- 1Cascadia Research Collective, Olympia, WA, United States
- 2Northwest ZooPath, Monroe, WA, United States
- 3Animal Health Centre, British Columbia Ministry of Agriculture, Abbotsford, BC, Canada
- 4Washington Department of Fish and Wildlife Marine Mammal Investigations, Lakewood, WA, United States
- 5Marine-Med: Marine Research, Epidemiology, and Veterinary Medicine, Bothell, WA, United States
- 6Northwest Fisheries Science Center, National Oceanic and Atmospheric Administration, Seattle, WA, United States
- 7SeaDoc Society, UC Davis Wildlife Health Centre, Eastsound, WA, United States
- 8The Whale Museum, Friday Harbor, WA, United States
- 9Vancouver Aquarium, Vancouver, BC, Canada
Primary fungal diseases in marine mammals are rare. Mucormycosis, a disease caused by fungi of the order Mucorales, has been documented in few cetaceans and pinnipeds. In 2012, the first case of mucormycosis in the Pacific Northwest was documented in a dead stranded harbor porpoise (Phocoena phocoena) in Washington state. Since then, mucormycosis has been detected in a total of 21 marine mammals; fifteen harbor porpoises, five harbor seals (Phoca vitulina), and one southern resident killer whale (Orcinus orca). Infected animals were predominately found in the inland waters of Washington and British Columbia, and one harbor seal was recovered in northern Oregon. Fungal hyphae were detected histologically in a variety of tissues, including brain, lung, spleen, pancreas, kidneys, muscle, lymph nodes, and skin. Three fungal species were identified from seven cases by PCR screening or fungal culture; Rhizomucor pusillus (four cases), Lichtheimia corymbifera (two cases), and Cunninghamella bertholletiae. Underlying conditions such as emaciation, current or recent pregnancy, multisystemic parasitism, protozoal infection, and herpesvirus were found in several affected animals. Reasons for the appearance and subsequent increase of these fungal infections in marine mammals are unknown. The emergence of this disease as a source of marine mammal mortality in the Pacific Northwest is of particular concern for endangered southern resident killer whales that spend time in this region. Current population-level stressors such as insufficient prey, high levels of contaminants, and noise pollution, could predispose them to these fatal infections.
Introduction
Mucormycosis is a disease caused by fungi of the order Mucorales and its global incidence is increasing, particularly on the Asian continent (Prakash and Chakrabarti, 2019). These fungi are found in a variety of decaying organic matter such as leaves, rotting wood and vegetation, and in animal excrement (Ribes et al., 2000; El-Herte et al., 2012; Petrikkos et al., 2012; Seyedmousavi et al., 2018; Hassan and Voigt, 2019). Modes of infection include inhalation of sporangiospores, direct inoculation into the skin, or, less frequently, ingestion (Roden et al., 2005; Guarner and Brandt, 2011; El-Herte et al., 2012; Petrikkos and Tsioutis, 2018). Despite the ubiquity of Mucorales in the environment, mucormycosis is uncommon, accounting for only 8–13% of all fungal infections in humans (Petrikkos et al., 2012). However, documented human cases have been increasing worldwide (Bitar et al., 2009; Petrikkos and Tsioutis, 2018; Prakash and Chakrabarti, 2019).
Mucoralean fungi are considered to be opportunistic pathogens, primarily causing disease in immunocompromised hosts (Ribes et al., 2000; Binder et al., 2014; Seyedmousavi et al., 2018). In susceptible individuals the disease is aggressive and spreads hematogenously, causing thrombosis and infarction (Guarner and Brandt, 2011; Ibrahim et al., 2012; Binder et al., 2014; Ibrahim and Voelz, 2017). Virulence can vary depending on the fungal agent involved (Gomes et al., 2011; Ibrahim and Voelz, 2017) but mortality rates are generally high, particularly in pulmonary or disseminated forms (Roden et al., 2005; Hassan and Voigt, 2019). Primary risk factors for mucormycosis in humans include hematological malignancies, organ or bone marrow transplants, unregulated diabetes mellitus, intravenous drug use, and iron overload (Guarner and Brandt, 2011; El-Herte et al., 2012; Lanternier et al., 2012; Petrikkos et al., 2012). In immunocompetent individuals, cutaneous mucormycosis may develop when the agent is introduced with penetrating wounds, injections, or other localized trauma (Skiada et al., 2012).
Mucormycosis in marine mammals is rare but has been documented in captive and wild stranded cetaceans and pinnipeds. Huckabone et al. (2015), reported only two cases in over 7000 marine mammal necropsies conducted in California, and a single case was documented in 444 examined harbor porpoises (Phocoena phocoena) in the Baltic Sea (Wunschmann et al., 1999). Affected captive animals include killer whales (Orcinus orca), finless porpoise (Neophocaena phocaenoides), bottlenose dolphins (Tursiops truncatus), Pacific white-sided dolphins (Neophocaena phocaenoides), a harp seal (Pagophilus groenlandicus), and a hooded seal (Cystophora cristata) (Kaplan et al., 1960; Robeck and Dalton, 2002; Naota et al., 2009; Barnett et al., 2011; Abdo et al., 2012a, b). Isolated cases in wild stranded marine mammals have been described in a Southern right whale (Eubalaena australis) (Best and McCully, 1979), a bottlenose dolphin (Eubalaena australis) (Isidoro-Ayza et al., 2014), an Atlantic spotted dolphin (Stenella frontalis) (Cerezo et al., 2018), a harbor porpoise (Wunschmann et al., 1999), a harbor seal (Phoca vitulina) and a California sea lion (Zalophus californianus) (Huckabone et al., 2015).
In the Pacific Northwest, which includes Washington and Oregon states in the United States and British Columbia, Canada, fatal fungal diseases are infrequent. Mycotic infections in Dall’s porpoises (Phocoenoides dalli), harbor porpoises, and harbor seals in this region have chiefly been attributed to an outbreak of Cryptococcus gattii that began in humans and animals in southern British Columbia in 1999 and subsequently spread to Washington state (Stephen et al., 2002; Datta et al., 2009; Norman et al., 2011; Huggins et al., 2015; Rosenburg et al., 2016). In 2012, the first case of mucormycosis was documented in a stranded harbor porpoise in Washington. Since then, the number of cases and species involved have increased, and the disease has been recorded throughout the Pacific Northwest. We provide details of the emergence, gross and histologic lesions, and culture and molecular characterization of the disease. Implications for local marine mammals, specifically the endangered southern resident killer whale population, are discussed.
Materials and Methods
Marine mammals included in this study were documented during routine stranding response activities authorized by the Department of Fisheries and Oceans (Canada) and the National Oceanic and Atmospheric Administration Marine Mammal Health and Stranding Response Program (United States). Systematic gross necropsies were performed according to established protocols (Geraci and Lounsbury, 2005; Pugliares et al., 2007; Raverty et al., 2018). Nutritional condition was determined by a combination of morphometric measurements, visual assessment of the carcass and microscopic evaluation of blubber and visceral adipose stores (Raverty et al., 2014). Emaciated animals feature loss of nuchal fat (peanut head) and bilaterally symmetric atrophy of the epaxial musculature with more prominent ribs and vertebral processes; whereas, more robust individuals present with a rounded contour of the torso and fat oozes on incision of the blubber. Representative samples of all available organs and lymph nodes, including all gross lesions were collected in 10% neutral buffered formalin for routine histology, conducted at Northwest ZooPath in Monroe, WA and the Animal Health Center (AHC) in Abbotsford, British Columbia. An additional set of tissues was frozen from most cases for ancillary studies.
For histological evaluation, tissues were embedded in paraffin, sectioned at 3–5 μm thickness, mounted on frosted glass slides and stained with hematoxylin and eosin (HE). For select cases, sections were also stained with Gomori’s methenamine silver technique (GMS).
Samples of targeted tissues from 14 infected animals were analyzed via polymerase chain reaction (PCR) and sequencing; these included samples stored in RNAlater, frozen in Whirl-Pak bags, or paraffin blocks. PCR analyses were performed primarily at the Northwest Fisheries Science Center (Seattle, WA) and the British Columbia Centre for Disease Control (Vancouver, BC). Some duplicate samples were screened at the Washington Animal Disease Diagnostic Laboratory (WADDL, Pullman, WA). Tissue subsamples were minced to ∼ 1 mm and homogenized with a handheld rotor-stator (Tissue Tearor, Biospec) in 2 ml of lysis buffer (400 ml sodium chloride, 750 mM sucrose, 20 mM ethylenediaminetetraacetic acid, 50 mM Tris-hydrochloride, pH 9). Homogenates were sequentially digested with 1 mg ml–1 lysozyme at 37°C, and 100 μg ml–1 proteinase K with 1% sodium dodecylsulfate at 55°C. After digestion, homogenates were subjected to three freeze-thaw cycles with liquid nitrogen, then phenol:chloroform extraction (Green and Sambrook, 2017). DNA was recovered from tissue samples in paraffin blocks using a protocol adapted from Bialek et al. (2002, 2005), and Pikor et al. (2011).
End-point PCR for fungal species employed multiple primer sets targeting internal transcribed regions of ribosomal RNA genes (White et al., 1990). Amplicons were cleaned by spin columns (Qiagen) and analyzed by Sanger sequencing (Molecular Cloning Labs). Taxonomic identifications were made by comparison to NCBI’s nucleotide database using their Basic Local Alignment Search Tool® and to the ISHAM Barcoding Database for curated sequences. Amplicon sequences were submitted to NCBI GenBank (SUB7506891, SUB7518690; 06/30/20 release date).
Fungal cultures were attempted on tissues from seven cases, six at AHC (includes Canadian and Washington cases), and one Washington case at Phoenix Labs in Mukilteo, WA. Growth from the latter case was forwarded to the Fungus Testing Laboratory at the University of Texas Health Science Center at San Antonio (San Antonio, Texas, United States), for identification.
Results
Stranding Demographics
Between 2012 and 2019, 1,801 necropsies were conducted on stranded harbor porpoises, harbor seals, and killer whales in the Pacific Northwest (Table 1). A total of 21 cases of mucormycosis were documented; 13 in Washington state, 7 in British Columbia, and 1 in Oregon (Figure 1 and Table 1). From 2012–2015, cases only occurred in Washington. Harbor porpoises were the most frequently affected species and represent 71% (n = 15) of the total cases. The annual number of infections in the region peaked in 2016 (n = 6), the same year that mucormycosis was detected in other marine mammal species (harbor seal and killer whale) and locations (British Columbia). The single Oregon case, a harbor seal, occurred in 2017.
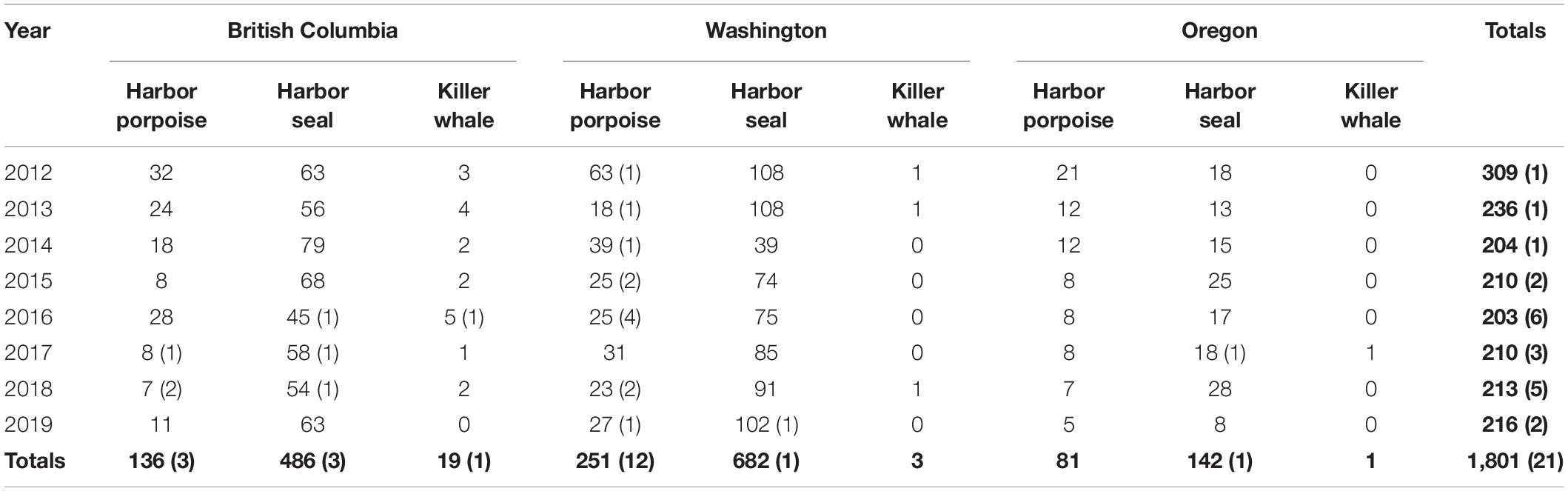
Table 1. Necropsies conducted and mucormycosis cases (in parentheses) in the Pacific Northwest, 2012–2019, by year and subarea.
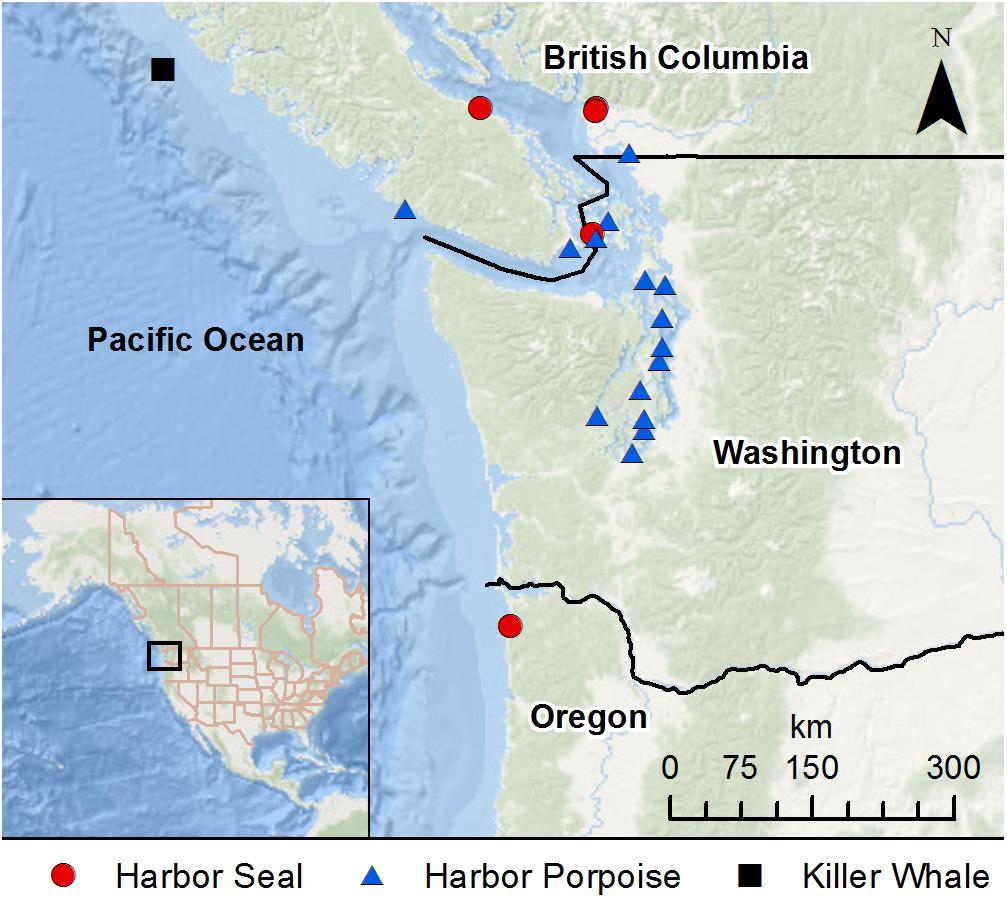
Figure 1. Stranding locations of harbor porpoises, harbor seals, and killer whale with mucormycosis infection.
Infected animals included both sexes and all age classes, although there were some differences in age class distribution between harbor porpoises and harbor seals (Table 2). Among harbor porpoises, mucormycosis was only seen in adults (n = 10) and subadults (n = 5). Harbor seals that presented with the disease were primarily pups (n = 3), and one each of the subadult and adult age classes. The killer whale was a 20-year-old adult male.
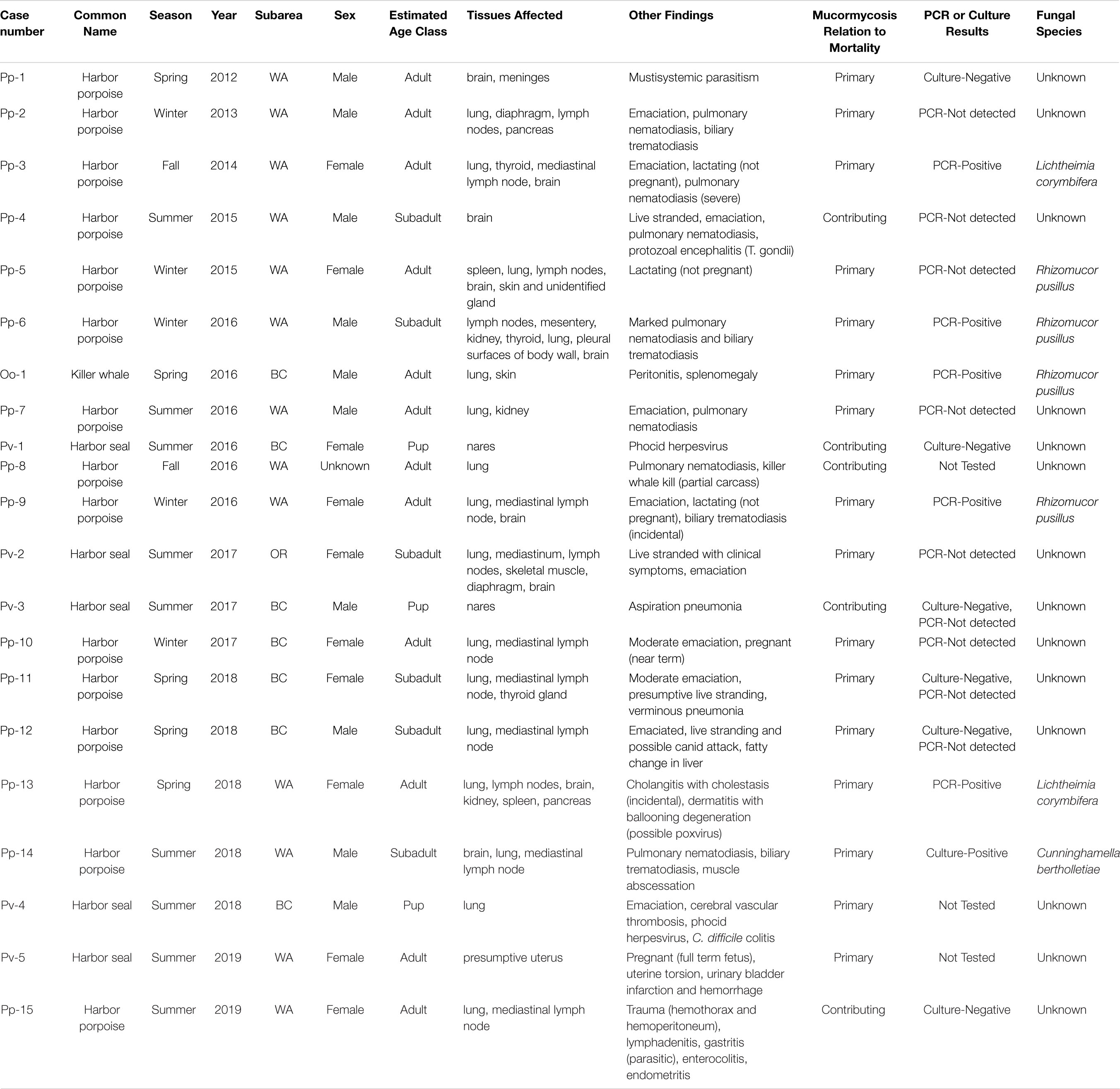
Table 2. Details of mucormycosis cases found in the Pacific Northwest, 2012–2019, in order of occurrence.
Mucormycosis was diagnosed in marine mammals in all seasons, although more cases occurred during the summer months (June–August) than other times of the year (Table 2). This was driven by harbor seal cases, all five of which occurred during the summer. The highest number (n = 5) of harbor porpoise cases were documented in the winter months (December-February), followed by spring (n = 4), summer (n = 4) and fall (n = 2). The killer whale died in the spring.
Gross and Histologic Findings
On gross examination, the lesions caused by mucormycosis appeared similar to those caused by other types of mycotic infections. In general, gross lesions were pale (cream or yellowish) raised firm masses of varying sizes (Figure 2). Gross lesions were most commonly noted in the lungs and associated lymph nodes, but in cases of disseminated mucormycosis, fungal lesions were seen in other organs, including brain, kidney, spleen, pancreas, uterus, diaphragm, skeletal muscle and skin (Table 2). In two harbor seal pups, the fungal infections were localized to the nasal cavity with occasional aspiration of fungal elements, deep into bronchoalveolar spaces.
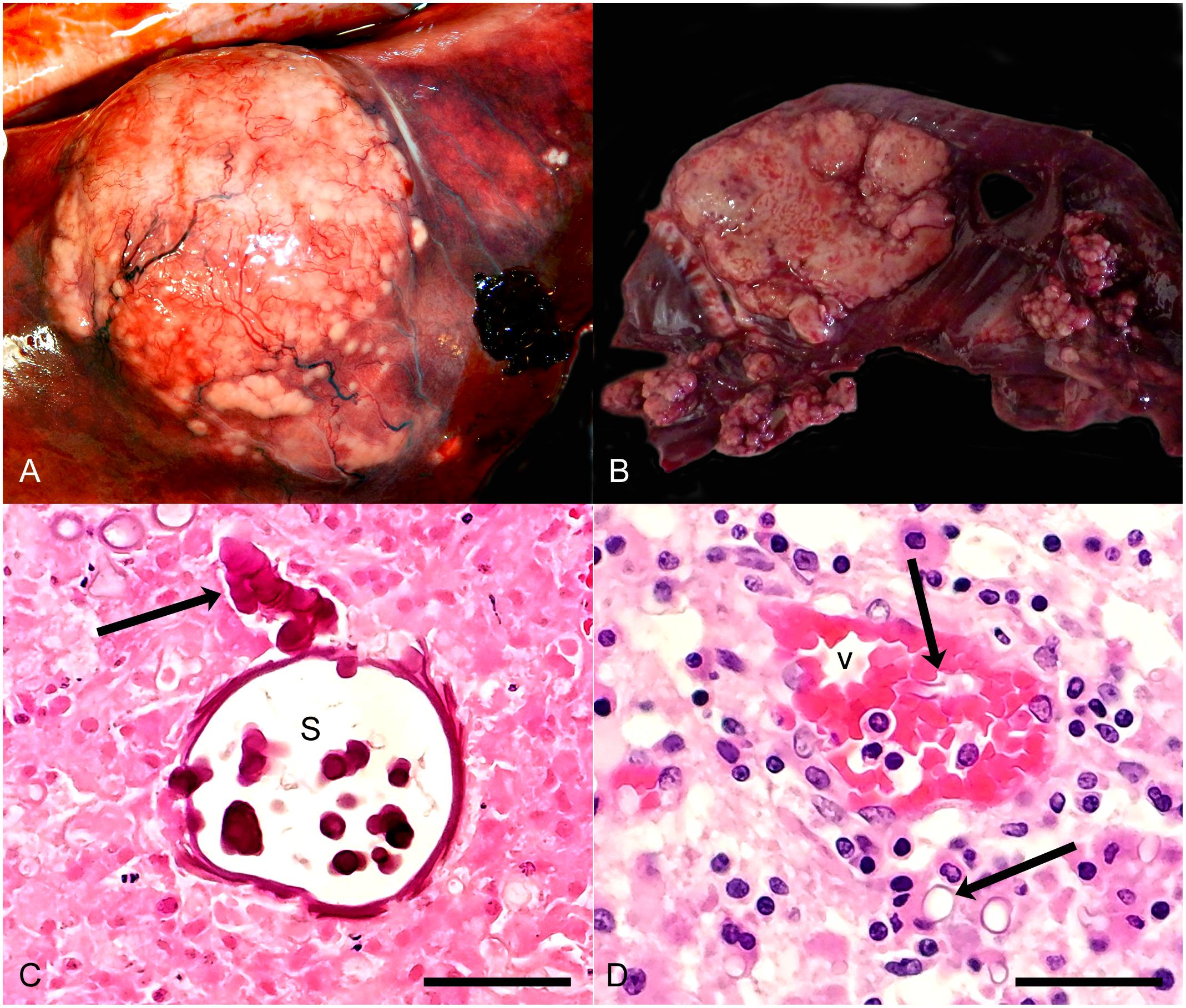
Figure 2. (A) Harbor porpoise, pulmonary abscess. Note large bulging white nodular mass in lung. (B) Harbor seal, Pulmonary abscesses. Note variably sized irregular nodular white masses. (C) Harbor porpoise, lung. Note undulating, broad, septate intensely eosinophilic fungal element (arrow), terminating in a large spherical sporangium (s). Pulmonary tissue around the fungi is necrotic. HE, bar = 75 μ. (D) Harbor porpoise, brain. Note small blood vessel (v) with luminal and perivascular fungal elements (arrows). The neuropil around the vessel is infiltrated by low numbers of plasma cells, lymphocytes and histiocytes. HE, bar = 75 μ.
Histologic lesion morphology was variable depending on the chronicity of the lesion (Figure 2). Grossly visible lesions were typically abscesses or granulomas and comprised of large numbers of neutrophils and histiocytes with fewer lymphocytes and plasma cells, frequently oriented around accumulations of necrotic cellular or caseous debris. Undulating somewhat flattened large eosinophilic septate and branching fungal elements were occasionally detected in the necrotic foci. In the lung these lesions were commonly associated with peripheral fibrin deposition and edema in the alveoli and fibrinoid necrosis of vessel walls and fibrinocellular thrombi. Similar lesions were seen in other viscera of some of the animals. Acute lesions in various tissues, including the brain, generally were associated with necrosis of small vessel walls, thrombosis, and fungal elements within the thrombi, vessel walls and adjacent tissue. Tissue surrounding the thrombi was variably necrotic and infiltrated by low numbers of neutrophils.
Additional Findings and Causes of Mortality
Mucormycosis was determined to be the primary cause of mortality in 76% (n = 16) of the documented cases (Table 2). In these animals, the proximate cause of death was due to massive pulmonary infections, presumptive disruption of the perfusion-ventilation coefficient, secondary microbial involvement, fungemia with embolism to the brain, toxin production and other factors. In the remaining cases (n = 5), mucormycosis was identified as a contributing factor to morbidity and mortality; with primary cause attributed to traumatic injuries and infectious disease.
In addition to the mycotic infections, other conditions were documented in most individuals (Table 2). Suboptimal nutritional status was noted in nine cases. Parasitism was a common finding (n = 11), primarily involving the liver and the lungs. Three adult female harbor porpoises were lactating but not pregnant, and three animals (two harbor porpoises and one harbor seal) were pregnant, with no evidence of fungal infection in the fetal tissues. Phocid herpesvirus was isolated in two harbor seal pups in BC, and one harbor porpoise in Washington presented with protozoal encephalitis caused by Toxoplasma gondii. One porpoise (Pp-5), an adult female in good nutritional condition, did not appear to have any underlying conditions other than active lactation, but represented one of the most severe disseminated mucormycosis infections in our study.
Molecular and Culture Results
The fungal species involved could only be determined in seven cases (Table 2), six of them by PCR sequencing and the other by culture. Three cases of Rhizomucor pusillus and two cases of Lichtheimia corymbifera were detected by PCR sequencing of frozen harbor porpoise tissue (≥ 99% identity in 580 nucleotides and 100% identity in 455 nucleotides, respectively; GenBank Accession numbers MT537730, MT537731, MT537732, MT548024, and MT548025). One case of Rhizomucor pusillus in killer whale was detected by PCR sequencing of histology sections of lung (> 96% identity in 202 nucleotides). Scant growth from culture was obtained from the tissues from one harbor porpoise; the species Cunninghamella bertholletiae was identified by combined phenotypic characterization of wide (10–20 μm), aseptate, or partially septate hyphae with erectile sporangiophores, and DNA sequencing of the following targets: ITS, D1/D2.
Discussion
The recent emergence of mucormycosis as a source of mortality in marine mammals in the Pacific Northwest is unusual and unexplained. There have been no apparent increases in mortality that would suggest that populations of harbor porpoises and harbor seals are less healthy, and therefore more susceptible to this fungal infection, than observed in the past. As non-migratory animals, harbor porpoises and harbor seals are considered sentinel species for the local ecosystem and causes of mortality have been monitored for decades. The distinctive histologic appearance of mucormycosis likely would have been identified if present prior to 2012. Stranded harbor porpoises are of particular interest and are prioritized for collection and necropsy. Examinations of stranded harbor seals are a slightly lower priority due to high numbers of animals and limited funding, so the disease prevalence in harbor seals could be underrepresented in this report.
A variety of conditions in addition to the mucormycosis were documented, but none were consistently present among the infected animals. Some were obviously compromised by viral, parasitic, or bacterial agents and many showed signs of nutritional stress. Risk factors for mucormycosis in marine mammals are poorly understood, and it is possible that an emergent underlying component or unusual combination of factors exists that has made these animals more susceptible to this disease than their cohorts. Geraghty et al. (2011) assessed numerous health and environmental parameters for infection in Tasmanian platypuses (Ornithorhynchus anatinus) and found that higher altitude, low trypanosome numbers and reduced tail fat content appeared to be associated with ulcerative mucormycosis. Chemical contaminants, which are known immunosuppressants in marine mammals, were suggested as a possible contributor to mucormycosis in a harbor porpoise in the Baltic Sea (Wunschmann et al., 1999). Free iron plays a key role in pathogenesis of Mucorales in humans (Ibrahim et al., 2012; Kousser et al., 2019), and zinc, copper and manganese are important elements for the growth of many fungal species (Spellberg et al., 2005; Kousser et al., 2019). Serum is difficult to obtain from stranded animals; however, all of these trace elements are stored in marine mammal livers and analysis of liver tissue could possibly be used as a proxy for serum values. More research is needed to evaluate the possible roles and interplay of conditions such as chemical contaminants, metal/trace element accumulations, infectious disease, parasitism, pregnancy and malnutrition in the susceptibility of the individual hosts. Efforts are currently underway to identify potential demographic, environmental, and other risk factors that may be associated with mucormycosis in harbor porpoises in Washington State.
Lung was the most commonly affected tissue in this study, and inhalation was the suspected route of fungal infiltration in all cases except the killer whale, in which case fungal spores were introduced via percutaneous attachment of a satellite tag. Spores may have come from the environmental air, on the surface of the water, or both. Surveys conducted in the region by Raverty et al. (2017) found Mucor sp. in the sea surface microlayer (SML), and hypothesized that the breathing patterns (several quick breaths followed by a deep breath for diving) and anatomy (direct link between the blowhole and lungs) of cetaceans may allow small amounts of the SML to be aspirated deep into the lung tissue. Harbor seals also may aspirate the SML but are more frequently exposed to mucoralean fungi while hauled out on land. Ingestion of infectious spores seems a less likely route of infection in the study population, as gut lesions associated with mucormycosis were not seen.
Fungal displacement caused by environmental disturbances such as construction activities, flooding, earthquakes, tornadoes and other natural disasters may increase concentrations of spores or distribute the fungi to new areas through wind or runoff of the fungi into bodies of water (Miller and Lodge, 2007; Benedict and Park, 2014; Engelthaler and Casadevall, 2019). Clusters of mucormycosis cases in humans have been documented in the aftermath of environmental disasters, when infections are the result of inhalation or injury-related inoculation (Benedict and Park, 2014; Walsh et al., 2019). The majority of marine mammal cases occurred in the inland waters of Washington and British Columbia. Among harbor porpoises in Washington, mucormycosis infections were only documented in inland waters, despite relatively equal numbers of necropsies conducted between the inland and outer coast regions of the state (n = 125 and n = 126, respectively). Screening of water samples from inland marine waters of Washington (in 2011) and nearshore to the outer coast from norther California to British Columbia (in 2016) detected mucoralean fungal DNA in 1.8% of the inland water samples but no evidence of these fungal general in outer coast samples (L. Rhodes, pers. comm.). The inland waters have highly populated coastlines with large cities that have undergone tremendous growth in recent years. Construction projects are numerous (Puget Sound Business Journal, 2020) and construction-associated soil disruption could be contributing to increased dissemination of these pathogenic fungi.
The seasonality of human mucormycosis in the Middle East (El-Herte et al., 2012) shows most cases occur in the late summer, fall and early winter. When evaluating seasonality in marine mammals, seasonal stranding trends for each species must be considered. In harbor porpoises, there was little difference in the number of mucormycosis cases that occurred in the spring, summer and winter months, but winter numbers were slightly higher. However, harbor porpoise strandings are substantially lower in the winter than in other seasons (Norman et al., 2004; Huggins et al., 2015), suggesting a higher proportion of infections during the winter months, when heavy rain and flooding is common, which could be a mode of fungal dispersal into the marine environment. All of the infected harbor seal cases occurred in summer, coincident with pupping season and highest seasonal harbor seal strandings (Warlick et al., 2018). Given the low number of harbor seals with mucormycosis in this study, we could not determine seasonality.
The three species of Mucorales identified have also been documented in humans and terrestrial animals. L. corymbifera is one of the most common causes of mucormycosis and is frequently seen in trauma-related infections (Walsh et al., 2019), while infections caused by R. pusillus and C. bertholletiae are less frequent but have higher mortality rates (Ribes et al., 2000; Gomes et al., 2011). R. pusillus is a common cause of mucormycosis in mammals and has been linked to mycotic abortion and mastitis in cattle (Ribes et al., 2000; Gomes et al., 2011). This agent was responsible for fatal pneumonia in a captive harp seal (Kaplan et al., 1960). Disseminated C. bertholletiae infection was documented in a stranded bottlenose dolphin in Spain (Isidoro-Ayza et al., 2014) and a captive killer whale in Japan (Abdo et al., 2012a). Although human infections caused by C. bertholletiae are rare, they are almost always fatal; its virulence is attributed to very fast germination (Ibrahim and Voelz, 2017). Mucormycosis is not a reportable disease in the United States or Canada, and a corresponding emergence in human or terrestrial animals has not been determined.
To our knowledge, this increase in cases in populations under surveillance is the first documented multi-year and multi-species occurrence of mucormycosis in free-ranging marine mammals. The prevalence in this study (1.16%) is much higher than was observed in similar studies in California (<0.025%, Huckabone et al., 2015) and the Baltic Sea (0.23%, Wunschmann et al., 1999). Harbor porpoises in inland waters appear to be disproportionately vulnerable. Although the population is not threatened or endangered, they have recently recolonized the southern portion of Washington’s inland waters after virtually disappearing several decades ago (Evenson et al., 2016) and a larger-scale epizootic could negatively affect this portion of the inland water stock. This disease is of particular concern for endangered southern resident killer whales that seasonally occur these waters (Olsen et al., 2018). This small population has been in a declining trend for the past several years, with few carcasses recovered for necropsy and limited information available on causes of mortality (Raverty et al. in prep). It is at risk to nutritional, immunological, and anthropogenic stressors (NMFS, 2008) that could increase their susceptibility to fatal infection by Mucorales. Additional research to understand what causes increased fungal dispersion and what risk factors are most associated with infection is needed to evaluate and potentially mitigate the risks to these and other marine mammal species in the region.
Data Availability Statement
All datasets generated for this study are included in the article/supplementary material.
Ethics Statement
The marine mammals included in this study were documented during routine stranding response activities conducted under the authorization of the Department of Fisheries and Oceans (Canada) and the National Oceanic and Atmospheric Administration Marine Mammal Health and Stranding Response Program (United States). The authors adhered to all guidelines for animal use provided by the governing agencies. No living animals were used for this study; samples were collected during necropsy of deceased animals only.
Author Contributions
JH conducted the necropsies on stranded marine mammals in Washington, collected the data, and prepared the manuscript. MG provided histological analyses for cases in Washington and Oregon. SR necropsied harbor porpoises and the killer whale in Canada and analyzed histology samples from marine mammals in Canada and a few cases from Washington. DL, SN, JG, and JO conducted necropsies on stranded marine mammals from Washington. LR conducted PCR sequencing to identify fungal species. MH conducted necropsies on harbor seals in Canada. MBH was involved in tagging the killer whale. All authors contributed to manuscript revisions and read and approved the final submission.
Funding
This project was supported in part by grants from the John H. Prescott Marine Mammal Rescue Assistance Grant Program and the SeaDoc Society, a program of the Karen C. Drayer Wildlife Health Center, School of Veterinary Medicine, University of California, Davis, and by support from the NMFS West Coast Region’s Office of Protected Resources.
Conflict of Interest
MG founded the company Northwest ZooPath.
The remaining authors declare that the research was conducted in the absence of any commercial or financial relationships that could be construed as a potential conflict of interest.
Acknowledgments
The authors would like to thank the local stranding networks for assistance with carcass recovery and sample collection; particularly Erin Keene (Cascadia Research Collective), Susan Berta and Sandy Dubpernell (Central Puget Sound Marine Mammal Stranding Network), Debbie Duffield (Portland State University), Keith Chandler (Seaside Aquarium), Jim Rice (Oregon State University), the volunteers of the San Juan County Marine Mammal Stranding Network, and the staff and volunteers of the Vancouver Aquarium’s Marine Mammal Rescue Centre. Undergraduate interns Augustus Pendleton, Alexa Dietz, and Shaun Christean (NOAA Office of Education) assisted in processing tissues and PCR sequencing. The authors also thank David Anderson of Cascadia Research Collective for map generation, and Cathy Minogue and Christie Buie of Northwest ZooPath for data retrieval and plate illustration, respectively.
References
Abdo, W., Kakizoe, Y., Ryono, M., Dover, S. R., Fukusi, H., Okuda, H., et al. (2012a). Pulmonary zygomycosis with Cunninghamella bertholletiae in a killer whale (Orcinus orca). J. Comp. Pathol. 147, 94–99. doi: 10.1016/j.jcpa.2011.08.012
Abdo, W., Kawachi, T., Sakai, H., Fukusi, H., Kano, R., Shibahara, T., et al. (2012b). Disseminated mycosis in a killer whale (Orcinus orca). J. Vet. Diagn. Invest. 24, 211–218. doi: 10.1177/1040638711416969
Barnett, J. E. F., Davison, N. J., Thornton, S. M., Riley, P., Cooper, T., and Wessels, M. E. (2011). Systematic mucormycosis in a hooded seal (Cystophora cristata). J. Zoo Wild. Med. 42, 338–341.
Benedict, K., and Park, B. J. (2014). Invasive fungal infections after natural disasters. Emerg. Infect. Dis. 20, 349–355. doi: 10.3201/eid2003.131230
Best, P. B., and McCully, R. M. (1979). Zygomycosis (phycomycosis) in a right whale (Eubalaena australis). J. Comp. Pathol. 89, 341–348. doi: 10.1016/0021-9975(79)90023-9
Bialek, R., Feucht, A., Aepinus, C., Just-Nübling, G., Robertson, V. J., Knobloch, J., et al. (2002). Evaluation of two nested PCR assays for detection of Histoplama capsulatum DNA in human tissue. J. Clin. Microbiol. 40, 1644. doi: 10.1128/jcm.40.5.1644-1647.2002
Bialek, R., Konrad, F., Kern, J., Aepinus, C., Cecenas, L., Gonzalez, G. M., et al. (2005). PCR based identification and discrimination of agents of mucormycosis and aspergillosis in paraffin wax embedded tissue. J. Clin. Pathol. 58:1180. doi: 10.1136/jcp.2004.024703
Binder, U., Maurer, E., and Lass-Florl, C. (2014). Mucormycosis-from the pathogens to the disease. Clin. Microbiol. Infect. 20, 60–66. doi: 10.1111/1469-0691.12566
Bitar, D., Cauteren, D. V., Lanternier, F., Dannaoui, E., Che, D., Dromer, F., et al. (2009). Increasing incidence of zygomycosis (mucormycosis), France, 1997-2006. Emerg. Infect. Dis. 15, 1395–1401. doi: 10.3201/eid1509.090334
Cerezo, A., Quesada-Canales, O., Sierra, E., Diaz-Delgado, J., Fernandez, A., Henningson, J., et al. (2018). Pyogranulomatous obliterative laryngotracheitis by Rhizopus arrhizus (syn. R. oryzae) in a free-ranging atlantic spotted dolphin Stenella frontalis. Dis. Aquat. Org. 130, 153–158. doi: 10.3354/dao03268
Datta, K., Bartlett, K. H., Baer, R., Byrnes, E., Galanis, E., Heitman, J., et al. (2009). Spread of Cryptococcus gattii into Pacific Northwest region of the United States. Emerg. Infect. Dis. 15, 1185–1191. doi: 10.3201/eid1508.081384
El-Herte, R. I., Baban, T. A., and Kanj, S. S. (2012). Mucormycosis: a review on environmental fungal spores and seasonal variation of human disease. Adv. Infect. Dis. 2, 76–81. doi: 10.4236/aid.2012.23012
Engelthaler, D. M., and Casadevall, A. (2019). On the emergence of Cryptococcus gattii in the Pacific Northwest: ballast tanks, tsunamis, and black swans. mBio 10:e02193-19.
Evenson, J. R., Anderson, D., Murphie, B. L., Cyra, T. A., and Calambokidis, J. (2016). Disappearance and Return of Harbor Porpoise to Puget Sound: 20 Year Pattern Revealed From Winter Aerial Surveys. Technical Report. Olympia, WA: Washington Department of Fish and Wildlife.
Geraci, J., and Lounsbury, V. (2005). Marine Mammals Ashore: A Field Guide for Strandings, 2nd Edn. Baltimore: National Aquarium, 371.
Geraghty, D. P., Griffiths, J., Stewart, N., Roberson, I. K., and Gust, N. (2011). Hematologic, plasma biochemical, and other indicators of the health of Tasmanian platypuses (Ornithorhynchus anatinus): predictors of mucormycosis. J. Wild. Dis. 47, 483–493. doi: 10.7589/0090-3558-47.3.483
Gomes, M. Z. R., Lewis, R. E., and Kontoyiannis, D. P. (2011). Mucormycosis caused by unusual mucormycetes, non-Rhizopus, -Mucor, and -Lichtheimia species. Clin. Microbiol. Rev. 33, 3341–3344. doi: 10.1128/CMR.00056-10
Green, M. R., and Sambrook, J. (2017). Isolation of high-molecular-weight DNA using organic solvents. Cold Spring Harb. Protoc. 2017:db.rot093450. doi: 10.1101/pdb.prot093450
Guarner, J., and Brandt, M. E. (2011). Histopathologic diagnosis of fungal infections in the 21st Century. Clin. Microbiol. Rev. 24, 247–280. doi: 10.1128/CMR.00053-10
Hassan, M. I. A., and Voigt, K. (2019). Pathogenicity patterns of mucormycosis: epidemiology, interaction with immune cells and virulence factors. Med. Mycol. 57, S245–S256. doi: 10.1093/mmy/myz011
Huckabone, S., Gulland, F. M. D., Johson, S. M., Colegrove, K. M., Dodd, E. M., Pappagianis, D., et al. (2015). Coccidioidomycocosis and other systemic mycoses of marine mammals stranding along the central California, USA coast: 1998-2012. J. Wildl. Dis. 51, 295–308. doi: 10.7589/2014-06-143
Huggins, J. L., Raverty, S. A., Norman, S. A., Calambokidis, J., Gaydos, J. K., Duffield, D. A., et al. (2015). Increased harbor porpoise mortality in the Pacific Northwest, USA: understanding when higher levels may be normal. Dis. Aquat. Org. 115, 93–102. doi: 10.3354/dao02887
Ibrahim, A. S., Spellberg, B., Walsh, T. J., and Kontoyiannis, D. P. (2012). Pathogenesis of mucormycosis. Clin. Infect. Dis. 54, S16–S22. doi: 10.1093/cid/cir865
Ibrahim, A. S., and Voelz, K. (2017). The mocormycete-host interface. Curr. Opin. Microbiol. 40, 40–45. doi: 10.1016/j.mib.2017.10.010
Isidoro-Ayza, M., Perez, L., Cabanes, J., Castella, G., Andres, M., Vidal, E., et al. (2014). Central nervous system mucormycosis caused by Cunninghamella Bertholletiae in a Bottlenose Dolphin (Tursiops truncatus). J. Wild. Dis. 50, 634–638. doi: 10.7589/2013-10-284
Kaplan, W., Goss, L. J., Jello, L., and Ivens, M. S. (1960). Pulmonary mucormycosis in a harp seal caused by Mucor pusillus. Micopahologia 12, 101–110. doi: 10.1007/bf02051389
Kousser, C., Clark, C., Sherrington, S., Voelz, K., and Hall, R. A. (2019). Pseudomonas aeruginosa inhibits Rhizopus microsporus germination through sequestration of free environmental iron. Sci. Rep. 9:5714. doi: 10.1038/s41598-019-42175-0
Lanternier, F., Dannaoui, E., Morizot, G., Elie, C., Garcia-Hermoso, D., Huerre, M., et al. (2012). A global analysis if mucormycosis in France: the retroygo study (2005-2007). Clin. Infect. Dis. 54, S35–S43. doi: 10.1093/cid/cir880
Miller, R. M., and Lodge, D. J. (2007). “Fungal responses to disturbance: agriculture and forestry,” in Environmental and Microbial Relationships. The Mycota, Vol. 4, eds C. Kubicek and I. Druzhinina (Berlin: Springer), 47–68. doi: 10.1007/978-3-540-71840-6_4
Naota, M., Shimada, A., Morita, T., Kimura, K., Ochiai, K., and Sano, A. (2009). Granulomatous pericarditis associated with systemic mucormycosis in a finless porpoise (Neophocaena phocaenoides). J. Comp. Pathol. 140, 64–66. doi: 10.1016/j.jcpa.2008.09.006
NMFS (2008). Recovery Plan for Southern Resident Killer Whales (Orcinus orca). Seattle, WA: National Marine Fisheries Service.
Norman, S. A., Bowlby, E., Brancato, M. S., Calmbokidis, J., Duffield, D., Gearin, P., et al. (2004). Cetacean strandings in Oregon and Washington between 1930 and 2002. J. Cet. Res. Man. 6, 87–99.
Norman, S. A., Raverty, S., Zabek, E., Etheridge, S., Ford, J. K. B., Hoang, L. M. N., et al. (2011). Maternal–fetal transmission of Cryptococcus gattii in harbor porpoise. Emerg. Infect. Dis. 17, 304–305. doi: 10.3201/eid1702.101232
Olsen, J., Wood, J., Osborne, R. W., Barrett-Lennard, L., and Larson, S. (2018). Sightings of southern resident killer whales in the Salish Sea 1976-2014: the importance of a long-term opportunistic dataset. Endang Species R 37, 105–118. doi: 10.3354/esr00918
Petrikkos, G., Skiada, A., Lortholary, O., Roilides, E., Walsh, T. J., and Kontoyiannis, D. P. (2012). Epidemiology and clinical manifestations of mucormycosis. Clin. Infect. Dis. 54, S23–S34. doi: 10.1093/cid/cir866
Petrikkos, G., and Tsioutis, C. (2018). Recent advances in the pathogenesis of mucormycoses. Clin. Ther. 40, 894–902. doi: 10.1016/j.clinthera.2018.03.009
Pikor, L. A., Enfield, K. S. S., Cameron, H., and Lam, W. L. (2011). DNA extraction from paraffin embedded material for genetic and epigenetic analyses. J. Vis. Exp. 26:2763. doi: 10.3791/2763
Prakash, H., and Chakrabarti, A. (2019). Global epidemiology of mucormycosis. J. Fungi 5:26. doi: 10.3390/jof5010026
Puget Sound Business Journal (2020). Crane Watch: Search for Seattle-Area Construction Projects, Details, Costs, Timelines, Developers, Links to Stories and More. Available online at: https://www.bizjournals.com/seattle/feature/crane-watch (accessed February 6, 2020).
Pugliares, K., Bogomolni, A., Touhey, K., Herzig, S., Harry, C., and Moore, M. (2007). Marine Mammal Necropsy: An Introductory Guide for Stranding Responders and Field Biologists: Technical. Report WHOI-2007-06. Woods Hole, MA: Woods Hole Oceanographic Institution, doi: 10.1575/1912/1823
Raverty, S., Duignan, P., Morell, M., and Jepson, P. (2018). “Gross necropsy and specimen collection,” in CRC Handbook of Marine Mammal Medicine, 3rd Edn, eds F. M. D. Gulland, L. A. Dierauf, and K. L. Whitman (Boca Raton, FL: CRC Press), 249–266.
Raverty, S., Gaydos, J., and St Leger, J. (2014). Killer Whale Necropsy and Disease Testing Protocol. 82. Available online at: https://www.seadocsociety.org/blog/killer-whale-necropsy-protocol-2014 (accessed May 31, 2020).
Raverty, S. A., Rhodes, L. D., Zabek, E., Eshghi, A. Z., Cameron, C. E., Hanson, M. B., et al. (2017). Respiratory microbiome of endangered southern resident killer whales and microbiota of surrounding sea surface microlayer in the Eastern North Pacific. Sci. Rep. 7:394. doi: 10.1038/s41598-017-00457-5
Ribes, J. A., Vanover-Sams, C., and Baker, D. J. (2000). Zygomycetes in human disease. Clin. Microbiol. Rev. 13, 236–301. doi: 10.1128/cmr.13.2.236
Robeck, T. R., and Dalton, L. M. (2002). Saksenaea vasiformis and Apophsomyces elegans zygomycotic infections in bottlenose dolphins (Tursiops truncatus), a killer whale (Orcinus orca) and Pacific white-sided dolphins (Lagenorhynchus obliquidens). J. Zoo. Wildl. Med. 33, 356–366.
Roden, M. M., Zaoutis, T. E., Buchannan, W. L., Knudsen, T. A., Sarkisova, T. A., Schaufele, R. L., et al. (2005). Epidemiology and outcome of zygomycosis: a review of 929 reported cases. Clin. Infect. Dis. 41, 634–653. doi: 10.1086/432579
Rosenburg, J. F., Haulena, M., Hoang, L. M. N., Morshed, M., Zabek, E., and Raverty, S. A. (2016). Cryptococcus gattii type VGIIa Infection in Harbor Seals (Phoca vitulina) in British Columbia, Canada. J. Wildl. Dis. 52, 677–681. doi: 10.7589/2015-11-299
Seyedmousavi, S., Bosco, S. M. G., de Hoog, S., Ebel, F., Elad, D., Gomes, R., et al. (2018). Fungal infections in animals: a patchwork of different situations. Med. Mycol. 56, 165–187. doi: 10.1093/mmy/myx104
Skiada, A., Rigopoulos, D., Larios, G., Petrikkos, G., and Katsambas, A. (2012). Global epidemiology of cutaneous zygomycosis. Clin. Dermatol. 30, 628–632. doi: 10.1016/j.clindermatol.2012.01.010
Spellberg, B., Edwards, J. Jr., and Ibrahim, A. (2005). Novel perspectives on mucormycosis: pathophysiology, presentation, and management. Clin. Microbiol. Rev. 18, 556–569. doi: 10.1128/CMR.18.3.556-569.2005
Stephen, C., Lester, S., Black, M., Fyfe, M., and Ravety, S. (2002). Multispecies outbreak of cryptococcosis on southern Vancouver Island, British Columbia. Can. Vet. J. 43, 792–794.
Walsh, T. H., Hospenthal, D. R., Petraitis, V., and Kontoyiannis, D. P. (2019). Necrotizing mucormycosis of wounds following combat injuries, natural disasters, burns, and other trauma. J. Fungi 5:57. doi: 10.3390/jof5030057
Warlick, A. J., Duffield, D. A., Lambourn, D. M., Jeffries, S. J., Rice, J. M., Gaydos, J. K., et al. (2018). Spatio-temporal characterization of pinniped strandings and human interaction cases in the Pacific Northwest, 1991-2016. Aquat. Mamm. 44, 299–318. doi: 10.1578/AM.44.3.2018.299
White, T. J., Bruns, T., Lee, S., and Taylor, J. (1990). “Amplification and direct sequencing of fungal ribosomal RNA genes for phylogenetics,” in PCR Protocols: A Guide to Methods and Application, eds M. A. Innis, D. H. Gelfand, J. J. Sninsky, and T. J. White (New York, NY: Academic Press), 315–322. doi: 10.1016/b978-0-12-372180-8.50042-1
Keywords: mucormycosis, harbor porpoise, harbor seal, killer whale, fungus, marine mammal, strandings
Citation: Huggins JL, Garner MM, Raverty SA, Lambourn DM, Norman SA, Rhodes LD, Gaydos JK, Olson JK, Haulena M and Hanson MB (2020) The Emergence of Mucormycosis in Free-Ranging Marine Mammals of the Pacific Northwest. Front. Mar. Sci. 7:555. doi: 10.3389/fmars.2020.00555
Received: 16 February 2020; Accepted: 16 June 2020;
Published: 07 July 2020.
Edited by:
Celine Frere, University of the Sunshine Coast, AustraliaReviewed by:
Ursula Siebert, University of Veterinary Medicine Hanover, GermanyDaniel Powell, University of the Sunshine Coast, Australia
Copyright © 2020 Huggins, Garner, Raverty, Lambourn, Norman, Rhodes, Gaydos, Olson, Haulena and Hanson. This is an open-access article distributed under the terms of the Creative Commons Attribution License (CC BY). The use, distribution or reproduction in other forums is permitted, provided the original author(s) and the copyright owner(s) are credited and that the original publication in this journal is cited, in accordance with accepted academic practice. No use, distribution or reproduction is permitted which does not comply with these terms.
*Correspondence: Jessica L. Huggins, amh1Z2dpbnNAY2FzY2FkaWFyZXNlYXJjaC5vcmc=