- 1Department of Biological, Geological and Environmental Sciences, University of Catania, Catania, Italy
- 2Section of Biochemistry, Department of Biomedical and Biotechnological Sciences, University of Catania, Catania, Italy
Fish skin mucus is an important component of the innate immune mechanism and provides a first barrier against pathogens. Although several studies investigated and demonstrated the antimicrobial properties of skin mucus of fishes, most of them tested antimicrobial properties against marine microbial strains as potential use in aquaculture. Furthermore, most of these studies concerned freshwater species, and far less attention was given to skin mucus of marine fishes for potential application in human health and research toward its antimicrobial properties. This review outlines the importance of marine fishes as an important and effective source of antimicrobial drugs against several human pathogens, thus providing a highly available and low-cost tool to counteract clinical infections.
Introduction
Skin mucus is an important component of the innate immune mechanism in fish and provides a first physical and chemical barrier against pathogens, thus playing an important role in fish health (Subramanian et al., 2007). It is secreted by three different cell types, namely, goblet cells, sacciform cells, and club cells. Goblet cells are abundant on all external surfaces and on the gill’s surface; these cells produce mucus granules and contain glycoproteins. The sacciform cells mix their secretions with those of goblet cells, and the club cells mainly secrete proteinaceous components (Fasulo et al., 1993; Zaccone et al., 2001). Skin mucus performs its role by a continuous production and slough off, preventing the pathogens’ attacks, and containing several antimicrobial factors, such as proteins, lysozyme, immunoglobulin, and lectins (Dash et al., 2018). Furthermore, it is also involved in osmoregulation and chemical communication, improves swimming performance by reducing water resistance, and acts as a physical barrier against abrasion and as a physical and biochemical barrier against pollutants (Menzies and Hood, 2012). In some species, such as cichlids and blennies, it is involved also in parental care (Chong et al., 2006; Giacomello et al., 2006; Buckley et al., 2010; Pizzolon et al., 2010).
Depending on the species, skin mucus varies considerably in viscosity, thickness, and glycoprotein (mucin) content which also represents the major components of mucus (Dash et al., 2018). Mucins are glycoproteins with high molecular weight, which give the mucus viscoelastic and rheological properties. Other components found in fish mucus are lysozyme, glycosaminoglycans, immunoglobulins, complements, carbonic anhydrase, lectins, and calmodulin (Shephard, 1994). However, the composition of the fish skin mucus is very variable between species and within species, sex, developmental stages, and environmental conditions (Blackstock and Pickering, 1982; Reverter et al., 2018). In recent years, interest in antimicrobial properties of fish skin mucus has increased (Kumari et al., 2011; Reverter et al., 2018), playing an important role against microbes that can alter the mucosal microbiome of fish, making the fish more vulnerable to several diseases (Llewellyn et al., 2017; Reid et al., 2017). The antimicrobial effect of mucus appears to be a good candidate for the development not only of new therapeutic agents to treat infections in humans (Fuochi et al., 2017) but also of new agents for commercial use (Pethkar and Lokhande, 2017). Therefore, the number of marine species studied in search of secondary metabolites is growing (Gates, 2010). It was estimated that with respect to terrestrial species, the success rate in finding undescribed metabolites in marine organisms is 500 times higher. The biochemical resources from the marine environment represent an important source of potential medical and industrial products, thus the importance of bioprospecting studies in the search of novel therapeutic agents, such as antimicrobial and anti-proliferative compounds (da Cunha et al., 2013). Furthermore, the anti-microbial resistance is one of the greatest threats to global health, as well as a major contributor to rising healthcare costs worldwide (Marston et al., 2016), hence the urgency to discover new antimicrobial compounds. In this review, we analyze all the main aspects of the antimicrobial properties of mucus in marine fishes.
Antimicrobial Activity in Marine Fishes
Several studies have shown the potential use of antimicrobial properties of fish skin mucus in the treatment of infections in humans, and most of them concern freshwater species (Dhanaraj et al., 2009; Kumari et al., 2011; Nwabueze, 2014; Pethkar and Lokhande, 2017). In recent years, some authors focused on the antimicrobial properties of marine and catadromous fish species (Fuochi et al., 2017; Pethkar and Lokhande, 2017).
Antimicrobial Activity in Marine Elasmobranchs
The elasmobranchs represent a distinct group of cartilaginous fish that host a remarkable ability to heal wounds quickly and without infection (Ritchie et al., 2017). Several studies have shown the antimicrobial properties of fish mucus from elasmobranchs (Table 1). In this regard, Vennila et al. (2011) showed the antimicrobial activity of crude aqueous, acidic, and organic mucus extract of Maculabatis gerrardi (Gray, 1851) and Pastinachus sephen (Forsskål, 1775) against several strains of bacterial and fungal pathogens. The higher inhibition of bacterial pathogens’ growth was obtained with the acidic mucus extracts of both species against Salmonella typhi, Escherichia coli, Vibrio cholerae, Klebsiella pneumoniae, and Streptococcus aureus. The acid extracts of both species showed a potent activity also against fungal pathogens: Candida tropicalis, Aspergillus niger, Penicillium sp., Trichophyton mentagrophytes, Alternaria alternata, Candida albicans, Rhizopus sp., Mucor sp., and Trichophyton rubrum. Authors suggested that the antimicrobial activity of the acidic mucus extract was due to the presence of basic antimicrobial peptides or acidic soluble proteins. On the other hand, no antimicrobial effect was shown for the organic extracts hypothesizing that the antimicrobial activity is due to the microbial flora which remains in the mucus of the stingrays.
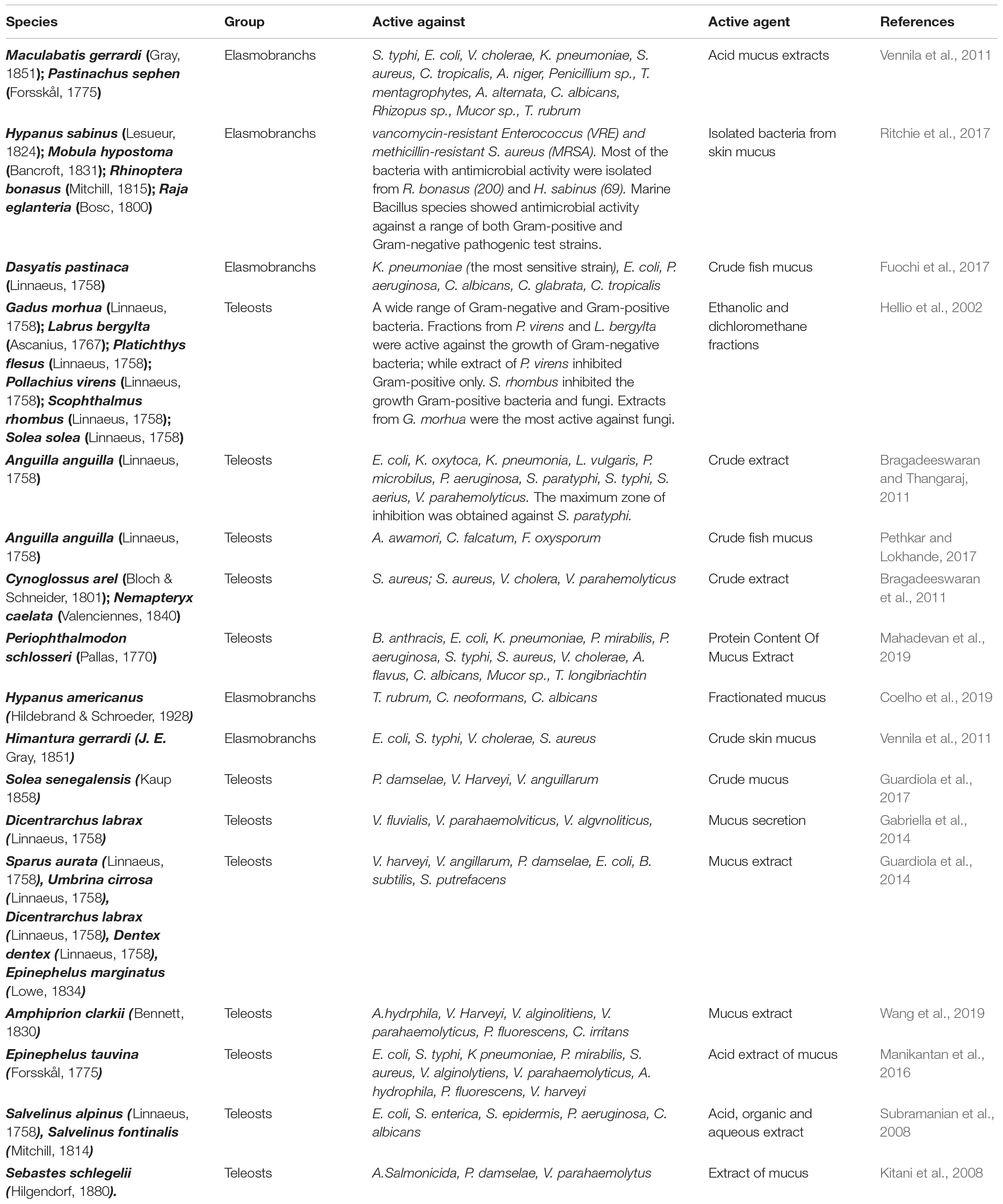
Table 1. List of principal studies of skin mucus antimicrobial activities from elasmobranchs and teleosts.
Consistently, Ritchie et al. (2017) isolated a total of 1860 bacteria from the epidermal mucus of Hypanus sabinus (Lesueur, 1824), Mobula hypostoma (Bancroft, 1831), Rhinoptera bonasus (Mitchill, 1815), and Raja eglanteria (Bosc, 1800). All isolated bacteria were screened for their antimicrobial activity vs. a range of pathogenic test strains. Out of the total bacteria analyzed, 311 showed antimicrobial activity and 57 of these produced either broad-spectrum antibiotics or activities against vancomycin-resistant Enterococcus (VRE) and methicillin-resistant Staphylococcus aureus (MRSA). However, most of the bacteria with antimicrobial activity were isolated from R. bonasus (200) and H. sabinus (69), even if this apparent higher diversity is likely due to the higher sample size of these species. Furthermore, marine Bacillus species showed antimicrobial activity against a range of both Gram-positive and Gram-negative pathogenic test strains (Das et al., 2008). Similarly, we also showed that an aqueous protein extract of the epidermal mucus layers of Dasyatis pastinaca (Linnaeus, 1758), had a good inhibition only for Gram-negative bacteria, such as Klebsiella pneumoniae (the most sensitive strain), Escherichia coli, and Pseudomonas aeruginosa (Fuochi et al., 2017). This was explained by the strong interaction between the outer membrane (present only in the Gram-negative strains) and the active molecules present in the fish skin mucus. In the same work, a great inhibition against fungal pathogens of the genus Candida namely C. albicans, C. glabrata and C. tropicalis was also demonstrated. The inhibition of the fungal growth was potentially explained by the presence of chitinase in the skin mucus of D. pastinaca.
Coelho et al. (2019) studied the biochemical and biological characteristics of the mucus of Hypanus americanus (Hildebrand and Schroeder, 1928), showing its pro-inflammatory characteristics, probably due to the presence in the mucus of peptidases and peptides derived from immune-related proteins. In fact, fractionated mucus improved phagocytosis in macrophages and showed antimicrobial activity against Trichophyton rubrum, Cryptococcus neoformans, and C. albicans in vitro. All fractions were tested on four mushrooms. Fraction 3 was able to inhibit the growth of T. rubrum and C. neoformans. Conversely, fraction 5 is active only against C. albicans and no antibiotic effect was observed for the other fractions (Coelho et al., 2019).
Several studies have also focused on mucus extraction methods, which are mainly three: aqueous, organic, and acidic. Three stingray species (Order: Myliobatiformes; Family: Dasyatidae), namely Dasyatis pastinaca (common stingray), P. sephen (cowtail stingray), and H. gerrardi (whitespotted whipray) were also reviewed for their mucosal antibacterial properties.
The crude mucus extract (16.50 μg/μL) of common stingray (Fuochi et al., 2017) was reported to strongly inhibit the growth of Gram-negative bacteria such as E. coli, K. pneumoniae, and P. aeruginosa, while the acidic mucus extracts of P. sephen and H. gerrardi (Vennila et al., 2011) revealed varying MIC values against various pathogens. In this study, the extracts of both stingray species had shown lower MIC value against Gram-negative bacteria (E. coli, S. typhi and V. cholerae) than Gram-positive bacteria (S. aureus). Nevertheless, no activity was shown by the aqueous and organic mucus extracts for the two species (Lee et al., 2020).
Sharks also produce epidermal mucus, but due to the characteristic presence of superficial skin denticles on the skin, their mucus is not so extractable. Nevertheless, in two recent studies, the authors observed that the bacteria associated with the mucus of six shark species showed antibiotic activity (Carcharodon carcharias, Carcharhinus acronotus, Carcharhinus leucas, Carcharhinus limbatus, Galeocerdo cuvier, Negaprion brevirostris) (Ritchie et al., 2017). Such a growing database of marine bacteria that produce antibiotics has implications for host microbe associations among elasmobranch fish and could reveal promising candidates for future drug discovery initiatives.
Antimicrobial Activity in Marine Teleosts
Seventy-eight skin mucus extracts from a total of 13 marine teleosts was tested against a total of 15 pathogen bacterial (Bacillus subtilis, Bacillus cereus, Bacillus megaterium, Streptococcus sp., Staphylococcus aureus, Escherichia coli, Klebsiella pneumoniae, Serratia marcescens, Proteus vulgaris, Pseudomonas aeruginosa) and fungal (Candida brusei, Candida albicans, Candida tropicalis, Saccharomyces cerevisiae and Issatchenkia orientalis) strains (Hellio et al., 2002) (Table 1). Fifteen out of a total of 78 extracts tested showed antimicrobial activity at 30 μg/ml. On the other hand, the aqueous, ethanol, and dichloromethane fractions of Conger conger (Linnaeus, 1758), Lophius piscatorius (Linnaeus, 1758), Pleuronectes platessa (Linnaeus, 1758), Pollachius pollachius (Linnaeus, 1758), Scombrus scombrus (Linnaeus, 1758), Trachurus trachurus (Linnaeus, 1758), and Trisopterus luscus (Linnaeus, 1758) were inactive against all the tested strains. None of the aqueous extracts from skin mucus of fishes showed antimicrobial activity. Active samples were extracted by Gadus morhua (Linnaeus, 1758), Labrus bergylta (Ascanius, 1767), Platichthys flesus (Linnaeus, 1758), Pollachius virens (Linnaeus, 1758), Scophthalmus rhombus (Linnaeus, 1758), and Solea solea (Linnaeus, 1758). One third of such extracts were ethanolic fractions, and three quarters were dichloromethane fractions. Most of the active fractions were obtained from dichloromethane extracts, and this suggests that antimicrobial activity was negatively correlated with the polarity of extracts. Fractions from P. virens and L. bergylta were active against the growth of Gram-negative bacteria, while the extract of P. virens inhibited Gram-positive only. These results suggested that several compounds were involved in bacterial defense, and different specialized compounds acted against both Gram-negative and Gram-positive bacteria. Hence, it could be possible to combine molecules with different complementary activities or molecules with a wide range of microbial targets. Other fractions from P. virens, P. flesus, and S. solea were active against the growth of both Gram-negative and Gram-positive bacteria. Fraction from S. rhombus inhibited the growth Gram-positive bacteria and fungi. The results of the minimum inhibitory concentration (MIC) determinations showed that the most active extracts against Gram-positive bacteria were from S. rhombus and S. solea. The extracts of this latter species were also, together with that of L. bergylta, the most active against Gram-negative bacteria; while the extracts from G. morhua were the most active against fungi.
The study of Katra et al. (2016) showed that the aqueous extract and the DMC phase obtained from L. bergylta mucus have no bactericidal effect on the microorganisms tested in the study. In fact, it has been discovered that the aqueous extract, the aqueous phase, and the DMC phase, obtained from L. bergylta (ballan wrasse), have no effect on E. coli, Yersinia ruckeri, Edwardsiella tarda, Listonella anguillarum, Aeromonas sobria, Shemanella baltica, Enterobacter sp., Citrobacter Sp., Bacillus subtilis, and Lactobacillus plantarum. However, this study runs counter to the study by Hellio et al. (2002) which instead showed that various extracts obtained from the skin mucus of the L. bergylta fish were analyzed, found that extracts had no effect on Bacillus subtilis, B. cereus, B. megaterium, Streptococcus sp., Staphylococcus aureus, Candida brusei, C. albicans, C. tropicalis, Saccharomyces cerevisiae, and Issatchenkia orientalis strains. However, the aqueous phase was reported to have antimicrobial effect on E. coli, K. pneumoniae, S. marcescens, and P. vulgaris, and the DCM phase was found to have that effect on P. aeruginosa strains; this difference, the authors explain, could be due to several reasons including the development of antimicrobial resistance in the strains that were used or, again, the season in which the fish were caught (Hellio et al., 2002). Furthermore, the reason why the aqueous extracts have no antimicrobial effect could be explained by the possibility that the enzyme content of the extracts would not transform into the active form due to the inappropriate temperature and pH conditions or even the levels of these enzymes that were too low (Katra et al., 2016).
The mucus of Solea senegalensis (Kaup, 1858) was also studied by Guardiola A.F. (Guardiola et al., 2017); in particular, they studied its bactericidal activity against pathogenic and non-pathogenic fish bacteria. The study showed that S. senegalensis mucus has high bactericidal activity against pathogenic bacteria, in particular for Photobacterium damselae, Vibrio harveyi, and Vibrio anguillarum, and a weak activity against non-pathogenic bacteria (E. coli, B. subtilis and S. putrefaciens) (Guardiola et al., 2017). The skin mucus of Anguilla anguilla (Linnaeus, 1758) was tested against ten different bacteria strains (Bragadeeswaran et al., 2011), namely, Escherichia coli, Klebsiella oxytoca, Klebsiella pneumonia, Lactobacillus vulgaris, Proteous microbilus, Pseudomonas aeruginosa, Salmonella paratyphi, Salmonella typhi, Staphylococcus aerius, and Vibrio parahemolyticus. The maximum zone of inhibition (crude extract) was obtained against S. paratyphi and was 10 mm. On the other hand, the lower inhibitory effect (aqueous extract) was obtained against S. aerius and was 1 mm. Another study on the skin mucus of A. anguilla (Linnaeus, 1758) tested its antimicrobial properties against some phytopathogenic fungi, namely, Aspergillus awamori, Colletotrichum falcatum and Fusarium oxysporum and showed a good antifungal activity on all these species, especially on C. colletotrichum (Pethkar and Lokhande, 2017).
Also, Gabriella et al. (2014) studied the antimicrobial activities of the mucus of A. anguilla, together with that of Dicentrarchus labrax and Pagellus bogaraveo. Both the mucus of A. anguilla and that of D. labrax have shown a wide spectrum of antibacterial activity against V. fluvialis, V. parahaemolyticus and V. algynoliticus (Gabriella et al., 2014). Similarly, Guardiola et al. (2014) studied the antimicrobial properties of the mucus of five marine teleostei: gilthead seabream (Sparus aurata), European sea bass (Dicentrarchus labrax), shi drum (Umbrina cirrosa), common dentex (Dentex dentex), and dusky grouper (Epinephelus marginatus) against fish pathogenic Vibrio harveyi, Vibrio angillarum, and Photobacterium damselae and non-pathogenic bacteria Escherichia coli, Bacillus subtilis, and Shewanella putrefaciens (Guardiola et al., 2014), suggesting that the increased bactericidal activity against pathogenic bacteria has been shown for Sparus aurata. In the case of cutaneous mucus by D. dentex, the activity was low against V. harveyi but high against P. damselae. In non-pathogenic bacteria, the lowest activity was found for Sparus aurata, and the highest, for E. marginatus. On the contrary, in the mucus of U. cirrosa the bactericidal activity was very high toward E. coli but very low toward B. subtilis. Furthermore, a significant antibacterial effect against human pathogens was also demonstrated for the skin mucus of two marine teleosts, namely, Cynoglossus arel (Bloch and Schneider, 1801) and Nemapteryx caelata (Valenciennes, 1840). In particular, out of a total of 10 human pathogens tested, four showed a strong inhibition in the growth when exposed to the mucus of C. arel and N. caelata (Bragadeeswaran et al., 2011): Vibrio cholera (9 and 2 mm in diameter), Staphylococcus aureus (6 and 3 mm), Streptococcus aureus (5 and 4 mm), and Vibrio parahemolyticus (4 and 5 mm), respectively. A small antibacterial effect was observed against Salmonella typhi: the zone of inhibition was of 2 mm for the mucus of both species.
The protein content of mucus extracts of Periophthalmodon schlosseri (Pallas, 1770) showed a great antibacterial activity against eight human pathogenic bacteria (Bacillus anthracis, Escherichia coli, Klebsiella pneumoniae, Proteus mirabilis, Pseudomonas aeruginosa, Salmonella typhi, Staphylococcus aureus, and Vibrio cholerae) and four fungal strains (Aspergillus flavus, Candida albicans, Mucor sp. and Trichoderma longibriachtin) (Mahadevan et al., 2019). Between bacteria, the highest inhibition zone was observed against P. aeruginosa, S. typhi, with an inhibition zone of 13 and 18 mm, respectively. Between fungi, the highest inhibition zone was observed for Mucor sp. and was 16 mm.
Several other studies have focused on the study of the antimicrobial activity of the mucus of teleost fish. Wang et al. (2019) studied the antibacterial activity of Amphiprion clarkii mucus on two Gram-positive bacteria (S. aureus and M. lysocleiktiens) and five Gram-negative bacteria (A. hydrophila, V. harveyi, V. alginolitiens, V. parahaemolyticus and P. fluorescens), showing a strong antibacterial activity against Gram-negative but no effect against Gram-positive. It also showed an apparent antiparasitic activity against C. irritans. In addition, Manikantan et al. (2016) studied the activity of Epinephelus tauvina mucus on five human pathogens (E. coli, S. typhi, K. pneumoniae, P. mirabilis and S. aureus) and five pathogens for fish (V. alginolytiens, V. parahaemolyticus, A. hydrophila, P. fluorescens and V. harveyi). Only the acid extract showed significant antimicrobial activity. Among human pathogens, the maximum inhibition zone (26.0 mm) against P. mirabilis was observed, while for fish pathogens, the greatest activity was found for V. parahaemolytius (Manikantan et al., 2016). Consistently, another study focused on the antimicrobial activities of Arctic char (Salvelinus alpinus), brook trout (Salvelinus fontinalis), koi carp (Cyprinus carpio), striped bass (Morone saxatilis), haddock (Melanogrammus aeglefinus) and hagfish (Myxine glutinosa), extracted with acidic, organic, and aqueous solvents against human pathogens (E. coli, Salmonella enterica, Staphylococcus epidermis, P. aeruginosa, C. albicans) and fish pathogens (Aeromonas salmonicida, L. anguillarum and Y. ruckeri). The acidic extracts of S. fontinalis, M. aeglefinus, and M. glutinosa showed bactericidal activity, while the organic extracts of S. fontinalis, M. saxatilis, and C. carpio show bacteriostatic activity, while no activity has been detected for aqueous extracts. In comparison to brook trout and haddock, the minimum bactericidal concentrations of hagfish acidic mucus extracts were found to be ∼1.5–3.0 times lower against fish pathogens and ∼1.6–6.6-fold lower for human pathogens (Subramanian et al., 2008).
Finally, Kitani et al. (2008) in his study isolated L-amino acid oxidase (LAO) from rockfish mucus Sebastes schlegelii (called SSAP) and was shown to be active on Gram-negative bacteria. SSAP inhibited the growth of A. salmonicida, P. damselae, and V. parahaemolytivus with a minimum inhibitory concentration (MIC) of 0.078, 0.16, and 0.63 μg/mL, respectively.
In conclusion, the study showed that organic mucus extracted with the polar solvent ethanol and non-polar solvents DMSO exhibited greater inhibitory activity against both bacterial and fungal pathogens than the aqueous mucus extract against human pathogens tested.
Conclusion
Although the antibacterial properties of skin mucus of fishes have been known for many years, previous studies focused on antibacterial properties against marine microbial strains (Ingram, 1980; Fouz et al., 1990), and no attention was paid to investigate the antimicrobial properties of skin mucus of fishes for human health. To date, most studies focused on freshwater species, and little attention was paid to the marine ones. More generally, in the marine environment, while a handful of drugs have been developed from marine invertebrates, marine vertebrates remain largely underutilized as a potential source for new therapeutic agents (Luer and Walsh, 2018). Fishes discarded from fisheries could be a relevant, easily-fond, and low-cost source for the isolation of new biologically active compounds (Hellio et al., 2002). The main studies concerning the investigation of antimicrobial properties of the skin mucus of marine fishes are quite limited, especially in comparison to the studies performed on the skin mucus of freshwater fishes. However, as outlined in the present review, marine fishes are a potentially important resource for the development of new and effective antimicrobial compounds. Overall, marine fishes have shown they have good antimicrobial properties against different human pathogens including Gram-negative and Gram-positive bacterial and fungal pathogens. Therefore, the marine environment represents a great resource for the production of new antimicrobial clinically relevant compounds (Figure 1). In this perspective, the skin mucus of fishes, due to its considerable and recognized antimicrobial effect, is a good candidate for providing new antimicrobial compounds for human health. In conclusion, further studies and efforts are still needed in order to investigate and exploit the biological properties contained in the low-cost and largely available skin mucus of fishes.
Author Contributions
FT, DT, GM, and LL: conceptualization. FT, DT, BL, and GL: writing – original draft preparation. All authors have read and agreed to the published version of the manuscript.
Funding
This work was supported by the University of Catania linea di intervento 3 “Piano PIACERI 2020-2022”.
Conflict of Interest
The authors declare that the research was conducted in the absence of any commercial or financial relationships that could be construed as a potential conflict of interest.
References
Blackstock, N., and Pickering, A. D. (1982). Changes in the concentration and histochemistry of epidermal mucous cells during the alevin and fry stages of the brown troutSalmo trutta. J. Zool. 197, 463–471. doi: 10.1111/jzo.1982.197.4.463
Bragadeeswaran, S., Priyadharshini, S., Prabhu, K., and Rani, S. R. (2011). Antimicrobial and hemolytic activity of fish epidermal mucus cynoglossus arel and arius caelatus. Asian Pac. J. Trop. Med. 4, 305–309. doi: 10.1016/s1995-7645(11)60091-6
Bragadeeswaran, S., and Thangaraj, S. (2011). Hemolytic and Antibacterial studies on skin mucus of Eel fish, Anguilla anguilla Linnaues, 1758. Asian J. Biol. Sci. 4, 272–276. doi: 10.3923/ajbs.2011.272.276
Buckley, J., Maunder, R. J., Foey, A., Pearce, J., Val, A. L., and Sloman, K. A. (2010). Biparental mucus feeding: a unique example of parental care in an Amazonian cichlid. J. Exp. Biol. 213, 3787–3795. doi: 10.1242/jeb.042929
Chong, K., Joshi, S., Jin, L. T., and Shu-Chien, A. C. (2006). Proteomics profiling of epidermal mucus secretion of a cichlid (Symphysodon aequifasciata) demonstrating parental care behavior. Proteomics 6, 2251–2258. doi: 10.1002/pmic.200500591
Coelho, G. R., Neto, P. P., Barbosa, F. C., Dos Santos, R. S., Brigatte, P., Spencer, P. J., et al. (2019). Biochemical and biological characterization of the Hypanus americanus mucus: a perspective on stingray immunity and toxins. Fish Shellfish Immunol. 93, 832–840. doi: 10.1016/j.fsi.2019.08.049
da Cunha, M. G., Franchin, M., De Carvalho Galvao, L. C., De Ruiz, A. L., De Carvalho, J. E., Ikegaki, M., et al. (2013). Antimicrobial and antiproliferative activities of stingless bee Melipona scutellaris geopropolis. BMC Complement. Altern. Med. 13:23.
Das, S., Ward, L. R., and Burke, C. (2008). Prospects of using marine actinobacteria as probiotics in aquaculture. Appl. Microbiol. Biotechnol. 81, 419–429. doi: 10.1007/s00253-008-1731-8
Dash, S., Das, S. K., Samal, J., and Thatoi, H. N. (2018). Epidermal mucus, a major determinant in fish health: a review. Iran. J. Vet. Res. 19, 72–81.
Dhanaraj, M., Hannifa, M. A., Arun Singh, S. V., Muthu Ramakrishnan, C., Manikandaraja, D., and James Milton, M. (2009). Antibacterial activity of skin and intestinal mucus of five different freshwater fish species viz., Channa striatus, C. micropeltes, C. marulius, C. Punctatus and C. gachua. Malays. J. Sci. 28, 256–262. doi: 10.22452/mjs.vol28no3.3
Fasulo, S., Tagliafierro, G., Contini, A., Ainis, L., Ricca, M. B., Yanaihara, N., et al. (1993). Ectopic expression of bioactive peptides and serotonin in the sacciform gland cells of teleost skin. Arch. Histol. Cytol. 56, 117–125. doi: 10.1679/aohc.56.117
Fouz, B., Devesa, S., Gravningen, K., Barja, J., and Toranzo, A. (1990). Antibacterial action of the mucus of the turbot. Bull. Eur. Ass. Fish. Pathol. 10, 56–59.
Fuochi, V., Li Volti, G., Camiolo, G., Tiralongo, F., Giallongo, C., Distefano, A., et al. (2017). Antimicrobial and anti-proliferative effects of skin mucus derived from Dasyatis pastinaca (Linnaeus, 1758). Mar. Drugs 15:342. doi: 10.3390/md15110342
Gabriella, C., Giulia, M., Lucrezia, G., Rosalba, C., Maria Gabriella, D., Santi, D., et al. (2014). Comparative study of antibacterial and haemolytic activities in sea bass, european eel and blackspot seabream. Open Mar. Biol. J. 8, 10–16. doi: 10.2174/1874450801408010010
Gates, K. W. (2010). Marine products for healthcare: functional and bioactive nutraceutical compounds from the ocean, vazhiyil venugopal. J. Aquat. Food Product Technol. 19, 48–54. doi: 10.1080/10498850903517528
Giacomello, E., Marchini, D., and Rasotto, M. B. (2006). A male sexually dimorphic trait provides antimicrobials to eggs in blenny fish. Biol. Lett. 2, 330–333. doi: 10.1098/rsbl.2006.0492
Guardiola, F. A., Cuartero, M., Del Mar Collado-González, M., Díaz Baños, F. G., Cuesta, A., and Moriñigo, M. Á, et al. (2017). Terminal carbohydrates abundance, immune related enzymes, bactericidal activity and physico-chemical parameters of the Senegalese sole (Solea senegalensis, Kaup) skin mucus. Fish Shellfish Immunol. 60, 483–491. doi: 10.1016/j.fsi.2016.11.025
Guardiola, F. A., Cuesta, A., Abellán, E., Meseguer, J., and Esteban, M. A. (2014). Comparative analysis of the humoral immunity of skin mucus from several marine teleost fish. Fish & Shellfish Immunol. 40, 24–31. doi: 10.1016/j.fsi.2014.06.018
Hellio, C., Pons, A. M., Beaupoil, C., Bourgougnon, N., and Gal, Y. L. (2002). Antibacterial, antifungal and cytotoxic activities of extracts from fish epidermis and epidermal mucus. Int. J. Antimicrob. Agents 20, 214–219. doi: 10.1016/s0924-8579(02)00172-3
Ingram, G. A. (1980). Substances involved in the natural resistance of fish to infection-a review. J. Fish Biol. 16, 23–60. doi: 10.1111/j.1095-8649.1980.tb03685.x
Katra, N., Hisa, O., Karatas, S., Turgay, E., and Sarvan, C. (2016). In vitro antimicrobial activities of extracts from ballan wrasse (Labrus bergylta) skin mucus. Mar. Sci. Technol. Bull. 5, 13–15.
Kitani, Y., Kikuchi, N., Zhang, G., Ishizaki, S., Shimakura, K., Shiomi, K., et al. (2008). Antibacterial action of L-amino acid oxidase from the skin mucus of rockfish Sebastes schlegelii. Comp. Biochem. Physiol. B Biochem. Mol. Biol. 149, 394–400. doi: 10.1016/j.cbpb.2007.10.013
Kumari, U., Nigam, A. K., Mitial, S., and Mitial, A. K. (2011). Antibacterial properties of the skin mucus of the freshwater fishes, rita rita and Channa punctatus. Eur. Rev. Med. Pharmacol. Sci. 15, 781–786.
Lee, Y., Bilung, L. M., Sulaiman, B., and Chong, Y. L. (2020). The antibacterial activity of fish skin mucus with various extraction solvents and their in-vitro evaluation methods. Int. J. Acqua. Res. 12, 1–21.
Llewellyn, M. S., Leadbeater, S., Garcia, C., Sylvain, F. E., Custodio, M., Ang, K. P., et al. (2017). Parasitism perturbs the mucosal microbiome of Atlantic Salmon. Sci. Rep. 7:43465.
Luer, C., and Walsh, C. (2018). Potential human health applications from marine biomedical research with elasmobranch fishes. Fishes 3:47. doi: 10.3390/fishes3040047
Mahadevan, G., Mohan, K., Vinoth, J., and Ravi, V. (2019). Biotic potential of mucus extracts of giant mudskipper Periophthalmodon schlosseri (Pallas, 1770) from Pichavaram, southeast coast of India. J. Basic Appl. Zool. 80:13.
Manikantan, G., Lyla, S., Khan, S. A., Vijayanand, P., and Jothi, G. E. G. (2016). Bioactive potency of epidermal mucus extracts from greasy grouper, Epinephelus tauvina (Forsskal, 1775). J Coast. Life Med. 4, 510–520. doi: 10.12980/jclm.4.2016j6-34
Marston, H. D., Dixon, D. M., Knisely, J. M., Palmore, T. N., and Fauci, A. S. (2016). Antimicrobial resistance. J. Am. Med. Assoc. 316, 1193–1204.
Menzies, K. J., and Hood, D. A. (2012). The role of SirT1 in muscle mitochondrial turnover. Mitochondrion 12, 5–13. doi: 10.1016/j.mito.2011.03.001
Nwabueze, A. A. (2014). Antimicrobial action of epidermal mucus extract of clarias gariepinus (Burchell, 1822) Juveniles-Fed ginger inclusion in diet. Int. J Biol. 6, 42–48.
Pethkar, M. R., and Lokhande, M. V. (2017). Antifungal activity of skin mucus of three cultivable fish species (Catla-catla, cirrhinus mrigala and anguilla-anguilla). Int. J. Zool. Stud. 2, 01–03.
Pizzolon, M., Giacomello, E., Marri, L., Marchini, D., Pascoli, F., Mazzoldi, C., et al. (2010). When fathers make the difference: efficacy of male sexually selected antimicrobial glands in enhancing fish hatching success. Funct. Ecol. 24, 141–148. doi: 10.1111/j.1365-2435.2009.01608.x
Reid, K. M., Patel, S., Robinson, A. J., Bu, L., Jarungsriapisit, J., Moore, L. J., et al. (2017). Salmonid alphavirus infection causes skin dysbiosis in Atlantic salmon (Salmo salar L.) post-smolts. PLoS One 12:e0172856. doi: 10.1371/journal.pone.0172856
Reverter, M., Tapissier-Bontemps, N., Lecchini, D., Banaigs, B., and Sasal, P. (2018). Biological and ecological roles of external fish mucus: a review. Fishes 3:41. doi: 10.3390/fishes3040041
Ritchie, K. B., Schwarz, M., Mueller, J., Lapacek, V. A., Merselis, D., Walsh, C. J., et al. (2017). Survey of antibiotic-producing bacteria associated with the epidermal mucus layers of rays and skates. Front. Microbiol. 8:1050.
Shephard, K. L. (1994). Functions for fish mucus. Revi. Fish Biol. Fish. 4, 401–429. doi: 10.1007/bf00042888
Subramanian, S., Mackinnon, S. L., and Ross, N. W. (2007). A comparative study on innate immune parameters in the epidermal mucus of various fish species. Comp. Biochem. Physiol. B. Biochem. Mol. Biol. 148, 256–263. doi: 10.1016/j.cbpb.2007.06.003
Subramanian, S., Ross, N. W., and Mackinnon, S. L. (2008). Comparison of antimicrobial activity in the epidermal mucus extracts of fish. Comp. Biochem. Physiol. B Biochem. Mol. Biol. 150, 85–92. doi: 10.1016/j.cbpb.2008.01.011
Vennila, R., Kumar, K. R., Kanchana, S., Arumugam, M., Vijayalakshmi, S., and Balasubramaniam, T. (2011). Preliminary investigation on antimicrobial and proteolytic property of the epidermal mucus secretion of marine stingrays. Asian Pac. J. Trop. Biomed. 1, S239–S243.
Wang, H., Tang, W., Zhang, R., and Ding, S. (2019). Analysis of enzyme activity, antibacterial activity, antiparasitic activity and physico-chemical stability of skin mucus derived from Amphiprion clarkii. Fish Shellfish Immunol. 86, 653–661. doi: 10.1016/j.fsi.2018.11.066
Keywords: skin fish mucus, marine fish, antimicrobial activity, elasmobrachii, teleostean fish
Citation: Tiralongo F, Messina G, Lombardo BM, Longhitano L, Li Volti G and Tibullo D (2020) Skin Mucus of Marine Fish as a Source for the Development of Antimicrobial Agents. Front. Mar. Sci. 7:541853. doi: 10.3389/fmars.2020.541853
Received: 10 March 2020; Accepted: 24 August 2020;
Published: 18 September 2020.
Edited by:
Antonio Trincone, Institute of Biomolecular Chemistry (ICB), ItalyReviewed by:
Maria Angeles Esteban, University of Murcia, SpainSeyed Hossein Hoseinifar, Gorgan University of Agricultural Sciences and Natural Resources, Iran
Copyright © 2020 Tiralongo, Messina, Lombardo, Longhitano, Li Volti and Tibullo. This is an open-access article distributed under the terms of the Creative Commons Attribution License (CC BY). The use, distribution or reproduction in other forums is permitted, provided the original author(s) and the copyright owner(s) are credited and that the original publication in this journal is cited, in accordance with accepted academic practice. No use, distribution or reproduction is permitted which does not comply with these terms.
*Correspondence: Francesco Tiralongo, ZnJhbmNlc2NvLnRpcmFsb25nb0B1bmljdC5pdA==; Giuseppina Messina, Z2l1c2VwcGluYS5tZXNzaW5hQHVuaWN0Lml0