation. When statistically assessed, the interaction between OR and habitats/years (i.e., independent variables) were not significant in neither of the linear models assessed. Comparisons of the SL-OR relationships across habitats for each year sampled revealed inter-habitat differences. The MPAN-BB evidenced higher SL at equal OR compared to TDF in 2016 (ANCOVA, F1,51 = 26.645; p < 0.001), whereas it was the other way around in 2017 (ANCOVA, F1,45 = 5.446; p = 0.024). Also, a significant inter-annual difference was detected across older larvae sampled in the autumn surveys only in the MPAN-BB, attaining equal SL with smaller OR in 2016 than 2017 (ANCOVA, F1,70 = 145.012; p < 0.001). Post-larvae, on the other hand, exhibited a linear relation between SL and OR. The estimated age at which the transition between growth models occurred was approximately 110 days.
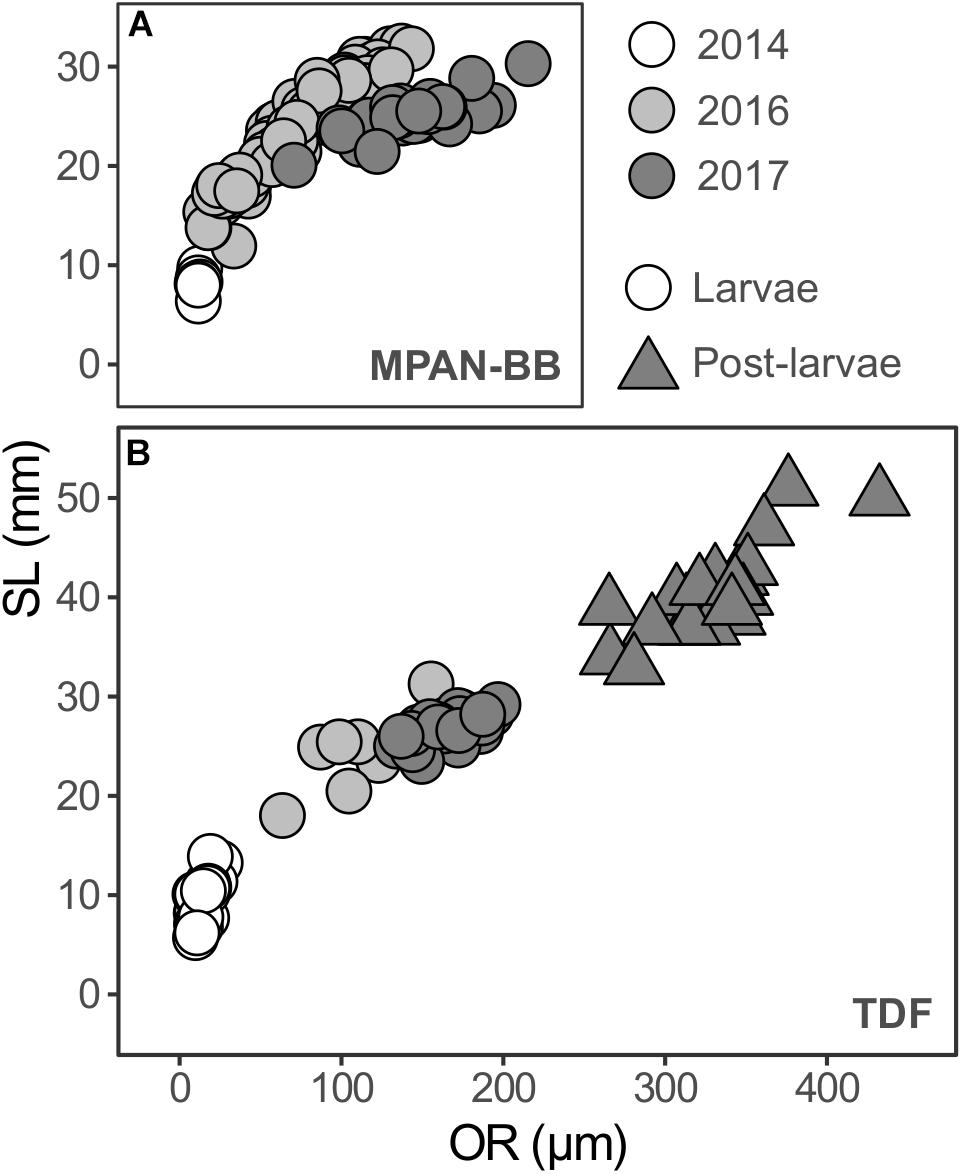
Figure 3. Otolith radius (OR; μm) and standard length (SL; mm) relationships of Fuegian sprat Sprattus fuegensis in the Southwest Atlantic Ocean. Data is differentiated according to developmental stage, the survey in which sprats were captured and the habitat sampled: the Marine Protected Area Namuncurá-Burdwood Bank (MPAN-BB) (A) and Tierra del Fuego (TDF) (B).
Increment Width Variability
Otolith IW of sprats sampled in the autumn surveys (see Table 1) varied between 0.17 and 6.23 μm overall, with the highest values measured in sprats captured in TDF in 2017 (Table 2). Both differences and similarities were recognized in otolith growth trajectories across habitats, hatching seasons and/or years sampled (Figure 4). Small IW were observed during the early larval phase (first 20 days) and did not vary significantly across the groups assessed in general, although they did differ across habitats when comparing summer-born larvae in 2016 and 2017, with values in both years being higher in TDF (Table 3). Further differences were observed across habitats and/or hatching seasons. Spring-born sprats sampled in TDF in 2017 displayed a clear dome-shaped deposition pattern, with major IW deposited around 90 days of age (Figure 4D) and with values close to the peak of the dome (60, 80, and 100 days) being significantly higher than those of summer-born sprats of that same year and habitat (Table 3). A similar vaulted pattern was exhibited by the only spring-born sprat analyzed in the MPAN-BB (Figure 4A), though the post-peak decline was more gentle. In contrast, summer-born sprats (Figures 4B,E) achieved a plateau of relatively slow variation in their IW after the first 40–50 days of age. Sprats from TDF (Figure 4E) remained in that plateau when captured, while a slow decrease was evidenced posterior to the plateau in the MPAN-BB (Figure 4B). Also, inter-habitat variability was statistically corroborated for summer-born sprats, with higher IW deposited in TDF in 2017 for all ages compared, and for the increments at 20 and 40 days of age in 2016 (Table 3). Inter-annual variability among summer-born sprats was graphically observed for both habitats, with curves in 2017 exhibiting higher values than 2016. When statistically assessed, such variability was corroborated at 60 and 80 days of age in TDF and only at 40 days of age in the MPAN-BB (Table 3). Finally, sprats born in autumn in the MPAN-BB evidenced constant IW of approximately 0.75 μm in spite of increasing age (Figure 4C).
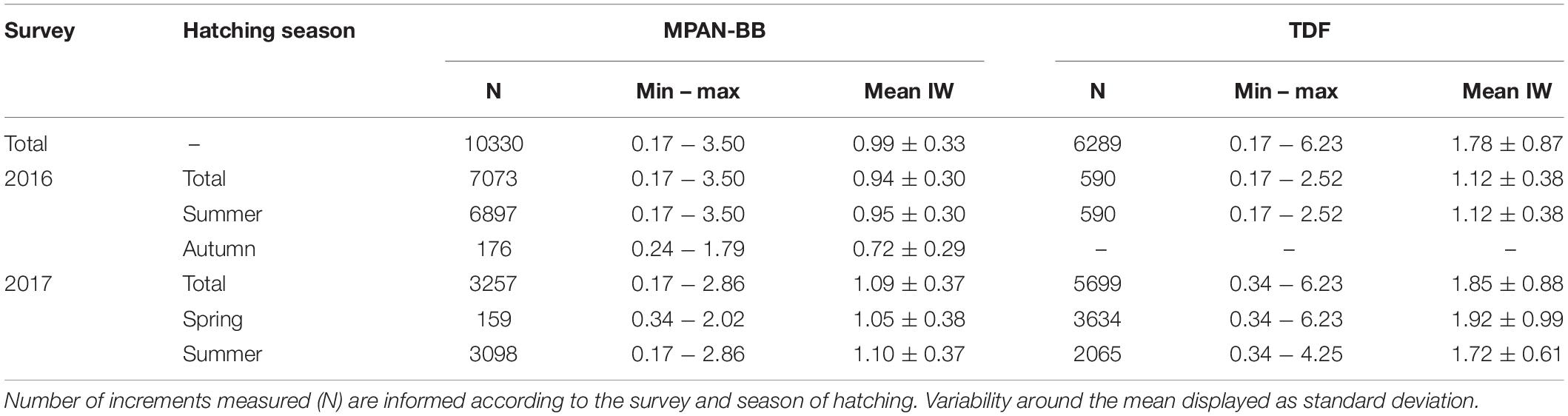
Table 2. Minimum (min), maximum (max) and mean otolith increment widths (IW; μm) of Fuegian sprats Sprattus fuegensis analyzed in the Marine Protected Area Namuncurá-Burdwood Bank (MPAN-BB) and Tierra del Fuego (TDF).
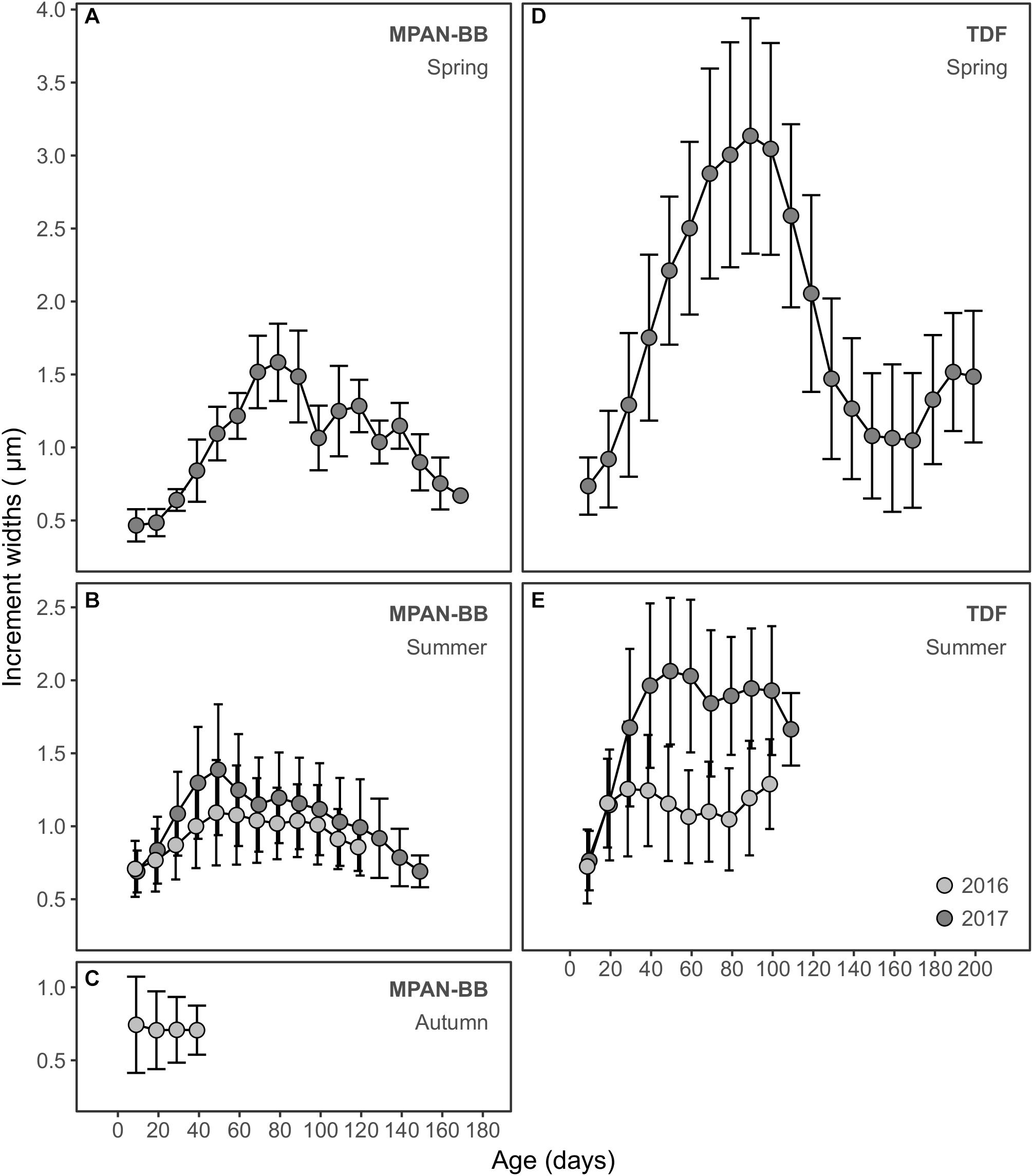
Figure 4. Otolith increment widths (μm) in relation to age (days) of Fuegian sprat Sprattus fuegensis in the Marine Protected Area Namuncurá-Burdwood Bank (MPAN-BB) (A–C) and Tierra del Fuego (TDF) (D–E). Records are grouped according to the year sampled (colors) and season of hatching: spring (A,D), summer (B,E) and autumn (C). Increment widths are averaged every 10 days, with variation around the mean displayed as standard deviations.
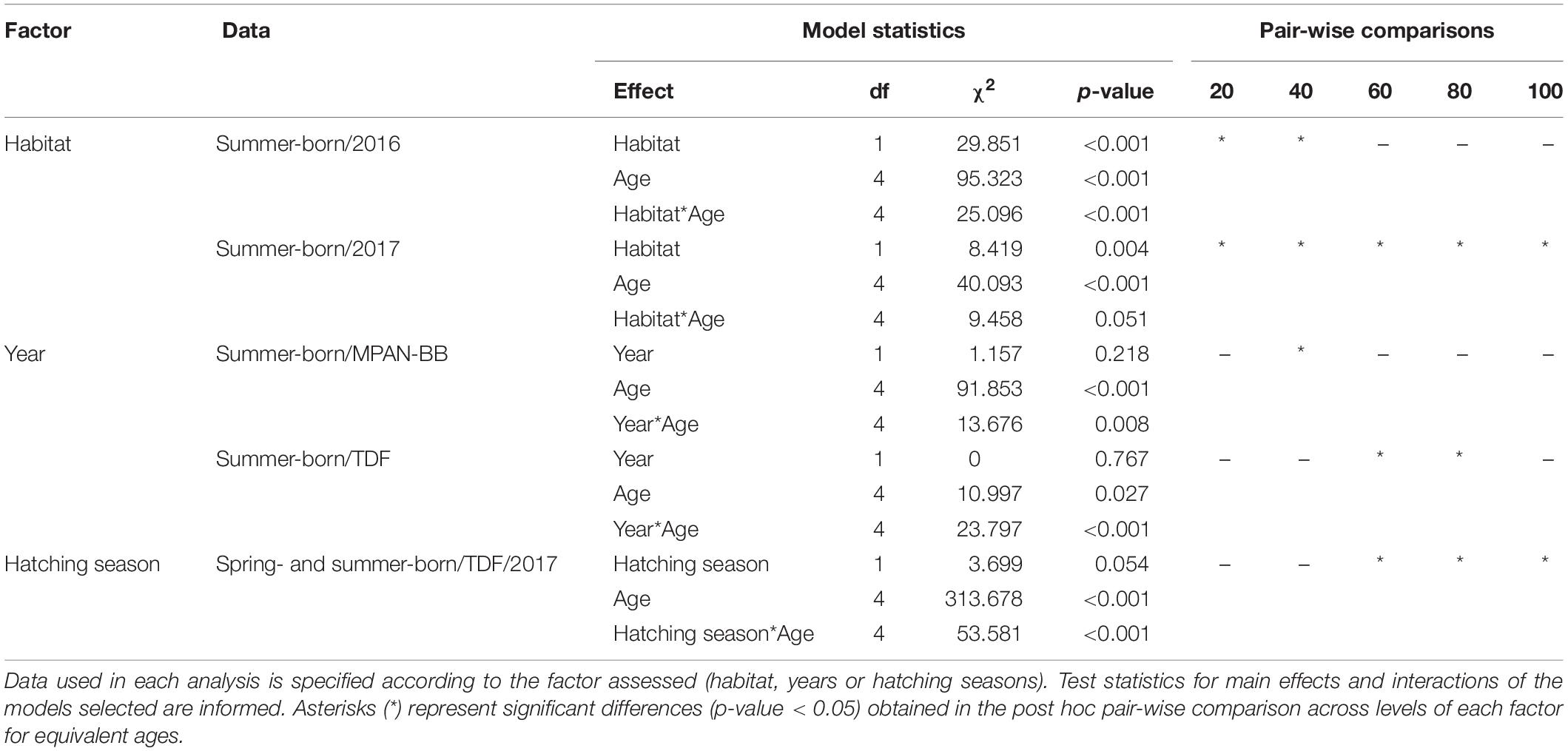
Table 3. Results of repeated measure analyses of otolith increment widths at 20, 40, 60, 80, and 100 days of age of Fuegian sprats Sprattus fuegensis in the Marine Protected Area Namuncurá-Burdwood Bank (MPAN-BB) and Tierra del Fuego (TDF).
Comparisons of Daily Growth
Estimated DG varied between 0.02 and 1.52 mm d–1 overall, exhibiting different ranges across the groups assessed (Table 4). Growth trajectories also varied spatially and seasonally (Figure 5). Spring-born sprats displayed a dome-shaped pattern in their DG trajectories, similar to the one described for their IW, though in this case both habitats attained the highest DG at 60 days of age and their maximum values were not as uneven (Figures 5A,D). When compared with summer-born sprats captured in 2017 in TDF, DG at 60 and 80 days of age of spring-born sprats in TDF were significantly higher in the latter case (Table 5). DG of summer-born sprats did not resemble their IW growth patterns. In TDF (Figure 5E), DG increased from hatching until 20–30 days of age and decreased afterward, while in the MPAN-BB growth was maximum at the early larval phase and declined after the first 50 days of age (Figure 5B). Inter-annual variability also differed according to the habitat analyzed. The dissimilarity recognized in TDF laid in the position of the peak of maximum DG, occurring later for sprats sampled in 2017 than in those captured in 2016. However, DG did not statistically differ in neither of the ages assessed (20, 40, 60, 80, and 100 days of age) (Table 5). On the contrary, differences in the MPAN-BB did not involve variability in the shape of the trajectory but rather in the magnitude of the estimated values, with DG being significantly higher in sprats sampled in 2016 at all ages compared (Table 5). Finally, DG of sprats born in the MPAN-BB in autumn of 2016 decreased from hatching to capture (Figure 5C). Although they exhibited the maximum DG rates estimated, this group also displayed the major variability observed (in standard deviations) among all the groups considered.
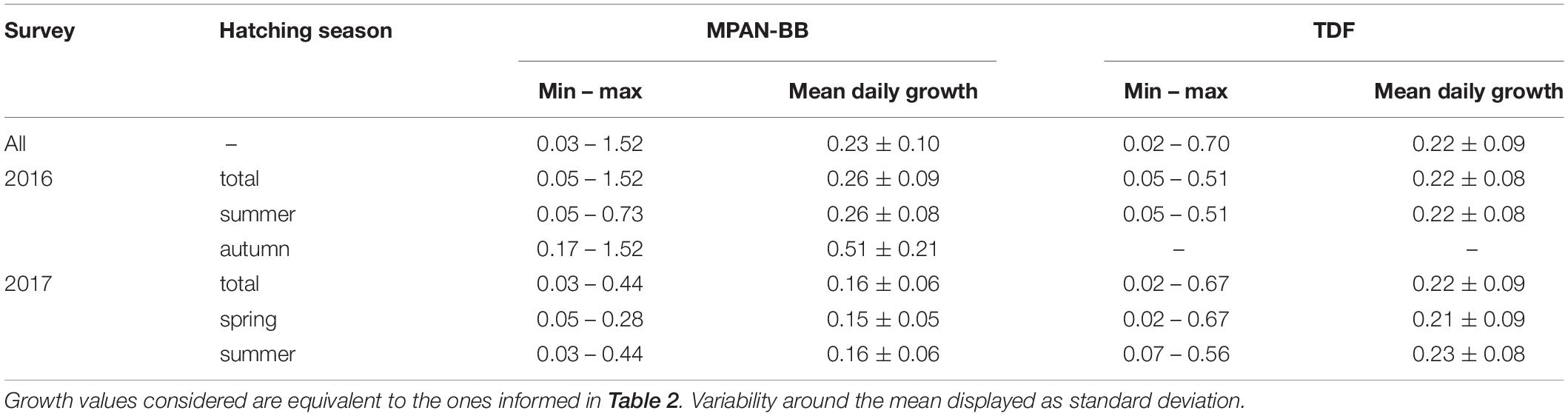
Table 4. Minimum (min), maximum (max) and mean daily growth rates (mm d–1) of Fuegian sprats Sprattus fuegensis sampled in the autumn surveys in the Marine Protected Area Namuncurá-Burdwood Bank (MPAN-BB) and Tierra del Fuego (TDF).
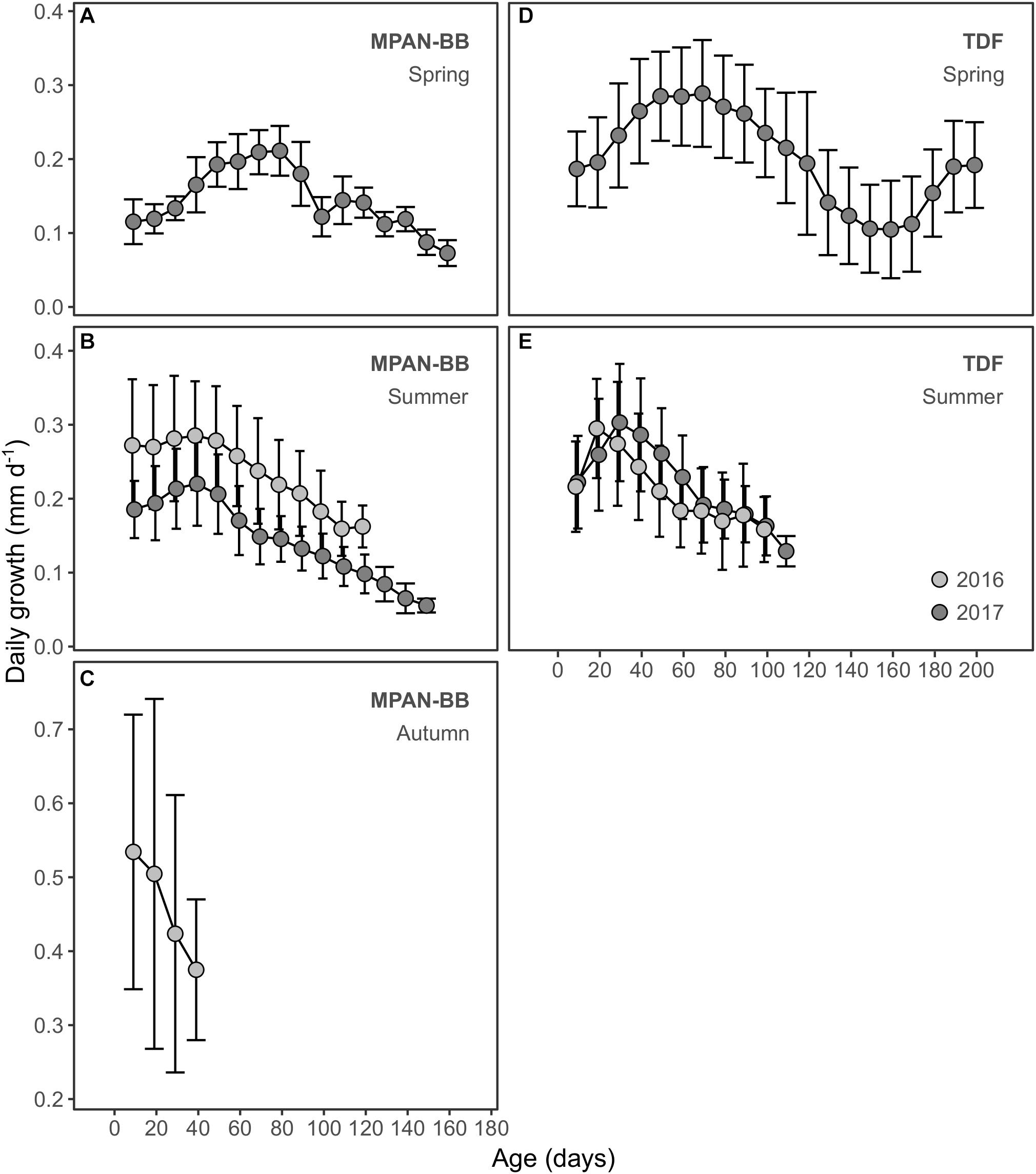
Figure 5. Daily growth (mm d–1) in relation to age (days) of Fuegian sprat Sprattus fuegensis in the Marine Protected Area Namuncurá-Burdwood Bank (MPAN-BB) (A–C) and Tierra del Fuego (TDF) (D–E). Records are grouped according to the year sampled (colors) and season of hatching: spring (A,D), summer (B,E) and autumn (C). Daily growths are averaged every 10 days, with variation around the mean displayed as standard deviations.
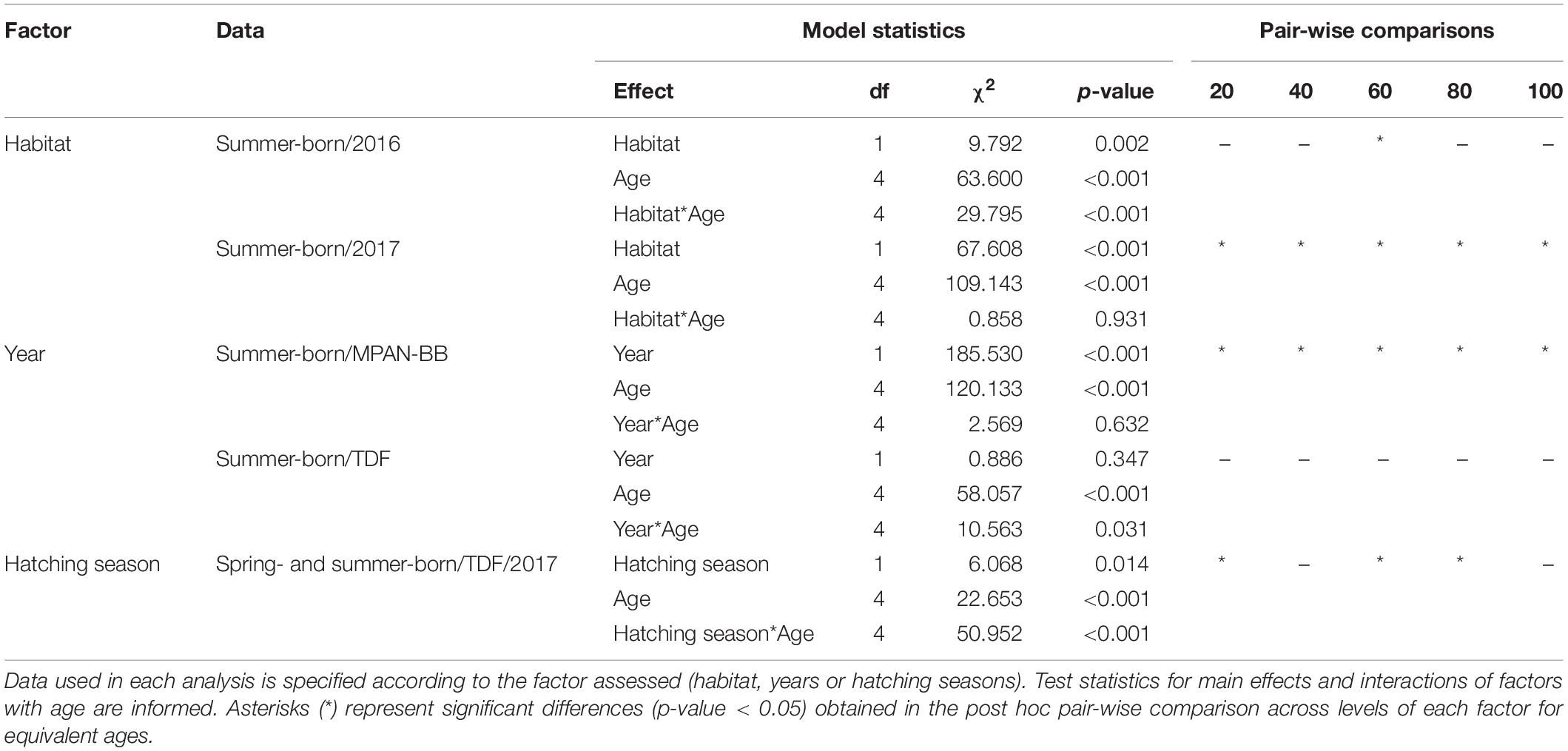
Table 5. Results of repeated measure analyses of daily growth rates at 20, 40, 60, 80, and 100 days of age of Fuegian sprats Sprattus fuegensis in the Marine Protected Area Namuncurá-Burdwood Bank (MPAN-BB) and Tierra del Fuego (TDF).
Growth Trajectories and Temperature Time Series
Sea-surface temperature was assessed from December of 2015 (month of the first estimated hatching date) to June of 2017 (subsequent month to the last sampled date in the 2017 survey) in both habitats (Figures 6A,B). Temperatures differed significantly across habitats (chi-square = 123.66, df = 1, p < 0.0001), being higher in TDF (8.10 ± 1.94°C) than in the MPAN-BB (6.35 ± 1.19°C). Minimum temperatures registered (winter) were similar across habitats (approximately 3°C). In contrast, maximum temperatures (summer) were higher in TDF (11.69°C) (Figure 6B) than in the MPAN-BB, where they did not surpass the 9°C (Figure 6A). This difference was responsible for the wider temperature range of the former (8.33°C) compared to the latter (5.87°C). Moreover, inter-annual variability was also observed within habitats, with higher temperatures being registered in the summer of 2017 (compared to 2016) (chi-square = 38.49, df = 1, p = 0.0024 for TDF and chi-square = 60.61, df = 1, p < 0.0001 for the MPAN-BB).
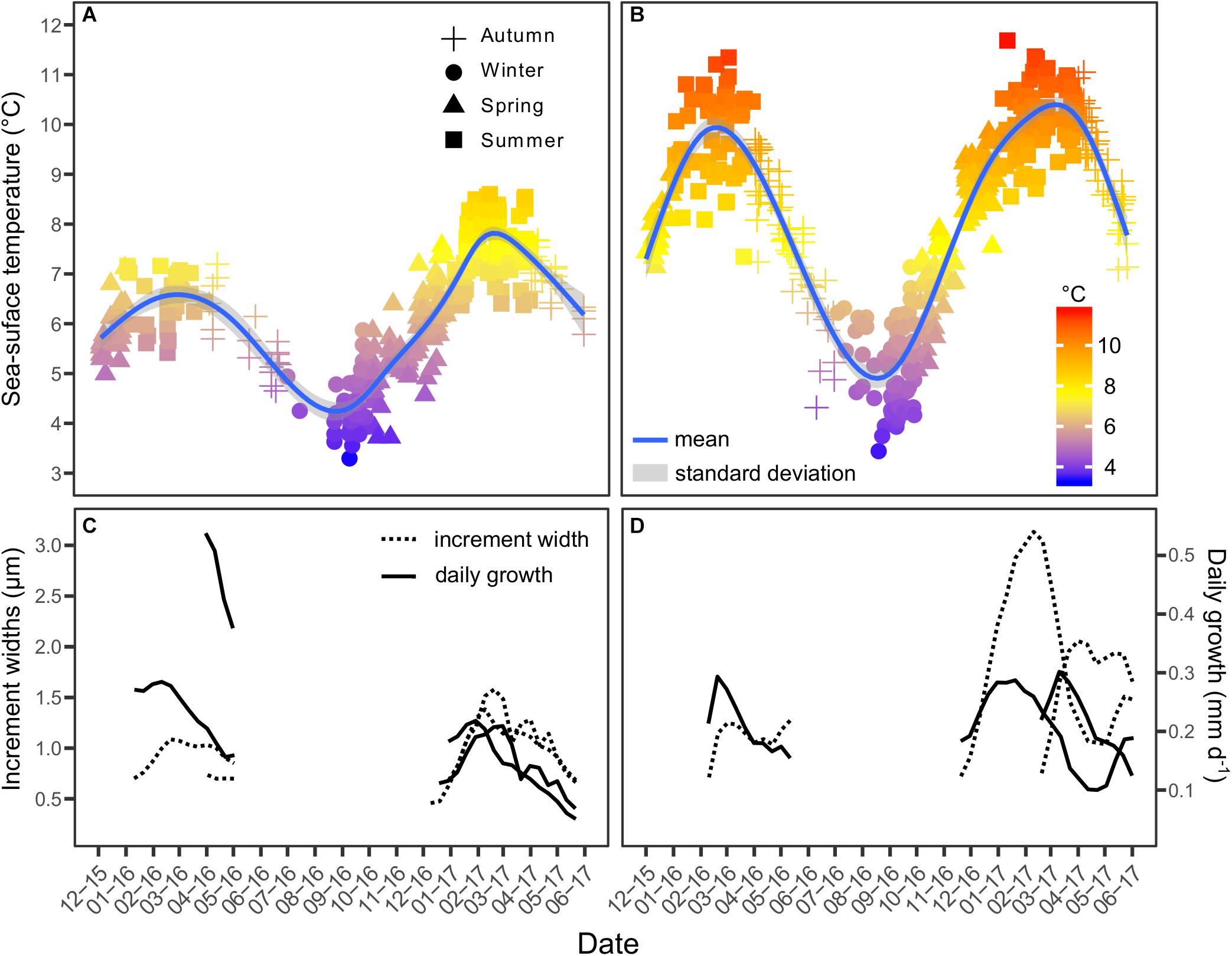
Figure 6. Sea-surface temperature (°C) time series in the Marine Protected Area Namuncurá-Burdwood Bank (MPAN-BB) (A) and in Tierra del Fuego (TDF) (B). Sites from which data was obtained are shown in the reference maps. Shapes indicate the season of the record. Mean trajectories of increment widths (μm; dotted lines) and daily growths (mm d–1; solid lines) for each habitat, survey and season of hatching are displayed (C–D).
Major coupling between mean growth trajectories of Fuegian sprats and SST was found for most of the groups considered (i.e., sprats according to the habitat, year sampled and season of hatching) (Figures 6C,D). The moment at which maximum IW and DG were first attained occurred roughly at the same time at which SST did in both habitats. The only exception was observed in spring-born sprats of TDF (larger trajectories in Figure 6D) sampled in 2017, which attained IW and DG peaks more than a month before temperature did. Although IW trajectories varied considerably after these peaks, DG consistently declined after these maximum values in all cases considered, therefore following the decrease in SST after its peak in summer.
Pearson correlation coefficients evidenced a significant positive correlation between SST and age-detrended IW and DG in 2017 for all the groups considered (Table 6), year in which maximum SST temperatures were registered. On the contrary, coefficients estimated for 2016 differed across groups, being positive for sprats from TDF, but mainly negative for sprats from the NMPA-BB. However, only the correlation between summer-born sprats’ IW from the NMPA- BB was significant for the groups assessed in 2016.
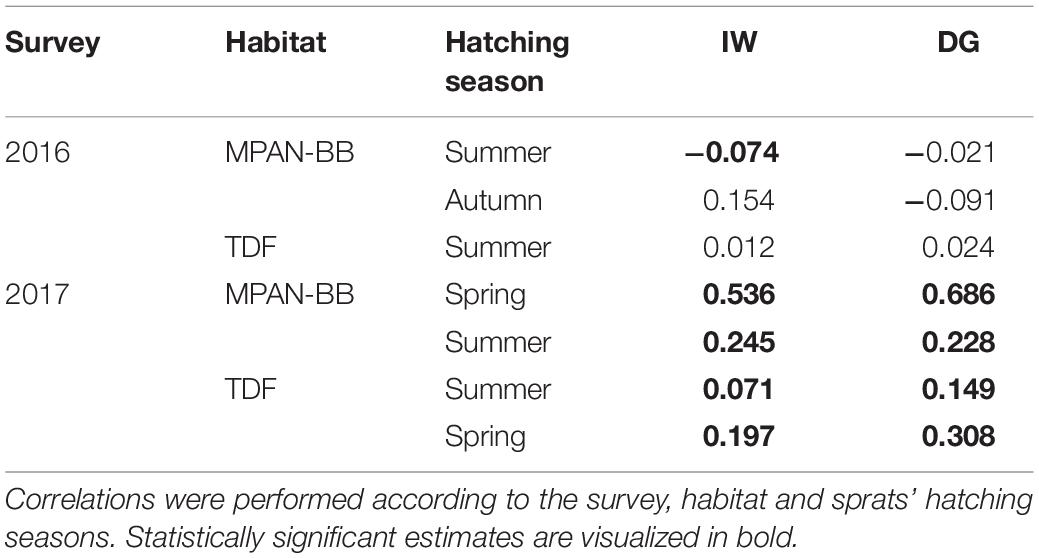
Table 6. Pearson correlation coefficients for age-detrended increment widths (IW) and daily growth rates (DG) of Fuegian sprats Sprattus fuegensis in relation to sea surface temperature in the Marine Protected Area Namuncurá-Burdwood Bank (MPAN-BB) and Tierra del Fuego (TDF).
Discussion
Otolith microstructure analyzes provide important life-history knowledge (Campana and Neilson, 1985; Stevenson and Campana, 1992; Morales-Nin, 2000; Sponaugle, 2010), with information from field-caught fishes being particularly valuable for species which are difficult to rear in the laboratory, such as sprats (Peck et al., 2004; Baumann et al., 2005; Petereit et al., 2008; Leal et al., 2017). In this context, collecting samples in two environmentally distinct habitats represented a unique opportunity to evaluate early growth variability of Fuegian sprats in relation to physical characteristics such as temperature. However, sampling in an open-sea area as the MPAN-BB is both logistically and meteorologically challenging. Recurrent oceanographic surveys were recently carried out in this submarine plateau of high biodiversity and conservational importance (e.g., Schejter et al., 2016; Fraysse et al., 2018; Di Luca and Zelaya, 2019; Schejter and Bremec, 2019) and its adjacent areas as a result of the implementation of the Argentinian national initiative “Pampa Azul”. Results shown here not only represent the first comparison of age-0 S. fuegensis growth trajectories from these spawning/nursery grounds of the SWAO, but also represent an update on early sprats’ knowledge sampled for the last time over 20 years ago.
Determining when successful recruits are predominantly produced represents crucial information in the attempt to understand the recruitment dynamic of batch spawner fishes (Baumann et al., 2008) such as the Fuegian sprat (Shirokova, 1978; Hansen, 1999). Survivors sampled in the autumn surveys (2016 and 2017) were born during spring/summer in TDF or spring/autumn in the MPAN-BB. In the latter, only one spring-born specimen was captured during these surveys, with no post-larvae being captured at all, thus suggesting a selective survival of later born sprats. Colder conditions experienced in spring may be disadvantageous for larval development (Baumann et al., 2008), although this warrants a more detailed assessment since the response to cold water temperatures of clupeid larvae can vary rapidly and is species-specific (Molina-Valdivia et al., 2020). Also, density-dependent control (Baumann et al., 2007) could be taking place during this period of major spawning in the MPAN-BB (García Alonso et al., 2018). However, in the light of recent results from numerical models addressing water circulation in the bank (Matano et al., 2019), combined with the fact that sprats generally reside close to coastlines (Whitehead, 1985), an alternative explanation for this outcome implies that nursery of these older/bigger specimens may not occur within the bank. In this regard, the Malvinas (Falkland) Islands area arises as the most probable destination given their geographic proximity and estimated water circulation pattern. In TDF, similar cautions should be taken before jumping into conclusions over selective survival. Spring-born were only captured with the IKMT net in 2017, an appropriate sampling gear for bigger/faster specimens (Fey, 2015). Although the absence of spring-born sprats in 2016 could be the result of selective survival of summer-born specimens, this outcome is most likely to be reflecting the selectivity of the net employed. Employing the IKMT net and broadening the sampled areas are therefore necessary to untangle these uncertainties.
As previously mentioned, otolith microstructure analysis is a powerful tool which can provide growth information of life-history events and environmental effects over growth. Before analyzing otolith growth trajectories, sprats’ ages and developmental stages already provided strong evidence of differential growth across habitats. Spring-born sprats of 155 days or older were post-larval specimens in TDF, with transition estimated to occur around 110 days of age. However, several sprats captured in the MPAN-BB were more than 110 days old, reaching similar ages (152 days) or even older (165 days) than post-larvae from TDF, yet still evidencing larval morphology, thus further supporting a protracted larval stage in the former habitat (García Alonso et al., 2018). Stage durations tend to be both longer and potentially more variable in higher latitudes subject to colder temperatures (Houde, 1989). Although the MPAN-BB occupies a similar latitudinal range than TDF, this habitat is subject to colder temperatures which would account for this difference in larval stage duration.
Modeling age-size relations constitutes one of the most common methodologies used to assess early growth of S. fuegensis in both the Southeast Pacific Ocean (Cerna et al., 2014; Leal et al., 2017) and the SWAO (Sánchez et al., 1995, 1997; Brown and Sánchez, 2010; García Alonso et al., 2018). Given that collected sprats corresponded to different cohorts and the restricted SL ranges covered, back-calculating previous SL based on SL-OR relations (Stevenson and Campana, 1992) was the chosen methodology to estimate daily growth rates in this study. Many authors employed otolith growth (i.e., IW or mean standardized IW) as a direct proxy for somatic growth (e.g., Baumann et al., 2006a, 2008; Hinrichsen et al., 2010). However, in the light of differential SL-OR relationships observed among larval and post-larval stages (Günther et al., 2012; this study) and with IW being highly autocorrelated and age dependent (Hinrichsen et al., 2010), modeling SL-OR relations and generating SL daily back-calculations arises as a more adequate approach in the estimation of DG rates of Fuegian sprats. Furthermore, inter-annual variability should also be considered when pooling data from a particular population. Increment deposition during the firsts 20 days of age of Fuegian sprats did not vary considerably across habitats nor hatching seasons. Even more, they closely resembled the pattern described for the Chilean Patagonian population of S. fuegensis (Landaeta et al., 2012). However, when comparing data from the autumn surveys, OR in the year 2017 were wider that in sprats of equivalent SL in 2016 in both habitats. This was a direct consequence of wider IW being deposited in 2017 (Table 2) in accordance to higher temperatures registered in both habitats during that year (Figure 6).
Besides selecting adequate SL-OR models (Günther et al., 2012), other considerations should also be taken when assessing sprats’ growth on the basis of otolith IW such as otolith growth-somatic growth decoupling. Decoupling in length and otolith growth of age-0 Fuegian sprats has been previously reported (García Alonso et al., 2018), with variable environmental conditions including feeding condition, salinity and vertical stratification (Baumann et al., 2005; Landaeta et al., 2012; Zenteno et al., 2014) and/or ontogenetic shifts (Günther et al., 2012) being among the possible causes for such decoupling. Our results not only yield evidence supporting the existence of otolith length-fish length decoupling in Fuegian sprats in all the groups considered (Contreras et al., 2017), but also suggest that this decoupling becomes more pronounced toward the end of the hatching period. Spring-born sprats from TDF only evidenced a dissociation between IW and DG peaks but did not display major variations in the overall trajectories, with moderate decoupling exhibited in the MPAN-BB overall. On the contrary, summer-born sprats in both habitats did show distinct IW and DG patterns. For sprats sampled in TDF, decoupling was observed after the first 30 days of age during which IW remained in a plateau of high values, but DG constantly decreased. A similar decoupling was detected for sprats sampled in the MPAN-BB, but this discrepancy took place between 50 and 100 days, after which IW trajectories started decreasing. Moreover, autumn-born sprats not only evidenced discordant growth trajectories patterns, but displayed the lowest IW and highest DG (Figure 6), further supporting the idea that estimating somatic growth by direct comparison with IW values could lead to major inconsistencies.
Notwithstanding the particular differences mentioned above, an important general coupling was observed between these growth trajectories’ patterns and SST time series, thus corroborating an important effect of temperature variability over them. With the exception of spring-born sprats sampled in 2017 in TDF, the dates at which maximum temperatures were registered correlated with the moment at which maximum IW and DG values were first attained, with DG trajectories closely resembling temperature patterns overall (Figure 6). Furthermore, significant positive correlations were estimated for both IW and DG with SST in most of the cases considered (Table 6) further supporting such association. However, although higher DG are expected to occur in warmer environmental conditions, highest mean DG values of 0.26 and 0.51 mm d–1 were estimated for summer- and autumn-born sprats in the MPAN-BB in 2016, habitat and year with the coldest maximum temperatures registered. In fact, the mean growth of autumn-born sprats from the MPAN-BB was higher than the one estimated for S. fuegensis from Chilean Patagonia (0.45 mm d–1) where temperatures measured were about 2°C above the ones measured in the NMPA-BB (Landaeta et al., 2012). Lower temperatures could be advantageous for faster growth in the NMAP-BB by setting lower food ingestion requirements to support growth in such a cold habitat (Houde, 1989). Salinity could also be considered a possible factor affecting growth, however, Landaeta et al. (2012) found that this environmental forcing did not significantly correlate with larval otolith growth of S. fuegensis. Another possible explanation for this outcome lies in the high phytoplankton biomass detected during the 2016 survey over the MPAN-BB, composed mainly of Rhizosolenia crassa diatom (Bértola pers. comm.). Although these organisms are not the most adequate food source for Fuegian sprats, their high abundances indicates the existence of favorable environmental conditions for phytoplankton proliferation and, thus, zooplankters which are the main prey items in S. fuegensis diet.
Further analyzes are still necessary to unravel underlying mechanisms during early development of Fuegian sprats not evaluated yet. However, the results from the present study comprise a fundamental baseline in the understanding of environmental variation over growth of age-0 S. fuegensis in the SWAO. Strong correlation with temperature appears to be a key factor implied in growth modulation, but the habitat, seasonal and inter-annual variability observed revealed a high complexity in the early development of this species, which in turn can influence recruitment success. Biological mechanisms involved in the multiple processes simultaneously affecting recruitment need to be thoroughly understood before predictions and/or suggestions can be proposed (Baumann et al., 2006b; Voss et al., 2012). Nonetheless, otolith microstructure analyzes emerges as powerful tool in the assessment of life-history of Fuegian sprats, and information gathered in this study provides keystone information upon which adequate ecosystem based management strategies for the different habitats existing in the SWAO can be based on.
Data Availability Statement
The datasets generated for this study are available on request to the corresponding author.
Ethics Statement
The research reported here has been conducted in an Ethical and responsible manner, complying with all applicable international, national, and/or institutional guidelines for the care and use of fish larvae and post-larvae.
Author Contributions
VG and FC conceived and designed the study. VG and DB conducted the field work and performed the otolith microstructure analyzes. MP and FC provided the necessary sampling gear and materials. VG organized the database, performed the data analyzes, and wrote the manuscript. All authors participated in the edition and discussion of the manuscript making valuable contributions.
Funding
Operating costs were afforded with funds from the MPA Namuncurá-Banco Burdwood creation law (Law 26.875). This research is part of the Pampa Azul interministerial initiative promoted by Argentine Ministry for Science, Technology and Productive Innovation. This study is part of the Ph.D. by VGA who was supported by a Doctoral Fellowship awarded by the National Scientific and Technical Research Council-Argentina (CONICET-Argentina). FC was funded by CONICET-Argentina (PIP 11220150100109CO 2015-2017) and the University of Buenos Aires, Argentina (UBACYT 20020160100045BA 2017-2020).
Conflict of Interest
The authors declare that the research was conducted in the absence of any commercial or financial relationships that could be construed as a potential conflict of interest.
The handling Editor declared a shared affiliation, though no other collaboration, with the authors VG and FC, at time of review.
Acknowledgments
The authors would like to thank Luciano Padovani and Alejandro Martinez for their collaboration during sample collection and preparation and to Pablo S. Milla Carmona for his statistical advices. We extend our thanks to both reviewers for their suggestions and corrections, which greatly improved the manuscript. This is INIDEP contribution N° 2207 and Marine Protected Area Namuncurá-Burdwood Bank (Law 26,875) contribution N° 35 MODIS data was distributed by the NASA Ocean Biology Processing Group. Parts of these results were presented in the “II Workshop Latinoamericano de Otolitos.”
Footnotes
References
Acha, E. M., Mianzan, H. W., Guerrero, R. A., Favero, M., and Bava, J. (2004). Marine fronts at the continental shelves of austral South America: physical and ecological processes. J. Mar. Syst. 44, 83–105. doi: 10.1016/j.jmarsys.2003.09.005
Acha, E. M., Pájaro, M., and Sánchez, R. P. (1999). “The reproductive response of clupeoid fishes to different physical scenarios. three study cases in the Southwest Atlantic,” in Proceedings of the ICES Annual Science Conference, ICES CM 1997_K:12, Copenhagen.
Akaike, H. (1974). A new look at the statistical model identification. IEEE Trans. Autom. Control 19, 716–723. doi: 10.1109/TAC.1974.1100705
Alheit, J., and Hagen, E. (2001). “The effect of climatic variation on pelagic fish and fisheries,” in History and Climate, eds P. D. Jones, A. E. J. Ogilvie, T. D. Davies, and K. R. Briffa (Boston, MA: Springer), 247–265.
Alshuth, S. (1988). Daily growth increments on otoliths of laboratory reared sprat, Sprattus sprattus L., larvae. Meeresforsch 32, 23–29.
Amante, C., and Eakins, B. W. (2009). ETOPO1 1 Arc-Minute Global Relief Model: Procedures, Data Sources and Analysis. NOAA Technical Memorandum NESDIS NGDC-24. Silver Spring: NOAA.
Anderson, J. T. (1988). A review of size dependent survival during pre-recruit stages of fishes in relation to recruitment. J. Northwest Atlant. Fish. Sci. 8, 55–66.
Antezana, T. (1999). Hydrographic features of Magellan and Fuegian inland passages and adjacent Subantarctic waters. Sci. Mar. 63, 23–34.
Aranis, A., Meléndez, R., Pequeño, G., and Cerna, F. (2007). Sprattus fuegensis en aguas interiores de Chiloé, Chile (Osteichthyes: Clupeiformes: Clupeidae). Gayana 71, 102–113.
Bakun, A. (2006). Wasp-waist populations and marine ecosystem dynamics: navigating the “predator pit” topographies. Prog. Oceanogr. 68, 271–288. doi: 10.1016/j.pocean.2006.02.004
Balestrini, C., Manzella, G., and Lovrich, G. A. (1998). Simulación de corrientes en el Canal Beagle y Bahía Ushuaia, mediante un modelo bidimensional. Serv. Hidrografía Naval 98, 1–58. doi: 10.13140/RG.2.1.1196.272
Baumann, H., Gröhsler, T., Kornilovs, G., Makarchouk, A., Feldmann, V., and Temming, A. (2006a). Temperature-induced regional and temporal growth differences in Baltic young-of-the-year sprat Sprattus sprattus. Mar. Ecol. Prog. Ser. 317, 225–236. doi: 10.3354/meps317225
Baumann, H., Hinrichsen, H. H., Möllmann, C., Köster, F. W., Malzahn, A. M., and Temming, A. (2006b). Recruitment variability in Baltic Sea sprat (Sprattus sprattus) is tightly coupled to temperature and transport patterns affecting the larval and early juvenile stages. Can. J. Fish. Aquat. Sci. 63, 2191–2201. doi: 10.1139/f06-112
Baumann, H., Hinrichsen, H. H., Voss, R., Stepputtis, D., Grygiel, W., Clausen, L. W., et al. (2006c). Linking growth to environmental histories in central Baltic young-of-the-year sprat, Sprattus sprattus: an approach based on otolith microstructure analysis and hydrodynamic modelling. Fish. Oceanogr. 15, 465–476. doi: 10.1111/j.1365-2419.2005.00395.x
Baumann, H., Peck, M. A., Götze, H. E., and Temming, A. (2007). Starving early juvenile sprat Sprattus sprattus (L.) in western Baltic coastal waters: evidence from combined field and laboratory observations in August and September 2003. J. Fish Biol. 70, 853–866. doi: 10.1111/j.1095-8649.2007.01346.x
Baumann, H., Peck, M. A., and Herrmann, J. P. (2005). Short-term decoupling of otolith and somatic growth induced by food level changes in postlarval Baltic sprat, Sprattus sprattus. Mar. Freshw. Res. 56, 539–547. doi: 10.1071/MF04140
Baumann, H., Voss, R., Hinrichsen, H. H., Mohrholz, V., Schmidt, J. O., and Temming, A. (2008). Investigating the selective survival of summer-over spring-born sprat, Sprattus sprattus, in the Baltic Sea. Fish. Res. 91, 1–14. doi: 10.1016/j.fishres.2007.11.004
Belleggia, M., Figueroa, D. E., Irusta, G., and Bremec, C. (2014). Spatio-temporal and ontogenetic changes in the diet of the Argentine hake Merluccius hubbsi. J. Mar. Biol. Assoc. U. K. 94, 1701–1710. doi: 10.1017/S0025315414000629
Bellisio, N. B., López, R. B., and Torno, A. (1979). Peces Marinos Patagónicos. Secretaria de Estado de Intereses Maítimos, Subsecretaría de Pesca. Argentina: Buenos Aires.
Bezzi, S. I. (1984). Aspectos biológicos pesqueros de la merluza de cola del Atlántico Sudoccidental. Rev. Investig. Desarrollo Pesquero INIDEP 4, 63–80.
Brown, D. R., and Sánchez, R. P. (2010). Larval and juvenile growth of two Patagonian small pelagic fshes: Engraulis anchoita and Sprattus fuegensis. Rev. Investig. Desarrollo Pesquero INIDEP 20, 35–50.
Bruno, D. O., Victorio, M. F., Acha, E. M., and Fernández, D. A. (2018). Fish early life stages associated with giant kelp forests in sub-Antarctic coastal waters (Beagle Channel, Argentina). Polar Biol. 41, 365–375. doi: 10.1007/s00300-017-2196-y
Campana, S. E. (1990). How reliable are growth back-calculations based on otoliths? Can. J. Fish. Aquat. Sci. 47, 2219–2227. doi: 10.1139/f90-246
Campana, S. E. (1992). “Measurement and interpretation of the microstructure of fish otoliths,” in Otolith Microstructure Examination and Analysis, eds D. K. Stevenson and S. E. Campana (Ottawa: Canada Communication Group - Publishing), 59–71.
Campana, S. E., and Neilson, J. D. (1985). Microstructure of fish otoliths. Can. J. Fish. Aquat. Sci. 42, 1014–1032. doi: 10.1139/f85-127
Cerna, F., Leal, E., López, A., and Plaza, G. (2014). Age, growth and natural mortality of the Patagonian sprat Sprattus fuegensis (Jenyns, 1842) in Chiloé inland sea, southern Chile. Latin Am. J. Aquat. Res. 42, 580–587. doi: 10.3856/vol42-issue3-fulltext-15
Ciancio, J. E., Pascual, M. A., Botto, F., Frere, E., and Iribarne, O. (2008). Trophic relationships of exotic anadromous salmonids in the southern Patagonian Shelf as inferred from stable isotopes. Limnol. Oceanogr. 53, 788–798. doi: 10.4319/lo.2008.53.2.0788
Ciechomski, J. D., Cassia, M. C., and Weiss, G. (1975). Distribución de huevos, larvas y juveniles de peces en los sectores surbonaerenses, patagónico y fueguino del mar epicontinental argentino, en relación con las condiciones ambientales, en noviembre 1973-enero 1974. Ecosur 2, 219–248.
Ciechomski, J. D., and Weiss, G. (1974). Consideraciones sobre la reproducción y distribución cuantitativa de huevos y larvas de la sardina fueguina Sprattus fuegensis, en sector patagónico del Atlántico. FAO/CARPAS/6. Docu. Técn. 12, 1–11.
Contreras, J. E., Rodriguez-Valentino, C., Landaeta, M. F., Plaza, G., Castillo, M. I, and Alvarado-Niño, M. (2017). Growth and mortality of larval anchoveta Engraulis ringens, in northern Chile during winter and their relationship with coastal hydrographic conditions. Fish. Oceanogr. 26, 603–614. doi: 10.1111/fog.12219
Contreras, T., Castro, L. R., Montecinos, S., Gonzalez, H. E., Soto, S., Muñoz, M. I., et al. (2014). Environmental conditions, early life stages distributions and larval feeding of patagonian sprat Sprattus fuegensis and common sardine Strangomera bentincki in fjords and channels of the northern Chilean patagonia. Prog. Oceanogr. 129, 136–148. doi: 10.1016/j.pocean.2014.10.005
Cousseau, M. B. (1982). Revisión taxonómica y análisis de los caracteres morfométricos y merísticos de la sardina fueguina, Sprattus fuegensis (Jenyns, 1842) (Pisces, Clupeidae). Rev. Investig. Desarrollo Pesquero INIDEP 3, 77–94.
Cury, P., Bakun, A., Crawford, R. J., Jarre, A., Quinones, R. A., Shannon, L. J., et al. (2000). Small pelagics in upwelling systems: patterns of interaction and structural changes in “wasp-waist” ecosystems. ICES J. Mar. Sci. 57, 603–618. doi: 10.1006/jmsc.2000.0712
Cushing, D. H. (1990). Plankton production and year-class strength in fish populations: an update of the match/mismatch hypothesis. Adv. Mar. Biol. 26, 249–293. doi: 10.1016/S0065-2881(08)60202-3
Di Luca, J., and Zelaya, D. G. (2019). Gastropods from the Burdwood Bank (southwestern Atlantic): an overview of species diversity. Zootaxa 4544, 41–78. doi: 10.11646/zootaxa.4544.1.2
Fauchald, P., Skov, H., Skern-Mauritzen, M., Johns, D., and Tveraa, T. (2011). Wasp-waist interactions in the North Sea ecosystem. PLoS One 6:e22729. doi: 10.1371/journal.pone.0022729
Fey, D. P. (1999). Effects of preservation technique on the length of larval fish: methods of correcting estimates and their implication for studying growth rates. Arch. Fish. Mar. Res. 47, 17–29.
Fey, D. P. (2015). Size and growth rate differences of larval Baltic sprat Sprattus sprattus collected with bongo and MIK nets. J. Fish Biol. 86, 355–359. doi: 10.1111/jfb.12528
Fox, J., and Weisberg, S. (2011). An {R} Companion to Applied Regression, Second Edition. Thousand Oaks, CA: Sage.
Francis, R. I. C. C. (1990). Back-calculation of fish length: a critical review. J. Fish Biol. 36, 883–902. doi: 10.1111/j.1095-8649.1990.tb05636.x
Fraysse, C., Calcagno, J., and Pérez, A. F. (2018). Asteroidea of the southern tip of South America, including Namuncurá Marine Protected Area at Burdwood Bank and Tierra del Fuego Province, Argentina. Polar Biol. 41, 2423–2433. doi: 10.1007/s00300-018-2377-3
García Alonso, V. A., Brown, D., Martín, J., Pájaro, M., and Capitanio, F. L. (2018). Seasonal patterns of Patagonian sprat Sprattus fuegensis early life stages in an open sea Sub-Antarctic Marine Protected Area. Polar Biol. 41, 2167–2179. doi: 10.1007/s00300-018-2352-z
Glorioso, P. D., and Flather, R. A. (1995). A barotropic model of the currents off SE South America. J. Geophys. Res. Oceans 100, 13427–13440. doi: 10.1029/95JC00942
Gorelick, N., Hancher, M., Dixon, M., Ilyushchenko, S., Thau, D., and Moore, R. (2017). Google Earth Engine: planetary-scale geospatial analysis for everyone. Remote Sens. Environ. 202, 18–27. doi: 10.1016/j.rse.2017.06.031
Guerrero, R. A., Baldoni, A. G., and Benavides, H. R. (1999). Oceanographic conditions at the southern end of the Argentine continental slope. Docu. Científico INIDEP 5, 7–22.
Guerrero, R. A., and Piola, A. R. (1997). “Masas de agua en la plataforma continental,” in El Mar Argentino y Sus Recursos Pesqueros. Tomo 1. Antecedentes Históricos de las Exploraciones en el Mar Argentino y las Características Ambientales, ed. E. E. Boschi (Mar del Plata, BA: Instituto Nacional de Investigación y Desarrollo Pesquero), 107–118.
Günther, C. C., Temming, A., Baumann, H., Huwer, B., Möllmann, C., Clemmesen, C., et al. (2012). A novel length back-calculation approach accounting for ontogenetic changes in the fish length–otolith size relationship during the early life of sprat (Sprattus sprattus). Can. J. Fish. Aquat. Sci. 69, 1214–1229. doi: 10.1139/f2012-054
Halekoh, U., Højsgaard, S., and Yan, J. (2006). The R package geepack for generalized estimating equations. J. Stat. Softw. 15, 1–11. doi: 10.18637/jss.v015.i02
Hansen, J. E. (1999). Estimación de parámetros poblacionales del efectivo de sardina fueguina (Sprattus fuegensis) de la costa continental Argentina. Informe Técn. INIDEP 27, 1–18.
Hinrichsen, H. H., Voss, R., Huwer, B., and Clemmesen, C. (2010). Variability of larval Baltic sprat (Sprattus sprattus L.) otolith growth: a modeling approach combining spatially and temporally resolved biotic and abiotic environmental key variables. Fish. Oceanogr. 19, 463–479. doi: 10.1111/j.1365-2419.2010.00557.x
Hjort, J. (1926). Fluctuations in the year classes of important food fishes. ICES J. Mar. Sci. 1, 5–38. doi: 10.1093/icesjms/1.1.5
Hothorn, T., Bretz, F., and Westfall, P. (2008). Simultaneous inference in general parametric models. Biometr. J. 50, 346–363. doi: 10.1002/bimj.200810425
Houde, E. D. (1987). Fish early dynamics and recruitment variability. Am. Fish. Soc. Symp. 2, 17–29.
Houde, E. D. (1989). Comparative growth, mortality and energetics of marine fish larvae: temperature and implied latitudinal effects. Fish. Bull. 87, 471–495.
Isla, F., Bujalesky, G., and Coronato, A. T. (1999). Procesos estuarinos en el canal Beagle, Tierra del Fuego. Rev. Asoc. Geol. Argent. 54, 307–318.
Kendall, A. W., Ahlstrom, E. H., and Moser, H. G. (1984). ““Early life history stages of fishes and their characters,”,” in Ontogeny and Systematics of Fishes, eds H. G. Moser, W. J. Richards, D. M. Cohen, M. P. Fahay, A. W. Kendall, and S. L. Richardson (Lawrence, KS: Allen Press Inc), 11–22.
Köster, F. W., Hinrichsen, H. H., Schnack, D., John, M. A. S., Mackenzie, B. R., Tomkiewicz, J., et al. (2003). Recruitment of Baltic cod and sprat stocks: identification of critical life stages and incorporation of environmental variability into stock-recruitment relationships. Sci. Mar. 67, 129–154. doi: 10.3989/scimar.2003.67s1129
Laidig, T. E., Ralston, S., and Bence, J. R. (1991). Dynamics of growth in the early life history of shortbelly rockfish Sebastes jordani. Fish. Bull. 89, 611–621.
Landaeta, M. F., López, G., Suárez-Donoso, N., Bustos, C. A., and Balbontín, F. (2012). Larval fish distribution, growth and feeding in Patagonian fjords: potential effects of freshwater discharge. Environ. Biol. Fishes 93, 73–87. doi: 10.1007/s10641-011-9891-2
Landaeta, M. F., Martínez, R. A., Bustos, C. A., and Castro, L. R. (2013). Distribution of microplankton and fish larvae related to sharp clines in a Patagonian fjord. Rev. Biol. Mar. Oceanogr. 48, 401–407. doi: 10.4067/S0718-19572013000200020
Leal, E., Muñoz, C., Moyano, G., Bernal, C., and Aranis, A. (2017). A first experience of Patagonian sprat Sprattus fuegensis spawning in captivity: adult acclimation, egg and larval measurements. Rev. Biol. Mar. Oceanogr. 52, 641–645. doi: 10.4067/S0718-19572017000300021
Lebour, M. V. (1921). The larval and post-larval stages of the pilchard, sprat and herring from Plymouth district. J. Mar. Biol. Assoc. U. K. 12, 427–457. doi: 10.1017/S0025315400006299
Leggett, W. C., and Deblois, E. (1994). Recruitment in marine fishes: is it regulated by starvation and predation in the egg and larval stages? Netherlands J. Sea Res. 32, 119–134. doi: 10.1016/0077-7579(94)90036-1
Lenth, R. V. (2016). Least-squares means: the R Package lsmeans. J. Stat. Softw. 69, 1–33. doi: 10.18637/jss.v069.i01
Matano, R. P., Palma, E. D., and Combes, V. (2019). The Burdwood bank circulation. J. Geophys. Res. Oceans 124, 6904–6926. doi: 10.1029/2019JC015001
Molina-Valdivia, V., Landaeta, M. F., Castillo, M. I., Alarcón, D., and Plaza, G. (2020). Short-term variations in the early life history traits of common sardine Strangomera bentincki and anchoveta Engraulis ringens off central Chile. Fish. Res. 224:105460. doi: 10.1016/j.fishres.2019.105460
Morales-Nin, B. (2000). Review of the growth regulation processes of otolith daily increment formation. Fish. Res. 46, 53–67. doi: 10.1016/S0165-7836(00)00133-8
NASA (2019). Data from: Goddard Space Flight Center, Ocean Ecology Laboratory, Ocean Biology Processing Group. Greenbelt, MD: NASA.
Nissling, A. (2004). Effects of temperature on egg and larval survival of cod (Gadus morhua) and sprat (Sprattus sprattus) in the Baltic Sea - implications for stock development. Hydrobiologia 514, 115–123. doi: 10.1023/B:hydr.0000018212.88053.aa
Pan, W. (2001). Akaike’s information criterion in generalized estimating equations. Biometrics 57, 120–125. doi: 10.1111/j.0006-341X.2001.00120.x
Peck, M. A., Baumann, H., Bernreuther, M., Clemmesen, C., Herrmann, J. P., Haslob, H., et al. (2012). The ecophysiology of Sprattus sprattus in the Baltic and North Seas. Prog. Oceanogr. 103, 42–57. doi: 10.1016/j.pocean.2012.04.013
Peck, M. A., Clemmesen, C., Baumann, H., Herrmann, J. P., Stäcker, S., and Temming, A. (2004). The Growth-Feeding Relationship in Post-Larval Baltic sprat (Sprattus sprattus L.): Comparison of Somatic, Nucleic Acid- and Otolith-Based Growth Rates. ICES CM 2004/L:25. Available at http://www.ices.dk/products/cmdocs/2004/CM[[AQ16]]
Peck, M. A., Reglero, P., Takahashi, M., and Catalán, I. A. (2013). Life cycle ecophysiology of small pelagic fish and climate-driven changes in populations. Prog. Oceanogr. 116, 220–245. doi: 10.1016/j.pocean.2013.05.012
Petereit, C., Haslob, H., Kraus, G., and Clemmesen, C. (2008). The influence of temperature on the development of Baltic Sea sprat (Sprattus sprattus) eggs and yolk sac larvae. Mar. Biol. 154, 295–306. doi: 10.1007/s00227-008-0923-1
Pinheiro, J., Bates, D., DebRoy, S., Sarkar, D., and R Core Team (2018). nlme: Linear and Nonlinear Mixed Effects Models_. R package version 3.1-137.
Piola, A. R., and Falabella, V. (2009). “The Patagonian Sea,” in Atlas of the Patagonian Sea, Species and Spaces, eds V. Falabella, C. Campagna, and J. Croxall (Buenos Aires: Wildlife Conservation Society and Bird Life International), 55–75.
Piola, A. R., and Gordon, A. L. (1989). Intermediate waters in the southwest South Atlantic. Deep Sea Res. A Oceanogr. Res. Pap. 36, 1–16. doi: 10.1016/0198-0149(89)90015-0
Piola, A. R., Palma, E. D., Bianchi, A. A., Castro, B. M., Dottori, M., Guerrero, R. A., et al. (2018). ““Physical oceanography of the SW Atlantic shelf: a review,”,” in Plankton Ecology of the Southwestern Atlantic, eds M. Hoffmeyer, M. Sabatini, F. Brandini, D. Calliari, and N. Santinelli (Cham, ZG: Springer), 37–56.
Piola, A. R., and Rivas, A. L. (1997). “Corrientes en la plataforma continental,” in El Mar Argentino y Sus Recursos Pesqueros. Tomo 1. Antecedentes Históricos de las Exploraciones en el Mar Argentino y las Características Ambientales, ed. E. E. Boschi (Mar del Plata, BA: Instituto Nacional de Investigación y Desarrollo Pesquero), 119–132.
R Core Team (2019). R: A Language and Environment for Statistical Computing. Vienna: R Foundation for Statistical Computing.
Ramírez, F. C. (1976). Contribución al conocimiento del espectro alimenticio de la sardina fueguina (Clupeidae, Sprattus fuegensis Jenyns). Neotrópica 22, 137–141.
Reta, R., Guerrero, R., Molinari, G., Fenco, H., Allega, L., Cozzolino, E., et al. (2014). Oceanografía del Banco Burdwood: Estado Actual del Conocimiento y Perspectivas. Buenos Aires: Informe Técnico INIDEP, 15.
Robinson, D., and Hayes, A. (2020). broom: Convert Statistical Analysis Objects into Tidy Tibbles. R package version 0.5.5.
Sánchez, R. P., and Ciechomski, J. D. (1995). Spawning and nursery grounds of pelagic fish species in the sea-shelf off Argentina and adjacent areas. Sci. Mar. 59, 455–478.
Sánchez, R. P., Madirolas, A., Reta, R., Ehrlich, M. D., Álvarez Colombo, G., and Macchi, G. (1997). “The reproductive biology of the Patagonian sprat (Sprattus fuegensis): several facts and still some speculations,” in Proceedings of the ICES Annual Science Conference, ICES CM 1997_HH:22, Copenhagen.
Sánchez, R. P., Remeslo, A., Madirolas, A., and de Ciechomski, J. D. (1995). Distribution and abundance of post-larvae and juveniles of the Patagonian sprat, Sprattus fuegensis and related hydrographic conditions. Fish. Res. 23, 47–81. doi: 10.1016/0165-7836(94)00339-X
Schejter, L., and Bremec, C. S. (2019). Stony corals (Anthozoa: Scleractinia) of Burdwood Bank and neighbouring areas, SW Atlantic Ocean. Sci. Mar. 83, 247–260. doi: 10.3989/scimar.04863.10A
Schejter, L., Rimondino, C., Chiesa, I., Díaz de Astarloa, J. M., Doti, B., Elías, R., et al. (2016). Namuncurá Marine Protected Area: an oceanic hot spot of benthic biodiversity at Burdwood Bank, Argentina. Polar Biol. 39, 2373–2386. doi: 10.1007/s00300-016-1913-2
Schiavini, A. C., Goodall, R. N. P., Lescrauwaet, A. K., and Alonso, M. K. (1997). Food habits of the Peales dolphin Lagenorhynchus australis; review and new information. Rep. Int. Whal. Comm. 47, 827–834.
Scioscia, G., Raya Rey, A., Saenz Samaniego, R. A., Florentín, O., and Schiavini, A. (2014). Intra-and interannual variation in the diet of the Magellanic penguin (Spheniscus magellanicus) at Martillo Island, Beagle Channel. Polar Biol. 37, 1421–1433. doi: 10.1007/s00300-014-1532-8
Shirokova, E. N. (1978). Some biological features of Tierra del Fuego sprat. Soviet J. Mar. Biol. 4, 697–702.
Sogard, S. M. (1997). Size-selective mortality in the juvenile stage of teleost fishes: a review. Bull. Mar. Sci. 60, 1129–1157.
Sponaugle, S. (2010). Otolith microstructure reveals ecological and oceanographic processes important to ecosystem-based management. Environ. Biol. Fish. 89, 221–238. doi: 10.1007/s10641-010-9676-z
Stevenson, D. K., and Campana, S. E. (1992). Otolith Microstructure Examination and Analysis. Ottawa: Canada Communication Group - Publishing.
Voss, R., Clemmesen, C., Baumann, H., and Hinrichsen, H. H. (2006). Baltic sprat larvae: coupling food availability, larval condition and survival. Mar. Ecol. Prog. Ser. 308, 243–254. doi: 10.3354/meps308243
Voss, R., Peck, M. A., Hinrichsen, H. H., Clemmesen, C., Baumann, H., Stepputtis, D., et al. (2012). Recruitment processes in Baltic sprat–A re-evaluation of GLOBEC Germany hypotheses. Prog. Oceanogr. 107, 61–79. doi: 10.1016/j.pocean.2012.05.003
Watanabe, Y., and Kuroki, T. (1997). Asymptotic growth trajectories of larval sardine (Sardinops melanostictus) in the coastal waters off western Japan. Mar. Biol. 127, 369–378. doi: 10.1007/s002270050023
Whitehead, P. J. P. (1985). King herring: his place amongst the clupeoids. Can. J. Fish. Aquat. Sci. 42, s3–s20. doi: 10.1139/f85-258
Wickham, H., Averick, M., Bryan, J., Chang, W., McGowan, L., François, R., et al. (2019a). Welcome to the Tidyverse. J. Open Source Softw. 4:1686. doi: 10.21105/joss.01686
Wickham, H., François, R., Henry, L., and Müller, K. (2019b). dplyr: A Grammar of Data Manipulation. R package version 0.8.0.1.
Wickham, H., and Henry, L. (2018). tidyr: Easily Tidy Data with ‘spread()’ and ‘gather()’ Functions. R package version 0.8.2.
Keywords: Sprattus fuegensis, otolith microstructure, growth variability, environmental effect, Southwest Atlantic Ocean, Marine Protected Area Namuncurá-Burdwood Bank
Citation: García Alonso VA, Brown DR, Pájaro M and Capitanio FL (2020) Growing Up Down South: Spatial and Temporal Variability in Early Growth of Fuegian Sprat Sprattus fuegensis From the Southwest Atlantic Ocean. Front. Mar. Sci. 7:322. doi: 10.3389/fmars.2020.00322
Received: 14 December 2019; Accepted: 21 April 2020;
Published: 19 May 2020.
Edited by:
Alejandra Vanina Volpedo, University of Buenos Aires, ArgentinaReviewed by:
Mauricio F. Landaeta, University of Valparaíso, ChileGuido Plaza, Pontificia Universidad Católica de Valparaíso, Chile
Copyright © 2020 García Alonso, Brown, Pájaro and Capitanio. This is an open-access article distributed under the terms of the Creative Commons Attribution License (CC BY). The use, distribution or reproduction in other forums is permitted, provided the original author(s) and the copyright owner(s) are credited and that the original publication in this journal is cited, in accordance with accepted academic practice. No use, distribution or reproduction is permitted which does not comply with these terms.
*Correspondence: Virginia A. García Alonso, garciaalonso.v.a@gmail.com